- 1School of Mining & Civil Engineering, Liupanshui Normal University, Liupanshui, China
- 2Guizhou Panjiang Coal Power Group Technology Research Institute Co. Ltd, Guiyang, China
- 3School of Energy, China University of Geosciences (Beijing), Beijing, China
The organic-rich shales in the northern Dongpu Depression were deposited in deep and semi-deep lakes based upon evidence from the geochemical characteristic and rock mineral of the Es3 member. The results show that these rocks gradually decrease in abundance and deterioration of organic matter types from the center of the salt lake towards the edges. The development of high-quality shales in Es3 member is due to stratified sedimentation of a deep water, narrow basin water body. The saline lake region has a high TOC content, primarily type I derived from low-grade aquatic organisms. The minerals consist of a variety of mainly clay minerals (31–71%), quartz (11.2–57.9%), plagioclase (3.3–22.1%), dolomite (1.8–19.9%), and pyrite (1–4.1%). The water body was mainly brackish to super-brackish, which has solid limitations and severe salinity stratification, resulting in stable anoxic conditions of the bottom water and conducive to preserving organic matter. The shales were divided into massive silty shale facies, laminated paste shale facies, and laminated gray shale facies according to the mineral composition and microstructure. Comparing the geochemical characteristics, the laminated calcareous shale lithofacies of the deep to semi-deep lacustrine environments favor shale oil and gas occurrence. Pores consist of inorganic pores (intergranular mineral pores, dissolution pores, clay mineral interlayer cracks, mineral intergranular pores micropores, dissolution pores, clay mineral layer cracks, and cracks) with relatively few organic pores. There are many types of natural gas in the study area. The oil-type gas came from the lacustrine shale rock in Es3 member of Shahejie Formation, the coal-derived gas came from the high mature coal-based gas in the deep Carboniferous-Permian system, and the mixed gas was the mixed source of the above two source rocks. Multiple sets of overlying halite provided adequate seals within the depression and were conducive to the accumulation and preservation of hydrocarbons throughout the basin.
1 Introduction
Shale gas mainly occurs in the matrix’s micropores, microfractures, and other lithological interbeds and structural fractures by dissociation and absorption. The result is that gas generated by organic-rich shale cannot be completely discharged and retained or only migrate after a very short distance within the shale and accumulate on the spot. Shale gas are a type of hydrocarbon that is self-generated and self-stored (Schmoker, 2002; Zou et al., 2011; Guo and Zhang, 2014; Tang et al., 2016; Zhou, 2022). Chinese sedimentary basins are characterized by multi-cycle tectonic evolution and develop the three types of organic-rich shales: marine, continental, and marine-continent transitional facies (Guo, 2016; He et al., 2016; Zhang et al., 2016; Zhang et al., 2020; Li et al., 2022). Breakthroughs have been made in shale gas exploration and developing both marine and marine-continent transitional facies. The continental organic-rich shales mainly formed in the water intake system tracts of the lacustrine sedimentary environment. Although the plane distribution is limited to the strongly separated continental environment, organic matter maturity varies significantly due to the large cumulative shale thickness and high organic carbon content (Tian et al., 2017; Zhang et al., 2021). The Dongpu Depression is a saline lake hydrocarbon basin controlled by the water salinity and sedimentary facies belts. Significant differences exist in the development degree of the shale in different regions and periods, and the differences control gas distribution. In the basins where gases coexist with salt, 46% of the oil and gas layers occur below the salt strata; 41% of the basin’s oil and gas layers occur above the salt strata; 13% of the basin’s oil and gas layers occur between the salt strata, indicating the salt influence the reservoirs (Sarg, 2001; Chen et al., 2014).
The salt rock development affects the hydrocarbon accumulation of mud shales in many aspects, including the salt rock origin, the influence on the shale thermal evolution, the influence on reservoir development, and the influence on the migration and accumulation of oils and gases (Volozh et al., 2003; Manzi et al., 2005; Feng et al., 2014; Li et al., 2014; Gao et al., 2015; Wu et al., 2016). In the early stage of the salt rock formation, the bottom water body was stagnant due to the increased water salinity or the water bodies mixed with different salinity, causing extensive anoxia throughout the area. It was beneficial to the preservation of organic matter in a weak oxidizing-reducing environment (Murray, 1991). The original productivity in these types of environments can exceed the high value of a typical marine environment. Furthermore, the salt rock preserves abnormally high pressure under the salt and acts as a good caprock. When the salt structure is active, overpressure is released, creating an extensive oil-gas driving force (Harry and Carney, 1997; Leng et al., 2006; Zhang et al., 2018). The continental shale gas in the northern Dongpu Depression has geological peculiarities in its occurrence state, enrichment strata, reservoir characteristics, accumulation mechanism, recoverable conditions, and development technology. The characteristics and models of the shale gas reservoir conditions of Es3 in the northern Dongpu Depression will be studied, and the reservoir mechanism of continental shale gas should be explored. The results lay an essential foundation for developing new replacement fields of oil and gas in the Dongpu Depression and forming the unconventional exploration theories and hydrocarbon technology in this region.
2 Geological background
The Dongpu Depression is a Mesozoic-Cenozoic continental faulted basin in eastern China, located at the southwest end of the Bohai Bay Basin. It is about 140 km long, 62 km wide to the south, and 14–18 km wide in the north, covering an area of 5,300 km2 (Figure 1). Nearly 90% of the proven hydrocarbon reserves are distributed in the northern salt-bearing area, and the southern salt-free area is poor in resources, where the proven reserves’ abundance per square kilometer is only 1/20 of the northern salt-bearing area. There is a strong relationship between the saline lake environment and the accumulation of oils and gases (Tan et al., 2011; Lu et al., 2012; Tang et al., 2017). The Paleogene’s tectonic evolution history can be divided into four stages: initial rifting stage (sedimentary period of Es4), main rifting stage (sedimentary period of Es3), fault-depression, and atrophy period (sedimentary period of Es2 to Es1), and fault-depression and decline period (sedimentary period of Ed). The Cenozoic Depression is composed of the Paleogene Shahejie Formation (Es), Dongying Formation (Ed), Neogene Guantao Formation (Ng), Minghuazhen Formation (Nm), and Quaternary Plain Formation (Qp).
Shahejie Formation is the leading shale development and oil and gas exploration target in the depression. Es is divided into Es4, Es3, Es2, and Es1 in ascending order, with a thickness ranging between 2,000 and 5,000 m Es3 is the most widely distributed and thickest, which is often further subdivided into Es3U, Es3M, and Es3L. It was deposited in semi-deep and deep lake depositional settings. The sedimentary period of Es3 in the northern Dongpu Depression can be divided into a saline water area, half-salt water area, and light saltwater area. The upper sub-member of Es3 in the saline water area is mainly interbedded with mudstone and sand mudstone, with a thick sandstone layer, limestone, and dolomite at the bottom. The middle sub-member of Es3 is mainly interbedded with mudstone and sand mudstone, with many developed salt beds throughout. The lower sub-member of Es3 is primarily interbedded with mudstone and sand mudstone. The upper sub-member of Es3 in the half-salt water area and the light saltwater area is mostly sand mudstone. The middle sub-member of Es3 is interbedded with siltstone and mudstone. The lower sub-member of Es3 consists of siltstone and mudstone.
3 Materials and methods
Seven exploration wells in the northern part of the Dongpu depression were selected, and samples were taken for different lithofacies shale in the third member of the Shahejie formation. A total of 32 cores were obtained consisting of black, brownish-gray, and gray shale and dark gray and brown salt shale. Geochemistry, scanning electron microscopy (SEM), X-ray diffraction, trace element, and inorganic carbon isotope analysis was performed on the core samples.
3.1 Rock-eval and TOC analysis
The soluble organic matter of a rock sample powder was extracted with the mixed solvent of chloroform and ethanol with a volume ratio of 98:2 (extracted continuously by the Soxhlet extractor for 72 h). After the asphaltene was precipitated by chloroform asphalt “A,” the extract was continuously eluted with n-hexane, toluene, and chloroform on a neutral alumina chromatographic column to separate saturated hydrocarbon, aromatic hydrocarbon, and non-hydrocarbon components, respectively.
3.2 X-ray diffraction
XRD was conducted with a D8 ADVANCE X-ray diffractometer (Bruker, Germany) under the conditions of a Cu-Kα radiation X-ray tube at a voltage of 40 kV and an electric current of 30 mA. A comparative analysis was carried out based on the standard powder diffraction data provided by the International Centre for Diffraction Data (ICDD) and according to its standard analysis methods and diffraction criteria (consistent interplanar spacing and diffraction intensity). About 50–100 g of samples were taken at each sampling site and were crushed and divided into samples of about 5 g each. The collected samples were ground in a grinding bowl to a particle size of 48p.m.
3.3 SEM
The sample surface was scanned using a Fei quanta 200f field emission scanning electron microscope. The working voltage was 10–15 kV at a working distance of 8–10 mm. The energy spectrometer consisted of EDAX ternary integrated system to enlarge and image the sample surface.
3.4 Trace element analysis
Dry and clean samples (<200 mesh) were used for trace element determination. First, the dried samples were placed into a 700°C high-temperature furnace for 3 h to remove all organic matter. Approximately 45 mg of a sample was placed into a dry and Teflon bottle, and a mixed solution of HNO3, HF and HClO4 was added to dissolve the sample. The dissolved and diluted samples were measured by PE Elan 6,000 inductively coupled plasma mass spectrometer (ICP-MS) for trace elements.
3.5 Inorganic carbon isotope analysis
The determination of carbon and oxygen isotopic composition was completed on a MAT-252 stable isotope mass spectrometer from the Finnigan Mat Company. The standard was carbon dioxide (PDB) made from American Archery carbonate fossils from the Cretaceous Pidi Formation, South Carolina, with allowable errors of less than ±0.5% respectively 2‰ and ±0.5% 3‰.
4 Development characteristics of mud shales
4.1 Distribution characteristics of mud shales
In the northern Dongpu Depression, the thickness of the Es3 middle sub-member shales is the greatest, with a maximum thickness of about 800 m. The shales are mainly distributed in the Liutun, Haitongji, and Qianliyuan Depressions. The thickness of the Es3 lower sub-member has a maximum thickness of about 700 m, mainly distributed in the northern Haitongji and Qianliyuan Depression. The thickest dark mudstone of the Es3 upper sub-member is about 500 m, of which Haitongji and Liutun Depression are the thickest, and the thickness of Qianliyuan Depression is only 300 m. There are five primary sets of gypsum salt beds developed in the northern Dongpu Depression, including saltⅠof Es1, salt Ⅰ of the upper sub-member of Es3, salt Ⅱ, and Ⅲ of the middle sub-member of Es3, and salt Ⅳ of the lower sub-member of Es3. In addition to the limited distribution of saltⅠof Es3, the other four sets of gypsum-salt layers are widely distributed. Wenliu and Weicheng are the thickness centers, and the accumulated thickness of the gypsum-salt layers can reach 950 m.
The lithology of salt rock and mud shale assemblage of the Es3 in the northern Dongpu Depression primarily consists of in ascending order sand mudstone, gypsum mudstone, gypsum salt rock, salt rock, gypsum salt rock, gypsum mudstone, and sand mud. They are mainly distributed in the lower sub-member and the middle sub-member of Es3, and the upper sub-member of Es3 is limited (Figure 2). The lithological changes can be divided into the gypsum salt rock, transition zone of gypsum salt rock and sand mud shale (Liu et al., 2014), and sand mudstone, reflecting the cyclical change of ancient water salinity from freshwater to light saltwater, saltwater, light saltwater, and finally back to freshwater. The depositional lake basin has always been a balanced system of freshwater injection and evaporation. When evaporation exceeded the freshwater injection, the water body was concentrated and salinized. Furthermore, carbonate-sulfate-chlorate and other evaporites were deposited with increased salinity. Carbonate-sulfate-evaporite assemblages are mainly distributed in Es2 and Es1, while Es3 does not contain evaporites.
4.2 Geochemical characteristics
4.2.1 Organic matter abundance
The organic matter abundance of the mud shales in the northern Dongpu Depression is greater than that in the southern salt-free area. The TOC content of more than 20% of samples is greater than 1.0%, and 6.6% of the samples are greater than 2.0% in the northern depression. Nearly 70% of samples have a TOC content of less than 0.4%, and only 10% of the samples are greater than 1.0% in the southern salt-free area. In the lower and middle sub-member of Es3, the organic matter abundance of the source rocks is high and is classified as “good” source rocks (Liu et al., 2020). However, the organic matter abundance of the shale in the undeveloped area of the Es3 upper sub-member is relatively low, which is classified as a “poor” source rock (Table 1).
The TOC content of the shales in the saline area of the northern depression is closely related to the gypsum salt rock layer. The organic matter abundance of the gypsum mudstone and saline mudstone in the transition zone of the gypsum mudstone-sand mudstone is the greatest, followed by inter-salt mudstone, supra-salt, or sub-salt mudstone in the transition zone of gypsum mudstone-sand mudstone, or the gypsum salt developed zone. The organic matter abundance of the mudstone or silty shale in the sand mudstone developed zone is lower. The distance between the mud shales and the gypsum salt rocks has an apparent negative correlation with the shales’ organic matter abundance. The closer the mud shale is to the gypsum salt rock, the higher the organic matter abundance of mud shale.
The organic carbon content also increases in the areas with the high chloride content, namely the area with the high paleosalinity. Ancient salinity controls the abundance of organic matter, with increased salinity corresponding to increase organic matter abundance. The distance between shale and gypsum salt rock has an obvious negative correlation with shale organic matter abundance; that is, the closer the shale is to gypsum salt rock, the higher the shale organic matter abundance. When the distance between shale and gypsum salt rock exceeds 40 m, the influence of gypsum salt rock on shale organic matter abundance is significantly weakened. Three TOC-rich intervals are well developed (Lu et al., 2021), and the most important TOC-rich strata are located in the middle sub-member of Es3, below the Wen nine Salt and above the Wen 23 Salt. The maximum TOC content can reach 8% (Figure 3).
Shale samples from different environments were selected for analysis finding the higher TOC content of the shale samples in saline lake facies (Wei 79–13) and transition facies (Well Pu 6–33). The TOC contents of the deep lake muds (Pu 148) and silty mud shales (Well Wei 79) were lower. The TOC contents of the mud shale samples from Well Wei 79–13 were 0.12–3.14%, with an average of 1.06%. Well Pu 6–33 had TOC contents ranging from 0.2 to 2.72%, with an average of 0.92%. Well Pu 148 ranged from 0.15 to 0.87%, with an average of 0.46. Well Wei 79 ranged from 0.17 to 0.54%, with an average of 0.29% (Figure 4).
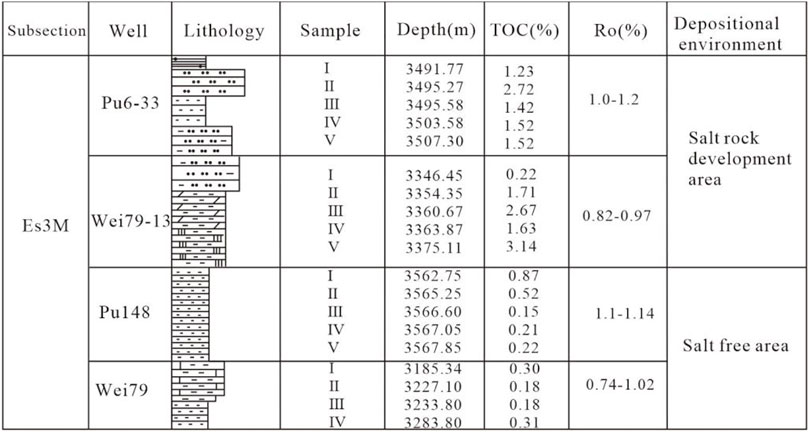
FIGURE 4. Geochemical characteristics of shales with different sedimentary environments in the northern Dongpu Depression.
4.2.2 Organic matter types
The sources of organic matter in shales from different zones of the Dongpu Depression varied widely due to different depositional environments. Cyanobacteria, coccolithophores, and dinoflagellates lived in the saltwater environment, while dinoflagellates were the most abundant in the half saltwater environment. The systems had abundant organic matter, providing adequate potential for hydrocarbon generation. The freshwater environment was dominated by higher plant inputs, mainly green algae. The shales in the northern Dongpu Depression were mainly formed in the saltwater and half saltwater environment, and the kerogen types are generally preferred. TypesⅠand type Ⅱ1 are dominant, accounting for about 40–80% of the total shale samples. TypeⅡ2 is second, accounting for about 10–35% of the total shales. Type Ⅲ is the least, generally less than 20% (Table 2).
According to the Pr/nC17-Ph/nC18 relationship diagram (Figure 5), the sedimentary environment in the northern Dongpu Depression is classified as a reducing environment consisting primarily of type I kerogen. High-quality source rocks in saltwater and half saltwater environments are more conducive to generating liquid hydrocarbons in the mature stage. Furthermore, the Paleogene source rocks in the Dongpu Depression are mainly mature-highly mature, and the most high-quality source rocks are found throughout the northern Dongpu Depression. These factors allow for more abundant oil and gas resources in the northern Dongpu Depression (Liu et al., 2020; Lu et al., 2021).
4.2.3 Biomarker compounds
The regular sterane mass spectrum among the mud shale steranes in the northern salt-bearing area usually presents as an “L" or asymmetric “V" shape, with the characteristics of C27 > C29. The 4-Methyl sterane’s mass fraction is relatively high, with 4-Methyl sterane/C29 greater than 0.4, indicating the lower aquatic algae biogenesis’s higher contribution under high salinity and strong reduction environment (Figures 6A,B). The mass fraction of γ-cerane and 4-methyl sterane is relatively high, indicating half salt water-light saltwater shales, with γ-cerane/C 30>0.5 and 4-methyl sterane/C 29>0.4. The dominance of C27 in regular steranes is weakened and presents a “V" shaped distribution, indicating the mixed sources are dominated by low-grade aquatic organisms and supplemented by high-grade terrestrial plants in a reducing environment with medium salinity (Figures 6C,D).
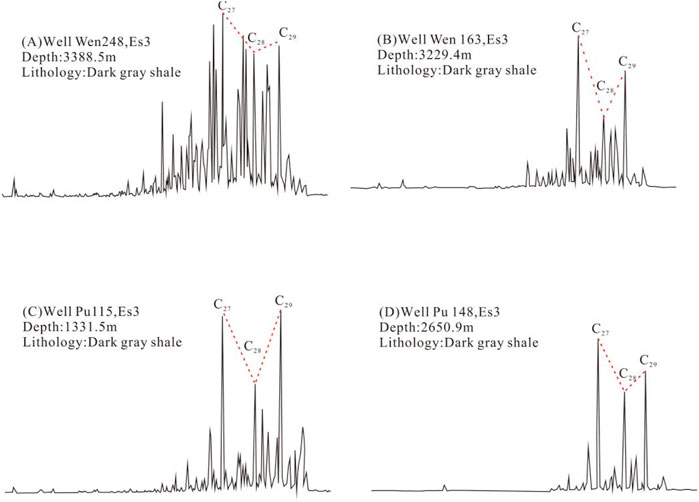
FIGURE 6. Typical biomarker combinations of source rocks in different zones of the Dongpu Depression.
4.3 Organic matter maturity
Geothermal temperature is the most effective and lasting factor in thermal evolution. Gypsum salt rocks have high thermal conductivity. If there are gypsum salt rocks in the formation, deep subsurface temperatures under the salt layer are easily transferred to the formation above the salt layer, as the salt layer can quickly transfer the heat. As a result, the formation temperature adjacent to the upper salt layer is higher than the salt-free layer at the same depth. The abnormally high temperature above the salt promotes source rock thermal evolution, while the abnormally low temperature under the salt hinders source rock thermal evolution and delays organic matter maturation (Chen et al., 2018). Overall, salt bodies significantly increase the range of hydrocarbon generation windows across a region. Therefore, the hydrocarbon generation peak in salt-bearing regions and over-maturity threshold depth is deeper than that of an underdeveloped gypsum-salt rock area (Figure 7).
4.4 Carbon isotope characteristics
The carbon isotope characteristics of natural gas can effectively reflect the characteristics of natural gas genesis, source, and maturity. It is an effective gas source correlation method and identifies the genesis. Natural gas in the study areaδ13C1 values range between −41.9 and −29.0‰,δ13C2 values range between-29.4∼−23.1‰, δ13C3 values range between −28.3 and −21.3‰ (Table 3).
According to the identification chart of hydrocarbon isotope origin of natural gas, the natural gas of the Es3 member in the northern Dongpu Depression is classified as coal-derived gas and mixed gas. Natural gas is mainly sourced from coals formed by humic organic matter. However, some of the natural gas in this area has mixed sources. The carbon isotope composition has a positive sequence distribution, indicating typical organic genetic characteristics. Overall, the local carbon isotope inversion of natural gas may be caused by non-homologous or mixed sources from different periods of homology (Figure 8). From the source of parent material, the organic matter type of the Carboniferous Permian coal seam and carbonaceous mudstone is type III kerogen. The carbon isotope value of ethane from the region is −26∼−24‰, and it is generally difficult to reach values greater than -20‰. Only based on the reaction, the ethane is “heavy”, as indicated by the carbon isotopes. The early reservoir forming natural gas in the northern Dongpu depression comes from the hydrocarbon generation of Carboniferous Permian coal measure source rocks in the over mature stage. It is a highly mature region, with the natural gas more enriched in heavy carbon isotopes.
Near the depth of 3,500 m, the Ro of Es3 shale is 1%–1.3%. The oil-type in the northern Dongpu depression comes from lacustrine shale. Mixed natural gas: firstly, lacustrine mudstone and shale are common in Es3 as high-quality source rocks; secondly, regional salt beds were developed in ES2, which effectively prevents a large amount of vertical migration of hydrocarbons and ensures that Es3 can store gas generated by source rocks in this layer; at the same time, highly mature coal-based gas from Carboniferous Permian system in the lower part migrates upward along the fault and forms a reservoir mixed with oil-based gas in this layer. The mixed gas reservoirs are widely distributed in the study area.
5 Lithofacies characteristics
5.1 Mineral composition
The Es3 shale is mainly comprised of clay minerals, quartz, plagioclase, dolomite, and pyrite. The content of clay minerals ranges from 31 to 71%, quartz 11.2–57.9%, plagioclase 3.3–22.1%, dolomite 1.8–19.9%, and pyrite 1–4.1%. Clay minerals include illite, illite/smectite mixed layer, kaolinite, and chlorite. The content of the illite/smectite mixed layer is greater, and the clay mineral percentage and types vary with diagenesis. Illite increases from the upper sub-member to the lower sub-member of Es3, while illite/smectite mixed-layer decreases.
5.2 Lithofacies
The shale layers can be divided into three typical facies: laminated limestone shale facies, massive silty shale facies, and laminated gypsum mudstone facies. Laminated limestone shale-facies rocks are mainly composed of argillaceous, limestone, and clastic particles. Microscopic stratification is developed in the rocks, and argillaceous and limestone are interbedded. Light green fluorescence occurs in bedding cracks, and yellow-green fluorescence occurs in fractures (Figures 9A,D). The massive silty shale facies are mainly composed of argillaceous and clastic particles with a massive structure and medium luminous intensity (Figures 9B,E). Laminated gypsum mudstone facies are located in the salt-bearing environment, mainly composed of gypsum, and grey micrite. Micro-laminated and micro-cracks are developed throughout the samples, and the fractures are mostly filled by gypsum. The rock does not fluoresce as a whole, but parts do fluoresce yellow-green, with extremely dark luminous intensity (Figures 9C,F). The laminated limestone shale facies appear to have a better oil content, followed by the silty shale facies and the laminated gypsum mudstone facies.
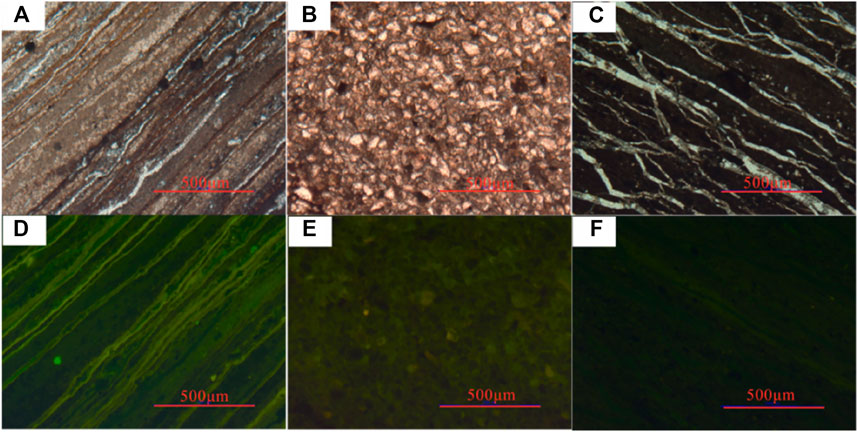
FIGURE 9. Microscopic characteristics of different shale facies of Shahejie Formation in the northern Dongpu Depression.
By analyzing the hydrocarbon generation potential of three typical lithofacies, the limestone shales have higher TOC content (0.15–5.72%, with an average of 1.512%), greater oil production potential (0.05–16.67 mg/g, with an average of 3.978 mg), and higher core measured oil content (0.007–1.667%, with an average of 0.398%) than the silty mudstone. The limestone shales are the dominant lithofacies of the developed shale oils and gases (Table 4).
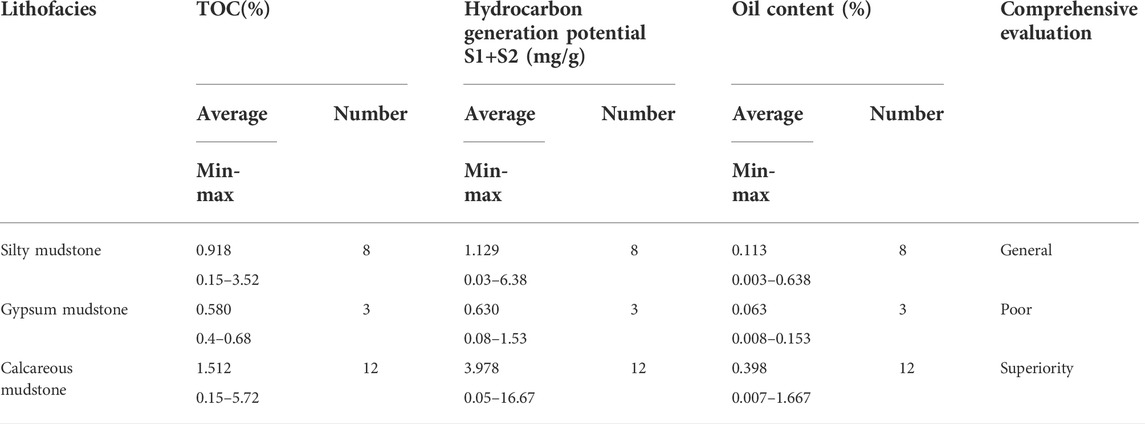
TABLE 4. Comprehensive evaluation of hydrocarbon generation potential of different lithofacies types.
5.3 Formation mode
The lithofacies types and characteristics are directly related to the sedimentary environment, and its formation is related to the bottom water oxygen content, organic matter supply, and the chemical properties of the lake, changing with environmental temperatures. The climate of Es3 in the northern Dongpu Depression was dry, with high salinity and halocline (Ma et al., 2019). Under sunlight catalysis, the autotrophic organisms in the eutrophic surface water develop a large amount of organic matter. The organic matter can be used as food for aquatic animals to provide specific nutrients for algae growth to promote breeding. However, excessive organic matter can easily develop. When granular and dissolved organic matters exist, it can severely affect solar radiation and weaken photosynthesis. After the death of numerous organisms, the degradation process consumed a large amount of oxygen in the water. If the dissolved oxygen in the water is insufficient, it causes an aerobic environment, which reduces poison accumulation and produces CO, H2S, NH3, and other harmful substances. The water quality deteriorates, affecting the organic growth and even causing extensive mortality. Organic-rich laminae are deposited, resulting in the sedimentary hiatus of organic matter and clay mineral or carbonate deposition. The growth environment of algal blooms forms again with improved oxygen conditions. Over and over, organic-rich laminated shales were formed, interbedded with organic-poor shales, siltstones and carbonates [Figure 10].
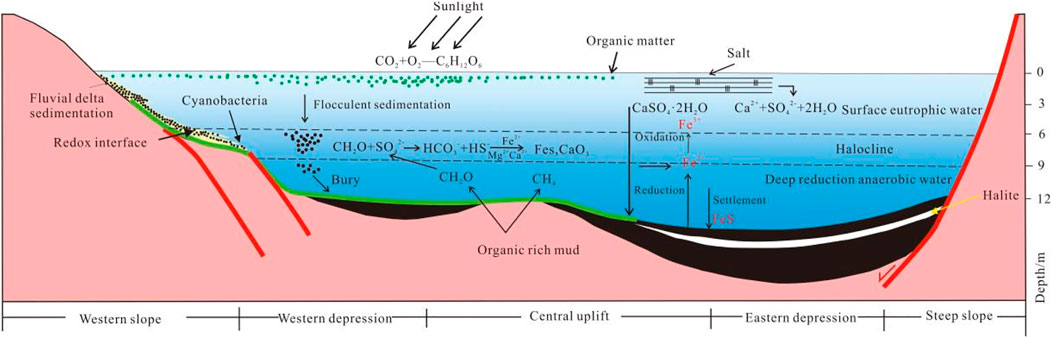
FIGURE 10. Sedimentary environment and redox model of salt-bearing of Es3 of Shahejie Formation of Paleogene in the northern Dongpu Depression.
6 Pore characteristics
The micro-reservoir space of Es3 in the Dongpu Depression is composed of inorganic pores, organic pores, and micro-cracks. Overall, the pore space can be divided into organic and inorganic pores. The porosity of the Es3 shale in the northern Dongpu Depression ranges from 1.1 to 9.3%, with an average of 6.6%. The permeability is mostly 0.0078×10−3∼0.159×10−3 μm2, with an average of 0.827×10−3 μm2. The average pore diameter is 3.404–8.40 nm, with an average of 11.29 nm, and is generally dominated by mesopores. Inorganic pores are the primary pore type, which can be divided into mineral intergranular micropores (Figure 11A), dissolution pores (Figure 11B), and clay mineral interlayer cracks (Figure 11C). Organic pores are generally not developed throughout the shale (Figure 11D). Pores and cracks are generally unfilled (Figure 11E) or partially filled (Figure 11F), consisting of calcite and pyrite. Organic matter mainly covers the clay minerals surface in free and absorbed states or is coated and adhered with carbonate and pyrite in the form of lumpy organic matter.
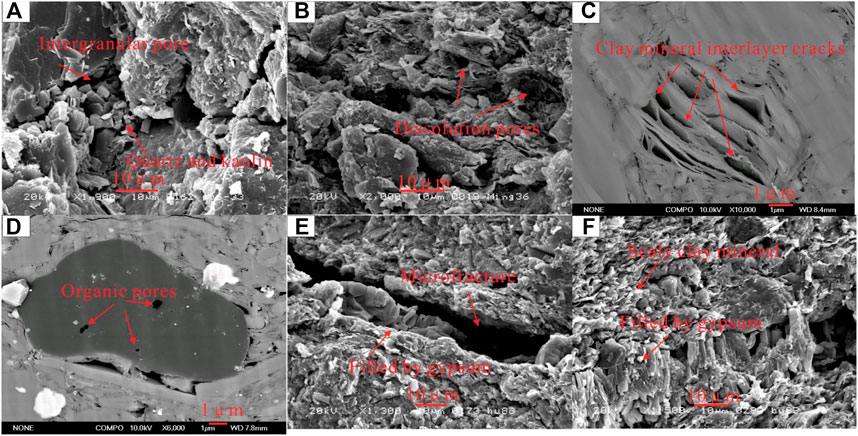
FIGURE 11. Pore SEM photography of Es3 of Shahejie Formation of Paleogene in the northern Dongpu Depression.
The porosity of the lower reservoir in the gypsum-salt layer is 2–10% greater than that of the normal compacted layer in the gypsum-salt rock underdeveloped area. The greater porosity of the lower reservoir is influenced by three characteristics. 1) The gypsum-salt layer is relatively dense, and there is obvious under-compaction in the lower strata, so the lower strata maintain a higher porosity. 2) The gypsum-salt rock’s high thermal conductivity causes the lower strata’s heat to be quickly released, and the diagenetic evolution is inhibited, so the porosity of the gypsum-salt layer’s lower reservoir can be preserved. 3) The abnormally high pressure under the gypsum-salt layer partly exceeds the rocks’ fracture pressure, which is prone to cracks and increases the total porosity (Figure 12).
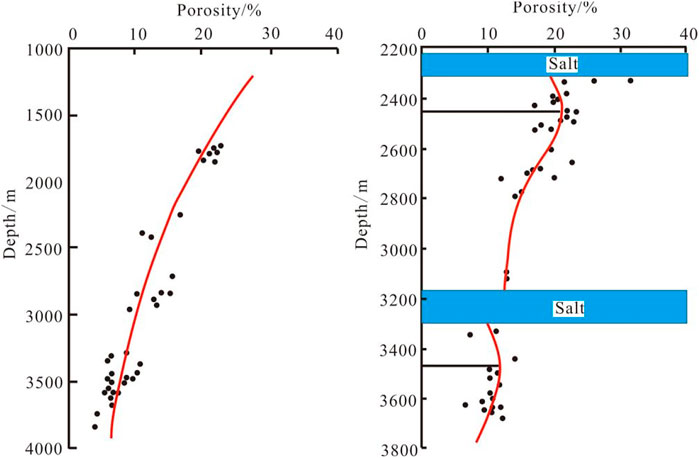
FIGURE 12. Porosity vs. depth diagram for reservoirs in different environments of Dongpu Depression.
Diagenesis also enhances porosity due to dissolution. The comparison of vitrinite reflectance between the salt-bearing and salt-free areas of the diagenetic stage in the study area shows that the primary and secondary dissolution zones mainly range from 2,500 to 2,600 m to 3,200–4,000 m, and the abnormal pore zone and calcite content correlate. The result indicates that the dissolution materials are mostly calcite, and both primary and secondary dissolutions positively affect porosity and permeability.
7 Conclusion
1) Es3 was a period of rapid subsidence, with “large basin and deep water.” The upper sub-member of Es3 was mainly composed of clastic rocks and evaporite rocks, and the lithology from bottom to top was mainly characterized by sand mudstone, gypsum mudstone, gypsum salt rock, salt rock, gypsum salt rock, gypsum mudstone, and sand mudstone, reflecting the cyclical change of ancient water salinity between freshwater, light saltwater, saltwater, light saltwater, and back to freshwater. The mudstone facies in the saline lake consist of laminated gray shale facies, laminated shale facies, and laminated paste shale facies. The mudstone’s microscopic structure is mostly lamellar or laminated.
2) Gypsum-salt obviously influences hydrocarbon generation and evolution of the source rock. It was found that the oil generation window would be expanded if the thickness of gypsum salt was over 50 m. The mud shales in the saline lake area of northern Dongpu Depression have high TOC content sourced from lower aquatic organisms in a strongly reducing environment. Organic matters are classified as typeⅠandⅡ kerogen. The salt rock has good thermal conductivity, and the hydrocarbon generation peak of the mud shales in the salt-bearing area and over-maturity threshold depth is deeper than that of the underdeveloped gypsum salt rock area. The mud shales’ micro-reservoir space is mainly inorganic pores, organic pores, and micro-fracture, and the pores are divided into organic pores and inorganic pores. Inorganic pores are the primary type of pores, divided into mineral intergranular micropores, dissolution pores, and clay-mineral interlayer cracks. The pores are mostly unfilled or semi-filled, and organic pores are generally not developed throughout the shale reservoir. The abnormal pore zone and the calcite content are highly correlated, indicating that the calcite was the main dissolution material, and both primary and secondary dissolution positively affected porosity and permeability.
3) The origin of natural gas in the northern Dongpu depression is complex. There are not only typical coal-derived gas but also oil-type gas and mixed gas in the formation of Es3 member; The gas source comparison shows that the shale of Es3 and the coal of Carboniferous Permian are jointly supplied, and the oil-type gas comes from Es3 of lacustrine sedimentary shale; The mixed gas is jointly supplied by the shale of Es3 and the coal of Carboniferous Permian; The coal of the Carboniferous Permian strata in the study area migrated upward along the faults developed in the area to Es3 of Shahejie formation, and formed a coal-derived gas under the cover of the overlying salt rock.
Data availability statement
The original contributions presented in the study are included in the article/supplementary material, further inquiries can be directed to the corresponding author.
Author contributions
All authors listed have made a substantial, direct, and intellectual contribution to the work and approved it for publication.
Funding
This research was jointly supported by the Open fund of provincial and ministerial Key Laboratory of China University of Geosciences (Beijing) (20210113), Guizhou Education Department Youth Science and Technology Talents Growth Project [grant numbers QJH No. KY (2020)122], Fund Program of the Science and Technology Department ofLiupanshui City (52020-2018-03-03).
Conflict of interest
ED was employed by Guizhou Panjiang Coal Power Group Technology Research Institute Co. Ltd.
The remaining authors declare that the research was conducted in the absence of any commercial or financial relationships that could be construed as a potential conflict of interest.
Publisher’s note
All claims expressed in this article are solely those of the authors and do not necessarily represent those of their affiliated organizations, or those of the publisher, the editors and the reviewers. Any product that may be evaluated in this article, or claim that may be made by its manufacturer, is not guaranteed or endorsed by the publisher.
References
Chen, X. F., Li, S. M., Zhang, H. G., Xu, T. W., Zhang, Y. X., Wan, Z. H., et al. (2018). Controlling effects of gypsum-salt on hydrocarbon generation of source rocks in Dongpu Sag and its significance on petroleum heology. Geoscience 32, 1125–1136. doi:10.19657/j.geoscience.1000-8527.2018.06.02
Chen, Y. C., Yang, Z. M., Wang, S. H., Zheng, H. Q., Tong, X. J., Hu, Y., et al. (2014). Discussion on the lower limit of organic abundance of effective hydrocarbon expulsion for the source rock from Majiagou Formation in Ordos Basin:Taking the well Longtan 1 as an example. Nat. Gas. Geosci. 25, 1718–1726. doi:10.11764/j.issn.1672-1926.2014.11.1718
Feng, J. L., Cao, J., Hu, K., Peng, X. Q., Chen, Y., Wang, Y. F., et al. (2014). Dissolution and its impacts on reservoir formation in moderately to deeply buried strata of mixed siliciclastic-carbonate sediments, northwestern Qaidam Basin, northwest China. Mar. Petroleum Geol. 39, 124–137. doi:10.1016/j.marpetgeo.2012.09.002
Gao, H. C., Zheng, R. C., Xiao, Y. K., Meng, F. W., Chen, F. L., Bai, G., et al. (2015). Origin of the salt rock of palcogene shahejic Formation in Dongpu sag, Bohai Bay basin, evidences from sedimentology and geochemistry. Acta Pet. Sin. 36, 19–32. doi:10.7623/syx6201501003
Guo, T. L., and Zhang, H. R. (2014). Formation and enrichment mode of Jiaoshiba shale gas field, Sichuan Basin. Petroleum Explor. Dev. 41, 28–36. doi:10.11698/PED.2014.01.03
Guo, T. L. (2016). Key geological issues and main controls on accumulation and enrichment of Chinese shale gas. Petroleum Explor. Dev. 43, 349–359. doi:10.1016/s1876-3804(16)30042-8
Harry, H., and Carney, R. S. (1997). Evidence of episodic fluid, gas, and sediment venting on the northern gulf of Mexico continental slope. Econ. Geol. 92, 863–879. doi:10.2113/gsecongeo.92.7-8.863
He, Z. L., Nie, H. K., and Zhang, Y. Y. (2016). The main factors of shale gas enrichment of Ordovician Wufeng Formation-Silurian Longmaxi Formation in the Sichuan Basin and its adjacent areas. Earth Sci. Front. 23, 8–17. doi:10.13745/j.esf.2016.02.002
Leng, J. G., Liu, X. F., Pang, X. Q., and Song, D. Y. (2006). Over pressured fractured mudstone reservoir in saltmud interbed:A case from Wenliu structure in Dongpu Sag. Petroleum Explor. Dev. 33, 692–696. doi:10.1177/1090820X12448817
Li, J., Zhou, S. X., Fu, D. L., Chen, K. F., Zhang, C., Sun, Z., et al. (2022). Quantitative gas-in-place comparison of original and bitumen-free lacustrine shale. Acta Geol. Sin. Engl. Ed. 96, 559–570. doi:10.1111/1755-6724.14680
Li, S. J., Sun, D. S., Zheng, M. L., and Meng, X. W. (2014). Salt-related structure and its control on hydrocarbon of the cambrian in sichuan basin. Oil Gas Geol. 35, 622–631. doi:10.11743/ogg20140506
Liu, J. D., Jiang, Y. L., Tan, Y. M., and Mu, X. S. (2014). Relationship between gypsum-salt rock and oil-gas in Dongpu depression of Bohai gulf basin. Acta Sedimentol. Sin. 32, 126–137. doi:10.14027/j.cnki.cjxb.2014.01.015
Liu, X. W., Wang, X. J., LiZhang, H. L. Y. X., Zhang, W. B., and Huang, Z. (2021). Characteristics and formation environment analysis of Paleogene source rocks in Dongpu Depression. Fault-Block Oil Gas Field 28, 452474–453455. doi:10.6056/dkyqt202104004
Liu, X. Y., Chen, H. H., Xiao, X. W., Zhang, H. G., and Xu, T. W. (2020). Mixing characteristics of oil inclusions with different thermal maturities in the wenliu uplift, Dongpu depression, Bohai Bay basin, north China. J. Earth Sci. 31, 1251–1258. doi:10.1007/s12583-020-1356-0
Lu, K., Li, C. C., Zhou, F., Zhang, C. F., and Xu, T. W. (2021). Mechanism of high efficient reservoir formation of low abundance source rocks in the north of Dongpu Depression. Fault-Block Oil Gas Field 28, 470–474. doi:10.6056/dkyqt202104007
Lu, S. F., Ma, Y. L., Cao, R. C., Li, J. J., Wang, W. M., Li, H. G., et al. (2012). Evaluation criteria of higlr quality source rocks and its applications: Taking the wuerxun sag in hailaer basin as an example. Earth Science-Journal China Univ. Geosciences 37, 535–544. doi:10.3799/dqkx.2012.060
Ma, X. X., Yao, S. P., Zang, B. L., Zhang, Y. X., and Peng, J. (2019). Redox conditions of paleogene paleolake and development models of high - quality source rocks in the Dongpu Sag, Bohai Bay Basin. Geol. J. China Univ. 25, 801–812. doi:10.16108/j.issn1006-7493.2019081
Manzi, V., Lugli, S., Lucchi, F. R., and Roveri, M. (2005). Deep-water clastic evaporites deposition in the messinian adriatic foredeep (northern apennines, Italy): Did the mediterranean ever dry out? Sedimentology 52, 875–902. doi:10.1111/j.1365-3091.2005.00722.x
Murray, J. W. (1991). Ecology and palaeoecology of benthic foraminifera. Holocene 2, 185–186. doi:10.4324/9781315846101
Sarg, J. F. (2001). The sequence stratigraphy, sedimentology, and economic importance of evaporite–carbonate transitions: A review. Sediment. Geol. 140, 9–34. doi:10.1016/S0037-0738(00)00170-6
Schmoker, J. W. (2002). Resource-assessment perspectives for unconventional gas systems. Am. Assoc. Pet. Geol. Bull. 86, 1993–1999. doi:10.1306/61EEDDDC-173E-11D7-8645000102C1865D
Tan, Y. M., Cheng, X. S., Chen, S. P., and He, F. (2011). Complex fault block groups in Dongpu Sag and their exploration potential. Oil &Gas Geol. 32, 584–592. doi:10.11743/ogg20110412
Tang, L., Pang, X. Q., Xu, T. W., Hu, T., Pan, Z. H., Guo, K. Z., et al. (2017). Hydrocarbon generation thresholds of Paleogene Shahejie Fm source rocks and their north–south differences in the Dongpu Sag, Bohai Bay Basin. Nat. Gas. Ind. 37, 26–37. doi:10.3787/j.issn.1000-0976.2017.02.004
Tang, S. H., Fan, E. P., and Zhang, S. H. (2016). Reservoir characteristics and gas-bearing capacity of the Lower Palaeozoic marine shales in Northwestern Hunan. Earth Sci. Front. 23, 135–146. doi:10.13745/j.esf.2016.02.014
Tian, Z. B., Wei, S. H., Wang, J. Q., Li, L. Y., Tang, S. H., and Li, J. (2017). Characteristics of micro-scale pore structures of marine-continental transitional shale from the mid-eastern area, Qinshui Basin. J. China Coal Soc. 42, 1818–1827. doi:10.13225/j.cnki.jccs.2016.1430
Volozh, Y., Talbot, C., and Ismail-Zadeh, A. (2003). Salt structures and hydrocarbons in the pricaspian basin. Am. Assoc. Pet. Geol. Bull. 87, 313–334. doi:10.1306/09060200896
Wang, J. B., Gao, Z. Q., Kang, Z. H., Yang, Y.-X., Wei, D., and Qin, N. T. (2017). The sedimentary environment and geochemical characteristics of the source rocks in the Pusige Formation in Hetian Sag, southwestern Tarim Basin, China. Nat. Gas. Geosci. 28, 1723–1734. doi:10.11764/j.issn.1672-1926.2017.04.011
Wu, H., Zhao, M. J., Zhuo, Q. G., Lu, X. S., Gui, L. L., Li, W. Q., et al. (2016). Quantitative analysis of the effect of salt on geothermal temperature and source rock evolution:A case study of Kuqa foreland basin, Western China. Petroleum Explor. Dev. 43, 602–610. doi:10.1016/s1876-3804(16)30070-2
Zhang, J. C., Yang, C., Chen, Q., Zhao, Q. R., Wei, P. F., and Jiang, S. L. (2016). Deposition and distribution of potential shales in China. Earth Sci. Front. 23, 74–86. doi:10.13745/j.esf.2016.01.007
Zhang, L., Xiang, C. F., DongY, X., Zhang, M. Y., Lyu, Y., ZhaoZ, X., et al. (2018). Abnormal pressure system and its origin in the nanpu sag, Bohai Bay basin. Oil Gas Geol. 39, 664–675. doi:10.11743/ogg20180404
Zhang, Q., Fink, R., Krooss, B., Jalali, M., and Littke, R. (2021). Reduction of shale permeability by temperature-induced creep. SPE J. 26, 750–764. doi:10.2118/204467-PA
Zhang, Q., Grohmann, S., Xu, X., and Littke, R. (2020). Depositional environment and thermal maturity of the coal-bearing Longtan Shale in southwest Guizhou, China: Implications for shale gas resource potential. Int. J. Coal Geol. 231, 103607. doi:10.1016/j.coal.2020.103607
Zhou, Q. F. (2022). Discussion on key issues of shale oil/gas resource assessment. Oil Gas Geol. 43, 26–33. doi:10.11743/ogg20220102
Keywords: reservoir model, geochemistry, shale oils and gases, lithofacies characteristics, the northern
Citation: Zhang P, Deng E, Yang J, Huang Y, Tang X and Liu C (2022) Study on gas accumulation conditions of continental shale in Es3 member in the northern dongpu depression, eastern China. Front. Earth Sci. 10:977194. doi: 10.3389/feart.2022.977194
Received: 24 June 2022; Accepted: 02 August 2022;
Published: 24 August 2022.
Edited by:
Yu Song, China University of Geosciences Wuhan, ChinaCopyright © 2022 Zhang, Deng, Yang, Huang, Tang and Liu. This is an open-access article distributed under the terms of the Creative Commons Attribution License (CC BY). The use, distribution or reproduction in other forums is permitted, provided the original author(s) and the copyright owner(s) are credited and that the original publication in this journal is cited, in accordance with accepted academic practice. No use, distribution or reproduction is permitted which does not comply with these terms.
*Correspondence: Ende Deng, cGptZGp0ZGVkQGZveG1haWwuY29t