- 1School of Earth and Space Sciences, University of Science and Technology of China, Hefei, Anhui, China
- 2Key Laboratory of Atmospheric Optics, Anhui Institute of Optics and Fine Mechanics, HFIPS, Chinese Academy of Sciences, Hefei, Anhui, China
- 3Collaborative Innovation Center on Forecast and Evaluation of Meteorological Disasters, Nanjing University of Information Science and Technology, Nanjing, Jiangsu, China
- 4Department of Mechanics and Aerospace Engineering, Southern University of Science and Technology, Shenzhen, Guangdong, China
- 5Beijing Meteorological Observation Center, Beijing, China
- 6Caribbean TLE Observatory, Cabo Rojo, PR, United States
- 7Guangzhou Power Supply Bureau, Guangzhou Power Grid Co, Ltd, China Southern Power Grid, Guangzhou, Guangdong, China
- 8State Key Laboratory of Space Weather, National Space Science Center, Chinese Academy of Sciences, Beijing, China
The observations of transient luminous events from space-borne platform extend our exploration on the mysteries of sprite phenomenology from continental thunderstorms to oceanic thunderstorms. By combining with ground-based measurements of causative strokes for hundreds of red sprites observed by the Imager of Sprites and Upper Atmospheric Lightnings (ISUAL) during 2004–2016, there is a consensus that negative cloud-to-ground (CG) strokes spawned by oceanic thunderstorms are more readily to produce sprites. The existing ground-based observations in both Caribbean Sea and near the coast of South China, mainly due to the contributions from numerous amateurs, are generally consistent with the implications of ISUAL observations. However, the physical mechanisms that might cause the enhancement of negative CG strength in the ocean remain not completely understood. There have been analyses on several cases of oceanic thunderstorms abundant in producing negative sprites. It seems that the production of negative sprites heavily depends on the size of parent thunderstorms, and they are often generated by thunderstorm conditions that are also favorable for gigantic jets.
Introduction
Red sprites are one primary category of transient luminous events (TLEs) appearing at altitudes of 50–90 km in the near space that are believed to be caused by intense tropospheric lightning (Pasko et al., 1997; Huang et al., 1999). In the past 3 decades, the efforts of many researchers all over the world, including numerous amateurs, have confirmed that red sprites are a fairly common phenomenon that could be produced by energetic cloud-to-ground (CG) lightning strokes spawned by various types of thunderstorms (Lyons, 1996; Huang et al., 2018a, Huang et al. 2018b; Wang et al., 2021b, Wang et al 2021c). Coordinated observations combining with the measurement of radio-frequency (RF) electromagnetic fields radiated by sprite-producing lightning strokes have revealed many details regarding the mechanism of sprite formation in the mesosphere (e.g., Li et al., 2012; Cummer et al., 2013; 2016; Ren et al., 2019; Kuo et al., 2021).
Lightning strokes of either polarity (i.e., positive or negative) could produce sprites. When the electric field (E-field) change caused by the charge transfer from thundercloud to ground exceeds the critical value of conventional breakdown (Ek) at certain altitude, the ionization will be initiated and became sustained only if the ambient E-field remains higher than Ek (Pasko et al., 1997; Qin et al., 2013). However, the ground-based observations over continental thunderstorms show that, despite of the well-known dominance of negative CG lightning on the land, the vast majority of sprites observed over continental thunderstorms are produced by positive CG strokes (e.g., Li et al., 2012). In particular, the documented fraction of sprites produced by negative CG strokes is substantially smaller than that inferred from the ground-based measurement of lightning strength on a global scale (e.g., Füllekrug et al., 2002; Sato and Fukunishi, 2003), constituting a sprite polarity paradox (Williams et al., 2007).
Halos, as relatively non-structured phenomena of diffusive emission that often accompany sprites (in the form of halo sprites), usually appear at relatively high altitudes (Frey et al., 2007). The formation of halos is suggested to follow a similar process to sprites, except for that the duration of lightning-induced E-field perturbation surpassing the critical E-field is relatively short (Li et al., 2012; Qin et al., 2013). Based on the space-borne observations from Imager of Sprites and Upper Atmospheric Lightnings (ISUAL) and ground-based observations of Photometric Imager of Precipitated Electron Radiation (PIPER) (Chen et al., 2008; Kuo et al., 2013; Newsome and Inan, 2010), Williams et al. (2012) proposed that the occurrence of halos might resolve the aforementioned sprite polarity paradox. That is, most halos are not registered by the conventional ground-based video observation due to the relatively short duration. Indeed, pure halos are rarely reported in ground-based observations by means of video cameras at regular frame rates (for example, 25 or 30 frames per second, depending on the encoding format).
In this paper, we mainly address the contrast between continental and oceanic thunderstorms as reflected by the observations of red sprites and halos from both space-borne and ground-based platforms. This contrast might be in line with the high incidence rate of high-peak current negative CG strokes in the ocean as found on the basis of ground-based lightning detections (Said et al., 2013). Therefore, the further efforts to characterize the oceanic sprite-producing thunderstorms might shed light on the meteorological conditions that favor the occurrence of intense negative CG strokes in the ocean (e.g., Chronis et al., 2016).
Implications from space-borne observations
The analysis of red sprites captured by the ISUAL payload on the FORMOSAT-2 satellite indicates that there is a much higher population of sprites produced by negative CG strokes over oceanic thunderstorms (Lu et al., 2017). As for halos, a similar category of TLEs that typically endure less than 1 ms (Frey et al., 2007; Newsome and Inan, 2010; Kuo et al., 2013), their causative strokes are predominantly of negative polarity and tend to crowd in the ocean (Lu et al., 2018).
Figure 1 shows the geographic distribution of halos, sprites, and halo/sprite events observed by ISUAL during 2004–2016. The sprite location is estimated by the ISUAL team according to the procedures described by Chen et al. (2008). By comparing with the location of causative CG stroke given by the National Lightning Location Network (NLDN), Liu et al. (2017) showed that the location of ISUAL-detected events has been determined with error typically less than 100 km. As shown in Figure 1A, there are totally 1,099 pure halo events that are mainly (791, or 72%) observed over oceanic regions. According to the results of Lu et al. (2018), intense negative CG strokes in the ocean might made a major contribution. For pure sprites (Figure 1B), however, most (686 out of 1,157, or 59.3%) were observed over land, which is primarily due to a remarkable high concentration in central Africa. It merits further investigation regarding the dominant polarity of sprites in this region. There were 470 sprites whose occurrence was accompanied by a preceding halo feature (Figure 1C), and these events were almost evenly distributed over land and ocean.
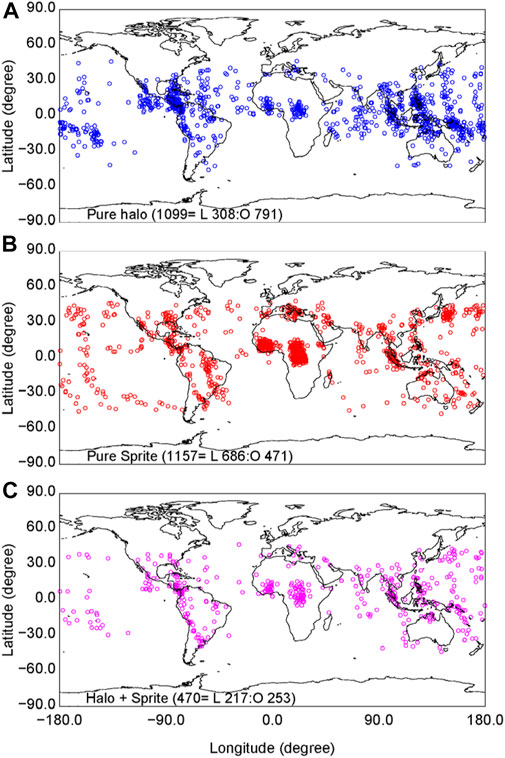
FIGURE 1. Geographic distribution of (A) pure halos, (B) pure sprites, and (C) halo-sprite events observed by ISUAL during 2004–2016.
The analysis of Lu et al. (2017) with respect to sprites observed in the vicinity of North America shows that the continental thunderstorms primarily produce sprites through positive CG strokes, which is consistent with the ground-based observations in 2008–2013 (Huang et al., 2018a). The mesoscale convection systems (MCSs) prevailing in the Great Plain of North America are prolific sprite-producers, and many sprite-related studies at the early stage were conducted based on the observations of sprites over them (e.g., Lyons, 1996; Huang et al., 1999; Hu et al., 2002; Miyasato et al., 2002; Cummer and Lyons, 2005; Li and Cummer, 2011; Kuo et al., 2013, Kuo et al., 2016).
The distribution pattern of halos and sprites observed by ISUAL is substantially different from that of global lightning as indicated by both space observations (e.g., Christian et al., 2003) and ground-based lightning location results (Said et al., 2013), implying that lightning strokes in different places bear varying energies to impose electromagnetic stress on the lower ionosphere. Figure 2 shows the distribution of various TLE types with latitude. Both pure halos and sprites with halo feature were predominantly produced at low latitudes (Figures 2A,C). As shown in Figure 2B, the geographic distribution of pure sprite observations exhibits a secondary peak at middle latitudes especially in north hemisphere, which is mainly caused by the concentration of pure sprites in the Mediterranean.
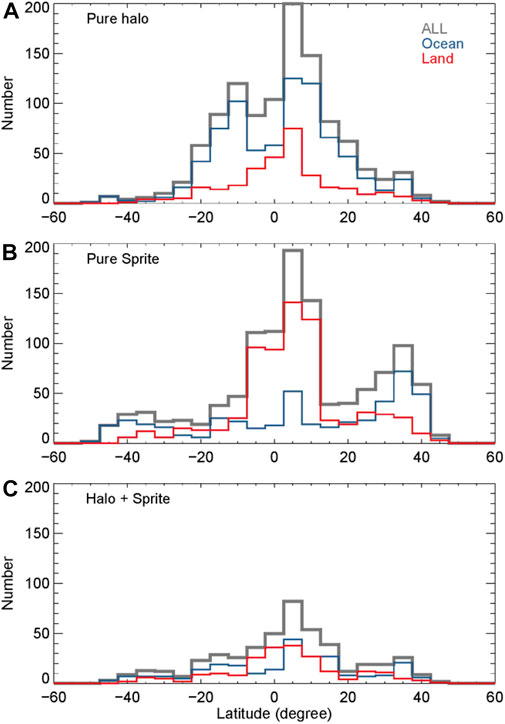
FIGURE 2. Distribution of ISUAL-observed TLEs along latitudes. The ISUAL TLEs are classified into three categories, i.e., (A) pure halo, (B) pure sprite, and (C) halo with sprite.
Based on the identification of transient Schumann Resonances (SRs) in the extremely low-frequency (ELF) magnetic field waveform at two stations during a 1-year period, Sato and Fukunishi (2003) estimated the average global occurrence rate of sprites to be around 720 events/day. It is noticed that their results indicate a region with high occurrence rates of sprites beyond the east coast of southern Africa, which is however not clear in Figure 1. Instead, the ISUAL observations indicate the high occurrence rate of sprites in central Africa, which is also the capital of lightning in the world as seen from the space observation (Christian et al., 2003).
Ground-based observations of negative sprites
The first ground-based observation of sprite was probably serendipitously obtained by a research team who was testing their low-light-level TV camera designed for tracking rocket flight. This fortunate recording, along with existing reports from space-born observations, inspired more scientists from various continents to conduct the ground-based observations over thunderstorms. Sprites made by negative CG strokes were extremely rarely captured at the initial stage of sprite hunting (Barrington-Leigh et al., 1999), and the dataset of negative sprites with coordinated lightning sferic measurements slowly accumulated (Taylor et al., 2008; Li et al., 2012; Lu et al., 2016).
However, although the space-borne observations indicate a population of negative sprites in the ocean, there is generally an absence of ground-based observations of red sprites over oceanic thunderstorms before 2016. While Huang et al. (2012), examined the occurrence of several gigantic jets over Typhoon Lionrock (2010), they also mentioned the observation of many sprites, while unfortunately, these events were not examined in details. Boggs et al. (2016) reported the observations of five sprites produced by negative CG strokes hosted in two coastal thunderstorms both associated with a tropical disturbance. All these sprite-producing negative CG strokes belonged to the categories recognized earlier by Lu et al. (2012). Huang et al. (2018b) reported the observations of tens of sprites over Hurricane Matthew (2016), whereas these events all turned out to be produced by positive CG strokes. However, in a further study on this particular thunderstorm case, Huang et al. (2021) showed that Hurricane Matthew (2016) could have produced hundreds of sprites during its lifetime, including quite many negative CG strokes with impulsive charge transfer in excess of the known threshold for sprite production.
Negative sprites produced by continental thunderstorms
Negative sprites were rarely produced by CG strokes hosted in continental thunderstorms. According to the survey of Williams et al. (2007), the percentage of confidential negative sprite observations is estimated to be about 0.1% before (Cummer et al., 2006b).
Barrington-Leigh et al. (1999) reported the first observation of negative sprites in North America, which was followed by Miyasato et al. (2002) who obtained the array photometric measurement of the sprite halo event produced by a negative CG stroke. Taylor et al. (2008) reported the observation of a negative halo-sprite event in South America. Li et al. (2012) also reported that during the 4-years observations (from 2008 to 2011) at several ground-based stations in the continental United States, among 1,651 sprites captured, only six events (and thus a ratio of 0.36%) were confirmed to be produced by negative CG strokes. The inclusion of ground-based sprite observations in the following 2 years (2012–2013) does not significantly change the percentage of negative sprites in the ground-based observations over continental thunderstorms. So far, there was no report of ground-based observations yet with respect to negative sprites over thunderstorms in Europe.
Almost all the sprite-producing negative CG strokes appear to bear several similar features: 1) the peak current usually reaches above -80 kA, and 2) the causative charge transfer is usually high (greater than −450 C km) and impulsive (with time scale <1 ms), and there is no evidence of long continuing current as often observed for intense positive CG strokes (Lu et al., 2009, 2013).
Li et al. (2012) examined six cases of sprites produced by negative CG strokes, and found that these negative sprites show similar features in their morphology: negative sprites are always accompanied by halos, and the downward streamers usually terminate at higher altitudes (approximately 55–60 km) than their positive counterparts. Li et al. (2012) also suggested that the timescale of causative CG strokes, primarily the impulse current, has a significant impact on the morphology of sprites (e.g., Qin et al., 2013). First of all, as shown in Figure 3 regarding one of the cases examined in details by Li et al. (2012), due to the relatively short duration and therefore a less influence by the relatively high conductivity at high altitudes, the lightning-induced E-field perturbation can penetrate into higher region; secondly, the short duration of charge transfer results in a greater induction component in the lightning-induced E-field perturbation (e.g., Contreras-vidal et al., 2021), and therefore the overall region of dielectric breakdown extends to a higher altitude.
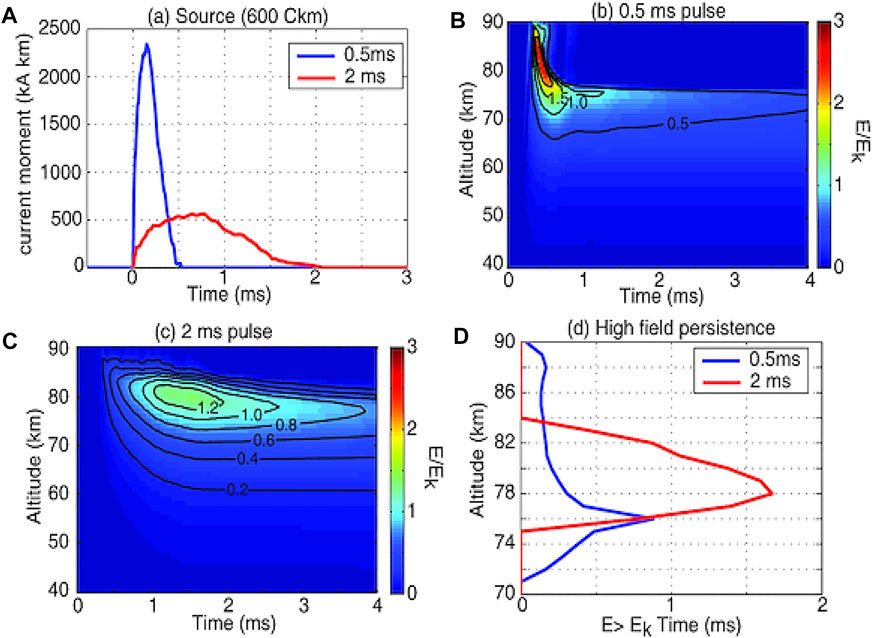
FIGURE 3. Comparison between two impulse charge transfer with different time scales to show the influence of charge transfer timescale on the lightning-induced E-field perturbation at the altitude of sprite initiation. The current moments with different time scales are shown in Panel (A), and the lightning-induced E-field perturbation associated with each case is shown in panels (B,C), respectively. Panel (D) shows the persistent time for the lightning-induced E-field to exceed the local E-field threshold of dielectric breakdown at different altitudes. Adopted from Li et al. (2012).
Later on, Lu et al. (2016) reported an unusual case of negative sprite that was actually produced by the joint effect of a moderate negative CG stroke along with its ensuing current surge (likely associated with an M-component). This particular observation actually implies that the duration of causative stroke might be very critical for a particular CG stroke to produce sprites. To demonstrate this, we present the simulation results for three different waveforms of causative CG stroke (Figure 4A). The length of vertical lightning channel is assumed to be 5 km in all cases. All three current moments accumulate to the same total charge moment change of +500 C km (Figure 4B). As shown in Figure 4C, the computation results for current waveform of type 1 and type 2 shows the comparison between different durations (corresponding to different polarities). The implications of this comparison are generally consistent with Li et al. (2012).
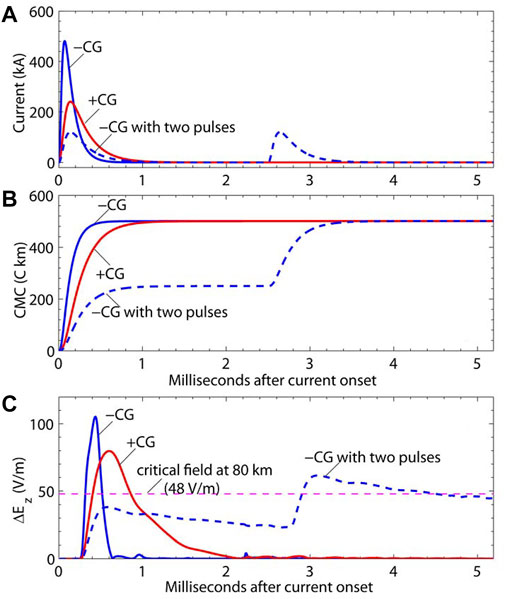
FIGURE 4. Vertical E-field perturbation caused by lightning current moment with different forms: type 1 for normal negative CG strokes, type 2 for normal positive CG strokes, and type 3 for a negative CG stroke with two consecutive current pulses as discussed by Lu et al. (2016). The current waveform of each type is shown in panel (A), and the lightning channel is assumed to be vertical with a length of 5 km. The cumulated charge moment change (CMC) and resulted E-field perturbation at 80 km altitude are shown in panels (B,C), respectively.
Also, the third type of current moment exhibits an interesting non-linear effect that merits further discussions. As shown in Figure 4C, because the sub-critical E-field perturbation caused by the first current pulse actually reduces the conductivity in the region of interest, the E-field perturbation generated by the second current pulse will endure longer than usual. Indeed, the streamer feature of negative sprite examined by Lu et al. (2016) was more distinct than most cases previously reported (e.g., Taylor et al., 2008; Li et al., 2012). The discussions above also have some implications for the general scenario that the typical sprite-producing lightning flashes, either of positive polarity or negative polarity, usually develop an extensive intra-cloud channel system prior to the CG stroke (e.g., Lu et al., 2009, 2012).
Boggs et al. (2016) reported the observations of totally five negative sprites near the coast of Florida over two thunderstorms associated with a tropical disturbance. The parent lightning flashes of all these events were characterized by a high-level progression in the upper positive region. Their analyses indicate that an excess amount of midlevel negative charge in comparison with the upper positive charge is essential for the production of negative CG strokes with sprite-producible impulse charge transfer. This “charge imbalance” could be favored jointly by the high wind shear at the middle to upper regions and the low negative CG flash rate.
Ground-based observations from Puerto Rico
Huang et al. (2018b) examined the massive sprite production (about 40 events totally on two consecutive nights) over Hurricane Matthew (2016), as captured by an amateur photographer, Frankie Lucena, who began to capture TLEs from his places in Puerto Rico since May of 2016. After examining the broadband lightning sferics of these sprites recorded in Duke Forest, it was surprisingly found that all these events were produced by positive CG strokes. For approximately 86% of these sprites, the causative CG strokes were detected by the World-Wide Lightning Location Network (WWLLN) (e.g., Hutchins et al., 2012). As shown in Figure 5, these sprite-producing CG strokes were all located in the outer rainband region defined by relatively cold cloud-top brightness temperature (≤194 K).
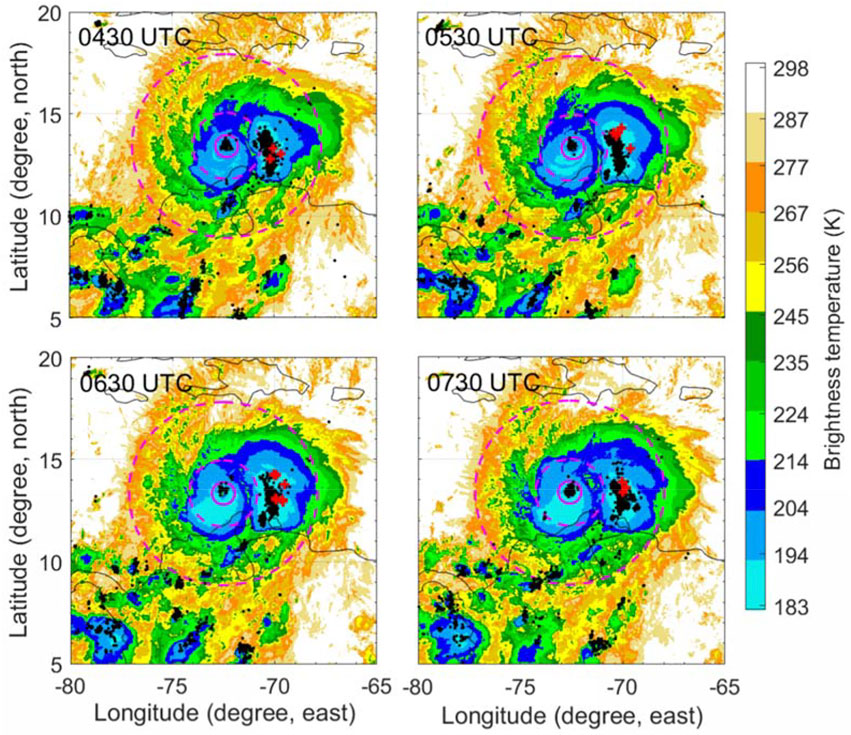
FIGURE 5. Distribution of parent strokes for the sprites observed during four consecutive 1-hour time windows (centered at the time specified in each panel) over Hurricane Matthew (2016). Adapted from Huang et al. (2018b).
The absence of negative sprite observation for this particular thunderstorm arose more curiosity, and therefore all the available observations from the very beginning were collected to make a long-term survey regarding the sprite phenomenology in the Caribbean area. After examining the sferic waveform recorded coordinately with these observations, as well as the characteristics of sprite morphology from existing studies, the polarity of causative lightning strokes is determined for the majority of sprites captured in the 5-years time period of 2016–2020. Nearly 100 red sprites produced by negative CG strokes were identified from this data set. Recently, with the data obtained by Frankie Lucena, (Wang et al., 2019), reported the observations of several negative sprites over a tropical depression that eventually developed into Hurricane Harvey (2017). It should also be noted that, as indicated in Figure 6B, this particular thunderstorm also produced quite a few gigantic jets during the nighttime observation on August 19, similar to the case examined by Boggs et al. (2016), which provides further implications regarding the attributes of thunderstorm charge structure that might be favorable for producing negative sprites. One negative sprite and one gigantic jet were shown in the inset of Figure 6A, which shows the overall track of Hurricane Harvey (2017) since August 18, 2017.
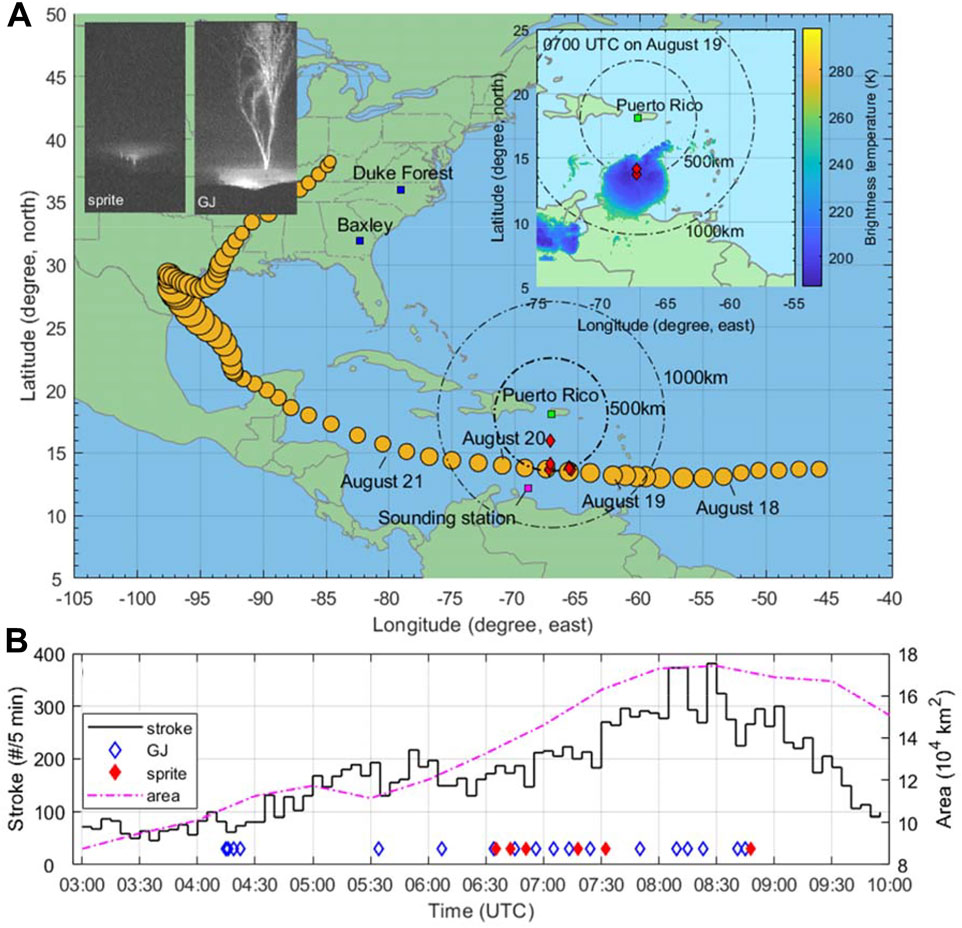
FIGURE 6. Observations of negative sprites over a tropical thunderstorm that eventually developed into Hurricane Harvey (2017). Panel (A) shows the overall track of the hurricane, and the parent lightning of TLE observations from Puerto Rico on the night of August 19. The inset images of panel (A) show the observations of one negative sprite and one gigantic jet, respectively. Panel (B) summarizes the observations in comparison with the lightning occurrence during the nighttime observation. Adapted from Wang et al. (2021b).
In comparison with space-borne observations that cannot keep track of the sprite production over a specific thunderstorm, the ground-based observations remain more valuable in diagnosing the dynamics of a particular thunderstorm that could be essential for producing negative sprites. As more and more ground-based observations of negative sprites accumulate, more sophisticated measurements will be incorporated to diagnose the meteorological conditions and thunderstorm properties conducive to the generation of negative sprite-producible CG strokes.
Contributions from Chinese amateurs
The amateurs have made important contributions to the exploration of spatial and temporal connection between TLEs and their parent lightning (e.g., van der Velde et al., 2007; Lu et al., 2011; Lu et al., 2013). With critical observations of several amateurs residing in New Mexico, Texas, and Florida, respectively, a detailed understanding on the in-cloud lightning evolution associated with gigantic jets and red sprites has been achieved.
Since the summer season of (Chen et al., 2014), the amateurs in China, including some professional photographers, have been enthusiastically contributing to the research of TLEs, either by providing the critical observations (e.g., Chou et al., 2016; Yang et al., 2020), or arousing the attention from the publicity. Yang et al. (2020) reported the analysis of a gigantic jet event captured by two amateurs concurrently from different sites in southern part of China.
In 2021, there was a tremendous surge in the number of TLEs recorded by the amateurs. Although the timing is not perfectly in line with GPS, the uncertainty is relatively small so that the parent CG strokes can be readily identified in most cases. Figure 7 shows the distribution of identified causative CG strokes for the sprites captured by the amateurs in China. Note that the negative CG stroke received special attention by carefully inspecting the consistency between the location and direction of sprite observation. We can see a relatively clear abundance of negative sprite-producing CG strokes in the open sea near the coast of Guangdong Province. Exactly 20 years ago, Su et al. (2002) have reported the observations of red sprites in the similar region, while there was no confirmative report yet of sprites produced by negative CG strokes.
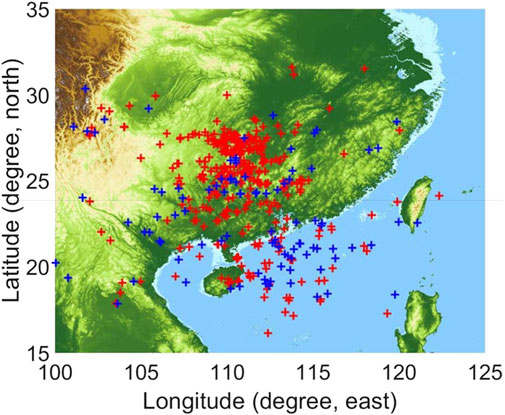
FIGURE 7. Distribution of sprites observed by the Chinese amateurs during 2021. Red pluses and blue pluses represent the detection of positive and negative sprite-producing CG strokes, respectively.
With the dramatic enhancement of captured events, some rare TLEs were also recorded, including quite a few gigantic jets and halos. In particular, on the night of three consecutive days in May of 2021, the massive sprite generation was recorded over a mesoscale convective system, which is the first observation of >100 sprites produced by one thunderstorm. This particular observation suggests that thunderstorms in East Asia could produce red sprites as actively as those in North America. Moreover, due to the influence of South Asian monsoon and East Asian monsoon, the unique three-step staircase topography in the mainland of China might exhibit some interesting patterns of sprite-producible CG strokes.
Implications on sprite phenomenology
The detailed development of sprite morphology can be resolved by means of high-speed video observations with enhanced sensitivity (e.g., Stanley et al., 1999; Stenbaek-Nielsen et al., 2000; Cummer et al., 2006a; Cummer et al., 2006b). Based on the high-speed video observations of sprites over a mesoscale convective system in the central United States, Ren et al. (2021) examined the time-resolved evolution of two sprite halo events in comparison with the charge moment change retrieved from the broadband sferic measurement. For the case shown in Figure 8, as an example, the halo feature appeared approximately at about 0.5 ms after the return stroke (see Figures 8A–C), and then the brightness remained growing until the sprite streamer became visible another 0.5 ms later (see Figures 8D–E). From this analysis, it can be seen that the transition from halo to sprite considerably depends on the duration of lightning-induced E-field perturbation exceeding the threshold of dielectric breakdown (e.g., Adachi et al., 2004; Hiraki, 2010). According to the analysis of Ren et al. (2021) based on the transmission line model of CG strokes, both induction and static component of lightning-induced E-field change contributed to the formation of halo; as time elapsed (about half millisecond after the return stroke), the electrostatic lightning E-field became dominant in supporting the streamer development.
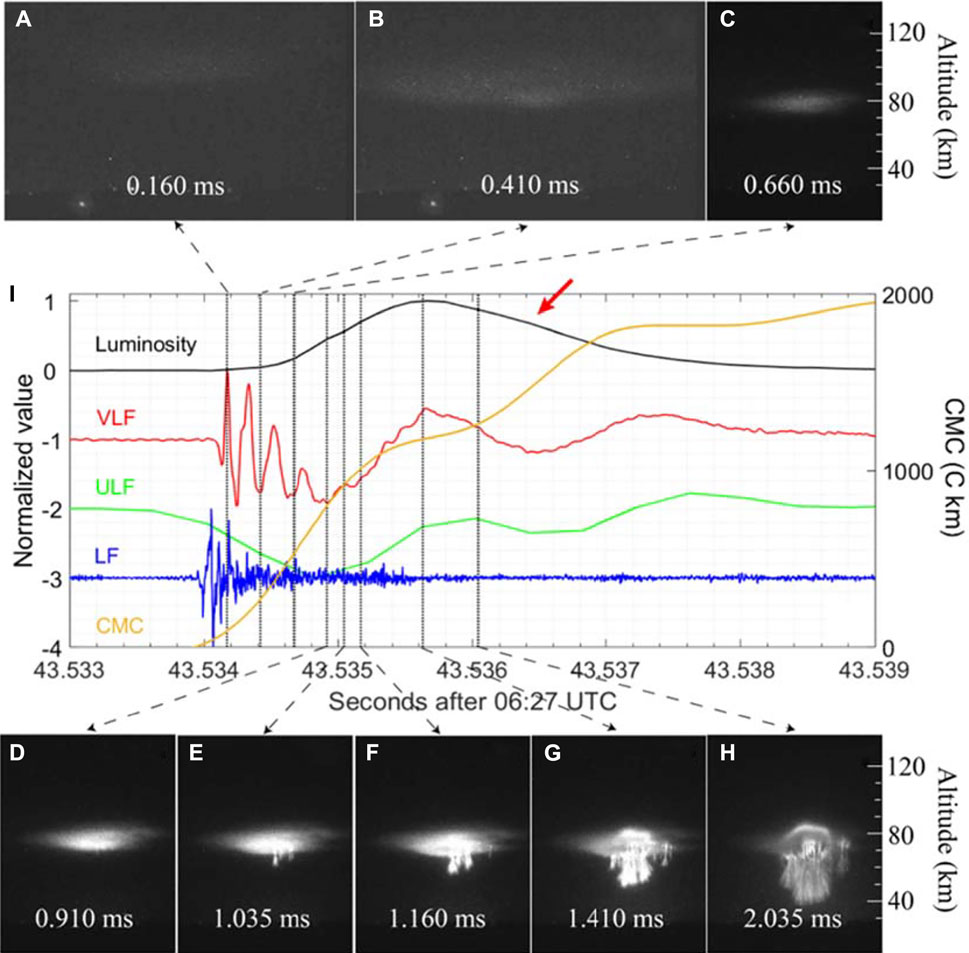
FIGURE 8. Examining the evolution of a halo-sprite event captured with the high-speed camera by comparing with the broadband lightning sferics [panel (I)]. The static images obtained by the high-speed observations were given in panels (A–H). Adopted from Ren et al. (2021).
Kuo et al. (2021) also examined the evolution of several TLE events (including two elves, one halo, and one halo sprite, and two sprites) captured with the high-speed video observations at speed of 10,000 fps from the Yushan weather station (at an altitude of 3,845 m) in Taiwan. The similar image sequences were obtained for two halo events produced by positive CG strokes with peak current of +118 kA and +172 kA, respectively. Their observations indicate that the elevation for the edge of halo emissions gradually declined over a time period of approximately 1.5 ms.
Critical electric field for sprite production
The analysis of Qin et al. (2012) with a two-dimensional (2D) plasma fluid model indicates that, under the same upper atmospheric ambient conditions, the sprite production requires a much larger charge moment change (CMC) for negative CG strokes than their positive counterpart (e.g., −300 C km vs. +200 C km).
It has been suggested that the impulsive charge transfer within 2 ms after the return stroke can be used as a metric to evaluate the potential of a particular CG stroke in producing sprites (Hu et al., 2002). This is probably because the ensuing impulse current of CG strokes usually drive a major pulse of approximately 2 ms duration as measured by the very-low-frequency (VLF) or ultra-low-frequency (ULF) magnetic field sensor (e.g., Price et al., 2002; Hu et al., 2002; Li and Cummer, 2011; Lu et al., 2013), or E-field change measurement with slow antenna (e.g., Hager et al., 2012). In fact, as also pointed out by Li et al. (2012), such pulses detected for negative sprite-producing CG strokes usually appear to be more impulsive than those driven by positive CG strokes. Therefore, based on the analysis of Ren et al. (2021), the contribution of induction component to the lightning E-field perturbation at the formation height of halo will be more important for negative CG strokes.
It should be mentioned that due to the different duration of halos and sprites, it is not quite reasonable to determine the threshold for negative sprite production with a time interval of 2 ms to calculate the impulse charge moment change (iCMC). As we can see from Figure 8, the halo production usually reaches the greatest brightness within 1 ms after the causative return stroke. Therefore, the iCMC over a time period of 2 ms might slightly overestimate the critical charge transfer for halo production, as well as negative sprites that are usually characterized by a halo feature (Li et al., 2012).
There are some other criteria that have been applied to evaluate the potential of individual lightning strokes in producing sprites or halos, such as peak current, which, however, has been shown not to be a good indicator for sprite production (Lu et al., 2013), especially for negative CG strokes (e.g., Lu et al., 2012). For the lightning detection of WWLLN, the energy of a specific lightning stroke was also estimated according to the received sferic waveform (e.g., Abarca et al., 2010; Hutchins et al., 2013), whereas it remains unknown whether this parameter can be used as an effective tool to evaluate the potential of a lightning stroke in producing sprite.
On the phenomenology of negative sprites
The morphology of negative sprites, since their first recording by Barrington-Leigh et al. (1999), has been fairly consistent by composing a pancake-shape diffusive region atop short vertical tendrils. The streamer region of negative sprites is usually very dim, and its termination altitude is also usually relatively high (e.g., Taylor et al., 2008; Li et al., 2012).
Wang et al. (2021a) reported the observation of an atypical negative sprite for which the broadband very low-frequency (VLF) magnetic sferics exhibit the signature of “sprite current,” namely a secondary VLF pulse (see Figures 9B,D–H). This feature was typically observed for bright sprites produced by positive CG strokes (e.g., Cummer et al., 1998; Lu et al., 2013). The examination of optical signal radiated by the causative stroke indicates that its duration (5.25 ms, see Figure 9I) is significantly longer than that (about 2.5 ms, see Figure 9J) of regular negative parent strokes for sprites observed by ISUAL. Also, the streamer portion descended to an altitude of 50 km, which is the lowest ever recorded for negative sprites. Nevertheless, the analysis of this event indicates that provided a relatively long duration of intense charge transfer after the return stroke, the sprite produced by the negative CG could also be very bright, suggesting that the aforementioned dependence of sprite morphology on the duration of causative CG charge transfer is also present for negative sprites.
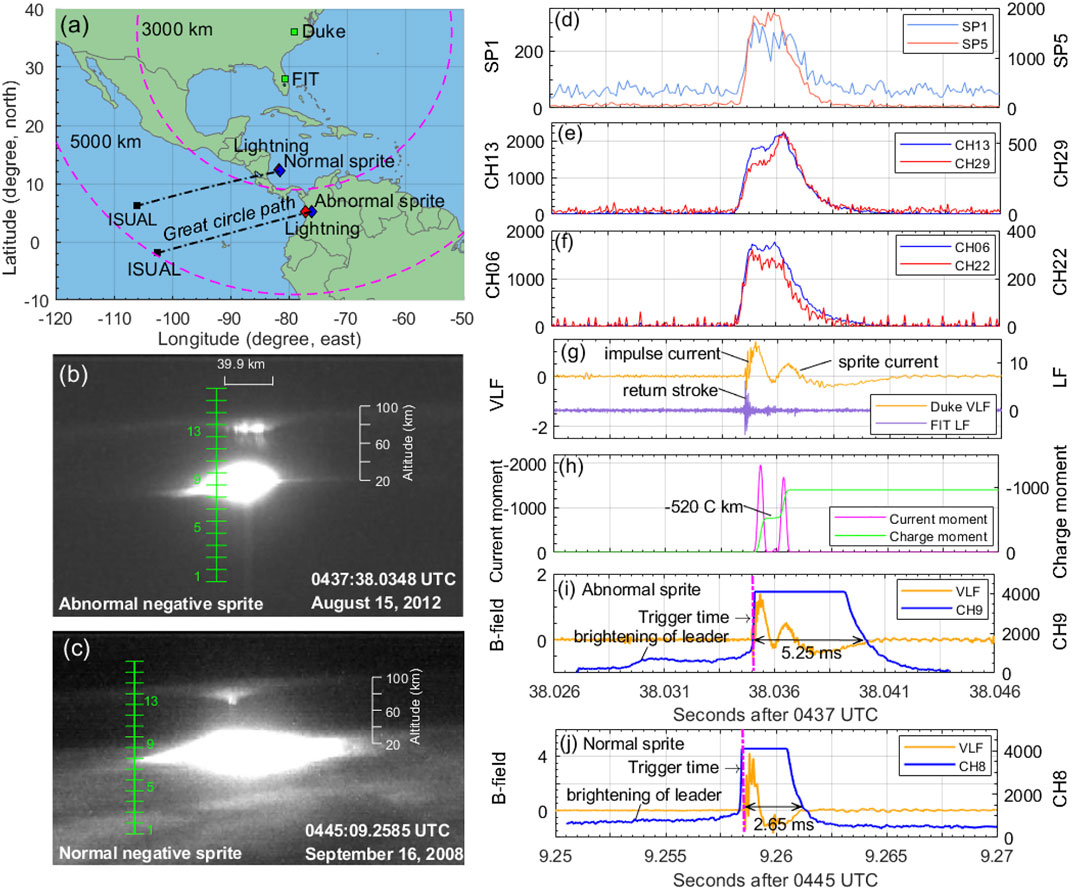
FIGURE 9. ISUAL observation of an unusual negative sprite for which the simultaneous measurement of VLF sferics also shows the signature of “sprite current.”. Panel (A) shows the ISUAL observations of two negative sprites, including one abnormal event [panel (B)] demonstrating the sprite current feature shown in the very low-frequency (VLF) sferic signal recorded in Duke Forest [panel (G)] and one normal event [panel (C)]. The selected other ISUAL measurements for the abnormal event were plotted in panels (D–F). The comparison between VLF signal and brightness curve is shown in panels (I,J), respectively. Adapted from Wang et al. (2021a).
In the relatively large data set of negative sprites based on the observations of ISUAL, negative sprites could also bear a different morphology. It should be noted that despite of a quite standard morphology with a pancake-shaped cap atop vertical compact streamers, there are also some negative sprites taking on appearance that remain to be explained. Interestingly, these abnormal events were mostly produced by sub-critical impulse charge moment change (i.e., iCMC < −300 C km) (Lu et al., 2017), and the underlying physical mechanism remains unknown.
Thoughts for future studies
Apparently, more ground-based observations of sprites produced by vigorous negative CG strokes spawned by oceanic thunderstorms are desired to characterize the physical connection between impulse charge transfer of sprite-producing CG strokes and time-resolved evolution of sprites. There are no conclusive results yet regarding why the oceanic thunderstorms are so productive in spawning intense negative CG strokes. Chronis et al. (2016) discussed several factors that might contribute to making oceanic thunderstorm prolific in producing negative CG strokes with high peak current and probably also large impulse charge moment change. Their conclusion is that the enhancement of negative CG strength in the ocean is most likely caused by the modulation of thundercloud potential by sodium chloride (NaCl) and ice crystal size. The general connection between lightning strength and thunderstorm potential is consistent with that oceanic thunderstorms are prone to spawn intense negative CG strokes favorable for sprites (i.e., negative CG strokes with particularly large impulse charge transfer), as a greater difference between thunderstorm potential and ocean surface (with zero potential) is bound to cause a higher charge transfer. A better understanding on the lightning phenomenology related to oceanic thunderstorms is desired (e.g., Han et al., 2021).
Sufficient ground-based observations of red sprites, especially that with coordinated measurement of broadband lightning sferics to quantify the strength of parent lightning strokes, are still lacking in many places around the world. For negative CG flashes spawned by continental thunderstorms, they are known to usually deposit the in-cloud negative charge through several discrete strokes, and the number of CG strokes contained in individual CG flashes is referred to as “multiplicity.” Will the negative CG flashes generated by oceanic thunderstorms, on a statistical manner, exhibit a substantial difference from those produced by continental thunderstorms?
It also remains necessary to examine the characteristic time scale of impulse charge transfer for both negative and positive CG strokes. The existing work indicates that the charge transfer of negative sprite-producing CG stroke appears to be significantly more impulsive than their positive counterparts. Will the negative CG strokes produced by oceanic thunderstorms be less impulsive that produced by continental thunderstorms?
According to the ISUAL observations in 2004–2016, the maritime continent (MC) in South China Sea is a broad prolific region of red sprites and halos (as shown in Figure 1). However, the ground-based observations are relatively scarce in this region. Therefore, it is highly desired to conduct coordinated ground-based campaigns regarding discharge properties of lightning strokes around the maritime continent, including the coastal regions of South China. Wang et al. (2021c) reported some preliminary results of sprite observations over tropical thunderstorms from a station located in Malacca, Malaysia, and only a handful of red sprites produced by positive CG strokes have been recorded from this station.
Concluding remarks
By combining the ISUAL observations and existing ground-based observations, there have been sufficient evidence to show that there is a considerable difference between continental and oceanic thunderstorms in posing electrical stress on the middle atmosphere. In particular, continental thunderstorms mainly impact on middle atmosphere with positive CG strokes, whereas oceanic thunderstorms are mainly through negative CG strokes. Primarily due to the lack of sufficient ground-based platforms, transient luminous events (TLEs) produced by oceanic thunderstorms are substantially less studied than their continental counterparts. However, there is increasing evidence, from both space-borne and ground-based observations, showing that red sprites and halos produced by negative CG strokes could be very popular in the ocean, where thunderstorms very likely bear some features that are favorable for spawning negative CG strokes with relatively large impulse charge transfer to the seawater. This is partially in line with the abundance of high peak-current negative strokes indicated by various lightning detection networks, but it remains necessary to explore the exact cause of enhanced peak current for negative CG strokes in the ocean.
Both ground-based and space-borne observations of TLEs are important to further investigate the exact physical mechanism for the enhancement of negative CG strength in the ocean. Although the observations from space over years reveal some peculiarities of sprite genesis over oceanic thunderstorms, the underlying mechanism remains to be investigated through ground observations by combining with concurrent measurements regarding the lightning morphology and time-resolved charge transfer of causative CG strokes. In particular, it is desired to conduct more ground-based observations of TLEs over the maritime continent in South China Sea. Some general understandings on the difference in the typical charge structure between oceanic and continental thunderstorms could also be promoted by examining the remote sensing data of satellites.
Author contributions
Conceptualization and design of study, GL; Data Collection, GL, K-MP, FL, and XZ; Data analysis and interpretation, YW, MZ, K-MP, and HR; Writing and preparation of original draft, GL, YW, and HR; Funding acquisition, GL. All authors contributed to article revision, read and approved the submitted version.
Funding
This work was supported by National Key R&D Program of China (No. 2019YFC1510103), the CAS Project of Stable Support for Youth Team in Basic Research Field (No. YSBR-018), Natural Science Foundation of China (Nos. 41875006 and U1938115), the Chinese Meridian Project, and the International Partnership Program of Chinese Academy of Sciences (No. 183311KYSB20200003).
Acknowledgments
We truly appreciate the persistent efforts from many amateurs in China, whose contributions substantially boost the progress in the understanding on the difference between continental and oceanic thunderstorms in driving the transient luminous events. We acknowledge for the data resources from National Space Science Data Center, National Science and Technology Infrastructure of China (https://www.nssdc.ac.cn).
Conflict of interest
Author XZ was employed by Guangzhou Power Grid Co, Ltd.
The remaining authors declare that the research was conducted in the absence of any commercial or financial relationships that could be construed as a potential conflict of interest.
Publisher’s note
All claims expressed in this article are solely those of the authors and do not necessarily represent those of their affiliated organizations, or those of the publisher, the editors and the reviewers. Any product that may be evaluated in this article, or claim that may be made by its manufacturer, is not guaranteed or endorsed by the publisher.
References
Abarca, S. F., Corbosiero, K. L., and Galarneau, T. J. (2010). An evaluation of the worldwide lightning location network (WWLLN) using the national lightning detection network (NLDN) as ground truth. J. Geophys. Res. 115, D18206. doi:10.1029/2009JD013411
Adachi, T., Fukunishi, H., Takahashi, Y., and Sato, M. (2004). Roles of the EMP and QE field in the generation of columniform sprites. Geophys. Res. Lett. 31, L04107. doi:10.1029/2003GL019081
Barrington-Leigh, C. P., Inan, U. S., Stanley, M., and Cummer, S. A. (1999). Sprites triggered by negative lightning discharges. Geophys. Res. Lett. 26, 3605–3608. doi:10.1029/1999gl010692
Boeck, W. L., Vaughan, O. H., Blakeslee, R., Vonnegut, B., and Brook, M. (1992). Lightning induced brightening in the airglow layer. Geophys. Res. Lett. 19, 99–102. doi:10.1029/91gl03168
Boggs, L. D., Liu, N., Splitt, M., Lazarus, S., Glenn, C., Rassoul, H., et al. (2016). An analysis of five negative sprite-parent discharges and their associated thunderstorm charge structures. J. Geophys. Res. Atmos. 121, 759–784. doi:10.1002/2015JD024188
Chen, A. B.-C., Su, H.-T., and Hsu, R.-R. (2014). Energetics and geographic distribution of elve-producing discharges. J. Geophys. Res. Space Phys. 119, 1381–1391. doi:10.1002/2013JA019470
Chen, A. B., Kuo, C. L., Lee, Y. J., Su, H. T., Hsu, R. R., Chern, J. L., et al. (2008). Global distributions and occurrence rates of transient luminous events. J. Geophys. Res. 113, A08306. doi:10.1029/2008JA013101
Chern, R. J., Lin, S., and Wu, A. (2015). Ten-year transient luminous events and Earth observations of FORMOSAT-2. Acta Astronaut. 112, 37–47. doi:10.1016/j.actaastro.2015.02.030
Chou, C.-C., Dai, J., Kuo, C.-L., and Huang, T.-Y. (2016). Simultaneous observations of storm-generated sprite and gravity wave over Bangladesh. J. Geophys. Res. Space Phys. 121, 9222–9233. doi:10.1002/2016JA022554
Christian, H. J., Koshak, W. J., Hall, J. M., Driscoll, K. T., Boeck, W. L., Buechler, D., et al. (2003). Global frequency and distribution of lightning as observed from space by the Optical Transient Detector. J. Geophys. Res. 108 (D1), 4005. doi:10.1029/2002JD002347
Chronis, T., Koshak, W., and McCaul, E. (2016). Why do oceanic negative cloud-to-ground lightning exhibit larger peak current values? J. Geophys. Res. Atmos. 121, 4049–4068. doi:10.1002/2015JD024129
Contreras-Vidal, L., Sonnenfeld, R. G., da Silva, C. L., McHarg, M. G., Jensen, D., Harley, J., et al. (2021). Relationship between sprite current and morphology. JGR. Space Phys. 126, e2020JA028930. doi:10.1029/2020JA028930
Cummer, S. A., Frey, H. U., Mende, S. B., Hsu, R.-R., Su, H.-T., Chen, A. B., et al. (2006b). Simultaneous radio and satellite optical measurements of high-altitude sprite current and lightning continuing current. J. Geophys. Res. 111, A10315. doi:10.1029/2006JA011809
Cummer, S. A., Inan, U. S., Bell, T. F., and Barrington-Leigh, C. P. (1998). ELF radiation produced by electrical currents in sprites. Geophys. Res. Lett. 25 (8), 1281–1284. doi:10.1029/98GL50937
Cummer, S. A., Jaugey, N., Li, J., Lyons, W. A., Nelson, T. E., and Gerken, E. A. (2006a). Submillisecond imaging of sprite development and structure. Geophys. Res. Lett. 33, L04104. doi:10.1029/2005GL024969
Cummer, S. A., and Lyons, W. A. (2005). Implications of lightning charge moment changes for sprite initiation. J. Geophys. Res. 110, A04304. doi:10.1029/2004JA010812
Cummer, S. A., Lyons, W. A., and Stanley, M. A. (2013). Three years of lightning impulse charge moment change measurements in the United States. J. Geophys. Res. Atmos. 118, 5176–5189. doi:10.1002/jgrd.50442
Frey, H. U., Mende, S. B., Cummer, S. A., Li, J., Adachi, T., Fukunishi, H., et al. (2007). Halos generated by negative cloud-to-ground lightning. Geophys. Res. Lett. 34, L18801. doi:10.1029/2007GL030908
Füllekrug, M., and Constable, S. (2000). Global triangulation of intense lightning discharges. Geophys. Res. Lett. 27 (3), 333–336. doi:10.1029/1999gl003684
Füllekrug, M., Price, C., Yair, Y., and Williams, E. R. (2002). <i>Letter to the Editor</i> Intense oceanic lightning. Ann. Geophys. 20, 133–137. doi:10.5194/angeo-20-133-2002
Hager, W. W., Sonnenfeld, R. G., Feng, W., Kanmae, T., Stenbaek-Nielsen, H. C., McHarg, M. G., et al. (2012). Charge rearrangement by sprites over a north Texas mesoscale convective system. J. Geophys. Res. 117, D22101. doi:10.1029/2012JD018309
Han, Y., Luo, H., Wu, Y., Zhang, Y., and Dong, W. (2021). Cloud ice fraction governs lightning rate at a global scale. Commun. Earth Environ. 2, 157. doi:10.1038/s43247-021-00233-4
Hiraki, Y., and Fukunishi, H. (2006). Theoretical criterion of charge moment change by lightning for initiation of sprites. J. Geophys. Res. 111, A11305. doi:10.1029/2006JA011729
Hiraki, Y. (2010). Phase transition theory of sprite halo. J. Geophys. Res. 115, A00E20. doi:10.1029/2009JA014384
Hu, W., Cummer, S. A., Lyons, W. A., and Nelson, T. E. (2002). Lightning charge moment changes for the initiation of sprites. Geophys. Res. Lett. 29 (8), 120. doi:10.1029/2001GL014593
Huang, E. E., Williams, R., Boldi, S., Heckman, W., Lyons, M., Taylor, T., et al. (1999). Criteria for sprites and elves based on Schumann resonance observations. J. Geophys. Res. 104 (D14), 16943–16964. doi:10.1029/1999JD900139
Huang, S.-M., Hsu, R.-R., Lee, L.-J., Su, H.-T., Kuo, C.-L., Wu, C.-C., et al. (2012). Optical and radio signatures of negative gigantic jets: Cases from Typhoon Lionrock (2010). J. Geophys. Res. 117, A08307. doi:10.1029/2012JA017600
Huang, A., Lu, G., Yue, J., Lyons, W. A., Lucena, F., Lyu, F., et al. (2018b). Observations of red sprites above hurricane Matthew. Geophys. Res. Lett. 45, 13158–13165. doi:10.1029/2018GL079576
Huang, A., Lu, G., Zhang, H., Liu, F., Fan, Y., Zhu, B., et al. (2018a). Locating parent lightning strokes of sprites observed over a mesoscale convective system in Shandong Province, China. Adv. Atmos. Sci. 35, 1396–1414. doi:10.1007/s00376-018-7306-4
Huang, A., Yang, J., Cummer, S. A., Lyu, F., and Liu, N. (2021). Examining the capacity of hurricane Matthew (2016) in spawning halo/sprite-producible lightning strokes during its lifetime. Geophys. Res. Atmos. 126, e2021JD035097. doi:10.1029/2021JD035097
Hutchins, M. L., Holzworth, R. H., Rodger, C. J., and Brundell, J. B. (2012). Far-field power of lightning strokes as measured by the world wide lightning location network. J. Atmos. Ocean. Technol. 29, 1102–1110. doi:10.1175/jtech-d-11-00174.1
Hutchins, M. L., Holzworth, R. H., Virts, K. S., Wallace, J. M., and Heckman, S. (2013). Radiated VLF energy differences of land and oceanic lightning. Geophys. Res. Lett. 40, 2390–2394. doi:10.1002/grl.50406
Kuo, C.-L., Huang, T.-Y., Hsu, C.-M., Sato, M., Lee, L.-C., and Lin, N.-H. (2021). Resolving elve, halo and sprite halo images at 10, 000 fps in the taiwan 2020 campaign. Atmosphere 12, 1000. doi:10.3390/atmos12081000
Kuo, C. L., Williams, E., Bor, J., Lin, Y. H., Lee, L. J., Huang, S. M., et al. (2013). Ionization emissions associated with N2+ 1N band in halos without visible sprite streamers. J. Geophys. Res. Space Phys. 118, 5317–5326. doi:10.1002/jgra.50470
Li, J., and Cummer, S. (2011). Estimation of electric charge in sprites from optical and radio observations. J. Geophys. Res. 116, A01301. doi:10.1029/2010JA015391
Li, J., Cummer, S., Lu, G., and Zigoneanu, L. (2012). Charge moment change and lightning-driven electric fields associated with negative sprites and halos. J. Geophys. Res. 117, A09310. doi:10.1029/2012JA017731
Liu, N. Y., Dwyer, J. R., and Cummer, S. A. (2017). Elves accompanying terrestrial gamma ray flashes. J. Geophys. Res. Space Phys. 122 (10), 10563–10576. doi:10.1002/2017JA024344
Lu, G., Cummer, S. A., Blakeslee, R. J., Weiss, S., and Beasley, W. H. (2012). Lightning morphology and impulse charge moment change of high peak current negative strokes. J. Geophys. Res. 117, D04212. doi:10.1029/2011JD016890
Lu, G., Cummer, S. A., Chen, A. B., Lyu, F., Huang, S., Hsu, R. R., et al. (2017). Analysis of lightning strokes associated with sprites observed by ISUAL in the vicinity of North America. Terr. Atmos. Ocean. Sci. 28 (4), 583–595. doi:10.3319/TAO.2017.03.31.01
Lu, G., Cummer, S. A., Li, Jingbo, Han, Feng, Blakeslee, Richard J., and Christian, Hugh J. (2009). Charge transfer and in-cloud structure of large-charge-moment positive lightning strokes in a mesoscale convective system. Geophys. Res. Lett. 36, L15805. doi:10.1029/2009GL038880
Lu, G., Cummer, S. A., Lyons, W. A., and Krehbiel, P. R. (2011). Lightning development associated with two negative gigantic jets. Geophys. Res. Lett. 38, L12801. doi:10.1029/2011GL047662
Lu, G., Cummer, S. A., Li, J., Zigoneanu, L., and Lyons, W. A. (2013). Coordinated observations of sprites and in-cloud lightning flash structure. J. Geophys. Res. 118, 1–26. doi:10.1002/jgrd.50459
Lu, G., Cummer, S. A., Tian, Y., Zhang, H., Lyu, F., Wang, T., et al. (2016). Sprite produced by consecutive impulse charge transfers in a negative stroke: observation and simulation. J. Geophys. Res. Atmos., 121. doi:10.1002/2015JD024644
Lu, G., Yu, B., Cummer, S. A., Chen, A. B., Lyu, F., Liu, F., et al. (2018). On the causative strokes of halos observed by ISUAL in the vicinity of North America. Geophys. Res. Lett. 45, 10781. doi:10.1029/2018GL079594
Lyons, W. A. (1996). Sprite observations above the U.S. High Plains in relation to their parent thunderstorm systems. J. Geophys. Res. 101 (23), 29641–29652. 641–29. doi:10.1029/96jd01866
Mende, S. B., Frey, H. U., Hsu, R. R., Su, H. T., Chen, A. B., Lee, L. C., et al. (2005). D region ionization by lightning-induced electromagnetic pulses. J. Geophys. Res. 110, A11312. doi:10.1029/2005JA011064
Miyasato, R., Taylor, M. J., Fukunishi, H., and Stenbaek-Nielsen, H. C. (2002). Statistical characteristics of sprite halo events using coincident photometric and imaging data. Geophys. Res. Lett. 29 (21), 2033. doi:10.1029/2001GL014480
Newsome, R. T., and Inan, U. S. (2010). Free-running ground-based photometric array imaging of transient luminous events. J. Geophys. Res. 115, A00E41. doi:10.1029/2009JA014834
Pasko, V. P., Inan, U. S., Bell, T. F., and Taranenko, Y. N. (1997). Sprites produced by quasi-electrostatic heating and ionization in the lower ionosphere. J. Geophys. Res. 102, 4529–4561. doi:10.1029/96ja03528
Price, C., Asfur, M., Lyons, W., and Nelson, T. (2002). An improved ELF/VLF method for globally geolocating sprite-producing lightning. Geophys. Res. Lett. 29 (3), 1031. doi:10.1029/2001GL013519
Qin, J., Celestin, S., and Pasko, V. P. (2013). Dependence of positive and negative sprite morphology on lightning characteristics and upper atmospheric ambient conditions. J. Geophys. Res. Space Phys. 118, 2623–2638. doi:10.1029/2012JA017908
Qin, J., Celestin, S., and Pasko, V. P. (2012). Minimum charge moment change in positive and negative cloud to ground lightning discharges producing sprites. Geophys. Res. Lett. 39, L22801. doi:10.1029/2012GL053951
Ren, H., Tian, Y., Lu, G., Zhang, Y., Fan, Y., Jiang, R., et al. (2019). Examining the influence of current waveform on the lightning electromagnetic field at the altitude of halo formation. J. Atmos. Sol. Terr. Phys. 189, 114–122. doi:10.1016/j.jastp.2019.04.010
Ren, H., Lu, G., Cummer, S. A., Peng, K.-M., Lyons, W. A., Liu, F., et al. (2021). Comparison between high-speed video observation of sprites and broadband sferic measurements. Geophys. Res. Lett. 48, e2021GL093094. doi:10.1029/2021GL093094
Said, R. K., Cohen, M. B., and Inan, U. S. (2013). Highly intense lightning over the oceans: Estimated peak currents from global GLD360 observations. J. Geophys. Res. Atmos. 118, 6905–6915. doi:10.1002/jgrd.50508
Sato, M., and Fukunishi, H. (2003). Global sprite occurrence locations and rates derived from triangulation of transient Schumann resonance events. Geophys. Res. Lett. 30 (16), 1859. doi:10.1029/2003GL017291
Sato, M., Ushio, T., Morimoto, T., Kikuchi, M., Kikuchi, H., Adachi, T., et al. (2015). Overview and early results of the global lightning and sprite measurements mission. J. Geophys. Res. Atmos. 120, 3822–3851. doi:10.1002/2014JD022428
Stanley, M., Krehbiel, P., Brook, M., Moore, C., Rison, W., and Abrahams, B. (1999). High speed video of initial sprite development. Geophys. Res. Lett. 26, 3201–3204. doi:10.1029/1999gl010673
Stenbaek-Nielsen, H. C., Moudry, D. R., Wescott, E. M., Sentman, D. D., and Sabbas, F. T. S. (2000). Sprites and possible mesospheric effects. Geophys. Res. Lett. 27, 3829–3832. doi:10.1029/2000gl003827
Su, H.-T., Hsu, R.-R., Chen, A. B., Lee, Y.-J., and Lee, L. C. (2002). Observation of sprites over the Asian continent and over oceans around Taiwan. Geophys. Res. Lett. 29 (4), 1044. doi:10.1029/2001gl013737
Surkov, V. V., and Hayakawa, M. (2020). Progress in the study of transient luminous and atmospheric events: A review. Surv. Geophys. 41, 1101–1142. doi:10.1007/s10712-020-09597-2
Taylor, M. J., Bailey, M. A., Pautet, P. D., Cummer, S. A., Jaugey, N., Thomas, J. N., et al. (2008). Rare measurements of a sprite with halo event driven by a negative lightning discharge over Argentina. Geophys. Res. Lett. 35, L14812. doi:10.1029/2008GL033984
University of Science and Technology of China Transient luminous events over high-impact thunderstorms systems. V1.0. Beijing: National Space Science Data Center. doi:10.12176/01.05.00070-V01.2021-09-25
van der Velde, O. A., Lyons, W. A., Nelson, T. E., Cummer, S. A., Li, J., and Bunnell, J. (2007). Analysis of the first gigantic jet recorded over continental North America. J. Geophys. Res. 112, D20104. doi:10.1029/2007JD008575
Wang, Y., Lu, G., Cummer, S. A., Lucena, F., Cohen, M., Ren, H., et al. (2021b). Ground observation of negative sprites over a tropical thunderstorm as the embryo of hurricane Harvey (2017). Geophys. Res. Lett. 48, e2021GL094032. doi:10.1029/2021GL094032
Wang, Y., Lu, G., Ma, M., Liu, F., Zhu, B., Zhou, R., et al. (2019). Triangulation of red sprites observed above a mesoscale convective system in North China. Earth Planet. Phys. 3, 111–125. doi:10.26464/epp2019015
Wang, Y., Lu, G., Peng, K.-M., Cheng, Z., Wang, R., Ahmad, M. R., et al. (2021c). Observations of red sprites in Melacca area in 2019 and analyses of parent thunderstorms [J]. J. Trop. Meteorology 37 (3), 370–380. doi:10.16032/j.issn.1004-4965.2021.036
Wang, Y., Lu, G., Peng, K.-M., Ma, M., Cummer, S. A., Chen, A. B., et al. (2021a). Space-based observation of a negative sprite with an unusual signature of associated sprite current. JGR. Atmos. 126, e2020JD033686. doi:10.1029/2020JD033686
Williams, E., Downes, E., Boldi, R., Lyons, W., and Heckman, S. (2007). Polarity asymmetry of sprite-producing lightning: A paradox? Radio Sci. 42, RS2S17. doi:10.1029/2006RS003488
Williams, E., Kuo, C. L., Bor, J., Satori, G., Newsome, R., Adachi, T., et al. (2012). Resolution of the sprite polarity paradox: The role of halos. Radio Sci. 47, RS2002. doi:10.1029/2011RS004794
Keywords: red sprites and halos, oceanic thunderstorms, cloud-to-ground (CG) lightning strokes, impulse charge moment change (iCMC), charge structure of thunderstorm
Citation: Lu G, Peng K-M, Xian T, Wang Y, Ren H, Tian Y, Zhang M, Lucena F, Zhang X, Huang X and Cheng Z (2022) Contrast between continental and oceanic thunderstorms in producing red sprites and halos. Front. Earth Sci. 10:961566. doi: 10.3389/feart.2022.961566
Received: 04 June 2022; Accepted: 08 August 2022;
Published: 12 September 2022.
Edited by:
Guihua Wang, Fudan University, ChinaReviewed by:
Fanchao Lyu, Chinese Academy of Meteorological Sciences, ChinaYongbo Tan, Nanjing University of Information Science and Technology, China
Copyright © 2022 Lu, Peng, Xian, Wang, Ren, Tian, Zhang, Lucena, Zhang, Huang and Cheng. This is an open-access article distributed under the terms of the Creative Commons Attribution License (CC BY). The use, distribution or reproduction in other forums is permitted, provided the original author(s) and the copyright owner(s) are credited and that the original publication in this journal is cited, in accordance with accepted academic practice. No use, distribution or reproduction is permitted which does not comply with these terms.
*Correspondence: Gaopeng Lu, Z2FvcGVuZ2x1QGdtYWlsLmNvbQ==