- Departamento de Estratigrafía y Paleontología, Facultad de Ciencias, Universidad de Granada, Granada, Spain
Serravallian (middle Miocene) coralline algal assemblages at the southern margin of the Guadalquivir Basin (southern Spain) occur as rhodoliths preserved in situ or very close to their growth habitats (autochthonous–parautochthonous assemblages) and also as reworked remains (allochthonous assemblages). The former assemblages consist of spherical rhodoliths built up by encrusting to warty plants and also of large fragments of branches, whereas the latter are mostly unrecognizable small fragments occurring in channeled packstone–grainstone beds. In both cases, the most abundant components are members of the order Hapalidiales (Mesophyllum roveretoi, Mesophyllum sp., Lithothamnion ramosissimum, and less frequently Phymatolithon group calcareum and Lithothamnion group corallioides). Laminar growths of Lithoporella minus and branches of Spongites group fruticulosus and Sporolithon sp. occur very rarely. There are also anecdotal records of Subterraniphyllum thomasii, extending its upper stratigraphic range up to the Serravallian in the western Tethys. The autochthonous–parautochthonous coralline algal assemblages formed in a middle ramp, at several tens of meters of water depth, as suggested by the dominance of Hapalidiales. The allochthonous assemblages represent fragments of coralline algae derived from the middle ramp and redeposited in deeper settings, most likely the outer ramp, due to storm-generated currents.
Introduction
The Serravallian (middle Miocene) is a time interval of global temperature decline following the middle Miocene climatic transition. Superimposed to the climatic trend, deep paleogeographic transformations affected the Mediterranean during the middle Miocene, mostly due to tectonism associated with the relative movements of the African, Arabian, and Eurasian Plates: 1) restriction of the Paratethys to become a megalake during the late Miocene (Rögl, 1998; Meulenkamp and Sissingh, 2003; Palcu et al., 2011), 2) disconnection of the Mediterranean from the Indian Ocean (Rögl, 1998; Cornacchia et al., 2021), and 3) folding and thrusting at the western Mediterranean that led to the uplifting of the Betic-Rif mountain belt (Galindo-Zaldívar et al., 2000; Pedrera et al., 2012; Ruiz-Costán et al., 2012). The interplay of the climatic evolution, tectonic–paleogeographic changes, and sea-level fluctuations during the middle Miocene had critical effects on the paleoceanography of the Mediterranean, which, in turn, controlled carbonate production (Cornacchia et al., 2021).
In the Betic Cordillera, several carbonate ramps with varying siliciclastic supplies developed associated with the newly emerging reliefs during the Serravallian. These carbonate deposits, although widespread in the region, are poorly known, except for the Serravallian carbonate ramp located at the western end of the North-Betic strait (Braga et al., 2010a), which connected the Mediterranean Basin with the Atlantic Ocean at the front of the uplifting Betic orogen (Martín et al., 2009). Further west–southwest, coeval Serravallian carbonates occur at the southern margin of the Guadalquivir Basin, the foreland basin of the Betic Cordillera. They are heterozoan carbonates dominated by coralline algae, larger benthic foraminifera (LBF), echinoderms, bryozoans, serpulids, and mollusks.
In this study, we investigated the coralline algae in Serravallian limestones at the southern margin of the Guadalquivir Basin that crop out in the vicinity of Jimena, Bedmar, and Jódar (Jaén Province, SE Spain). The coralline algae are major biotic components in these carbonates, occurring in densely packed rhodolith beds and as dispersed fragments together with other bioclasts. Rhodolith coralline algal composition, shape, and size are valuable tools to infer the paleoenvironmental contexts in which they grew (e.g., Aguirre et al., 2017). In a regional context, the paleogeography and tectonic scenario at the southern margin of the Guadalquivir Basin during the middle Miocene played an important role in controlling carbonate deposition and the main biotic carbonate producers. At last, rhodolith-dominated deposits were widespread in the Mediterranean during the middle Miocene (Braga, 2017; Cornacchia et al., 2021). Therefore, the study of the Serravallian coralline algae and rhodolith beds, together with the analysis of their lithofacies and stratal geometry, is key to understanding the significance of this type of deposit in carbonate production within the western Mediterranean.
Geographic location and geological settings
The Serravallian carbonates were studied in three areas of the Jaén Province (SE Spain): 1) Cerro de la Atalaya, a hill immediately south of Jimena, 2) southeast of Serrezuela de Jódar, and 3) Cerro Hernando–Cerro de Fontanar, east of Jódar (Figure 1).
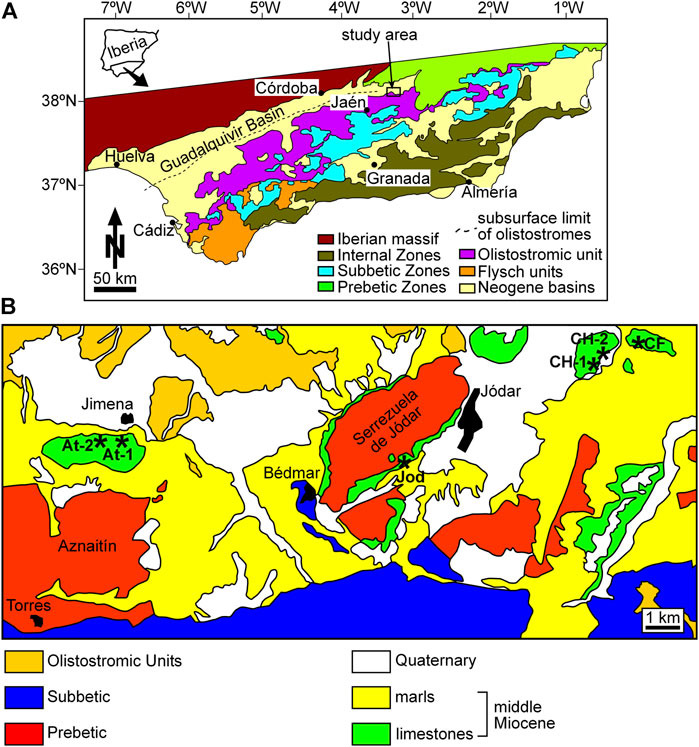
FIGURE 1. (A) geological map of the Betic Cordillera with indication of the study area NE Jaén. (B) geological map of the study area. Asterisks indicate the location of the stratigraphic columns.
These areas are located at the southern margin of the Guadalquivir Basin, the foreland basin of the Betic Cordillera (Figure 1A). This is a mountain belt in the western Mediterranean Alpine orogen that can be divided into three main tectonic domains: 1) the Internal Zones, mostly made up of metamorphic rocks; 2) the External Zones, consisting of the thrusted and folded sediments accumulated at the southern margin of the Iberian Plate; and 3) the Campo de Gibraltar or Flysch units, formed in deep-water fan systems in areas between the two former domains (García-Hernández et al., 1980) (Figure 1A). The External Zones can be divided, in turn, into the Prebetic Zones, the paleogeographic area closest to the continent, and the Subbetic Zones, corresponding to more distal settings further to the south.
During the formation of the Betic Cordillera, the overgrowth of the orogenic wedge led to the flexure of the crust underneath, originating the Guadalquivir foreland Basin between the passive margin to the north and the active margin of the orogen to the south (Perconing, 1960-62; García-Castellanos et al., 2002). Large amounts of allochthonous deposits derived from the orogen frontal wedge, the Olistostrome unit (Figure 1A), were transported into the Guadalquivir Basin (Perconing, 1960-62; Roldán, 1995, 2008; Berasátegui et al., 1998; Rodríguez-Fernández et al., 2012, 2013). The study limestones unconformably overlies chaotic deposits of the Olistostrome unit and Mesozoic carbonates of the Prebetic Zones. They are folded and faulted due to the northward thrusting of the Betic front units (García-Tortosa et al., 2008; Sanz de Galdeano et al., 2013). Marls in the lower part of the study sections contain rich planktonic foraminifer assemblages of the late Serravallian, attributable to biozone M9 of Wade et al. (2011), including Fohsella fohsi, Sphaeroidinellopsis spp., Paragloborotalia siakensis, Globorotalia praemenardii, and Globigerina bulloides.
Materials and methods
Six sections were logged in the three study areas (Figure 1B). One hundred and five limestone samples were collected from the distinguished lithofacies intervals throughout sections. One rock sample was collected in each of these intervals, except in the rhodolith beds, where several samples were taken in the selected sampling sites. Ultrathin sections were prepared from all collected samples to analyze their microfacies and coralline algal assemblages. Relative abundance of bioclastic and terrigenous components was estimated using the comparison charts of Baccelle and Bosellini (1956). Coralline algae were identified at the lowest taxonomic level possible. Taxonomic identification of fragments was difficult because, in most cases, there were insufficient diagnostic characters preserved. The relative species abundance in lithified rhodolith beds is difficult to estimate as any quantification of component proportions had to be performed in thin sections under the microscope. The collection of rock samples and thin-section preparation is conditioned by several factors that prevent a simple random or systematic sampling and, therefore, bias the results. Taxonomic subdivisions follow the most recent molecular phylogenetic schemes (Peña et al., 2020; Jeong et al., 2021). The cementation of the study rocks precluded the extraction of isolated rhodoliths; therefore, the rhodolith shape has been analyzed in 2D sections at the outcrops. Rhodolith description (external shapes, algal growth forms, inner arrangement, etc.) and rhodolith bed characterization follow the terminology reviewed by Aguirre et al. (2017).
Stratigraphic sections
Both in the Cerro de la Atalaya and in the Cerro Hernando–Cerro Fontanar areas, Serravallian successions show similar stratigraphic patterns, consisting of marls at the base that change upwards to limestones and, finally, to silts and marls (Figure 2A). In the Serrezuela de Jódar area, Serravallian sediments unconformably overlie Cretaceous limestones (Figure 2B).
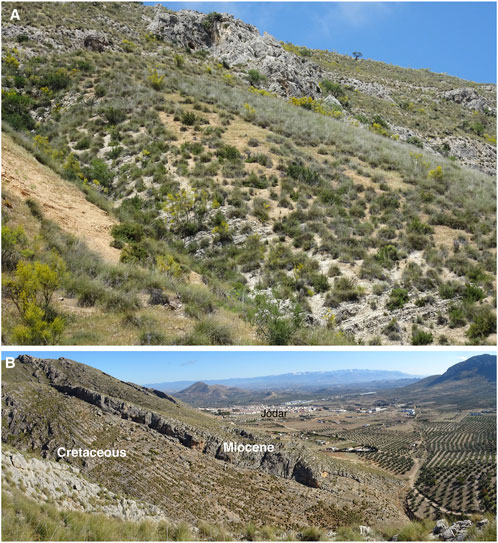
FIGURE 2. (A) panoramic view of the stratigraphic section corresponding to the CH-2 section: Cerro Hernando–Cerro Fontanar area. Note the whitish marls in the lower part of the section and the carbonates on top of the marls. (B) panoramic view of the Serravallian carbonates unconformably overlying Cretaceous limestones in the Serrezuela de Jódar area.
Cerro de la Atalaya
The Serravallian deposits in Cerro de la Atalaya form a northward-verging anticline north of Sierra del Aznaitín, which is made up of Cretaceous limestones of the Prebetic Zones (García-Tortosa et al., 2008; Sanz de Galdeano et al., 2013) (Figure 1B). The lower part of the sections consists of marls rich in planktonic foraminifers. In the transition to the carbonate above, thin planktonic foraminifera-rich packstone beds intercalate with the marls (Figure 3). They change upwards to channeled fine- to coarse-grained packstones, rarely rudstones (Figure 3). The beds are decimeters up to a meter in thickness. The major components are benthic foraminifera (LBF and small forms), bryozoans, coralline algae, echinoderms, and bivalves, with varying proportions of planktonic foraminifera. Siliciclastic content, consisting of fine angular quartz grains, ranges from 10 to 50%. Above the channeled deposits, the coralline algal content substantially increases. In a lower interval, they occur in rudstone beds, 20–50-cm thick, as branch fragments and small rhodoliths (1–2 cm in diameter). This facies changes upwards to densely packed rhodolith beds (rudstones with rhodoliths embedded in a packstone matrix), up to 2-m thick (Figure 4A). The algal nodules reach up to 17 cm in the longest diameter (Figures 4B and C). The matrix includes LBF, bryozoans, echinoderms, bivalves, Ditrupa worm tubes and other encrusting serpulids, and scarce barnacles. On occasions, planktonic foraminifera are significant. Above the rhodolith beds, the Serravallian sections end with marls rich in planktonic foraminifers (Figure 3).
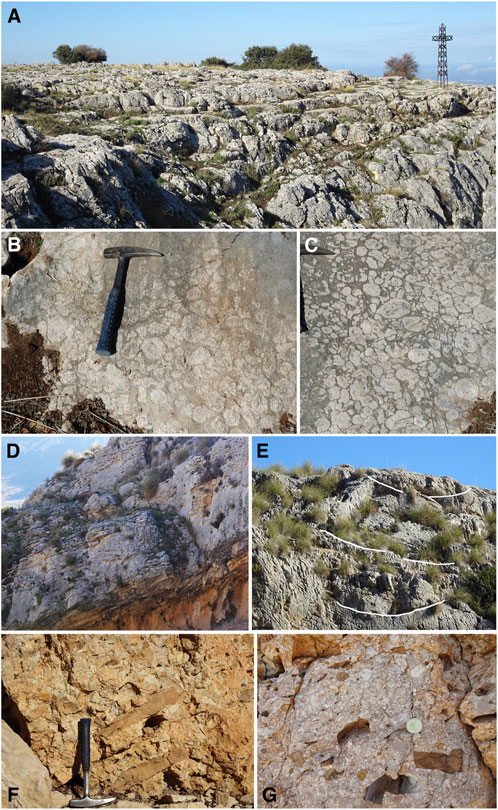
FIGURE 4. (A) plane-parallel densely packed rhodolith beds from the La Atalaya area: At-2 section. (B,C) massive rhodolith beds in the La Atalaya area: At-1 section. (D) trough crossbedding at the lower part of the Serravallian carbonates in the Jódar section. (E) channeled beds in the Jódar section. (F) Breccia including large angular boulders and cobbles in the CF section (Cerro Hernando–Cerro Fontanar area). (G) same breccia bed as in F but including numerous rhodoliths and coralline algal debris (whitish spots).
Serrezuela de Jódar
The Serravallian limestones crop out in a belt surrounding Serrezuela de Jódar and Serrezuela de Bédmar (also called Sierra de Bédmar–Jódar), a series of hills made up of Prebetic Cretaceous limestones (García-Tortosa et al., 2008; Sanz de Galdeano et al., 2013) (Figures 1B and 2B). One stratigraphic section, about 3 km south of Jódar, was logged (Figure 5). The lower part of the limestones consists of centimeter–decimeter-thick packstone–grainstone beds displaying rough trough cross-bedding (Figure 4D). The major components are LBF, bryozoans, echinoderms, bivalves, fragments of coralline algae, and scattered barnacles and serpulids (including Ditrupa). The terrigenous content (angular, fine-grained particles of quartz), although scarce, reaches up to 10%. Higher up in the section, decimeter-thick channeled beds of packstones occur (Figure 4E). They are rich in LBF, echinoderms, and bryozoans. The coralline algal content progressively increases upwards, and rhodoliths become the main components, originating densely packed rhodolith rudstone beds up to several meters in thickness (Figure 5) with a packstone matrix dominated by LBF, bryozoans, echinoderms, and, less frequently, serpulids and bivalves (pectinids and oysters). Above the rhodolith beds, the grain size decreases passing to centimeter–decimeter-thick packstone beds rich in planktonic foraminifera. Silts and marls end the Serravallian succession (Figure 5).
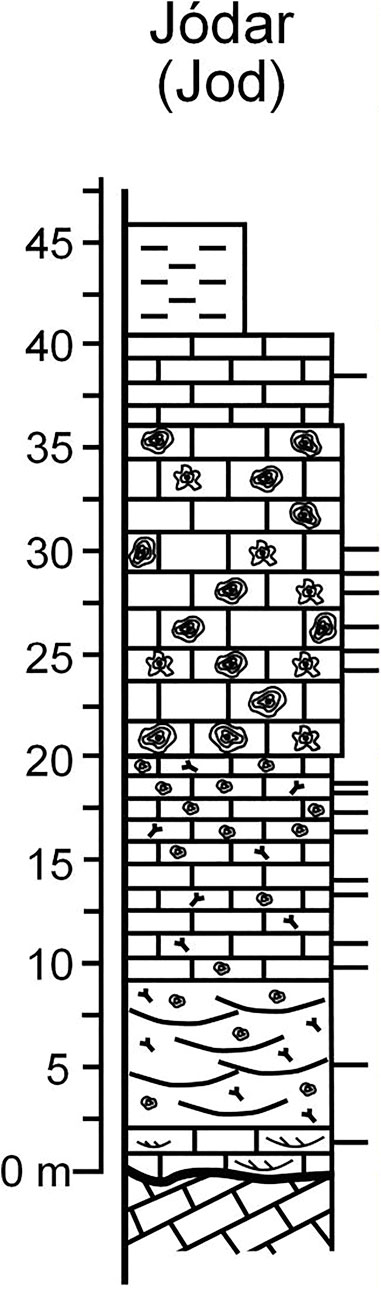
FIGURE 5. Stratigraphic section in the Jódar area (symbols as in Figure 3).
Cerro Hernando–Cerro Fontanar
The Serravallian deposits form the hills named Cerro Hernando and Cerro Fontanar, ENE of Jódar, and unconformably overlie chaotic materials of the Olistostrome unit (Figure 1B). The study carbonates form a homoclinal sequence dipping ∼15° to the NNW in Cerro Hernando and to the E in Cerro Fontanar. The study sections can be divided into two parts: the lower part made up of planktonic foraminifera-bearing marls and the upper part consisting of bioclastic limestones (Figures 2A and 6). The transition between both is gradual, characterized by fine-grained packstone beds, centimeter to decimeter in thickness, intercalated in the marls. The packstone beds are made up of planktonic foraminifer ooze and show a rough parallel lamination. Higher up in the section, marls pass to decimeter-thick plane-parallel bedded packstone rich in planktonic foraminifera with abundant benthic foraminifera as well as fragments of coralline algae, echinoderms, bryozoans, bivalves, and serpulids. Limestones change upward to decimeter–meter-thick channeled beds formed by alternating finer-grained (packstone) and coarser-grained (rudstone) beds up to the top of the Serravallian succession. Some beds show incomplete Bouma sequences, preserving layers A and B and, occasionally, layer C. The proportion of the terrigenous components can reach up to 5% of the rock volume. They are quartz sand grains and angular pebbles to boulders of Mesozoic limestone and sandstone. In the Cerro Fontanar, a 3-m-thick breccia containing cobbles and boulders (up to 1.2 m in diameter) is observed (Figures 4F and G). This breccia contains rhodoliths and abundant coralline algal debris, LBF, and bryozoans with varying amounts of echinoderms, bivalves, serpulids (including Ditrupa), and rare barnacles.
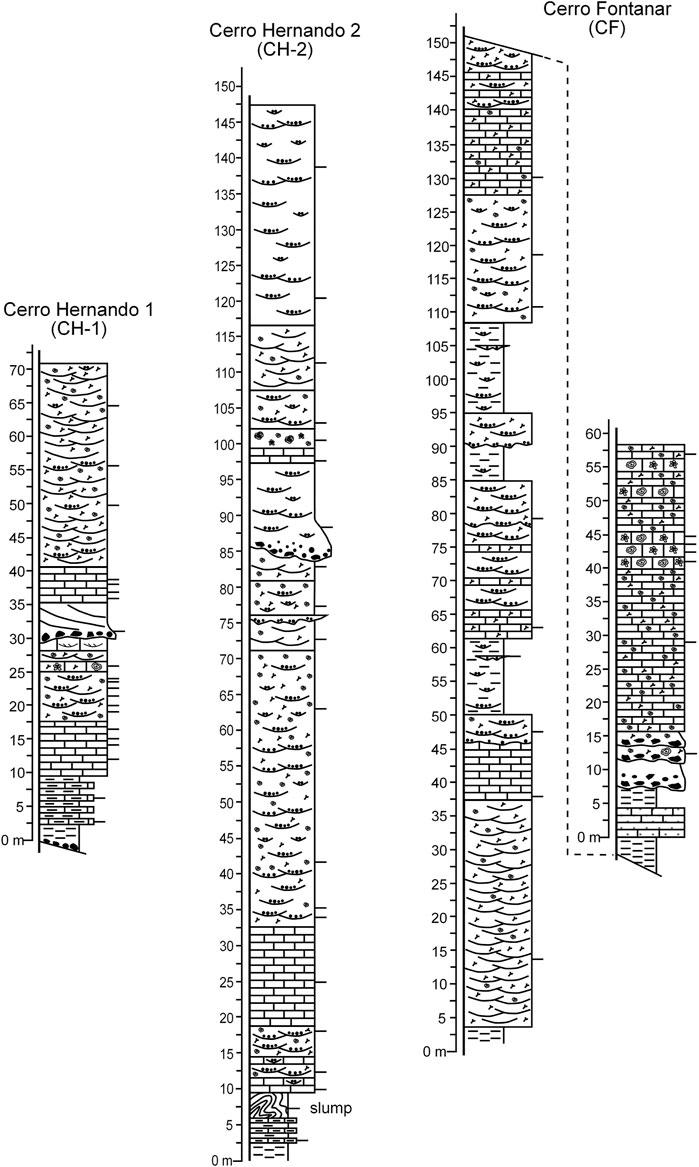
FIGURE 6. Stratigraphic sections in the Cerro Hernando–Cerro Fontanar area (symbols as in Figure 3).
In the upper half of the Serravallian succession, some channeled beds, 25–50-cm thick, contain rhodoliths and fragments of coralline algal branches as the main components, together with angular lithoclasts derived from the basement (Figure 6).
Coralline algal occurrence and taxonomic composition
In the Cerro de la Atalaya and Serrezuela de Jódar areas, rhodoliths occur in loosely to densely packed massive rudstone beds (Figures 7A–D), as well as in channeled facies. In the Cerro Hernando–Cerro Fontanar, rhodoliths are only found in channeled beds (Figures 7E and F). In all cases, rhodoliths are mostly spheroidal in shape with some ellipsoidal nodules. On average, the ratio between the shorter and larger axes is ca. 0.75, indicating the dominance of spheroidal nodules (Figure 8).
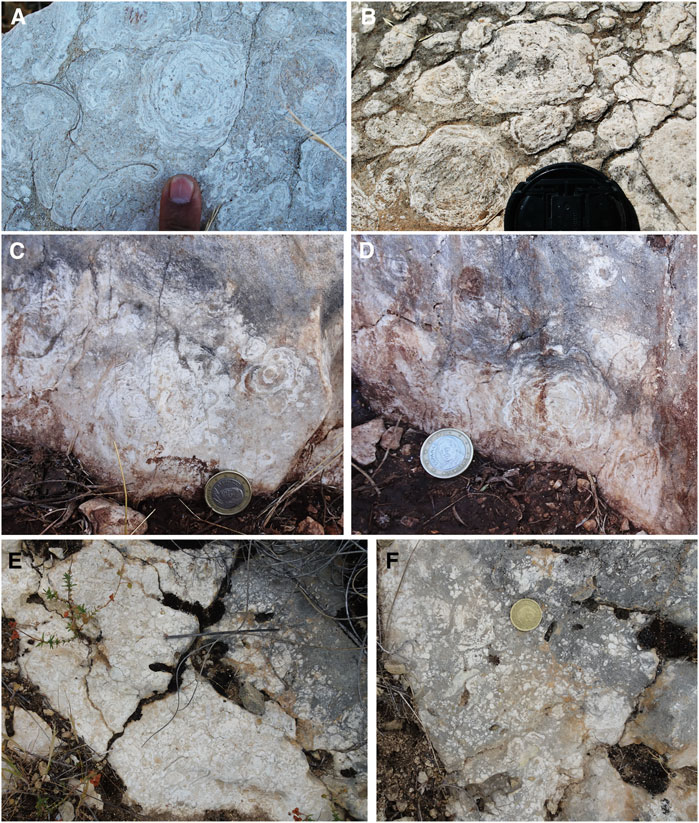
FIGURE 7. (A,B) rhodolith concentrations in the At-2 section (Cerro de la Atalaya area). Rhodoliths are spheroidal in shape, and some of them show an ellipsoidal morphology. Inner algal arrangement is characterized by concentric laminar-encrusting algal thalli. Rarely, i.e., right of the finger in (A), rhodoliths are made up of warty–fruticose algal plants. (C,D) rhodolith rudstones in the Jódar section (Serrezuela de Jódar area). As in Cerro de la Atalaya, rhodoliths are mostly spheroidal in shape and made up of laminar-encrusting algal thalli. (E,F) small rhodoliths and coralline algal fragments observed in the channeled facies of the Cerro Hernando–Cerro Fontanar area. Note that the size of the rhodoliths is smaller than the size of those present in Serrezuela de Jódar and Cerro de la Atalaya.
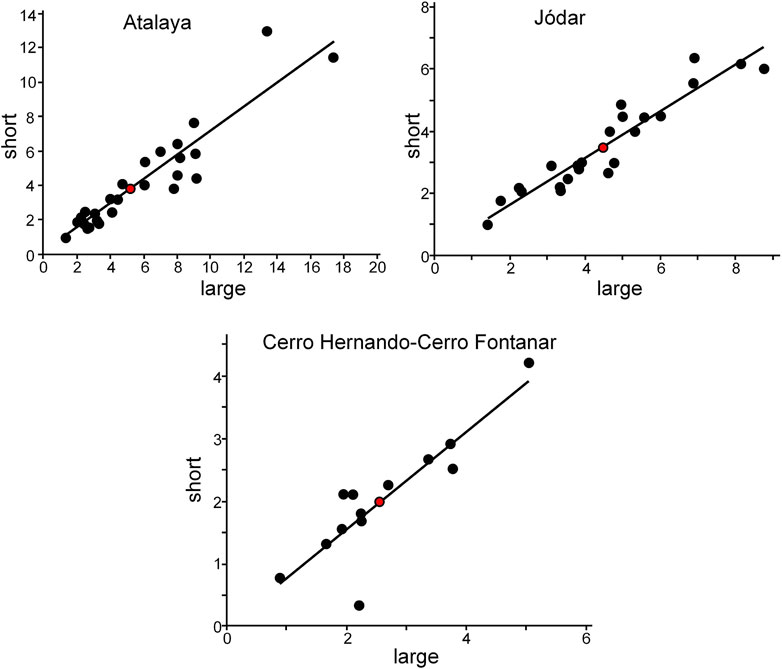
FIGURE 8. Size of the rhodoliths in the three study areas represented as the relationship between large and short axes. Red dots denote the average rhodolith sizes. Note the good adjustment of the size values to a straight line, indicating the dominance of spheroidal morphology. Number of measured rhodoliths: Atalaya, n = 25; Jódar, n = 23; Cerro Hernando–Cerro Fontanar, n = 13.
In the massive rhodolith rudstone beds, rhodoliths attain similar mean sizes both in Cerro de la Atalaya (5.2 × 3.8 cm) and Serrezuela de Jódar (4.5 × 3.5 cm), although the size range of the largest dimension is higher in Cerro de la Atalaya (from 1.3 to 17.4 cm) than in Serrezuela de Jódar (1.4–8.8 cm) (Figure 8).
Rhodoliths show nearly concentric inner arrangements with dominantly encrusting and warty coralline algal growth forms, less frequently fruticose, and small proportions of constructional voids (Figures 7A–D). Rhodoliths are built up by complex intergrowths of corallines with other encrusting organisms, such as benthic foraminifera, bryozoans, serpulids, and, occasionally, barnacles (Figure 9). In a very few cases, encrusting benthic foraminifera are more abundant than coralline algae, forming the so-called for-algaliths (Prager and Gingsburg, 1989) or macroids (Hottinger, 1983; Bassi et al., 2012). The nuclei of rhodoliths are bioclasts, mainly bivalves, bryozoans, coralline algae, and LBF, or lithoclasts.
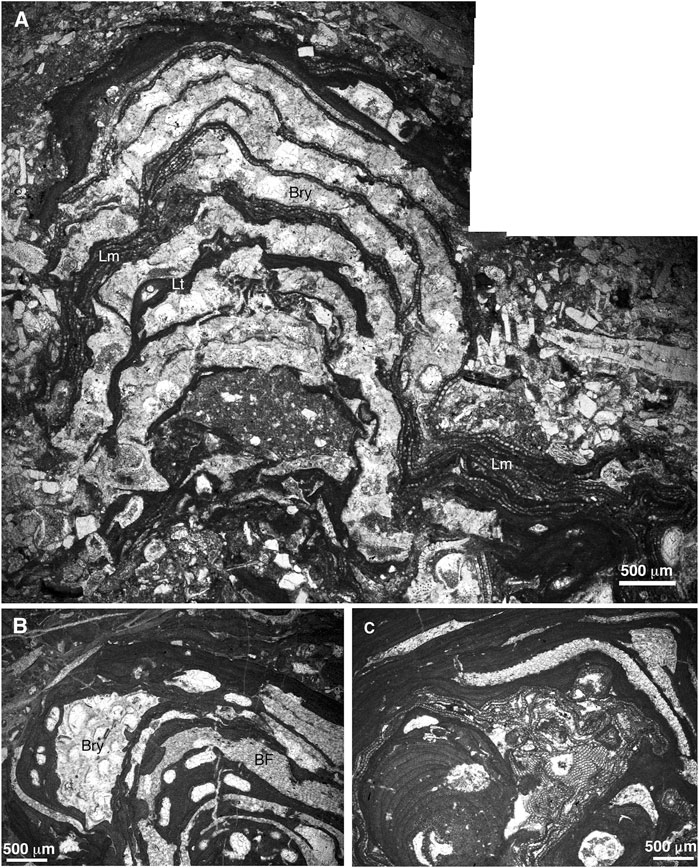
FIGURE 9. Intergrowth of different organisms building up the rhodoliths. (A) laminar-encrusting Lithoporella minus (Lm) and Lithothamnion sp. 1. (Lh) intergrowing with bryozoans (Bry). Sample JOD-5 (Serrezuela de Jódar section). (B) Lithothamnion sp. 1 growing with bryozoan (Bry) and benthic foraminifera (BF). Sample JOD-8ii (Serrezuela de Jódar section). (C) intergrowth of different coralline algal species and BF. Sample JOD-9ii (Serrezuela de Jódar section).
Sixteen species of coralline algae have been identified, with representatives of the three calcified orders Sporolithales, Corallinales, and Hapalidiales (Table 1). However, in terms of taxonomic composition, rhodoliths are generally monospecific or paucispecific, with two to three species. Hapalidiales is the dominant group, with Mesophyllum roveretoi, Mesophyllum sp., and Lithothamnion ramosissimum being the most abundant, followed by Lithothamnion group corallioides and Phymatolithon group calcareum, (Figure 10). Additional Hapalidiales are anecdotal: Lithothamnion sp. 1 and Melobesia sp. (Figures 11A and B). The order Corallinales is scarcely represented, with Spongites group fruticulosus and Lithoporella minus standing out as the most frequent species (Figures 11C and D), with occasional records of Spongites spp., Hydrolithon lemoinei, and very rare fragments of calcified intergenicula of unidentifiable geniculates. It is also worth highlighting the scarce occurrence of Subterraniphyllum thomasii fragments in the lower part of the Cerro Fontanar (Figures 11E–G). At last, Sporolithales are only anecdotally present as branch fragments of Sporolithon sp. (Figure 11H).
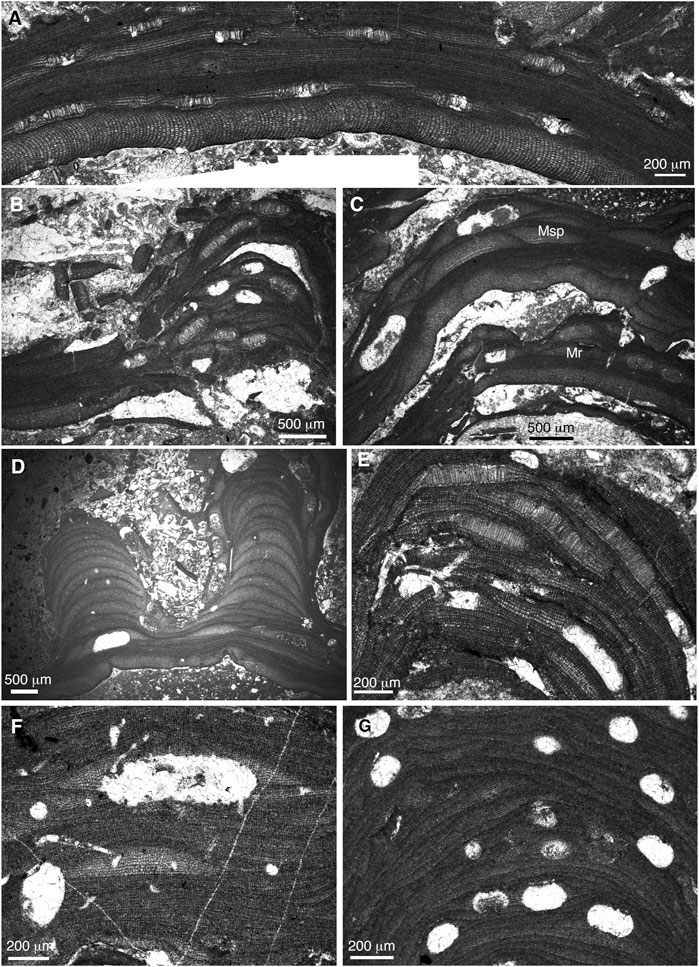
FIGURE 10. (A) encrusting plant of Mesophyllum roveretoi. Sample JIM-CR-3 (Cerro de la Atalaya-2 section). (B) encrusting–warty thallus of M. roveretoi. Sample JOD-7 (Serrezuela de Jódar section). (C) superposition of encrusting thalli of M. roveretoi (Mr) and Mesophyllum sp. (Msp). Sample JIM-CR-4 (Cerro de la Atalaya-2 section). (D) fruticose plant of Mesophyllum sp. Sample JIM-CR-4 (Cerro de la Atalaya-2 section). (E) Lithothamnion ramosissimum. Sample JOD-7i (Serrezuela de Jódar section). (F) Lithothamnion group corallioides. Sample JIM-CR-5i (Cerro de la Atalaya-2 section). (G) Phymatolithon group calcareum. Sample JIM-CR-3 (Cerro de la Atalaya-2 section).
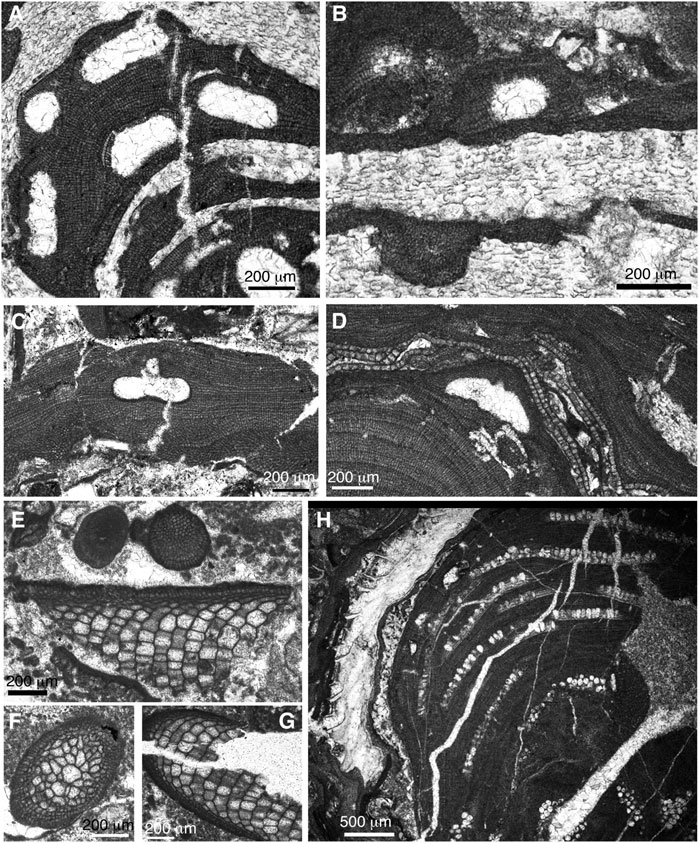
FIGURE 11. (A) Lithothamnion sp. 1. Sample JOD-8ii (Serrezuela de Jódar section). (B) Melobesia sp. Sample JOD-9ii (Serrezuela de Jódar section). (C) Spongites group fruticulosus. Sample CH-1.7 (Cerro Hernando-1 section). (D) Lithoporella minus. Sample JOD-9ii (Serrezuela de Jódar section). (E–G) Subterraniphyllum thomasii. Sample CH-3–1 (Cerro Hernando-1 section). (H) Sporolithon sp. Sample JOD-8 (Serrezuela de Jódar section).
Coralline algae in the packstone channeled lithofacies are mostly abraded fragments of branches and crusts. They occur in highly varying proportions, from 3% up to 50% of the rock volume. Small rhodoliths, from 0.9 to 5 cm in the largest diameter (mean, 2.6 cm) (Figure 8), and broken rhodoliths also occur. They show an inner concentric arrangement of thin encrusting and warty algal growth forms, with very rare fruticose plants. In some cases, the sediment filling up the internal voids of these rhodoliths is different (wackestone) from the sediment surrounding them (packstone), indicating that they are displaced from their growth places.
Most fragments do not show enough taxonomic features to be identified at any taxonomic level. Among the identifiable ones, the most abundant are branch fragments of L. ramosissimum, as well as crusts of M. roveretoi and Mesophyllum sp. On occasions, there are fragments of P. group calcareum, S. group fruticulosus, and Sporolithon sp.
Discussion
Paleoenvironmental interpretation and sedimentary model
In Cerro de la Atalaya and Cerro Hernando–Cerro Fontanar, Serravallian carbonates rest conformably on top of planktonic foraminifera-rich marls and marlstones, which were deposited in basinal settings. The sediments at the transition between the marls and the carbonates represent a gradual facies change, characterized by sediment gravity flow deposits intercalated in the marls.
The overlying channeled packstones are interpreted as sediments reworked during storm events and redeposited offshore in low-relief channels formed by storm rip currents (see below). Similar storm-related deposits were described in Oligocene–Miocene carbonate ramps from southern Italy and Malta (Pedley, 1998). In Cerro Hernando–Cerro Fontanar, channeled beds dominate the Serravallian carbonate successions. Some of them contain abundant rhodoliths and angular lithoclasts, indicating that they were reworked from shallower parts of the ramp.
In the Cerro de la Atalaya and Serrezuela de Jódar areas, densely and loosely packed rhodolith beds overlie the channeled facies. The external algal covers of the rhodoliths are well preserved. In the case of fruticose algal growth forms, they show well-preserved slender branches. In addition, they do not show significant inner erosive surfaces, indicating that rhodoliths grew nearly continuously, without substantial interruptions due to episodic reworking and/or temporal burial that hindered algal growth. These features suggest that rhodoliths formed autochthonous–parautochthonous accumulations (factory facies) in low-energy conditions in the middle ramp. In parallel, in the Mediterranean Sea, present-day rhodolith beds extend from 9 to 150 m of water depth, but they preferentially occur at 30–75-m depth on average (Bressan and Babbini, 2003; Aguilar et al., 2009; Basso et al., 2017; Rendina et al., 2020; Del Río et al., 2022).
The most abundant rhodolith-forming species belong to the order Hapalidiales, Mesophyllum roveretoi, Mesophyllum sp., and Lithothamnion ramosissimum, with accompanying Phymatolithon group calcareum and Lithothamnion group corallioides. In the present-day Mediterranean, the genera Lithothamnion and Phymatolithon preferentially live at tens of meters of water depth, mainly below 30–40-m depth (Basso, 1995, 1998; Peña et al., 2015; Basso et al., 2017; Bracchi et al., 2019; Rendina et al., 2020). Recent Mesophyllum species in the Mediterranean have a wide depth range, from a few meters down to 80–100 m (e.g., Fravega and Vannucci, 1989; Athanasiadis 1999; Athanasiadis and Neto, 2010), but they show the highest abundance at 30–50 m (Hamel and Lemoine, 1952; Basso, 1995). Therefore, the dominant coralline algal association indicates that rhodolith beds formed in middle-ramp settings, most likely at about 40–50 m of water depth. The presence of the serpulid Ditrupa, a calcified worm typically inhabiting in deep-water settings of shelves (Le Loc’h et al., 2008; Aguirre et al., 2015), is consistent with this paleobathymetric interpretation.
As observed in present-day settings, the movement of rhodoliths in deep-water, low-energy settings could be produced either by the action of vagile organisms (Prager and Gingsburg, 1989; Marrack, 1999; Pereira-Filho et al., 2015; Millar and Gagnon, 2018; O’Connell et al., 2020) or by exceptionally intense storm events (Basso and Tomaselli, 1994; Joshi et al., 2017). Some of these high-energy events would rework sediment from shallow settings, including siliciclastic particles of variable sizes and fragments of different organisms. In our study case, storm events transported particles to deeper settings forming the channeled facies. The abraded fragments of coralline algal branches and other organisms, together with the presence of angular terrigenous granules to pebbles, account for the allochthonous nature of the algae. Therefore, the channeled packstone beds represent more distal deposits formed offshore the rhodolith beds and fed by biogenic components produced in the middle ramp. The homoclinal strata geometry observed in the Cerro Hernando–Cerro Fontanar area can be interpreted as the most distal parts of the middle-ramp or upper outer-ramp growth due to the accumulation of these storm-dominated deposits. In the recent Bay of Naples, Toscano et al. (2006) documented a similar rhodalgal facies system, characterized by production areas (rhodolith factories) in shallow ramps connected with offshore-transported rhodoliths in channeled facies.
Rhodoliths in the channeled beds are smaller and better sorted (smaller variance in size) than those present in massive rhodolith beds in Cerro de la Atalaya and Serrezuela de Jódar. These differences in rhodolith size among areas represent a sorting of particles in a proximal–distal transect along the ramp. That is, storm events swept rhodoliths from shallower areas on the ramp, and the smaller ones were able to travel longer distances offshore.
Rhodolith beds in the context of the middle Miocene Mediterranean Sea
During the early–middle Miocene, rhodolith-dominated deposits were globally widespread (Halfar and Mutti, 2005). In the Mediterranean, rhodalgal lithofacies peaked during the Langhian, coinciding with the Miocene climatic optimum and the subsequent mid-Miocene climatic transition, up to the Tortonian, when they started to decline (Braga, 2017). The final closure of the Eastern Mediterranean and Indian Ocean connections during the middle Miocene led to significant paleoceanographic changes and a major shift in carbonate producers in the Mediterranean, with coralline algae being one of the major carbonate producers in carbonate platforms (Pedley, 1998; Cornacchia et al., 2021). In addition, tectonics in the Mediterranean generated shallow carbonate platforms with reduced or no terrigenous supply that promoted widespread development of rhodalgal facies (Braga, 2017).
The autochthonous–parautochthonous rhodolith beds in the study area formed in ramps located at the southeastern margin of the Guadalquivir foreland Basin, in a wide passage connecting the Atlantic and the Mediterranean. Coeval deposits occur further to the northeast, in the Prebetic Zone (Braga et al., 2010a). Here, rhodoliths and loose algal branches, dominated by Lithothamnion, Mesophyllum, and Sporolithon species, as well as bryozoan colonies, formed at several tens of meters of water depth in the middle ramp (Braga et al., 2010a). All these Serravallian ramps formed surrounding newly emerged reliefs as a consequence of the Betic Cordillera uplift.
In parallel, during the middle Miocene, major development of rhodolith beds throughout the entire Mediterranean occurred overall in the middle–outer ramps, from 30 to 80 m of water depth (Studenki, 1979, 1988; Scott and Govean, 1985; Bucur and Filipescu, 1994, 2011; Randazzo et al., 1999; BouDagher-Fadel and Clark, 2006; Ruchonnet and Kindler, 2010; Brandano et al., 2017; Cornacchia et al., 2021). The rhodoliths in these rhodalgal facies are mostly accompanied by large and small benthic foraminifera, mollusks, echinoids, serpulids, and bryozoans (Halfar and Mutti, 2005). On occasions, scattered coral patch-reefs also occur associated with these rhodolith-dominated facies (Pedley, 1979; Bosence and Pedley, 1982; Scott and Govean, 1985; Studencki, 1988; Bucur and Nigorici, 1992; Buchbinder et al., 1993).
Offshore-transported rhodoliths, together with coralline algal debris, have been described in lower–middle Miocene deposits from the Piedmont Basin (NW Italy) (Vannucci, 1980; Fravega and Vannucci, 1982; Fravega et al., 1984, 1993; Basso et al., 2012), southern Apennines (SW Italy) (Checconi et al., 2010; Bassi et al., 2010, 2017), and Sardinia (Murru et al., 2015). In all these cases, reworked rhodoliths are mostly spheroidal in shape and built up by encrusting–laminar and warty plants concentrically arranged. Inferred basinward transport mechanisms are sediment gravity flows (Bassi et al., 2010, 2017; Basso et al., 2012; Murru et al., 2015) and, occasionally, storm currents (Checconi et al., 2010; Bassi et al., 2017). As aforementioned in the study of Serravallian deposits, allochthonous rhodoliths concentrate in wide and shallow channels piled up in continuous carbonate successions formed in the distal middle ramp or upper outer ramp due to storm events. Just in one particular case, in the Cerro Fontanar section, rhodoliths are embedded in a breccia with angular pebbles to cobbles (sometimes boulders). In this case rhodoliths were redeposited due to a high-density mass transport.
In terms of taxonomic composition and species abundance, it is difficult to compare our results with those previously published in other Mediterranean areas due to discrepancies in taxonomic practices and lack of chronologic precision (Braga et al., 2010b). For instance, middle Miocene Paratethys coralline algal assemblages from different areas in Poland are represented by up to 62 species (Studencki, 1979, 1988). Other studies, however, show more conservative figures, indicating approximately 20 coralline algal species from different Mediterranean areas (Vannucci, 1980; Fravega and Vannucci, 1982; Fravega et al., 1984, 1993; Bucur and Filipescu, 1994, 2011; Basso et al., 2012; Hrabovsky, 2019), a species richness similar to that identified in the Betic Serravallian coralline algal assemblages (16 species).
At last, it is worth highlighting the presence of Subterraniphyllum thomasii in the Cerro Hernando–Cerro Fontanar area (Figures 11E–G). This species was originally described by Elliott (1957) to refer to a geniculate coralline alga with a “distinctive vey coarse and irregular cell-mesh of the medullary tissue” (Elliott, 1957; p.73). Despite disagreements concerning its thallus organization, either geniculate (Elliott, 1957; Vannucci et al., 2000) or nongeniculate (Bassi et al., 2000), S. thomasii has been traditionally cited in upper Eocene–lowermost Miocene (Aquitanian) deposits, with its highest abundance and largest expansion during the Oligocene (Elliott, 1957; Bassi et al., 2000; Vannucci et al., 2000). Nonetheless, this species has been recently cited in middle Eocene deposits of the Betic Cordillera (S Spain), extending its lower stratigraphic range (Aguirre et al., 2020). In this study, we recorded S. thomasii in Serravallian carbonate deposits from SE Spain; thus, also widening the stratigraphic range of the species.
Conclusion
The coralline algae of Serravallian (middle Miocene) carbonate deposits cropping out in Jimena and in the vicinity of Jódar (Jaén Province, S Spain) have been studied. They occur forming rhodoliths and as coralline algal debris in two different paleoenvironmental contexts. Autochthonous–parautochthonous rhodolith beds, up to 2-m thick, were originated by the in situ production of the algal nodules in the middle ramp, at several tens of meters of water depth. The rhodoliths, averaging 4.5–5 cm in size, are spheroidal in shape and are built up by the intergrowth of laminar-encrusting coralline algae, less frequently warty and fruticose plants, with other encrusting organisms, such as benthic foraminifera, bryozoans, serpulids and, rarely, barnacles. Rhodolith-forming algal species are dominated by Hapalidiales: Mesophyllum roveretoi, Mesophyllum sp., Lithothamnion ramosissimum, and, less frequently, Lithothamnion group corallioides and Phymatolithon group calcareum. Accompanying species are Lithothamnion sp. 1, Spongites group fruticulosus, Spongites spp., Lithoporella minus, Hydrolithon lemoinei, and Sporolithon sp. It is worth mentioning the presence of a few fragments of Subterraniphyllum thomasii, a species previously considered extinct in the early Miocene.
Coralline algae also occur as rhodoliths and algal fragments in channeled beds characterized by rudstone containing angular cobbles to pebbles. Large and small benthic foraminifera, echinoderms, bryozoans, bivalves, serpulids (including Ditrupa), and barnacles occur with the algae. Rhodoliths are smaller than those formed in the middle ramp, averaging 2.6 cm in the largest diameter, spherical in shape, and formed by laminar-encrusting algal plants. These rhodoliths are composed of the same species as those described above. The rhodoliths and algal debris in the channeled facies are interpreted as elements formed in the middle ramp and transported offshore by storm currents. These mechanisms of transport produced segregation by rhodolith size; thus, the smaller ones traveled longer distances downslope.
Data availability statement
The original contributions presented in the study are included in the article/supplementary material; further inquiries can be directed to the corresponding author.
Author contributions
All authors listed have made a substantial, direct, and intellectual contribution to the work and approved it for publication.
Funding
We acknowledge the reviewers Brandano and Pereira-Filho for their valuable comments and suggestions that have improved the quality of the article. This study was funded by the research project PGC2018-099391-B-100 of the Spanish Ministerio de Ciencia e Innovación and by the research group RNM-190 of the Junta de Andalucía.
Conflict of interest
The authors declare that the research was conducted in the absence of any commercial or financial relationships that could be construed as a potential conflict of interest.
Publisher’s note
All claims expressed in this article are solely those of the authors and do not necessarily represent those of their affiliated organizations, or those of the publisher, the editors, and the reviewers. Any product that may be evaluated in this article, or claim that may be made by its manufacturer, is not guaranteed or endorsed by the publisher.
References
Aguilar, R., Pastor, X., Torriente, A., and García, S. (2009). “Deep-sea coralligenous beds observed with rov on four seamounts in the Western Mediterranean,” in UNEP – MAP – RAC/SPA Proc. 1st Mediterranean symp. on the conservation of the coralligenous and other calcareous bio-concretions. Editors C. Pergent-Martini, and T. Brichet (Tabarka, Tunisia: UNEP - MAP - RAC/SPA, 148–150.
Aguirre, J., Braga, J. C., and Bassi, D. (2017). “Rhodoliths and rhodolith beds in the rock record,” in Rhodolith/maërl beds: A global perspective. Editors R. Riosmena-Rodríguez, W. Nelson, and J. Aguirre (Basel, Switzerland): Springer Intern. Publ.), 105–138.
Aguirre, J., Braga, J. C., Pujalte, V., Orue-Etxebarria, X., Salazar-Ortiz, E., Rincón-Martínez, D., et al. (2020). Middle Eocene rhodoliths from tropical and mid-latitude regions. Diversity 12, 117. doi:10.3390/d12030117
Aguirre, M., J., Braga, J. C., Martín, J. M., Puga-Bernabéu, Á., Pérez-Asensio, J. N., Sánchez-Almazo, I. M., et al. (2015). An enigmatic kilometer-scale concentration of small mytilids (Late Miocene, Guadalquivir Basin, S Spain). Palaeogeogr. Palaeoclimatol. Palaeoecol. 436, 199–213. doi:10.1016/j.palaeo.2015.07.015
Athanasiadis, A. (1999). Mesophyllum macedonis, nov. sp. (Rhodophyta, Corallinales), a putative Tethyan relic in the north Aegean Sea. Eur. J. Phycol. 34, S0967026299002103–S0967026299002252. doi:10.1017/s0967026299002103
Athanasiadis, A., and Neto, A. I. (2010). On the occurrence of Mesophyllum expansum (Philippi) Cabioch et Mendoza (Melobesioideae, Corallinales, Rhodophyta) in the Mediterranean Sea, the Canary Isles and the Azores. Bot. Mar. 53, 333–341. doi:10.1515/bot.2010.042
Baccelle, L., and Bosellini, A. (1956). Diagrammi per la stima visiva della composizione percentuale nelle rocche sedimentary. Ann. Univ. Ferrara (Nuova Ser.), Sez. 9, Sc. Geol. Paleontol. 1, 59–62.
Bassi, D., Carannante, G., Checconi, A., Simone, L., and Vigorito, M. (2010). Sedimentological and palaeoecological integrated analysis of a Miocene channelized carbonate margin, Matese Mountains, Southern Apennines, Italy. Sediment. Geol. 230, 105–122. doi:10.1016/j.sedgeo.2010.07.002
Bassi, D., Iryu, Y., Humblet, M., Matsuda, H., Machiyama, H., Sasaki, K., et al. (2012). Recent macroids on the Kikai-jima shelf, Central Ryukyu Islands, Japan. Sedimentology 59, 2024–2041. doi:10.1111/j.1365-3091.2012.01333.x
Bassi, D., Simone, L., and Nebelsik, J. H. (2017). “Re-sedimented rhodliths in channelized depositional systems,” in Rhodolith/maërl beds: A global perspective. Editors R. Riosmena-Rodríguez, W. Nelson, and J. Aguirre (Basel, Switzerland): Springer Intern. Publ.), 139–167.
Bassi, D., Woelkerling, W. J., and Nabelsik, J. H. (2000). Taxonomic and biostratigraphical re-assessments of Subterraniphyllum Elliott (Corallinales, Rhodophyta). Palaeontology 43, 405–425. doi:10.1111/j.0031-0239.2000.00133.x
Basso, D., Babbini, L., Ramos-Esplá, A. A., and Salomidi, M. (2017). “Mediterranean rhodolith beds,” in Rhodolith/maërl beds: A global perspective. Editors R. Riosmena-Rodríguez, W. Nelson, and J. Aguirre (Basel, Switzerland): Springer Intern. Publ.), 281–298.
Basso, D. (1998). Deep rhodolith distribution in the Pontian Islands, Italy: A model for the paleoecology of a temperate sea. Palaeogeogr. Palaeoclimatol. Palaeoecol. 137, 173–187. doi:10.1016/s0031-0182(97)00099-0
Basso, D., Quaranta, F., Vannucci, G., and Piazza, M. (2012). Quantification of the coralline carbonate from a Serravallian rhodolith bed of the Tertiary Piedmont Basin (Stazzano, Alessandria, NW Italy). Geodiversitas 34, 137–149. doi:10.5252/g2012n1a8
Basso, D. (1995). Study of living calcareous algae by a paleontological approach: The non-geniculate corallinaceae (Rhodophyta) of the soft bottoms of the Tyrrhenian Sea (Western Mediterranean). The genera Phymatolithon Foslie and Mesophyllum Lemoine. Riv. It. Paleontol. Strat. 100, 575–596.
Basso, D., and Tomaselli, V. (1994). “Palaeoecological potentiality of rhodoliths: A Mediterranean case history, in studies on ecology and paleoecology of benthic communities,”Boll. Soc. Paleontol. Ital. Spec. Editors L. Matteucci, M. G. Carboni, and J. S. Pignatti (Mucchi, Modena: Boll. Soc. Paleontol. Ital.), 2, 17–27.
Berasátegui, X., Banks, C. J., Puig, C., Taberner, C., Waltham, D., and Fernàndez, M. (1998). “Lateral diapiric emplacement of Triassic evaporites at the southern margin of the Guadalquivir Basin, Spain,” in Cenozoic foreland basins of western europe. Editors A. Mascle, C. Puigdefàbregas, H. P. Luterbacher, and M. Fernàndez, Geol. Soc. London (London: Sp. Publ.), 134, 49–68.
Bosence, D. W. J., and Pedley, H. M. (1982). Sedimentology and palaeoecology of a Miocene coralline algal biostrome from the Maltese Islands. Palaeogeogr. Palaeoclimatol. Palaeoecol. 38, 9–43. doi:10.1016/0031-0182(82)90062-1
BouDagher-Fadel, M., and Clark, G. N. (2006). Stratigraphy, paleoenvironment and paleogeography of maritime Lebanon: A key to Eastern Mediterranean Cenozoic history. Stratigraphy 3, 1–38.
Bracchi, V. A., Angeletti, L., Marchese, F., Taviani, M., Cardone, F., Hajdas, I., et al. (2019). A resilient deep-water rhodolith bed off the Egadi archipelago (Mediterranean Sea) and its actuopaleontological significance. Alp. Medit. Quater. 32, 131–150. doi:10.26382/AMQ.2019.09
Braga, J. C., Bassi, D., and Piller, W. E. (2010b). “Paleoenvironmental significance of Oligocene-Miocene coralline red algae – A review,” in Carbonate systems during the Oligocene-Miocene climatic transition. Editors M. Mutti, W. E. Piller, and C. Betzler (IAS Spec. Publ.), 42, 165–182.
Braga, J. C., Martín, J. M., Aguirre, J., Baird, C. D., Grunnaleite, I., Jensen, N. B., et al. (2010a). Middle-Miocene (Serravallian) temperate carbonates in a seaway connecting the Atlantic Ocean and the Mediterranean Sea (north Betic Strait, S Spain). Sediment. Geol. 225, 19–33. doi:10.1016/j.sedgeo.2010.01.003
Braga, J. C. (2017). “Neogene rhodoliths in the Mediterranean basins,” in Rhodolith/maërl beds: A global perspective. Editors R. Riosmena-Rodríguez, W. Nelson, and J. Aguirre (Basel, Switzerland: Springer Intern. Publ.), 169–193.
Brandano, M., Cornacchia, I., and Tomassetti, L. (2017). Global versus regional influence on the carbonate factories of Oligo-Miocene carbonate platforms in the Mediterranean area. Mar. Pet. Geol. 87, 188–202. doi:10.1016/j.marpetgeo.2017.03.001
Bressan, G., and Babbini, L. (2003). Corallinales del Mar Mediterraneo: Guida Alla Determinazione. Biol. Mar. Medit. 10 (Suppl. 2), 1–237.
Buchbinder, B., Martinotti, G. M., Siman-Tov, R., and Zilberman, E. (1993). Temporal and spatial relationships in Miocene reef carbonates in Israel. Palaeogeogr. Palaeoclimatol. Palaeoecol. 101, 97–116. doi:10.1016/0031-0182(93)90154-b
Bucur, I. I., and Filipescu, S. (2011). “Middle Miocene red algae from Lopadea Veche (western border of the Transylvanian Basin),” in Calcareous algae from Romanian Carpathians. Editors I. I. Bucur, and E. Sasaran (Cluj-Napoca, Romania: Press University Clujeana), 115–122.
Bucur, I. I., and Filipescu, S. (1994). Middle Miocene red algae from the Transylvanian Basin (Romania). Beitr. Paläont. 19, 39–47.
Bucur, I. I., and Nigorici, E. (1992). Calcareous algae from the Sarmatian deposits in the Smileu Basin (Romania). Stud. Univ. Babes-Bolyai, Geol. 37, 3–7.
Checconi, A., Bassi, D., Monaco, P., and Carannante, G. (2010). Re-deposited rhodoliths in the middle Miocene hemipelagic deposits of Vitulano (southern Apennines, Italy): Coralline assemblage characterization and related trace fossils. Sediment. Geol. 225, 50–66. doi:10.1016/j.sedgeo.2010.01.001
Cornacchia, I., Brandano, M., and Agostini, S. (2021). Miocene paleoceanographic evolution of the Mediterranean area and carbonate production changes: A review. Earth. Sci. Rev. 221, 103785. doi:10.1016/j.earscirev.2021.103785
De Río, J., Angelo Ramos, D., Sánchez-Tocino, L., Peñas, J., and Braga, J. C. (2022). The Punta de la Mona rhodolith bed: Shallow-water Mediterranean rhodoliths (Almuñecar, Granada, southern Spain). Front. Earth Sci. 10, 884685. doi:10.3389/feart.2022.884685
Fravega, P., Giammarino, S., and Vannucci, G. (1984). Episodi ad «algal balls» e loro significato al passaggio arenarie di Serravalle-Marne di S. Agata fossili a nord di Gavi (bacino Terziario del Piemonte). Atti Soc. Tosc. Sci. Nat. Ser. A 91, 1–20.
Fravega, P., Piazza, M., and Vannucci, G. (1993). “Importance and significance of the rhodolithic bodies in the miocenic sequences of Tertiary Piedmont Basin,” in Studies on fossil benthic algae. Editors F. Barattolo, P. De Castro, and M. Parente (Ital., Sp.: Boll. Soc. Paleontol), 1, 197–210.
Fravega, P., and Vannucci, G. (1989). Rhodophycee calcaree nelle biocenosi del Golfo di Calvi (Corsica). Atti 3° Simp. Ecol. Paleoecol. Com. Bent., 711–727.
Fravega, P., and Vannucci, G. (1982). Significato e caratteristiche degli episodi e rhodoliti al «top» del Serravalliano tipo. Geol. Romana 21, 705–715.
Galindo-Zaldívar, J., Ruano, P., Jabaloy, A., and López-Chinaco, M. (2000). Kinematics of faults between subbetic units during the Miocene (central sector of the Betic Cordillera). Comptes Rendus de l'Academie. des Sci. - Ser. IIA - Earth Planet. Sci. 331, 811–816. doi:10.1016/s1251-8050(00)01484-1
García-Castellanos, D., Fernàndez, M., and Torné, M. (2002). Modeling the evolution of the Guadalquivir foreland basin (southern Spain). Tectonics 21, 9-1–9-17. doi:10.1029/2001TC001339
García-Hernández, M., López-Garrido, A. C., Rivas, P., Sanz de Galdeano, C., and Vera, J. A. (1980). Mesozoic palaeogeographic evolution of the External Zones of the Betic Cordillera. Geol. Mijnbou 59, 155–168.
García-Tortosoa, F. J., Sanz de Galdeano, C., Sánchez-Gómez, M., and Alfaro, P. (2008). Tectónica reciente en el frente de cabalgamiento bético. Las deformaciones de Jimena y Bedmar (Jaén). Geogaceta 44, 59–62.
Halfar, J., and Mutti, M. (2005). Global dominance of coralline red-algal facies: A response to Miocene oceanographic events. Geol. 33, 481. doi:10.1130/g21462.1
Hamel, G., and Lemoine, M. P. (1952). Corallinacées de France et d’Afrique du Nord. Arch. Mus. Hist. Nat. Paris Sér. 7, 15–136.
Hottinger, L. (1983). “Neritic macroid genesis, an ecological approach,” in Coated grains. Editor T. M. Peryt (Berlin: Springer-Verlag), 38–55.
Hrabovsky, J. (2019). Reproductive phases of Miocene algae from central Paratethys and their bearing on systematics. Acta Palaeontol. Pol. 64, 417–439. doi:10.4202/app.00579.2018
Jeong, S. Y., Nelson, W. A., Sutherland, J. E., Peña, V., Le Gall, L., Diaz-Pulido, G., et al. (2021). Corallinapetrales and Corallinapetraceae: A new order and new family of coralline red algae including Corallinapetra gabriellii comb. nov. J. Phycol. doi:10.1111/jpy.13115-20-107
Joshi, S., Duffy, G. P., and Brown, C. (2017). Mobility of maerl-siliciclastic mixtures: Impact of waves, currents and storm events. Estuar. Coast. Shelf Sci. 189, 173–188. doi:10.1016/j.ecss.2017.03.018
Le Loc'h, F., Hily, C., and Grall, J. (2008). Benthic community and food web structure on the continental shelf of the Bay of Biscay (North Eastern Atlantic) revealed by stable isotopes analysis. J. Mar. Syst. 72, 17–34. doi:10.1016/j.jmarsys.2007.05.011
Marrack, E. (1999). The relationship between water motion and living rhodolith beds in the southwestern Gulf of California, Mexico. Palaios 14, 159. doi:10.2307/3515371
Martín, J. M., Braga, J. C., Aguirre, J., and Puga-Bernabéu, Á. (2009). History and evolution of the North-Betic Strait (Prebetic Zone, Betic Cordillera): A narrow, early Tortonian, tidal-dominated, Atlantic–Mediterranean marine passage. Sediment. Geol. 216, 80–90. doi:10.1016/j.sedgeo.2009.01.005
Meulenkamp, J. E., and Sissingh, W. (2003). Tertiary palaeogeography and tectonostratigraphic evolution of the Northern and Southern Peri-Tethys platforms and the intermediate domains of the African–Eurasian convergent plate boundary zone. Palaeogeogr. Palaeoclimatol. Palaeoecol. 196, 209–228. doi:10.1016/s0031-0182(03)00319-5
Millar, K. R., and Gagnon, P. (2018). Mechanisms of stability of rhodolith beds: Sedimentological aspects. Mar. Ecol. Prog. Ser. 594, 65–83. doi:10.3354/meps12501
Murru, M., Bassi, D., and Simone, L. (2015). Displaced/re-worked rhodolith deposits infilling parts of a complex Miocene multistorey submarine channel: A case history from the Sassari area (Sardinia, Italy). Sediment. Geol. 326, 94–108. doi:10.1016/j.sedgeo.2015.07.001
O’Connell, L. G., James, N. P., Harvey, A. S., Luick, J., Bone, Y., Shepherd, S. A., et al. (2020). Reevaluation of the inferred relationship between living rhodolith morphologies, their movements, and water energy: Implications for interpreting paleoceanographic conditions. Palaios 35, 543–556.
Palcu, D. V., Patina, I. S., Șandric, I., Lazarev, S., Vasiliev, I., Stoica, M., et al. (2011). Late Miocene megalake regressions in Eurasia. Sci. Rep. 11, 11471. doi:10.1038/s41598-021-91001-z
Pedley, H. M. (1998). “A review of sediment distributions and processes in Oligo-Miocene ramps of southern Italy and Malta (Mediterranean divide),”. Editors V. P. Wright, and T. P. Burchette. Carbonate ramps (London, United Kingdom: Geol. Soc. London, Sp. Publ.), 149, 163–179.
Pedley, H. M. (1979). Miocene bioherms and associated structures in the upper coralline limestone of the Maltese islands: Their lithification and palaeoenvironment. Sedimentology 26, 577–591. doi:10.1111/j.1365-3091.1979.tb00930.x
Pedrera, A., Marín-Lechado, C., Martos-Rosillo, S., and Roldán, F. J. (2012). Curved fold-and-thrust accretion during the extrusion of a synorogenic viscous allochthonous sheet: The Estepa Range (External Zones, western Betic Cordillera, Spain). Tectonics 31, TC4013. doi:10.1029/2012TC003119
Peña, V., Pardo, C., López, L., Carro, B., Hernández-Kantun, J., Adey, W. H., et al. (2015). Phymatolithon lusitanicum sp. nov. (Hapalidiales, Rhodophyta): The third most abundant maerl-forming species in the Atlantic Iberian peninsula. Cryptogam. Algol. 36, 429–459. doi:10.7872/crya/v36.iss4.2015.429
Peña, V., Vieira, C., Braga, J. C., Aguirre, J., Rösler, A., Baele, G., et al. (2020). Radiation of the coralline red algae (Corallinophycidae, Rhodophyta) crown group as inferred from a multilocus time-calibrated phylogeny. Mol. Phylogenet. Evol. 150, 106845. doi:10.1016/j.ympev.2020.106845
Perconing, E. (1960-62). “Sur la constitution géologique de L'Andalousie occidentale en particuler du bassin du Guadalquivir (Espagne méridionale),”. Livre a la Mémorie du Professeur Paul Fallot Editor M. Durand Delga. (Tome: Soc. Géol. Fr.), 1, 229–256.
Pereira-Filho, G. H., Veras, P. C., Francini-Filho, R. B., de Moura, R. L., Pinheiro, H. T., Gibran, F. Z., et al. (2015). Effects of the sand tilefish Malacanthus plumieri on the structure and dynamics of a rhodolith bed in the Fernando de Noronha Archipelago, tropical West Atlantic. Mar. Ecol. Prog. Ser. 541, 65–73.
Prager, E. J., and Gingsburg, R. N. (1989). Carbonate nodule growth on Florida’s outer shelf and its implications for fossil interpretations. Palaios 4, 310. doi:10.2307/3514555
Randazzo, A. F., Müller, P., Lelkes, G., Juhász, E., and Hámor, T. (1999). Cool-water limestones of the Pannonian basinal system, middle Miocene, Hungary. J. Sediment. Res. 69, 283–293. doi:10.2110/jsr.69.283
Rendina, F., Kaleb, S., Caragnano, A., Ferrigno, F., Appollino, L., Donnarumma, L., et al. (2020). Distribution and characterization of deep rhodolith beds off the Campania coast (SW Italy, Mediterranean Sea). Plants 9, 985. doi:10.3390/plants9080985
Rodríguez-Fernández, J., Azor, A., and Azañón, J. M. (2012). “The Betic intramontane basins (SE Spain): Stratigraphy, subsidence, and tectonic history,” in Tectonics of sedimentary basins: Recent advances. Editors C. Busby, and A. Azor (Blackwell Publishing Ltd.), Hoboken, New Jersey, United States, 461–479.
Rodríguez-Fernández, J., Roldán, F. J., Azañón, J. M., and García-Cortés, A. (2013). El colapso gravitacional del frente orogénico alpino en el Dominio Subbético durante el Mioceno medio-superior: El complejo extensional Subbético. Bol. Geol. Min. Esp. 124, 477–504.
Rögl, F. (1998). Palaeogeographic considerations for Mediterranean and Paratethys seaways (Oligocene to Miocene). Ann. Natur. Mus. Wien 99, 279–310.
Roldán, F. J. (1995). Evolución neógena de la Cuenca del Guadalquivir. Spain: Univ. Granada. Ph.D. Thesis.
Roldán, F. J. (2008). “Las unidades olistostrómicas del antepaís Bético,” in Contextos geológicos españoles. Una aproximación al patrimonio geológico español de relevancia internacional. Editor A. García-Cortés (Madrid): IGME), 124–132.
Ruchonnet, C., and Kindler, P. (2010). “Facies models and geometries of the Ragusa platform (SE Sicily, Italy) near the Serravallian–Tortonian boundary,”Carbonate systems during the Oligocene-Miocene climatic transition. Editors M. Mutti, W. E. Piller, and C. Betzler (IAS Spec. Publ.), 42, 71–88.
Ruiz-Costán, A., Pedrera, A., Galindo-Zaldívar, J., Pous, J., Arzate, J., Roldán-Garcia, F. J., et al. (2012). Constraints on the frontal crustal structure of a continental collision from an integrated geophysical research: The central-western Betic Cordillera (SW Spain). Geochem., Geophys., Geosyst. 13, Q08012. doi:10.1029/2012GC004153
Sanz de Galdeano, C., García-Tortosa, F. J., and Peláez, J. A. (2013). Estructura del Prebético de Jaén (sector de Bedmar). Su relación con el avance del Subbético y con fallas en el basamento. Rev. Soc. Geol. Esp. 26, 55–68.
Scott, R. W., and Govean, F. M. (1985). Early depositional history of a rift basin: Miocene in Western Sinai. Palaeogeogr. Palaeoclimatol. Palaeoecol. 52, 143–158. doi:10.1016/0031-0182(85)90035-5
Studencki, W. (1988). Facies and sedimentary environment of the Pinczow limestones (middle Miocene; Holy Cross Mountains, Central Poland). Facies 18, 1–25. doi:10.1007/bf02536793
Studencki, W. (1979). Sedimentation of algal limestones from Busko-Spa environs (middle Miocene, Central Poland). Palaeogeogr. Palaeoclimatol. Palaeoecol. 27, 155–165. doi:10.1016/0031-0182(79)90098-1
Toscano, F., Vigliotti, M., and Simone, L. (2006). “Variety of coralline algal deposits (rhodalgal facies) from the Bays of Naples and Pozzuoli (northern Tyrrhenian Sea, Italy),” Cool-water carbonates: Depositional systems and palaeoenvironmental controls. Editors H. M. Pedley, and G. Carannante (London, United Kingdom: Geol. Soc., London, Sp. Publ.), 255, 85–94.
Vannucci, G., Basso, D., and Fravega, P. (2000). New observations on the anatomy of the fossil calcareous alga Subterraniphyllum Elliott. Riv. It. Paleotol. Strat. 106, 237–246.
Vannucci, G. (1980). Prime indagini sulle rhodoliti del “Serravalliano” della Valle Scrivia. Quater. Ist. Geol. Univ. Genova 1, 59–64.
Keywords: rhodolith beds, autochthonous-allochthonous assemblages, carbonate ramps, Guadalquivir Basin, Jimena, Jódar, Betic Cordillera.
Citation: Aguirre J and Braga JC (2022) Middle Miocene (Serravallian) rhodoliths and coralline algal debris in carbonate ramps (Betic Cordillera, S Spain). Front. Earth Sci. 10:958148. doi: 10.3389/feart.2022.958148
Received: 31 May 2022; Accepted: 30 June 2022;
Published: 26 August 2022.
Edited by:
Sebastian Teichert, Friedrich-Alexander Universität Erlangen-Nürnberg, GermanyReviewed by:
Marco Brandano, Sapienza University of Rome, ItalyGuilherme Henrique Pereira-Filho, Universidade Federal de São Paulo, Brazil
Copyright © 2022 Aguirre and Braga. This is an open-access article distributed under the terms of the Creative Commons Attribution License (CC BY). The use, distribution or reproduction in other forums is permitted, provided the original author(s) and the copyright owner(s) are credited and that the original publication in this journal is cited, in accordance with accepted academic practice. No use, distribution or reproduction is permitted which does not comply with these terms.
*Correspondence: Julio Aguirre, amFndWlycmVAdWdyLmVz