- 1Sichuan Huadi Construction Engineering Co., Ltd., Chengdu, Sichuan, China
- 2Chengdu Center of Hydrogeology and Engineering Geology Sichuan Burean of Geology and Mineral Exploration and Development, Chengdu, Sichuan, China
- 3Sichuan Institute of Geological Disaster Prevention and Control Engineering Technology, Chengdu, Sichuan, China
- 4Sichuan Communication Surveying and Design Institute, Chengdu, Sichuan, China
- 5School of Emergency Management, Xihua University, Chengdu, Sichuan, China
- 6State Key Laboratory of Geohazard Prevention and Geoenvironment Protection, Chengdu University of Technology, Chengdu, Sichuan, China
- 7Key Laboratory of Geohazard Prevention of Hilly Mountains, Ministry of Natural Resources, Fuzhou, Fujian, China
- 8College of Architecture and Environment, Sichuan University, Chengdu, Sichuan, China
- 9Research Institute No. 280, CNNC, Guanghan, Sichuan, China
Slope cutting is becoming more common in engineering construction to obtain a large floor area. Slope cutting disrupts a slope’s inherent stability, causing instability and sliding. To solve the problem of geological disasters caused by artificial slope cutting, Cangxi County is used as an example to analyze the cutting slope and the height of different slope structures in the county, to study the disaster mode and disaster factors caused by slope cutting, and to analyze the influence of slope cutting height and slope cutting gradient on the disaster process. This study demonstrates that the stability of cutting on different types of slopes is weakened to different degrees, with mixed rocky-soil slopes having the strongest resistance to weakening, thick-soil slopes the second strongest, and thin-soil slopes the weakest. Slope cutting is the main factor of disaster, and rainwater infiltration promotes landslide formation. Numerical simulation is employed to determine the proximities of the slope cutting height and slope cutting gradient, and 75% of the critical value is taken as the proposed threshold value of slope cutting. The recommended cut slope threshold value corresponds to the real stable cut slope gradient on site, and the research findings can be used to guide engineering cut slope decisions in Cangxi County and other red-layer areas.
Introduction
Slope cutting excavation on slopes is becoming more common for acquiring a large floor area during construction, and high and steep artificial slope cutting has created safety hazards for landslides and geological disasters (Gao et al., 2022). The horizontal displacement of the cut slope is larger than the vertical displacement, deformation gradually increases with the gradation of the cut slope, and the deformation area of the slope is primarily concentrated near the cut slope surface, according to a study of the evolution of disaster-causing slope cutting on red-layer slopes (Shao et al., 2022). Thus, the height and gradient of the cut slope and slope structure become the key factors that trigger geological hazards, and it is especially important to limit the height and gradient of the cut slope according to the characteristics of a slope structure.
With the rapid development of the engineering construction industry, the use of engineering slope cutting is increasing, which leads to the frequent occurrence of geological hazards. Applying single prevention and control measures to the complex and changeable engineering geological hazards is difficult. Nevertheless, quantitative analysis, which is a good method (Li et al., 2021a a/b; Li, 2022a), is extensively employed to determine the dangers of landslides. The risk prediction value is used to investigate the preventive and control methods for landslides (Strouth and McDougall, 2021). The safety factor of slopes is controlled by numerous factors (Cui et al., 2021; Li, 2022), and it has been found that the friction angle φ has the greatest influence on the safety factor of slopes, followed by the horizontal seismic coefficient Kh, cohesion c, and saturation permeability ks (Liu et al., 2021). Zhang and Wang (2020) found that the critical conditions for the propagation of catastrophic shear zones on engineering slopes are related to the gravitational shear stress ratio of the slope, which is mainly controlled by the slope toe. Panthee (2016) investigated the relationship between the slope safety factor and the engineering cut slope’s height and gradient and found that reducing the engineering cut slope’s gradient significantly enhanced the slope safety factor and stability (He et al., 2014). When dampened by water, loess produces a bigger sink, and the stability of a loess slope becomes more susceptible to water than other soil. The erosion of loess slopes is influenced by three elements, namely, slope material, slope structure, and slope hydrology (Li et al., 2020). The consolidation deformation of ultra-high fill slopes of wet sinking loess is the main factor causing its deformation (Mei et al., 2022). The intrinsic cause of landslides induced by loess cut slopes is the structural characteristics of small pores in loess (Cao et al., 2016). The instability volume of a loess slope is affected by slope and height; the larger the slope and height, the larger the instability volume (Zhang et al., 2021). The three-dimensional properties of slopes are critical determinants of slope stability, rainfall infiltration is one of the main causal factors of slope damage, and rainfall patterns regulate slope damage patterns, according to a study by Xu et al. (2021). On slopes with very high soil erosion rates, a vegetation cover protects the soil from rainfall and enhances soil stability (Solgi et al., 2021). After the slope cutting, the rock is exposed, and the strength of the rock decreases with time under weathering, according to Awang et al. (2021). Research has demonstrated that the stability of soil agglomerates is important in the resistance to soil erosion of engineering graben slopes in geologically hazardous areas, thus indicating that rock strength and soil erosion resistance are important factors in determining slope stability (Ai et al., 2021; Zhou et al., 2021). Postill et al. (2021) investigated the effect of supervoid water pressure formed after slope excavation on slope stability. Disaster-induced slope cutting damages the stable state of the slope and gives favorable spatial geometric conditions for disaster occurrence; rainfall infiltration decreases soil strength and also promotes disaster occurrence (Wang et al., 2021). Consequently, it can be understood that after slope cutting, rainfall becomes the primary factor of disaster occurrence. The OP-ELM algorithm is used to predict rainfall and build a deep belief network for predicting future displacements to provide a theoretical basis for landslide disaster prevention and control (Li et al., 2021b). Rock bridges on the potential sliding surface control the stability of rocky slopes (Tang et al., 2020), and the shear capacity decreases with increasing dispersion/number of bridges, which is caused by numerous bridges fracturing individually (Tang et al., 2021). The stability of slopes is controlled by key blocks (Qin et al., 2020), and the slope toe as a key block of slope stability plays a vital role in maintaining slope stability.
The existing study on disaster-causing factors on a cut slope is dominated by disaster processes in specific areas, analyzes the influence of external factors on slope stability, and lacks research on disaster-causing factor threshold values. Therefore, this research considered slope cutting gradient and slope cutting height as disaster-causing factors to study the geological hazards induced by engineering slope cutting. The cut slope height and cut slope gradient of disasters that have and have not occurred were compared and assessed; in addition, numerical simulation was employed to estimate the cut slope height and gradient limit threshold. The numerical simulation results were discounted considering the slope safety reserve, and a set of slope cutting height and slope cutting gradient limits were suggested for different slope types in the red-layer area.
Overview of the study area
Cangxi County is located 80 km south of Guangyuan City. It is a red-layer area. Its northern part is primarily a medium-low mountainous area, its central part is a primarily low mountainous area on the east and west sides, and its southern part is a primarily deep-hill area and flat dam area. Slope cutting is a typical practice in Cangxi County to expand the building area. It primarily involves soil slopes and soil-rock mixed slopes, with a few pure rocky slope cuttings with significant heights and steep slopes.
Slope cutting characteristics
Distribution characteristics
A total of 108 slope cutting spots exist in Cangxi County, which are spread among 23 townships. According to the distribution characteristics, engineering slope cutting in Cangxi County is primarily concentrated in the county’s urban areas and towns, with a relatively limited distribution in the countryside. The following three factors contribute to this distribution characteristic: the flexible site selection for construction in the countryside, which makes it possible to build on a flat and open land to avoid slope cutting; the high cost and low cost-effectiveness of slope cutting for widening floor area; and the dense construction of houses in urban areas and towns in the county, poor choice of building sites, and high necessity of slope cutting. The main traffic hubs exist in the county city and town, traffic lines are intertwined, line layout option is poor, and the road in the process of construction of the slope excavation necessity is high. Figure 1 presents the distribution characteristics of engineering cut slopes in Cangxi County.
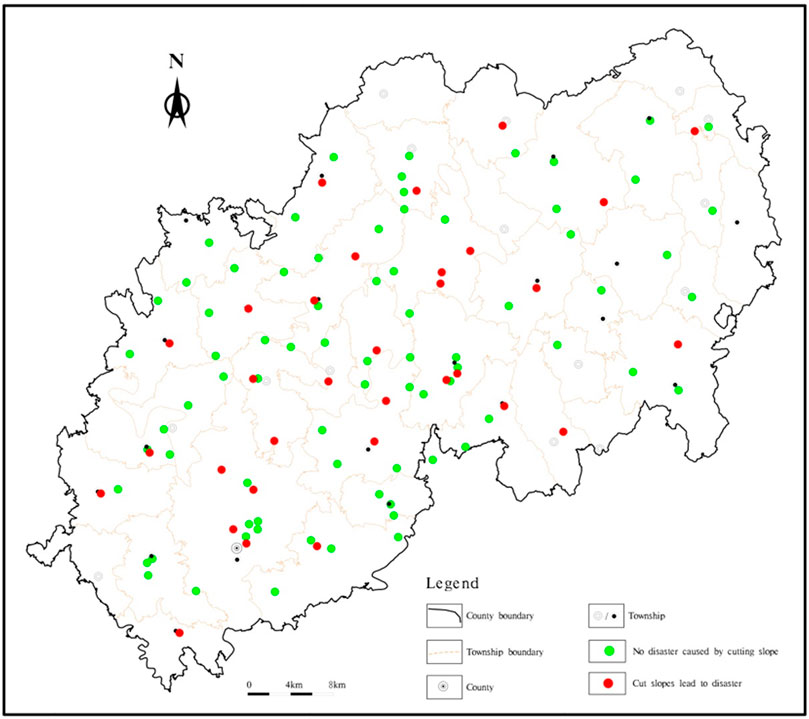
FIGURE 1. Cangxi County engineering cut slope distribution map. Note: The red points in the map are the points where slope cutting has caused disaster, and the green points are the points where slope cutting has not caused disaster.
The distribution characteristics of different structural types of slopes within Cangxi County are controlled by topography and geomorphology. The slopes in the medium-low mountainous landscape area have large slopes, and it is difficult for the overburden layer to attach to the surface of the slope in large quantities, and the slope types in the medium-low mountainous landscape area are mainly thin-soil layer slopes. The slope gradient in the deep mound landform area is small, and the overburden layer can be attached to the slope surface in a large amount. The slope types in the deep mound landform area are mainly rock-soil mixed slopes and thick-soil layer slopes.
Slope cutting height and slope cutting gradient characteristics
The cutting slopes in the studied area range from 4 to 20 m in height, with most of them falling between 5 and 8 m, with 78 cut slopes in this height range, accounting for 72.2% of the total, which is consistent with the region’s general topographic slope. Figure 2 presents the cutting slope height statistics. To reduce slope cutting excavation, residents mostly adopt near-vertical excavation when cutting slopes, resulting in a large cut slope ratio, and the slope cutting ratio of rocky slopes is more than 1:0.3. Most of the cut soil slopes are not protected, and the cut rock slopes are close to 90°; a layer of concrete mortar is sprayed on the cut slopes to prevent the slopes from weathering and spalling, as shown in Figure 3.
The mudstone and sandstone that make up Cangxi County’s rocky slopes are prone to differential weathering and spalling, and a study found that differential weathering is the primary cause of slope instability (Admassu and Shakoor, 2015). In the research region, cut rocky slopes range from 60° to 80°, with some of the cut slopes approaching 90°. The aim of engineering slope cutting is mainly to widen the construction area; thus, the location of slope cutting is close to the residents’ houses, which poses a great threat to the residents’ lives and properties when disasters occur.
Typical cut slope hazards
Cut slope disaster characteristics
The first is the thin-soil slope, with a soil layer thickness of 1–5 m; the upper layer of the slope is powder clay, whereas the lower layer is sandstone or mudstone. The second one is the thick-soil slope, with a soil layer thickness of 5–15 m. The third is a thick-soil slope, with a soil layer thickness of 5–15 m.
As presented in Figure 4A, the landslide behind Li Dekai’s house is a typical slope with a thin-soil layer. The soil layer of the slope surface is powder clay, and the thickness of the soil layer at the slope toe is much larger than that at the top of the slope due to slope cutting. The landslide body has an average thickness of 3.5 m, and the front edge shear outlet is in the drainage ditch behind the house, 1 m away from the house’s back wall, with a depth of 40–80 cm under the improper rear edge. The slope cutting gradient height is 7.7 m, and the cutting slope of 40°. When the vegetation on the slope surface and the slope toe are removed, the slope surface cover soil loses the support of the slope toe, there is a downward trend, and rainwater infiltration reduces the strength of the soil body, increases the self-weight of the soil body, and aggravates the soil layer decline.
As presented in Figure 4B, the landslide behind Yang Julan’s house is a typical rock-soil mixed landslide. The upper layer of the slope is an interlayer structure of powder clay and sandstone, the thickness of the soil layer is 25–45 cm, the thickness of the rock layer is 40–60 cm, the dip angle of the rock layer is 8°, and the tendency is consistent with the slope direction. The cut slope dip angle is 75°, and the cut slope height is 8.2 m. The cut slope breaks the slope’s equilibrium condition and creates a high and steep cut slope surface, which significantly reduces the slope’s stability. Rainwater infiltration along the cut slope surface to the inside of the slope increases the self-weight and reduces the strength of the soil body, loses the support of the toe of the surface layer of soil first destabilization slide, drives the lower broken rock layer together with the slide, and lastly forms the overall destabilization slide.
As presented in Figure 4C, the landslide behind Zhang Dachun’s house is a typical thick-soil layer landslide. The soil layer is powder clay and is 7–12 m thick, the slope cutting height is 6.5 m, and the slope cutting gradient is 50°. The rainwater infiltrates into the soil layer along the cut slope surface and reduces the strength of the soil near the cut slope surface, resulting in the destabilization and sliding of the surface soil of the slope after experiencing frequent rainfall. Currently, the slope has received management measures to repair and reinforce the slope toe with dry-laid blocks and to release the slope at multiple levels, with a slope release of 20° and a slope release height of 1.5 m. The slope surface is compacted to prevent it from collapsing locally and thereby causing the overall slide.
The landslide volume of thin-soil slopes and rocky-soil mixed slopes is comparatively small, and that of thick-soil slopes is larger. The cut slope height and gradient of the three typical cut slope hazard points all surpass the safety value, which are originally used as the upper limit of cut slope for these three slope types.
Disaster pattern
The disaster formation mode of the thin-soil slope is presented in Figure 5A. The slope surface cover loses the toe support after the slope cutting, as well as the cover sliding along the underlying bedrock surface. The formation of the thick-soil slope is presented in Figure 5B. After the slope cutting, the cover layer of the slope surface loses part of the support of the slope toe, and the cover layer of the hollow part of the slope toe slides downward, causing the slope to become locally unstable. Figure 5C presents the rocky-soil mixed slope disaster formation. After the slope cutting, the slope surface rocky-soil body loses the support of the slope toe; first, the slope surface soil slides; the soil sliding process then carries the lower broken rock sliding; finally, the entire unstable slide is formed.
Numerical simulation analysis
Modeling and parameter values
The Mohr–Coulomb theory was chosen as the computational model, which is generally used to describe shear damage to soils and rocks. The damage envelope of the model matches the Mohr–Coulomb strength criterion and the tensile damage criterion.
The strength reduction method is employed to study the stability of slopes under natural and storm conditions after slope cutting. In the calculation model of the thin-soil slope, group 1 is the cut slope excavation area; group 2, the powder clay layer; and group 3, the bedrock, as shown in Figure 6A. In the calculation model of the slope with mixed rocky-soil structure, groups 1, 2, 3, and 4 are cut slope excavation areas; groups 1, 3, 5, and 7 are pulverized clay layers; groups 2 and 6 are mudstones; and groups 4 and 8 are bedrock (Figure 6B). In the computational model of the thick-soil slope, group 1 is the cut slope excavation area; group 2, the powder clay; and group 3, the bedrock (Figure 6C). The X, Y, and Z directions of the model are presented in Figure 6.
The deformation of the slope along the horizontal direction of the cut slope prograde is mostly indicated by the X direction displacement. The deformation characteristics of the slope body in the horizontal direction can be used to identify the deformation characteristics of the slope body to the prograde, and the displacement vector can reflect the body’s deformation direction. When the displacement in the Z direction is positive, the slope is displaced upward, and when the displacement in the Z direction is negative, the slope is deformed by settlement.
As presented in Table 1, the geotechnical parameters were obtained via in situ drilling and sampling (soil and rock samples) and via indoor tests. Water-filled treatment of soil and rock samples is used to measure the geotechnical parameters during storm conditions. The geotechnical parameters are used in numerical simulations.
Analysis of simulation results
After the slope cutting, the substantial deformed part of the slope is situated at the prograde of the cut slope. The maximum X displacement of the thin-soil layer slope (natural working condition) is 3.02 m, the maximum X displacement of the thick-soil layer slope (natural working condition) is 2.74 m, and the maximum X displacement of the rocky-soil mixed slope (natural working condition) is 2.24 m. The plastic deformation zone of all types of slopes is situated at the slope toe, and the deformation nature is primarily tensile damage and shear damage; howover, the plastic deformation zone of the thin-soil layer slope has the largest range. The slope cutting causes serious deformation of the slope toe, the X displacement also far exceeds the safety value, and the slope is in a state of instability, which is consistent with the actual situation onsite. This indicates that the geotechnical parameters and numerical simulation methods employed are realistic. Figure 7 presents the calculation result clouds for the thin-soil slope;Figure 8, the computed result clouds for the thick-soil slope; and Figure 9, the calculation result clouds for the rocky-soil mixed slope. Table 2 presents the results of numerical simulations for slopes of different structure types.
Determination of the threshold value
Methodology for determining the threshold value
When the slope stability coefficient is larger than 1.1, FLAC3D is used to compute the critical height of slopes at different slope ratios from the stability coefficients of red-bedded soft rock slopes at different heights and slopes (Wu et al., 2011). Because the cut slope height and gradient are the most important influencing elements of slope stability, this research presents a method for determining the cut slope height and gradient threshold values. The slope cutting height and gradient are computed based on the collected data, and the slope cutting disaster points are screened; then, the typical slope cutting disaster points are extracted, and their disaster modes and mechanisms are investigated. The disaster process of typical slope cutting is investigated via numerical simulation, and the values of the geotechnical parameters are modified according to the results of the numerical simulation to make the simulation results consistent with the actual situation. The modified geotechnical parameters are used to simulate and compute the slope cutting disaster process. In addition, the critical slope cutting height and gradient under different slope structures are determined and then taken as the theoretical threshold values for safe slope cutting. Figure 10 presents the network diagram of the evolutionary relationship of the cut slope indicator threshold.
Figure 11 presents the step-by-step search method for determining the critical cut slope gradient and height. First, the slope of a slope structure is determined, the cut slope height h is kept unchanged, the cut slope gradient α is modified, the magnitude is increased by 0.1° each time until the slope is unstable, and the unstable slope gradient α1 under the cut slope height h is obtained; then, the cut slope height is changed from h to h1, and the magnitude of 0.1 m is increased each time. This is the same method used to obtain the unstable slope gradient α2 under the cut slope height h1. This continues until the critical cut slope height and critical cut slope have been determined.
Threshold results
The theoretical threshold values of the three slope structures were obtained using the method presented in Figure 11. The landslide control engineering survey specifies that the safety factor of the slope under natural and storm conditions is Fs ≥ Fst, and Fst is 1.25. Therefore, 75% is used as the threshold value, which is discounted by 25% as the safety reserve; the proposed threshold value is taken as the guideline value for engineering slope cutting in Cangxi County and similar red-layer areas. The slope needs to be treated with multistage slope release, and the slope gradient and slope height of each stage shall not exceed the recommended threshold value when the height of the cut slope is much larger than the threshold value. As presented in Table 3, the threshold values of slope cutting indicators for various slope structures are obtained.
Analysis of the disaster-causing mechanisms
It was found that there are differences in the degree of stability weakening of different slope structures by slope cutting and rainfall, with slope cutting being the main factor causing disasters and rainfall promoting the occurrence of disasters.
1) Engineering cut slope: Consolidation and settlement have stabilized the natural slope. Slope cutting undermines the slope’s stable state, which causes stress redistribution inside the slope and the formation of a dangerous critical air surface. Slope cutting results in the loss of slope toe support, an increase in the sliding tendency of prospective landslides, and a decrease in slope stability (Cui et al., 2018).
2) Rainfall factor: The root system and the stems and leaves of the vegetation on the slope surface generate a mulching film that prevents precipitation infiltration. The cover film generated by vegetation is destroyed by slope cutting, making it easier for rainwater to infiltrate into the interior of the slope through the cut slope surface, thus reducing the slope’s stability. Rainwater infiltration leads to a rise in soil void water pressure, and as the pore water pressure rises, the cohesion and internal friction angle of the soil decrease with time, ultimately leading to slope instability and sliding (Cui et al., 2018). It was found that desiccation cracks can lead to shallow slope damage; nevertheless, plant roots can efficiently prevent the formation of desiccation cracks (Gao et al., 2021). Therefore, when a fissure already has developed on the slope, planting a lawn on the slope is an effective and economical means of preventing rainwater infiltration into the body of the slope (Leng et al., 2021).
3) Slope structure: The degree of weakening of slope cutting on the structural stability of different slopes varies. The slope toe loses support to the possible landslide body after the slope cutting of the thin-soil layer, and the potential landslide body slides down the sliding surface. The slope partially loses the support to the prospective landslide body after the thick-soil layer slope is cut, the degree of consolidation of the surface soil is reduced, and a sliding surface develops along with a specific depth, generating local instability. After the slope cutting of soil-rock mixed slope, the surface soil layer is the first to slip, and the soil sliding process interrupts the underlying broken rock layer; moreover, as the scale of sliding rises, the underlying broken rock layer incidentally slides together.
The surface of the broken rock layer in the rocky-soil mixed slope is rough, which stops the sliding, and the sliding trend of the slope is relatively small after the slope cutting. The junction of incompletely and fully consolidated soil, with a high coefficient of friction between the soil layers, is the possible sliding surface of a thick-soil slope. The residual slope deposit on the surface is the possible landslide body of the thin-soil slope. The residual slope deposit loses its support near the slope toe and slips over the bedrock surface after the slope is cut. The stability of cut slopes on different structural types of slopes is weakened to different degrees, with thin-soil slopes being the toughest, thick-soil slopes the second toughest, and rocky-soil mixed slopes the weakest.
An engineering cut slope causes the slope to lose toe support, resulting in the sliding force of potential landslide body being greater than the maximum static friction, and the slope changes from stable to unstable state. Therefore, an engineering cut slope is the main initiating factor of landslide disaster. The rainwater infiltration will reduce the strength of the soil, increase the self-weight of the soil, and promote the formation of landslide hazards. Slopes of different structural types are affected by shear slope to different degrees, with thin-soil layer slopes being affected to the highest degree, followed by thick-soil layer slopes, and rocky-soil mixed slopes being affected to the lowest degree. The relationship between the contributing factors and slope instability is shown in Figure 12.
Conclusion
In this research, Cangxi County was used as the research area, and the threshold values of the disaster-causing indicators of engineering slope cutting in the region were determined based on survey data and numerical simulations. The following conclusions were drawn:
1) In Cangxi County, there are a total of 108 engineering cut slope points, of which 32 have caused disasters and 76 are less stable. The slope cutting points are mainly distributed in urban areas and towns, and the rural distribution is relatively small. The height of the cut slope is 4–20 m, mainly concentrated in 5–7 m, and a total of 78 sites were developed, accounting for 72.2% of the total number of surveys. Thin-soil, thick-soil, and rocky-soil mixed slopes are the three types of slope structures in Cangxi County. The impact of slope cutting on the stability of slopes of various structural types varies, with mixed rocky-soil slopes being the most robust, followed by thick-soil slopes, and finally thin-soil slopes.
2) Slope cutting reduces the slope’s inherent stability, leading to a lack of toe support, which is the primary cause of disaster. Furthermore, rainwater infiltration reduces the strength of the soil, increases the self-weight of the soil, and promotes disaster formation as the main driving factor.
3) The theoretical threshold value of slope cutting height and slope cutting gradient is determined through a numerical simulation, and 75% is suggested as the threshold value, which is used to guide the engineering slope cutting in the red-layer area. Table 3 presents the slope cutting thresholds for the three slope structure types.
Data availability statement
The original contributions presented in the study are included in the article/Supplementary Material. Further inquiries can be directed to the corresponding authors.
Author contributions
YP and KC were responsible for writing. ZW, YW, and CD provided engineering data and geophysical analysis. NC and XX were responsible for numerical simulation analysis. QH and FL were responsible for determining the threshold and mechanism. MG and GZ were responsible for review and proofreading.
Funding
This research was supported by the National Natural Science Foundation of China (No. 42107281), the Opening Fund of State Key Laboratory of Geohazard Prevention and Geoenvironment Protection (Chengdu University of Technology) (No. SKLGP 2022K014), the Opening Foundation of Key Laboratory of Geohazard Prevention of Hilly Mountains, Ministry of Natural Resources (Fujian Key Laboratory of Geohazard Prevention) (Grant No. FJKLGH 2022K005), and the On-campus Talent Introduction Project at Xihua University (No. Z201125).
Conflict of interest
YP, ZW, NC, and XX were employed by Sichuan Huadi Construction Engineering Co., Ltd.
The remaining authors declare that the research was conducted in the absence of any commercial or financial relationships that could be construed as a potential conflict of interest.
Publisher’s note
All claims expressed in this article are solely those of the authors and do not necessarily represent those of their affiliated organizations, or those of the publisher, the editors, and the reviewers. Any product that may be evaluated in this article, or claim that may be made by its manufacturer, is not guaranteed or endorsed by the publisher.
References
Admassu, Y., and Shakoor, A. (2015). Cut slope design for stratigraphic sequences subject to differential weathering: a case study from Ohio. Environ. Eng. Geoscience 21 (4), 311–324. doi:10.2113/gseegeosci.21.4.311
Ai, X. Y., Wang, L., Xu, D. P., Rong, J. J., Ai, S., Liu, S., et al. (2021). Stability of artificial soil aggregates for cut slope restoration: a case study from the subalpine zone of southwest China. Soil Tillage Res. 209 (2), 104934. doi:10.1016/j.still.2021.104934
Awang, H., Salmanfarsi, A. F., Aminudin, M. S., and Ali, M. I. (2021). Stability of weathered cut slope by using kinematic analysis. IOP Conf. Ser. Earth Environ. Sci. 682 (1), 012018. doi:10.1088/1755-1315/682/1/012018
Cao, C. S., Wu, S. R., Pan, M., and Liang, C. Y. (2016). Mechanism research on artificial slope cutting-induced loess landslide. Rock Soil Mech. 37 (04), 1049–1060. In Chinese with English Abstract. doi:10.16285/j.rsm.2016.04.018
Cui, S. H., Pei, X. J., Wu, H. Y., and Huang, R. Q. (2018). Centrifuge model test of an irrigation-induced loess landslide in the heifangtai loess platform, northwest China. J. Mt. Sci. 15 (1), 130–143. doi:10.1007/s11629-017-4490-0
Cui, S., Pei, X., Jiang, Y., Wang, G., Fan, X., Yang, Q., et al. (2021). Liquefaction within a bedding fault: Understanding the initiation and movement of the daguangbao landslide triggered by the 2008 wenchuan earthquake (ms = 8.0). Eng. Geol. 295, 106455. doi:10.1016/j.enggeo.2021.106455
Gao, M. B., Zhang, H., Cui, S. H., Wu, Z. T., Liu, J., Feng, L. X., et al. (2022). Investigation on deformation mechanism and treatment effect of a scattered slope based on continuum–discontinuum element method and finite difference method. Front. Earth Sci. 10, 894923. doi:10.3389/feart.2022.894923
Gao, Q. F., Zeng, L., and Shi, Z. N. (2021). Effects of desiccation cracks and vegetation on the shallow stability of a red clay cut slope under rainfall infiltration. Comput. Geotechnics 140 (1), 104436. doi:10.1016/j.compgeo.2021.104436
He, L., Ren, Y., Feng, Z. X., and Han, Z. W. (2014). Numerical simulation and Design optimization research of slope ratio and platforms influence on a high rock cut slope engineering stability. Adv. Mat. Res. 1065-1069, 151–158. doi:10.4028/www.scientific.net/amr.1065-1069.151.net/AMR.1065-1069.151
Leng, X. L., Wang, C., Zhang, J., Sheng, Q., Cao, S., and Chen, J. (2021). Deformation development mechanism in a loess slope with seepage fissures subjected to rainfall and traffic load. Front. Earth Sci. (Lausanne). 9, 769257. doi:10.3389/feart.2021.769257
Li, H. (2022a). SCADA data based wind power interval prediction using LUBE-based deep residual networks. Front. Energy Res. 10, 920837. doi:10.3389/fenrg.2022.920837
Li, H. (2022). Short-term wind power prediction via spatial temporal analysis and deep residual networks. Front. Energy Res. 10, 920407. doi:10.3389/fenrg.2022.920407
Li, H., Deng, J., Feng, P., Pu, C., Arachchige, D., and Cheng, Q. (2021a). Short-term nacelle orientation forecasting using bilinear transformation and ICEEMDAN framework. Front. Energy Res. 9, 780928. doi:10.3389/fenrg.2021.780928)
Li, H., Deng, J., Yuan, S., Feng, P., and Arachchige, D. (2021b). Monitoring and identifying wind turbine generator bearing faults using deep belief network and EWMA control charts. Front. Energy Res. 9, 799039. doi:10.3389/fenrg.2021.799039
Li, L. P., Lan, H. X., and Peng, J. B. (2020). Loess erosion patterns on a cut-slope revealed by LiDAR scanning. Eng. Geol. 268, 105516. doi:10.1016/j.enggeo.2020.105516
Liu, W., Hu, Y. X., He, S. M., Zhou, J. W., and Chen, K. T. (2021). A numerical study of the critical threshold for landslide dam formation considering landslide and river dynamics. Front. Earth Sci. (Lausanne). 9, 651887. doi:10.3389/feart.2021.651887
Mei, Y., Zhou, D. B., Hu, C. M., Wang, X. Y., Zhang, Y., Xiao, N., et al. (2022). Study on deformation characteristics of loess ultrahigh-fill slope based on large-scale undisturbed soil centrifugal model tests. Front. Earth Sci. (Lausanne). 10, 848542. doi:10.3389/feart.2022.848542
Panthee, S. (2016). Parametric evaluation of shear strength parameters on the stability of cut slope: A case study from mahabaleshwar road section, India. J. Nepal Geol. Soc. 51, 73–76. doi:10.3126/jngs.v51i0.24094
Postill, H., Helm, P. R., Dixon, N., Glendinning, S., Smethurst, J., Rouainia, M., et al. (2021). Forecasting the long-term deterioration of a cut slope in high-plasticity clay using a numerical model. Eng. Geol. 280, 105912. doi:10.1016/j.enggeo.2020.105912
Qin, C. A., Chen, G. Q., Zhu, J., and Tang, P. (2020). A precursor of bedding rockslide: Rock spalling in the key block triggered by tensile cracks. Bull. Eng. Geol. Environ. 79 (2), 2513–2528. doi:10.1007/s10064-019-01703-y
Shao, Y. L., Yang, H. R., Wang, J. J., and Yang, Y. (2022). Deformation evolution process of a gently inclined red-bed slope influenced by cutting. Adv. Sci. Technol. Water Resour. 42 (02), 95–100. In Chinese with English Abstract. doi:10.3880/j.issn.1006-7647.2022.02.015
Solgi, A., Reza, N., Zenner, E. K., Hemmati, V., Behjou, F. K., and Masumian, A. (2021). Evaluating the effectiveness of mulching for reducing soil erosion in cut slope and fill slope of forest roads in hyrcanian forests. Croat. j. eng. 42 (2), 259–268. doi:10.5552/crojfe.2021.756
Strouth, A., and McDougall, S. (2021). Historical landslide fatalities in British columbia, Canada: Trends and implications for risk management. Front. Earth Sci. 9, 1-8. doi:10.3389/feart.2021.606854
Tang, P., Chen, G. Q., Huang, R. Q., and Wang, D. (2021). Effect of the number of coplanar rock bridges on the shear strength and stability of slopes with the same discontinuity persistence. Bull. Eng. Geol. Environ. 80(5), 3675–3691. doi:10.1007/s10064-021-02180-y
Tang, P., Chen, G. Q., Huang, R. Q., and Zhu, J. (2020). Brittle failure of rockslides linked to the rock bridge length effect. Landslides 17 (4), 793–803. doi:10.1007/s10346-019-01323-3
Wang, L., Li, G., Chen, Y., Tan, J. M., Wang, S. M., and Guo, F. (2021). Field model test on failure mechanism of artificial cut-slope rainfall in southern Jiangxi. Rock Soil Mech. 42(03), 846–854. (In Chinese). doi:10.16285/j.rsm.2020.1129
Wu, G. X., Chen, X. S., Ding, J. S., and Ding, W. F. (2011). Determination of critical height of slope filled by red-bed soft rock under seismic loading. Adv. Mat. Res. 261-263, 1655–1659. doi:10.4028/www.scientific.net/amr.261-263.1655.net/AMR.261-263.1655
Xu, J. S., Zhao, X., Li, P. F., and Zhang, M. J. (2021). Stability of a 3D unsaturated vertical cut slope subjected to variable rainfall infiltration. Comput. Geotechnics 134 (1), 104110. doi:10.1016/j.compgeo.2021.104110
Zhang, W. C., and Wang, D. (2020). Stability analysis of cut slope with shear band propagation along a weak layer. Comput. Geotechnics 125 (1), 103676. doi:10.1016/j.compgeo.2020.103676
Zhang, X. C., Li, Y. R., Liu, Y. S., Huang, Y. X., Wang, Y., and Lu, Z. (2021). Characteristics and prevention mechanisms of artificial slope instability in the Chinese loess plateau. Catena 207 (1), 105621. doi:10.1016/j.catena.2021.105621
Keywords: landslide, artificial slope cutting, failure mode, numerical simulation, threshold
Citation: Pan Y, Chen K, Gao M, Wu Z, Zheng G, He Q, Lu F, Wan Y, Du C, Cao N and Xie X (2022) Study on the threshold value of disaster-causing factors of engineering slope cutting in red-layer areas. Front. Earth Sci. 10:961615. doi: 10.3389/feart.2022.961615
Received: 05 June 2022; Accepted: 20 July 2022;
Published: 22 August 2022.
Edited by:
Jingren Zhou, Sichuan University, ChinaReviewed by:
Peng Tang, Jiangxi University of Science and Technology, ChinaChang’An Qin, Beijing University of Civil Engineering and Architecture, China
Copyright © 2022 Pan, Chen, Gao, Wu, Zheng, He, Lu, Wan, Du, Cao and Xie. This is an open-access article distributed under the terms of the Creative Commons Attribution License (CC BY). The use, distribution or reproduction in other forums is permitted, provided the original author(s) and the copyright owner(s) are credited and that the original publication in this journal is cited, in accordance with accepted academic practice. No use, distribution or reproduction is permitted which does not comply with these terms.
*Correspondence: Kezhu Chen, NjA5MjE1MjA1QHFxLmNvbQ==; Meiben Gao, Z2FvbWJAbWFpbC54aHUuZWR1LmNu