- 1State Key Laboratory of Oil and Gas Reservoir Geology and Exploitation, Chengdu University of Technology, Chengdu, China
- 2Xihua University, Chengdu, China
- 3Key Laboratory for Mineral Deposits Research, School of Earth Sciences and Engineering, Nanjing University, Nanjing, China
The research on the pre-collisional tectonic evolution of basin-mountain system at the margin of Tibetan plateau provides insights into the upward and outward growth mechanism of the plateau in the Cenozoic. Lower Jurassic Baitianba Formation quartz-rich conglomerate exposed along the edge of the northwest Sichuan Basin whereas these deposits were incompatible with peripheral carbonatite-dominated orogenic belts, which aroused our great interest. To better understand the origin of quartz-rich conglomerate and early erosional unroofing history of northeastern Tibetan Plateau margin, this study focuses on sandstone petrography analysis along with U-Pb age dating of the detrital zircons of Baitianba Formation gravel and sandstone sample from northwestern Sichuan Basin, which is located to north Longmen Shan thrust belt. In the distinctive Lower Jurassic quartz gravel samples, there are at least two distinct provenance sources. One is characterized by a unimodal age population at ca. 400–1,000 Ma but the absence of younger detrital zircons (<395 Ma), resembling the Early Paleozoic marine quartz sandstone due to the uplift and erosion of the Paleozoic basement of the Longmen Shan thrust belt. The other yield age clusters of ca. 1.8 Ga, 950–750 Ma, 450–420 Ma, 280–240 Ma, which is identical to the detrital zircons from the Upper Triassic strata. Furtherly, detrital zircon dating of our sandstone samples also exhibit strong similarity to the Upper Triassic strata detrital record. Detrital zircons linked with the sedimentological data show these quartz-rich conglomerate mainly recycled from the underlying Upper Triassic through long-term weathering.
1 Introduction
The early uplift of the boundary faults and orogenic belts of the Tibetan Plateau did not typically form during the Cenozoic. In reality, the strong collisions of terranes afforded the early uplift of the boundaries of the Tibetan Plateau starting mostly during the Mesozoic. This is attributed to the Qiangtang and Lhasa terranes constituting the middle part of the Tibetan Plateau collapsed sequentially to the southern margin of paleo-Asia during the Mesozoic (Ritts and Biffi, 2000; Tian et al., 2013; Zhu et al., 2013; Qi et al., 2016; Cheng et al., 2019).
Notably, the tectonic evolution of the Sichuan Basin, China’s largest natural gas producer located on the eastern margin of the Tibetan Plateau, was mainly controlled by the tectonic evolution of the Longmen Shan thrust belt (Liu et al., 2021). Therefore, the study of the early uplift history of the Longmen Shan thrust belt provides an insight into the formation and preservation of natural gas in the deep Sichuan Basin. Unfortunately, whether the early uplift of the Longmen Shan thrust belt formed the eastern margin of the Tibetan Plateau since the Mesozoic or not remains controversial. First, during the Late Triassic, the Longmen Shan thrust belt was uplifted due to the collision between the Qiangtang and Kunlun terranes during the closure of the paleo-Tethys ocean (Weislogel et al., 2006; Li et al., 2007; Zhan et al., 2018). Second, since the Early Jurassic, the continued northward extrusion of the Qiangtang terrane after the Qiangtang–Kunlun collision led to the early uplift of the Longmen Shan thrust belt (Meng et al., 2005; Deng et al., 2012; Yan et al., 2018).
The crux of this controversy is the question of which Early Mesozoic conglomerate (Upper Triassic or Lower Jurassic) most closely represents the early uplift and unroofing of the Longmen Shan thrust belt. The lack of research on the quartz-rich conglomerate of the Lower Jurassic contributes to the uncertainty. Thus, herein, the Jinzishan ancient alluvial fan preserved on the northwestern margin of the Sichuan Basin was selected for the detailed analyses of sedimentology, detrital components and geochronology to trace the early uplift and unroofing history of the Longmen Shan thrust belt.
2 Geological background
The Sichuan Basin is located at the northwestern margin of the South China Block, separated from the Songpan-Ganze and Bikou terranes to the northwest by the Longmen Shan thrust belt and from the North China Block to the north by the Qinling Orogen (Figure 1C). Due to the oblique collision between North China and South China Block at the end of Triassic, the basin evolved from Sinian (Ediacaran)-Middle Triassic passive margin into a Late Triassic-Cenozoic foreland basin (Mu et al., 2019; Yan et al., 2019; Liu et al., 2021 and references therein). The Qinling Orogenic belt was also the result of this collision overprinted by the Late Jurassic to Cenozoic deformation. Micang-Daba Shan, as well as North Longmen Shan, was shaped as the foreland belts of the SW Qinling orogenic belt (Yan et al., 2018), and the main tectonic style subsequently transformed into extensional collapse during the Early Jurassic (Meng et al., 2005; Dong et al., 2011; Yan et al., 2011; Dong and Santosh, 2016).
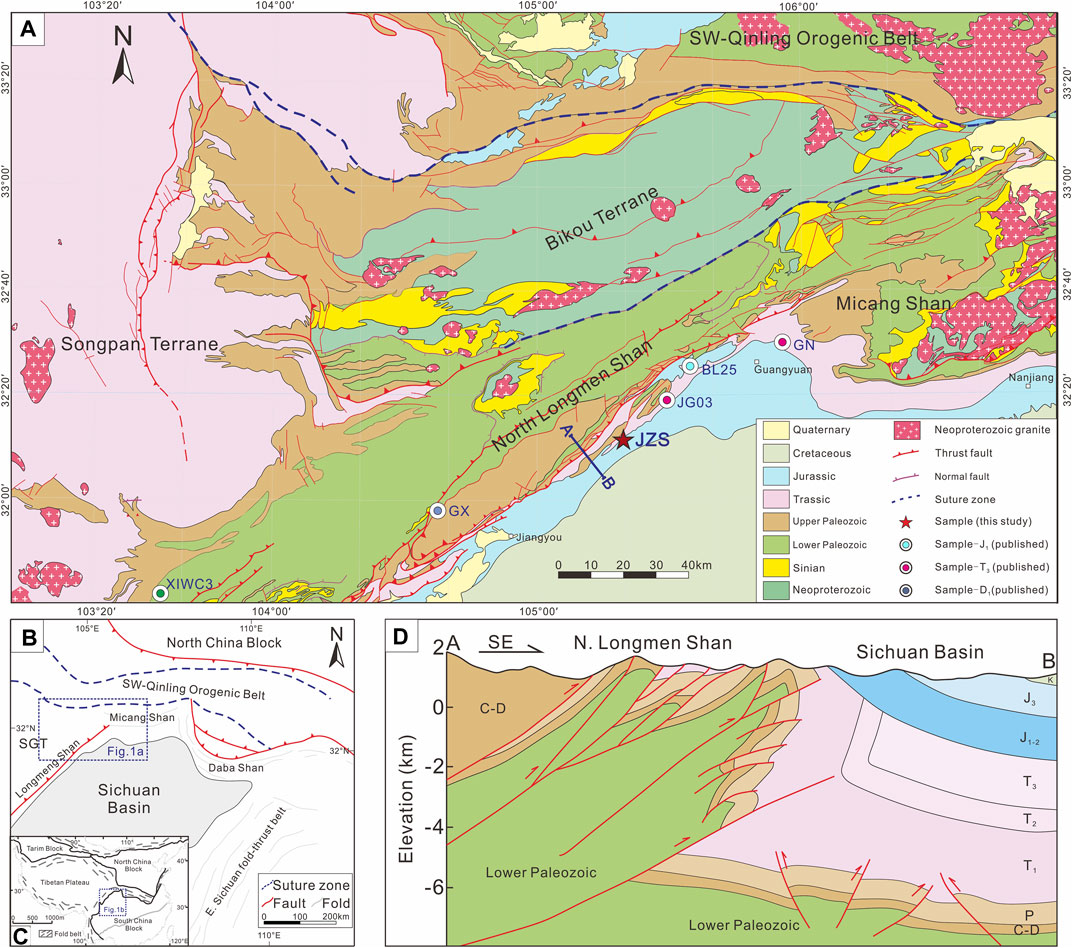
FIGURE 1. (A) Geological map of the northwestern Sichuan Basin and north Longmen Shan orogenic belt (adapted from Yan et al., 2018). The red star indicates the Jinzishan outcrop (JZS) analysed herein. (B) Tectonic setting of Sichuan basin and adjoining regions (adapted from Ma et al., 2019). (C) Simplified tectonic framework map (adapted from Yan et al., 2018). (D) Cross shows tectonic features and stratigraphic distribution in the study area, modified from Wang et al., 2014.
In the study area, the Lower Jurassic rest unconformably on deformed Upper Triassic and older rocks along the front zone of the North Longmen Shan thrust belt (Figure 2A). It consists of the thickly bedded conglomerate (Figures 2B,C), cross-bedded sandstone (Figure 2E) and interbed coal (Figure 2F) deposited in alluvial fan whereas changes to clastic rocks and limestone dominate in the basin interior (Meng et al., 2005). Samples were collected from the Jinzishan (N32°11′17″, E105°19′24″) Jurassic paleo-alluvial fan for conglomerate compositions, sandstone detritals and geochronology (Figure 3). Paleocurrent measurements indicate southeastward paleoflows.
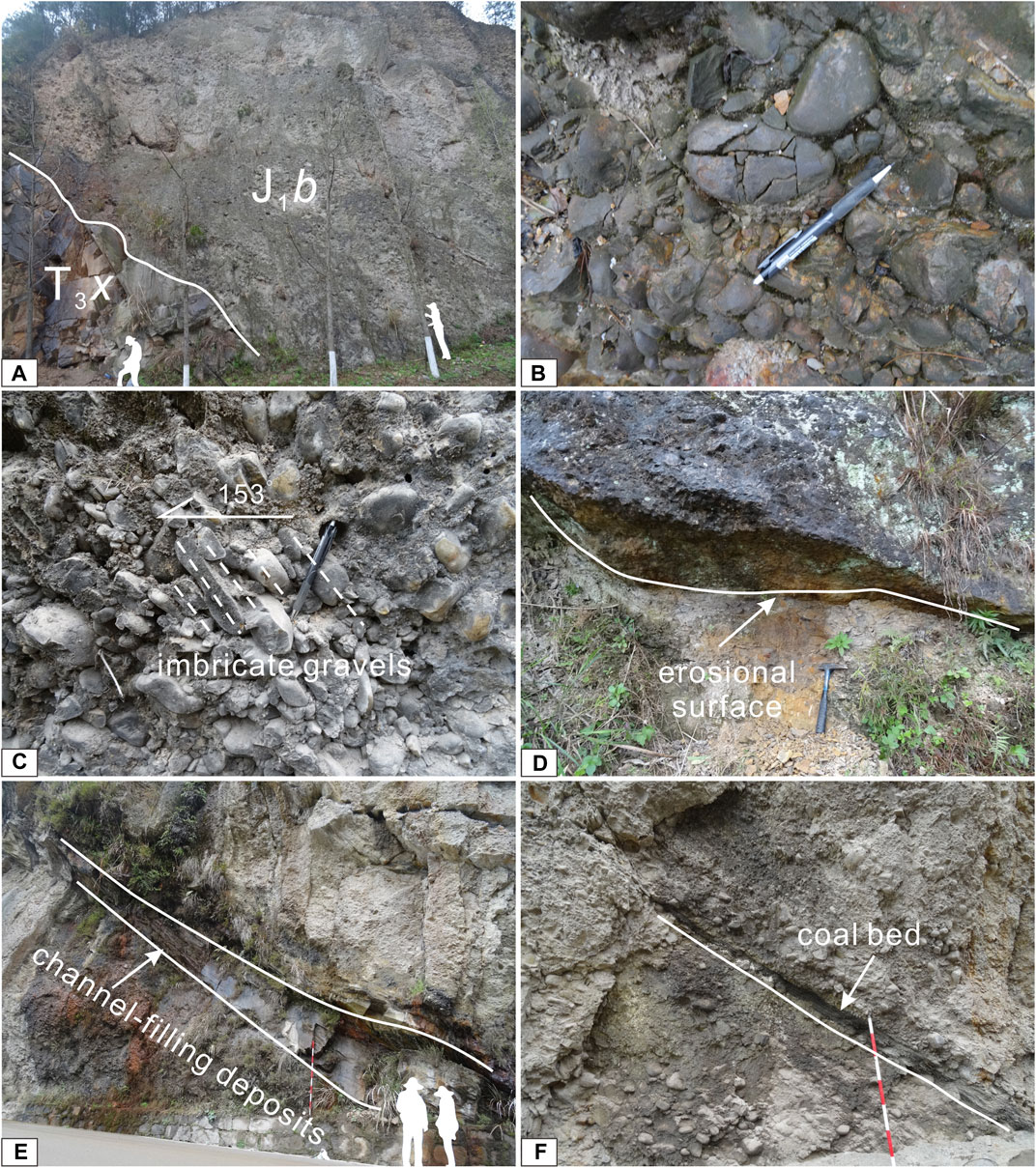
FIGURE 2. Photographs of the Lower Jurassic strata in the Jinzishan outcrop, northwestern Sichuan Basin. (A) The unconformity between Upper Triassic Xujiahe Fm and the overlying Baitianba Fm. (B) The well-rounded nature of the quartz clasts forming the Baitianba conglomerate. (C) Gravel imbrication indicates southeastward paleoflows. (D) Represents an erosional basal surface. (E) Channel-filling deposits. (F) Shows an interbedded coal bed.
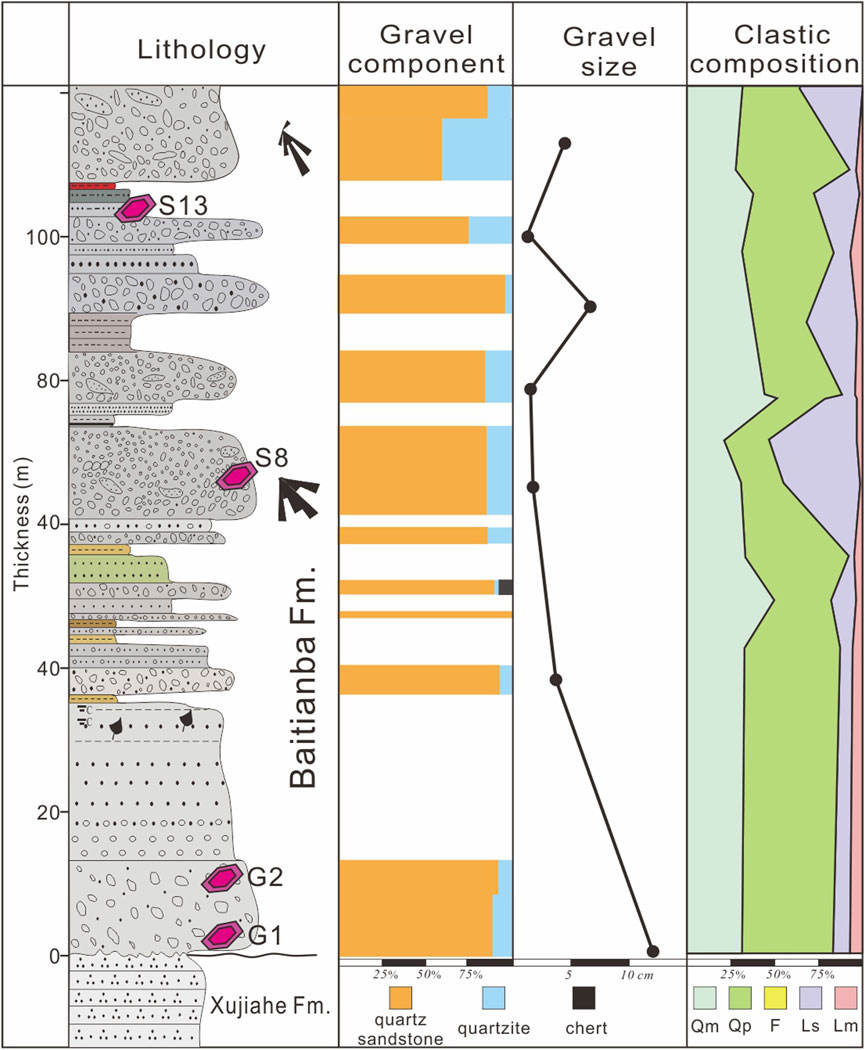
FIGURE 3. Lithostratigraphy, gravel component and sandstone composition of the Jinzishan outcrop. Qm: monocrystalline quartz; Qp: polycrystalline quartz; F: feldspar; Ls: sedimentary rock fragments; Lm: metamorphic rock fragments.
3 Methods and results
3.1 Gravel and sandstone petrography
The field investigation observed that Baitianba Formation conglomerate is composed of the well-rounded nature of the quartz clasts in Jinzishan outcrop, especially quartz-sandstone (Figure 3), and differ from the Baitianba formation dominated by quartzite and chert gravels in the Micang-Daba Shan (Qian et al., 2015). In order to determine trustier component of the gravels, twenty-two quartz gravel samples were collected from the lower part of the Jinzishan outcrop from which polished thin sections were composed to analyse microscopic features. First, the detrital composition of 19 gravel samples was coarse–fine quartz sandstones, dominated by siliceous cemented dense quartz sandstones with durability similar to vein quartz, chert and quartzite (Figure 3, Figures 4A–C), consistent with quartz-pebble conglomerates (QPC) (Cox et al., 2002; Youngson et al., 2006). Second, another two gravel samples are fine conglomerates. Finally, only one gravel is lithic sandstone.
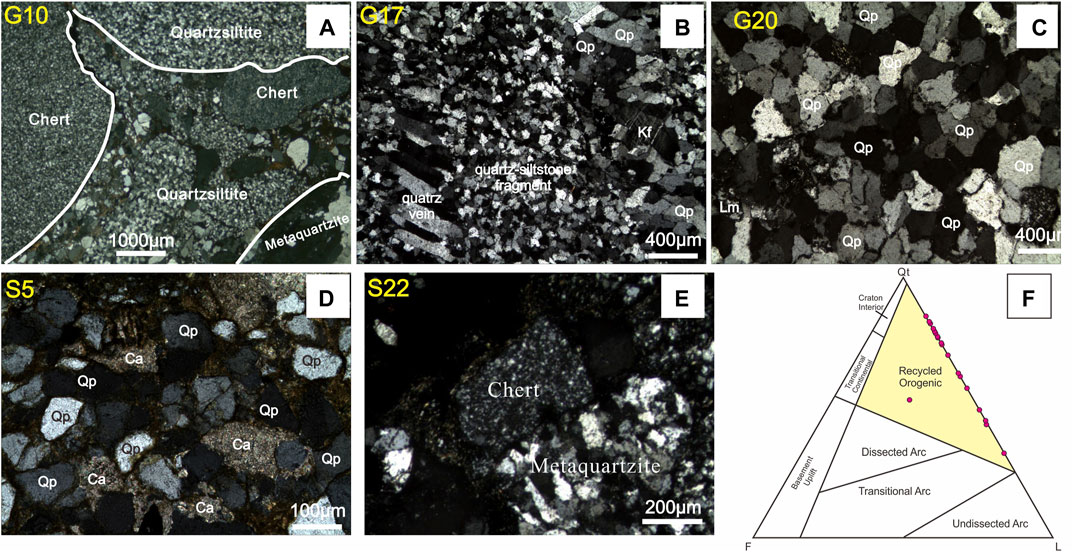
FIGURE 4. (A–C) Representative cross-polarized light photomicrograph of gravel samples. (D,E) Representative cross-polarized light photomicrograph of sandstone samples. (F) Qt-F-L ternary diagram displays the relative abundance of framework grains in sandstone, Qt: total quartz, F, feldspar, L: lithic rock fragments, Ca: carbonate fragment.
Twenty-nine sandstone samples were collected from the lithic sandstone layer in the Jinzishan. The microscopic characteristics and debris components divided the sandstone into 3 types, including rock-fragment sandstone, lithic quartz sandstone and lithic feldspathic sandstone. We used cross-polarization microscope to observe the petrographic features, including mineral composition, grain size, sorting and roundness. In general, the detrital components of Baitianba Formation sandstone are quartz rich with a percentage composition range 33–85, whereas the feldspar content is extremely low, the lithics observed in the samples are mostly the sandstone, siltstone, argillite, chert and mica. Significantly, carbonate fragment is only found in the top of the Jinzishan outcrops (Figure 4D). The modal compositions of sandstone samples plot near the boundary of “recycled orogenic” field in the QtFL diagram (Figure 4F).
3.2 Detrital zircon geochronology
Quart-rich conglomerates can be attributed to potential source in the thrust belt on the basis of their detrital zircon age population (Lawton et al., 2010). In this study, two gravel and two sandstone samples (about 5 kg each) were collected from Lower Jurassic Jinzishan outcrops, and zircon grains were extracted following the standard procedures outlined in Li et al. (2007). Chengxin Geology Service Co. Ltd., Langfang, China performed this work. To avoid sampling bias, zircon crystals (N200 grains) were mounted in epoxy resin without handpicking and polished to obtain a smooth internal surface. Zircon U–Pb dating was performed using laser ablation-inductively coupled plasma mass spectrometry (LA-ICPMS) at the CUGB LaserChron Center. All four samples were ablated using a 30-μm diameter laser beam. The U–Pb fractionation results were corrected using the zircon standard 91500 reference, affording 324 concordant data points for the four samples. The U-Pb concordia diagrams for zircon grains of each sample are shown in Figure 5 and U–Pb age data set is provided as supplementary files in excel format (Supplementary File S1).
3.2.1 gravel sample G1
Gravel sample G1 was taken from the bottom of the Lower Jurassic outcrop. 87 valid DZ age data points ranging from 231 to 3,069 Ma, with peaks in five major age intervals: 230–290 Ma; 425–460 Ma; 740–1,100 Ma; 1,650–1,700 Ma; and 1,860–2,000 Ma were obtained, with minimum zircon ages constraining the age of deposition of its parent rock not earlier than the Late Triassic.
3.2.2 gravel sample G2
Gravel sample G2 was also derived from the bottom of this outcrop with 85 valid DZ age data points ranging from 395 to 2,829 Ma, with peaks at 540–640 Ma; 760–1,200 Ma; 1,500–1,690 Ma; 2,430–2,500 Ma, and about 53% of clastic zircons of Neoproterozoic age.
3.2.3 Sandstone sample S8
Sample S8 was taken from the lenticular sandstone formation within the conglomeratic strata, identified as lithic sandstone. Ninety valid DZ ages ranged from 211 to 2,647 Ma, with peaks in five main age intervals of 211–350 Ma; 410–490 Ma; 600–900 Ma; 1,700–2,000 Ma; and 2,400–2,600 Ma, with weaker peaks in 1,000–1,150 Ma.
3.2.3 Sandstone sample S13
Sample S13 was collected from the sandstone layer with 92 valid DZ ages ranging from 232 to 2,647 Ma, of which detrital zircons from 1,800 to 2,000 Ma account for 43% of the total quartz grains, showing a obvious age peak. The remaining zircon ages are distributed within the 200–300 Ma, 350–500 Ma and 2,300–2,400 Ma sub-age intervals.
4 Discussion
In previous studies, based on the siliceous characteristics of the Lower Jurassic Baitianba Formation in the northwestern part of the Sichuan Basin, these QPC were arbitrarily considered to be mainly derived from Paleozoic Devonian quartz-sandstones deposited at the western margin of the Sichuan Basin (Cui et al., 1991) and combined with the underlying carbonate-rich conglomerates of the Upper Triassic Xujiahe Formation (Figure 6). Previous studies have proposed that these two sets of lithologically distinct conglomerates should represent the uplift erosion of the Longmen Shan thrust belt (Meng et al., 2005; Deng et al., 2012). However, this explanation for the detrital provenance of the Lower Jurassic QPC could not resolve the presence of carbonate strata over a 4-km distance from Triassic to Devonian (Liu et al., 2021), implying that Lower Devonian quartz sandstones from a 1.0–1.5 km range could not be the sole provenance of the Baitianba QPC. To further explore this paradox, the detrital zircon U–Pb ages from the gravel and sandstone samples were used to trace true provenance matching published detrital zircon data from possible provenance areas.
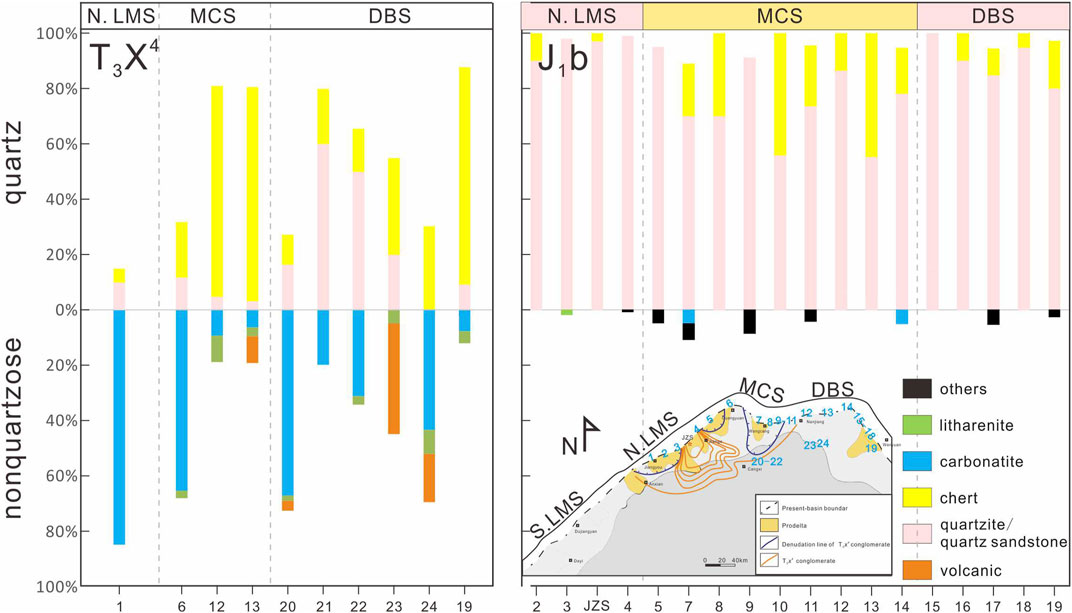
FIGURE 6. Comparison of conglomerate compositions between the Upper Triassic Xujiahe Formation and the Lower Jurassic Baitianba Formation in the NW Sichuan Basin. JZS-Jinzishan, 1-Jiangyou Qinglingkou, 2- Jiangyou Daliangshang, 3- Jiangyou Erlangmiao, 4- Baitianba, 5- Guangyuan Baolun, 6- Gongnong, 7- Guangyuan Baishui, 8-Wangcang, 9-Cangxi Puji, 10- Wangcang Sangjiang, 11- Wangcang Jinxi, 12-Nanjiang; 13- Tongjiang Pingxi; 14- Tongjiang Lianghekou; 15- Tongjiang Tiexi, 16- Wanyuan Zhuyu, 17- Wanyuan Huangzhong; 18- Wanyuan Jiuyuan, 19- Wanyuan Gujun, 20- Well YB6, 21- Well YB123, 22- Well YB203, 23- Well YB204, 24- Well M201.
We performed multiple comparisons between the kernel density estimate (KDE, Figures 7A–H), cumulative age distribution (CAD, Figure 7I), and multidimensional scaling analysis (MDS, Figure 7J) of detrital zircons to demonstrate a similarity between the Lower Jurassic Baitianba Formation and probable provenance strata (Vermeesch, 2018). On the MDS diagram, the data points are distinctly divided into two different parts: 1) samples G1, S8 and S13 are highly correlated with the Upper Triassic Xujiahe Formation with notable peaks within 1,800–2,000 Ma; 2) sample G2 shows a typical “Paleozoic basement-type” feature of Lower Paleozoic quartz sandstone similar with sample BL25 from the northern Sichuan Basin (Li et al., 2012). However, the MDS interpretation reflects that the age spectra of the detrital zircons from the gravel samples were significantly different, indicating derivation from the underlying Upper Triassic Xujiahe Formation and the deeper Paleozoic basement of the Longmen Shan thrust belt, respectively. In addition, it is necessary to evaluate the topographic relief and denudation degree of the Longmen Shan thrust belt to quantify the mixing ratio of the two different provenances to the detrital material of the Lower Jurassic Baitianba Formation based on recycled features of the QPC. The DZ ages of gravel G1 and G2 represent the age spectra of the Upper Triassic Xujiahe sandstone and the Lower Paleozoic basement, respectively, which could be defined as a provenance zircon feature. The sandstone samples (S8 and S13) derived from the sandstone lenticular in the lower part of the alluvial fan and the sandstone layer in the upper part of the Jinzishan outcrop as well as the other sample (BL25, Figure 7F) from the Baolun outcrop are regarded as mixed sediments and statistically simulated based on the DZmix program of MATLAB (Sundell and Saylor, 2017). All of S13 was from the Upper Triassic Xujiahe Formation; all of Bl25 was derived from the Lower Paleozoic basement and S8 was collected from the above two provenances: 80.3% from eroded Xujiahe Formation strata and 19.7% from the Lower Paleozoic basement (Figure 7K).
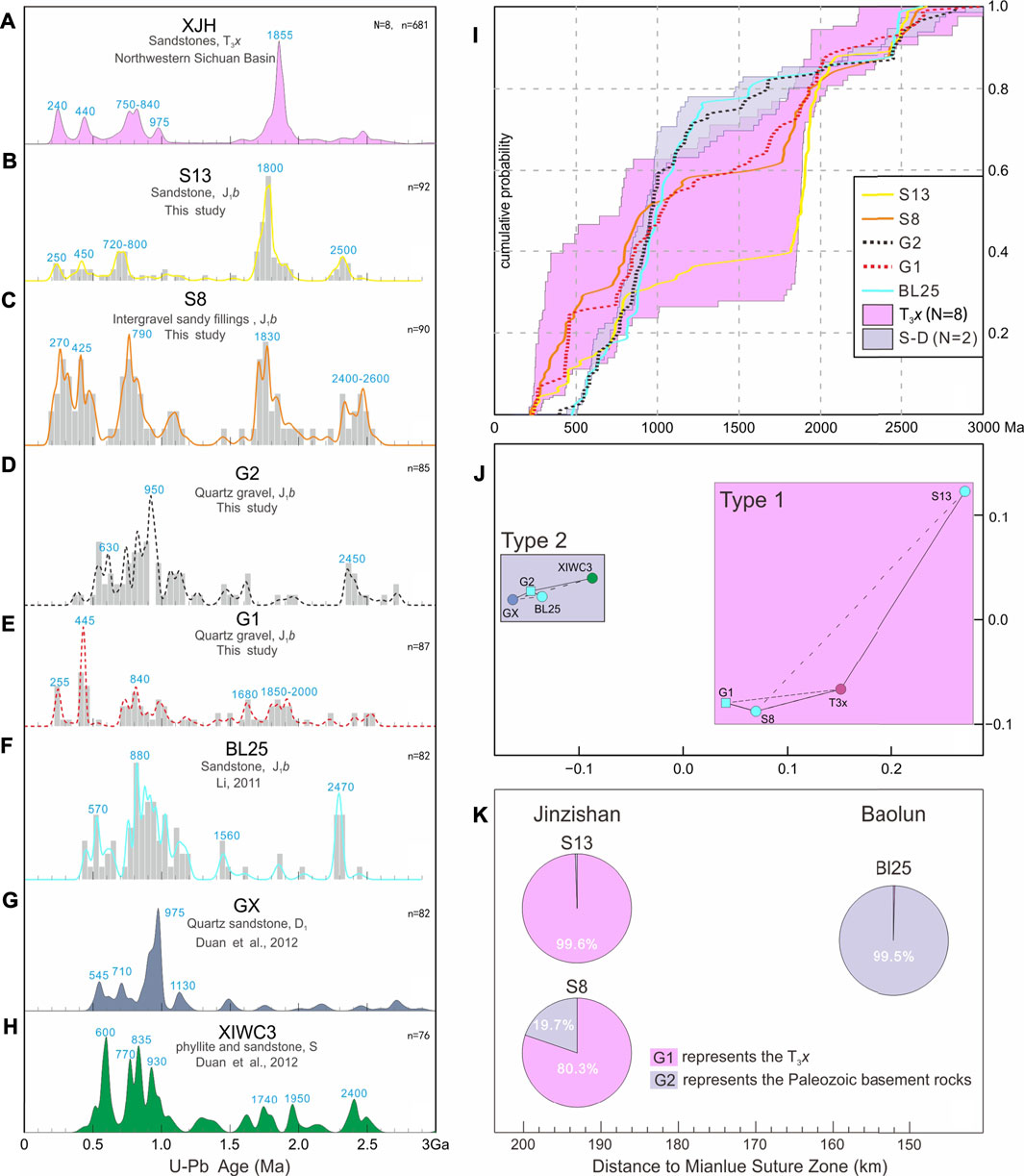
FIGURE 7. (A–H) Zircon U–Pb age cumulative probability distribution for each sample. XJH: Spectra of Upper Triassic Xujiahe Formation sediments in northwestern Sichuan Basin are taken from previous studies (Chen et al., 2011; Luo et al., 2014; Shao et al., 2016; Mu et al., 2019); G1, G2, S8 and S13 are new samples collected from the Lower Jurassic strata in the Jinzishan section; BLl25 is a Lower Jurassic sandstone sample from Li. (2013); Lower Devonian sample GX and Silurian sample XIWC3 are thrust belt source units in Longmen Shan, from Vermeesch, 2018 and Duan et al. (2012), respectively. (I) Cumulative distribution plot (CDF) of all samples; (J) Multidimensional scaling map (Vermeesch, 2018), showing the distinct signature of Baitianba Formation, Type 1 is similar to Upper Triassic continental deposits, and Type 2 is a population distribution similar to that of Lower Paleozoic Marine quartz-rich sandstones of the Longmen Shan thrust belt. The multi-dimensional scaling (MDS) map uses the Kolmogorov–Smirnov (KS) statistic for detrital zircon U–Pb datasets (Stevens et al., 2013; Vermeesch, 2018). (K) Unmixing detrital geochronology age distribution.
The above spatial features of detrital zircons have indicated the following. First, the northern Guangyuan area was eroded to the Paleozoic basement in the Early Jurassic and second, the detritus of the Lower Jurassic in the southern Jinzishan area should be doubly supplied from the Upper Triassic and Lower Paleozoic quartz sandstones. However, the Upper Triassic Xujiahe sandstone mainly comprises lithic sandstone with <5% of quartz sandstone resembling the detritus of the Lower Jurassic QPC. At the same time, marine carbonates over a 4-km distance between the Lower Devonian and Upper Triassic only appear in the detrital grains of the upper sandstone member and not be found in the Jinzishan conglomerates. Thus, neither the Upper Triassic nor the Lower Devonian could directly supply the Lower Jurassic QPC. Therefore, the lithologic features of the Lower Jurassic QPC imply that only those quartz-rich conglomerates with high resistance to weathering and abrasion that are among the composite conglomerates in periphery provenance areas could have been retained to form the Lower Jurassic QPC (Cui et al., 1991; Qian et al., 2015).
Herein, a new depositional model of the Lower Jurassic QPC was reconstructed. First, during the depositional process of the provenance area, the southwardly thrusting South Qinling orogenic belt formed a foreland basin in the northern Longmen Shan thrust belt as well as provided a large amount of detrital sediment during the Late Triassic (Qian et al., 2015; Mu et al., 2019). Meanwhile, the southward expansion of the thrust front afforded the absence of the upper part of the Xujiahe Formation (V and VI members) in the northwestern Sichuan Basin (Li Y et al., 2003; Deng et al., 2012). Second, during the weathering and leaching process of the provenance area, the Upper Triassic composite conglomerates were exposed to the surface and subjected to weathering and leaching, dissolving unstable carbonate and sandstone gravels in rainwater, except for hard quartz conglomerates (Cox et al., 2002; Youngson et al., 2006). Finally, as QPC were deposited, a southeast-tilting gently oblique zone formed in the northern Longmen Shan thrust belt. This occurred because the Bikou terrane was uplifted during regional extension in the Early Jurassic (Yan et al., 2018), allowing the weathering conglomerates to be transported into the Sichuan Basin. In the transporting process, the unstable components of the former composite conglomerates continued to be diluted, directly increasing the percentage of stable quartz-rich conglomerates and eventually forming a QPC alluvial fan in the study area underlying the gently sloping topography (Figure 8).
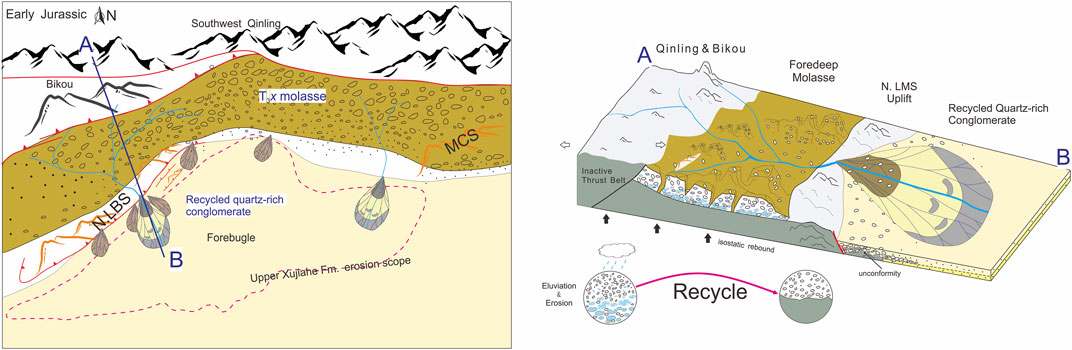
FIGURE 8. Depositional model of the QPC of the Lower Jurassic Baitianba Formation in the northern part of the Longmen Shan orogenic belt.
5 Conclusion
1) Detrital zircon U–Pb geochronology and petrography of tow Lower Jurassic gravel samples from Jinzishan outcrop reveal at least two source of quartz-rich conglomerate. One sample is characterized by the absence of younger detrital zircons (<395 Ma), resembling the Early Paleozoic marine quartz sandstone due to the uplift and erosion of the Paleozoic basement in the North Longmen Shan thrust belt. The other yield age clusters of ca. 1.8 Ga, 950–750 Ma, 450–420 Ma, and 280–240 Ma, which is identical to the detrital zircons from the Upper Triassic strata. Our results indicated Upper Triassic to Early Paleozoic strata had uplifted and suffered from erosion in adjacent Longmen Shan thrust belt before the Early Jurassic.
2) U–Pb dating of Baitianba Formation sandstone also exhibit strong similarity to the Upper Triassic strata detrital record.
3) The feature of Baitianba conglomerate fits the definition of QPCnew depositional model of the Lower Jurassic conglomerates mainly recycled from the uplifted Upper Triassic strata in the north Longmen Shan thrust belt through long-term weathering.
Data availability statement
The original contributions presented in the study are included in the article/Supplementary Material, further inquiries can be directed to the corresponding author.
Author contributions
BR and SL conceived and designed the study. ZW measured Chronological data. FL and BR wrote the first draft of the manuscript. XL provided constructive suggestions to improve the work. YH, KL, TS, and FL edited and reviewed the manuscript.
Funding
This study was financially supported by the National Natural Science Foundation of China (No. 42072129).
Acknowledgments
We thank Liangliang Zhang for contribution to the laboratory work. And we thank the reviewers for review suggestions of this work.
Conflict of interest
The authors declare that the research was conducted in the absence of any commercial or financial relationships that could be construed as a potential conflict of interest.
Publisher’s note
All claims expressed in this article are solely those of the authors and do not necessarily represent those of their affiliated organizations, or those of the publisher, the editors and the reviewers. Any product that may be evaluated in this article, or claim that may be made by its manufacturer, is not guaranteed or endorsed by the publisher.
Supplementary material
The Supplementary Material for this article can be found online at: https://www.frontiersin.org/articles/10.3389/feart.2022.982354/full#supplementary-material
References
Chen, Q., Sun, M., Long, X. P., Zhao, G. C., and Yuan, C. (2016). U–Pb ages and Hf isotopic record of zircons from the late neoproterozoic and silurian–devonian sedimentary rocks of the western yangtze block: Implications for its tectonic evolution and continental affinity. Gondwana Res. 31, 184–199. doi:10.1016/j.gr.2015.01.009
Chen, Y., Liu, S. G., Li, Z. W., Deng, B., Zeng, X. L., and Lin, J. (2011). LA-ICP-MS detrital zircon U-Pb geochronology approaches to the sediment provenance of the Western Sichuan foreland Basin and limited uplift of the longmen mountains during the early stage of Late Triassic. Geotecton. Metallog. 35 (2), 315–323. in Chinese with English abstract. doi:10.3969/j.issn.1001-1552.2011.02.017
Cheng, F., Garzione, C., Jolivet, M., Wang, W., Dong, J., Richter, F., et al. (2019). Provenance analysis of the Yumen Basin and northern Qilian Shan: Implications for the pre-collisional paleogeography in the NE Tibetan plateau and eastern termination of Altyn Tagh fault. Gondwana Res. 65, 156–171. doi:10.1016/j.gr.2018.08.009
Cox, R., Gutmann, E. D., and Hines, P. G. (2002). Diagenetic origin for quartz-pebble conglomerates. Geology 30 (4), 323–326. doi:10.1130/0091-7613(2002)030<0323:DOFQPC>2.0.CO;2
Cui, B. Q., Long, X, M., and Li, Y. (1991). The subsidence of western sichuan depression and the rise of longmenshan mountains. Jour. Chengdu Coll. Geol. 18, 39–45.
Deng, B., Liu, S., Jansa, L., Cao, J., Cheng, Y., Li, Z., et al. (2012). Sedimentary record of Late Triassic transpressional tectonics of the Longmenshan thrust belt, SW China. J. Asian Earth Sci. 48, 43–55. doi:10.1016/j.jseaes.2011.12.019
Dong, Y., and Santosh, M. (2016). Tectonic architecture and multiple orogeny of the qinling orogenic belt, Central China. Gondwana Res. 29 (1), 1–40. doi:10.1016/j.gr.2015.06.009
Dong, Y., Zhang, G., Neubauer, F., Liu, X., Genser, J., and Hauzenberger, C. (2011). Tectonic evolution of the qinling orogen, China: Review and synthesis. J. Asian Earth Sci. 41 (3), 213–237. doi:10.1016/j.jseaes.2011.03.002
Duan, L., Meng, Q. R., Wu, G. L., Ma, S. X., and Li, L. I. N. (2012). Detrital zircon evidence for the linkage of the South China block with Gondwanaland in early palaeozoic time. Geol. Magaz. 149 (6), 1124–1131. doi:10.1017/S0016756812000404
Lawton, T. F., Hunt, G. J., and Gehrels, G. E. (2010). Detrital zircon record of thrust belt unroofing in Lower Cretaceous synorogenic conglomerates, central Utah. Geology 38 (5), 463–466. doi:10.1130/G30684.1
Li, X. H., Chen, F., Guo, J. H., Li, Q. L., Xie, L. W., and Siebel, W. (2007). South China provenance of the lower-grade Penglai Group north of the Sulu UHP orogenic belt, eastern China: Evidence from detrital zircon ages and Nd-Hf isotopic composition. Geochem. J. 41 (1), 29–45. doi:10.2343/geochemj.41.29
Li, Z. W., Liu, S., Chen, H., Deng, B., Hou, M., Wu, W., et al. (2012). Spatial variation in Meso-Cenozoic exhumation history of the Longmen Shan thrust belt (eastern Tibetan Plateau) and the adjacent western Sichuan basin: Constraints from fission track thermochronology. J. Asian Earth Sci. 47, 185–203. doi:10.1016/j.jseaes.2011.10.016
Liu, S., Yang, Y., Deng, B., Zhong, Y., Wen, L., Sun, W., et al. (2021). Tectonic evolution of the sichuan basin, southwest China. Earth. Sci. Rev. 213, 103470. doi:10.1016/j.earscirev.2020.103470
Luo, L., Qi, J. F., Zhang, M. Z., Wang, K., and Han, Y. Z. (2014). Detrital zircon U–Pb ages of Late Triassic–Late Jurassic deposits in the western and northern Sichuan Basin margin: constraints on the foreland basin provenance and tectonic implications. Int. J. of Earth Sci. 103 (6), 1553–1568. doi:10.1007/s00531-014-1032-7
Ma, H. M., Wang, Y., Huang, Y. J., and Xie, Y. T. (2019). Three-stage Mesozoic intracontinental tectonic evolution of South China recorded in an overprinted basin: evidence from stratigraphy and detrital zircon U–Pb dating. Geol. Magaz. 156 (12), 2085–2103. doi:10.1017/S0016756819000451
Meng, Q. R., Wang, E., and Hu, J. M. (2005). Mesozoic sedimentary evolution of the northwest Sichuan basin: Implication for continued clockwise rotation of the South China block. Geol. Soc. Am. Bull. 117 (3-4), 396–410. doi:10.1130/B25407.1
Mu, H., Yan, D. P., Qiu, L., Yang, W. X., Kong, R. Y., Gong, L. X., et al. (2019). Formation of the late triassic western sichuan foreland basin of the qinling orogenic belt, SW China: Sedimentary and geochronological constraints from the Xujiahe Formation. J. Asian Earth Sci. 183, 103938. doi:10.1016/j.jseaes.2019.103938
Qi, B., Hu, D., Yang, X., Zhang, Y., Tan, C., Zhang, P., et al. (2016). Apatite fission track evidence for the Cretaceous–Cenozoic cooling history of the Qilian Shan (NW China) and for stepwise northeastward growth of the northeastern Tibetan Plateau since early Eocene. J. Asian Earth Sci. 124, 28–41. doi:10.1016/j.jseaes.2016.04.009
Qian, T., Liu, S., Li, W., Gao, T., and Chen, X. (2015). Early–middle jurassic evolution of the northern yangtze foreland basin: A record of uplift following triassic continent–continent collision to form the qinling–dabieshan orogenic belt. Int. Geol. Rev. 57 (3), 327–341. doi:10.1080/00206814.2015.1006270
Ritts, B. D., and Biffi, U. (2000). Magnitude of post–Middle Jurassic (Bajocian) displacement on the central Altyn Tagh fault system, northwest China. Geol. Soc. Am. Bull. 112 (1), 61–74. doi:10.1130/0016-7606(2000)112<61:MOPJBD>2.0.CO;2
Shao, T. B., Cheng, N. F., and Song, M. S. (2016). Provenance and tectonic-paleogeographic evolution: Constraints from detrital zircon U–Pb ages of Late Triassic-Early Jurassic deposits in the northern Sichuan basin, central China. J. Asian Earth Sci. 127, 12–31. doi:10.1016/j.jseaes.2016.05.027
Sundell, K. E., and Saylor, J. E. (2017). Unmixing detrital geochronology age distributions. Geochem. Geophys. Geosyst. 18 (8), 2872–2886. doi:10.1002/2016GC006774
Stevens, T., Carter, A., Watson, T. P., Vermeesch, P., Andò, S., and Bird, A. F. (2013). Genetic linkage between the Yellow River, the Mu Us desert and the Chinese loess plateau. Quat. Sci. Rev. 78, 355–368. doi:10.1016/j.quascirev.2012.11.032
Tian, Y., Kohn, B. P., Gleadow, A. J., and Hu, S. (2013). Constructing the Longmen Shan eastern Tibetan Plateau margin: Insights from low-temperature thermochronology. Tectonics 32 (3), 576–592. doi:10.1002/tect.20043
Vermeesch, P. (2018). IsoplotR: A free and open toolbox for geochronology. Geosci. Front. 9 (5), 1479–1493. doi:10.1016/j.gsf.2018.04.001
Wang, L., Chen, Z., Li, B., Lei, Y., and Yan, S. (2014). Structural characteristics of the northern Longmenshan fold-thrust belt and the favorable exploration areas, Sichuan Basin, Southwest China. Petroleum Explor. Dev. 41 (5), 591–597. doi:10.1016/S1876-3804(14)60070-7
Weislogel, A. L., Graham, S. A., Chang, E. Z., Wooden, J. L., Gehrels, G. E., and Yang, H. (2006). Detrital zircon provenance of the late triassic songpan-ganzi complex: Sedimentary record of collision of the north and South China blocks. Geol. 34 (2), 97–100. doi:10.1130/G21929.1
Yan, D. P., Zhou, M. F., Li, S. B., and Wei, G. Q. (2011). Structural and geochronological constraints on the Mesozoic‐Cenozoic tectonic evolution of the Longmen Shan thrust belt, eastern Tibetan Plateau. Tectonics 30 (6). doi:10.1029/2011TC002867
Yan, D. P., Zhou, Y., Qiu, L., Wells, M. L., Mu, H., and Xu, C. G. (2018). The longmenshan tectonic complex and adjacent tectonic units in the eastern margin of the Tibetan plateau: A review. J. Asian Earth Sci. 164, 33–57. doi:10.1016/j.jseaes.2018.06.017
Yan, Z., Tian, Y., Li, R., Vermeesch, P., Sun, X., Li, Y., et al. (2019). Late Triassic tectonic inversion in the upper Yangtze Block: Insights from detrital zircon U–Pb geochronology from south-western Sichuan Basin. Basin Res. 31 (1), 92–113. doi:10.1111/bre.12310
Youngson, J. H., Craw, D., and Falconer, D. M. (2006). Evolution of Cretaceous–Cenozoic quartz pebble conglomerate gold placers during basin formation and inversion, southern New Zealand. Ore Geol. Rev. 28 (4), 451–474. doi:10.1016/j.oregeorev.2005.02.004
Zhan, Q. Y., Zhu, D. C., Wang, Q., Cawood, P. A., Xie, J. C., Li, S. M., et al. (2018). Constructing the eastern margin of the Tibetan plateau during the late triassic. J. Geophys. Res. Solid Earth 123 (12), 10–449. doi:10.1029/2018JB016353
Keywords: early jurassic, quartz-rich conglomerate, detrital zircon, Longmen Shan thrust belt, tectonic evolution
Citation: Lv F, Ran B, Liu S, Wang Z, Sun T, Li X, Han Y and Liang K (2022) Provenance of the lower jurassic quartz-rich conglomerate in northwestern sichuan basin and its link with the pre-collisional unroofing history of the north longmen shan thrust belt, NE tibetan plateau margin. Front. Earth Sci. 10:982354. doi: 10.3389/feart.2022.982354
Received: 30 June 2022; Accepted: 25 July 2022;
Published: 02 September 2022.
Edited by:
Shengyao Yu, Ocean University of China, ChinaReviewed by:
Licheng Wang, Institute of Tibetan Plateau Research (CAS), ChinaYushuai Wei, China University of Geosciences, China
Copyright © 2022 Lv, Ran, Liu, Wang, Sun, Li, Han and Liang. This is an open-access article distributed under the terms of the Creative Commons Attribution License (CC BY). The use, distribution or reproduction in other forums is permitted, provided the original author(s) and the copyright owner(s) are credited and that the original publication in this journal is cited, in accordance with accepted academic practice. No use, distribution or reproduction is permitted which does not comply with these terms.
*Correspondence: Bo Ran, cmFuYm8wOEBjZHV0LmNu