- 1Petroleum Engineering School, South West Petroleum University, Chengdu, China
- 2International Business Center, Sinopec Huadong Oilfield Service Corporation, Nanjing, China
- 3Changqing Oilfield Branch of Petrochina, Xi’an, China
- 4Shengli OilProduction Plant, Shengli Oilfield Company, SINOPEC, Dongying, China
- 5Gepetto Oil Technology Group Co Ltd., Chengdu, China
- 6Fracturing Company, Great Wall Drilling Corp, CNPC, Panjin, China
The beach-bar sand reservoir of the Sha 4 Member of the Shahejie Formation in the Dongying Sag is the main oil-bearing formation in this area. In recent years, its proven reserves have been getting lower and lower, and the poor petrophysical properties of the reservoir have made water injection development difficult. In turn, it results in a rapid decline in elastic development productivity and low oil recovery. In this study, the experimental evaluation and numerical simulation research on the adaptability of CO2 flooding in beach-bar sand reservoirs are carried out on the basis of fully investigating the successful examples of CO2 flooding conducted by the previous. According to the geological characteristics of the reservoir in the CL area of the Dongying Sag, the reasonable reservoir engineering parameters and surface injection procedures for the CO2 flooding have been formulated. Experiments show that after the completion of water flooding, the recovery factor of the CO2 continuous flooding is 85.64%. It proves that the recovery factor of the CO2 flooding is higher than that of the water flooding. Field tests have shown that CO2 molecules in beach-bar sand reservoirs behave in a supercritical state underground, which is easier to being injected into the reservoir than water. In addition, the displacement distance of the CO2 is obviously larger than that of the water injection development. The gas-oil ratio variation of different flooding types is different, and CO2 flooding can effectively increase the formation energy, and improve the oil recovery and economic benefits of this type of reservoir.
Introduction
The average recovery factor of water-flooding reservoirs is usually only 30.0%, and its remaining oil saturation is high, so the potential of tertiary oil recovery is great (Dicharry et al., 1973; Al-Abri et al., 2009; Al-Bayati et al., 2019; Gao and Pu., 2021; Li et al., 2021). CO2 miscible flooding belongs to tertiary oil recovery technology. By injecting CO2 into the reservoir, the interfacial tension, viscosity and fluidity of the crude oil can be improved, and the ultimate recovery of the crude oil is enhanced. Because CO2 miscible flooding has good oil-increasing effect and wide adaptability, it has become the main measure for tertiary oil recovery in different oilfields (Al-Abri and Amin, 2010; Hu et al., 2015; Yongle et al., 2019; Chen et al., 2020; Qin et al., 2021). In the past 20 years, laboratory and field experiments of CO2 miscible flooding have been carried out in the Daqing, Jilin, Zhongyuan and Huadong Oilfields in China. However, there are few reports on field trials of CO2 miscible flooding in ultra-high water-cut reservoirs (Stalkup., 1978; Desch et al., 1984; Akbarabadi and Piri., 2013; Wang et al., 2019; Jia et al., 2021; Huang et al., 2022).
The CO2 injection development of low permeability sandstone reservoirs is a reliable and effective method to enhance oil recovery. The main mechanisms include the reduction of interfacial tension, the reduction of crude oil viscosity, the swelling effect of crude oil, and the extraction of light hydrocarbons (Song et al., 2012; Chen et al., 2017; Zhang et al., 2018; Chen et al., 2019; Hou et al., 2020). Under miscible conditions, CO2 and crude oil can be mixed in any ratio, thereby eliminating interfacial tension and obtaining high CO2 flooding efficiency. However, CO2 immiscible flooding is likely to cause early CO2 breakthrough. Crude oil is considered a colloidal system, the dispersion medium is saturated and aromatic hydrocarbons, and the asphaltenes are in a dispersed phase (Paxton et al., 2002; Golkari and Riazi., 2017; Fakher and Imqam., 2020). When CO2 is injected into the reservoir, it will dissolve into the crude oil, and its extraction of the light components of the crude oil will lead to changes in the composition of the crude oil system. Furthermore, the asphaltene molecules in the crude oil cannot continue to be stably dispersed in the crude oil. At this point, the asphaltene molecules collide with each other and flocculate into aggregates that eventually precipitate out of the crude oil and then solid precipitates are formed. The size of these precipitated particles will gradually increase as the fluid migrates in the rock pores (Lashkarbolooki et al., 2016; Ding et al., 2019; Du et al., 2021; Liu et al., 2022). When the size of the asphaltene precipitation particles approaches or exceeds the size of the pore throats, the particles can become trapped and form blockages at the narrow throats, and the pore throat connectivity of the rock will be reduced. At the same time, asphaltene precipitation is also continuously adsorbed on the surface of rock minerals, resulting in a gradual decrease in the size of pores and throats. Asphaltene precipitation particles are much more likely to block pore throats. In addition, the adsorption of these asphaltene precipitates on the pore surface can also cause changes in rock wettability. Therefore, asphaltene precipitation can change the pore-throat structures of the rock by forming plugging and adsorption, thereby impairing the reservoir permeability and increasing the water cut and residual oil ratio (Lv et al., 2015; Wang et al., 2018; Hong et al., 2020; Hu et al., 2020).
Typical beach-bar sand reservoirs are developed in the Sha 4 Member of the Shahejie Formation in the CL area of the Dongying Sag, China. In recent years, the quality of the newly added proven reserves in the Sha 4 Member of the Shahejie Formation has been getting worse and worse. This is because the burial depth of the reservoir is relatively deep (between 2,700–3,500 m), and the permeability of the reservoir drops sharply from 10 to 0.3 mD. Due to the inability to achieve high-efficiency water injection development, oilfield engineers can only select some units with relatively good petrophysical properties to carry out large-scale fracturing (Kazemzadeh et al., 2015; Qiao et al., 2019; Xu and Gao., 2020; Yoshida and Santosh., 2020; Lan et al., 2021). From the perspective of development status, for low-permeability reservoirs developed only by natural energy, the average monthly decline rate of their productivity reaches 4%. Therefore, many newly discovered low-permeability reserves are poorly developed, resulting in a huge potential of remaining reserves in unproduced beach-bar sand reservoirs. For ultra-low permeability beach-bar sand reservoirs, water flooding development is greatly restricted (Li et al., 2020; Mahmud et al., 2020; Wang et al., 2020; Li., 2022; Wang et al., 2022). The development method that relies solely on elastic energy results in rapid decline in production and low recovery of crude oil. Therefore, it is necessary to find new energy supplement methods to improve the development level and production degree of low-permeability reservoirs.
In this study, the experimental evaluation and numerical simulation research on the adaptability of CO2 flooding in beach-bar sand reservoirs are carried out on the basis of fully investigating the successful examples of CO2 flooding conducted by the previous. According to the geological characteristics of the reservoir in the CL area of the Dongying Sag, the reasonable reservoir engineering parameters and surface injection procedures for CO2 flooding have been formulated. The research results have important reference value for formulating an efficient development plan for beach-bar sand reservoirs in similar areas.
Materials and methods
The target layer of this study is the beach-bar sand body in the upper part of the Sha 4 Member of the Shahejie Formation, which is widely distributed in the Dongying Sag. This set of sand bodies is located in the interlayer of the source rocks, and the thickness of the single layer is generally between 2 and 5 m, and it has the characteristics of large-area oil-bearing properties. The residual amount of original formation water in the sand body is extremely low, so the CO2 flooding technology is suitable for improving the oil recovery of this set of reservoirs.
Feasibility of CO2 flooding development
Usually, the waterflooding development effect of low-permeability and ultra-low- permeability beach-bar sand reservoirs is not ideal. When the petrophysical properties of the sandstone reservoirs are less than 3 mD, the development benefit of water flooding is quite poor, and the permeability of the Sha 4 Formation reservoir in the study area is between 0.3–10 mD, so it is not suitable for water flooding development. For the XX-1 Block with relatively good petrophysical properties of the target layer in the study area, the large-scale anti-swelling method is adopted, and the initial oil pressure via water injection is between 20–30 MPa. In a short period of time, the water injection pressure can be increased to more than 35 MPa, and the wellhead can meet the injection requirements after the booster pump is used. However, with the deepening of development, the excessive water injection pressure caused serious damage to the casing of the water injection well, and the reservoir began to suffer from under-injection and under-production in a large area (Mahmi et al., 2018; Wang et al., 2021; Li et al., 2022). Therefore, it is urgent to explore the development method of CO2 flooding for ultra-low permeability beach-bar sand reservoirs.
In this study, the reasons for the ultra-low permeability beach-bar sand reservoirs suitable for CO2 miscible flooding in the Sha 4 Member of the Shahejie Formation in the CL area are as follows:
(1) The research block is in the early stage of crude oil development, and the degree of crude oil recovery is high. Moreover, its crude oil basically does not contain water, which is conductive to the development of CO2 flooding technology.
(2) The connectivity of the beach-bar sand reservoirs in the study area is good, and the connectivity rate of the main sand bodies is 93%. At the same time, the beach-bar sand reservoir has no edge and interlayer water, which is conducive to reducing the corrosion of equipments, so it is suitable for CO2 injection.
(3) The main reservoir of the ultra-low permeability beach-bar sand reservoir in the Sha 4 Member of the Shahejie Formation in the study area is buried between 2,700–3,400 m, and its original formation pressure is 32–45 MPa. Its ground saturation pressure difference is large, so it is easy to form miscible phase after CO2 injection into the formation. Therefore, it is beneficial to carry out CO2 miscible flooding.
Design of minimum CO2 miscible pressure experiment
(1) Model parameters
The experimental model adopts a thin tube model with a length of 16 m and a diameter of 6.34 mm. The air permeability was maintained at <9.9 mD, the porosity was 32.25%, the experimental temperature was 126°C, and the displacement speed was 0.873 m/h.
(2) Criteria for determining the minimum miscible pressure for CO2 flooding
When 1.2 times the pore volume of CO2 was injected, there was a sudden change in the slope between the pressure curve and the displacement efficiency. Moreover, the final recovery factor is >95%, and the breakthrough value is later than 0.74 PV. In addition, a transition zone formed by immiscible gas and liquid phases can be seen in the observation port, which gradually diffuses to form a single phase after complete miscibility.
Design of long core flooding tests
The long core displacement test adopts a long core displacement device, which is mainly composed of a displacement system, a constant temperature device, a long core holder system, a back pressure controller and an oil measurement and gas measurement device. The maximum working pressure is 50 MPa and the temperature is 150°C.
The displacement tests of long cores were carried out under the conditions of simulated formation temperature of 125°C and pressure of 30 MPa. A total of three different long core flooding experiments were carried out. The experiments were used to evaluate the effect of injection timing and injection method on the enhanced oil recovery effect of miscible flooding.
A total of 3 sets of plans have been designed:
(a) After the water is flooded to 100% water content, CO2 is continuously injected until no oil is produced;
(b) First, continuous injection of CO2 large slug initially, when no oil is produced, it is transferred to water flooding;
(c) Initially, alternate injection of CO2 and water is carried out, and when no oil is produced, it is transferred to the subsequent water flooding.
Numerical simulation of CO2 flooding scheme
The numerical simulation in this study was completed using the PVT analysis results of laboratory experimental fluids and the CMG reservoir numerical simulation software. The construction of the equation of state for the CMG reservoir is based on the phase equilibrium software package Winprop. Furthermore, it is used for fluid characterization, compositional fitting, regression fitting of PVT experimental data, and simulation of multiple contact processes. It generates the data required by the component simulator GEM by establishing the equation of state of the crude oil in the target layer.
Results
Properties of CO2 and formation crude oil
(1) Variation of saturation pressure of formation crude oil
Indoor CO2 miscible flooding experiments show that the saturation pressure of formation crude oil will gradually increase after increasing the injection amount of CO2. Therefore, CO2 has strong mutual solubility in the formation crude oil in this block (Figure 1).
(2) Variation of volume expansion coefficient of formation crude oil
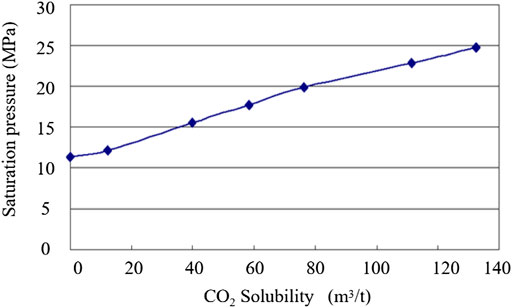
FIGURE 1. Relationship between CO2 solubility and saturation pressure of formation crude oil (125°C).
Through laboratory experiments, the relationship between CO2 solubility and formation oil volume expansion coefficient (Figure 2) was plotted. The expansion coefficient is basically positively correlated with the solubility of CO2, indicating that CO2 has a better expansion ability of the crude oil in the formation.
(2) Changes in the viscosity of formation crude oil
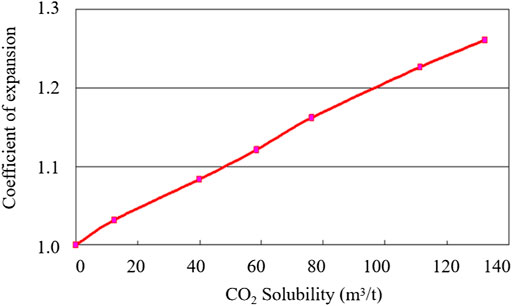
FIGURE 2. Relationship between CO2 solubility and volume expansion coefficient of formation crude oil (125°C).
In general, the greater the dissolved amount of CO2 contained in crude oil, the lower its viscosity (Paul et al., 1984; Santosh and Feng., 2020; Yin and Wu., 2020; Mirzaei-Paiaman and Ghanbarian., 2021). The relationship between the viscosity of crude oil and the solubility of CO2 in the study area shows that (Figure 3), the viscosity and solubility of crude oil are negatively correlated. Therefore, CO2 can improve the flowability of formation crude oil.
According to this study, the crude oil in the study area has a relatively strong dissolving ability to CO2, and the volume expansion and viscosity reduction of the crude oil are caused by the injection of CO2.
Experimental results of minimum CO2 miscible pressure
When the experimental pressure was gradually increased, the oil displacement effect of CO2 began to increase significantly. When the pressure was increased to 29.0 MPa, the oil displacement efficiency at 1.1 PV was over 97%, and it had reached complete miscibility (Figure 4). From the relationship between oil displacement efficiency and gas injection pressure at 1.1 PV, it can be concluded that the minimum miscible pressure in block XX is 28.94 MPa, and the corresponding oil displacement efficiency is 95.4% (Figure 5).
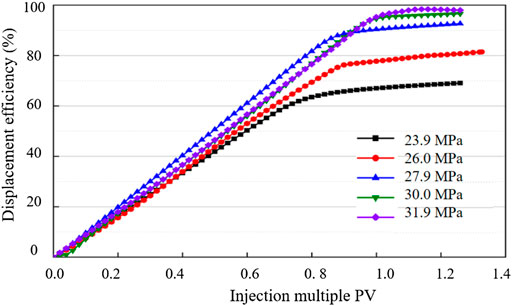
FIGURE 4. Relationship between oil displacement efficiency and injection multiple under different pressures.
Therefore, it is easier to form a miscible state when the minimum miscible pressure is weaker than the original formation pressure.
Experimental results of CO2 miscible oil displacement efficiency
The results of three long core flooding experiments show that (Figure 6), when the pressure and temperature are kept constant, the water flooding recovery factor is 33.50%; after the completion of water flooding, the recovery factor of the CO2 continuous flooding is 85.64%; after CO2 continuous flooding, the recovery factor when changed to water flooding is 79.58%; after the initial alternate injection of CO2 and water, the recovery factor when changed to water flooding is 81.56%. The test results fully demonstrate that the oil recovery factor of the CO2 flooding is much higher than1 that of water flooding. Considering the actual situation of the Block XX, the optimal injection method is continuous CO2 flooding.
Discussion
Parameter optimization of CO2 flooding scheme
For the simulation of the CO2 flooding scheme in this study, the basic conditions of the production well are set as: the minimum limit bottom-hole pressure: 12 MPa; the minimum limit oil production: 3 t/d; the limit gas-oil ratio: 2000 m3/m3. The basic conditions of the gas injection well are set as: the maximum injection pressure at the bottom of the well: 60 MPa.
(1) Optimization of pressure maintenance level
It can be clearly seen from the curve of recovery factor versus formation pressure (Figure 7) that when the miscible pressure is lower than 28.95 MPa, the pressure rises. And the recovery factor increases linearly and the growth rate is large, which is much higher than the recovery factor after miscible pressure. Therefore, the oil-reservoir pressure must be maintained above the miscible pressure (Trevisan et al., 2014; Qie et al., 2021; Wang and Wang., 2021).
(2) Optimization of CO2 injection amount
According to the relationship among the PV value of CO2 injection, the recovery factor and the daily oil production rate, it can be seen that the oil recovery factor increases with the increase of the injected PV value, and the daily oil production rate shows a slight downward trend. When the daily oil production rate dropped to 0.33, its changing trend began to stabilize, and the maximum value of the PV of injected CO2 was 0.33.
(3) Optimization of gas injection and oil recovery rates
The optimization simulation results of different gas injection and oil recovery rates show that the oil recovery efficiency is the best when the oil recovery rate is maintained at 2% and the gas injection rate is maintained at 20 t/d.
Field application and effect analysis of CO2 miscible flooding
In this study, we carried out the pilot experiment of CO2 flooding and enhanced recovery in the study area. The three-stage test development mode of single well experiment-well group expansion-block implementation was adopted. The application uses procedures implemented from individual wells to groups of wells to blocks.
The first stage: single-well test injection of CO2 in well A1.
Well A1 in the middle of Block XX is selected as the CO2 injection test well. The well spacing between this well and the surrounding 6 production wells is 350–700 m, the daily gas injection volume is 30–60 m3, and the gas injection pressure in the initial stage is low and maintained at 2–8 MPa. Except for the one well with the farthest distance of 700 m, which was not effective, the other five wells were all effective to varying degrees (Table 1).
The second and third stages: After the measure wells in the first stage are effective, the well group expansion will begin. 2 gas injection wells and 1 production well were newly drilled in Block XX, forming 4 central gas injection wells and 6 surrounding production wells. After that, the injection-production well pattern was gradually improved, and the overall development of the XX Block was realized.
(1) Gradual recovery of formation energy
The static pressure measured in Well A1 was 14.78 MPa at the time of transfer injection, and it changed to 25.628 MPa after 2 years, with an increase of 10.848 MPa and an average monthly increase of 0.638 MPa. For another gas injection well of Well A-16, the static pressure measured at the start-up stage was 26.03 MPa, and the static pressure measured after 2 years of gas injection was 28.3 MPa. It can be seen from the pressure tests of a single well that the formation pressure in the block is gradually recovered. According to the numerical simulation results, the formation pressure recovers faster near the bottom of the injection hole (Zekri et al., 2009; Zhang et al., 2020; Zhao et al., 2021).
In the design scheme, when the designed oil production rate of the central well group scheme is 2% and the injection-production ratio is 2.0, the time for the pressure to recover to miscibility is 1.25 years. At present, the oil production rate in the central well area is 1.76%, and the injection-production ratio is 1.23. The estimated time to start pressure recovery to miscibility at current production rates is 1.5 years. If the injection-production ratio is 2.0 according to the design, the pressure recovery time is 1 year (Figure 8).
(2) Analysis of oil-increasing effect
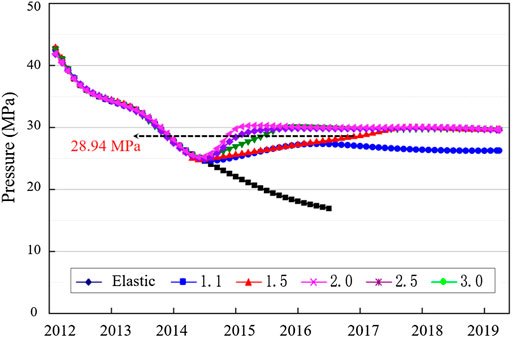
FIGURE 8. Variation law of designed pressure curves in different oil production rate schemes. The production methods include elastic development and development methods with oil recovery rates of 1.1–3.0% respectively.
Judging from the effective time of a single well of the gas injection well group in the Block XX, the effective time is 2–8 months, the average effective time is 4.4 months, and the effective time is positively correlated with the well spacing. In addition, the decline of gas injection well groups is 15% slower than that of elastic development well groups. According to the production status and monitoring results of the oil wells, the surrounding wells have seen very significant oil increases. According to the calculation results of the exponential decreasing method, the cumulative oil increase of the well group is 10,200 t. Comparing the effects of water injection and gas injection, the first-line well with gas injection effect has a stable daily production of 10 t, and the single well with water injection effect has a stable daily production of 3–5 t. After the gas injection took effect, the liquid volume increased by an average of 2 times; and after the water injection took effect, the liquid volume increased by 0.5 times.
(3) Analysis of economic benefit
Of the three development stages, the oil change ratio was 4.2:1 in the first stage, 5.9:1 in the second stage, and 3.8:1 in the third stage. Currently, the block has an overall oil change ratio of 4.9:1. The second stage is mainly due to the reduction of oil change rate due to the phenomenon of gas channeling. With the increase of CO2 injection amount, the formation energy gradually recovered and the oil change rate increased (Zhao et al., 2015; Xue et al., 2021; Yang et al., 2021).
Under the condition of production time of 6 years, the CO2 gas injection in the XX block is compared with the water injection in the adjacent block. Under the same conditions of single well investment, the cost per 1 m3 of water injection is 11 yuan, and the cost per 1 m3 of gas injection is 300 yuan. There are 7 water injection wells corresponding to the 7 production wells in the adjacent block, while there are 7 CO2 injection production wells in the XX Block, which corresponds to 4 gas injection wells. According to the current decreasing calculation of the block, the accumulated oil of water injection and gas injection after 6 years is 7.1736 × 104 t and 11.6916 × 104 t respectively, the income is 179.52 million yuan and 292.58 million yuan respectively, and the input-output ratio is 1:3.05 and 1: 2.95 respectively. It shows good economic benefits, and with the extension of time, the economic benefits of gas injection will be better than water injection.
Conclusion
(1) In this study, the experimental evaluation and numerical simulation research on the adaptability of CO2 flooding in beach-bar sand reservoirs are carried out on the basis of fully investigating the successful examples of CO2 flooding conducted by the previous. According to the geological characteristics of the reservoir in the CL area of the Dongying Sag, the reasonable reservoir engineering parameters and surface injection procedures for CO2 flooding have been formulated.
(2) Experiments show that after the completion of water flooding, the recovery factor of the CO2 continuous flooding is 85.64%. It proves that the recovery factor of the CO2 flooding is higher than that of water flooding. Field tests have shown that CO2 molecules in beach-bar sand reservoirs behave in a supercritical state underground, which is easier to being injected into the reservoir than water.
(3) The displacement distance of the CO2 is obviously larger than that of the water injection development. The gas-oil ratio variation of different flooding types is different, and CO2 flooding can effectively increase the formation energy, and improve the oil recovery and economic benefits of this type of reservoir.
Data availability statement
The original contributions presented in the study are included in the article/Supplementary Material, further inquiries can be directed to the corresponding author.
Author contributions
SY and QZ are responsible for the idea and writing of this paper and YX, ZH, TL, and XL are responsible for the data interpretation.
Funding
We gratefully acknowledge National Science and Technology of China (2016ZX05070) financial support.
Conflict of interest
Author SY was employed by the Sinopec Huadong Oilfield Service Corporation, author YX was employed by the Changqing Oilfield Branch, author ZH was employed by the Shengli Oilfield Company, author QZ was employed by the Gepetto Oil Technology Group Co. Ltd., and authors TL and XL were employed by the Great Wall Drilling Corp, CNPC.
The remaining authors declare that the research was conducted in the absence of any commercial or financial relationships that could be construed as a potential conflict of interest.
Publisher’s note
All claims expressed in this article are solely those of the authors and do not necessarily represent those of their affiliated organizations, or those of the publisher, the editors and the reviewers. Any product that may be evaluated in this article, or claim that may be made by its manufacturer, is not guaranteed or endorsed by the publisher.
References
Akbarabadi, M., and Piri, M. (2013). Relative permeability hysteresis and capillary trapping characteristics of supercritical CO2/brine systems: An experimental study at reservoir conditions. Adv. Water Resour. 52, 190–206. doi:10.1016/j.advwatres.2012.06.014
Al-Abri, A., and Amin, R. (2010). Phase behaviour, fluid properties and recovery efficiency of immiscible and miscible condensate displacements by SCCO2 injection: Experimental investigation. Transp. Porous Media 85 (3), 743–756. doi:10.1007/s11242-010-9589-5
Al-Abri, A., Hiwa, S., and Robert, A. (2009). Experimental investigation of the velocity-dependent relative permeability and sweep efficiency of supercritical CO2 injection into gas condensate reservoirs. J. Nat. Gas Sci. Eng. 1 (4-5), 158–164. doi:10.1016/j.jngse.2009.10.002
Al-Bayati, D., Saeedi, A., Myers, M., White, C., and Xie, Q. (2019). An experimental investigation of immiscible-CO2-flooding efficiency in sandstone reservoirs: Influence of permeability heterogeneity. SPE Reserv. Eval. Eng. 22 (3), 990–997. doi:10.2118/190876-pa
Chen, H., Li, B., Duncan, I., Elkhider, M., and Liu, X. (2020). Empirical correlations for prediction of minimum miscible pressure and near-miscible pressure interval for oil and CO2 systems. Fuel 278, 118272. doi:10.1016/j.fuel.2020.118272
Chen, H., Li, B., Zhang, X., Wang, Q., Wang, X., Yang, S., et al. (2019). Effect of gas contamination and well depth on pressure interval of CO2 near-miscible flooding. J. Petroleum Sci. Eng. 176, 43–50. doi:10.1016/j.petrol.2019.01.062
Chen, H., Zhang, X., Chen, Y., Tang, H., Mei, Y., Li, B., et al. (2017). Study on pressure interval of near-miscible flooding by production gas Re-injection in QHD offshore oilfield. J. Petroleum Sci. Eng. 157, 340–348. doi:10.1016/j.petrol.2017.07.045
Desch, J. B., Larsen, W. K., Lindsay, R. F., and Nettle, R. L. (1984). Enhanced oil recovery by CO2 miscible displacement in the little knife field, billings county, north Dakota. J. petroleum Technol. 36 (09), 1592–1602. doi:10.2118/10696-pa
Dicharry, R. M., Perryman, T. L., and Ronquille, J. D. (1973). Evaluation and design of a CO2 miscible flood project-SACROC unit, Kelly-Snyder field. J. Petroleum Technol. 25 (11), 1309–1318. doi:10.2118/4083-pa
Ding, M., Gao, M., Wang, Y., Qu, Z., and Chen, X. (2019). Experimental study on CO2-EOR in fractured reservoirs: Influence of fracture density, miscibility and production scheme. J. Petroleum Sci. Eng. 174, 476–485. doi:10.1016/j.petrol.2018.11.039
Du, D., Li, C., Song, X., Liu, Q., Ma, N., Wang, X., et al. (2021). Experimental study on residue oil distribution after the supercritical CO2 huff-n-puff process in low permeability cores with Nuclear Magnetic Resonance (NMR). Arabian J. Chem. 14 (10), 103355. doi:10.1016/j.arabjc.2021.103355
Fakher, S., and Imqam, A. (2020). An experimental investigation of immiscible carbon dioxide interactions with crude oil: Oil swelling and asphaltene agitation. Fuel 269, 117380. doi:10.1016/j.fuel.2020.117380
Gao, H., and Pu, W. (2021). Experimental study on supercritical CO2 huff and puff in tight conglomerate reservoirs. ACS omega 6 (38), 24545–24552. doi:10.1021/acsomega.1c03130
Golkari, A., and Riazi, M. (2017). Experimental investigation of miscibility conditions of dead and live asphaltenic crude oil–CO2 systems. J. Pet. Explor. Prod. Technol. 7 (2), 597–609. doi:10.1007/s13202-016-0280-4
Hong, D., Cao, J., Wu, T., Dang, S., Hu, W., and Yao, S. (2020). Authigenic clay minerals and calcite dissolution influence reservoir quality in tight sandstones: Insights from the central Junggar Basin, NW China. Energy Geosci. 1 (1–2), 8–19. doi:10.1016/j.engeos.2020.03.001
Hou, E., Cong, T., and Xie, X. (2020). Ground surface fracture development characteristics of shallow double coal seam staggered mining based on particle flow. J. Min. Strata Control Eng. 2 (1), 013521. doi:10.13532/j.jmsce.cn10-1638/td.2020.01.002
Hu, Y., Guo, C., and Xu, X. (2015). Pore throat structure and flow characteristics of sandstone reservoirs. Petroleum Geol. Exp. 37 (3), 390–393.
Hu, Y., Patmonoaji, A., Zhang, C., and Suekane, T. (2020). Experimental study on the displacement patterns and the phase diagram of immiscible fluid displacement in three-dimensional porous media. Adv. Water Resour. 140, 103584. doi:10.1016/j.advwatres.2020.103584
Huang, X., Zhang, Y., He, M., Li, X., Yang, W., Lu, J., et al. (2022). Asphaltene precipitation and reservoir damage characteristics of CO2 flooding in different microscopic structure types in tight light oil reservoirs. Fuel 312, 122943. doi:10.1016/j.fuel.2021.122943
Jia, Z., Ning, Z., Gao, X., Wang, Q., Zhang, W., Cheng, Z., et al. (2021). Experimental investigation on molecular-scale mechanism of wettability alteration induced by supercritical carbon dioxide-water-rock reaction. J. Petroleum Sci. Eng. 205, 108798. doi:10.1016/j.petrol.2021.108798
Kazemzadeh, Y., Parsaei, R., and Riazi, M. (2015). Experimental study of asphaltene precipitation prediction during gas injection to oil reservoirs by interfacial tension measurement. Colloids Surfaces A Physicochem. Eng. Aspects 466, 138–146. doi:10.1016/j.colsurfa.2014.10.053
Lan, S. R., Song, D. Z., Li, Z. L., and Liu, Y. (2021). Experimental study on acoustic emission characteristics of fault slip process based on damage factor. J. Min. Strata Control Eng. 3 (3), 033024. doi:10.13532/j.jmsce.cn10-1638/td.20210510.002
Lashkarbolooki, M., Vaezian, A., Hezave, A. Z., Ayatollahi, S., and Riazi, M. (2016). Experimental investigation of the influence of supercritical carbon dioxide and supercritical nitrogen injection on tertiary live-oil recovery. J. Supercrit. Fluids 117, 260–269. doi:10.1016/j.supflu.2016.07.004
Li, H. (2022). Research progress on evaluation methods and factors influencing shale brittleness: A review. Energy Rep. 8, 4344–4358. doi:10.1016/j.egyr.2022.03.120
Li, L., Chen, Z., Su, Y. L., Fan, L. Y., Tang, M. R., Tu, J. W., et al. (2021). Experimental investigation on enhanced-oil-recovery mechanisms of using supercritical carbon dioxide as prefracturing energized fluid in tight oil reservoir. SPE J. 26 (05), 3300–3315. doi:10.2118/202279-pa
Li, X., Xue, J., Wang, Y., Yang, W., and Lu, J. (2022). Experimental study of oil recovery from pore of different sizes in tight sandstone reservoirs during CO2 flooding. J. Petroleum Sci. Eng. 208, 109740. doi:10.1016/j.petrol.2021.109740
Li, Y., Zhou, D., Wang, W., Jiang, T. X., and Xue, Z. J. (2020). Development of unconventional gas and technologies adopted in China. Energy Geosci. 1 (1–2), 55–68. doi:10.1016/j.engeos.2020.04.004
Liu, Y., Chen, L., Tang, Y., Zhang, X., and Qiu, Z. (2022). Synthesis and characterization of nano-SiO2@octadecylbisimidazoline quaternary ammonium salt used as acidizing corrosion inhibitor. Rev. Adv. Mater. Sci. 61 (1), 186–194. doi:10.1515/rams-2022-0006
Lv, G., Li, Q., Wang, S., and Li, X. (2015). Key techniques of reservoir engineering and injection–production process for CO2 flooding in China's SINOPEC Shengli Oilfield. J. CO2 Util. 11, 31–40. doi:10.1016/j.jcou.2014.12.007
Mahmi, O., Dypvik, H., and Hammer, E. (2018). Diagenetic influence on reservoir quality evolution, examples from Triassic conglomerates/arenites in the Edvard Grieg field, Norwegian North Sea. Mar. Petroleum Geol. 93, 247–271. doi:10.1016/j.marpetgeo.2018.03.006
Mahmud, H., Hisham, M., Mahmud, M., Leong, V. H., and Shafiq, M. U. (2020). Petrophysical interpretations of subsurface stratigraphic correlations, Baram Delta, Sarawak, Malaysia. Energy Geosci. 1 (3–4), 100–114. doi:10.1016/j.engeos.2020.04.005
Mirzaei-Paiaman, A., and Ghanbarian, B. (2021). A new methodology for grouping and averaging capillary pressure curves for reservoir models. Energy Geosci. 2 (1), 52–62. doi:10.1016/j.engeos.2020.09.001
Paul, G. W., Lake, L. W., and Gould, T. L. (1984). “A simplified predictive model for CO2 miscible flooding,” in SPE annual technical conference and exhibition (Houston: OnePetro).
Paxton, S. T., Szzbo, J. O., Ajdukiewicz, J. M., and Klimentidis, P. E. (2002). Construction of an intergranular volume compaction curve for evaluating and predicting compaction and porosity loss in rigid-rain sandstone reservoirs. AAPG Bull. 86 (12), 2047–2067. doi:10.1306/61EEDDFA-173E-11D7-8645000102C1865D
Qiao, J. C., Zeng, J. H., Jiang, S., Feng, S., Feng, X., Guo, Z., et al. (2019). Heterogeneity of reservoir quality and gas accumulation in tight sandstone reservoirs revealed by pore structure characterization and physical simulation. Fuel 253, 1300–1316. doi:10.1016/j.fuel.2019.05.112
Qie, L., Shi, Y. N., and Liu, J. S. (2021). Experimental study on grouting diffusion of gangue solid filling bulk materials. J. Min. Strata Control Eng. 3 (2), 023011. doi:10.13532/j.jmsce.cn10-1638/td.20201111.001
Qin, Z., Arshadi, M., and Piri, M. (2021). Near-miscible supercritical CO2 injection in oil-wet carbonate: A pore-scale experimental investigation of wettability state and three-phase flow behavior. Adv. Water Resour. 158, 104057. doi:10.1016/j.advwatres.2021.104057
Santosh, M., and Feng, Z. Q. (2020). New horizons in energy geoscience. Energy Geosci. 1 (1–2), 1. doi:10.1016/j.engeos.2020.05.005
Song, Y., Jiang, L., Liu, Y., Yang, M., Zhao, Y., Zhu, N., et al. (2012). An experimental study on CO2/water displacement in porous media using high-resolution Magnetic Resonance Imaging. Int. J. Greenh. Gas Control 10, 501–509. doi:10.1016/j.ijggc.2012.07.017
Stalkup, F. I. (1978). Carbon dioxide miscible flooding: Past, present, and outlook for the future. J. Petroleum Technol. 30 (08), 1102–1112. doi:10.2118/7042-pa
Trevisan, L., Pini, R., Cihan, A., Birkholzer, J. T., Zhou, Q., Illangasekare, T. H., et al. (2014). Experimental investigation of supercritical CO2 trapping mechanisms at the intermediate laboratory scale in well-defined heterogeneous porous media. Energy Procedia 63, 5646–5653. doi:10.1016/j.egypro.2014.11.597
Wang, H., Tian, L., Huo, M., Xu, S., Liu, Z., Zhang, K., et al. (2022). Dynamic track model of miscible CO2 geological utilizations with complex microscopic pore-throat structures. Fuel 322, 124192. doi:10.1016/j.fuel.2022.124192
Wang, J., and Wang, X. L. (2021). Seepage characteristic and fracture development of protected seam caused by mining protecting strata. J. Min. Strata Control Eng. 3 (3), 033511. doi:10.13532/j.jmsce.cn10-1638/td.20201215.001
Wang, J., Zhang, Y., and Xie, J. (2020). Influencing factors and application prospects of CO2 flooding in heterogeneous glutenite reservoirs. Sci. Rep. 10 (1), 1839. doi:10.1038/s41598-020-58792-z
Wang, Q., Yang, S., Lorinczi, P., Glover, P. W., and Lei, H. (2019). Experimental investigation of oil recovery performance and permeability damage in multilayer reservoirs after CO2 and water–alternating-CO2 (CO2–WAG) flooding at miscible pressures. Energy fuels. 34 (1), 624–636. doi:10.1021/acs.energyfuels.9b02786
Wang, S., Jiang, L., Cheng, Z., Liu, Y., Zhao, J., Song, Y., et al. (2021). Experimental study on the CO2-decane displacement front behavior in high permeability sand evaluated by magnetic resonance imaging. Energy 217, 119433. doi:10.1016/j.energy.2020.119433
Wang, Y., Wei, N., Zhang, C., Wietsma, T. W., Bonneville, A., Li, X., et al. (2018). Experimental study of drying effects during supercritical CO2 displacement in a pore network. Microfluid. Nanofluidics 22 (9), 101. doi:10.1007/s10404-018-2122-9
Xu, N., and Gao, C. (2020). Study on the special rules of surface subsidence affected by normal faults. J. Min. Strata Control Eng. 2 (1), 011007. doi:10.13532/j.jmsce.cn10-1638/td.2020.01.011
Xue, F., Liu, X. X., and Wang, T. Z. (2021). Research on anchoring effect of jointed rock mass based on 3D printing and digital speckle technology. J. Min. Strata Control Eng. 3 (2), 023013. doi:10.13532/j.jmsce.cn10-1638/td.20201020.001
Yang, J. X., Luo, M. K., Zhang, X. W., and Huang, N. (2021). Mechanical properties and fatigue damage evolution of granite under cyclic loading and unloading conditions. J. Min. Strata Control Eng. 3 (3), 033016. doi:10.13532/j.jmsce.cn10-1638/td.20210510.001
Yin, S., and Wu, Z. (2020). Geomechanical simulation of low-order fracture of tight sandstone. Mar. Petroleum Geol. 100, 104359. doi:10.1016/j.marpetgeo.2020.104359
Yongle, H. U., Mingqiang, H. A. O., Guoli, C. H. E. N., Ruiyan, S. U. N., and Shi, L. I. (2019). Technologies and practice of CO2 flooding and sequestration in China. Petroleum Explor. Dev. 46 (4), 753–766. doi:10.1016/s1876-3804(19)60233-8
Yoshida, M., and Santosh, M. (2020). Energetics of the solid Earth: An integrated perspective. Energy Geosci. 1 (1–2), 28–35. doi:10.1016/j.engeos.2020.04.001
Zekri, A. Y., Shedid, S. A., and Almehaideb, R. A. (2009). Investigation of supercritical carbon dioxide, aspheltenic crude oil, and formation brine interactions in carbonate formations. J. Petroleum Sci. Eng. 69 (1-2), 63–70. doi:10.1016/j.petrol.2009.05.009
Zhang, B., Shen, B., and Zhang, J. (2020). Experimental study of edge-opened cracks propagation in rock-like materials. J. Min. Strata Control Eng. 2 (3), 033035. doi:10.13532/j.jmsce.cn10-1638/td.20200313.001
Zhang, N., Wei, M., and Bai, B. (2018). Statistical and analytical review of worldwide CO2 immiscible field applications. Fuel 220, 89–100. doi:10.1016/j.fuel.2018.01.140
Zhao, F., Hao, H., Hou, J., Hou, L., and Song, Z. (2015). CO2 mobility control and sweep efficiency improvement using starch gel or ethylenediamine in ultra-low permeability oil layers with different types of heterogeneity. J. Petroleum Sci. Eng. 133, 52–65. doi:10.1016/j.petrol.2015.05.014
Keywords: CO2 flooding technology, beach-bar sand reservoir, CO2 miscible flooding, recovery factor, field study
Citation: Yang S, Xu Y, Huang Z, Zhang Q, Liu T and Li X (2022) Application of CO2 miscible flooding in ultra-low permeability beach-bar sand reservoir. Front. Earth Sci. 10:970719. doi: 10.3389/feart.2022.970719
Received: 16 June 2022; Accepted: 15 July 2022;
Published: 15 August 2022.
Edited by:
Wenlong Ding, China University of Geosciences, ChinaCopyright © 2022 Yang, Xu, Huang, Zhang, Liu and Li. This is an open-access article distributed under the terms of the Creative Commons Attribution License (CC BY). The use, distribution or reproduction in other forums is permitted, provided the original author(s) and the copyright owner(s) are credited and that the original publication in this journal is cited, in accordance with accepted academic practice. No use, distribution or reproduction is permitted which does not comply with these terms.
*Correspondence: Qunshuang Zhang, enFzX2hhMTIzQDE2My5jb20=