- 1College of Geosciences and Engineering, North China University of Water Resources and Electric Power, Zhengzhou, China
- 2Henan Yukuang Geological Exploration Investment Co., Ltd., Zhengzhou, China
At present, the Carboniferous-Permian shale gas in the South North China Basin is still in the exploration stage, and the understanding of the microscopic pore structures, mineral composition and hydrocarbon enrichment law of the marine-continental transitional shale gas reservoirs in this area is extremely limited. In this paper, taking the Carboniferous-Permian shale gas reservoir in the South North China Basin as an example, the geological conditions of shale gas accumulation have been systematically studied using a large amount of sedimentary, logging, seismic, core, geochemical, physical property, and scanning electron microscope data. The study shows that the transitional dark shale is stable and widely distributed in the South North China Basin. Among them, the average thickness of the high-quality shale in the Taiyuan Formation is 67 m, while that of the Shanxi Formation is 56 m. Carboniferous and Permian are the main strata for the distribution of shale gas resources. Among them, the dark shale developed in the Taiyuan and Shanxi Formations has the largest thickness and the highest organic matter abundance. In addition, the organic matter types of the Upper Paleozoic coal-measure source rocks in the South North China are mainly II2-III types, and a small part of them belong to II1 types. The brittle minerals in the target shale are mainly quartz, and the content of illite is the highest among the clay minerals. Porosity is negatively correlated with clay mineral content, and positively correlated with quartz and TOC content. In addition, the permeability is negatively correlated with the organic matter content. Finally, the criteria for key indicators of the transitional shale gas reservoirs in the South North China Basin were developed.
Introduction
There are three types of sedimentary facies for organic-rich shales: marine, continental and marine-continental transitional facies (Bernard et al., 2012; Clarkson et al., 2013; Jiang et al., 2015; Li., 2022). Coal-measure shale is a typical marine-continental transitional facies deposit. At present, coal-measure shale gas in China is still in the exploratory stage (He et al., 2012; Zou et al., 2019). Compared with marine shale, it is generally believed that coal-measure shale gas reservoirs have the characteristics of rapid lithofacies change, thin single-layer thickness and high content of clay minerals (Chalmers and Bustin, 2007; Curtis et al., 2012; Wang et al., 2015; Kong et al., 2016). With the improvement of oil and gas development technology in tight reservoirs, the commercial development of coal-measure shale gas has gradually become possible. Shale gas will become one of the clean energy sources with the most development potential and prospects in China due to its huge resources, extensive gas-bearing areas and strong sustainable production (Yan et al., 2015; He et al., 2020; Li et al., 2020; Zheng et al., 2020).
The Carboniferous-Permian was a critical period for the transition of sedimentary systems from marine to continental in China. Transitional organic-rich shale is widely developed in China, for example, it is widely developed in the Tarim Basin, Ordos Basin, Qinshui Basin in the central and western China, and the Lower Yangtze and Yunnan-Guizhou areas in the southern China (Lu et al., 2015; Xi et al., 2017; Zou et al., 2019; Nie et al., 2020). Generally, the accumulation scale of transitional shale gas is limited, so its own shale gas reserves do not have high development and economic value. However, it is usually interbed with gas-bearing coal seams and tight sandstone layers, so the “multi-type-gas co-production” can be developed (Kruk and Jaroniec., 2001; Loucks et al., 2009; Fu et al., 2013; Fu et al., 2015). From this point of view, transitional shale gas still has good resource potential and economic benefits.
The main part of the South North China Basin is located in the central and eastern part of the Henan Province, and the Carboniferous-Permian is the main stratum for the distribution of shale gas resources (He et al., 2012). Shale gas in the Taiyuan and Shanxi Formations in the South North China Basin has great exploration potential. For example, the fracturing production of the Well MY1 is 1 200 m3/d, and the production of the Well ZD2 after fracturing is 3 000 m3/d. In addition, the current drilling in the Taikang Uplift, Luyi Depression and Zhoukou Depression have obtained good shale gas shows.
At present, there is insufficient understanding of the microscopic pore structures, mineral composition and hydrocarbon enrichment law of the Carboniferous-Permian shale gas reservoirs in the South China Basin. In this paper, taking the Carboniferous-Permian shale gas reservoir in the South North China Basin as an example, the geological conditions of the shale gas accumulation in the basin have been systematically studied using a large amount of sedimentary, logging, seismic, core, geochemical, physical property, and scanning electron microscope data. This study can provide scientific guidance for promoting the development of transitional shale gas in the South North China Basin and similar areas.
Geological background and methods
Structural and sedimentary background
The South North China Basin is located in the central and southern parts of the Henan Province, China, with a total area of about 150,000 km2 (Figure 1). From north to south, the basin is divided into five sub-structural units: Kaifeng Depression, Taikang Uplift, Zhoukou Depression, Changshan Uplift and Xinyang-Hefei Depression. The ZM Block in the study area is located near the Kaifeng Depression, and the target layers are the Late Paleozoic Carboniferous and Permian strata.
The South North China Basin was part of the North China Craton Basin in the Late Paleozoic (He et al., 2012; Zou et al., 2019). Affected by the global sea level rise in the Late Carboniferous, extensive transgression gradually began to occur in the study area. During the depositional period of the Benxi Formation in the Late Carboniferous, the North China Plate was a gentle monoclinic paleotopography with high in the northwest and low in the southeast. At this time, seawater intruded from the northeast, and a set of marine-continental alternating strata was formed. In the early Permian, affected by the uplift of the Yinshan Ancient Land, the surface sea water of the North China continent retreated from the northwest to the southeast (He et al., 2012). Furthermore, a set of sedimentary strata dominated by marine and transitional facies developed in the Shanxi Formation of the South North China Basin. In the early stage, the tidal flat and peat swamp facies deposits formed on the basis of the gradual withdrawal of the surface sea water; in the late stage, the delta facies deposits under the action of rivers were formed (Wang et al., 2015; Yan et al., 2015). By the late Permian, the South North China Basin was uplifted as a whole, the paleotopography was higher in the north and lower in the south, the seawater completely retreated, and the basin entered the stage of continental sedimentary development. At this time, a set of red clastic deposits dominated by fluvial facies were deposited. The Carboniferous-Permian coal measure strata in the South North China Basin have experienced a complex evolution process of deposition→deep burial→uplifting and denudation transformation→second deep burial. It has the basic geological conditions for secondary hydrocarbon generation in the process of secondary deep burial.
Distribution characteristics of shale series in the taiyuan and shanxi formations
The Carboniferous and Permian in the southern part of the North China are widely distributed with diverse sedimentary types and rich biological fossils. Therefore, this area is one of the best areas to study the Carboniferous and Permian marine-continental transitional shale in China. Shales of the Taiyuan Formation is mainly distributed in the Taikang Uplift, Luyi Depression and Shenqiu Depression. Among them, the cumulative thickness of dark shales in the Taiyuan Formation in the Wells MY1 and ZX1 are 82 and 50 m, respectively. The burial depth of shale is mainly concentrated above 2000 m, and the maximum depth can reach 6,000 m. The shale with the maximum depth is mainly distributed in the Kaifeng and Zhoukou Depressions.
The thickness of the shale in the Shanxi Formation ranges from 5 to 100 m, and the deposition centers are concentrated in the northern part of the basin, such as the Taikang Uplift, Luyi Depression, Luoyang Basin and Xiangcheng Depression. In other areas, the average thickness of the shale is 30 m. Among them, the thickness of dark shale in the Wells MY1 and ZX1 are 91 and 70 m, respectively.
Experimental methods
The samples collected in this study were all from the lower Permian Taiyuan and Shanxi Formations of the Well MY1 in the Zhongmu Shale Gas Exploration Area, Henan Province. Organic carbon content testing and field emission scanning electron microscopy observations were carried out on all samples. Mineral composition analysis was carried out by X-ray diffraction (XRD) with the instrumental parameter of Cu Kα radiation (λ = 1.540 6 for CuKα1) in a stepwise scan at a rate of 4°/min in the range of 3°–85° (2θ). The total organic carbon content (TOC) was measured using a LECO carbon-sulfur analyzer. Before the test, the samples were treated with hydrochloric acid to remove carbonate minerals. Vitrinite reflectance (Ro) testing was performed with a DM LPWITH MSP20 Vitrinite Reflectometer. In addition, the morphological features of the pores of the samples were observed using a Quanta 250FEG SEM field emission scanning electron microscope. Before the experiment, an argon ion spectrometer was used to profile the sample surface to ensure smooth sample surface and improve imaging quality.
Results
Organic abundance of source rocks
The dark shale of the Upper Paleozoic is mainly distributed in the Taiyuan and Shanxi Formations of the Permian, with an average organic carbon content of 0.52%–3.21%. The abundance of organic matter gradually increased from top to bottom perpendicular to the bedding plane (Figure 2). The limestone and marl of the Upper Paleozoic are distributed in the Taiyuan Formation, with an average thickness of 30 m. Its organic carbon content is distributed between 0.07% and 4.54%, with an average value of 0.74%. The TOC content of 1.5%–3% represents medium source rocks, while that of 3%–6% represents good source rocks. Therefore, the target layer develop medium-good source rocks (Wang and Yin., 2019). On the whole, the organic matter abundance of the Upper Paleozoic coal-measure dark shale from bottom to top increases from high to low, and the source rock grade changes from good to poor. The dark shale of the Taiyuan Formation belongs to medium source rocks, while limestone and marl belong to the medium to good source rocks; in addition, the shale of the Shanxi Formation belongs to the poor to medium source rocks (Figure 3).
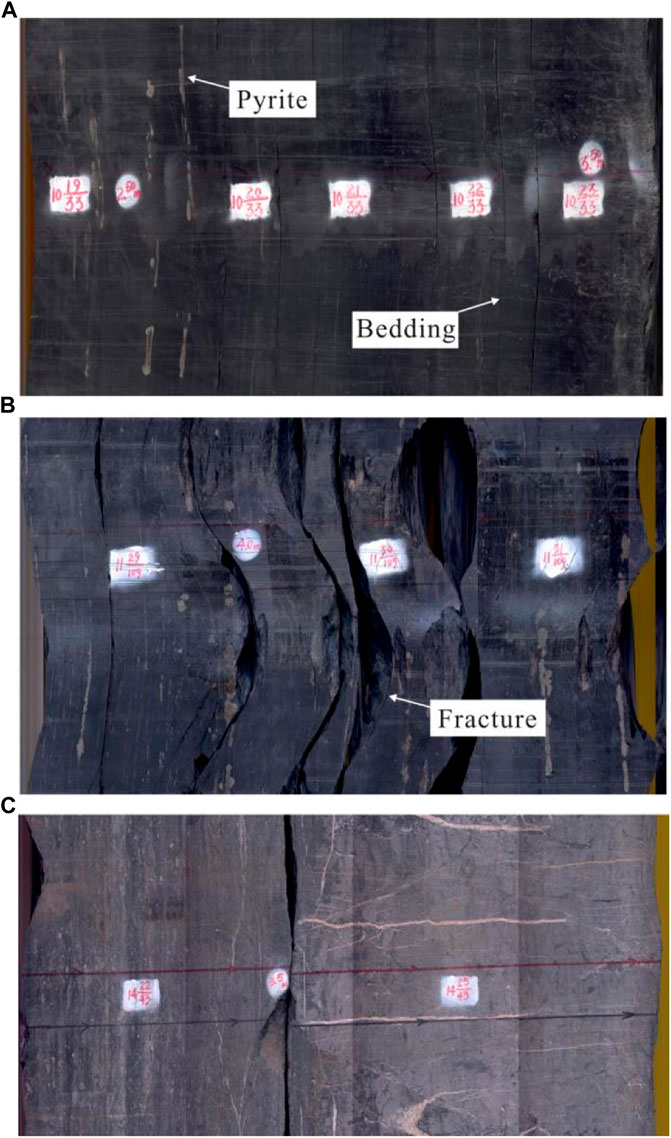
FIGURE 3. Development characteristics of different types of shale in Well MY1. (A) 2,895.25–2,895.91 m; (B) 2,916.6–2,917.4 m; (C) 2,959.24–2,969.13 m.
Types of organic matter in source rocks
For the organic matter types of the Upper Paleozoic coal-measure source rocks in the South North China Basin, dark shale is generally better than coal and carbonaceous shale. The microscopic composition of coal is mainly vitrinite, and the content is usually more than 60%; the second is inertite, its content is less than 30%; and the content of chitinite + sapropel group is generally less than 20%, and most of which are only 2%–15%. The organic matter component of coal is Type III kerogen.
Statistics show that the content of crustal group + sapropel group in the micro-component of dark shale is higher than that of coal and carbonaceous shale (generally more than 40%). It reflects that the organic matter type of dark shale is good. It is mainly Type II2, and a small part is Type III. In addition, the H/C atomic ratios of the organic elements are between 0.51 and 0.91, and the O/C atomic ratios are between 0.06 and 0.14. It also reflects that the types of organic matter are mainly Type II2-III.
On the whole, the organic matter types of the Upper Paleozoic coal measure source rocks in the South North China Basin are mainly Type II2-III, and a small part is Type II1. Moreover, the organic matter type of the black shale is better than that of the arbonaceous shale and coal.
Evolution of organic matter in source rocks
The thermal evolution of the Upper Paleozoic coal measure source rocks in the South North China Basin is uneven in different regions, which is caused by the superposition of the normal pleogenesis and various abnormal thermal evolutions. The Ro value in the Zhongmu-Taikang-Luyi areas is above 2%, and the source rocks are in the high-mature- overmature stage (Figure 4). It is mainly caused by the superposition of normal pleogenesis, mantle thermal uplift, and abnormal thermal evolution of igneous rock thermal contact metamorphism. The Ro value in the Xiangcheng-Tanzhuang-Shenqiu areas is 0.7%–1.5%, and the source rocks are mainly in the mature stage, which belongs to normal pleogenesis.
On the whole, the Upper Paleozoic coal measure source rocks in the South North China Basin are generally in the low-mature-over-mature stage. The general trend of maturity shows a ring-shaped distribution along the Jiyuan-Jiaozuo-Taikang high evolution belt. It has the characteristics of high in the north and low in the south, and high in the west and low in the east. Among them, the high thermal evolution of the Zhongmu-Taikang-Luyi northern region is related to the thermal uplift caused by the thinning of the lithosphere and the upwelling of the asthenosphere. However, the high thermal evolution in the Tanzhuang-Shenqiu area is mainly related to the normal pleogenesis of the huge thick sediments in the Yanshanian- Himalayan period.
Physical properties
The porosity distribution of the Taiyuan Formation gas-bearing shale in Well MY1 ranges from 0.4% to 4.5%, with an average value of 2.1% (Figure 5); the permeability distribution ranges from 1.21 × 10–6 to 0.11 mD, with an average value of 7.48 × 10–3 mD (Figure 6). The porosity distribution of the gas-bearing shale intervals in the Shanxi Formation ranges from 0.3% to 8.8%, with an average value of 2.3% (Figure 5); the permeability distribution ranges from 23×10–6 to 0.92 mD, with an average value of 0.045 mD (Figure 6). The permeability of shale reservoirs in the area is usually less than 0.1 mD, which belongs to the ultra-low permeability type. On the whole, the porosity and permeability of the reservoirs in the area are low, and the shale reservoirs are low-porosity and low-permeability reservoirs.
Total desorption gas volume on site
Core observations in the Well MY1 revealed that the thickness of high-quality shale in the Taiyuan Formation is 77 m, and the thickness of high-quality shale gas in the Shanxi Formation is 67.5 m. The total gas content of high-quality shale ranges from 0.42 to 4.44 m3/t, with an average of 1.93 m3/t (Figure 7).
Discussion
Classification of pore types
The brittle minerals in the transitional shale of the target layer are mainly quartz, and the content of shale in the Taiyuan Formation in Well MY1 of the Zhongmu Block is 6%–55%; the content of clay minerals is 32%–64%. The content of clay minerals and quartz in the shale of the Shanxi Formation ranges from 35% to 89% and 3%–52%, respectively. The clay minerals in the Taiyuan Formation are dominated by illite, kaolinite, imonite mixed layers, and a small amount of chlorite. Among them, the average contents (volume fraction) of illite, kaolinite and imonite mixed layer are 28%, 22.17%, and 20.17%, respectively. For the Shanxi Formation, the clay minerals in the shale are mainly illite and kaolinite, and a small amount of chlorite and imonite mixed layers. Among them, the average volume fractions of illite, kaolinite and imonite mixed layer are 67.36%, 28.18%, and 25.09%, respectively.
Inorganic pores, organic pores and micro-fractures are the main storage spaces in the transitional shale gas reservoirs in the study area (Figure 8) (Zhang et al., 2021).
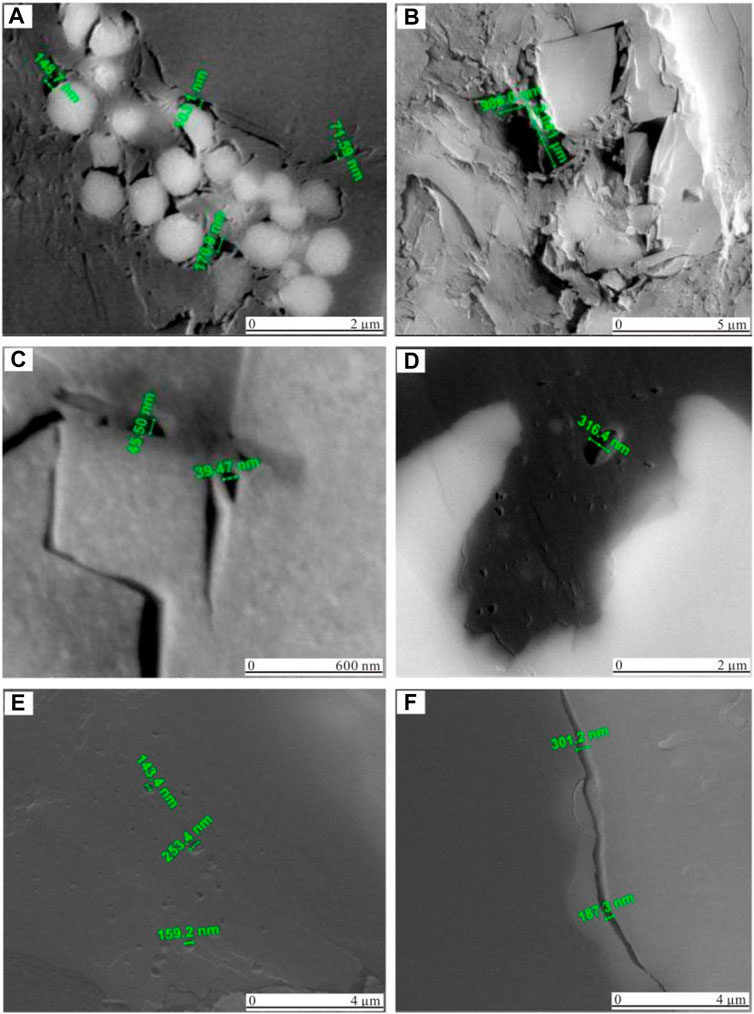
FIGURE 8. Microscopic images of pore characteristics of the Taiyuan and Shanxi Formation shales from Well MY1. (A) Pyrite intergranular pores, 2,934 m; (B) Intergranular pores, 2,937 m; (C) Calcite edge mold pores, 2,874 m; (D) Organic hydrocarbon generation pores, 2,944 m; (E) Organic hydrocarbon generation pores, 2,945 m; (F) Organic matter shrinkage crack, 2,948 m.
1) Inorganic pores: The minerals in the shale of the Taiyuan and Shanxi Formations are mainly composed of clay and quartz, which form a large number of micro-sedimentary structures. The incomplete cementation between various grains or the later diagenetic transformation will generate a large number of intergranular pores. Ductile minerals such as clay minerals, organic matter, and brittle minerals such as quartz, feldspar, and pyrite constitute the inorganic pores (Figures 8A–C).
2) Organic pores and micro-fractures: The shale samples of the Taiyuan and Shanxi Formations develope organic hydrocarbon-generating pores and a large number of organic shrinkage micro-fractures (Figure 8D–F). Organic micro-fractures are fractures formed by the shrinkage of organic matter in the process of hydrocarbon generation. It can not only provide space for the storage of shale gas, but also provide main channels for the seepage of gas (Tissot., 1978; Chalmers et al., 2009; Qi et al., 2019; Li., 2021).
Analysis of controlling factors of physical properties
Statistics show that the average volume fraction of quartz in the Shanxi Formation shale reservoirs in Well MY1 is 30%, while that in the Taiyuan Formation shale reservoirs is 37%. The clay minerals in the Shanxi Formation are dominated by illite and kaolinite, and the clay minerals in the Taiyuan Formation are dominated by illite, kaolinite and imon mixed layer. It fully shows that the shale reservoir in the area has strong brittleness and fracture-making ability, and is suitable for hydraulic fracturing.
Generally, clay minerals are favorable for the adsorption of shale gas (Yang et al., 2010; Yu et al., 2019). Montmorillonite has the strongest adsorption capacity, followed by illite, then chlorite and kaolinite. The composition of clay minerals in shale is relatively complex, with high content of quartz, which are mostly clay grade and often appear in the form of laminar structures (Yang et al., 2016; Xiong et al., 2017; Wang et al., 2018). However, shale with high organic matter and quartz content is highly brittle, and it is easy to form natural and induced fractures under the action of external force. In turn, it facilitates the percolation of natural gas.
There are many factors affecting the porosity of tight reservoirs, including framework particle size, organic matter content, clay mineral content, and dissolution diagenesis. The study found that the porosity of shale was negatively correlated with clay mineral content (Figure 9A), positively correlated with quartz content (Figure 9A) and TOC content (Figure 9B). During the original deposition of shale, the original porosity was very large, and during the later burial compaction and diagenesis, the porosity will continue to decrease. Quartz is a brittle mineral with strong compressive ability. Therefore, as the quartz content in the shale increases, the preservation of the original porosity is better.
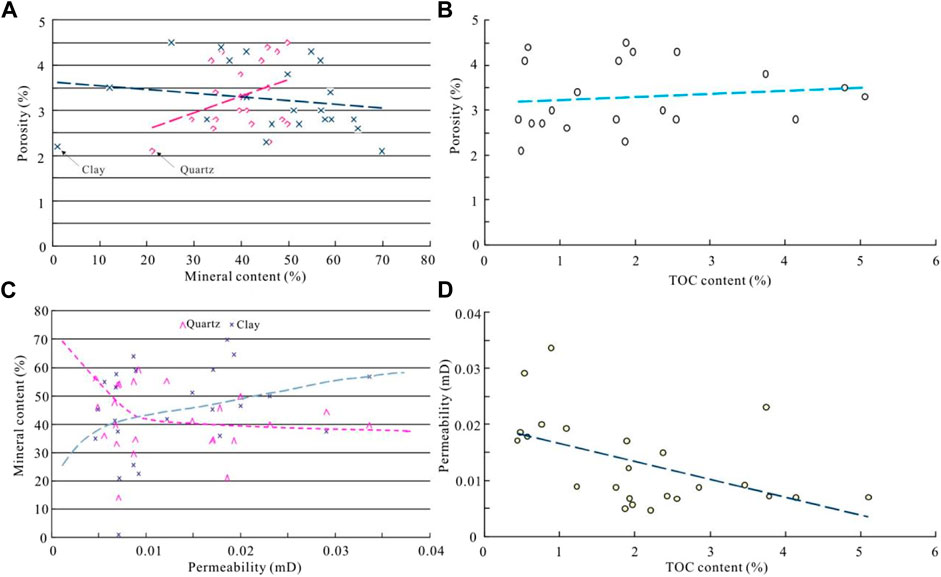
FIGURE 9. Characteristics of physical parameters, TOC content and mineral composition content of shale samples in the target layer. (A) Relationship between mineral composition content and porosity; (B) Relationship between TOC content and porosity; (C) Relationship between mineral composition content and permeability; (D) Relationship between TOC content and permeability.
Similarly, for permeability, interlayer pores with abundant clay minerals will greatly enhance the permeability of the reservoir. Therefore, the permeability increases continuously with the increase of authigenic clay content, and it tends to stabilize after reaching 50% (Figure 9C). There is a negative correlation between permeability and quartz content. The main reason is still the lack of connectivity between the intergranular pores. Therefore, in the process of deep burial, the intergranular pores are basically exhausted by compaction. In addition, the study also found that the permeability was negatively correlated with the organic matter content (Figure 9D). This is because the intercrystalline pores of clay minerals are mainly developed in the transitional shale, and the organic pores are not the main type of pores. When the degree of development of organic pores is high, it represents a strong reducing environment, and the degree of development of intercrystalline pores in clay minerals will decrease.
Accumulation conditions and indexes of shale gas
There is a good positive correlation between the maximum adsorbed gas volume and the desorption gas volume of the Taiyuan and Shanxi Formation shales in Well MY1 and the organic carbon content (Figure 10). This is caused by the presence of a large number of micropores and cracks in the organic matter. Moreover, with the increase of organic carbon content, the type of microscopic pores and fractures and the porosity will also increase, the specific surface area for natural gas adsorption increases sharply, and the maximum adsorbed gas content and desorption gas content of shale increase accordingly. Since the desorption gas volume includes adsorbed gas and free gas, the storage of free gas in pores is greatly affected by formation pressure (Guo et al., 2015; Gai et al., 2016; Ma et al., 2019; Xu et al., 2019). Therefore, the correlation between the desorption gas volume and the organic carbon content is weaker than that of the maximum adsorption gas volume.
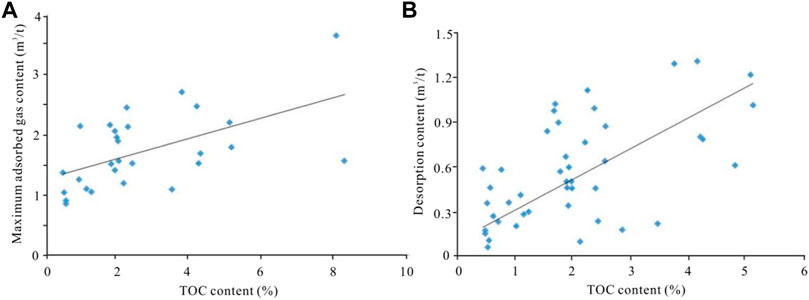
FIGURE 10. Effect of TOC content on the adsorption and desorption gas content of shale samples. (A) TOC content has a positive correlation with the maximum total adsorbed gas; (B) There is also a good positive correlation between TOC content and desorption gas volume.
The geological conditions required for the accumulation of shale gas include: large-scale development of dark shale, favorable structural and depositional background, good reservoir continuity, large thickness, and wide distribution areas. These geological conditions are the material basis for the large-scale accumulation of shale gas. At the same time, the good organic geochemistry, porosity and permeability properties, fracture development, and mineral composition are the key factors for the shale gas accumulation. They comprehensively control the hydrocarbon generation capacity, storage performance, oil and gas potential and production rate of shale, and determine the enrichment degree and exploration prospect of shale oil and gas. In addition, the burial depth, formation temperature, and pressure conditions of shale are also important factors that affect the accumulation of shale gas and determine whether it has industrial exploration and development value.
This study proposes that the key indicators of transitional shale gas include: 1) Organic matter abundance: organic carbon (TOC) content >1.0%, organic matter types are Type II2 and Type III (these two types are the main types in the South North China Basin); 2) Organic matter maturity: vitrinite reflectance Ro>0.9%, which is to ensure the quality of the gas source; 3) Brittle mineral content>40%, clay mineral content <40%, this is to ensure the quality of the reservoir; 4) Porosity>2%, permeability>100 nd, gas content>1.0 m3/t, water saturation <45%, oil saturation <10%; in addition, shale gas resource abundance>2.0×108 m3/km2, which is to ensure the potential and prospects of shale gas resources; 5) Formation pressure: normal pressure to overpressure, continuous effective shale thickness >15 m, interlayer thickness <3.0 m; 6) Lithology and thickness of roof and floor: the thickness of impermeable rock layer is >10 m; 7) Preservation conditions: stable structure, low degree of transformation.
Conclusion
1) The transitional dark shale is stable and widely distributed in the South North China Basin. Among them, the average thickness of the high-quality shale of the Taiyuan Formation is 67 m, while that of the Shanxi Formation is 56 m. Carboniferous and Permian are the main strata for the distribution of shale gas resources. Among them, the dark shale developed in the Taiyuan and the Shanxi Formations has the largest thickness and the highest organic matter abundance.
2) The organic matter types of the Upper Paleozoic coal-measure source rocks in the South North China are mainly the II2-III Types, and a small part of them belong to the II1 Type.
3) The brittle minerals in the target shale are mainly quartz, and the content of illite is the highest among the clay minerals. Porosity is negatively correlated with clay mineral content, and positively correlated with the quartz content and TOC content. In addition, the permeability is negatively correlated with the organic matter content.
4) The criteria for key indicators of transitional shale gas reservoirs in the South North China Basin were developed. This study can provide scientific guidance for efficient exploration and development of transitional shales.
Data availability statement
The original contributions presented in the study are included in the article/supplementary material, further inquiries can be directed to the corresponding author.
Author contributions
YP and JP are responsible for the idea and writing of this paper and XA is responsible for the field investigation and experiments.
Acknowledgments
This research was funded by the Natural Science Foundation of Xinjiang Uygur Autonomous Region—Surface Project (2019D01A34) “Accumulation Mechanism, Occurrence Characteristics and Basin Dynamics Environment of Shale (Stratification) Gas Source-Reservoir Complex.”
Conflict of interest
Author XA was employed by the Henan yukuang geological exploration investment Co., Ltd.
The remaining authors declare that the research was conducted in the absence of any commercial or financial relationships that could be construed as a potential conflict of interest.
Publisher’s note
All claims expressed in this article are solely those of the authors and do not necessarily represent those of their affiliated organizations, or those of the publisher, the editors and the reviewers. Any product that may be evaluated in this article, or claim that may be made by its manufacturer, is not guaranteed or endorsed by the publisher.
References
Bernard, S., Wirth, R., Schreiber, A., Schulz, H. M., and Horsfield, B. (2012). Formation of nanoporous pyrobitumen residues during maturation of the barnett shale (fort worth basin). Int. J. Coal Geol. 103 (23), 3–11. doi:10.1016/j.coal.2012.04.010
Chalmers, G., Bustin, R. M., and Powers, I. (2009). A pore by any other name would be as small: the importance of meso-and microporosity in shale gas capacity,” in AAPG annual convention and exhibition. Denver, Colorado, 7–10.
Chalmers, G., and Bustin, R. M. (2007). The organic matter distribution and methane capacity of the lower cretaceous strata of northeastern British Columbia, Canada. Int. J. Coal Geol. 70 (1-3), 223–239. doi:10.1016/j.coal.2006.05.001
Clarkson, C., Solano, N., Bustin, R., Bustin, A. M. M., Chalmers, G., He, L., et al. (2013). Pore structure characterization of North American shale gas reservoirs using USANS/SANS, gas adsorption, and mercury intrusion. Fuel 103, 606–616. doi:10.1016/j.fuel.2012.06.119
Curtis, M., Cardott, B., Sondergeld, C., and Rai, C. (2012). Development of organic porosity in the Woodford Shale with increasing thermal maturity. Int. J. Coal Geol. 103, 26–31. doi:10.1016/j.coal.2012.08.004
Fu, C. Q., Zhu, Y. M., and Chen, S. (2015). Diagenesis controlling mechanism of pore characteristics in the qiongzhusi Formation shale, east yunnan. J. China Coal Soc. 40, 439–448. doi:10.13225/j.cnki.jccs.2014.1588
Fu, J., Guo, S., Liu, X., and Wang, Y. (2013). Shale gas accumulation condition and exploration potential of the upper paleozoic Shanxi Formation in Ordos Basin. J. Jilin Univ. (Earth Sci. Ed. 43 (02), 382–389. doi:10.13278/j.cnki.jjuese.2013.02.001
Gai, S., Liu, H., He, S., Mo, S., Chen, S., Liu, R., et al. (2016). Shale reservoir characteristics and exploration potential in the target: A case study in the longmaxi formation from the southern sichuan basin of China. J. Nat. Gas Sci. Eng. 31, 86–97. doi:10.1016/j.jngse.2016.02.060
Guo, W., Liu, H., Xue, H., Lan, C., and Tang, X. (2015). Depositional facies of permian Shanxi formation gas shale in the Northern Ordos Basin and its impact on shale reservoir. Acta Geol. Sin. 89 (05), 931–941. doi:10.1108/WJE-06-2016-031
He, M., Wang, M., and Qiu, R. (2012). Multi-stage composite superimposed basins in North South China and oil and gas. Beijing: Geological Publishing House, 45–47.
He, X., Zhang, P., He, G., Gao, Y., Liu, M., Zhang, Y., et al. (2020). Evaluation of sweet spots and horizontal-well-design technology for shale gas in the basin-margin transition zone of southeastern Chongqing, SW China. Energy Geosci. 1 (3–4), 134–146. doi:10.1016/j.engeos.2020.06.004
Jiang, S., Xu, Z., Feng, Y., Zhang, J., Cai, D., Chen, L., et al. (2015). Geologic characteristics of hydrocarbon-bearing marine, transitional and lacustrine shales in China. J. Asian Earth Sci. 115, 404–418. doi:10.1016/j.jseaes.2015.10.016
Kong, L., Wan, M., Yan, Y., Zou, C., Liu, W., Tian, C., et al. (2016). Reservoir diagenesis research of silurian longmaxi Formation in sichuan basin, China. J. Nat. Gas Geoscience 23, 203–211. doi:10.1016/j.jnggs.2016.08.001
Kruk, M., and Jaroniec, M. (2001). Gas adsorption characterization of ordered Organic−Inorganic nanocomposite materials. Chem. Mat. 13, 3169–3183. doi:10.1021/cm0101069
Li, H. (2022). Research progress on evaluation methods and factors influencing shale brittleness: A review. Energy Rep. 8, 4344–4358. doi:10.1016/j.egyr.2022.03.120
Li, Y. (2021). Mechanics and fracturing techniques of deep shale from the Sichuan Basin, SW China. Energy Geosci. 2 (1), 1–9. doi:10.1016/j.engeos.2020.06.002
Li, Y., Zhou, D. H., Wang, W. H., Jiang, T. X., and Xue, Z. J. (2020). Development of unconventional gas and technologies adopted in China. Energy Geosci. 1 (1–2), 55–68. doi:10.1016/j.engeos.2020.04.004
Loucks, R., Reed, R., Ruppel, S., and Jarvie, D. (2009). Morphology, genesis, and distribution of nanometer-scale pores in siliceous mudstones of the mississippian barnett shale. J. Sediment. Res. 79, 848–861. doi:10.2110/jsr.2009.092
Lu, J., Ruppel, S. C., and Rowe, H. D. (2015). Organic matter pores and oil generation in the Tuscaloosa marine shale. Am. Assoc. Pet. Geol. Bull. 99, 333–357. doi:10.1306/08201414055
Ma, X., Guo, S., Shi, D., Zhou, Z., and Liu, G. (2019). Investigation of pore structure and fractal characteristics of marine-continental transitional shales from Longtan Formation using MICP, gas adsorption, and NMR (Guizhou, China). Mar. Petroleum Geol. 107, 555–571. doi:10.1016/j.marpetgeo.2019.05.018
Nie, H., Li, D., Liu, G., Lu, Z., Hu, W., Wang, R., et al. (2020). An overview of the geology and production of the Fuling shale gas field, Sichuan Basin, China. Energy Geosci. 1 (3–4), 147–164. doi:10.1016/j.engeos.2020.06.005
Qi, Y., Ju, Y., Huang, C., Zhu, H., Bao, Y., Wu, J., et al. (2019). Influences of organic matter and kaolinite on pore structures of transitional organic-rich mudstone with an emphasis on S2 controlling specific surface area. Fuel 237, 860–873. doi:10.1016/j.fuel.2018.10.048
Tissot, B. P. (1978). Petroleum formation and occurrence. Eos Trans. AGU. 55, 643–645. doi:10.1029/eo066i037p00643
Wang, F., and Yin, S. (2019). Hydrocarbon generation potential of the C-P continental source rocks in the Qinshui Basin. Petroleum Sci. Technol. 37 (2), 208–214. doi:10.1080/10916466.2018.1533865
Wang, G., Ju, Y., Yan, Z., and Li, Q. (2015). Pore structure characteristics of coal–bearing shale using fluid invasion methods: A case study in the huainan–huaibei coalfield in China. Mar. Petrol. Geol. 62, 1–13. doi:10.1016/j.marpetgeo.2015.01.001
Wang, P., Chen, Z., Jin, Z., Jiang, C., Jia, Z., Guo, Y., et al. (2018). Shale oil and gas resources in organic pores of the Devonian Duvernay Shale , Western Canada Sedimentary Basin based on petroleum system modeling. J. Nat. Gas. Sci. Eng. 50, 33–42. doi:10.1016/j.jngse.2017.10.027
Xi, Z., Tang, S., Zhang, S., and Li, J. (2017). Nano-scale pore structure of marine-continental transitional shale from liulin area, the eastern margin of Ordos Basin, China. J. Nanosci. Nanotechnol. 17, 6109–6123. doi:10.1166/jnn.2017.14501
Xiong, F., Jiang, Z., Li, P., Wang, X., Bi, H., Li, Y., et al. (2017). Pore structure of transitional shales in the Ordos Basin, NW China: Effects of composition on gas storage capacity. Fuel 206, 504–515. doi:10.1016/j.fuel.2017.05.083
Xu, H., Zhou, W., Zhang, R., Liu, S., and Zhou, Q. (2019). Characterizations of pore, mineral and petrographic properties of marine shale using multiple techniques and their implications on gas storage capability for Sichuan Longmaxi gas shale field in China. Fuel 241, 360–371. doi:10.1016/j.fuel.2018.12.035
Yan, D., Huang, W., and Zhang, J. (2015). Characteristics of marine continental transitional organic rich shale in the Ordos Basin and its shale gas significance. Earth Sci. Froniters 22 (6), 197–206. doi:10.13745/j.esf.2015.06.015
Yang, C., Zhang, J., Tang, X., Ding, J., Zhao, Q., Dang, W., et al. (2016). Comparative study on micro-pore structure of marine, terrestrial, and transitional shales in key areas, China. Int. J. Coal Geol. 171, 76–92. doi:10.1016/j.coal.2016.12.001
Yang, H., Li, S. X., and Liu, X. Y. (2010). Characteristics and resource prospects of tight oil and shale oil in Ordos Basin. Acta Pet. Sin. 34 (1), 1–11. doi:10.7623/syxb201301001
Yu, K., Shao, C., Ju, Y., and Qu, Z. (2019). The Genesis and controlling factors of micropore volume in transitional coal-bearing shale reservoirs under different sedimentary environments. Mar. Petroleum Geol. 102, 426–438. doi:10.1016/j.marpetgeo.2019.01.003
Zhang, Q., Liu, Z., Liu, C., Zhu, X., Steel, R., Tian, H., et al. (2021). Experimental study on the development characteristics and controlling factors of microscopic organic matter pore and fracture system in shale. Front. Earth Sci. (Lausanne). 7, 1–12. doi:10.3389/feart.2021.773960
Zheng, H., Zhang, J., and Qi, Y. (2020). Geology and geomechanics of hydraulic fracturing in the Marcellus shale gas play and their potential applications to the Fuling shale gas development. Energy Geosci. 1 (1–2), 36–46. doi:10.1016/j.engeos.2020.05.002
Keywords: South North China Basin, marine-continental transitional facies, shale gas, organic matter, pore structure
Citation: Peng Y, Pan J and An X (2022) A preliminary study on the geological conditions and indexes for the accumulation of shale gas in the marine-continental transitional facies sediments in the South North China Basin. Front. Earth Sci. 10:985302. doi: 10.3389/feart.2022.985302
Received: 03 July 2022; Accepted: 19 July 2022;
Published: 22 August 2022.
Edited by:
Hu Li, Southwest Petroleum University, ChinaCopyright © 2022 Peng, Pan and An. This is an open-access article distributed under the terms of the Creative Commons Attribution License (CC BY). The use, distribution or reproduction in other forums is permitted, provided the original author(s) and the copyright owner(s) are credited and that the original publication in this journal is cited, in accordance with accepted academic practice. No use, distribution or reproduction is permitted which does not comply with these terms.
*Correspondence: Jishun Pan, jspan123@126.com