- MNR Key Laboratory of Metallogeny and Mineral Assessment, Institute of Mineral Resources, CAGS, Beijing, China
South China is a well-known grand felsic igneous rocks province. However, it is still controversial and not well understood whether the Mesozoic tectono-magmatic pattern is dominated by the subduction of the paleo-Pacific oceanic plate. In this study, we address this question by concentrating on the long-term evolutionary Guandimiao batholith, which has complex lithofacies with different formation ages and can be a superb record of the Mesozoic tectonic evolution in South China. Geochronologically, four stages of magmatism can be identified combined with previous reports: granodiorite (G1, 239 Ma), biotite monzogranite (G2-1) and two-mica monzogranite (G2-2) (230–203 Ma), granite porphyry (G3, 211–190 Ma), and lamprophyre (L4, 121 Ma). G1 and G2-1 have an affinity with I-type granite and were derived from metabasaltic to metatonalitic sources, whereas G2 and G3 show S-type granite characteristics and were derived from the para-metamorphic basement of the Cathaysia block. The L4 was derived from partial melting of garnet and spinel lherzolite and underwent mixing between Mesoproterozoic pelagic and/or terrigenous sediments and the subcontinental lithosphere mantle (SCLM) of South China. The granitoids of the Guandimiao batholith underwent intensely fractional crystallization of feldspar, Ti-bearing minerals, allanite and monazite. The zircon U–Pb dating of L4 in the Guandimiao batholith completely records the six stages of pre-Mesozoic tectonic events in the SCB. During the Mesozoic, the main body of the Guandimiao batholith (G1, G2-1 and G2-2) recorded the closure of the paleo-Tethys Ocean in the Triassic and the subsequent regional extension of the postcollision. G-3 and L4 of the Guandimiao batholith documented the transition of tectonic and dynamic regimes in the early Yanshanian and the rollback and steep subduction of the paleo-Pacific Ocean in the late Yanshanian.
1 Introduction
South China is a felsic large igneous rock province that developed large intrusions and volcanics with a total outcrop area of nearly 218,090 km2. In particular, the outcrop area of granitoids occupies more than 90% of the total area of igneous rock (Zhou et al., 2006). Multiple stages of magmatic activity have been determined using accurate geochronological data (Zhou et al., 2006; Wang et al., 2010; Mao et al., 2011; Mao et al., 2014). Zhou et al. (2006) proposed four main stages of granitic magmatism: 251–234, 234–205, 180–142, and 142–67 Ma. Moreover, the mafic rocks contain three main clusters: the 190–160 Ma group (Zhao et al., 1998; Meng et al., 2012), the 160–110 Ma group (Wang et al., 2003; Wang et al., 2008; Ma et al., 2013) and the 110–80 Ma group (Chen et al., 2008a; Meng et al., 2012; Qin et al., 2020). Numerous models have been proposed for the systematic understanding of the evolutionary history of the regional geology. However, to date, the tectonic regime is not consistently well understood. Li and Li (2007) and Jiang et al. (2015) interpreted extensive igneous rocks in the early Mesozoic as a continual constraint of the plate subduction model of the Paleo-Pacific oceanic plate of the SCB. Wang et al. (2003), Mao et al. (2014), and Li et al. (2014) confirmed that the SCB suffered from the convergence of multiple plates in Indosinia. Subsequently, the postcollisional and postorogenic regimes occurred 234–203 Ma and 190–170 Ma, respectively (Zhou et al., 2006; Mao et al., 2014; Shu et al., 2019). Two supportable mechanisms have been presented with the controversy of whether the subduction of the Pacific Ocean dominated the tectono-magmatic pattern of the SCB in the middle to late Mesozoic (Li, 1990; Gilder et al., 1996; Chen et al., 2014; Li et al., 2014; Qin et al., 2020; Yang et al., 2020).
Batholith generally has complex lithofacies assemblage and long time span. It recorded the imprint of multiple stages of magmatism and thus can be considered an extremely advantageous object for studying the history of regional tectono-magmatic evolution (Hervé et al., 2007; Whalen et al., 2010; Zhao et al., 2012; Deng et al., 2017). Typical batholiths, such as South Patagonian batholiths, recorded magmatic activity over 100 Ma, which is essential for understanding the development of subduction from 157 to 40 Ma (Hervé et al., 2007). The Cumberland batholith (CB) in Canada provides evidence for Paleoproterozoic crustal and orogenic processes of the Trans-Hudson Orogen (Whalen et al., 2010), and the Lincang batholith in West China reveals the suture process of the Changning-Menglian belt (Deng et al., 2017). Thus, further research on Mesozoic batholiths of South China might be the key point for addressing the controversial geodynamic issues in the Mesozoic (Barbarin, 2005; Zhao et al., 2012).
The Guandimiao batholith is located in the middle Qing-Hang paleostylolitic zone in the SCB. Conspicuously, it is a sizeable Mesozoic batholith with a total area of approximately 300 km2 that, with formation ages throughout the Mesozoic, could be considered a complete record of Mesozoic tectonic and magmatic events (Wang et al., 2003; Dai et al., 2008; Zhao et al., 2017). Spatially, mafic rocks (MME, lamprophyre) and felsic rocks (granitoids) are concomitant in the Guandimiao batholith. Geochemically, the Guandimiao batholith contains two distinct granitic end-members, I-type granite from the Jingtou area and S-type granite from the Shizhuqiao area (Zhao et al., 2017). Thus, it could also effectively reveal deep crust–mantle interactions and generally trace complicated regional tectonic evolution. Wang et al. (2003), Bai et al. (2014), and Zhao et al. (2017) proposed that the Guandimiao batholith was formed at the 239–220 Ma, which is associated with the post-orogeny regime. Miao (2014) obtained the zircon U-Pb age of 203 ± 1.4 Ma from bitotite granite in Zhoujialing area, which is nearly 20 Ma younger than the previous studies. Dai et al. (2008) reported the occurance of the granite porphyry and lamprophyre and claimed that the dikes are formed at 153 Ma. However, due to lack of systematic geochronological and geochemical investigations, the petrogenesis, geological framework and geodynamic backgrounds for various types of magmatic rocks in the Guandimiao batholith still remain quite unclear.
To confirm the exact chronological sequence, geochemical characteristics, and further discussion of the relationship between intrusions and multiple stages of tectonic setting, we report new LA-ICP–MS zircon U-Pb data and geochemical and Sr-Nd-Pb isotope data for the Guandimiao batholith.
2 Regional geology
The Guandimiao batholith is situated in the northern part of the middle SCB. The SCB consists of the Yangtze Block (YZB) to the west and the Cathaysia Block (CAB) to the east (Figure 1A). The area experienced closure of the YZB and subsequently, the South China folded orogenic multistage intraplate cleavage occurred in the late Neoproterozoic (Li et al., 1999; Li et al., 2001; Li et al., 2002; Greentree et al., 2006; Meng et al., 2012). The Jiuling batholith recorded magmatism in the Neoproterozoic with ages ranging from 848 to 794 Ma (Li et al., 2003; Zhong et al., 2005). During the Phanerozoic, the SCB was in a stable intraplate tectonic environment in South China, dominated by early Paleozoic tectonic stress and early Mesozoic closure of the ancient Tethys Ocean in East Asia (Liu, 1994; Rowley, 1997; Wang et al., 2005a; Mao et al., 2005; Shu, 2012). Subsequently, in the late Mesozoic, the tectonic environment was characterized by multiple periods of compressional and extensional orogeny and the development of widespread depression red basins and depression belts (Xia, 1991; Cheng, 1994; Gilder et al., 1995; Gilder and Courtillot, 1997; Wang et al., 2005b; Yang et al., 2019; Yang et al., 2020). Correspondingly, the famous Zhuguangshan batholith formed 500–90 Ma with a large, exposed area of more than 1,000 km2 (Li, 1990; Deng et al., 2012; Zhang et al., 2020). Miao’shan-Yuechengling batholith is exposed in the western part of the SCB with emplacement ages from 421.8 to 212 Ma (Yang, 2012). The Fogang batholith had a large volume with a single temporal distribution in the middle Mesozoic (Li et al., 2007). The Guandimiao batholith developed from 239 to 153 Ma (Wang et al., 2003; Dai et al., 2008; Bai et al., 2014; Zhao et al., 2017) (Figure 1B).
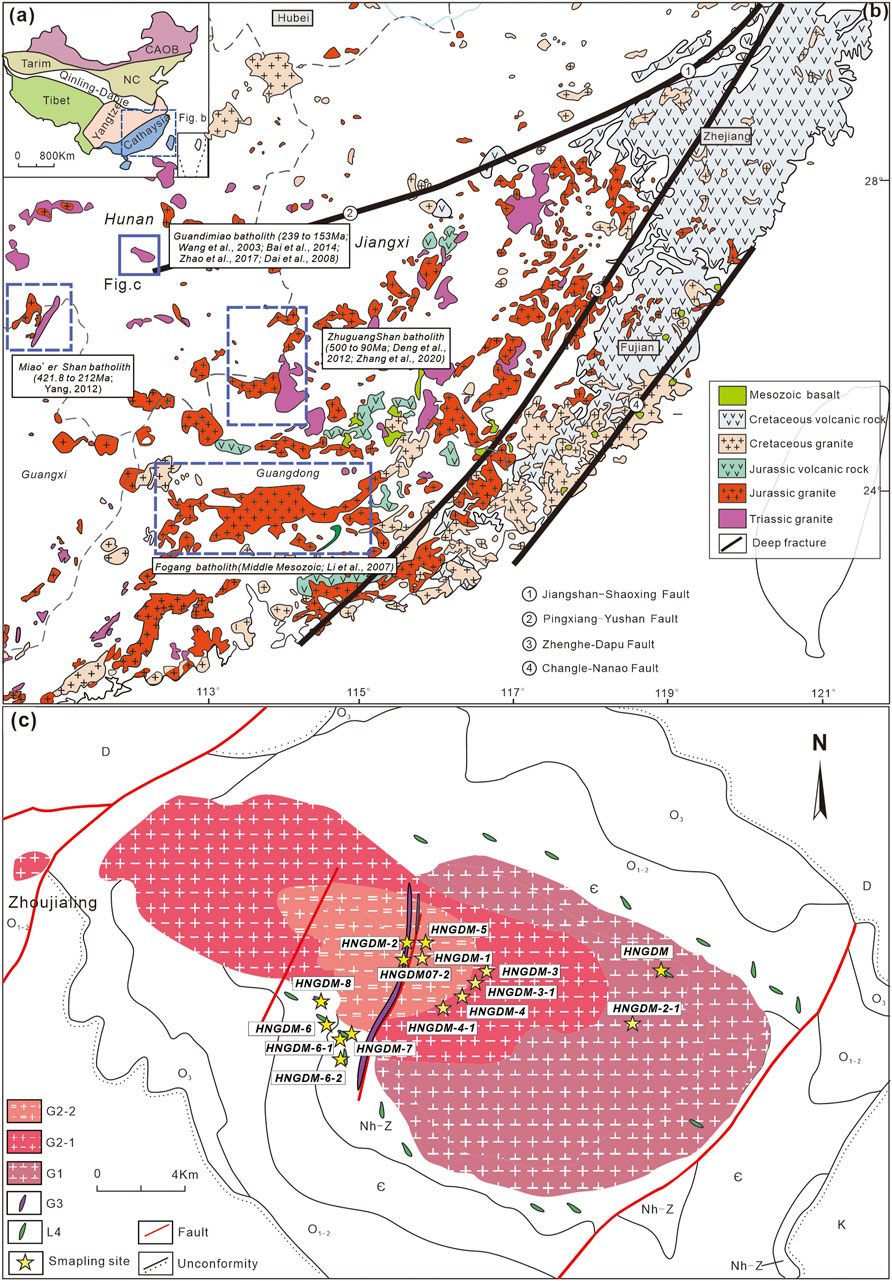
FIGURE 1. (A), Tectonic map of China (after Shu and Zhou, 2002); (B), Regional tectonic map of south China (after Sun, 2006; Wang et al., 2013); (C), Geological map of Guandimiao batholith (after Bai et al., 2014).
Multiple tectonic events and magmatic processes led to the unique geological features around the Guandimiao batholith. Numerous large abyssal faults, including basement faults and surface-cutting faults, are oriented in NE, NW, and E-W directions. “Checkerboard structure pattern” and “circular structures” are typical structural patterns (Zhang, 1984; Liang and Guo, 2002). NE- to NNE-directed subfaults and fractures closely connect with the abyssal faults and serve as necessary conduits for deep fluids. Precambrian to Cenozoic strata crop out. The Guandimiao batholith is located in a typical uplifted dome. In the center of the uplifted region, Precambrian strata are exposed as epi-metamorphic rocks in the Jiangkou Formation (Zaj) and carbonate in the Dengying Formation (Zd). The Phanerozoic strata are Permian to Ordovician in age and include sandstone of the Tiaomajian Formation (D2t), limestone and marble of the Qiziqiao Formation (D2q) and Xikuangshan Formation (D3x), and limestone of the Hutian Formation (C2+3h). The exposed rock assemblages range from acidic to basic and are dominated by five lithofacies: granodiorite (G1), biotite monzogranite (G2-1), two-mica monzogranite (G2-2), dikes [granite porphyry (G3) and lamprophyre (L4)] (Figure 1C).
3 Petrography
Granodiorite (G1) is the marginal facies and is distributed along the eastern edge of the Guandimiao batholith. It has a medium-coarse granular texture with a mineral assemblage of quartz (∼30%) + plagioclase (andesine, An≈40, 40%) + K-feldspar (∼20%) + amphibole (∼5%) + biotite (∼5%) and minor apatite, zircon and magnetite/ilmenite (Figure 2K). Biotite monzogranite (G2-1) was exposed in the center of the Guandimiao batholith and intruded into Lower Ordovician and Upper Cambrian strata (Figure 2B). The mineral assemblage is dominantly plagioclase (∼40%), perthitic K-feldspar (∼30%), quartz (∼20%), and biotite (∼10%) with accessory zircon, garnet, magnetite or ilmenite (Figures 2G,I). MMEs, generally 2 × 3 cm in size, are exposed in the interior of the biotite monzogranite in Shuanghekou village (Figure 1C). Two-mica monzogranite (G2-2) occurs locally in Maohedian town (approximately 3 km in length, 1.5 km in width), which is the central facies of the batholith. The central lithological facies is structurally massive with the occurrence of muscovite grains. The mineral assemblage of two-mica granites consists of perthitic K-feldspar (∼30%), plagioclase (∼35%), quartz (∼20%), biotite (∼5%), muscovite (∼5%) and magnetite/ilmenite (∼2%) with accessory zircon and monazite (Figure 2J). Granite porphyry (G3) occurs in the interior and surroundings of the Guandimiao batholith. The granite porphyry vein is oriented NNE with a width of 5–10 m and intruded into biotite monzogranite (Figures 2A,H). Granite porphyry presents a typical porphyritic structure, and the phenocryst minerals are quartz (20%), K-feldspar (∼15%), plagioclase (∼10%), and biotite (<5%). Lamprophyre (L4) is exposed in the interior and around the granitic batholith and is 0.5–2 m in width and vertical in occurrence (Figures 2E,F). They are gray–green in color and intruded into Paleozoic silty slate and granitic batholith. Lamprophyre presents lamprophyric texture. The phenocrysts are mainly biotite and plagioclase, occupying 50% vol. Of the whole rocks. Biotite occurs as brown-light brown sheets 0.2–0.4 mm in size (30% vol). Plagioclase occurs as subhedral crystals that are 0.1–0.2 mm in size (approximately 30% vol). The matrix is mafic with extensive epidotization, chloritization, and carbonation (Figure.2L).
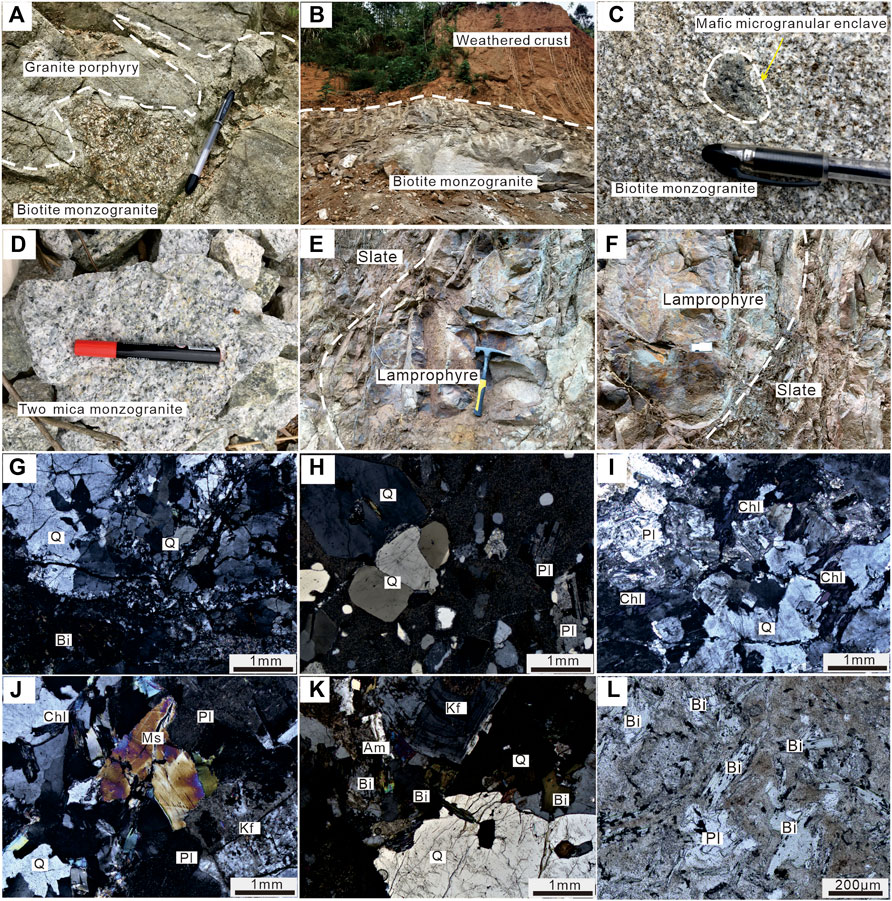
FIGURE 2. Petrographic photograph of Guandimiao batholith; (A), Granite porphyry intruded into biotite monzogranite; (B), Biotite monzogranite profile; (C), MME (mafic microgranular enclave) in biotite monzogranite; (D), Weathered two-mica monzogranite; (E,F,L), Lamprophyre; (G,I) Biotite monzogranite; (H), Granite prophyry; (J), Two-mica monzogranite; (K), Granodiorite. Abbreviation: Q-Quarz; Bi-Biotite; Pl-Plagioclase; Chl-Chlorite; Ms-Muscovite; Kf- Potassium feldspar; Am- Amphibole.
4 Sampling and method
15 samples, no obvious weathering or alteration, were collected from the Guandimiao batholith. The granodiorite sample (HNGDM-2-1) and biotite monzogranite samples (HNGDM-4, HNGDM-4-1, HNGDM-3 and HNGDM-3-1) were collected at Lingguandian town. The two-mica monzogranite samples (HNGDM-1 and HNGDM-5) were collected from the outside of Shiqiaopu lead-zinc ore deposit. The granite porphyry samples (HNGDM-2 and HNGDM07-2) were from Shuangjiangkou village. The lamprophyre samples (HNGDM, HNGDM-6, HNGDM-6-1, HNGDM-6-2, HNGDM-7, and HNGDM-8) were collected from the north of Qidong county. 8 out of 15 samples were chosen for accurately geochronological dating, 12 samples were chosen for geochemical analysis and 8 samples for Sr-Nd-Pb isotopic analysis.
4.1 LA–ICP–MS
Zircon U-Pb dating was performed the Institute of Mineral Resources, CAGS, Beijing, using a Finnigan, Neptune inductively coupled plasma mass spectrometer (MC LA-ICP-MS) with a new wave UP213 laser-ablation system. Helium was used as the carrier gas, and the beam diameter was 30 μm with 10-Hz repetition rate and a laser power of 2.5 J/cm2. Eight ion counters were used to receive the 238U, 235U, 232Th, 208Pb, 207Pb, 206Pb, 204Pb, and 202Hg signals simultaneously, whereas data for 208Pb, 232Th, 235U, and 238U were collected on a Faraday cup. Zircon GJ-1 was used as standard, and Plešovice zircon was used to optimize the mass spectrometer. U, Th, and Pb concentrations were calibrated using 29 Si as internal standard and zircon M127 U: 923 ppm; Th: 439 ppm; Th/U: 0.475 (Nasdala et al., 2008) as external standard. 207Pb/206Pb and 206Pb/238U were calculated using the ICP-MS DataCal 4.3 program. Common Pb was not corrected because of the high 206Pb/204Pb. Abnormally high 204Pb data were deleted. The Plešovice zircon was dated as unknown and yielded a weighted mean 206Pb/238U age of 337 ± 2 Ma (2SD, n = 12), which is in good agreement with the recommended 206Pb/238U age of 337.13 ± 0.37 Ma (2SD) (Sláma et al., 2008). Age calculations were performed, and concordia diagrams were generated using the Isoplot/Ex 3.0 software (Ludwig, 2003).
4.2 Geochemstry
Whole-rock major, trace and rare earth element concentrations were analyzed at the National Geological Experiment Test Center, Beijing. Whole-rock major elements were analyzed using a plasma spectrometer (PE8300). All results were normalized against the Chinese rock reference standard JY/T015-1996; among them, H2O+ is analyzed on the basis of GB/T 14,506.2-2010, FeO contents are normalized to GB/T 14,506.14-2010, LOI contents are normalized to LY/T 1253–1999 and CO2 contents are normalized to GB 9835–1988. The analytical uncertainties are less than ±2%.
4.3 Sr-Nd-Pb isotope
Fresh samples were ground with an agate mill, and powders were spiked with mixed isotope tracers, dissolved in Teflon capsules with HF + HNO3 acid, and separated by conventional cation exchange techniques. The isotopic measurements were performed on a VG-354 mass spectrometer at the Institute of Geology and Geophysics, Chinese Academy of Sciences. The mass fractionation corrections for Sr and Nd isotope ratios were based on 86Sr/88Sr = 0.1194 and 146Nd/144Nd = 0.7219. Repeat analyses yielded an 87Sr/86Sr ratio of 0.71023 ± 0.00006 for the NBS-987 Sr standard and a143Nd/144Nd ratio of 0.511845 ± 0.000012 for the La Jolla standard. For Pb isotope determinations, 200 mg of powder was weighed into a Teflon vial, spiked and dissolved in concentrated HF at 80°C for 72 h. Pb was separated and purified by conventional cation exchange techniques (AG1 × 8,200–400 resin) with diluted HBr as an eluant. Isotopic ratios were measured with a VG-354 mass spectrometer at the Institute of Geology and Geophysics. Repeat analyses of NBS981 yielded 204Pb/206Pb = 0.05897 ± 15, 207Pb/206Pb = 0.91445 ± 80, and 208Pb/206Pb = 2.16170 ± 180. Detailed descriptions of the analytical techniques are given elsewhere (Zhang et al., 2002, and references therein).
5 Results
5.1 U-Pb chronology
5.1.1 G2-1
Zircons are long and columnar with length-to-width ratios close to 2∶1. The sizes of zircons are between 100 and 150 µm. The edges of the zircons were obviously corroded. The U contents range from 446.6 to 1,515.8 ppm. Th contents range from 340.1 to 3,675.8 ppm. Th/U ratios varied from 0.4 to 1.7 (Table 1). The 206Pb/238U ages of sample HNGDM-2 are between 212.0 and 229.6 Ma, and yield the concordia age and mean age (n = 24) are 225.9 ± 1.28 Ma (MSWD = 0.49) and 225.9 ± 0.7 Ma (MSWD = 0.29), respectively (Figures 3A,B). The 206Pb/238U ages of Sample HNGDM-4 range from 236.1 to 207.6 Ma, and the concordia age and mean age (n = 19) of the zircon grains are 223.6 ± 1.3 Ma (MSWD = 1.5) and 223.7 ± 1.3 Ma (MSWD = 2.35), respectively (Figures 3C,D).
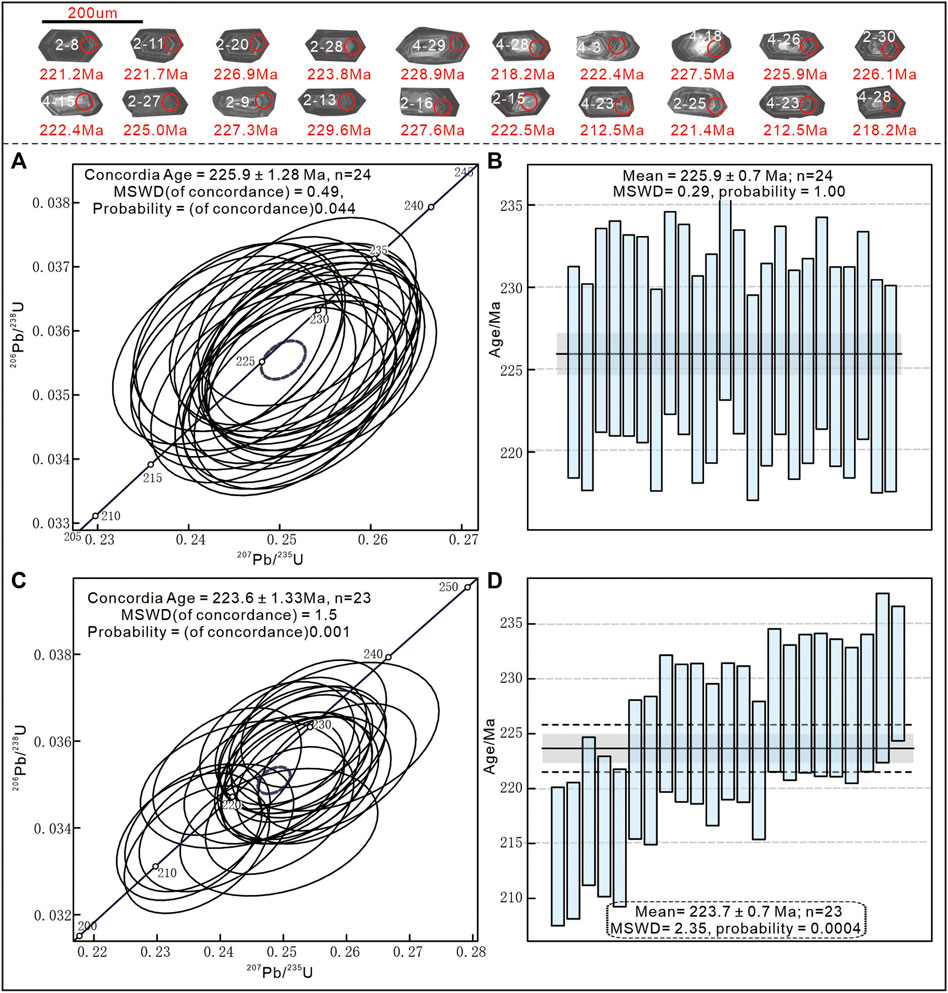
FIGURE 3. Zircon U–Pb concordia diagrams of Guandimiao biotite monzogranite. (A,C), Concordia age diagrams; (B,D), Mean age diagrams.
5.1.2 G2-2
Zircons exhibit length-to-width ratios between 1:1 and 2:1 and sizes of 100–200 μm. Most zircon grains developed obvious oscillatory zoning (Figure 4). The U contents range from 53.3 to 4,703.3 ppm (except for one spot value up to 9,301.8 ppm), Th contents range from 54.3 to 4,333.7 ppm (except for one spot value up to 6,293.6.8 ppm), and the Th/U ratios range from 0.5 to 1.9, suggesting an igneous origin (Rubatto, 2002; Wu and Zhen, 2004). Out of 30 spots in sample HNGDM-1, 14 analyses yield 206Pb/238U ages of 221.2–233.2 Ma, with a concordia U-Pb age of 227.4 ± 1.86 Ma (MSWD = 0.97) and a mean age of 227.41 ± 0.95 Ma (MSWD = 1.06) (Figures 4A,B). The 206Pb/238U ages of Sample HNGDM5-1 range from 217.3 to 228.7 Ma, with a concordia U-Pb age of 225.19 ± 1.61 Ma (MSWD = 0.72) and a mean age of 225.20 ± 0.82 Ma (MSWD = 0.99, n = 16) (Figures 4C,D).
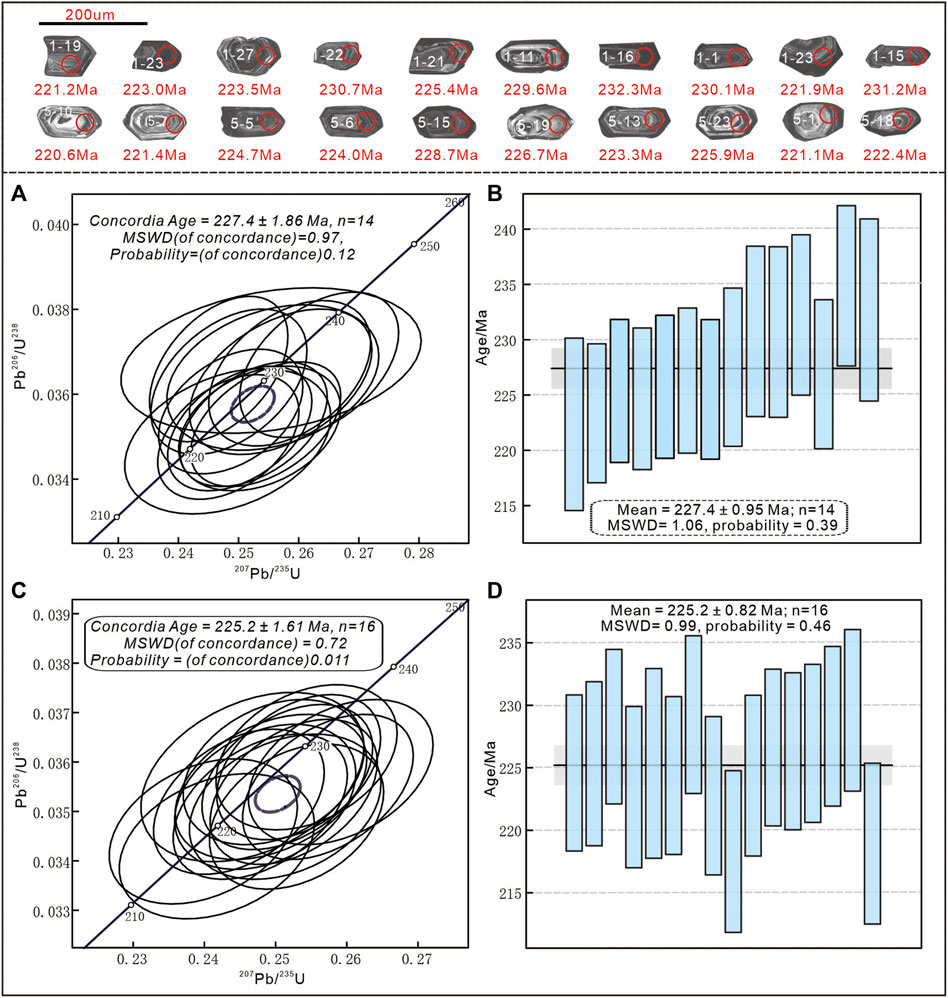
FIGURE 4. Zircon U–Pb concordia diagrams of Guandimiao two-mica monzogranite. (A,C), Concordia age diagrams; (B,D), Mean age diagrams.
5.1.3 G3
Zircons developed obvious oscillatory zoning. They exhibit length-to-width ratios from 1:1 to 2:1 with sizes from 100 to 200 μm (Figure 5B). U contents range from 516 to 3,309.6 ppm, Th contents range from 127.1 to 6,116.0 ppm, and Th/U ratios range from 0.1 to 2.2. Thirty spot analyses yield 206Pb/238U ages of 189.7–234.4 Ma. Two groups of 206Pb/238U ages can be identified. The first group is from 219.5 to 234.4 Ma with a concordia U-Pb age of 229.37 ± 0.71 Ma (MSWD = 2.6) (Figure 5A). The 206Pb/238U ages of the second group range from 189.7 to 211.4 Ma (Figure 5A).
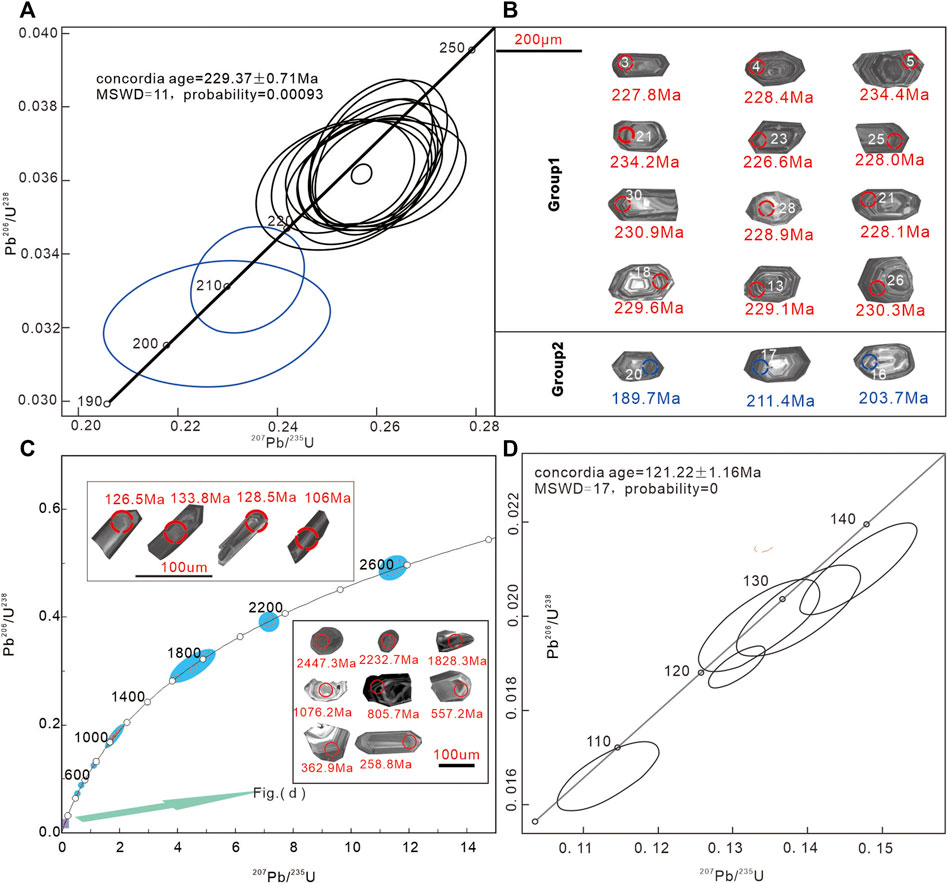
FIGURE 5. Zircon U–Pb concordia diagrams (A) and typical CL images (B) of Guandimiao granite porphyry; Zircon U–Pb concordia diagrams of Guandimiao lamprophyre (C,D). The black circles in a and b represent 206Pb/238U ages of group1 zircons, the blue circles represent 206Pb/238U ages of group 2 zircons.
5.1.4 L4
Two distinct groups of zircons were identified. The first group of zircon grains are complex and exhibits length-to-width ratios from 1:1 to 2:1 and sizes from 50 to 200 μm, Most of them developed obvious oscillatory zoning and are generally incomplete in shape, wheras a small amount is smaller in size (50–100 μm) and round in shape. The second group exhibits length-to-width ratios from 3:1 to 4:1 and sizes of approximately 100–150 μm with U contents from 249.8 to 1,195.2 ppm, Th contents from 324.4 to 1,348.3 ppm, and Th/U ratios from 1.0 to 1.3. Out of 61 spot analyses of L4, 4 analysis points yield 206Pb/238U ages of 106.0–133.8 Ma, with a concordia U-Pb age of 121.22 ± 1.16 Ma (MSWD = 7) (Figures 5C,D). The first group of zircons exhibits a long-term span and contains at least nine stages of magmatism 1) 217.7–227.8 Ma; 2) 240.6–268.0 Ma; 3) 295.9–379.6 Ma; 4) 405.3–466.8 Ma; 5) 539.1–615.4 Ma; 6) 769.7–842.8 Ma; 7) 972.4–1,135.0 Ma; 8) 1,625.9–1931.6 Ma, and 9) 2,114.4–2,573.1 Ma, which could reveal the long chronological evolutionary history of South China.
5.2 Geochemistry
The obtained geochemical result of samples from Guandimiao Batholith are listed in Table 2.
G1 has low SiO2 (69.29 wt%) and total alkalis (ALK = Na2O + K2O) (7.27 wt%) and higher Al2O3 (14.6 wt%), MgO (1.58 wt%), and CaO (2.7 wt%). The A/CNK and A/NK values are 1.00 and 1.51, respectively. G2-1 has high Al2O3 (14.36–14.71 wt%), ALK (7.76–8.53 wt%) and moderate SiO2 (67.9–70.1 wt%) and FeOT (2.96–3.39 wt%) contents and K/Na ratios (0.69–1.82). The ratios of A/CNK are 0.89–1.32, and those of A/NK are 1.24–1.58. The G2-2 have high contents of SiO2 (74.09–75.2 wt%), ALK (6.43–7.30) and K/Na ratios (1.37–1.67) but low MgO (0.39–0.44 wt%), CaO (0.26–0.41 wt%), and FeOT (1.22–1.47 wt%) contents.The G-3 is similar to the G2-2 but have higher content of SiO2 (76.7 wt%), K2O (4.84 wt%), ALK (7.63 wt%). L4 is characterized by low contents of SiO2 (55.43–57.14 wt%), K2O (0.11–1.01 wt%), ALK (4.12–6.70 wt%) and high contents of Al2O3 (12.83–17.02 wt%), FeOT (6.66–7.31 wt%) and MgO (0.86–7.19 wt%). The SiO2 versus (Na2O + K2O) and Zr/TiO2 versus Nb/Y diagrams suggest monzonite-diorite affinity for G1 and G2-1, granite affinity for G2-2 and G3, and calc-alkaline lamprophyre for L4 (Figures 6A,B). On the A/CNK versus A/NK diagram and SiO2 versus K2O diagram, all samples plot in the metaluminous-peraluminous field, whereas G1, G2-1, G2-2, and G3 hold middle-high K calc-alkaline (CA) characteristics, and L4 has middle to low K calc-tholeiitic series affinity (Figures 6C,D).
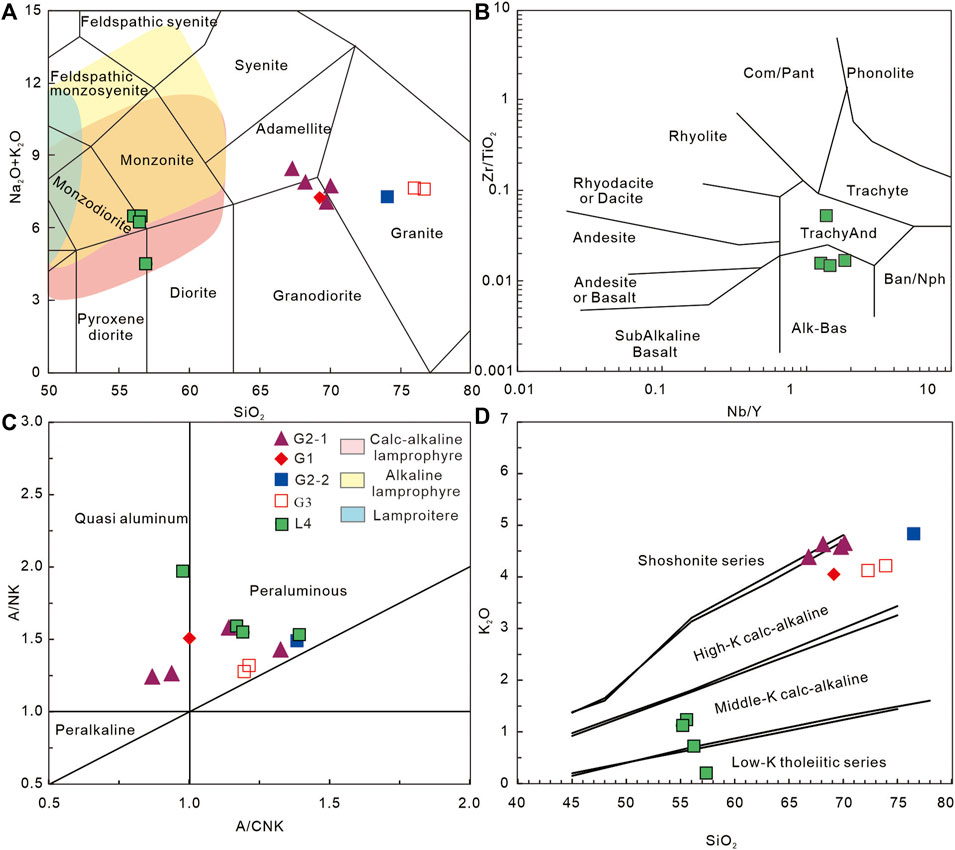
FIGURE 6. (A), TAS classification diagram of Guandimiao batholith; (B), SiO2 versus K2O diagram; (C), A/NK versus A/CNK diagram; (D), K2O versus SiO2 diagram.
The chondrite-normalized REE patterns of G2-2 and G3 show strongly negative Eu anomalies (δEu = 0.21–0.23) and have obviously lower differentiation of LREEs and HREEs [(La/Yb)N = 10.54–11.29, LREEs/HREEs = 8.84–9.81] than G1 and G2-1. The REE pattern of L4 has negative slopes with no obvious negative Eu anomalies (Eu/Eu* = 0.73–0.78). Except for HNGDM-7, L4 shows the lowest fractionation of REEs ((La/Yb)N = 9.66–10.98, LREEs/HREEs = 9.80–11.35). Spider diagrams normalized by a primitive mantle show entiched Rb, Th, U, La, Ce, Nd, Zr, and Hf and depleted Ba, Nb, Ce, P, and Ti for G1 and G2-1 (Figure 7A). G2-2 and G-3 are enriched in Rb, K, Th, U, La, Ce, Hf, and Y and depleted in Ba, Nb, Sr, Zr, and Ti (Figure 7B). L4 is enriched in Rb, Th, U, Nd, and Hf but depleted in K, Nb, Sr, and P. Sample HNGDM-7 of L4 obviously deviates from the main trend and presents stronger K, Nb, and Sr depletion and Th, U, La, Ce, Nd, and Hf enrichment (Figure 7C). The chondrite-normalized REE patterns of G1 and G2-1 are similar (Figure 7D), exhibiting negative slopes with strong light-heavy rare earth element fractionation [(La/Yb)N = 14.75–19.21; LREE/HREE = 12.00–14.08] and negative Eu anomalies (Eu/Eu* = 0.44–0.51). G1 and G2-1 have low Rb/Sr ratios (1.71–2.80) and high K/Rb ratios (136–155). However, G2-2 and G-3 have relatively higher Rb/Sr ratios (10.5–14.19) and lower K/Rb ratios (71.09–117). All granitic samples have Zr + Nb + Ce + Y values from 124.3 to 295.7 ppm, and the 10000 × Ga/Al ranges from 2.09 to 3.02, which is lower than the boundary value of 2.6 for A-type granite (Whalen et al., 1987),.
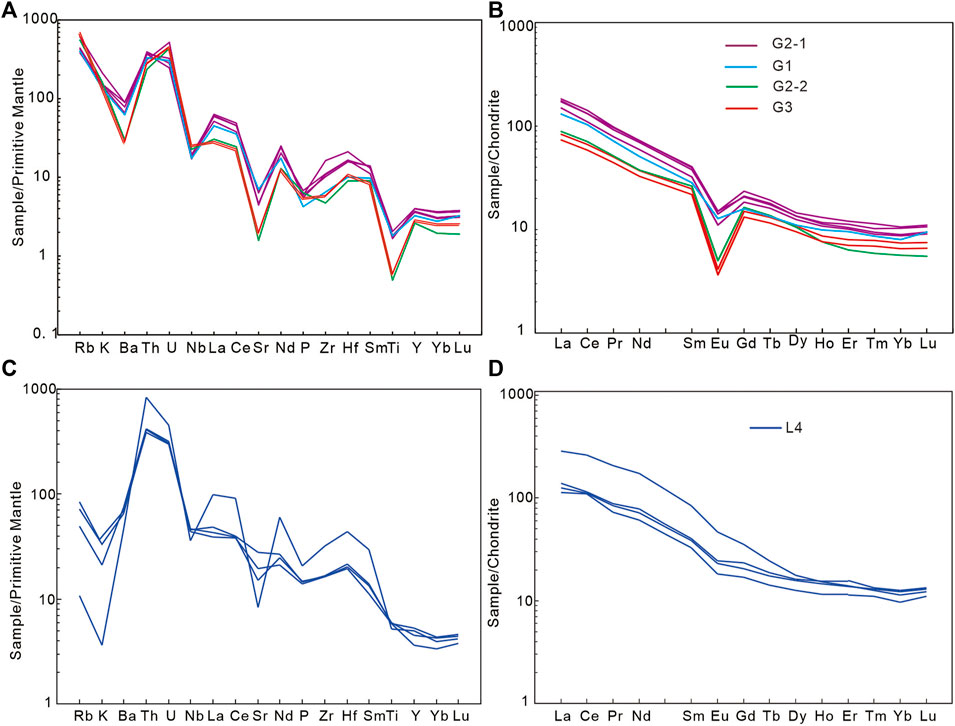
FIGURE 7. Rare earth element pattern and spidergrams of Guandimiao batholith (Standardized values according to Sun and McDonough, 1989); (A,B), Standardization diagram of chondrite meteorites of REE; (C,D), normalization diagram of the primitive mantle of trace elements.
5.3 Sr–Nd–Pb isotopes
The εNd(t) values were calculated at 226–224 Ma for G2-1, 227–225 Ma for G2-2, 239 Ma for G1, 190 Ma for the granite porphyry and 121 Ma for L4 on the basis of zircon U-Pb dating. The Sr-Nd-Pb isotope compositions of the rocks from the Guandimiao batholith are shown in Table 3. The 87Rb/86Sr ratios of the Guandimiao batholith range from 7.95 to 8.74, and the calculated ISr values range from 7.1229 to 7.1796 (G2-2 and G3 have high 87Rb/86Sr ratios, indicating that the calculated results cannot represent the actual isotopic compositions). The 87Rb/86Sr ratios of L4 range from 0.11 to 0.38, and the calculated ISr values range from 0.71449 to 0.71748. The calculated εNd(t) values are −9.37 to −10.54 for G2-1, −6.95 for G1 and −12.14 for G2-2. The 143Nd/144Nd ratios and calculated εNd(t) values are 0.512558 and −8.96 for G3 and 0.512042–0.512071 and −10.18 to −9.62 for L4, respectively. Since the fSm/Nd of the samples deviates from the mean value for continental crust, a two-stage model is used. Thus, we obtain two stages of depleted mantle model ages (TDM2) based on the model reported by Depaolo (1981). The calculated two-stage model ages are 1859–1764 Ma for G2-1, 1,568 Ma for G1, 1730 Ma for G2-1, 1928 Ma for G3 and 1,577–1,435 Ma for L4.
The 206Pb/204Pb, 207Pb/204Pb, and 208Pb/204Pb ratios of G2-1 vary from 18.821 to 18.611, 15.707–15.712, and 38.977–39.056, respectively. For G1, the 206Pb/204Pb ratio is 18.707, the 207Pb/204Pb ratio is 15.71, and the 208Pb/204Pb ratio is 39.109. For G2-2, the 206Pb/204Pb ratio is 18.701, the 207Pb/204Pb ratio is 15.701, and the 208Pb/204Pb ratio is 38.887. For G3, the 206Pb/204Pb ratio is 19.21, the 207Pb/204Pb ratio is 15.738, and the 208Pb/204Pb ratio is 39.081. For L4, the 206Pb/204Pb ratio is 18.95–19.96, the 207Pb/204Pb ratio is 15.71–15.78, and the 208Pb/204Pb ratio is 39.87–43.090. These data reflect relatively uniform Pb isotope ratios. The initial Pb isotope ratios of the samples were calculated using the formulas of Hart (1984). The calculated results for (206Pb/204Pb)i, (207Pb/204Pb)i, and (208Pb/204Pb)i range from 17.875 to 18.113, 15.664–15.682, and 37.75–37.787, respectively, for G2-1. For G1, the (206Pb/204Pb)i ratio is 18.36, the (207Pb/204Pb)i ratio is 15.692, and the (208Pb/204Pb)i ratio is 38.613. For G2-2, the (206Pb/204Pb)i ratio is 17.662, the (207Pb/204Pb)i ratio is 15.648, and the (208Pb/204Pb)i ratio is 36.992. For G3, the (206Pb/204Pb)i ratio is 18.588, the (207Pb/204Pb)i ratio is 15.707, and the (208Pb/204Pb)i ratio is 38.57. For L4, the (206Pb/204Pb)i ratios range from 18.31 to 19.72, the (207Pb/204Pb)i ratios range from 15.68 to 15.77, and the (208Pb/204Pb)i ratios range from 39.79 to 42.94. All analyzed Pb isotope compositions are shown in Table 4.
6 Discussion
6.1 Magmatism stages of the guandimiao batholith
Previous research on the biotite granodiorite of the Guandimiao batholith found that it formed 239 Ma (Wang et al., 2005a). The biotite monzogranite and two-mica monzogranite have a wide range of formation ages (230–220 Ma; Huang and Depaolo, 1989; Zhao et al., 2015). The Zhoujialing biotite granite exposed west of the Guandimiao batholith are formed at 208–203 Ma (Miao, 2014). Dai et al. (2008) determined that the formation age of lamprophyre was 153 Ma (Table 5).
In this study, high-precision LA-ICP-MS zircon U-Pb data were obtained for the Guandimiao batholith. The U-Pb data for G2-1 range from 225.9 ± 0.72 to 223.64 ± 1.33 Ma and for G2-2 from 227.5 ± 1.0 to 225.5 ± 2.2 Ma. These ages indicate that the main part of the batholith was formed in the Late Triassic. Due to the high emplacement and fast cooling of G3 and L4, the time was insufficient for the crystallization of sufficient zircon crystals. In addition, mafic magma is generally unsaturated silicon, and it is very hard to crystallize sufficient and well-formed zircon grains. In G3, two age groups were identified. The first group of ages yields a concordia U–Pb age of 229.37 ± 0.71 Ma, much closer to the main ages of the batholith within the allowed error range. These results suggest that the U-Pb ages of zircons were captured from G2-1 and G2-2. The second group of ages of zircons ranges from 189.7 to 211.4 Ma, which is significantly lower than those of G2-1 and G2-2. This more likely represents the crystallization age of G3. Four zircon grains in group one collected from lamprophyre formed 106.0 to 133.8 Ma and yield a concordia U-Pb age of 121.22 ± 1.16 Ma. However, the U-Pb ages of zircons from group two range from 217.7 to 2,573.1 Ma. Due to the same characteristics as those from felsic granite (G1, G2-1, G2-2, and G3) and the long-term temporal span, there is no doubt that it was from the succession of the source area or the capture crystals from the surrounding rocks.
Thus, four main stages of magmatism can be determined in the Guandimiao batholith: Stage 1 (G1; 239 Ma)→Stage 2 (G2-1, G2-2; 230–203 Ma)→Stage 3 (G3; 211–190 Ma)→Stage 4 (L4; 121 Ma) (Figure 8). Zhou et al. (2006) proposed that the formation ages of igneous rocks in the SCB contain two principal periods and five main stages. The Indosinian magmatism was between 251 and 205 Ma and can be divided into two stages (251–234 Ma and 234–205 Ma). The first stage formed 251–234 Ma and is defined as S-type granite with high aluminum contents. The 234–205 Ma stage was dominated by postcollisional granitoids without contemporaneous volcanic activity. The contemporaneous G1, G2-1, and G2-2 developed in the main body of the Guandimiao batholith. Lower Yanshanian igneous rocks (180–147 Ma) of the SCB, containing high K calc-alkaline I-type granitoids, are widespread and distributed as parallel belts. Upper Yanshanian igneous rocks contain early-stage granitic-volcanic rocks and late-stage mafic rocks in south-southeastern China. Correspondingly, the Yanshanian felsic and mafic dikes (G3 and L4) were emplaced at the center and margin of the Guandimiao batholith. Igneous rocks of the Guandimiao batholith contain a complete record of multiple stages of magmatism and tectonic events since the Indosinian.
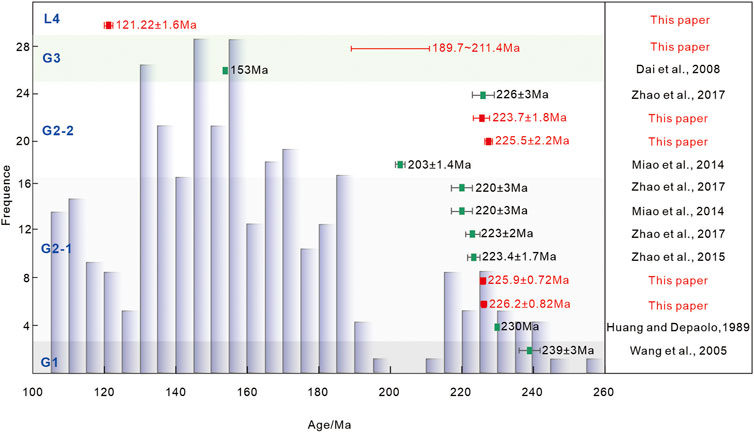
FIGURE 8. Histogram of formation ages of South China and distribution diagram of formation ages of Guandimiao batholith. The data of south China are from Zhou et al., 2006.
6.2 Petrogenesis
6.2.1 Genesis type of granitic rocks
The S- and I-type scheme was initially proposed by Chappell and White (1974); Chappell and White (1992), and this letter scheme classification has been widely developed in later studies (Loiselle and Wones, 1979; Whalen et al., 1987; Eby, 1990; Eby, 1992; King et al., 1997; Bonin, 2007; etc.). The mineral assemblage, with the absence of typical alkaline mafic minerals, indicates I- and S-type granitic characteristics for Guandimiao granitoids. In addition, G1 and G2-1 of the Guandimiao batholith have low SiO2 and high FeOT contents and K/Na and FeOT/MgO ratios; G2-2 and G3 are enriched in SiO2 contents, Al2O3 contents, and K/Na ratios but depleted in MgO and CaO contents. The values of (Zr + Nb + Ce + Y) for the Guandimiao granitoids range from 138 to 295.7 ppm. The ΣREEs are 96.2–193 ppm, and 10,000 × Ga/Al ratios are 2.09–3.02. These values are significantly lower than the lowest values observed in A-type granite (Whalen et al., 1987). Whole-rock Zr saturation temperatures suggest that crystallization temperatures (714–785°C) are consistent with crystallization temperatures < 800°C of I- and S-type granite rather than crystallization temperatures (>8 00°C, even possibly > 1,000°C) of A-type granite (Clemens et al., 1986). All samples plot in the S- and I-type granite fields on the FeOT/MgO versus Zr + Nb + Ce + Y (Figure 9A).
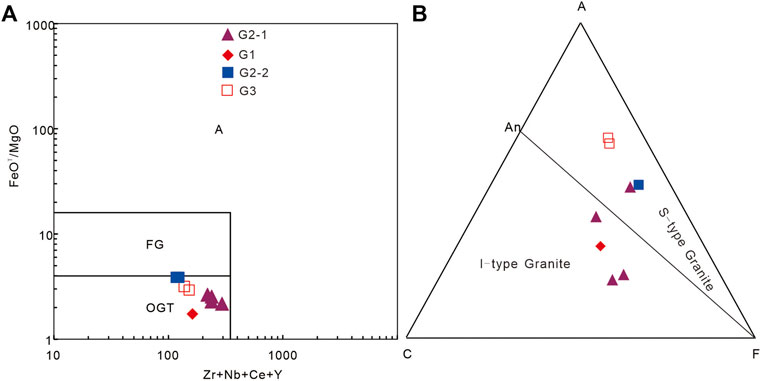
FIGURE 9. Discriminant diagram of type A granite (after Whalen et al., 1987); (A), FeOT/ MgO versus Zr + Nb + Ce + Y diagram; (B), ACF diagram of Guandimiao batholith (after Hine et al., 1978).
On the ACF classification diagram, G2-2 and G3 plot in the S-type granite field, whereas G1 and G2-1 plot within the I-type granite field (Figure 9B).
6.2.2 Origin of the guandimiao batholith
The Pb isotope compositions of the Guandimiao granitoids present higher radiogenic Pb isotope compositions than syntectic granite and plot in the remelting granite of the South China field on the (207Pb/204Pb)i versus (208Pb/204Pb)i diagram and 207Pb/204Pb versus 206Pb/204Pb diagram. It is also above the mantle evolution line proposed by Hart (1984) (Figures 10A,B). This suggests that the Pb was mainly derived from the upper crust.
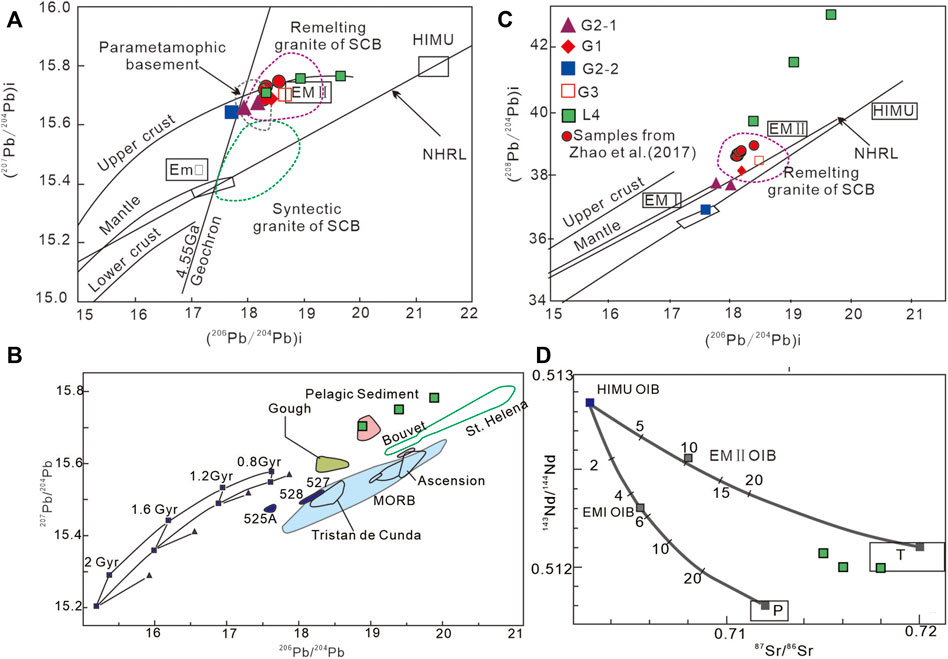
FIGURE 10. (A), (207Pb/204Pb)i versus (208Pb/204Pb)i diagram of Guandimiao batholith; (B), The 207Pb/204Pb versus 206Pb/204Pb diagram of Guandimiao batholith (lines are after Zartman and Doe, 1981); (C), 207Pb/204Pb versus 206Pb/204Pb diagram of lamprophyre; (D), 143Nd/144Nd versus 87Sr/86Sr diagram of lamprophyre (after Weaver, 1991). Lines are after Zartman and Doe, 1981; data sources: EM (Zindler and Hart, 1986; Hofmann, 2003), NHRL (Hart, 1984), the Cathaysia Block parametamorphic basement (Hu, 1998), remelting and syntectic granites in the SCB (Shen et al., 1992). The datas of Hawaii, MORB, Gough, Kerguelen, Iceland, Easter Island are from Sun (1980). The Hawaii-OIB, HIMU, EMI, and EMII datas are modified from Hanan et al. (2008). NHRL-northern hemisphere reference line, HIMU-high U/Pb Mantle, EM-enriched mantle, OIB-ocean ridge basalt, P-pelagic sediments, T-terrigenous sediments.
However, the existence of different genetic types (I- and S-type) and lithological compositions of intrusive rocks indicates diverse sources for the Guandimiao batholith (Chappell and White, 1974; Chappell et al., 1988). Zhou (2003) noted that the Indosinian granitoids are scattered across South China. Because of the uneven thickness of the Earth’s crust, the granitoids are characterized by multiple sources and are dominated by thickened continental crust. G1 and G2-1 of the Guandimiao batholith enclosed mafic microgranular enclave, which might have been formed by the rapid crystallization of mafic minerals upon chilling and reduction in viscosity instead of by the mixing of mantle-derived components (Vernon, 1984; Sparks and Marshall, 1986; Zhao et al., 2017).
In addition, G1 and G2-1 have obvious I-type granite affinities and are characterized by relatively high εNd(t) values (−6.17 to −10.54), low initial 87Sr/86Sr ratios (0.712005–0.71758) and TDM2 values (1.52 Ga for G1 and 1.85 to 1.76 Ga for G2-1). G2-2 and G3 show S-type granite affinities and have high initial 87Sr/86Sr ratios (0.7344) and TDM2 values (1.73 Ga for G2-2 and 1.93 Ga for G3) but lower εNd(t) values (−8.96 to −12.14). All samples show characteristics of crustal sources on the T versus εNd(t) diagram (Figure 11A). The AMF [molar Al2O3/(FeOT + MgO)] values of G1 and G2-1 range from 1.36 to 1.65, much lower than the values of G2-2 and G3 (3.59–4.63). The CMF [molar CaO/(FeOT + MgO)] values of G1 and G2-1 are between 0.47 and 0.62, whereas the G2-2 and G3 values range from 0.24 to 0.27. On the diagram of AFM versus CFM, G1 and G2-1 are derived from metabasaltic to metatonalitic source fields, which is consistent with the Jingtou granite, which was derived from a high-K calc-alkaline amphibolite source with metabasaltic to metatonalitic compositions (Figure 11B; Altherr et al., 2000; Zhao et al., 2017). In contrast, G2-2 and G3 plot near the Shizhuqiao granite field, indicating a source similar to the para-metamorphic basement of the Cathaysia Block (Shen et al., 1993; Altherr et al., 2000; Zhao et al., 2015; Zhao et al., 2017).
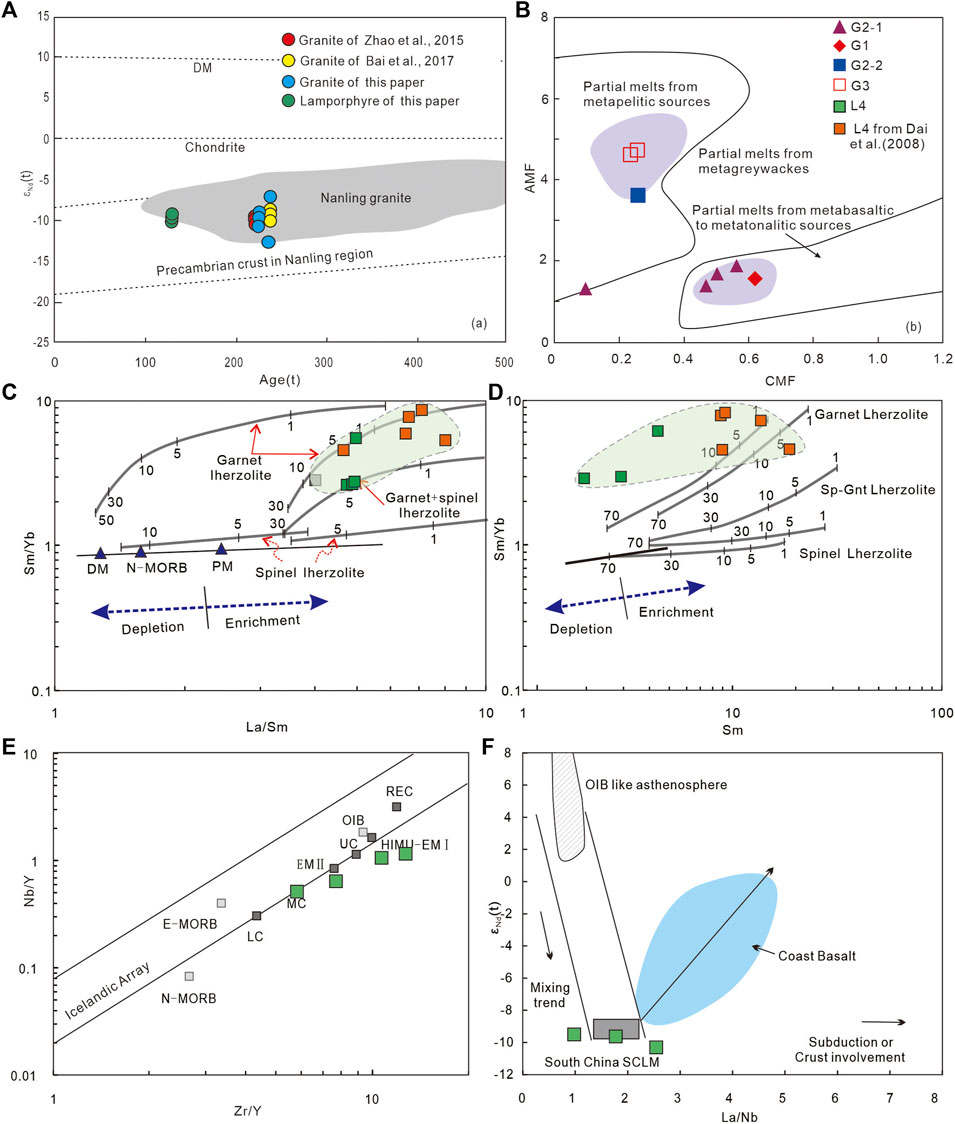
FIGURE 11. (A), εNd (t) versus Age diagram; (B), AMF versus CMF diagram; (C,D), Sm/Yb versus La/Yb diagram and Sm/Yb versus Sm of lamprophyre; (E), Nb/Y versus Zr/Y diagram; (F), εNd (t) versus La/Nb diagrams. Data source: Nanling granite (Yuan and Zhang, 1992), Precambrian crust in Nanling region (Shen et al., 1993). The gray field data from Zhao et al., 2015; Zhao et al., 2017; Orange samples from Dai et al., 2008; Coast Basalt field from Meng et al. (2012); South China SCLM from Chen et al. (2008b). Panels (C,D) are modified after Aldanmaz et al. (2000); DM from Mckenzie and Keith, 1995, N-MORB and PM from Sun and McDonough (1989). Melting curves of Spinel lherzolite (Ol53 + Opx 27 + Cpx 17 + Sp 11) and Garnet lherzolite (Ol 60 + Opx 20 + Cpx 10 + Gt 10) are from Aldanmaz et al. (2000).
Moreover, the variation features of two stages of Sm-Nd isotope model ages show an inhomogeneous source. The TDM2 of G1 is much younger than those of metamorphosed basement in the Cathaysia block (1.8–2.2 Ga) and might be derived from the remelting of meta-juvenile crust in the Mesoproterozoic. The TDM2 values of G2-1, G2-2 and G3 range from 1.74 to 1.93 Ga, which is consistent with the Paleoproterozoic metamorphosed basement of the Cathaysia block. These results amply justify their geological affiliation. L4 of the Guandimiao batholith has low εNd(t) values (−10.18 to −9.62) but high Isr (0.71449–0.71784) and TDM2 values (1.75–1.70 Ga), which represents significant deviation from the Mesozoic EM II-type OIB in the SCB (6.8–10.8; Meng et al., 2012; Qin et al., 2020). The 207Pb/204Pb versus 207Pb/204Pb and 143Nd/144Nd versus 87Sr/86Sr diagrams show that L4 was derived from a Mesoproterozoic pelagic and/or terrigenous sediment source (Figures 10C,D).
Garnet and spinel were mainly La-, Sm-, and Yb-bearing minerals in the magma source. They are significantly insensitive and can be used as an indicator to trace the melt source and the degree of partial melting (Aldanmaz et al., 2000; Zhao and Zhou, 2008). As the partial melting of a garnet lherzolite occurred in the source, the Sm/Yb ratio decreased sharply, while the La/Sm ratio decreased gradually. The partial melting of garnet lherzolite produces higher Sm/Yb ratios than spinel lherzolite melting (Johnson, 1994; Aldanmaz et al., 2000). The La/Sm versus Sm/Yb and Sm/Yb versus Yb diagrams show that the lamprophyre samples plot near the garnet lherzolite melting curves. These results suggest that the lamprophyre was mainly formed by partial melting of garnet lherzolite. However, a wide degree of partial melting is also presented in Figures 11C,D, which indicates extensive crustal contamination. The same conclusion can also be drawn from the Nb/Y versus Zr/Y and εNd(t) versus La/Nb diagrams. The lamprophyre samples deviate from the Iceland array and plot in the field of mixing trends between the OIB-like asthenosphere and South China SCLM. It shows strong crustal information (Condie, 2005; Meng et al., 2012; Figures 15E,F).
6.2.3 Magmatism
During granitic magmatism, the main Ti-bearing minerals (ilmenite, rutile, titanite, anatase, etc.) could lead to a significant depletion of Ti, Nb, Ta, etc. The fractional crystallization of apatite could significantly decrease the P concentration in the residual melt. Eu, Sr, and Ba could be depleted during fractional crystallization of feldspar, as they usually substitute K+ and Ca2+ sites into K-feldspar and/or plagioclase. In the Guandimiao batholith, there is significant depletion in Ba, Nb, Sr, and Ti for G2-2 and G3 but less depletion in Ba, Nb, Sr, P, and Ti for G1 and G2-1. This may suggest that the Guandimiao granitoids experienced obvious fractional crystallization of feldspar, Ti-bearing minerals and apatite at the early stages of magmatism. In addition, the negative correlations between Ba and Sr and between Rb and Sr suggest that the magmatic system underwent intense fractional crystallization of K-feldspar and plagioclase (Figures 12A,B). For the rare earth elements (La and Yb), carrier minerals include zircon, apatite, allanite, monazite, etc. The negative relationship between La and La/Yb suggests that the fractional crystallization of allanite and monazite also played an important role in the evolution of magmatism (Figure 12C). It is worth mentioning that G1 and G2-1 have higher K/Rb ratios and lower Rb/Sr ratios than G2-2 and G3. More proof is required that the late stage of magmatism was higher in the degree of fractionation than the early stage (Figure 12D). The granitoids of the Guandimiao batholith were the result of multistage differentiation of an identical long-term magma chamber.
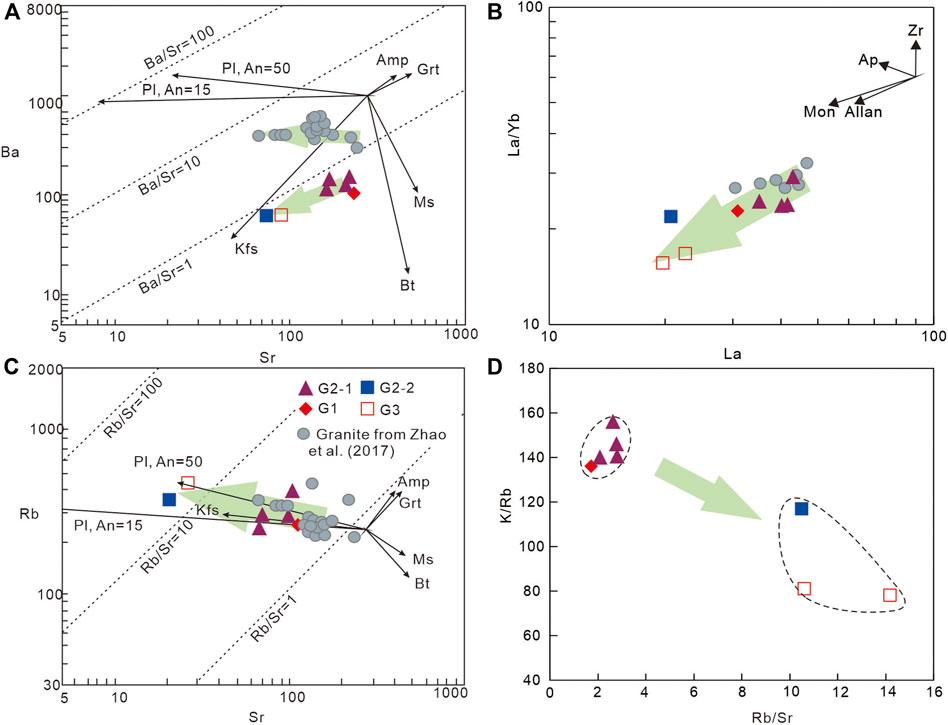
FIGURE 12. (A,B), Ba versus Sr diagram and Rb versus Sr diagram of granitoids from Guandimiao batholith; (C), La/Yb versus La diagram; (D), K/Rb versus Rb/Sr diagram.
6.3 Implications for tectonic evolution
6.3.1 Complete record of the pre-mesozoic tectonic event of the SCB
Nine stages of lamprophyre (L4) fully reveal the episodic growth of the South China plate (Figure 13B). Similar evidence has been reported by Shu (2012) and Shu et al. (2020). Five time intervals of detrital zircons dating from the Jinggangshan–Nanling region (2.56–2.38 Ga; 1.93–1.52 Ga; 1.3–0.9 Ga; 0.85–0.73 Ga; and 0.68–0.55 Ga) have been identified. The 5 time intervals could also be identified by detrital zircons from Hubei Province (Gaojiabei Formation), Jiangxi (Huangdongkou Formation, Jueshangou Formation and Tiaomajian Formation), Guangdong Province (gneiss), Sichuan Province (Pingyipu Formation), and Guizhou Province (Dazhuyuan Formation; Shu, 2012; Zhao et al., 2013; Wang D. H. et al., 2014; Wang X. L. et al., 2014) (Figure 13A).
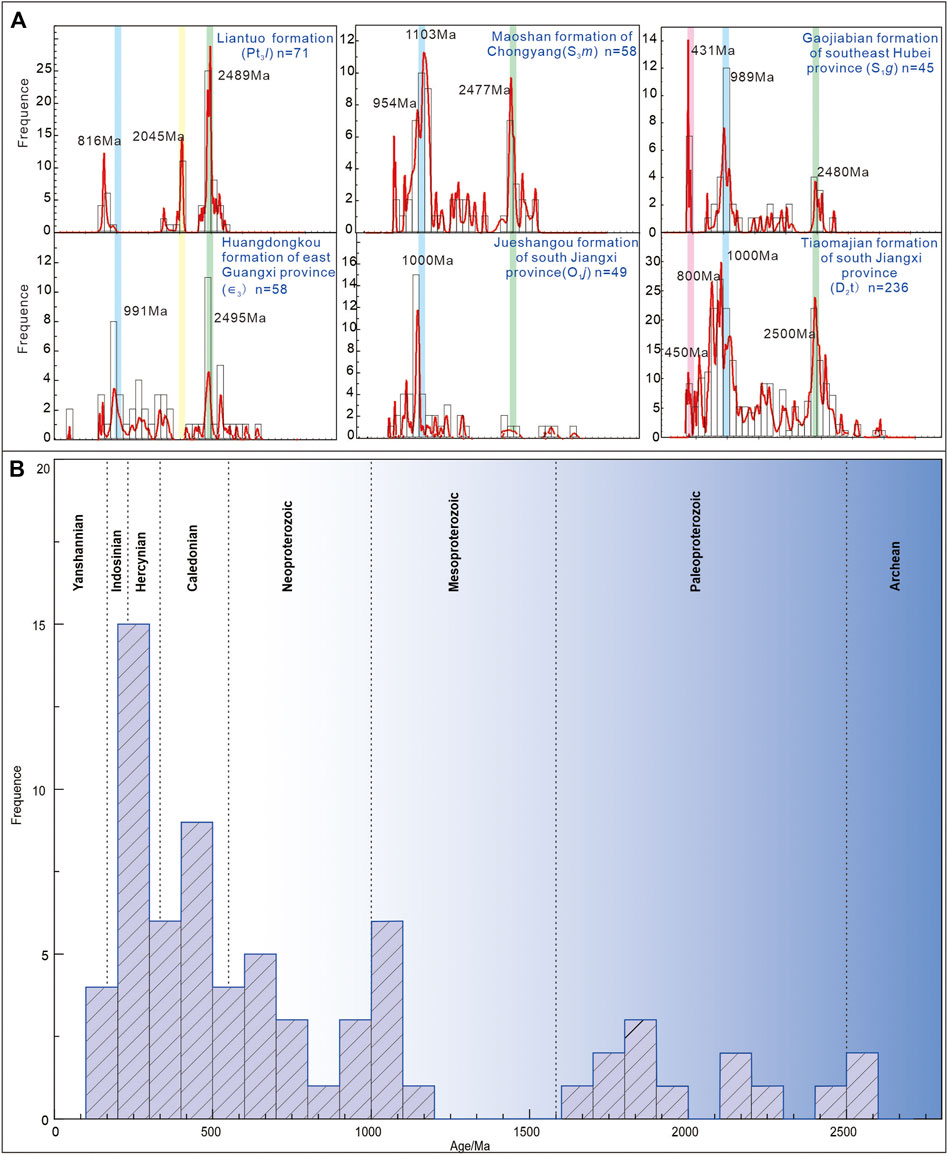
FIGURE 13. (A), Histogram of model ages of detrital zircons of South China, (B), histogram of zircon U-Pb ages of lamprophyre from Guandimiao batholith.
The Neoarchean to Paleoproterozoic ages (2,573.1–2,447.3 Ma) were extensively derived from xenolith crystals in mafic dikes and clastic zircons in Paleozoic strata (Zheng et al., 2008). They are obviously younger than the Kongling Group in the Yangtze block (3.3 Ga, Ma et al., 1997). They represent global continental nucleus growth events and mixing processes between Neoarchean mafic magma and more ancient crustal components.
The middle to late Paleoproterozoic data (2,232.7–2,114.4 Ma and 1931.6–1,625.9 Ma) is linked to the response of the convergence and breakup effect of the Columbia supercontinent. The convergence of the Columbia supercontinent began 2.1 Ga, which is represented by the formation of the Kora-Kareline conjunctive craton and West Australian craton and the outgrowth of the western Amazon ancient continental plate margin in South America (Hoffman, 1988). Correspondingly, significant tectonic magmatic events were also recorded in the North China craton (NCC) and Yangtze craton (Zhang et al., 2006; Yin et al., 2013). Subsequently, the tectonic regime transformed to breakup on a global scale 1.7–1.5 Ga. A large number of A-type granitoids and mafic dikes developed in the Yangtze craton, indicating the existence of intracontinental rifting (Rogers and Santosh, 2002; Zhao et al., 2002; Evans and Mitchell, 2011; Gibson et al., 2012; Geng et al., 2019).
During the early Neoproterozoic (1,135.0–769.7 Ma), the region experienced the suture of the Paleo-Huanan Ocean along the Jiangnan–Shaoxing large fault zone, and the collision between the Yangtze block and Cathaysia block created the initial appearance of the ancient SCB. This was completely documented by abundant ophiolites and associated pyroclastic rocks developed along the Dexin–Dongxiang and Zhuji–Lishui–Wuyi–Meixi belts in the southeastern Cathaysia block from 1.0–0.9 Ga. The I-type granite and mafic rocks of the southern Jiangnan orogenic belt, which formed 0.9–0.8 Ga, indicates the subduction of pristine oceanic crust (Ye et al., 2007; Shu et al., 2012; Zhou et al., 2018). The subsequent break-up of the supercontinent and extension events occurred along the paleo-suture and formed the Nanhua continental rift basin and three main tectonic domains (Nanling, Wuyi, and Yunkai) 825–760 Ma (Wang et al., 2017; Yang et al., 2018). The eventual Phanerozoic collage of the SCB (early Paleozoic, 466.8–405.3 Ma) and multistage intracontinental orogenic events (late Paleozoic, 268.0–258.8 Ma; 379.6–295.9 Ma) constituted the basic tectonic appearance in the Paleozoic (Liu et al., 1990; Shu et al., 2006; Shu et al., 2012).
6.3.2 Mesozoic tectonic evolution of the SCB
The Guandimiao batholith is the central part of the SCB. The G1, G2-1 and G2-2 phases were emplaced 239–203 Ma and were dominant in the period from 230 to 220 Ma. The structural discriminant diagram shows that these rocks formed in a postcollisional environment (Figure 14A; post-COLG field; Pearce, 1996). The lamprophyre plots near the WPG field and Ⅵ3 field on the Rb versus Y + Nb diagram (intraplate basalt) (Figure 14B), which indicates an intracontinental extensional setting.
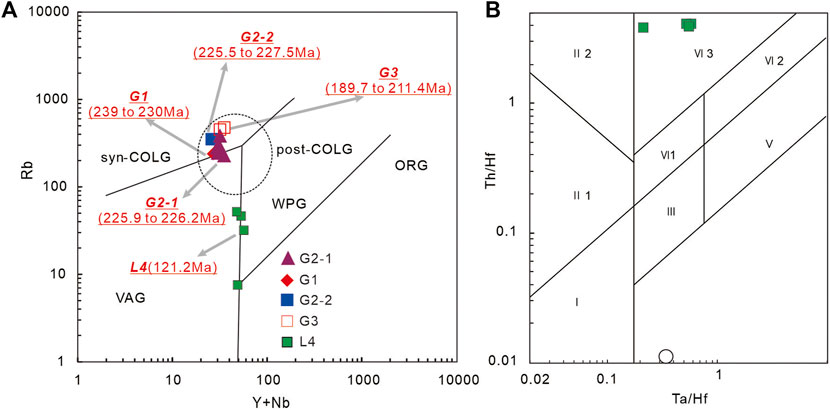
FIGURE 14. Rb versus Y + Nb diagram (A) and Th/Hf versus Ta/Hf diagrams (B) of Guandianmiao batholith. Syn-COLG, syncollisional granite, VAG, volcanic arc granite, WPG, within plate granite;ORG, ocean ridge granite. I, Extensional plate margin N-MORB; II1, Oceanic island arc basalt; II2, Continental margin island arc basalt; III, Oceanic intraplate basalt, seamount basalt and T-MORB, E-MORB; IV1, Intracontinental and continental marginal rift tholeiite basalt; IV2, Intracontinental rift alkaline basalt; IV3, Continental extensional basalt; V, Mantle plumes related basalt.
During the early Indosinian (250–240 Ma), the forceful collision and extensive metamorphism between the Indo-China block and the Sibumas-Qingtang block occurred in the Early-Middle Triassic and exerted a profound impact on the southeastern Eurasian continent (Chen et al., 2002; Li et al., 2006). Meanwhile, the closure of the Paleo-Tethys Ocean caused southeastward subduction and collision between the NCC and the SCB. The SCB underwent multidirectional compression and massive shortening, accompanied by an approximately 50 km thickening of the continental lithosphere (Tapponnier et al., 1982; Gilder et al., 1995; Carter et al., 2001; Wang et al., 2002; Li et al., 2017) (Figure 15A). The collisional orogenic events immensely shortened the crust and resulted in a series of thrust faults and uplift structures. There was an approximately 10 Myr. Interval before granitoids melted following the end of crustal thickening (Patio Dounce et al., 1990), and Sylvester (1998) proposed a possible relaxation and thinning of the thickened crust with a time interval of 10–20 Myr. The Guandimiao dome developed at the center of the SCB. The main part of the Guandimiao batholith was formed in a postcollision setting after the Indosinian crustal thickening events in the SCB. The thermal stress from crustal extension caused decompression and melting of thickened crust, along with continuous emplacement of mantle-derived magma (Pape et al., 2012). It induced extensive melting of Paleoproterozoic metamorphic basement in the SCB (Zhou et al., 2006; Guo et al., 2012; Qin et al., 2022) (Figure 15B).
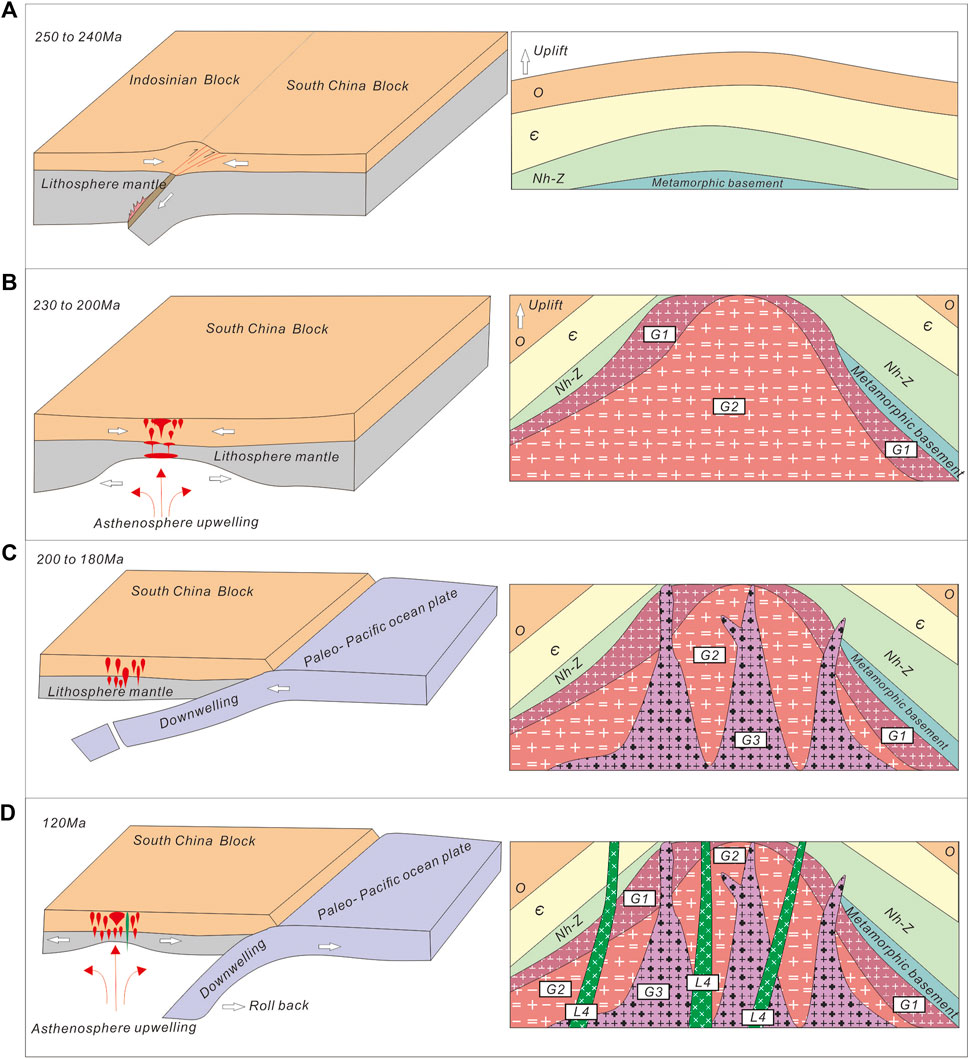
FIGURE 15. The brief model diagram of the tectonic evolution of south China at Mesozoic and the emplacement model of Guandimiao batholith. (A) the early Indosinian period (250–240 Ma); (B) the late Indosinian period (230–220 Ma); (C) the early Yanshanian period (200–180 Ma); (D) the late Yanshanian period (120 Ma).
Approximately 200–180 Ma, the transformation of the tectonic regime occurred from NE-directed paleo-Tethys ocean dominance to NE-directed paleo-Pacific ocean dominance. The continued subduction of the paleo-Pacific plate beneath the Eurasian plate affected more than 1,000 kilometers. Due to the stable structure and the shortage of transcrustal faults, there was a significant deficiency in magmatic rocks, and the volume and scale of magmatism were far less than those developed either in the early Insinian or late Mesozoic and have been confirmed as the break-in period (200–180 Ma) of igneous rocks in South China (Mao et al., 2004; Zhang et al., 2009) (Figure 15C). Scattershot intrusions occurred as stocks and dikes exposed in the interior batholith with limited scope.
In the Middle Jurassic, subduction of the paleo-Pacific oceanic plate converted to rollback steep subduction and caused large-scale extensional events in the back-arc basin. The uplift of asthenospheric mantle melted the ancient metamorphic basement and formed a large granitic melt 135–118 Ma (Zhou et al., 2006; Jiang et al., 2015; Yang, 2018; Li et al., 2019). Moreover, mafic magma rapidly intruded along the deeply penetrating faults and extensively contaminated the upper crustal materials. This made it possible for the formation of lamprophyre with intense crustal information (L4) (Figure 15D).
7 Conclusion
1 The Guandimiao batholith was dominated by four stages of magmatism. The granodiorite formed at 239 Ma (Stage 1). The biotite monzogranite (G2-1) and two-mica monzogranite (G2-2) formed at 230–203 Ma (Stage 2). The granite porphyry formed from 211 to 190 Ma (Stage 3). The lamprophyre (L4) formed 121 Ma (Stage 4).
2 G1 and G2-1 have I-type granite affinity, while G2-2 and G3 show S-type granite characteristics. G1 was derived from the remelting of meta-juvenile crust in the Mesoproterozoic. G2-1, G-2, and G3 were derived from the Paleoproterozoic metamorphosed basement of the Cathaysia block. The source of L4 was garnet and spinel lherzolite, which underwent mixing between the Mesoproterozoic pelagic and/or terrigenous sediment and SCLM of South China. The granitoids of the Guandimiao batholith underwent intensely fractional crystallization of feldspar, Ti-bearing minerals, allanite and monazite.
3 The zircon U-Pb dating of L4 in the Guandimiao batholith completely records the six stages of pre-Mesozoic tectonic events in the SCB. During the Mesozoic, the main body of the Guandimiao batholith (G1, G2-1, and G2-2) recorded the closure of the paleo-Tethys Ocean in the Triassic and the subsequent regional extension of the postcollision. The dikes of the Guandimiao batholith documented the transition of tectonic and dynamic regimes in the early Yanshanian and the rollback and steep subduction of the paleo-Pacific Ocean in the late Yanshanian.
Data availability statement
The original contributions presented in the study are included in the article/Supplementary Material, further inquiries can be directed to the corresponding author.
Author contributions
Conceptualization, J-HQ and FH; methodology, J-HQ; software, J-HQ; validation, FH and D-HW; formal analysis, J-HQ; investigation, J-HQ and FH; resources, J-HQ; data curation, D-HW; writing—original draft preparation, J-HQ; writing—review and editing, J-HQ and FH; visualization, J-HQ; supervision, D-HW; project administration, D-HW; funding acquisition, D-HW. All authors have read and agreed to the published version of the manuscript.
Funding
This study was financially supported by National Natural Science Foundation of China (42172097), China Geological Survey (DD20221695;DD20190379; DD20160346). We are grateful to Mr. Qin Jin-nin, Prof. Liu Shan-bao, Prof. Wang Cheng-hui and other geologists for their help during the field investigations and samples analysis.
Conflict of interest
The authors declare that the research was conducted in the absence of any commercial or financial relationships that could be construed as a potential conflict of interest.
Publisher’s note
All claims expressed in this article are solely those of the authors and do not necessarily represent those of their affiliated organizations, or those of the publisher, the editors and the reviewers. Any product that may be evaluated in this article, or claim that may be made by its manufacturer, is not guaranteed or endorsed by the publisher.
References
Aldanmaz, E., Pearce, J. A., Thirlwall, M. F., and Mitchell, J. G. (2000). Petrogenetic evolution of late cenozoic, post-collision volcanism in Western anatolia, Turkey. J. Volcanol. Geotherm. Res. 102 (1-2), 67–95. doi:10.1016/S0377-0273(00)00182-7
Altherr, R., Holl, A., Hegner, E., Langer, C., and Kreuzer, H. (2000). High-potassium, calc-alkaline I-type plutonism in the European Variscides, northern Vosges (France) and northern Schwarzwald (Germany). Lithos 50 (1), 51–73. doi:10.1016/s0024-4937(99)00052-3
Bai, D. Y., Zhong, X., Jia, P. Y., Xiong, X., and Huang, W. Y. (2014). Geochemistry and petrogenesis of the Indosinian Guandimiao granitic pluton in central Hunan. Sediment. Geol. Tethyan Geol. 34 (4), 93–105. (in Chinese with English abstract).
Barbarin, B. (2005). Mafic magmatic enclaves and mafic rocks associated with some granitoids of the central sierra Nevada batholith, California: Nature, origin, and relations with the hosts. Lithos 80 (1-4), 155–177. doi:10.1016/j.lithos.2004.05.010
Bonin, B. (2007). A-Type granites and related rocks: Evolution of a concept, problems and prospects. Lithos 97, 1–29. doi:10.1016/j.lithos.2006.12.007
Carter, A., Roques, D., Bristow, C., and Kinny, P. (2001). Understanding mesozoic accretion in southeast Asia, significance of triassic thermotectonism. Geology 29 (29), 211–214. doi:10.1130/0091-7613(2001)029<0211:UMAISA>2.0.CO;2
Chappell, B. W., White, A. J. R., and Hine, R. (1988). Granite provinces and basement terranes in the lachlan fold belt, southeastern Australia. Aust. J. Earth Sci. 35 (4), 505–521. doi:10.1080/08120098808729466
Chappell, B. W., and White, A. J. R. (1992). I- and s-type granites in the lachlan fold belt. Earth Environ. Sci. Trans. R. Soc. Edinb. 83 (1-2), 1–26. doi:10.1017/S0263593300007720
Chappell, B. W., and White, A. J. R. (1974). Two contrasting granite types, Pacific. Geology 8, 173–174. doi:10.1046/j.1440-0952.2001.00882.x
Chen, C. H., Lee, C. Y., Lu, H. Y., and Hsieh, P. S. (2008b). Generation of Late Cretaceous silicic rocks in SE China, age, major element and numerical simulation constraints. J. Asian Earth Sci. 31, 479–498. doi:10.1016/j.jseaes.2007.08.002
Chen, C. H., Lee, C. Y., and Shinjo, R. (2008a). Was there jurassic paleo-pacificsubduction in south China? Constraints from 40Ar/39Ar dating, elemental and Sr–Nd–Pb isotopic geochemistry of the mesozoic basalt. Lithos 106, 83–92. doi:10.1016/j.lithos.2008.06.009
Chen, P., Hua, R., Zhang, B., Lu, J., and Fan, C. (2002). Early Yanshanian post-orogenic granitoids in the Nanling region. Sci. China Ser. D-Earth. Sci. 45 (8), 755–768. doi:10.1007/bf02878432
Chen, Y. C., Wang, D. H., Xu, Z. G., and Huang, F. (2014). Outline of regional metallogeny of ore deposits associated with the Mesozoic magmatism in South China. Geotect. Metallogenia 38 (2), 219–229. (in Chinese with English abstract). doi:10.3969/j.issn.1001-1552.2014.02.002
Cheng, Y. Q. (1994). Concise regional geology of China. Beijing: Geological Publishing House, 1–539. (in Chinese).
Clemens, J. D., Holloway, J. R., and White, A. J. R. (1986). Origin of A type granites: Experimental constraints. Am. Mineral. 71, 317–324. (in Chinese with English abstract).
Condie, K. C. (2005). High field strength element ratios in Archean basalt, a window to evolving sources of mantle plumes? Lithos 79 (3-4), 491–504. doi:10.1016/j.lithos.2004.09.014
Dai, F. Y., Chen, B. H., and Liu, F. G. (2008). Characteristics and geological significance of vein rocks in guandimiao area, hunan province. Land Resour. Her. 5 (1), 33–36. (in Chinese with English abstract). doi:10.3969/j.issn.1672-5603.2008.01.013
Deng, J., Wang, C. M., Zi, J. W., Xia, R., and Li, Q. (2017). Constraining subduction-collision processes of the paleo-tethys along the changning-menglian suture: New zircon U-Pb ages and Sr-Nd-Pb-Hf-O isotopes of the Lincang batholith. Gondwana Res. 62, 75–92. doi:10.1016/j.gr.2017.10.008
Deng, P., Ren, J. S., Ling, H. F., Sun, L. Q., Zhu, B., and Tan, Z. Z. (2012). SHRIMP zircon U-Pb ages and tectonic implications for Indosinian granitoids of southern Zhuguangshan granitic composite, South China. Chin. Sci. Bull. 57 (14), 1542–1552. doi:10.1007/s11434-011-4951-8
Depaolo, D. J. (1981). Trace element and isotopic effects of combined wallrock assimilation and fractional crystallization. Earth Planet. Sci. Lett. 53 (2), 189–202. doi:10.1016/0012-821x(81)90153-9
Eby, G. N. (1992). Chemical subdivision of the A-type granitoids, Petrogenetic and tectonic implications. Geol. 20, 641. doi:10.1130/0091-7613(1992)020<0641:csotat>2.3.co;2
Eby, G. N. (1990). The A-type granitoids: A review of their occurrence and chemical characteristics and speculations on their petrogenesis. Lithos 26, 115–134. doi:10.1016/0024-4937(90)90043-z
Evans, D. A. D., and Mitchell, R. N. (2011). Assembly and breakup of the core of Paleoproterozoic-mesoproterozoic supercontinent Nuna. Geology 39 (5), 443–446. doi:10.1130/G31654.1
Geng, Y. S., Kuang, H. W., Du, L. L., Liu, Y. Q., and Zhao, T. P. (2019). On the Paleo-Mesoproterozoic boundary from the breakup event of the Columbia supercontinent. Acta Petrol. Sin. 35 (8), 2299–2324. (in Chinese with English abstract). doi:10.18654/1000-0569/2019.08.0210.18654/1000-0569/2019.08.02
Gibson, G. M., Henson, P. A., Neumann, N. L., Southgate, P. N., and Hutton, L. J. (2012). Paleoproterozoic-earliest Mesoproterozoic basin evolution in the Mount Isa region, northern Australia and implications for reconstructions of the Nuna and Rodinia supercontinents. Episodes 35 (1), 131–141. doi:10.18814/epiiugs/2012/v35i1/012
Gilder, S. A., Coe, R. S., Wu, H., Kuang, G., Zhao, X., Qi, W., et al. (1995). Triassic paleomagnetic data from south China and their bearing on the tectonic evolution of the Western circum-pacific region. Earth Planet. Sci. Lett. 131 (3), 269–287. doi:10.1016/0012-821x(95)00030-g
Gilder, S. A., and Courtillot, V. (1997). Timing of the north-south China collision from new middle to late mesozoic paleomagnetic data from the north China block. J. Geophys. Res. 102 (B8), 17713–17727. doi:10.1029/97JB01201
Gilder, S. A., Gill, J., Coe, R. S., Zhao, X. X., Liu, Z. W., and Wang, G. X. (1996). Isotopic and paleomagnetic constraints on the Mesozoic tectonic evolution of south China. J. Geophys. Res. 101 (B7), 16137–16154. doi:10.1029/96JB00662
Greentree, M. R., Li, Z. X., Li, X. H., and Wu, H. (2006). Late mesoproterozoic to earliest neoproterozoic basin record of the sibao orogenesis in Western south China and relationship to the assembly of rodinia. Precambrian Res. 151 (1), 79–100. doi:10.1016/j.precamres.2006.08.002
Guo, C. L., Zheng, J. H., Lou, F. S., and Zeng, Z. L. (2012). Petrography, genetic, types and geological dynamical setting of the Indosinian granitoids in South China. Geol. Metallogenia 36 (3), 457–472. (In Chinese with English abstract). doi:10.1007/s11783-011-0280-z
Hanan, B. B., Shervais, W. J., and Vetter, S. (2008). Yellowstone plume-continental lithosphere interaction beneath the snake river plain. Geol. 36 (1), 51–54. doi:10.1130/G23935A.1
Hart, S. R. (1984). A large-scale isotope anomaly in the southern hemisphere mantle. Nature 309 (5971), 753–757. doi:10.1038/309753a0
Hervé, F., Pankhurst, R. J., and Yaxley, G. (2007). the South patagonian batholith: 150 my of granite magmatism on a plate margin. Lithos 97 (3-4), 373–394. doi:10.1016/j.lithos.2007.01.007
Hine, R., Williams, I. S., Chappell, B. W., and White, A. J. R. (1978). Contrasts between I- and S-type granitoids of the kosciusko batholith. J. Geol. Soc. Aust. 25 (3-4), 219–234. doi:10.1080/00167617808729029
Hofmann, A.W. (2003). Sampling mantle heterogeneity through oceanic basalts: Isotopes and trace elements. Treatise on Geochem. 2, 1–44. doi:10.1016/B0-08-043751-6/02123-X
Hoffman, P. F. (2003). Continental transform tectonics: Great Slave Lake shear zone (ca. 1.9 Ga), northwest Canada. Geology 15, 7852–7889. doi:10.1130/0091-7613
Hu, G. R. (1998). Study on Geological Geochemistry, Isotopic chronogy and metamorphic P-T-t path of metamorphic rock belt in ccentral Jiangxi. China: Nanjing University. (in Chinese with English abstract).
Huang, X., and Depaolo, D. J. (1989). Study of sources of paleozoic granitoids and the basement of south China by mean of Nd-Sr isotope. Acta Petrol. Sin. 5 (1), 28–36. (in Chinese with English abstract).
Jiang, Y. H., Wang, G. C., Zheng, L., Ni, C. Y., Qing, L., Zhang, Q., et al. (2015). Repeated slab advance-retreat of the Palaeo-Pacific plate underneath SE China. Int. Geol. Rev. 57 (4), 472–491. doi:10.1080/00206814.2015.1017775
Johnson, K. T. M. (1994). Experimental cpx/ and garnet/ melt partitioning of REE and other trace elements at high pressures; petrogenetic implications. Mineral. Mag. 58, 454–455. doi:10.1180/minmag.1994.58A.1.236
King, P. L., White, A. J. R., Chappell, B. W., and Allen, C. M. (1997). Characterization and origin of aluminous A-type granites from the Lachlan Fold Belt, southeastern Australia. J. Petrology 38, 371–391. doi:10.1093/petroj/38.3.371
Li, S. Z., Jahn, B. M., Zhao, S. J., Da, L. M., Li, X. Y., Suo, Y. H., et al. (2017). Triassic southeastward subduction of north China block to south China block: Insights from new geological, geophysical and geochemical data. Earth-Science Rev. 166, 270–285. doi:10.1016/j.earscirev.2017.01.009
Li, X. H., Li, Z. X., Ge, W., Zhou, H., and Wingate, M. T. D. (2003). Neoproterozoic granitoids in South China: Crustal melting above a mantle plume at ca. 825 Ma? Precambrian Res. 122 (1), 45–83. doi:10.1016/s0301-9268(02)00207-3
Li, X. H., Li, Z. X., and Li, W. X. (2014). Detrital zircon U-Pb age and Hf isotope constrains on the generation and reworking of precambrian continental crust in the Cathaysia block, south China: A synthesis. Gondwana Res. 25 (3), 1202–1215. doi:10.1016/j.gr.2014.01.003
Li, X. H., Li, Z. X., Li, W. X., and Wang, Y. J. (2006). Initiation of the indosinian orogeny in south China: Evidence for a permian magmatic arc on hainan island. J. Geol. 114, 341–353. doi:10.1086/501222
Li, X. H., Li, Z. X., Li, W. X., Ying, L., Yuan, C., and Wei, G. (2007). U-Pb zircon, geochemical and Sr-Nd-Hf isotopic constraints on age and origin of jurassic I- and A-type granites from central guangdong, SE China: A major igneous event in response to foundering of a subducted flat-slab? Lithos 96 (1-2), 186–204. doi:10.1016/j.lithos.2006.09.018
Li, X. H., Li, Z. X., Zhou, H. W., Liu, Y., and Kinny, P. D. (2002). U-Pb zircon geochronology, geochemistry and Nd isotopic study of Neoproterozoic bimodal volcanic rocks in the Kangdian rift of South China, implications for the initial rifting of Rodinia. Precambrian Res. 113 (1), 135–154. doi:10.1016/s0301-9268(01)00207-8
Li, X. H. (1990). Magmatic age and crustal movement of the wanyangshan-Zhuguangshan granite complex batholith. Sci. China 7, 747–755. (In Chinese).
Li, X. H., Zhou, H. W., and Liu, Y. (2001). Mesozoic shoshonitic intrusives in the Yangchun basin, Western guangdong, and their tectonic significance, ii. trace elements and Sr-Nd isotopes. Geochimica 30, 57–65. (in Chinese with English abstract). doi:10.3321/j.issn:0379-1726.2001.01.007
Li, Z. X., and Li, X. H. (2007). formation of the 1300 km-wide intra-continental orogen and postorogenic magmatic province in mesozoic south China: A flat-slab subduction model. Geol. 35, 179. doi:10.1130/G23193A.1
Li, Z. X., Li, X. H., Kinny, P. D., and Wang, J. (1999). The breakup of Rodinia; did it start with a mantle plume beneath south China? Earth Planet. Sci. Lett. 173 (3), 171–181. doi:10.1016/S0012-821X(99)00240-X
Liang, X. Q., and Guo, D. L. (2002). Deep tectonic activation and its shellow response in Hunan province. Chin. J. Geol. 3, 332–342. (in Chinese with English abstract). doi:10.3321/j.issn:0379-1726.2001.01.007
Liu, B. J. (1994). Lithofacies paleogeographic atlas of south China. Beijing: Science Press, 1–239. (in Chinese with English abstract).
Liu, B. J., Zhou, M. K., and Wang, R. Z. (1990). Early palaeozoic palaeogeography and tectonic evolution of south China. Acta Geosci. Sin. 1, 97–98. (In Chinese with English abstract).
Loiselle, M. C., and Wones, D. R. (1979). Characteristics and origin of anorogenic granites. Geol. Soc. Am. Prog. Abstr. 12 (7), 468.
Ludwig, K. R. (2003). Isoplot/ex, a geochronological toolkit for microsoft excel, version 3.00. United States: Berkeley Geochronology Center.
Ma, D. H., Li, Z. C., and Xiao, Z. F. (1997). A Study on the boundary age between Jurassic and Cretaceous. Acta Geoscientia Sinica 3, 233–241. (in Chinese with English abstract).
Ma, X. X., Dong, C. W., Tang, L. M., Lv, Q., and Gu, H. Y. (2013). Petrological evidence of mesozoic tectonic extension in the coastal area of SE China, the spatial-temporal distribution and geochemical constraints on the mafic rocks from Hainan, Guangdong, Fujian and Zhejiang Provinces, South China. J. Zhejiang Univ. Sci. Ed. 40 (6), 683–692. doi:10.3785/j.issn.1008-9497.2013.06.015
Mao, J. R., Li, Z. L., and Ye, H. M. (2014). Mesozoic tectono-magmatic activities in South China: Retrospect and prospect. Sci. China Earth Sci. 44 (12), 2853–2877. doi:10.1007/s11430-014-5006-1
Mao, J. W., Chen, C. H., Yuan, S. D., and Guo, C. L. (2011). Characteristics and spatial-temporal regularity of mineral deposits in qinhang (or shihang) metallogenic belt, south China. Acta Geol. Sin. 85 (5), 636–658. (in Chinese with English abstract).
Mao, J. W., Xie, G. Q., Li, X. F., Zhang, C. Q., and Mei, Y. X. (2004). Meosozoic large scale mineralization and multiple lithospheric extension in South China. Earth Sci. Front. 11 (1), 45–55. China University of Geosciences, Beijing (in Chinese with English abstract). doi:10.3321/j.issn:1005-2321.2004.01.003
Mao, J. W., Xie, G. Q., Li, X. F., Zhang, Z. H., Wang, Y. T., and Wang, Z. L. (2005). Geodynamic process and metallogeny, history and present research trend, with a special discussion on continental accretion and related metallogeny throughout geological history in South China. Mineral. Deposits 24 (3), 193–205. (in Chinese with English abstract). doi:10.3969/j.issn.0258-7106.2005.03.001
Mckenzie, D., and Keith, O. R. (1995). The Source Regions of Ocean Island Basalts. J. Petrol. 1, 133–159. doi:10.1093/petrology/36.1.133
Meng, L. F., Li, Z. X., Chen, H., Li, X. H., and Wang, X. C. (2012). Geochronological and geochemical results from mesozoic basalts in southern south China block support the flat-slab subduction model. Lithos 132 (132-133), 127–140. doi:10.1016/j.lithos.2011.11.022
Miao, B. H. (2014). Geologic characteristic and genesis mechanism of the qingshuitang Pb-Zn deposit in Qidong county, hunan province. China: Nanjing University, 1–80. (in Chinese with English abstract).
Nasdala, L., Hofmeister, W., Norberg, N., Martinson, J. M., Corfu, F., Dörr, W., et al. (2008). Zircon M257–A homogeneous natural reference material for the ion microprobe U-Pb analysis of Zircon. Geostand. Geoanal. Res. 32 (3), 247–265. doi:10.1111/j.1751-908X.2008.00914.x
Pape, F. L., Jones, A. G., Vozar, J., and Wei, W. (2012). Penetration of crustal melt beyond the kunlun fault into northern tibet. Nat. Geosci. 5 (5), 330–335. doi:10.1038/NGEO1449
Patio Dounce, A. E., Humphreys, E. D., and Johnston, A. D. (1990). Anatexis and metamorphism in tectonically thickened continental crust exemplified by the Sevier hinterland, Western North America. Earth Planet. Sci. Lett. 97, 290–315. doi:10.1016/0012-821X(90)90048-3
Pearce, J. (1996). Sources and settings of granitic rocks. Episodes 19 (4), 120–125. doi:10.1002/(SICI)1096-9837(199612)21:12<1163::AID-ESP666>3.3.CO
Qin, J. H., Wang, D. H., and Chen, Y. C. (2022). Geochemical and geochronological constraints on a granitoid containing the largest indosinian tungsten (W) deposit in south China (SC): Petrogenesis and implications. Minerals 12 (1), 80. doi:10.3390/min12010080
Qin, J. H., Wang, D. H., and Chen, Y. C. (2020). Geochemical and Sr-Nd-Pb isotopic characteristics of basalt from eastern Hunan Province: New insight for the Late Cretaceous tectonic dynamic mechanism in South China. Geol. J. 55 (12), 8141–8168. doi:10.1002/gj.3914
Rogers, J. J. W., and Santosh, M. (2002). Configuration of Columbia, a mesoproterozoic super-continent. Gondwana Res. 5 (1), 5–22. doi:10.1016/S1342-937X(05)70883-2
Rowley, D. B., Jiayu, R., Jin, Z., Yuan-Dong, Z., and Ren-Bing, Z. (1997). Late precambrian through early paleozoic stratigraphic and tectonic evolution of the nanling region, Hunan Province, south China. Int. Geol. Rev. 39 (5), 469–478. doi:10.1080/00206819709465285
Rubatto, D. (2002). Zircon trace element geochemistry, Partitioning with garnet and the link between U-Pb ages and metamorphism. Chem. Geol. 84, 123–138. doi:10.1016/S0009-2541(01)00355-2
Shen, W. Z., Chen, F. R., Liu, C. S., and Wang, D. Z. (1992). Isotopic-geochemical characteristics and source material for two types of volcanic-intrusive complexes in Jiangxi Province. Acta Petrol. Sin. 8 (2), 177–184. (in Chinese with English abstract).
Shen, W. Z., Zhu, J. C., Liu, C. S., Xu, S. J., and Ling, H. F. (1993). Sm-Nd isotopic study of basement metamorphic rocks in south China and its constraint on material sources of granitoids. Acta Petrol. Sin. 9, 115–124. (in Chinese with English abstract).
Shu, L. S. (2012). An analysis of principal features of tectonic evolution in South China Block. Geol. Bull. China 31 (7), 1035–1053. (in Chinese with English abstract). doi:10.1111/j.1755-6724.2008.tb00581.x
Shu, L. S., Chen, X. Y., and Lou, F. S. (2019). Pre-jurassic tectonics of the south China. Acta Geol. Sin. 94 (2), 333–360. doi:10.1111/j.1755-6724.2008.tb00581.x
Shu, L. S., Zhou, X. M., Deng, P., and Yu, X. Q. (2006). Principal geological features of nanling tectonic belt, south China. Geol. Rev. 52 (2), 251–265. (in Chinese with English abstract). doi:10.3969/j.issn.1671-2552.2006.03.002
Shu, L. S., and Zhou, X. M. (2002). Late mesozoic tectonism of southeast China. Geol. Rev. 48 (3), 249–260. (in Chinese with English abstract). doi:10.3321/j.issn:0371-5736.2002.03.004
Shu, L. S. (2012). An analysis of pricipal features of tectonic evolution in South China Block. Geol. Bullet. China 31 (7), 1035–1053. (in Chinese with English abstract). doi:10.3969/j.issn.1671-2552.2012.07.003
Shu, L. S., Chen, X. Y., and Lou, F. S. (2020). Pre-Jurassic tectonics of the SouthChian. Acta Geologica Sinica 94 (2), 333–360.
Sláma, J., Košler, J., Condon, D. J., Crowley, J. L., Gerdes, A., Hanchar, J. M., et al. (2008). Plešovice zircon-a new natural reference material for U-Pb and Hf isotopic microanalysis. Chem. Geol. 249, 1–35. doi:10.1016/j.chemgeo.2007.11.005
Sparks, R. S. J., and Marshall, L. A. (1986). Thermal and mechanical constraints on mixing between mafic and silicic magmas. J. Volcanol. Geotherm. Res. 29 (1), 99–124. doi:10.1016/0377-0273(86)90041-7
Sun, S. S. (1980). Lead isotopic study of young volcanic rocks from midocean ridges, ocean islands and island arcs, Philos. Trans. R. Soc. Lond. 297, 409–445. doi:10.1098/rsta.1980.0224
Sun, S. S., and McDonough, W. F. (1989). Chemical and systematics of oceanic basalts, Implication for mantle 682 composition and processes, 42. London: Geological Society of London. Special Publication, 313–345. doi:10.1144/GSL.SP.1989.042.01.19
Sun, T. (2006). A new map showing the distribution of granites in South China and its egplauatory notes. Geol. Bull. China 25 (3), 332–337. (in Chinese with English abstract). doi:10.3969/j.issn.1671-2552.2006.03.002
Sylvester, P. J. (1998). Post-collisional strongly peraluminous granites. Lithos 45, 29–44. doi:10.1016/S0024-4937(98)00024-3
Tapponnier, P., Peltzer, G., Le Dain, A. Y., Armijo, R., and Cobbold, P. (1982). Propagating extrusion tectonics in Asia, new insights from simple experiments with plasticine. Geol. 10 (12), 611. doi:10.1130/0091-7613(1982)10<611:petian>2.0.co;2;2
Vernon, R. H. (1984). Microgranitoid enclaves in granites-globules of hybrid magma quenched in a plutonic environment. Nature 309 (5967), 438–439. doi:10.1038/309438a0
Wang, Y. J., Fan, W. M., Liang, X. Q., Peng, T. P., and Shi, Y. R. (2005). Zircon SHRIMP U-Pb age and genetic revelation of Indosinian granites in Hunan. Chinese Science Bulletin 50 (12), 1259–1266. (in Chinese with English abstract). doi:10.3321/j.issn:0023-074X.2005.12.018
Wang, D. H., Chen, Z. H., Chen, Y. C., Tang, J. X., Li, J. K., Ying, L. J., et al. (2010). New data of the rock forming and ore-forming chronology for China's important mineral resources areas. Acta Geol. Sin. 84 (7), 1030–1040. (in Chinese with English abstract).
Wang, D. H., Chen, Z. Y., Huang, F., Wang, C. H., Zhao, Z., Chen, Z. H., et al. (2014). Discussion on metallogenic specialization of the magmatic rocks and related issues in the Nanling Region. Geotect. Metallogenia 38 (2), 230–238. (in Chinese with English abstract).
Wang, X. L., Zhou, J. C., GriIIin, W. I., Zhao, G. C., Yu, J. H., Qiu, J. S., et al. (2014). Geochemical zonation across a Neoproterozoic orogenic belt isotopic evidence from granitoids and metasedimentary rocks of the Jiangnan orogen, China. Precambrian Res. 242, 154–171. doi:10.1016/j.precamres.2013.12.023
Wang, D. H., Li, P. G., Qu, W. J., Lei, Z. Y, Liao, Y. C., et al. (2013). Discovery and preliminary study of the high tungsten and lithium contents in the Dazhuyuan bauxite deposit, Guizhou, China. Sci. China Earth Sci. 56, 145–152. doi:10.1007/s11430-012-4504-2
Wang, X. L., Zhou, J. C., C'hen, X., Zhang, F. F., and Sun, Z. M. (2017). Formation and evolution of the jiangnan orogen. Bull. Mineralogy, Petrology Geochem. 360, 714–735. (in Chinese with English abstract). doi:10.3969/j.issn.1007-2802.2017.05.003
Wang, Y., Guan, T. Y., Liu, P. H., Yu, D. J., and Wu, J. H. (2002). Nd and Sr isopotic geochemical characteristics for Early Cretaceous volcanic rocks in Northeast Jiangxi Province. J. Mineralogy Petrology 22 (1), 36–40. (in Chinese with English abstract). doi:10.1080/12265080208422884
Wang, Y. J., Fan, W. M., Cawood, P. A., and Li, S. Z. (2008). Sr–Nd–Pb isotopic constraints on multiple mantle domains for Mesozoic mafic rocks beneath the South China Block hinterland. Lithos 106, 297–308. doi:10.1016/j.lithos.2008.07.019
Wang, Y. J., Fan, W. M., Guo, F., Peng, T. P., and Li, C. W. (2003). Geochemistry of Mesozoic mafic rocks adjacent to the Chenzhou-Linwu Fault, South China, implications for the lithospheric boundary between the Yangtze and Cathaysia blocks. Int. Geol. Rev. 45, 263–286. doi:10.2747/0020-6814.45.3.263
Wang, Y. J., Fan, W. M., Liang, X. Q., Peng, T. P., and Shi, Y. R. (2005a). Zircon SHRIMP U-Pb age and genetic revelation of Indosinian granites in Hunan. Chin. Sci. Bull. 50 (12), 1259–1266. (in Chinese with English abstract). doi:10.3321/j.issn:0023-074X.2005.12.018
Wang, Y. J., Fan, W. M., Peng, T. P., and Guo, F. (2005b). Elemental and Sr-Nd isotopic systematics of the early mesozoic volcanic sequence in southern jiangxi province, south China, petrogenesis and tectonic implications. Int. J. Earth Sci. 94 (1), 53–65. doi:10.1007/s00531-004-0441-4
Weaver, B. L. (1991). The origin of ocean Island basalt end-member compositions, trace element and isotopic constraints. Earth Planet. Sci. Lett. 104 (2-4), 381–397. doi:10.1016/0012-821X(91)90217-6
Whalen, J. B., Kenneth, L. C., and Bruce W, C. (1987). A-type granites, geochemical characteristics, discrimination and petrogenesis. Contrib. Mineral. Pet.. 95, 407–419. doi:10.1007/bf00402202
Whalen, J. B., Wodicka, N., Taylor, B. E., and Jackson, G. D. (2010). Cumberland batholith, trans-hudson orogen, Canada: Petrogenesis and implications for paleoproterozoic crustal and orogenic processes. Lithos 117 (1-4), 99–118. doi:10.1016/j.lithos.2010.02.008
Wu, Y. B., and Zhen, Y. F. (2004). Study on zirconium gallstone by mineralogy and its restriction on U-Pb age explanation. Chin. Sci. Bull. 49, 1589–1604. (in Chinese with English abstract).
Xia, W. C. (1991). Genetic stratigraphic framwork and its genetic relationship to oil and gas in Mesozoic and Cenozoic fault-controlled basin of Eastern China. Geol. Sci. Technol. Inf. 10 (1), 43–50. (in Chinese with English abstract).
Yang, F., Santosh, M., and Kim, S. W. (2018). Mesozoic magmatism in the eastern North China Craton: Insights on tectonic cycles associated with progressive craton destruction. Gondwana Res. 60, 153–178. doi:10.1016/j.gr.2018.04.003
Yang, F., Santosh, M., Kim, S. W., Zhou, H., and Jeong, Y. J. (2020). Late mesozoic intraplate rhyolitic volcanism in the north China craton: Far-field effect of the westward subduction of the paleo-Pacific plate. GSA Bull. 132 (1-2), 291–309. doi:10.1130/B35123.1
Yang, F., Xue, F., Santosh, M., Wang, G., Kim, S. W., Shen, Z., et al. (2019). Late mesozoic magmatism in the east qinling orogen, China and its tectonic implications. Geosci. Front. 10 (5), 1803–1821. doi:10.1016/j.gsf.2019.03.003
Yang, Z. (2012). Pre-yanshanian magmatism and its mineralization in the miaoershan-yuechengling area, northern guangxi province. China: Nanjing University, 1–64. (in Chinese with English abstract).
Yang, F., Santosh, M., and Kim, S. W. (2018). Mesozoic magmatism in the eastern North China Craton: Insights on tectonic cycles associated with progressive craton destruction. Gondwana Research 60, 153–178. doi:10.1016/j.gr.2018.04.003
Ye, M. F., Li, X. H., Li, W. X., Liu, Y., and Li, Z. X. (2007). SHRIMP zircon U-Pb geochronological and whole-rock geochemical evidence for an early Neoproterozoic Sibaoan magmatic arc along the southeastern margin of the Yangtze Block. Gondwana Res. 12 (1–2), 144–156. doi:10.1016/j.gr.2006.09.001
Yin, C. Q., Lin, S. F., Davis, D. W., Zhao, G. C., Xiao, W. J., Li, L. M., et al. (2013). 2.1–1.85Ga tectonic events in the Yangtze Block, South China: Petrological and geochronological evidence from the Kongling Complex and implications for the reconstruction of supercontinent Columbia. Lithos 183, 200–210. doi:10.1016/j.lithos.2013.10.012
Yuan, Z. X., and Zhang, Z. Q. (1992). Sm-Nd isotopic of granitoids in the nanling region and their petro-genetic analysis. Geol. Rev. 38 (1), 1–15. doi:10.16509/j.georeview.1992.01.001
Zartman, R. Z., and Doe, B. R. (1981). Plumbotectonics-themodel. Tectonophysics 75, 135–162. doi:10.1016/0040-1951(81)90213-4
Zhang, H. F., Sun, M., Zhou, X. H., Fan, W. M., Zhai, M. G., Yin, J. F., et al. (2002). Mesozoic lithosphere destruction beneath the north China craton, evidence from major-trace-element and Sr-Nd-Pb isotope studies of fangcheng basalts. Contrib. Mineral. Pet.. 144 (2), 241–254. doi:10.1007/s00410-002-0395-0
Zhang, L., Chen, Z. Y., Wang, F. Y., and Zhou, T. F. (2020). Apatite geochemistry as an indicator of petrogenesis and uranium fertility of granites: A case study from the zhuguangshan batholith, south China. Ore Geol. Rev. 128, 103886. doi:10.1016/j.oregeorev.2020.103886
Zhang, Y. X. (1984). Ring structiure and their genetic mechanism in the South China region. Geol. Rev. 30 (5), 437–445. (in Chinese with English abstract).
Zhang, S. B., Zheng, Y. F., Wu, Y. B., Zhao, Z. F., Gao, S., and Wu, F. Y. (2006). Zircon isotope evidence for ≥3.5Ga continental crust in the Yangtze craton of China. Precambrian Research 146 (1–2), 16–34. doi:10.1016/j.precamres.2006.01.002
Zhang, S. H., Zheng, Y. F., He, Z. F., and Wu, H. (2009). The 1.35Ga diabase sills from the northern North China Craton: Implications for breakup of the Columbia (Nuna) supercontinent. Earth and Planetary Science Letters 288 (3-4), 588–600. doi:10.1016/j.epsl.2009.10.023
Zhao, J. H., and Zhou, M. F. (2008). Neoproterozoic adakitic plutons in the northern margin of the Yangtze block, China, partial melting of a thickened lower crust and implications for secular crustal evolution. Lithos 104 (1-4), 231–248. doi:10.1016/j.lithos.2007.12.009
Zhao, K. D., Jiang, S. Y., Yang, S. Y., Dai, B. Z., and Lu, J. J. (2012). Mineral chemistry, trace elements and Sr–Nd–Hf isotope geochemistry and petrogenesis of Cailing and Furong granites and mafic enclaves from the Qitianling batholith in the Shi-Hang zone, South China. Gondwana Res. 22 (1), 310–324. doi:10.1016/j.gr.2011.09.010
Zhao, T. P., Zhou, M, F., Zhai, M. G., and Xia, B. (2002). Paleoproterozoic rift-related volcanism of the xiong'er group, north China craton: Implications for the breakup of Columbia. Int. Geol. Rev. 44 (4), 336–351. doi:10.2747/0020-6814.44.4.336
Zhao, Z. H., Bao, Z. H., and Zhang, B. Y. (1998). Geochemistry of the mesozoic basaltic rocks in southern Hunan province. Sci. China Ser. D-Earth. Sci. 41 (1), 102–112. doi:10.1007/BF02875640
Zhao, Z., Miao, B., Xu, Z., Lu, J., Lei, L., Zuo, C., et al. (2017). Petrogenesis of two types of late triassic granite from the guandimiao complex, southern hunan province, China. Lithos 282-283, 403–419. doi:10.1016/j.lithos.2017.02.021
Zhao, Z., Wang, D. H., Li, P. G., and Lei, Z. Y. (2013). Detrital zircon U-Pb geochronology of the Dazhuyuan formation in Northern Uuizhou; implications for bauxite mineralization. Rock Mineral analysis 32 (1), 166–173. (in Chinese with English abstract). doi:10.3969/j.issn.0254-5357.2013.01.027
Zhao, Z. X., Xu, Z. W., Miao, B. H., Zuo, C. H., Lu, J. J., Lu, R., et al. (2015). Diagenetic age and material source of the guandimiao granitic batholith, hengyang city, hunan province. Acta Geol. Sin. 89 (7), 1219–1230. (in Chinese with English abstract). doi:10.3969/j.issn.0001-5717.2015.07.006
Zheng, J. P., Griffin, W. L., Tang, H. Y., Zhang, Z. H., Su, Y. P., and Liu, G. P. (2008). Archean basement similar to the north China and Yangtze continents may Be existed beneath the western Cathaysia. Geol. J. China Univ. 4, 549–557. (in Chinese with English abstract). doi:10.3969/j.issn.1006-7493.2008.04.008
Zhong, Y. F., Ma, C. Q., Yu, Z. B., Lin, G. C., Xu, H. J., Wang, R. J., et al. (2005). SHRIMP U-Pb zircon geochronology of the jiuling granitic complex batholith in jiangxi province. Earth Science-Journal China Univ. Geosciences 30 (6), 685–681. (in Chinese with English abstract). doi:10.3321/j.issn:1000-2383.2005.06.005
Zhou, J. L., Li, X. H., Tang, U. Q., Uao, B. Y., Bao, Z. A., Ling, X. X., et al. (2018). Ca. 890 Ma magmatism in the northwest Yangtze block, south China: SIMS U-Pb dating, in-situ Hf-O isotopes, and tectonic implications. J. Asian Earth Sci. 151, 101–111. doi:10.1016/j.jseaes.2017.10.029
Zhou, X. M. (2003). My thinking about granite genesis of south China. Geol. J. China Univ. 9 (4), 556–565. (in Chinese with English abstract). doi:10.3321/j.issn:1000-2383.2005.06.005
Zhou, X. M., Sun, T., Shen, W. Z., Shu, L. S., and Niu, Y. L. (2006). Petrogenesis of Mesozoic granitoids and volcanic rocks in South China, a response to tectonic evolution. Episodes 29, 26–33. doi:10.18814/epiiugs/2006/v29i1/004
Keywords: Guandimiao batholith, granitoids, Lamprophyre, petrogenesis, tectonic evolution
Citation: Qin J-H, Huang F and Wang D-H (2022) Batholith recorded mesozoic multistage tectonic evolution of the South china block: A case study of the guandimiao intrusions. Front. Earth Sci. 10:948723. doi: 10.3389/feart.2022.948723
Received: 20 May 2022; Accepted: 30 June 2022;
Published: 09 August 2022.
Edited by:
Fan Yang, Lanzhou University, ChinaReviewed by:
Juquan Zhang, Hebei GEO University, ChinaAbulimiti Aibai, Xinjiang Institute of Ecology and Geography (CAS), China
Hua-Wen Cao, China Geological Survey, China
Copyright © 2022 Qin, Huang and Wang. This is an open-access article distributed under the terms of the Creative Commons Attribution License (CC BY). The use, distribution or reproduction in other forums is permitted, provided the original author(s) and the copyright owner(s) are credited and that the original publication in this journal is cited, in accordance with accepted academic practice. No use, distribution or reproduction is permitted which does not comply with these terms.
*Correspondence: Fan Huang, aGZoeW1uQDE2My5jb20=