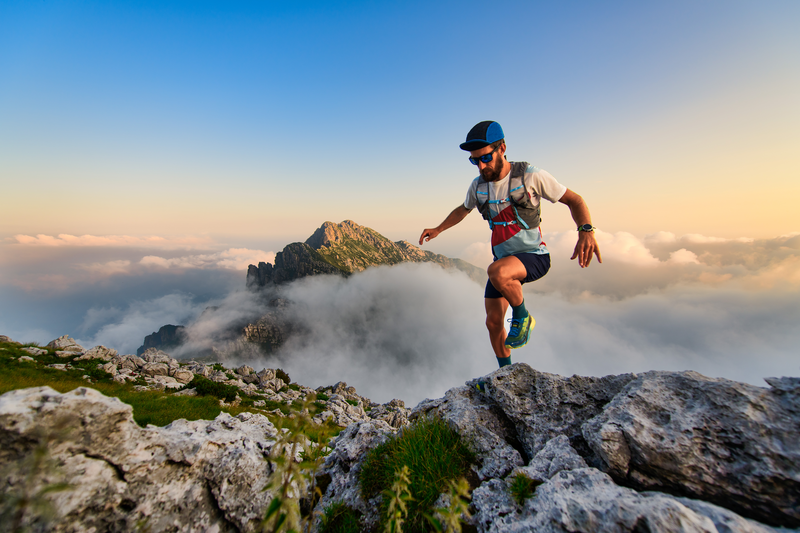
95% of researchers rate our articles as excellent or good
Learn more about the work of our research integrity team to safeguard the quality of each article we publish.
Find out more
EDITORIAL article
Front. Earth Sci. , 05 July 2022
Sec. Geochemistry
Volume 10 - 2022 | https://doi.org/10.3389/feart.2022.966090
This article is part of the Research Topic Refining the Interpretation of Nitrogen Isotopes in Deep Time Systems View all 11 articles
Editorial on the Research Topic
Refining the Interpretation of Nitrogen Isotopes in Deep-Time Systems
The nitrogen stable isotope composition in sedimentary rocks (δ15N) is increasingly used in deep-time studies for reconstructing changes in the N-biogeochemical cycle (e.g., reviews in Algeo et al., 2014; Ader et al., 2016; Stüeken et al., 2016). Analytical advances in EA-IRMS systems have greatly decreased the δ15N measurement time, complexity, and detection limit, while allowing simultaneous δ13C measurements, making the δ15N proxy a standard procedure in paleo-environmental studies (Mulvaney, 2012). Hence, it has already provided some insight into the Precambrian evolution of the marine nitrogen cycle (e.g., Thomazo et al., 2011; Ader et al., 2014; Stüeken et al., 2015, 2016, 2021a, Stüeken et al.; Michiels et al., 2017; Zerkle et al., 2017; Kipp et al., 2018; Luo et al., 2018) and its linkage to the history of Earth-surface oxygenation (Lyons et al., 2014) and biotic evolution (Stüeken, 2013). For the more recent Phanerozoic times, it has yielded detailed reconstructions of nitrogen cycling at the regional scale (e.g., Sachs and Repeta, 1999; Fulton et al., 2012), and documented wholesale reorganization of the marine nitrogen cycle in conjunction with major Phanerozoic biocrises (e.g., Xie et al., 2010, 2017; Luo et al., 2011; Schoepfer et al., 2016) as well as systematic changes related to major climate events (e.g., Algeo et al., 2008, 2014; Yao et al., 2015; Luo et al., 2016).
However, many uncertainties remain in the application of this proxy. The goals of the contributions to this Research Topic are thus to further refine the applicability of the δ15N proxy in sedimentary rocks and develop more nuanced interpretations and research questions for paleo-environmental reconstructions.
As reviewed in several studies (Ader et al., 2006; Robinson et al., 2012; Thomazo and Papineau, 2013; Ader et al., 2016; Stüeken et al.), including two of the present Research Topic (Stüeken et al.; Quan and Adeboye), one of the key uncertainties is the poor knowledge of the impact of diagenesis, catagenesis, metamorphism and fluid migration on primary δ15N signatures. Yet, it is of utmost importance to be able to precisely reconstruct primary δ15N values in order to make reliable paleo-environmental reconstructions, these aspects will therefore need to be better charaterized.
Another important uncertainty is the fact that, although the nitrogen fluxes operating at any given time in an ecosystem depend on the presence, absence or abundance of oxygen, several isotopic fractionations associated with N-cycling are not diagnostic of a particular redox state and hence of nitrogen speciation as nitrate or ammonium (Brandes et al., 2007; Thamdrup, 2012; Quan and Adeboye).
In addition, most of the current underlying assumptions supporting δ15N interpretation in the sedimentary rock record rely heavily on analogies with the present-day marine nitrogen cycle (e.g., Galbraith et al., 2008; Sigman et al., 2009; Robinson et al., 2012; Tesdal et al., 2013), which may not be the best analogue for deep-time lakes, restricted basins and oceans.
Two papers address the goal of refining the applicability of the δ15N proxy via the study of modern systems: the Dziani Dzaha, a saline and alkaline lake (Cadeau et al.), and the Coorong lagoon showing a strong salinity gradient (Priestley et al.). The results show that, while in the Coorong lagoon sediment δ15N records as expected the δ15N of primary producers (Priestley et al.), in the Dziani Dzaha lake the sediment δ15N values are more positive by three‰ compared to primary producers, possibly as a result of 15N-enriched ammonium assimilation in alkaline bottom waters (Cadeau et al.). Taken together, these two studies highlight that not only redox conditions but also salinity and pH may influence the nitrogen cycle. These aspects will need to be better integrated into future studies of deep-time nitrogen cycling.
The remaining papers address the goal of developing more nuanced interpretation of the δ15N proxy in deep-time by coupling it to other proxies, such as redox indicators, C/N ratio, δ13Corg and δ13Ccarb and biomarker δ15N. Two papers characterize nitrogen speciation (ammonium or nitrate) in the water column by coupling δ15N to other aqueous redox proxies. Johnson et al. use concentrations of U, V and Mo, along with Fe-speciation, in sedimentary successions of the Yangtze Platform during the Neoproterozoic Cryogenian Period, allowing them to show that nitrate was stable in the water column and to identify temporal variations in denitrification rates. Riquier et al. in a detailed multi-proxy study (δ13Corg, δ15Nbulk, Rock-Eval and trace metals) of the oceanic anoxic event (OAE-2) of the DSDP 367 succession from Cape Verde, show that δ15N values can be interpreted as evidence of ammonium assimilation. This implies that ammonium (and hence anoxia) reached the photic zone, corroborating molecular studies indicating photic zone anoxia at that time (Higgins et al., 2012).
Three studies identify feedbacks between the C- and N-cycles by coupling δ15N to δ13Ccarb and/or δ13Corg at high stratigraphic resolution. Xu et al. identify subtle changes in δ15N in the Yangtze platform that coincided with the Shuram Excursion, i.e., the largest negative δ13Ccarb excursion in Earth history (Grotzinger et al., 2011; Cao et al., 2020). This work is novel in that it highlights the variability in fractionation factors as a possible driver of subtle δ15N shifts in the paleoenvironment. Fraga-Ferreira et al. this volume also identify subtle changes in the δ15N values that coincided with the onset of an extreme δ13Ccarb positive isotope excursion and with variations in Sr isotope ratios and redox tracers in several sections of the Ediacarian/Cambrian Bambui Group (Brazil) (Caetano-Filho et al., 2021), further highlighting the close linkage between nitrogen and carbon cycling. In the same vein, Mercuzot et al. explore a combination of several proxies (δ15Nbulk to C/N, δ13Corg, Rock-Eval and palynofacies analyses) to deconvolute terrestrial from autochthonous organic matter in several sections of Late Carboniferous to Early Permian continental basins, providing insights into nitrogen cycling during times of enhanced autochthonous organic matter accumulation.
Finally, Hallmann et al., following the lead of a handful of previous studies (e.g., Sachs and Repeta, 1999; Higgins et al., 2012) reaffirmed the power of coupling of δ15N in bulk rocks and kerogens to that of N-containing biomarkers. In the case of Ediacaran sedimentary successions from Oman, the authors were also able to show that primary producers assimilated ammonium at a shallow redoxcline during times of enhanced water-column stratification. Furthermore, they identify and tentatively quantify the biomass produced by eukaryotic and cyanobacterial oxygenic primary producers, as well as anoxygenic primary producers.
The findings reported in these contributions show that providing context using other proxies allows the interpretation of even subtle changes in δ15N. Collectively, the studies in this Research Topic serve to further our understanding of the deep-time marine nitrogen cycle, providing a baseline for evaluating present-day anthropogenic changes in nitrogen cycling and their impacts on the environment and biosphere (Fowler et al., 2013).
MA drafted a first version of this editorial. All authors contributed to and approved the final version.
The authors declare that the research was conducted in the absence of any commercial or financial relationships that could be construed as a potential conflict of interest.
All claims expressed in this article are solely those of the authors and do not necessarily represent those of their affiliated organizations, or those of the publisher, the editors and the reviewers. Any product that may be evaluated in this article, or claim that may be made by its manufacturer, is not guaranteed or endorsed by the publisher.
We deeply thank all the authors, reviewers and Editors of Frontiers in Earth Sciences, who have participated in this Research Topic.
Ader, M., Cartigny, P., Boudou, J. P., Oh, J. H., Petit, E., and Javoy, M. (2006). Nitrogen Isotopic Evolution of Carbonaceous Matter during Metamorphism: Methodology and Preliminary Results. Chem. Geol. 232 (3-4), 152–169. doi:10.1016/j.chemgeo.2006.02.019
Ader, M., Sansjofre, P., Halverson, G. P., Busigny, V., Trindade, R. I. F., Kunzmann, M., et al. (2014). Ocean Redox Structure across the Late Neoproterozoic Oxygenation Event: A Nitrogen Isotope Perspective. Earth Planet. Sci. Lett. 396, 1–13. doi:10.1016/j.epsl.2014.03.042
Ader, M., Thomazo, C., Sansjofre, P., Busigny, V., Papineau, D., Laffont, R., et al. (2016). Interpretation of the Nitrogen Isotopic Composition of Precambrian Sedimentary Rocks: Assumptions and Perspectives. Chem. Geol. 429, 93–110. doi:10.1016/j.chemgeo.2016.02.010
Algeo, T. J., Meyers, P. A., Robinson, R. S., Rowe, H., and Jiang, G. Q. (2014). Icehouse-greenhouse Variations in Marine Denitrification. Biogeosciences 11 (4), 1273–1295. doi:10.5194/bg-11-1273-2014
Algeo, T., Rowe, H., Hower, J. C., Schwark, L., Herrmann, A., and Heckel, P. (2008). Changes in Ocean Denitrification during Late Carboniferous Glacial-Interglacial Cycles. Nat. Geosci. 1 (10), 709–714. doi:10.1038/ngeo307
Brandes, J. A., Devol, A. H., and Deutsch, C. (2007). New Developments in the Marine Nitrogen Cycle. Chem. Rev. 107 (2), 577–589. doi:10.1021/cr050377t
Caetano-Filho, S., Sansjofre, P., Ader, M., Paula-Santos, G. M., Guacaneme, C., Babinski, M., et al. (2021). A Large Epeiric Methanogenic Bambuí Sea in the Core of Gondwana Supercontinent? Geosci. Front. 12 (1), 203–218. doi:10.1016/j.gsf.2020.04.005
Cao, M., Daines, S. J., Lenton, T. M., Cui, H., Algeo, T. J., Dahl, T. W., et al. (2020). Comparison of Ediacaran Platform and Slope δ238U Records in South China: Implications for Global-Ocean Oxygenation and the Origin of the Shuram Excursion. Geochimica Cosmochimica Acta 287, 111–124. doi:10.1016/j.gca.2020.04.035
Fowler, D., Coyle, M., Skiba, U., Sutton, M. A., Cape, J. N., Reis, S., et al. (2013). The Global Nitrogen Cycle in the Twenty-First Century. Phil. Trans. R. Soc. B 368 (1621), 20130164. doi:10.1098/rstb.2013.0164
Fulton, J. M., Arthur, M. A., and Freeman, K. H. (2012). Black Sea Nitrogen Cycling and the Preservation of Phytoplankton δ15N Signals during the Holocene. Glob. Biogeochem. Cycles 26 (2), GB2030. doi:10.1029/2011gb004196
Galbraith, E. D., Sigman, D. M., Robinson, R. S., and Pedersen, T. F. (2008). Nitrogen in Past Marine Environments. Nitrogen Mar. Environ. 2, 1497–1535. doi:10.1016/b978-0-12-372522-6.00034-7
Grotzinger, J. P., Fike, D. A., and Fischer, W. W. (2011). Enigmatic Origin of the Largest-Known Carbon Isotope Excursion in Earth's History. Nat. Geosci. 4 (5), 285–292. doi:10.1038/ngeo1138
Higgins, M. B., Robinson, R. S., Husson, J. M., Carter, S. J., and Pearson, A. (2012). Dominant Eukaryotic Export Production during Ocean Anoxic Events Reflects the Importance of Recycled NH 4 +. Proc. Natl. Acad. Sci. U.S.A. 109 (7), 2269–2274. doi:10.1073/pnas.1104313109
Kipp, M. A., Stüeken, E. E., Yun, M., Bekker, A., and Buick, R. (2018). Pervasive Aerobic Nitrogen Cycling in the Surface Ocean across the Paleoproterozoic Era. Earth Planet. Sci. Lett. 500, 117–126. doi:10.1016/j.epsl.2018.08.007
Luo, G., Junium, C. K., Izon, G., Ono, S., Beukes, N. J., Algeo, T. J., et al. (2018). Nitrogen Fixation Sustained Productivity in the Wake of the Palaeoproterozoic Great Oxygenation Event. Nat. Commun. 9 (1), 978–979. doi:10.1038/s41467-018-03361-2
Luo, G., Algeo, T. J., Zhan, R., Yan, D., Huang, J., Liu, J., et al. (2016). Perturbation of the Marine Nitrogen Cycle during the Late Ordovician Glaciation and Mass Extinction. Palaeogeogr. Palaeoclimatol. Palaeoecol. 448, 339–348. doi:10.1016/j.palaeo.2015.07.018
Luo, G., Wang, Y., Algeo, T. J., Kump, L. R., Bai, X., Yang, H., et al. (2011). Enhanced Nitrogen Fixation in the Immediate Aftermath of the Latest Permian Marine Mass Extinction. Geology 39 (7), 647–650. doi:10.1130/g32024.1
Lyons, T. W., Reinhard, C. T., and Planavsky, N. J. (2014). The Rise of Oxygen in Earth's Early Ocean and Atmosphere. Nature 506 (7488), 307–315. doi:10.1038/nature13068
Michiels, C. C., Darchambeau, F., Roland, F. A. E., Morana, C., Llirós, M., García-Armisen, T., et al. (2017). Iron-dependent Nitrogen Cycling in a Ferruginous Lake and the Nutrient Status of Proterozoic Oceans. Nat. Geosci. 10 (3), 217–221. doi:10.1038/ngeo2886
Mulvaney, R. L. (2012). “Mass Spectrometry,” in Nitrogen Isotope Techniques. Editors E. Paul, J. Melillo, R. Knowles, and H. Blackburn (Amsterdam: Elsevier), 11–58.
Robinson, R. S., Kienast, M., Luiza Albuquerque, A., Altabet, M., Contreras, S., De Pol Holz, R., et al. (2012). A Review of Nitrogen Isotopic Alteration in Marine Sediments. Paleoceanography 27 (4), 002321. doi:10.1029/2012pa002321
Sachs, J. P., and Repeta, D. J. (1999). Oligotrophy and Nitrogen Fixation during Eastern Mediterranean Sapropel Events. Science 286 (5449), 2485–2488. doi:10.1126/science.286.5449.2485
Schoepfer, S. D., Algeo, T. J., Ward, P. D., Williford, K. H., and Haggart, J. W. (2016). Testing the Limits in a Greenhouse Ocean: Did Low Nitrogen Availability Limit Marine Productivity during the End-Triassic Mass Extinction? Earth Planet. Sci. Lett. 451, 138–148. doi:10.1016/j.epsl.2016.06.050
Sigman, D. M., Karsh, K. L., and Casciotti, K. L. (2009). Ocean Process Tracers: Nitrogen Isotopes in the Ocean. San Francisco: Academia.
Stüeken, E. E., Buick, R., Guy, B. M., and Koehler, M. C. (2015). Isotopic Evidence for Biological Nitrogen Fixation by Molybdenum-Nitrogenase from 3.2 Gyr. Nature 520 (7549), 666–669. doi:10.1038/nature14180
Stüeken, E. E. (2013). A Test of the Nitrogen-Limitation Hypothesis for Retarded Eukaryote Radiation: Nitrogen Isotopes across a Mesoproterozoic Basinal Profile. Geochimica Cosmochimica Acta 120, 121–139. doi:10.1016/j.gca.2013.06.002
Stüeken, E. E., Gregory, D. D., Mukherjee, I., and McGoldrick, P. (2021). Sedimentary Exhalative Venting of Bioavailable Nitrogen into the Early Ocean. Earth Planet. Sci. Lett. 565, 116963. doi:10.1016/j.epsl.2021.116963
Stüeken, E. E., Kipp, M. A., Koehler, M. C., and Buick, R. (2016). The Evolution of Earth's Biogeochemical Nitrogen Cycle. Earth-Science Rev. 160, 220–239. doi:10.1016/j.earscirev.2016.07.007
Stüeken, E. E., Zaloumis, J., Meixnerová, J., and Buick, R. (2017). Differential Metamorphic Effects on Nitrogen Isotopes in Kerogen Extracts and Bulk Rocks. Geochimica Cosmochimica Acta 217, 80–94. doi:10.1016/j.gca.2017.08.019
Tesdal, J.-E., Galbraith, E. D., and Kienast, M. (2013). Nitrogen Isotopes in Bulk Marine Sediment: Linking Seafloor Observations with Subseafloor Records. Biogeosciences 10 (1), 101–118. doi:10.5194/bg-10-101-2013
Thamdrup, B. (2012). New Pathways and Processes in the Global Nitrogen Cycle. Annu. Rev. Ecol. Evol. Syst. 43, 407–428. doi:10.1146/annurev-ecolsys-102710-145048
Thomazo, C., Ader, M., and Philippot, P. (2011). Extreme 15N-Enrichments in 2.72-Gyr-Old Sediments: Evidence for a Turning Point in the Nitrogen Cycle. Geobiology 9, 107–120. doi:10.1111/j.1472-4669.2011.00271.x
Thomazo, C., and Papineau, D. (2013). Biogeochemical Cycling of Nitrogen on the Early Earth. Elements 9 (5), 345–351. doi:10.2113/gselements.9.5.345
Xie, S., Algeo, T. J., Zhou, W., Ruan, X., Luo, G., Huang, J., et al. (2017). Contrasting Microbial Community Changes during Mass Extinctions at the Middle/Late Permian and Permian/Triassic Boundaries. Earth Planet. Sci. Lett. 460, 180–191. doi:10.1016/j.epsl.2016.12.015
Xie, S., Pancost, R. D., Wang, Y., Yang, H., Wignall, P. B., Luo, G., et al. (2010). Cyanobacterial Blooms Tied to Volcanism during the 5 m.Y. Permo-Triassic Biotic Crisis. Geology 38 (5), 447–450. doi:10.1130/g30769.1
Yao, L., Qie, W., Luo, G., Liu, J., Algeo, T. J., Bai, X., et al. (2015). The TICE Event: Perturbation of Carbon-Nitrogen Cycles during the Mid-Tournaisian (Early Carboniferous) Greenhouse-Icehouse Transition. Chem. Geol. 401, 1–14. doi:10.1016/j.chemgeo.2015.02.021
Keywords: nitrogen biogeochemical cycle, modern analog studies, paleoenvironments reconstructions, post depositional alteration, nitrogen isotopes (d15N)
Citation: Ader M, Stüeken E, Sansjofre P, Algeo TJ and Xie S (2022) Editorial: Refining the Interpretation of Nitrogen Isotopes in Deep Time Systems. Front. Earth Sci. 10:966090. doi: 10.3389/feart.2022.966090
Received: 10 June 2022; Accepted: 15 June 2022;
Published: 05 July 2022.
Edited and reviewed by:
Martyn Tranter, Aarhus University, DenmarkCopyright © 2022 Ader, Stüeken, Sansjofre, Algeo and Xie. This is an open-access article distributed under the terms of the Creative Commons Attribution License (CC BY). The use, distribution or reproduction in other forums is permitted, provided the original author(s) and the copyright owner(s) are credited and that the original publication in this journal is cited, in accordance with accepted academic practice. No use, distribution or reproduction is permitted which does not comply with these terms.
*Correspondence: Magali Ader, YWRlckBpcGdwLmZy
Disclaimer: All claims expressed in this article are solely those of the authors and do not necessarily represent those of their affiliated organizations, or those of the publisher, the editors and the reviewers. Any product that may be evaluated in this article or claim that may be made by its manufacturer is not guaranteed or endorsed by the publisher.
Research integrity at Frontiers
Learn more about the work of our research integrity team to safeguard the quality of each article we publish.