- Sustainable System Research Laboratory, Central Research Institute of Electric Power Industry, Abiko, Japan
Recent application of zircon U-Pb geochronology has contributed to deciphering the evolution of caldera systems worldwide. However, in the Tohoku region, NE Japan, this is not the case, although it is well studied as a typical island arc subduction system. Here, U-Pb dating of zircon suggests that the Sanzugawa caldera, the largest caldera in Tohoku since late Miocene, initiated its caldera-forming volcanic activity at ∼7 Ma and culminated its activity in late Pliocene to Quaternary (3.0–1.5 Ma). This is contrary to a previous notion that the caldera developed in late Miocene to Pliocene (6–3 Ma) based on mainly whole-rock K-Ar dating results. This finding may also question a current hypothesis of relatively subdued volcanism at 3.5–1.5 Ma in NE Japan and thus shows the need to date other calderas in the Tohoku region with zircon U-Pb and/or Ar-Ar methods to better understand the magmatic history of this region.
1 Introduction
In the past 2–3 decades, zircon U-Pb dating as well as sanidine Ar-Ar dating has been extensively used to decipher the history of Neogene-Quaternary caldera-forming eruptions (e.g., Long Valley caldera: Crowley et al., 2007, Mark et al., 2017; Toba caldera: Mark et al., 2014, Ito, 2020; Taupo Volcanic Zone: Milicich et al., 2013, 2020). The Tohoku region, NE Japan, is an archetypal island arc, where the Pacific Plate has been subducting beneath the Eurasian Plate at a rate of 8–9 cm/yr with a relatively shallow dip angle of about 30° since at least 2 Ma (Acocella et al., 2008; Yoshida et al., 2014). The resulting volcanism and tectonism have been well documented in the Tohoku region (e.g., Acocella et al., 2008; Yoshida et al., 2014, Yoshida et al., 2020). In essence, in this island arc tectonic setting, numerous (>80) calderas were formed under NE-SW compression during late Miocene-Pliocene, and subsequently a larger volume of andesite (mainly stratovolcanoes) was erupted under E-W compression during the Quaternary (Acocella et al., 2008) (Figure 1). Nevertheless, geochronological data for these Neogene caldera-forming eruptions are mostly based on whole-rock K-Ar and partly zircon fission-track (FT) (e.g., Takeno, 1988; Yamamoto, 1992; Kondo et al., 1998, 2004) with some exceptions (e.g., Takashima et al., 2020). The K-Ar method has a fundamental caveat that it is difficult to differentiate essential and detrital materials for dating caldera-forming pyroclastic deposits, leading to a calculated age which is older than the “true” eruption age. Although zircon FT method can circumvent this problem by analyzing on a grain-by-grain basis, it tends to offer larger uncertainty than the zircon U-Pb method for young (<5 Ma) grains. Therefore, the chronology of caldera-forming eruptions in the young Tohoku Arc should be reexamined using zircon U-Pb and/or Ar-Ar dating. The chronologies generated by reexamining eruptive products will help test current hypotheses about volcanism in the region over the last 5 Ma. For example, a hiatus in volcanic activity in NE Japan from 3.5 to 1.5 Ma was hypothesized (Kimura et al., 2015; Mahony et al., 2016), which seems unlikely considering that a compressive environment and likely increased crustal thickness have promoted favorable conditions for the accumulation of large volumes of silicic magma over the last 3–2 Ma (Mahony et al., 2016). In fact, Fujioka (1983, 1985) recognized more frequent large-scale felsic volcanism at 5–2 Ma in the Tohoku region than at 1–0 Ma using a marine core sediment drilled off the Tohoku region in the Pacific Ocean, whereas Mahony et al. (2016) recognized no intense volcanism at 4–2 Ma using more drill core sediments data than Fujioka (1983). This discrepancy may result from the fact that Fujioka (1983) discarded drill cores that may have suffered from resedimentation, while Mahony et al. (2016) may have used them.
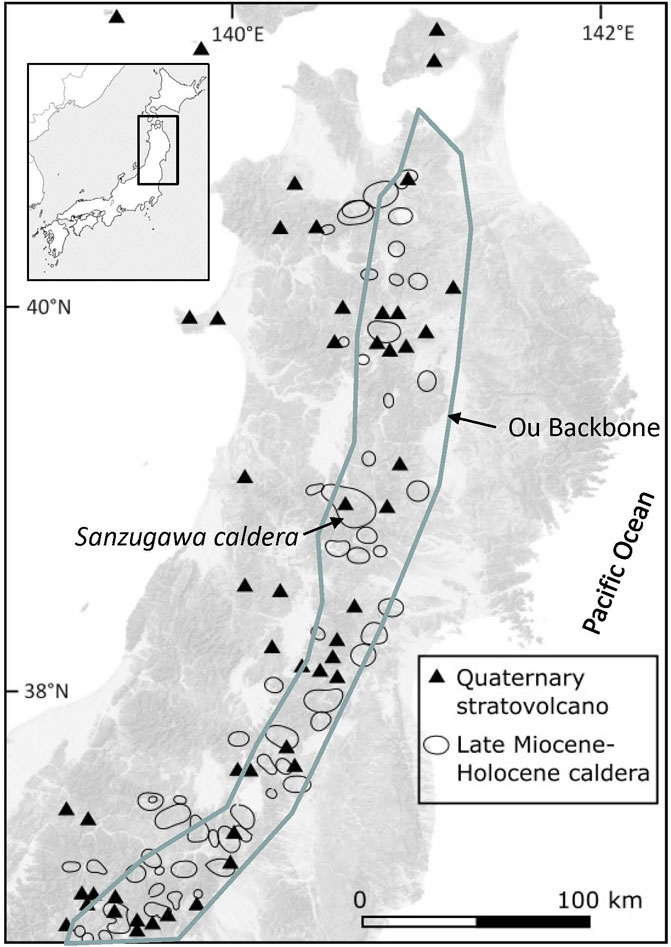
FIGURE 1. Distribution of the late Miocene to Quaternary volcanoes in the Tohoku region modified from Yamamoto (2009). Most volcanoes are situated in the Ou Backbone, an uplifted area of the central mountainous range. Inset map shows Japan and its corresponding area.
Here, I focus on the Sanzugawa caldera (Ito et al., 1989), which is the largest caldera system in the Tohoku region (Yoshida et al., 1999) (Figure 1), and reveal that the area experienced multiple large eruptions from ∼7 Ma to ∼1.5 Ma with no evidence of volcanic lull at 3.5–1.5 Ma; instead, intensive volcanic activities occurred at 3.5–1.5 Ma in and around the Sanzugawa caldera. This indicates the need to date other Tohoku caldera clusters with currently available more sophisticated dating tools (zircon U-Pb and/or Ar-Ar) and reconsider magmatism in the Tohoku region since the late Miocene.
2 Geological setting
2.1 The Sanzugawa caldera and its associates
The Sanzugawa caldera (also called Ogachi caldera) is situated in the middle of the Tohoku caldera cluster (Figure 1) with NW-SE elongation (long axis: ∼30 km; short axis: ∼20 km). It is accompanied by smaller calderas: the Innai caldera at the northwestern corner and the Onikobe caldera at the southeastern corner (Yoshida et al., 1999) (Figure 2). The Sanzugawa caldera is a Valles-type caldera (Smith and Bailey, 1968), because of the presence of pre-caldera “regional tumescence” (Ou Backbone: Figure 1), the pattern of gravity anomalies (lower gravity in the caldera), lithofacies of the caldera fill, and the existence of resurgence and associated volcanoes (Oki, 2016) (Quaternary volcanoes of Mt. Okumiya, Mt. Kobinai, and Mt. Takamatsu in ascending chronological order: Figure 2). The basement rocks, which predominantly bound the western margin of the caldera, are Cretaceous plutonic rocks, Miocene subaqueous volcaniclastic rocks and mudstones. The caldera fill is over 1,400 m thick and consists of the Torageyama Formation, the Sanzugawa Formation and post caldera volcanics (Takeno, 1988).
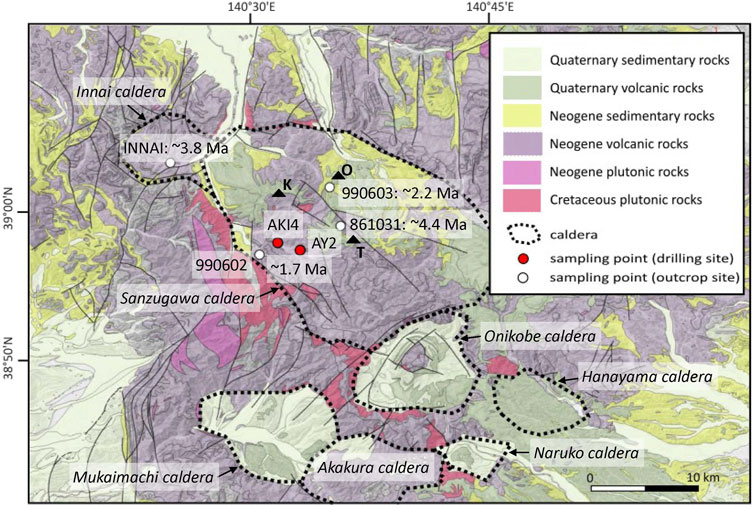
FIGURE 2. Geological map in and around the Sanzugawa caldera modified from Seamless digital geological map of Japan 1: 200,000 (Geological Survey of Japan, AIST). Sample names and zircon U-Pb ages are shown. Black triangles are Quaternary volcanoes (O: Mt. Okumiya, K: Mt. Kobinai, T: Mt. Takamatsu).
The Torageyama Formation consists of a thick (>1,100 m) pile of felsic pyroclastic flows, comprising dacite to rhyolite tuff which is partly welded. It contains abundant plagioclase and quartz, minor pyroxene and biotite, and sparse hornblende crystal fragments. Various kinds of lithic fragments and pumice shreds are also abundant (Takeno, 1988; Yamada, 1988). The Sanzugawa Formation is a lake deposit with a thick (>550 m) pile of volcanic rocks which partly fills the collapsed Sanzugawa basin (Takeno, 1988). The post-caldera volcanics, which are associated with resurgence, are distributed in the central part of the caldera.
Takeno (1988) considered that the caldera-forming eruption, forming the Torageyama Formation, occurred in two episodes (6–5 Ma and 4–3 Ma) based on whole-rock K-Ar dating, which has been widely accepted up until now (Nunohara et al., 2021) (Figure 3). Takeno (1988) also assumed that the uppermost part of the Torageyama Formation is contemporaneous with the Sanzugawa Formation. Therefore, the Torageyama Formation encompasses both caldera-forming and post-caldera ignimbrites. Oki (2016) found 7 pyroclastic flow units (each unit consisting of the following 5 lithofacies: eutaxitic, massive lapilli-tuff; massive lithic breccia; cross-stratified lapilli-tuff; parallel-stratified tuff; diffuse-stratified lapilli-tuff) in the Torageyama Formation and hypothesized that the Sanzugawa caldera was formed by repeated (>7 times) ignimbrite-forming eruptions. The post-caldera Sanzugawa Formation was dated at ∼3 Ma by zircon FT (Ito, 2001).
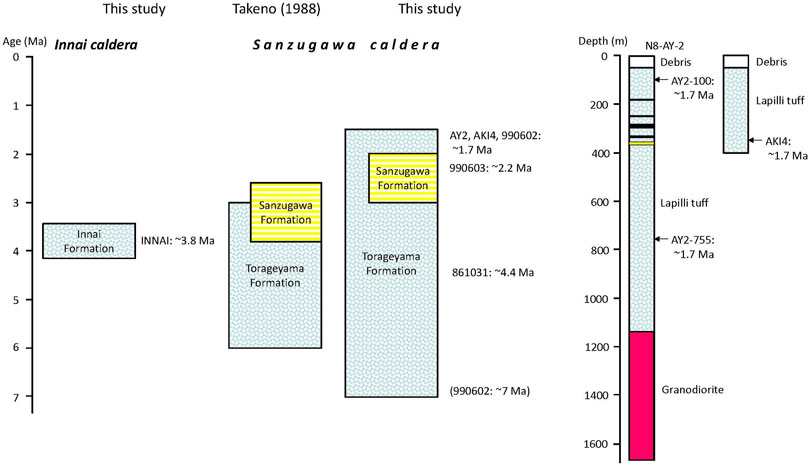
FIGURE 3. Lithostratigraphy related to caldera-forming eruptions in the Sanzugawa and Innai calderas. Sample names and zircon U-Pb ages are shown at their corresponding positions. The age of ∼7 Ma of sample 990602 corresponds to the old component age. Sampling elevations are shown in Supplementary Table S2.
The Innai caldera adjacent to the northwest of the Sanzugawa caldera is hypothesized to have been formed at ∼4 Ma based on whole-rock K-Ar dating (Takeno, 1988; Kondo et al., 2004). It is also assumed as a Valles-type caldera (Aizawa and Yoshida, 1999) and the caldera-fill volcanics are called the Innai Formation (Aizawa and Yoshida, 1999). The Onikobe caldera next to the southeast of the Sanzugawa caldera and the Naruko (or Narugo) caldera further to the southeast are believed to have been formed <1 Ma based on K-Ar, zircon FT, and thermoluminescence methods (Tsuchiya et al., 1997). The Akakura caldera to the south of the Sanzugawa caldera is thought to have been formed at ∼3–1.4 Ma based on whole-rock K-Ar and zircon FT (Otake, 2000). The Mukaimachi caldera, west of the Akakura caldera, is thought to have been formed after the Akakura caldera formation (Otake, 2007). Finally, the Hanayama caldera to the east of the Onikobe caldera is hypothesized to have been formed during the late Miocene (Tsuchiya et al., 1997; Yoshida et al., 2020).
2.2 Samples
Five samples (two are from a single drilling site) from the Torageyama Formation, one sample from the Sanzugawa Formation and one sample from the Innai Formation were used for U-Pb dating (Figure 2). All the samples were originally collected for the FT dating in 1990’s by the Author.
2.2.1 Torageyama Formation
Two drilling samples (sample names: AY2-100 and AY2-755) at depths of ∼100 m and ∼755 m, respectively, were collected from a wellbore (N8-AY-2; shown as AY2 in Figure 2) in the western basin of the Sanzugawa caldera. The N8-AY-2 was drilled for a geothermal exploration project to a depth of ∼1650 m and a granitic basement was encountered at a depth of ∼1140 m. From that depth to ∼50 m in depth, the Torageyama Formation was encountered (Ito, 2000a) (Figure 3). AY2-100 and AY2-755 were obtained as cuttings and a drill core, respectively. They are both dacite lapilli tuff (Figure 4A). In between the two samples, several andesite lavas at depths of 182 m, 255 m, 280 m–300 m, and 330 m–340 m and a siltstone at a depth of 355–368 m were encountered (Ito, 2000a). Therefore, these two samples likely represent different ignimbrite sequences. AY2-100 and AY2-755 were dated at 1.1 ± 0.4 Ma and 1.5 ± 0.4 Ma (errors are shown at the 2σ or 95% confidence level), respectively, by zircon FT dating (Ito, 2000a).
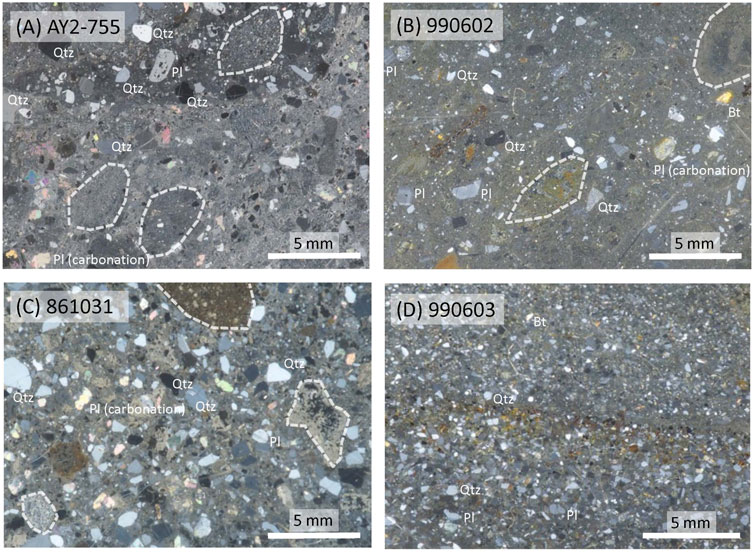
FIGURE 4. Thin section images of representative dated samples taken in cross-polarized light. (A–C) dacite lapilli tuff, (D) laminated sandy tuff. Some lithic fragments are encircled with dashed lines. Some plagioclases are partly altered to calcites. Abbreviations: Qtz, quartz; Pl, plagioclase; Bt, biotite.
A drill core sample (AKI4) was collected from a ∼400 m wellbore situated at ∼2 km northwest from the N8-AY-2 site. The Torageyama Formation was encountered from ∼50 m to ∼400 m (the bottom of the wellbore) (Figure 3). The AKI4 sample, composed of dacite lapilli tuff, was obtained at a depth of ∼350 m. No depositional hiatus was observed from the top of the Torageyama Formation (∼50 m in depth) to the AKI4 sampling position (Ito, 1996), and therefore, the AKI4 seems to belong to the latest ignimbrite sequence of the Torageyama Formation. AKI4 was dated at 1.2 ± 0.4 Ma by zircon FT dating (Ito, 1996).
An outcrop sample (990602), composed of dacite lapilli tuff (Figure 4B), was obtained near the western margin of the Sanzugawa caldera. At the caldera margin, Cretaceous granitic rocks (caldera wall) were intruded by several rhyolitic to andesitic dykes. The 990602 sample was obtained ∼500 m inside of the caldera wall. From the outcrop observation, it was difficult to assume whether the sampled lapilli tuff belongs to an early or a late stage of caldera formation. The 990602 sample was dated at 1.3 ± 0.4 Ma by zircon FT dating (Ito, 2001).
An outcrop sample (861031), composed of dacite lapilli tuff (Figure 4C), was obtained at a central part of the Sanzugawa caldera. Takeno (1988) assumed this sample belonging to an early stage of caldera formation although it may be difficult to pinpoint the stratigraphic position due to a limited exposure of the outcrop. It was dated at 6.0 ± 1.6 Ma by whole-rock K-Ar (Takeno, 1988) and 4.6 ± 0.6 Ma by zircon FT dating (Ito, 2000b).
2.2.2 Sanzugawa Formation
An outcrop sample (990603), composed of laminated sandy tuff (Figure 4D), was obtained at the eastern basin of the Sanzugawa caldera. Since the sample was obtained ∼5 m below Holocene talus deposits, I assume the sample is situated in the uppermost part of the >550 m Sanzugawa Formation. It was dated at 3.2 ± 0.6 Ma by zircon FT dating (Ito, 2001).
2.2.3 Innai Formation
An outcrop sample (INNAI), composed of rhyolite fine tuff, was obtained in the Innai caldera. Yoshida et al. (2020) assumed the caldera-forming volcanic activity of the Innai caldera occurred at 5.0–3.5 Ma.
3 U-Pb dating
Zircons prepared for FT dating at the Central Research Institute of Electric Power Industry (CRIEPI) were used. Zircons were handpicked and embedded in a PFA Teflon sheet and polished to 1 μm diamond paste finish except for some zircons (sample name: INNAI2 and FCT20) which were unpolished and unetched. Most zircons were etched in NaOH-KOH eutectic etchant at 225°C for ∼30–40 h to reveal spontaneous fission tracks (Figure 5). Note that etching does not affect U-Pb dating results (Ito et al., 2017). Zircon grains with low spontaneous fission track density were mainly selected for further analyses as these were expected to represent the eruption age or the most recent magmatic event (Ito et al., 2017). Some grains with a high spontaneous track density were intentionally selected so as to date old zircon grains (Figure 5B).
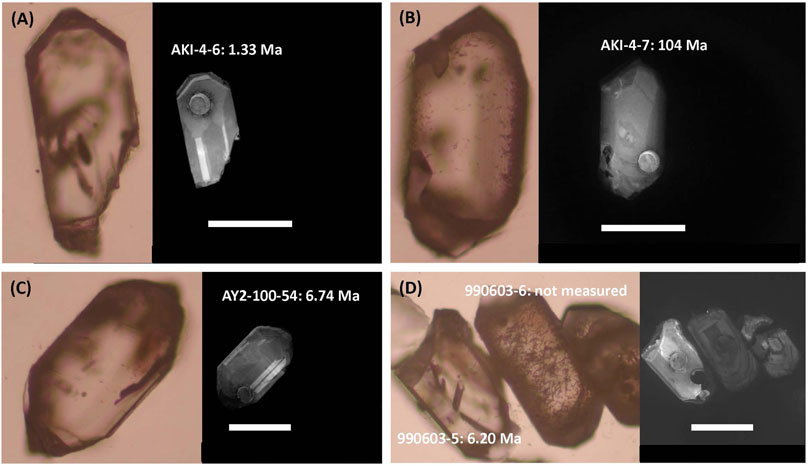
FIGURE 5. Representative etched zircon images (A–D) with sample names and U-Pb ages. Note that the sample 990603-6 in (D) was not U-Pb dated because of a high fission track density. Cathodoluminescence (CL) images were obtained using a Hitachi TM4000Plus electron microscope. Circular craters in CL images are laser-ablated pits of ∼30 μm in diameter. Scale bars in CL images are 100 μm.
Zircon U-Pb dating was performed at CRIEPI, using LA-ICP-MS (on a Thermo Fisher Scientific ELEMENT XR magnetic sector-field ICP-MS coupled to a New Wave Research UP-213 Nd-YAG laser) with experimental conditions primarily following Ito (2020) (Supplementary Table S1). Samples were ablated in helium gas using a 30 μm laser spot with 7–8 J/cm2 energy density and 10 Hz repetition rate. Data were acquired in electrostatic scanning (E-scan) mode over 1080 mass scans during a 30 s background measurement, followed by a 30 s sample ablation and then a 45 s background (washout) measurement. 235U was calculated from 238U assuming 238U/235U = 137.818 (Hiess et al., 2012). Both unknowns and standards were measured in the same conditions.
Data for the first 10 s of ablation were omitted to avoid surface Pb contamination and signal instability, and the following 10 s of data were used for age calculation. Approximately a depth of ∼24 µm for 30 μm laser beam was drilled during the 30 s laser ablation and therefore the U-Pb isotopic data are from approximately 8–16 µm in depth within the zircon crystal.
Individual U-Pb ages were corrected for common-Pb using a modified 207Pb-based method (Sakata, 2018) using values from Stacey and Kramers (1975) and measured 207Pb/206Pb. The modified 207Pb method employs both Th/U and Pa/U partitioning (fTh/U and fPa/U, respectively) for correcting initial 230Th and 231Pa disequilibrium in the zircon-magma system. The fTh/U was calculated assuming the Th/U of magma was 4.0 ± 2.0 and employing individually measured Th/U of zircon. The measured fTh/U with a 50% uncertainty and an assumed fPa/U of 3.36 ± 0.40 (Sakata, 2018) were used for all age calculations. Data with a high (>75%) common Pb contamination (f206%) or a high (>70%) uncertainty were excluded from further analyses (Ito, 2020) and the mean square weighted deviation (MSWD) is used as a statistical test of validity of weighted mean ages. The 91500 zircon (Wiedenbeck et al., 2004) was used as a primary standard with zircons of Plešovice (Sláma et al., 2008), Bishop Tuff (Crowley et al., 2007) and Fish Canyon Tuff (Schmitz and Bowring, 2001) used as secondary reference materials.
U and Th concentrations were quantified by comparing counts of 238U and 232Th for the sample relative to the standard 91500, which is assumed to have homogeneous U and Th concentrations of 80 and 30 ppm respectively (Wiedenbeck et al., 2004), followed by a correction relative to NIST SRM 610 glass standard. No down-hole isotopic (Pb/U, Th/U) fractionation correction was performed because data from the same depth range (or time span) was used for standards and unknowns in each analysis. The results are shown in Supplementary Table S2.
The U-Pb ages from the Plešovice, the Bishop Tuff, and the Fish Canyon Tuff zircons were 342.1 ± 2.3 Ma, 0.77 ± 0.01 Ma, and 28.6 ± 0.5 Ma (Supplementary Table S2), respectively, which are either close to or in accordance with their reference ages of 337.13 ± 0.37 Ma (Sláma, et al., 2008), 0.767 ± 0.001 Ma (Crowley et al., 2007), and 28.402 ± 0.023 Ma (Schmitz and Bowring, 2001).
4 Results
4.1 Torageyama Formation
A total of 51 grains were dated for the AY2-100 sample. Of these, 26 grains passed the criteria set out in Section 3 for both the common Pb and the uncertainty. Five grains showed >10 Ma (150–48 Ma), which I assumed to be xenocrysts and were discarded from further analyses. Taking the weighted mean from the remaining 21 grain ages yields an age of 2.22 ± 0.73 Ma (MSWD = 18) excluding 2 outliers (Figure 6A). The large MSWD of 18 implies that the weighted mean age does not represent the eruption age and is influenced by older magmatic activities.
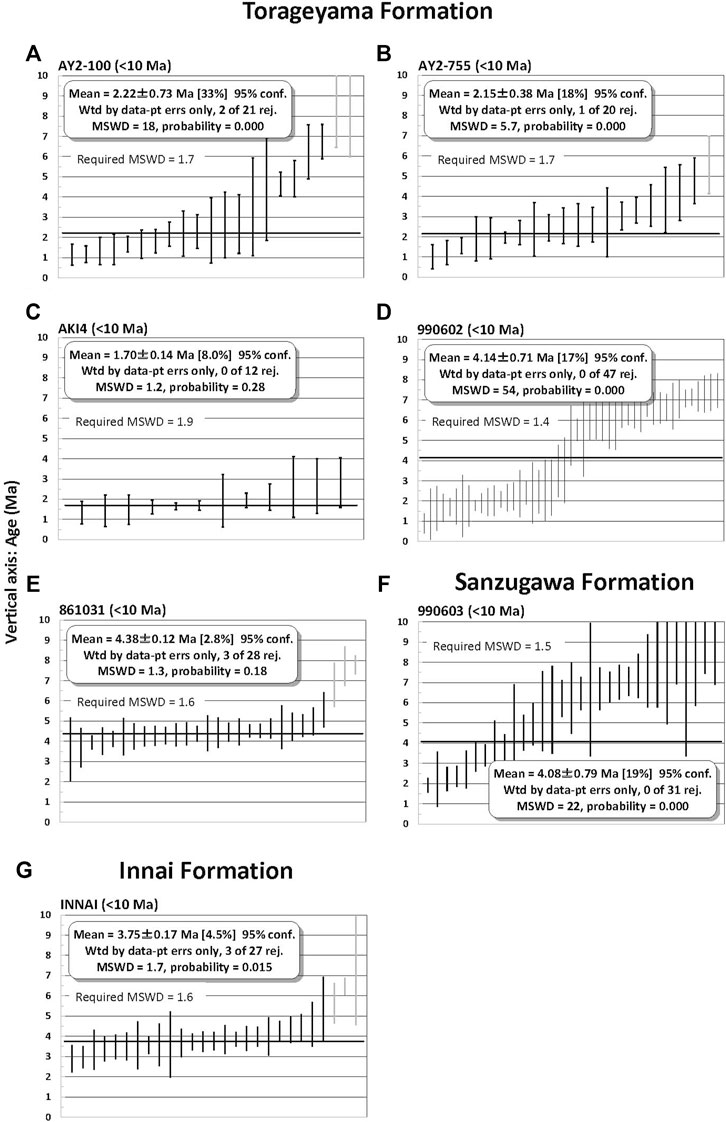
FIGURE 6. 238U-206Pb age distributions for zircons younger than 10 Ma. (A–E) Torageyama Formation, (F) Sanzugawa Formation, (G) Innai Formation. Individual grain ages with 2σ uncertainty are arranged in rank order. Analyses in grey represent statistical outliers and are excluded for weighted mean age calculation. Horizontal bars represent mean U-Pb ages. MSWD: mean square weighted deviation. “Required MSWD” is the largest MSWD value to be a single age population with 95% probability (Wendt and Carl, 1991).
A total of 30 grains were dated for the AY2-755 sample. Of these, 23 grains passed the initial criteria. Three grains showed >10 Ma (98–90 Ma), which were regarded as xenocrysts and were discarded from further analyses. Taking the weighted mean from the remaining 20 grain ages yields an age of 2.15 ± 0.38 Ma (MSWD = 5.7) excluding one outlier (Figure 6B). The large MSWD of 5.7 implies that the weighted mean age does not represent the eruption age and is influenced by older magmatic activities.
A total of 26 grains were dated for the AKI4 sample. Of these, 24 grains passed the initial criteria. Twelve grains showed >10 Ma (104–12 Ma), which were regarded as xenocrysts and were discarded from further analyses. Taking the weighted mean from the remaining 12 grain ages yields an age of 1.70 ± 0.14 Ma (MSWD = 1.2) (Figure 6C). Since the MSWD of 1.2 is close to unity it implies that the weighted mean age represents the eruption age.
A total of 60 grains were dated for the 990602 sample. Of these, 57 grains passed the initial criteria. Ten grains showed >10 Ma (97–12 Ma), which were regarded as xenocrysts and were discarded from further analyses. Taking the weighted mean from the remaining 47 grain ages yields an age of 4.14 ± 0.71 Ma (MSWD = 54) (Figure 6D). The large MSWD of 54 implies that the weighted mean age does not represent the eruption age and is influenced by older magmatic activities.
A total of 30 grains were dated for the 861031 sample. Of these, 28 grains passed the initial criteria. This sample contained no >10 Ma zircons. Taking the weighted mean from the 28 grain ages yields an age of 4.38 ± 0.12 Ma (MSWD = 1.3) excluding three outliers (Figure 6E). Since the MSWD of 1.3 is close to unity it implies that the weighted mean age represents the eruption age.
4.2 Sanzugawa Formation
A total of 50 grains were dated for the 990603 sample. Of these, 44 grains passed the initial criteria. Thirteen grains showed >10 Ma (149–11 Ma), which were regarded as xenocrysts and were discarded from further analyses. Taking the weighted mean from the remaining 31 grain ages yields an age of 4.08 ± 0.79 Ma (MSWD = 22) (Figure 6F). The large MSWD of 22 implies that the weighted mean age does not represent the eruption age and is influenced by older magmatic activities.
4.3 Innai Formation
A total of 36 grains were dated for the INNAI sample. Of these, 34 grains passed the initial criteria. Seven grains showed >10 Ma (119–42 Ma), which were regarded as xenocrysts and were discarded from further analyses. Taking the weighted mean from the remaining 27 grain ages yields an age of 3.75 ± 0.17 Ma (MSWD = 1.7) excluding three outliers (Figure 6G). Since the MSWD of 1.7 is close to unity it implies that the weighted mean age represents the eruption age. As for this sample, etched (INNAI series in Supplementary Table S2) and unetched (INNAI2) zircons were dated, yielding 3.91 ± 0.23 Ma (n = 12; MSWD = 1.3) and 3.66 ± 0.23 Ma (n = 11; MSWD = 1.4), respectively. They are in agreement within uncertainty, further corroborating that etching does not affect U-Pb dating result (Ito et al., 2017).
5 Discussion
5.1 Assessment of the zircon U-Pb age
5.1.1 Torageyama Formation
According to Wendt and Carl (1991), the MSWD is useful to detect any deviation from a single population. For n grain age data, the calculated weighted mean age has (n - 2) degrees of freedom (f) and the MSWD must be <1 + 2 (2/f)1/2 to be a single population with 95% probability (Wendt and Carl, 1991; Cocherie et al., 2009). The corresponding MSWD is shown as “required MSWD” in Figure 6.
Except for AKI4, 861031 and INNAI, the other samples yield MSWDs far larger than their required MSWDs. Therefore, their weighted mean ages show ages older than the eruption age. As for AY2-100 and AY2-755, individual grain ages increase gradually from ∼1 Ma to ∼6 Ma (Figures 6A, B). The age pattern is similar between AY2-100 and AY2-755, therefore it can be regarded that the Torageyama Formation from 100 m to 755 m in N8-AY-2 wellbore was deposited in a geologically instantaneous event (or events), although different lithofacies such as andesite lavas and siltstones are described in between in the original wellbore description (Ito, 2000a).
AKI4 is another wellbore sample near the N8-AY-2 wellbore, both situated in the western basin of the Sanzugawa caldera (Figure 2). The U-Pb age was obtained as 1.70 ± 0.14 Ma with its MSWD of 1.2 smaller than the required MSWD of 1.9 (Figure 6C). Therefore, the age can be regarded as a single event, most probably the eruption. This U-Pb age of 1.70 ± 0.14 Ma corresponds well with the youngest portions of the two N8-AY-2 wellbore samples (AY2-100 and AY2-755). Therefore, it seems that all the three samples derive from a single eruption event (or geologically instantaneous eruption events) and the eruption occurred at 1.70 ± 0.14 Ma. In order to check the validity of eruption age estimate, weighted mean ages using youngest n grains that pass the “required MSWD” are also calculated (Supplementary Table S3). The weighted mean ages of AY2-100 and AY2-755 are 1.62 ± 0.26 Ma (n = 14; MSWD = 1.8) and 1.42 ± 0.26 Ma (n = 5; MSWD = 1.15). These ages are in agreement with the estimated eruption age of 1.70 ± 0.14 Ma within uncertainty.
The sample 990602 was obtained near the western margin of the Sanzugawa caldera, which is also situated in the western basin that includes the above-mentioned three wellbore samples. The U-Pb age distribution for ages <10 Ma of this sample shows clear bimodal peaks of ∼1.8 Ma and ∼6.5 Ma (Figure 7A). The “Unmix Ages” program implemented in Isoplot 4.15 (Ludwig, 2012) calculates two age peaks of 1.88 ± 0.13 Ma and 6.60 ± 0.14 Ma. The younger peak of 1.88 ± 0.13 Ma is in agreement with the weighted mean age of 1.86 ± 0.18 Ma (n = 19, MSWD = 1.5) that passes the MSWD criteria (Supplementary Table S3). Therefore, it is probable that the caldera-forming volcanic activities initiated at ∼6.6 Ma and ended at 1.9–1.8 Ma in the western part of the Sanzugawa caldera. However, it may be possible that the older age of 6.60 ± 0.14 Ma does not represent an eruption age but an age from intrusive rocks (e.g., rhyolitic to andesitic dykes distributed near the sampling locality). Nevertheless, it is suggested here that this 6.60 ± 0.14 Ma represents a caldera-forming eruption because it occupies as high as a half proportion of <10 Ma zircon (Figure 7A) and the sample 990603 obtained in the eastern part of the Sanzugawa caldera also contains similar zircons with the same age and quantity (Figure 7B). This may mean coeval zircons were produced extensively in the entire Sanzugawa caldera which likely indicates a caldera-forming eruption happened at ∼6.6 Ma. This interpretation can also explain the age distributions from the above-mentioned three wellbore samples. From the four samples, it is inferred that the most recent caldera-forming volcanic activity occurred at 1.70 ± 0.14 Ma in the western basin of the Sanzugawa caldera.
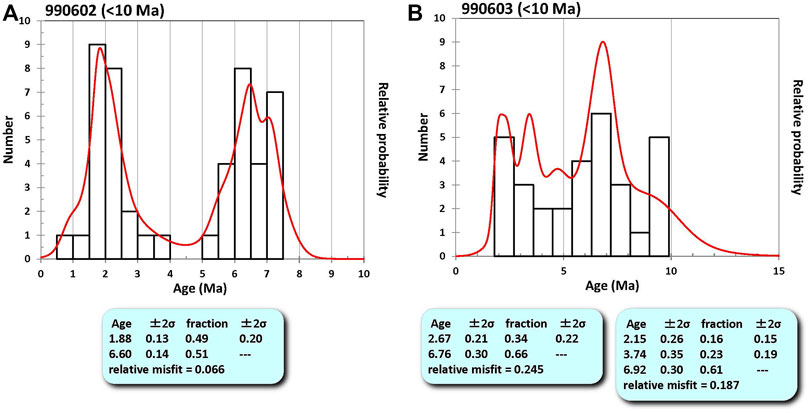
FIGURE 7. 238U-206Pb age distributions (histogram, probability density plot) for zircons younger than 10 Ma. (A) sample 990602, (B) sample 990603. Lower panels show the result of age peak deconvolution using Isoplot 4.15 (Ludwig, 2012).
The sample 861031 was obtained at a central uplifted part of the Sanzugawa caldera, situated ∼500 m higher than the present Sanzugawa caldera floor. The weighted mean U-Pb age of 4.38 ± 0.12 Ma can be regarded as the time of eruption, because the MSWD of 1.3 is smaller than the required MSWD of 1.6 (Figure 6E). This may also indicate a period of resurgence. The sample contains no <2 Ma zircons, which indicates the ∼1.7 Ma eruption that occurred in the western part of the caldera left no volcanic products in this part which may have been already situated at a relatively higher elevation.
5.1.2 Sanzugawa Formation
The U-Pb age distribution for ages <10 Ma of the sample 990603 shows three peaks of ∼2.2 Ma, ∼3.5 Ma and ∼7.0 Ma (Figure 7B). The Unmix Ages program (Ludwig, 2012) calculates two age peaks of 2.67 ± 0.21 Ma and 6.76 ± 0.30 Ma or three age peaks of 2.15 ± 0.26 Ma, 3.74 ± 0.35 Ma and 6.92 ± 0.30 Ma. The ∼7.0 Ma peak is indistinguishable from the ∼6.6 Ma volcanic activity that was confirmed in the western part of the caldera. Therefore, as mentioned above, it is plausible that the entire Sanzugawa caldera initiated volcanic activity at ∼7.0 Ma. It seems difficult to determine whether the younger two peaks reflect two different geologic events or they should be broadly assumed as one peak. If the former is true, the deconvolved two ages (2.15 Ma, 3.74 Ma) may reflect the sedimentation age of the Sanzugawa Formation and an older volcanic activity, respectively. The weighted mean age that passes the MSWD criteria is 2.15 ± 0.25 Ma (n = 5; MSWD = 0.92) (Supplementary Table S3), which seems to support the former assumption because of the agreement of 2.15 Ma. The age of 3.74 ± 0.35 Ma coincides with the age of the Innai caldera formation (discussed below). The sample was obtained in the eastern basin of the Sanzugawa caldera, located close to the Innai caldera (Figure 2). Therefore, a caldera-forming eruption occurred in the eastern part of the Sanzugawa caldera at ∼2.2 Ma, creating a collapsed basin, which deposited lake sediments of the Sanzugawa Formation at ∼2.2 Ma. The collapsed basin may have accumulated volcanic products of ∼7.0 Ma and ∼3.7 Ma derived from the Sanzugawa caldera and adjacent Innnai caldera, respectively.
5.1.3 Innai Formation
The sample INNAI showed a weighted mean age of 3.75 ± 0.17 Ma (Figure 6G). Although the MSWD of 1.7 slightly exceeds the required MSWD of 1.6, it is plausible that the age represents the eruption age that formed the Innai caldera, considering that the weighted mean age of 3.73 ± 0.16 Ma (n = 22; MSWD = 1.6) that passes the MSWD criteria (Supplementary Table S3) is indistinguishable.
5.2 Comparison with other dating methods
The zircon U-Pb ages in this study are compared with some of K-Ar and FT ages in the literature.
Ito (1996, 2000a) dated three wellbore samples (AY2-100, AY2-755 and AKI4) by zircon FT, yielding 1.1 ± 0.4 Ma, 1.5 ± 0.4 Ma, and 1.2 ± 0.4 Ma, respectively. These 1.5–1.1 Ma ages are in accordance with or slightly younger than the U-Pb age of 1.70 ± 0.14 Ma from AKI4 considering uncertainty. Ito (2000a) demonstrated that the FT age of 1.5 ± 0.4 Ma from AY2-755 is reliable even though its present wellbore temperature of ∼240°C matches the FT closure temperature of ∼240°C (Hurford, 1986), considering that the fission track length reduction from the AY2-755 zircon is small. The FT age of 1.3 ± 0.4 Ma (Ito, 1996) from the sample 990602 is also slightly younger than the younger component U-Pb age of 1.88 ± 0.13 Ma. Because of a high closure temperature of >900°C for zircon U-Pb (Lee et al., 1997), the slightly older zircon U-Pb ages than the FT ages may indicate they represent zircon crystallization ages in magma chamber, which are slightly older than the eruption age. Taking the U-Pb and FT ages into account, it seems most plausible that the caldera-forming eruption occurred at ∼1.5 Ma in the western half of the Sanzugawa caldera. This contradicts the whole-rock K-Ar result of Takeno (1988), which reported 6–5 Ma in the western half of the caldera.
The sample 861031 was dated at 4.6 ± 0.6 Ma by zircon FT (Ito, 2000b) and 6.0 ± 1.6 Ma by whole-rock K-Ar (Takeno, 1988). The U-Pb age of 4.38 ± 0.12 Ma is in agreement with the FT age. Although the K-Ar age marginally matches the U-Pb age considering uncertainty, its older age of 6.0 Ma may be influenced by xenolithic materials used for dating.
The sample 990603 from the Sanzugawa Formation was dated at 3.2 ± 0.6 Ma by zircon FT (Ito, 2001) by adopting a pooled age for grains that pass the chi-square (χ2) test at 5% level. The sample also yielded a pooled FT zircon age of 11.6 ± 1.4 Ma using all the grains (Ito, 2001). The zircon U-Pb age from this sample showed two or three age components. If the U-Pb age has two components of 2.67 ± 0.21 Ma and 6.76 ± 0.30 Ma, the FT age of 3.2 ± 0.6 Ma is in accordance with the younger component of the U-Pb age. Therefore, here again, it indicates that the zircon FT method can yield a reliable and robust age with larger uncertainty than U-Pb.
The sample INNAI from the Innai Formation was dated at 3.75 ± 0.17 Ma by zircon U-Pb in this study. This formation was dated by the whole-rock K-Ar method at 3.5 ± 0.4 Ma (Takeno, 1988) and at 3.9 ± 0.6 Ma, 4.0 ± 0.8 Ma, and 4.1 ± 0.6 Ma (Kondo et al., 2004), which are in agreement with the U-Pb dating result.
In summary, unlike whole-rock K-Ar, zircon FT can yield reliable ages for pyroclastic sediments including those affected by present geothermal activity if proper handling is performed (χ2 test, track length measurement etc).
5.3 Magmatic activity in and around the Sanzugawa caldera
From the literature and the U-Pb dating results in this study, caldera-forming volcanic activity in and around the Sanzugawa caldera since the late Miocene is suggested to be as follows (Figure 8).
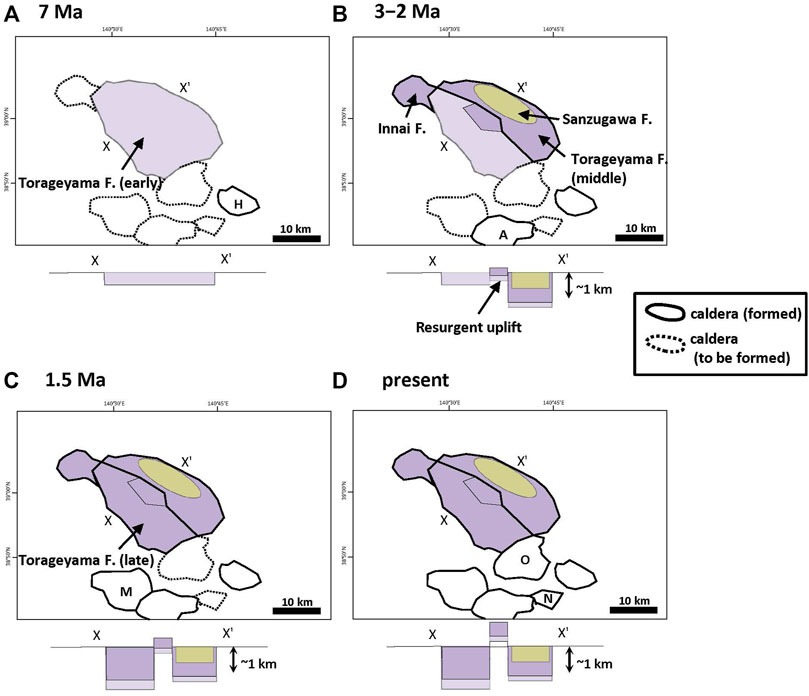
FIGURE 8. Schematics showing evolution of the Sanzugawa and nearby calderas. (A) 7 Ma, (B) 3–2 Ma, (C) 1.5 Ma, (D) present. Note that the evidence of ∼7 Ma caldera is weak. Caldera-fill pyroclastic sediments in the Sanzugawa and Innai calderas are shown in purple. Lake sediments are in yellow. X, X′ show vertical cross-section positions. Abbreviations for calderas are H: Hanayama, A: Akakura, M: Mukaimachi, O: Onikobe, N: Naruko.
The Hanayama caldera, the first caldera in this region since the Miocene, was formed at ∼9 Ma based on a zircon FT age of 9.2 ± 1.4 Ma using dacite breccia (Tsuchiya et al., 1997). At ∼7 Ma, the first stage of a caldera-forming eruption may have occurred at the Sanzugawa caldera (Figure 8A). Because ∼7 Ma zircons are abundant both in the west (sample 990602) and east (990603) of the caldera, I assume that the area of the preceding caldera was as large as the one seen today. However, this assumption is weak because no ignimbrite of this age determined by U-Pb was confirmed. Sporadic volcanic activities producing dacite lapilli tuffs ensued at 5–4 Ma at the latest in the central part of the caldera (evidenced by sample 861031), which may promote a resurgent uplift for this period. At ∼4 Ma, Innai caldera, to the northwest of the Sanzugawa caldera, was formed. At 3–2 Ma, another large caldera-forming eruption occurred in the eastern part of the Sanzugawa caldera, which caused the thick (>500 m) lacustrine sedimentation of the Sanzugawa Formation. From the distribution of the Sanzugawa Formation (Takeno, 1988; Ito et al., 1989), I assume the size of the 3–2 Ma caldera was >100 km2. The Akakura caldera to the south of the Sanzugawa caldera was also formed at this period (Otake, 2000) (Figure 8B). At ∼1.5 Ma, another large caldera-forming eruption occurred in the western part of the Sanzugawa caldera evidenced by large amounts of ∼2–1 Ma zircons there (samples: AY2-100, AY2-755, AKI4, 990602) (Figure 8C). In the N8-AY-2 wellbore, the Torageyama Formation is present from ∼50 m to ∼1140 m in depth, and a considerable portion of the wellbore (from <100 m to >755 m in depth) is comprised of ∼1.5 Ma eruption products. Therefore, I assume the ∼1.5 Ma eruption was voluminous and the ∼1.5 Ma caldera was equivalent to or larger than the 3–2 Ma caldera formed in the eastern part of the Sanzugawa caldera. The Mukaimachi caldera west of the Akakura caldera was also formed by this time (Otake, 2007). Since then, resurgent (or post-caldera) volcanism and uplifting probably accelerated in the central part of the Sanzugawa caldera, creating Quaternary volcanoes there, and the Onikobe and the Naruko calderas were formed in succession to the southeast of the Sanzugawa caldera (Tsuchiya et al., 1997) (Figure 8D). In summary, at least three, early (∼7 Ma), middle (5–2 Ma), and late (∼1.5 Ma) caldera-forming volcanic activities are thought to have occurred in the Sanzugawa caldera with the western part of the caldera being formed in the Quaternary (∼1.5 Ma).
5.4 Implications of the Tohoku caldera-forming volcanic activity
The western part of the Sanzugawa caldera was assumed to have been formed at 6–5 Ma based on the whole-rock K-Ar (Takeno, 1988), whereas zircon U-Pb dating in this study as well as zircon FT dating (Ito, 2000a) demonstrate that it was mainly formed at ∼1.5 Ma. In the Tohoku region, it is assumed that a migration of volcanism of ∼2 cm/yr occurred from the back arc to the arc side since ∼6 Ma (Honda and Yoshida, 2005). Repeated caldera-forming volcanic activities since ∼7 Ma to the present in and around the Sanzugawa caldera (Figure 8) may contradict this notion because no apparent migration occurred there. Moreover, a volcanic lull at 3.5–1.5 Ma in NE Japan was proposed (Kimura et al., 2015; Mahony et al., 2016). This also seems unacceptable because a volcanic “flare-up” occurred at 3–1.5 Ma in and around the Sanzugawa caldera. Yoshida et al. (2014) estimated the volume of volcanic products at 4–2 Ma in the Tohoku region to be ∼360 km3 per 200 km of length of the arc. Assuming the size of the Sanzugawa caldera formed at 4–2 Ma to be ∼200 km2 and the caldera collapse of 1 km, the volume is calculated to be ∼200 km3 for the Sanzugawa caldera alone. The volume of 4–2 Ma volcanic products should have been much larger considering volcanic products from the nearby Innai, Akakura and Mukaimachi calderas. The Innai and Akakura calderas are Valles-type (piston-type) calderas (Aizawa and Yoshida, 1999; Otake, 2000), as the Sanzugawa caldera. Assuming that the Mukaimachi caldera is also a piston-type caldera and these three calderas collapsed >1 km, considering their combined size of >200 km2 (Figures 2, 8), the total volume exceeds >200 km3. Therefore, the entire volcanic products in the Tohoku region at 4–2 Ma should have been much larger than those estimated by Yoshida et al. (2014). These discrepancies probably arise because some of the K-Ar data in the literature are dubious, as they tend to yield much older ages than eruption due to incorporation of xenocrystic materials (e.g., Gardner et al., 2002). Therefore, it is highly required to date other calderas in the Tohoku region with zircon U-Pb and/or Ar-Ar to better understand magmatic history of this region.
6 Conclusion
Zircon U-Pb dating was applied to the Sanzugawa and Innai calderas in the Tohoku region, NE Japan, where the geochronological framework was mostly based on whole-rock K-Ar dating results. U-Pb dating was performed mainly on etched zircons with low spontaneous fission track density in order to select grains that were assumed to represent the eruption age. In summary, six pyroclastic sediments from the Sanzugawa caldera and one from the Innai caldera, were dated and compared with previous K-Ar and fission track dating results. The main conclusions are as follows
(1) The Sanzugawa caldera may have initiated its caldera-forming activity at ∼7 Ma. Caldera-forming eruptions ensued in the eastern part of the caldera at 3–2 Ma, and in the western part at ∼1.5 Ma.
(2) Intensive caldera-forming eruptions occurred in and around the Sanzugawa caldera at 3.0–1.5 Ma, which may contradict the notion that volcanism was subdued at 3.5–1.5 Ma in NE Japan.
(3) Further zircon U-Pb dating and/or Ar-Ar dating studies are required to better understand magmatism in the Tohoku region.
Data availability statement
The original contributions presented in the study are included in the article/Supplementary Material, further inquiries can be directed to the corresponding author.
Author contributions
HI performed samplings, experiments and wrote the manuscript.
Funding
This work has been carried out under in-house research funding of Central Research Institute of Electric Power Industry.
Acknowledgments
I thank M. Yukawa for her help with sample preparation and LA-ICP-MS data collection, and Y. Adachi for her technical assistance on LA-ICP-MS. Sample 861031 was provided by N. Takeno. U-Pb ages were calculated using an Excel spreadsheet provided by S. Sakata. Comments by three reviewers were helpful to improve the manuscript. English assistance was provided by R. Marsden.
Conflict of interest
The author declares that the research was conducted in the absence of any commercial or financial relationships that could be construed as a potential conflict of interest.
Publisher’s note
All claims expressed in this article are solely those of the authors and do not necessarily represent those of their affiliated organizations, or those of the publisher, the editors and the reviewers. Any product that may be evaluated in this article, or claim that may be made by its manufacturer, is not guaranteed or endorsed by the publisher.
Supplementary material
The Supplementary Material for this article can be found online at: https://www.frontiersin.org/articles/10.3389/feart.2022.964773/full#supplementary-material
References
Acocella, V., Yoshida, T., Yamada, R., and Funiciello, F. (2008). Structural control on late Miocene to Quaternary volcanism in the NE Honshu arc, Japan. Tectonics 27, TC5008. doi:10.1029/2008TC002296
Aizawa, K., and Yoshida, T. (1999). Innai Caldera Complex composed of Valles type caldera and Glen-coe type caldera. Abstracts 1999 Japan Earth and Planetary Science Joint Meeting, Vc-P002.
Cocherie, A., Fanning, C. M., Jezequel, P., and Robert, M. (2009). LA-MC-ICPMS and SHRIMP U–Pb dating of complex zircons from Quaternary tephras from the French Massif Central: Magma residence time and geochemical implications. Geochim. Cosmochim. Acta 73, 1095–1108. doi:10.1016/j.gca.2008.11.028
Crowley, J. L., Schoene, B., and Bowring, S. A. (2007). U-Pb dating of zircon in the Bishop Tuff at the millennial scale. Geology 35, 1123–1126. doi:10.1130/g24017a.1
Fujioka, K. (1983). History of the explosive volcanism of the Tohoku arc from core sediment samples of the Japan Trench. Bull. Volcanol. Soc. Jpn. 28, 41–58.
Fujioka, K. (1985). “Synthesis of Neogene explosive volcanism of the Tohoku arc, deduced from the marine tephra drilled around the Japan trench region, DSDP, legs 56, 57 and 87B,” in Initial reports of the deep-sea drilling project. Editors H. Kagami, D. E. Karig, and W. T. Coulbourn (Washington: US Government Printing Office), 87, 703–723. http://deepseadrilling.org/87/volume/dsdp87_19.pdf.
Gardner, J. E., Layer, P. W., and Rutherford, M. J. (2002). Phenocrysts versus xenocrysts in the youngest Toba Tuff: Implications for the petrogenesis of 2, 800 km3 of magma. Geology 30, 347–350. doi:10.1130/0091-7613(2002)030<0347:pvxity>2.0.co;2
Hiess, J., Condon, D. J., McLean, N., and Noble, S. R. (2012). 238U/235U systematics in terrestrial U-bearing minerals. Science 335, 1610–1614. doi:10.1126/science.1215507
Honda, S., and Yoshida, T. (2005). Application of the model of small-scale convection under the island arc to the NE Honshu subduction zone. Geochem. Geophys. Geosyst. 6, Q01002. doi:10.1029/2004GC000785
Hurford, A. J. (1986). Cooling and uplift patterns in the Lepontine Alps South Central Switzerland and an age of vertical movement on the Insubric fault line. Contrib. Mineral. Petrol. 92, 413–427. doi:10.1007/bf00374424
Ito, H. (1996). Application of the fission-track dating method to evaluate the thermal history of three geothermal areas: North Kurikoma (Japan), Hijiori (Japan) and Valles caldera (USA). Kyoto, Japan: Ph. D. thesis, Kyoto Univ. Avaialable at: http://repository.kulib.kyoto-u.ac.jp/dspace/bitstream/2433/160910/2/D_Ito_Hisatoshi.pdf.
Ito, H. (2000a). Estimation of the sedimentation age of the Torageyama Formation, Akinomiya geothermal area, Akita prefecture, by the fission-track dating method. J. Geotherm. Res. Soc. Jpn. 22, 9–21. doi:10.11367/grsj1979.22.9
Ito, H. (2001). Fission-track ages on some pyroclastic rocks associated with the formation of Ogachi caldera (southern part of Akita Prefecture). Fission-Track Newslett 14, 15–18. Avaialable at: http://www.ftrgj.org/FTNLs/FTNLparts/ito01.pdf.
Ito, H. (2020). Magmatic history of the Oldest Toba Tuff inferred from zircon U–Pb geochronology. Sci. Rep. 10, 17506. doi:10.1038/s41598-020-74512-z
Ito, H., Spencer, C. J., Danišík, M., and Hoiland, C. W. (2017). Magmatic tempo of Earth’s youngest exposed plutons as revealed by detrital zircon U-Pb geochronology. Sci. Rep. 7, 12457. doi:10.1038/s41598-017-12790-w
Ito, H. (2000b). Study of the influence of the accidental grains in pyroclastic rocks on the fission-track dating result. Cent. Res. Inst. Electr. Power Ind. U99073, 16p. Avaialable at: https://criepi.denken.or.jp/hokokusho/pb/reportDetail?reportNoUkCode=U99073.
Ito, T., Utada, M., and Okuyama, T. (1989). Mio-Pliocene calderas in the Backbone region in northeast Japan. Mem. Geol. Soc. Jpn. 32, 409–429.
Kimura, J.-I., Nagahashi, Y., Satoguchi, Y., and Chang, Q. (2015). Origins of felsic magmas in Japanese subduction zone: Geochemical characterizations of tephra from caldera-forming eruptions <5 Ma. Geochem. Geophys. Geosyst. 16, 2147–2174. doi:10.1002/2015GC005854
Kondo, H., Kaneko, K., and Tanaka, K. (1998). Characterization of spatial and temporal distribution of volcanoes since 14 Ma in the Northeast Japan arc. Bull. Volcanol. Soc. Jpn. 43, 173–180.
Kondo, H., Tanaka, K., Mizuochi, Y., and Ninomiya, A. (2004). Long-term changes in distribution and chemistry of middle Miocene to Quaternary volcanism in the Chokai-Kurikoma area across the Northeast Japan Arc. Isl. Arc 13, 18–46. doi:10.1111/j.1440-1738.2003.00417.x
Lee, J. K. W., Williams, I. S., and Ellis, D. J. (1997). Pb, U and Th diffusion in natural zircon. Nature 390, 159–162. doi:10.1038/36554
Ludwig, K. R. (2012). User’s manual for Isoplot 3.75: A geochronological toolkit for Microsoft Excel. Berkeley Geochronol. Center Spec. Pub. 5, 75p.
Mahony, S. H., Sparks, R. S. J., Wallace, L. M., Engwell, S. L., Scourse, E. M., Barnard, N. H., et al. (2016). Increased rates of large-magnitude explosive eruptions in Japan in the late Neogene and Quaternary. Geochem. Geophys. Geosyst. 17, 2467–2479. doi:10.1002/2016GC006362
Mark, D. F., Petraglia, M., Smith, V. C., Morgan, L. E., Barfod, D. N., Ellis, B. S., et al. (2014). A high-precision 40Ar/39Ar age for the Young Toba Tuff and dating of ultra-distal tephra: Forcing of Quaternary climate and implications for hominin occupation of India. Quat. Geochronol. 21, 90–103. doi:10.1016/j.quageo.2012.12.004
Mark, D. F., Renne, P. R., Dymock, R. C., Smith, V. C., Simon, J. I., Morgan, L. E., et al. (2017). High-precision 40Ar/39Ar dating of pleistocene tuffs and temporal anchoring of the Matuyama-Brunhes boundary. Quat. Geochronol. 39, 1–23. doi:10.1016/j.quageo.2017.01.002
Milicich, S. D., Chamberfort, I., Wilson, C. J. N., Alcaraz, S., Ireland, T. R., Bardsley, C., et al. (2020). A zircon U-Pb geochronology for the Rotokawa geothermal system, New Zealand, with implications for Taupō Volcanic Zone evolution. J. Volcanol. Geotherm. Res. 389, 106729. doi:10.1016/j.jvolgeores.2019.106729
Milicich, S. D., Wilson, C. J. N., Bignall, G., Pezaro, B., Charlier, B. L. A., Wooden, J. L., et al. (2013). U–Pb dating of zircon in hydrothermally altered rocks of the Kawerau geothermal field, Taupo volcanic zone, New Zealand. J. Volcanol. Geotherm. Res. 253, 97–113. doi:10.1016/j.jvolgeores.2012.12.016
Nunohara, K., Okano, H., Yamada, R., Hirano, N., and Tsuchiya, N. (2021). Geothermal potential in southern part of the Sanzugawa caldera, Akita prefecture, northeast Japan. J. Geotherm. Res. Soc. Jpn. 43, 65–78.
Oki, F. (2016). Eruptive history and structural development of Quaternary Sanzugawa caldera, Yuzawa, Akita. Abstracts 2016 Japan Geoscience Union Meeting. SVC48-P06.
Otake, M. (2007). Sedimentary facies, processes and environments of the Akakura caldera lake, the South Kurikoma geothermal area, northeast Japan. J. Geol. Soc. Jpn. 113, 549–564. doi:10.5575/geosoc.113.549
Otake, M. (2000). Stratigraphy and styles of caldera-forming eruption and subsidence of the Akakura caldera in the South Kurikoma geothermal area, Northeast Japan. J. Geol. Soc. Jpn. 106, 205–222. doi:10.5575/geosoc.106.205
Sakata, S. (2018). A practical method for calculating the U-Pb age of Quaternary zircon: Correction for common Pb and initial disequilibria. Geochem. J. 52, 281–286. doi:10.2343/geochemj.2.0508
Schmitz, M. D., and Bowring, S. A. (2001). U-Pb zircon and titanite systematics of the Fish Canyon Tuff: An assessment of high-precision U-Pb geochronology and its application to young volcanic rocks. Geochim. Cosmochim. Acta 65, 2571–2587. doi:10.1016/s0016-7037(01)00616-0
Sláma, J., Košler, J., Condon, D. J., Crowley, J. L., Gerdes, A., Hanchar, J. M., et al. (2008). Plešovice zircon — a new natural reference material for U–Pb and Hf isotopic microanalysis. Chem. Geol. 249, 1–35. doi:10.1016/j.chemgeo.2007.11.005
Stacey, J. S., and Kramers, J. D. (1975). Approximation of terrestrial lead isotope evolution by a two-stage model. Earth. Planet. Sci. Lett. 26, 207–221. doi:10.1016/0012-821x(75)90088-6
Takashima, R., Kusakawa, H., Kuwabara, S., Orihashi, Y., Nishi, H., Niwano, M., et al. (2020). Identification of the source caldera for a Pliocene ash-flow tuff in Northeast Japan based on apatite trace-element compositions and zircon U-Pb ages. J. Volcanol. Geotherm. Res. 401, 106948. doi:10.1016/j.jvolgeores.2020.106948
Takeno, N. (1988). Geology of the North Kurikoma geothermal area, Akita Prefecture, Northeast Japan. Rept. Geol. Surv. Jpn. 268, 191–210.
Tsuchiya, N., Itoh, J., Seki, Y., and Iwaya, T. (1997). Geology of the Iwagasaki district. Quadrangle series, 1:50,000, Geological Survey of Japan, 96p.
Wendt, I., and Carl, C. (1991). The statistical distribution of the mean squared weighted deviation. Chem. Geol. Isot. Geosci. Sect.) 86, 275–285. doi:10.1016/0168-9622(91)90010-t
Wiedenbeck, M., Hanchar, J. M., Peck, W. H., Sylvester, P., Valley, J., Whitehouse, M., et al. (2004). Further characterisation of the 91500 zircon crystal. Geostand. Geoanalytical Res. 28, 9–39. doi:10.1111/j.1751-908x.2004.tb01041.x
Yamada, E. (1988). Geologic development of the Onikobe caldera, Northeast Japan, with special reference to its hydrothermal system. Rept. Geol. Surv. Jpn. 268, 61–190.
Yamamoto, T. (1992). Chronology of the Late Miocene–Pleistocene caldera volcanoes in the Aizu district, Northeast Japan. J. Geol. Soc. Jpn. 98, 21–38. doi:10.5575/geosoc.98.21
Yamamoto, T. (2009). Sedimentary processes caused by felsic caldera-forming volcanism in the Late Miocene to Early Pliocene intra-arc Aizu basin, NE Japan arc. Sediment. Geol. 220, 337–348. doi:10.1016/j.sedgeo.2009.04.021
Yoshida, T., Aizawa, K., Nagahashi, Y., Sato, H., Ohguchi, T., Kimura, J., et al. (1999). Geological history of Late Cenozoic island arc volcanism in Northeast Honshu arc and the formation of caldera swarm (in Japanese). Earth Monogr. 27, 123–129.
Yoshida, T., Kimura, J., Yamada, R., Acocella, V., Sato, H., Zhao, D., et al. (2014). Evolution of late Cenozoic magmatism and the crust-mantle structure in the NE Japan Arc. Geol. Soc. Spec. Publ. 385, 335–387. doi:10.1144/sp385.15
Keywords: U-Pb dating, caldera-forming eruption, zircon, Sanzugawa caldera, Tohoku
Citation: Ito H (2023) Quaternary caldera-forming eruptions at the Sanzugawa caldera, NE Japan, revealed by zircon U-Pb geochronology. Front. Earth Sci. 10:964773. doi: 10.3389/feart.2022.964773
Received: 09 June 2022; Accepted: 16 December 2022;
Published: 06 January 2023.
Edited by:
Derek Keir, University of Southampton, United KingdomReviewed by:
Dawid Szymanowski, ETH Zürich, SwitzerlandKata Molnar, Institute for Nuclear Research (MTA), Hungary
Copyright © 2023 Ito. This is an open-access article distributed under the terms of the Creative Commons Attribution License (CC BY). The use, distribution or reproduction in other forums is permitted, provided the original author(s) and the copyright owner(s) are credited and that the original publication in this journal is cited, in accordance with accepted academic practice. No use, distribution or reproduction is permitted which does not comply with these terms.
*Correspondence: Hisatoshi Ito, ito_hisa@criepi.denken.or.jp