- 1College of Chemical Engineering, Zhejiang University of Technology, Hangzhou, China
- 2School of Geoscience and Technology, Southwest Petroleum University, Chengdu, China
- 3Key Laboratory of Tectonic Controls on Mineralization and Hydrocarbon Accumulation of Ministry of Natural Resources, Chengdu, China
U–Pb ages of 637 new detrital zircons of Triassic sandstones from the Northwestern Sichuan Basin (NWSB), together with the petrology and paleocurrent data, are used to constrain the sediment provenance and tectonic–paleogeographic evolution of the western Yangtze Block. The U–Pb age data for the Lower Triassic detrital zircons generally show populations at ∼1,850 Ma, 980–705 Ma, 680–510 Ma, and 290–230 Ma with a minor cluster at ∼2.4 Ga. Such age spectrum features together with the eastward to northeastward paleocurrent direction implied that the Northwestern Sichuan Basin was dominantly fed by the uplifted Khamdian Paleoland. The Upper Triassic detrital zircons yield age populations at 855–730 Ma, 455–415 Ma, and 290–215 Ma and a prominent age group of 1.9–1.7 Ga. These age spectrum features together with paleocurrent data showed a provenance change with a major source of the Longmenshan thrust belt and Songpan-Ganzi terrane for the south area of NWSB and the Qinling orogenic belt for the north part of NWSB. Such transition of the provenance probably indicated the subsidence of the Khamdian Paleoland and uplift of the Songpan-Ganzi terrane and Qinling orogenic belt from the Early Triassic to the Late Triassic. This tectonic inversion most likely resulted from the gradually thermal subsidence of the Emeishan large igneous province (ELIP) and the gravitational loading triggered by the eastward shortening of the Songpan-Ganzi terrane as well as the ongoing convergence between Yangtze Block and North China Block.
1 Introduction
Clastic sedimentary rocks of basin bear valuable information about their sources and the basin evolution history, as well as the evolution and erosion history of the peripheral orogens (e.g., Wilson et al., 1968; Taylor and McLennan, 1985; Tuener et al., 1993). Detrital zircons in sedimentary rocks have proved to be a most effective medium to record such information due to their resistance to subsequent mechanical and chemical processes, such as erosion, transportation, metamorphism, and partial melting (Gehrels et al., 1998; Lee et al., 1997; Rubatto and Gebauer, 2000; Amidon et al., 2005; Kemp et al., 2006; Hawkesworth and Kemp, 2006; Hawkesworth et al., 2010). Hence, by analyzing the U–Pb age spectrum of detrital zircons from clastic rocks, we can trace the source characteristics and even their transport processes thus to reconstruct the tectonic evolution of basin and peripheral orogens (Wang et al., 2013; Peter-Jon et al., 2015; Zhu et al., 2016).
The Sichuan Basin is located at the Upper Yangtze Craton which is bounded by the Qinling orogen to the north, the Sanjiang orogenic belt to the southwest, and the Jiangnan-Xuefeng orogen to the southeast (Figure 1). The Sichuan Basin had experienced complex tectonic evolution from the Late Paleozoic to the Early Mesozoic (Zhu et al. (2017) and references therein), including the collision of the South China Block and the North China Block at the north margin, the tectono-magmatic thermal event of the Emeishan large igneous province (ELIP), and the closure of Paleo-Tethys Ocean that resulted in the formation of Sanjiang orogenic belt along the west margin. The Northwestern Sichuan Basin (NWSB) may be the ideal place to record the aforementioned tectonic activities, which resulted in the transformation of passive continental basin to peripheral foreland basin during the Early Mesozoic with thick clastic sediments (Yin and Nie, 1993; Chen and Wilson, 1996.; Guo et al., 1996; Lin et al., 2006; Yuan et al., 2010a; Luo et al., 2014).
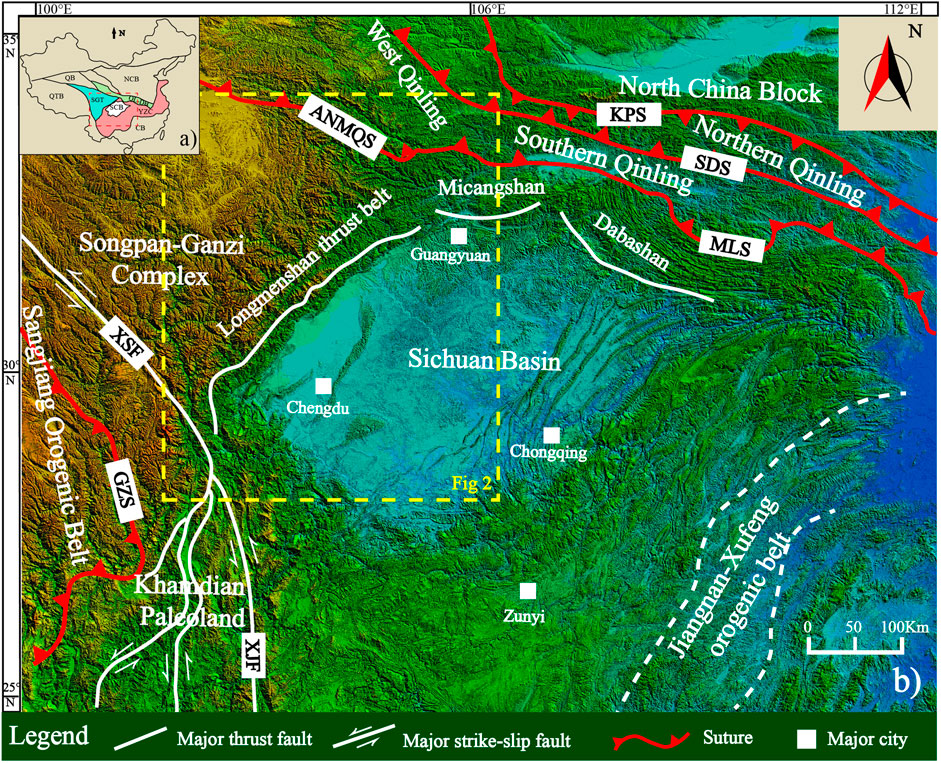
FIGURE 1. Geological sketch map showing the tectonic of the South China Block (A) and Upper Yangtze area (B) (modified from M. Faure et al., 2017); ANMQ, Animaqin suture; MLS, Mianlue suture; SDS, Shangdan suture; KPS, Kuanping suture;XSF, Xianshuihe fault; XJF, Xiaojiang fault; GZS, Ganzi-Litang suture.
Previous studies mainly focused on the Late Triassic detrital records to reveal the information of potential sources and tectonic processes of the NWSB during the Mesozoic era due to the lack of medium to coarse clastic rocks from the Early to the Middle Triassic. The results showed that the Sichuan Basin had a remarkably high correlative provenance with its adjacent orogens during the Late Triassic (Deng et al., 2008). However, with relatively rare research studies about provenance of Lower Triassic, some controversy is still going on concerning the depositional responses of the major orogeny and the time–spatial characteristics of the basin that received sedimentary material from the orogens during the Early Triassic (Zhao and Coe, 1987; Cui et al., 1991; Guo et al., 1996; Li et al., 2005; Luo et al., 2014; Zhang et al., 2015; Shao et al., 2016). Based on traditional sedimentology analyses, studies showed that the uplift of the Khamdian Paleoland controlled the evolution of the Early Triassic southwestern Sichuan (Ma et al., 2009). The first reported U–Pb geochronology and geochemistry of Early Triassic sedimentary rocks in Yanyuan (Zhu, 2010) and northern Yunnan (Zhang et al., 2016) of Southwestern Yangtze Block indicated that these regions mainly received materials from the Khamdian Paleoland rather than the exterior of the western Sanjiang orogen of the Yangtze Block during the Early Triassic. The subsequent study of U–Pb geochronology and geochemical characteristics of sandstone from the E’mei–Meigu region also suggested that the Early Triassic strata in the southwestern Sichuan probably originated from both the ELIP and the Khamdian Paleoland of the inner Yangtze Craton ( Zhu et al., 2017). However, the newly reported U–Pb geochronology of the Early Triassic sedimentary succession from the northern Sichuan Basin to South Qinling Orogen showed that the foreland basin of the northeastern Paleo-Tethys Ocean received recycled North Qinling Orogen detritus in a collisional tectonic setting (Zhang et al., 2022).
In this paper, seven sandstone samples from the Upper Triassic and three from the Lower Triassic were collected from the NWSB for sandstone petrology and detrital zircon geochronology analysis (Figures 2, 3, 4). Combined with other published paleogeography and basin analysis, this study not only traced the possible provenance information (Bruguier et al., 1997; Federico et al., 2004; Veevers et al., 2005) but also reconstructed the possible tectonic processes about the transition from the passive continental basin to the foreland basin during the Early Mesozoic.
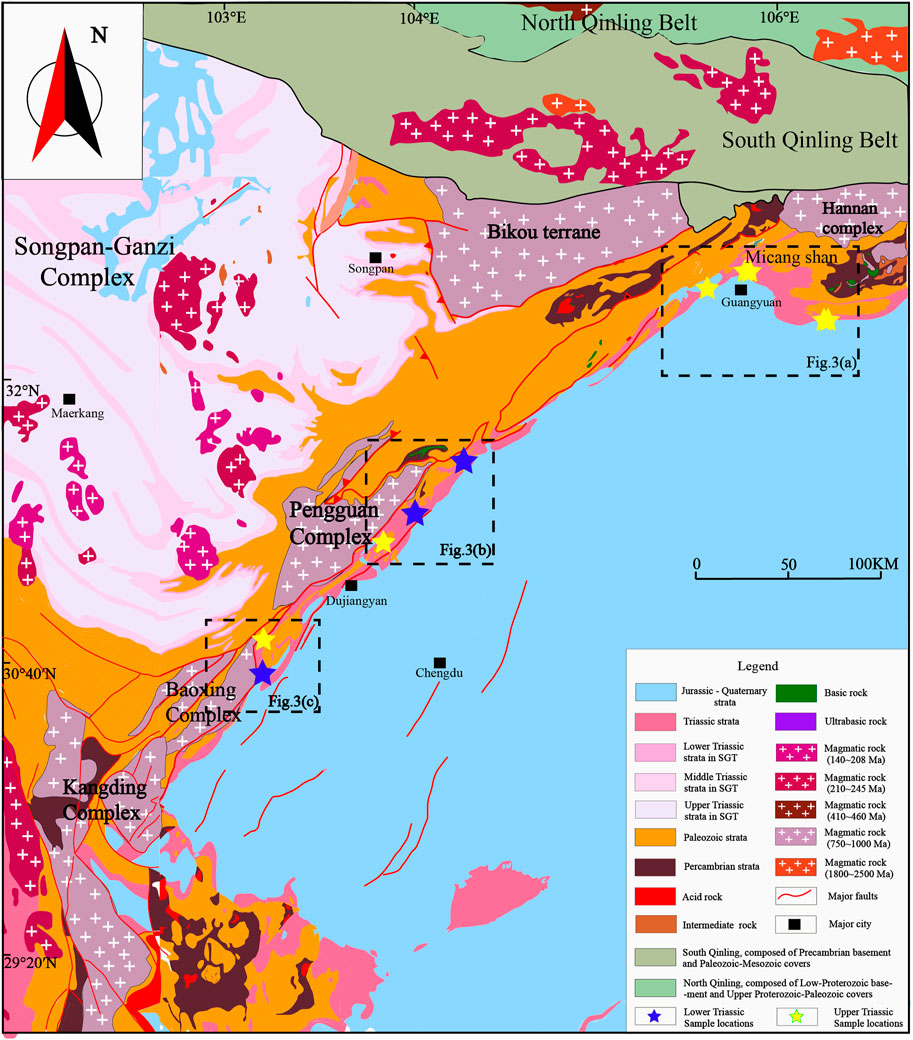
FIGURE 2. Geologic and peripheral igneous rocks’ sketch map of the Northwestern Sichuan Basin modified after geological map of Sichuan Province (Zhang et al., 2015) and Yu and Liang (2016) showing the peripheral igneous rocks, major faults, and locations of our samples. Abbreviations: PG, Pengguan massif; BX, Baoxing massif; KD, Kangding massif; BK, Bikou terrane; HN, Hannan massif. Specific geologic sketch map and sample locations in our study are shown in Figure 3.
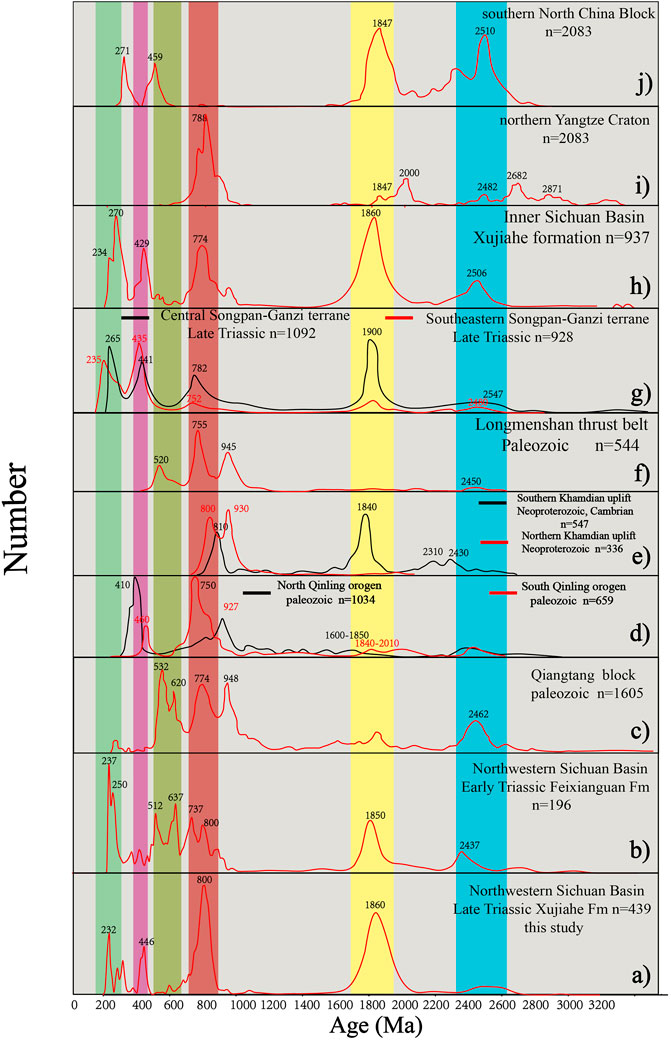
FIGURE 3. Relative probability of detrital zircon U–Pb ages for comparison with adjacent potential source areas. (A) Late Triassic sandstones and (B) Early Triassic sandstones in the Northwestern Sichuan Basin; (C) Paleozoic strata of Qiangtang Block (Gehrels et al., 2011); (D) red line: Paleozoic strata of South Qinling belt (Zhu et al., 2017; Shi et al., 2013); black line: Paleozoic strata of North Qinling belt (Shi et al., 2013; Zhu et al., 2011); (E) red line: Neoproterozoic sedimentary strata (Zhou et al., 2006; Sun et al., 2009); black line: Neoproterozoic and Cambrian sedimentary strata (Sun et al., 2009); (F) Paleozoic strata of Longmenshan thrust belt (Chen et al., 2011; Weislogel et al., 2010); (G) Late Triassic strata of Songpan-Ganzi terrane (Weislogel et al., 2010); (H) inner Sichuan foreland basin (Luo et al., 2014; Li et al., 2016; Deng et al., 2008); (I) north Yangtze Craton (Shi et al., 2013; Shao et al., 2016); (J) Permian–Middle Triassic strata in the southern North China Block (Shi et al., 2013; Zhang et al., 2015).
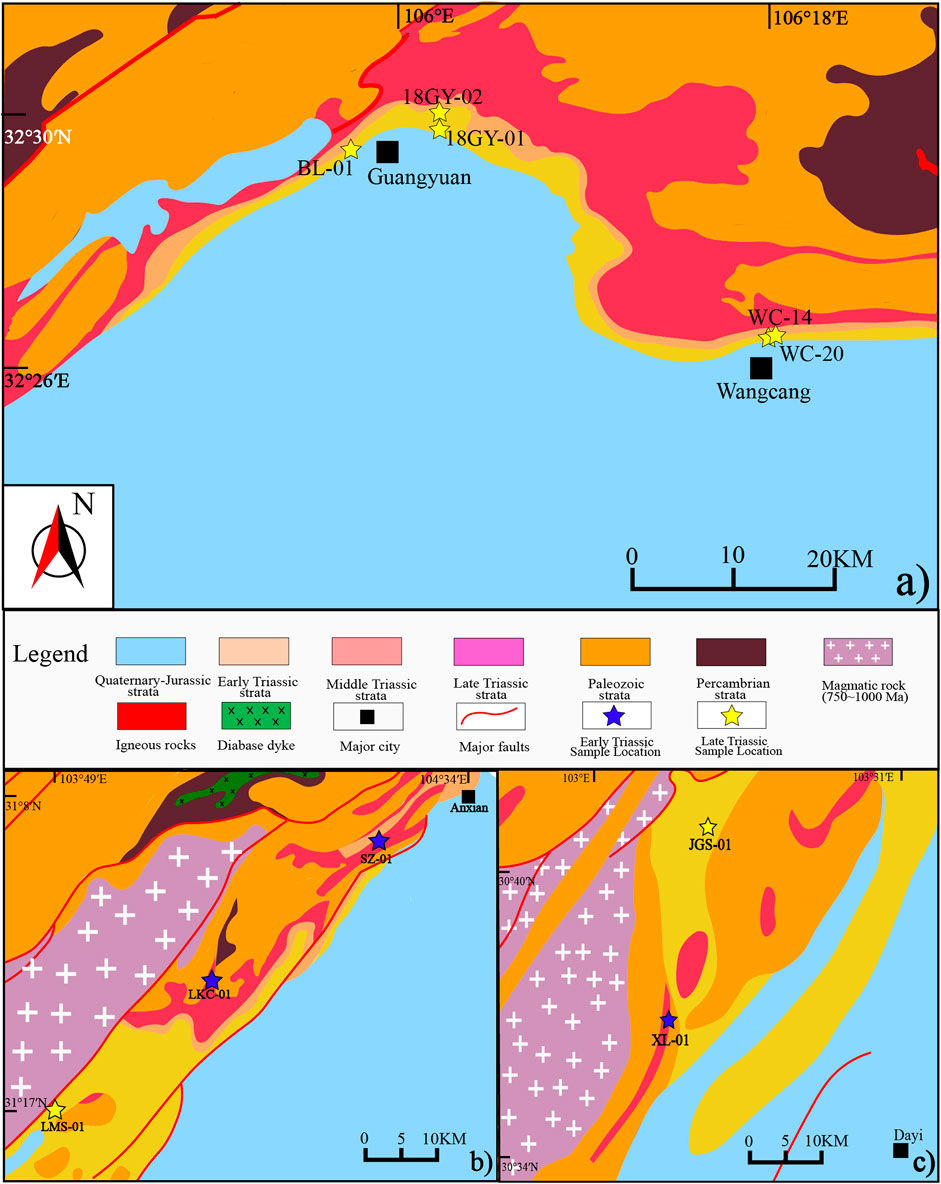
FIGURE 4. Specific geologic sketch map and sample locations of our study: Guangyuan (A), Qionglai (B), and Mianyang (C) (modified after the geological map of Sichuan Province).
2 Geological Settings
The Sichuan Basin is an NE–SW trending structural depression that occupies the northwest portion of Yangtze Craton. The NWSB, located at the northwestern part of the basin, is bounded by the Qinling orogen to the north, separated by the Micangshan–Dabashan structural belts, Songpan-Ganzi complex to the west divided by the Longmenshan thrust belt, Sanjiang orogen, and Khamdian Paleoland to the southwest by the Xianshuihe fault zone, and Central Sichuan uplift transitional zone to the east (Figure 1). As the NWSB turned into a foreland basin since the Late Triassic, it is an ideal target to study the depositional response of such transition as well as the relationship with the adjacent orogens since the Mesozoic era.
The convergence of the South China Block (SCB), the North China Block (NCB), and the Qiangtang Block (QTB) during the Mesozoic era has profoundly changed the Sichuan Basin (Pan et al., 2001; Shao et al., 2016). The diachronous collision between the SCB and the NCB led to the formation of the Qinling orogenic belt, which can be subdivided into the north margin of the South China Block, southern Qinling belt, northern Qinling belt, and south margin of the North China Block by Mianlue suture, Shangdan suture, and Kuanping complex, respectively (Zhang et al., 2001). This orogeny was accompanied by massive folding and intrusion of granitoids as well as thick Mesozoic sediments in the north of Sichuan Basin. This event also resulted in the transformation of the northern Sichuan Basin from shallow marine during the Late Permian to transitional environment during the Early and Middle Triassic, and finally got into the continental basin during the Late Triassic (Xu et al., 2013; Zhang et al., 2014; Yu and Liang, 2016). The convergence of the NCB and the QTB also formed the Songpan-Ganzi turbidite complex which expanded to the more rigid Yangtze Block (i.e., the western SCB) on the east and resulted in the development of the Longmenshan belt (Xu et al., 2013; Zhu et al., 2016; Yu and Liang, 2016). This tectonic activity also caused the transition from a passive continental marginal type basin into a foreland basin of the western Sichuan Basin (Zhao and Coe et al., 1987; Zhang et al., 2001; Yuan et al., 2010b).
The triangular-shaped Songpan-Ganzi turbidite complex (Weislogel, 2006; Zhu et al., 2016) is bounded by the Western Qinling–Eastern Kunlun orogen to the north, the Longmenshan structural belt and the Khamdian Paleoland complex to the east and southeast, respectively, and the Yidun arc to the west. The complex composed one of the largest continental slope-abyssal flysch turbidites, namely, Xikang Group during Ladinian through Norian Triassic (Zhang, 2013), which is followed by two-way compression of the Qiangtang Block and the North China Block–Kunlun complex from the Late Triassic to the Early Jurassic. Such tectonism resulted in massive folded and uplifted flysches with minor emplacement of Late Triassic (228∼185 Ma) granite (Roger et al., 2010; Zhong et al., 2014).
Located at the northwest margin of NWSB, the Longmenshan belt can be divided into three different structural parts from north to south with widely outcropped pre-Sinian complexes such as the Pengguan Complex at the center part (Xu et al., 1992; Liu, 1995; Jin et al., 2007; Li et al., 2008a; Luo et al., 2014). The Khamdian Paleoland, located at the southern end of Longmenshan belt, has a distance of over 700 km with an approximately N–S trending feature. A number of metamorphic complexes associated with Neoproterozoic granites occurred on the paleoland, including the Kangding complex, Mopanshan-Miyi complex, and Yuanmao complex. Those complexes were dominated by granitic gneiss and migmatitic granite (Zhou et al., 2002; Xu et al., 2010) with minor coeval mafic dykes. The mafic dykes intruding the coeval granitic rocks were roughly E–W trending. Together with numerous coeval granitic and volcanic units throughout the South China Block, this intraplate large igneous province most likely correlated with the breakup of Rodinia (Li et al., 2003; Lin et al., 2007).
The Lower Triassic units in the Sichuan Basin can be divided into the Feixianguan Formation and the Jialinjiang Formation. The Feixianguan Formation, which is conformably laid between the Lower Triassic Jialinjiang Formation carbonate platform deposition and the Upper Permian Changxing Formation, has a total thickness ranging from 100 to 573 m (BGMRSP, 1991). The Feixianguan Formation was composed of siltstone, mudstone, and marlstone that rhythmically interbedded in its lower part, while the middle part was mainly composed of siltstone and mudstone interlayered with silty limestone. The upper part consisted of shale with calcareous mudstone as well as siltstone. In general, the Feixianguan Formation is dominated by shallow-marine platform carbonate with terrigenous clastic rocks decreasing from the NWSB to the Eastern Sichuan Basin (Sun et al., 2009).
The Upper Triassic can be subdivided into the Maantang Formation and Xujiahe Formation in the NWSB. The Maantang Formation is in parallel unconformable contact with the shallow-marine shelf deposits of Middle Triassic Leikoupo Formation (Yu and Liang, 2016). The Xujiahe Formation mainly developed a set of coal-bearing continental deposits that consisted of conglomeratic sandstone, sandstone, siltstone, and mudstone (Yu and Liang, 2016; Luo et al., 2014). As the NWSB became a foreland basin during the Late Triassic, the Xujiahe Formation documented the features of significant vertical and lateral variations in both thickness and lithofacies (Zhang et al., 2006; Li et al., 2010a; Zhang et al., 2015; Zhu et al., 2017). The Xujiahe Formation can be further divided into six members from bottom to top. With member 6 generally missing in the NWSB, the Xujiahe Formation was generally uncomfortably overlain by Lower Jurassic succession. From member 1 to member 5, the sediments exhibited a primary coarse upward feature with member 1 consisting of gray to black shale interbedded with quartz sandstones and shed coals; member 2 is composed of sandstones and siltstones with apparent sedimentation structures; member 3 is dominated by sandstones with shale; and members 4 and 5 comprise sandstone and conglomerate interbedded with thin bedded mudstone and shale (Yu and Liang, 2016).
3 Samples and Analytical Methods
Nine fine to medium grained sandstones and one siltstone were collected from the NWSB for petrology analysis and point counting of compositions (quartz, feldspar, and lithic) (Figures 2, 4). By plotting triangle diagrams of the counting results with the method of Dickinson (1982), Dickinson et al. (1983), and Dickinson (1985), together with the paleocurrent restoration of oblique beddings and cross beddings (Figure 5) (Zhang, 2013), both the source region characteristics and their tectonic setting can be depicted (Figure 6).
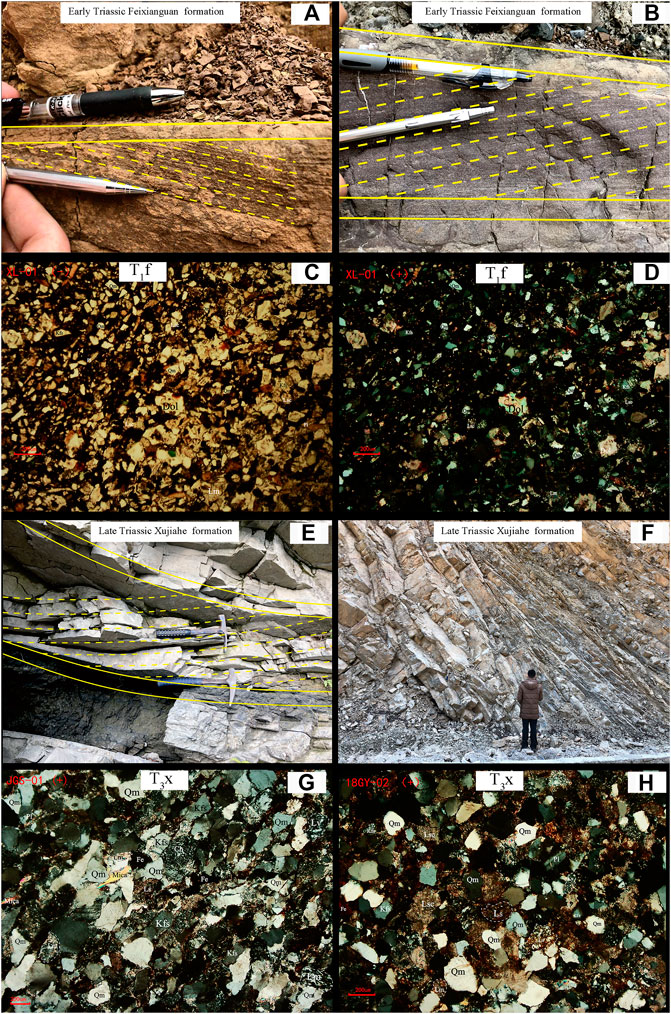
FIGURE 5. Field photographs of typical outcrops with sedimentary structures and photo-micrographs of Lower Triassic and Upper Triassic successions in the Northwestern Sichuan Basin: (A,B) siltstone and fine sandstone of Lower Triassic formation with cross bedding, middle sections of NWSB; (C,D) single and orthogonal polarized light images of Lower Triassic succession, sample XL-01; (E,F) sandstone of Late Triassic formation with the cross bedding, middle section and northern section of NWSB; (G) orthogonal polarized light image of Upper Triassic succession, sample JGS-01, middle section of NWSB; (H) orthogonal polarized light image of Upper Triassic succession, sample 18GY-02, northern section of NWSB. Abbreviations: Qm, monocrystalline quartz; Qp, polycrystalline quartz; Kfs, K-feldspar; Pl, plagioclase; Lv, volcanic lithic fragment; Ls, sedimentary lithic fragment; Lm, metamorphic lithic fragment; Lsc, carbonate rock; Dol, dolomite.
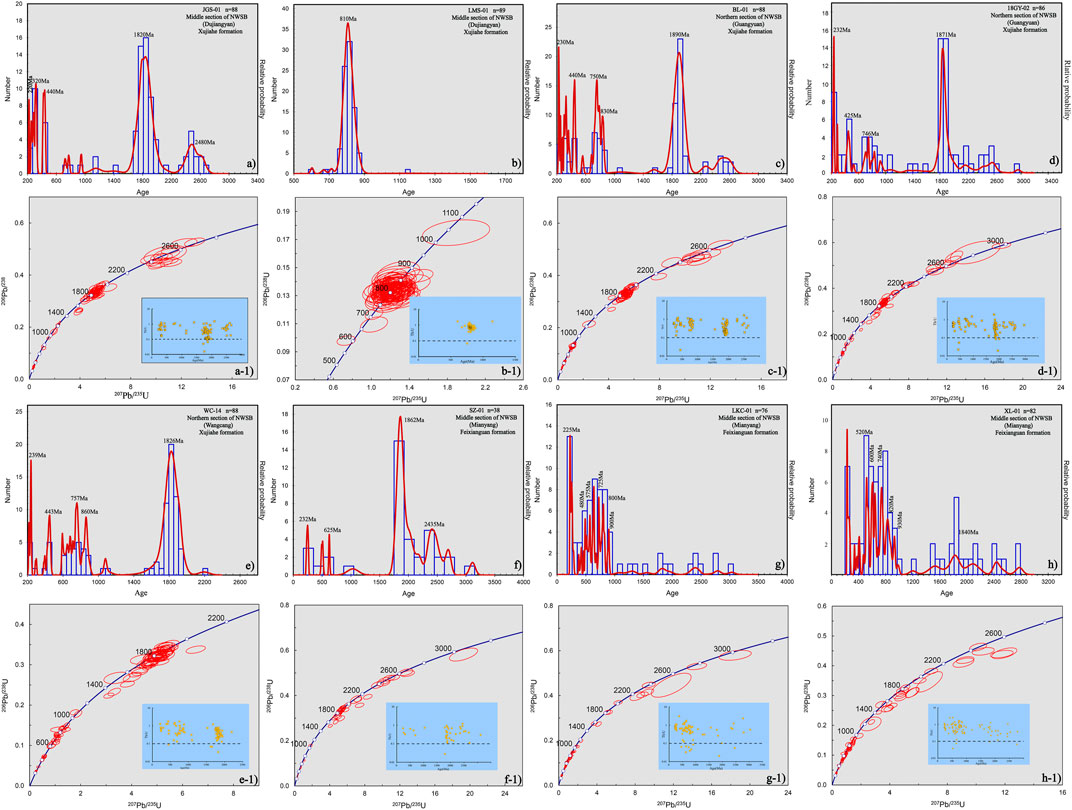
FIGURE 6. Histogram diagram of all detrital zircons in our study (A)-H)) and their corresponding U–Pb concordia with Th/U diagrams (A-1)-H-1)).
Five samples from the Upper Triassic Xujiahe Formation and three samples from the Lower Triassic Feixianguan Formation were collected from the Northwestern Sichuan Basin for detrital zircon U–Pb dating. The separation of zircons was carried out in ChengXin Geology in Hebei Province. After crushing the sample into 0.3∼0.1 mm and separating the zircons by the conventional density and magnetic approach, the zircon grains were mounted in epoxy resin for further analysis. The external properties (surface morphology, color, and crack) and the internal textures like oscillatory zoning of all the zircons were carefully observed on optical micrographs and cathodoluminescence (CL) images, respectively (Figure 7) (Rubattoo and Gebauer, 2000; Corfu et al., 2003; paul and urs, 2003). All the observations were done in the Southwest Petroleum University (SWPU) with the CL images by using a JXA-8230 Electron Microanalyzer in the Electronic Probe Laboratory of SWPU. LA-ICP-MS U–Pb age analysis was conducted using a GeoLas ProHD laser ablation system with an Agilent 7700 plasma mass spectrometer at Sample Solution Technology Company in Wuhan of Hubei Province. The detailed analysis conditions and procedures are similar to those in the studies of Liu et al. (2008), Hu et al. (2015), Zong et al. (2017), and Ludwig (2003). The ages of zircons were divided into two groups, with the ones older than 1,000 Ma corrected by the 207Pb/206Pb ratios while the zircons younger than 1,000 Ma based on their 206Pb/238U ratios. 100%* (206Pb/238U age)/(207Pb/206Pb age) is used for concordance calculation of the older ones and 100%* (206Pb/238U age)/(206Pb/235U) for the younger. Zircons with concordance ≥90% are used for further discussion (Figure 8). Totally, 450 and 224 new detrital zircon grains from the Upper Triassic (Supplementary Table S2) and Lower Triassic (Supplementary Table S1) were analyzed, respectively.
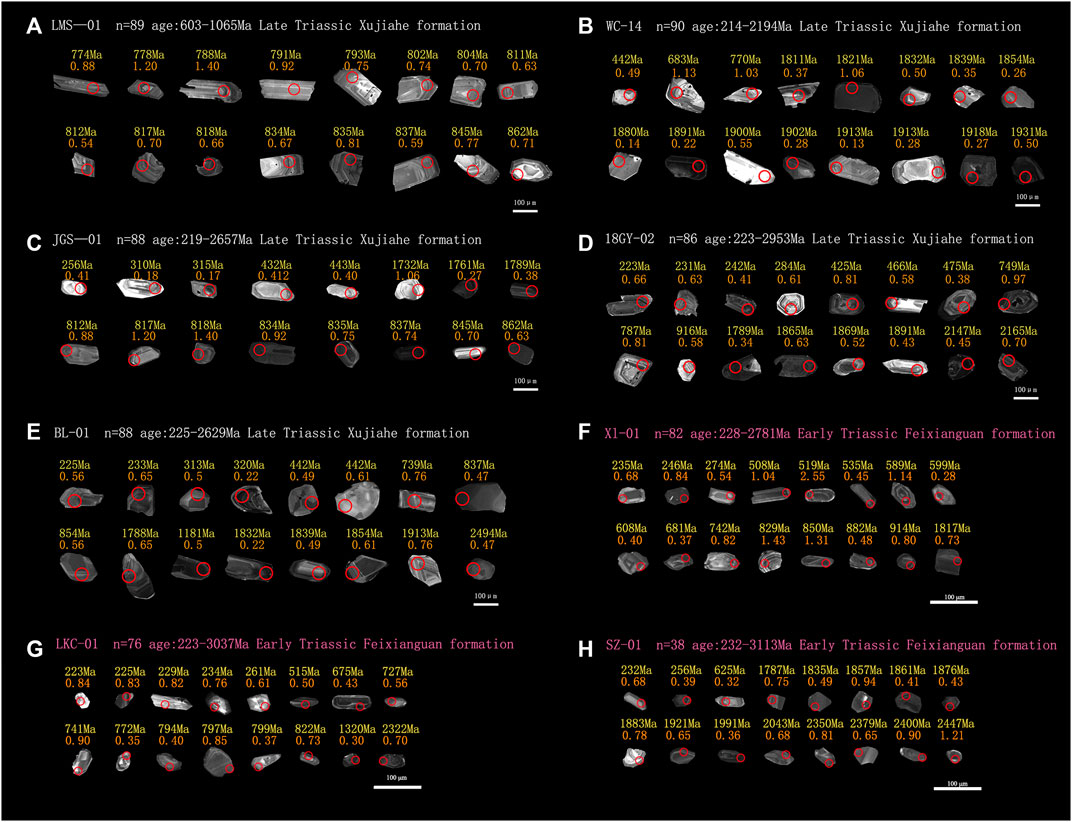
FIGURE 7. Representative cathodoluminescence (CL) images from samples of Late Triassic formation (A–E) and samples of Lower Triassic formation (F–H) showing the internal structure, texture, and morphology of zircon grains. The red circles represent the sites of U–Pb age analysis. The yellow figures and orange figures represent the measured ages and the ratio of Th/U, respectively.
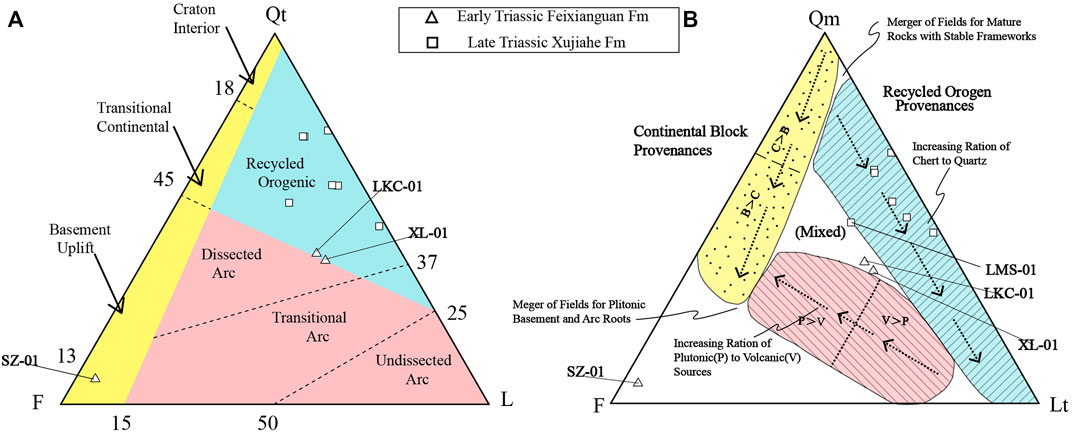
FIGURE 8. Modal triangle diagrams of detrital composition in sandstones: Qt-F-L (A) and Qm-F-Lt (B) (Dickinson et al., 1982; Dickinson et al., 1983; Dickinson et al., 1985). Abbreviations: Qt, total quartz (Qm + Qp); F, total feldspar; L, lithic fragment; Qm, monocrystalline quartz; Qp, polycrystalline quartz; Lt, L + Qp.
4 Results
4.1 Sandstone Petrology
Three sandstones from the Lower Triassic Feixianguan Formation are mainly fine-grained sandstone to siltstone, which have quartz, feldspar, and lithic components (Figures 5A-D). Sample SZ-01 is composed of high feldspar content (60%) with minor quartz and lithic fragments of few pyroxenes. Most of the feldspars are plagioclase with angular monocrystallines, quartz grains are generally sub-angular, and lithic fragments are irregular in shape. Other two samples shear similar characteristics with sub-angular, well-sorted quartz grains (30%–34%), lath-shaped or granular feldspars (16%–20%), and moderate abrasion and generally sub-rounded lithic fragments (8%–10%).
Seven medium to fine grained sandstones from the Upper Triassic Xujiahe Formation were collected for detrital components in Dujiangyan, Guangyuan, and Wangcang Counties (Figures 5E,F). Samples LMS-01 and JGS-01 were from the Dujiangyan area with LMS-01 poorly sorted with sub-angular moderate quartz (30%), sub-angular or sub-rounded low feldspars (15%), and lath-shaped or granular-shaped and irregular-shaped lithic fragments (45%). In contrast, sample JGS-01 has a higher quartz content (65%) and lower lithic components (10%–15%) with good abrasion (Figure 5G). Sample BL-01 collected from the west of Guangyuan contains high quartz content (60%), low feldspar content (5%), and low lithic fragments (20%–25%), of which quartz grains as well as feldspars appear to be sub-angular to sub-rounded monocrystallines with moderate abrasion, while lithic fragments tend to be good abrasive and basically sub-rounded with components of silexite, quartzite, metasiltstone, sericite, and carbonatite. Samples 18GY-01 and 18GY-02 collected from the Guangyuan area are fine-grained sandstones with moderate quartz grains (25%–30%), low feldspars (<5%), and high lithic fragments (50%) (Figure 5H). The quartz grains are mostly sub-angular to sub-rounded monocrystallines with moderate abrasion; most of feldspars are potassium feldspars that are lath-shaped or granular-grained. Lithic fragments, which contain limestone, silexite, and slate, are generally sub-rounded with good abrasion. Samples WC-14 and WC-20 were collected from Wangcang in the northern SCB, and both presented high quartz content (55%–60%) and moderate lithic fragments (20%–25%) with few feldspars (<5%), of which quartz grains tend to be mostly sub-angular and have poor abrasion; feldspars are sub-angular to sub-rounded in a lath shape, and lithic fragments mainly composed of silexite, quartzite, slate, and phyllite have a moderate abrasion.
4.2 U–Pb Ages of Detrital Zircons
4.2.1 Sample LMS-01 (T3x2)
Sample LMS-01, medium to fine grained sandstone, was collected from the Upper Triassic Xujiahe Formation adjacent to the Dujiangyan area of NWSB (Figure 3B). A total of 90 detrital zircons were analyzed; only one did not pass the <10% discordance filter. The ages of zircons were mainly distributed in the range of ∼880–751 Ma (95%) with a major peak at 800 Ma (Figure 8B). The features of these detrital zircons indicated a probably proximal source.
4.2.2 Sample JGS-01 (T3x4)
Sample JGS-01 collected from the Xujiahe Formation (Figure 3C) is the fine-grained lithic sandstone. As 90 detrital zircons were analyzed, only two did not pass the <10% discordance filter. With few zircons presenting Th/U < 0.1, others have high Th/U values (0.11–1.52), implying a magmatic origin. The ages of detrital zircons could be divided into five groups, ∼287–219 Ma group (6%), 326–307 Ma group (8%), 444–415 Ma group (6%), 1989–1720 Ma group (53%), and 2,540–2,362 Ma group (10%), with a major peak at ∼220 Ma, ∼320 Ma, ∼440 Ma, ∼1820 Ma, and ∼2,400 Ma, respectively, and there are some disperse clusters lying between 700 and 1,000 Ma (Figure 8A). All the zircon grains exhibit a relatively proximal source in that the zircons are almost slightly rounded with oscillatory zoning.
4.2.3 Sample BL-01 (T3x2)
Sample BL-01 collected from the Upper Triassic Xujiahe Formation in the west of Guangyuan (Figure 3A) is the fine-grained sandstone. About 88 out of 90 detrital zircons passed the <10% discordance filter. Zircons have high Th/U values (∼0.2–1.65) with few <0.1, suggesting a magmatic origin. The ages of zircons could be divided into four age groups, ∼250–225 Ma group (6%), 456–430 Ma group (7%), 854–730 Ma group (18%), and 1963–1788 Ma group (43%). The major peaks in the younger group (∼456–225 Ma) are at ∼230 Ma and 440 Ma, and those in other age groups are at ∼750 Ma, 830 Ma, and 1890 Ma (Figure 8C). The zircons in the young group (∼456–225 Ma) have mainly euhedral to subhedral morphology showing oscillatory zoning or sector zoning, suggesting a relatively proximal source. And those in the group from ∼1963 to 730 Ma are slightly rounded as a result of medium- to long-distance transportation or recycled materials origin.
4.2.4 Sample 18GY-02 (T3x1)
Sample 18GY-02 was collected from the Upper Triassic Xujiahe Formation near Guangyuan (Figure 3A), which is the fine-grained sandstone. Only 4 out of 90 detrital zircons did not pass the <10% discordance filter. With a mean value of 1.02, zircons display a Th/U ratio from ∼0.10 to 1.92. The ages of zircons could be classified into four groups, ∼242–223 Ma (10%), 475–423 Ma (10%), 837–741 Ma (6%), and 1943–1788 Ma (36%), with their major peaks at ∼232, ∼425, ∼746, and ∼1,871 Ma, respectively (Figure 8D). The zircons falling in the 242–223 Ma group generally have euhedral to subhedral morphology indicating a relatively proximal source, while in other groups, they are slightly rounded as a result of medium- to long-distance transportation or recycled materials origin.
4.2.5 Sample WC-14 (T3x3)
Sample WC-14 collected from the Upper Triassic Xujiahe Formation in Wangcang (Figure 3A) is medium to fine grained sandstone. About 90 zircons were analyzed in this sample, and all the grains passed the filter and could be put into following interpretation. With their Th/U ratio from 0.12 to 1.7 as well as a mean value of 1.11 in this sample, it is supposed to be magmatic protolith. The age of detrital zircons in this sample ranges from 214 to 2,194 Ma and could be divided into five groups, ∼242–214 Ma (6%), 455–423 Ma (6%), 782–722 Ma (9%), 896–817 Ma (8%), and 1,940–1,739 Ma (53%) groups, with their major peaks at ∼239, ∼443, ∼757, ∼860, and ∼1,826 Ma, respectively (Figure 8E). Similar to the former sample, the younger groups (∼242–214 Ma) are generally euhedral to subhedral with oscillatory or planer composition zoning, suggesting a relatively proximal source, while the older groups are sub-euhedral or slightly rounded displaying prominent oscillatory zoning, implying a proximal orogeny or a medium- to long-distance transportation.
4.2.6 Sample XL-01 (T1f)
Sample XL-01 was collected from the Lower Triassic Feixianguan Formation in Dayi at the southwest part of NWSB (Figure 3C), which belongs to the fine-grained sandstone. About 82 out of 90 zircons passed the <10% discordance filter, which could be put into following discussion. Combined with their high Th/U (0.11–2.55), the zircons in this sample display oscillatory or planer composition zoning, exhibiting a basically magmatic origin. Their ages range from 228 to 2,781 Ma with major peaks at ∼230, 520, 600, 740, 820, 930, and 1,840 Ma. The age spectrum could be divided into five groups, ∼280–230 Ma (10%), 681–508 Ma (26%), 882–704 Ma (23%), 983–914 Ma (5%), and 1,887–1,754 Ma (10%) (Figure 8H). The youngest group from 228 Ma to 296 Ma is generally euhedral to sub-euhedral and exhibits even a slightly rounded morphology due to a relatively proximal origin.
4.2.7 Sample LKC-01 (T1f)
Sample LKC-01 is the fine siltstone collected from the Lower Triassic Feixianguan Formation near Mianzhu (Figure 3B). About 90 grains were analyzed in this sample, in which only 76 passed the <10% discordance filter. With quite a few grains <0.1, others show Th/U ranging from 0.10 to 3.07 and most of the detrital zircons show oscillatory or planer composition zoning. Therefore, most of detrital zircons tend to be of magmatic origin. The ages of grains range from approximately 223 Ma to 3,037 Ma with the most group ranging from 223 Ma to 901 Ma. The age spectrum of zircons can be classified into four main groups, ∼285–223 Ma (21%), 679–508 Ma (25%), and 901–724 Ma (25%) with some minor clusters at 2.4 Ga (5%) (Figure 8G). Grains in the youngest group (∼285–223 Ma) are slightly sub-euhedral with a few euhedral morphology as a result of short transportation distance. But zircons in groups of 679–508 Ma and 901–724 Ma are basically sub-rounded suggesting a medium- to long-distance transportation.
4.2.8 Sample SZ-01 (T1f)
Sample SZ-01 collected from the Lower Triassic Feixianguan Formation near Anxian (Figure 3B) is the siltstone. About 45 zircon grains in this sample were analyzed, and 38 grains passed the <10% discordance filter. The feature of the Th/U ratio ranging from 0.11 to 1.2 except one implying that the zircons have the magmatic origin. All detrital zircons’ ages range from ∼232 to 3,113 Ma. Compared to other samples from Lower Triassic succession, the grains are from the dominated Paleoproterozoic era. The ages can be divided into two groups, ∼1,883–1787 Ma (37%) and 2,489–2,350 Ma (18%), as well as a sporadic cluster ranging from ∼689 to 232 Ma (13%) with major peaks at ∼232, 625, 1,862, and 2,435 Ma (Figure 8F). In this sample, most of the grains are sub-euhedral or slightly rounded; thus, it is supposed to have a relatively proximal origin or a medium-distance transportation.
5 Discussion
5.1 Possible Provenance of Lower Triassic Succession in NWSB
All the three samples were collected from the Lower Triassic Feixianguan Formation with two samples (XL-01, LKC-01) from the middle part and one (SZ-01) from the upper part. The detrital zircon ages of middle part exhibit similar features with major peaks ranging from 290 to 230 Ma, 679 to 508 Ma, 882 to 704 Ma, and 983 to 914 Ma and a minor peak from 2,532 to 2,376 Ma, while the sample from the upper part shows different age spectrum patterns with major peaks ranging from ∼1883 to 1787 Ma and ∼2,489 to 2,350 Ma and minor clusters at ∼260–232 Ma and 625 Ma (Figures 8F–H).
The zircons of 290–230 Ma age in all the samples of the Lower Triassic may probably be related to the Emeishan large igneous province (ELIP) which occurred at ca. 290–260 Ma (Zhou et al., 2002; Guynn et al., 2012; Tang et al., 2014; Xu et al., 2008; He et al., 2007) or related to the early-stage granitic rocks of the Yidun arc complex (Deng et al., 2008; Wang et al., 2013). Considering the ELIP constituted with massive clastic and basaltic rocks that overlain on the Khamdian Paleoland with intrusive bodies that occurred at the northern part, it may be the major source for the 290–230 Ma age zircon group (Xu et al., 2001; Yan et al., 2019).
It is widely shared that the 650–520 Ma age group is associated with the worldwide Pan-African orogenic event, also known as the East Gondwana event. Compared to the wide appearance of zircons within this age, such as in Mesozoic and Paleozoic strata from Indochina Block, Qiangtang Block, Sibumasu Block, and Baoshan Block (Gehrels et al., 2011; Hu et al., 2014), the synchronous igneous plutons sporadically occurred at adjacent blocks, e.g., Amdo terrane of Qiangtang Block (Gehrels et al., 2011; Guynn et al., 2006; 2012; Pullen et al., 2010) and some plutons aged between 580 and 500 Ma in the Eastern Kunlun Range (Ding et al., 2013; Wu et al., 2016). Such mismatch raised controversy to the interpretation of the widely distributed zircon group within this age in the South China Block (Zhu et al. (2017) and references therein). According to the studies of Burrett et al. (2014) and Zhang et al. (2014), as parts of the Gondwana continental group, the South China Block, Qiangtang Block, Sibumasu Block, and Indochina Block may have received recycled orogeny materials from the exotic East African orogenic belt (650–540 Ma) during the Early Paleozoic. As a few tuffaceous beds and metavolcanic strata also occurred in Early Cambrian–Neoproterozoic Ediacaran of Yangtze Craton and in Paleozoic strata of Khamdian Paleoland, respectively (BGMRSP, 1991), the uplifted Khamdian Paleoland can also be responsible for the 0.65–0.52 Ga detritus of the Lower Triassic. This interpretation is also consistent with the recycled orogenic mixed with the dissected arc and basement uplift origin from the Gazzi-Dickinson method and the northeastward to eastward restored paleocurrent measurements (Figures 4A; 6A,B). However, the Eastern Kunlun should be ruled out due to the opening of the Ganzi–Litang Ocean that block off most detritus (Leng et al., 2014). This is also supported by the lack of the 0.65–0.52 Ga detritus for the Lower Triassic succession at the adjacent area of Eastern Kunlun (Wu et al., 2016).
The zircon groups of 998–914 Ma and 882–704 Ma were in coincidence with the amalgamation and breakup of Rodinia supercontinent (Li et al., 2008b), respectively. The magmatism related to this event widely occurred not only in the South China Block but also in the adjacent area, such as the Khamdian Paleoland, the Longmenshan structural belt, and the Qiangtang Block (Figures 9A-I) (Li, 1999; Zhou et al., 2002; Li et al., 2003). Accordingly, the magmatism during this period of the Khamdian Paleoland has a good chance to play a major role of providing detritus for the Lower Triassic Feixianguan Formation of NWSB.
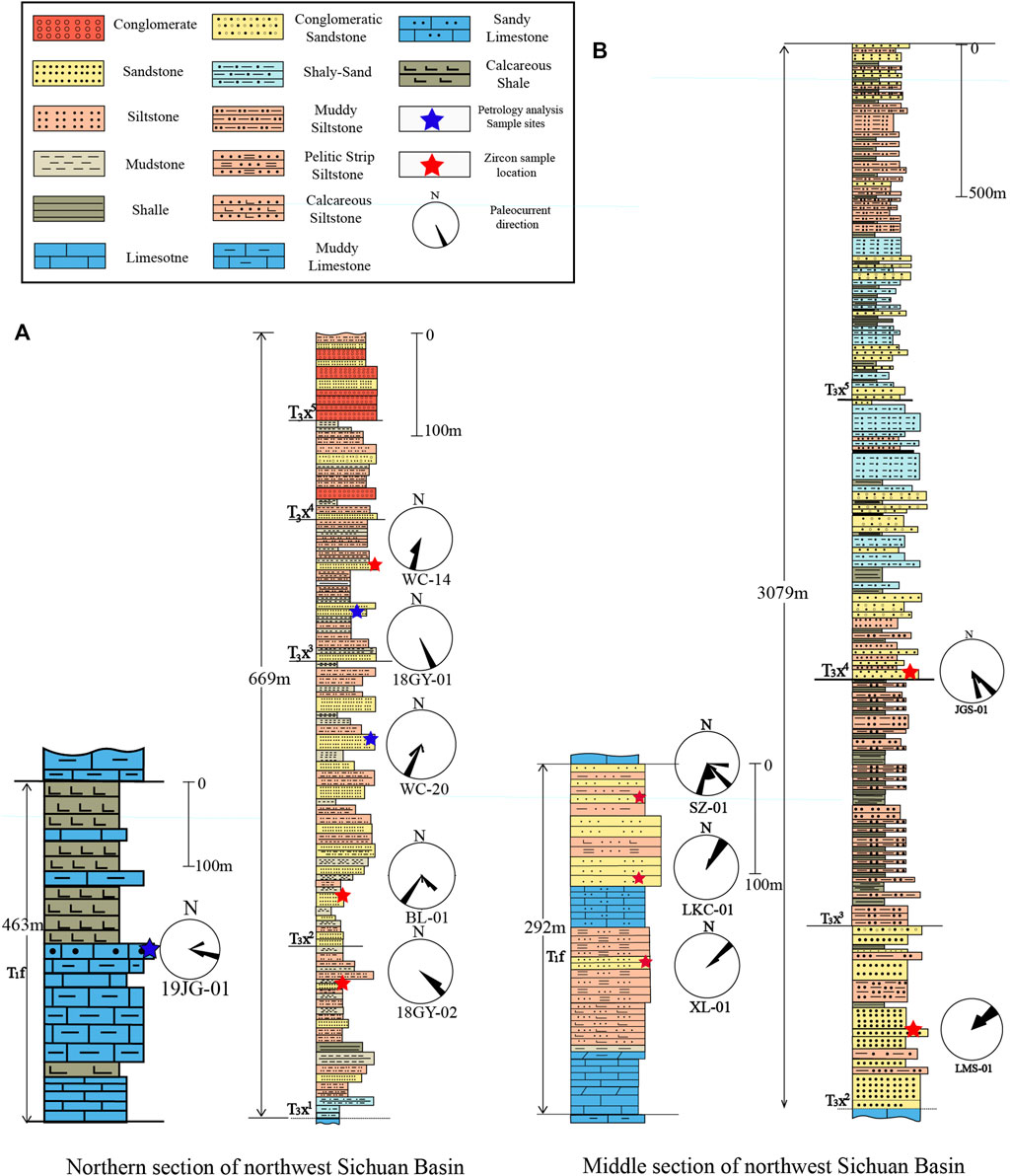
FIGURE 9. Sedimentary stratigraphy of the Lower and Upper Triassic in the study area of Northwestern Sichuan Basin (modified after BGMRSP, 1991; Yu and Liang, 2016). (A) Northern section of the Northwestern Sichuan Basin. (B) Middle section of the Northwestern Sichuan Basin.
The small population of zircons with peaks at ∼1.8 Ga and ∼2.4 Ga in the Early Triassic may probably be from the Qinling belt, the Southern NCB, and the Khamdian Paleoland. However, with the apparent increase of the ∼1.8 Ga zircons in sample XL-01 (Figure 8) at the south part of NWSB and the restored northeastward paleocurrent (Figures 4B, 10), the Khamdian Paleoland, which has 2.3–1.8 Ga, 1.7–1.4 Ga, and 1.2–0.7 Ga detritus, was most likely responsible for the deposition of early stage of the Early Triassic in the northwestern Sichuan Basin (Zhou et al., 2002). Sample SZ-01 from the upper part of the Feixianguan Formation displayed a major peak ranging from ∼1,883 to 1,787 Ma and a subordinate peak of ∼2,489–2,350 Ma. This probably implied a different detritus source input since the middle to the late stage of Early Triassic. The magmatic rocks with ages of ∼1.8 Ga and ∼2.4 Ga were relatively widely exposed in the southern North China Block, Qinling orogenic belt, and northern Yangtze Craton (Figures 2, 9A-J) (Tao, 1993; Dong and Zhao, 2002; Wang et al., 2008; Chen et al., 2016); thus, the increase of ∼1.8 Ga and ∼2.4 Ga zircons may be related to the southern North China Block and Qinling orogenic belt. This observation is consistent with the southward paleocurrent direction (Figures 4B, 6, 10) and basement uplift origin of Gazzi-Dickinson point counting results. The relatively minor peak at 260–232 Ma compared to the lower part of Feixianguan Formation also supports a great source input of the Qinling belt and the southern North China Block (Li and Li., 2003; Jin et al., 2003; Xiao et al., 2011).
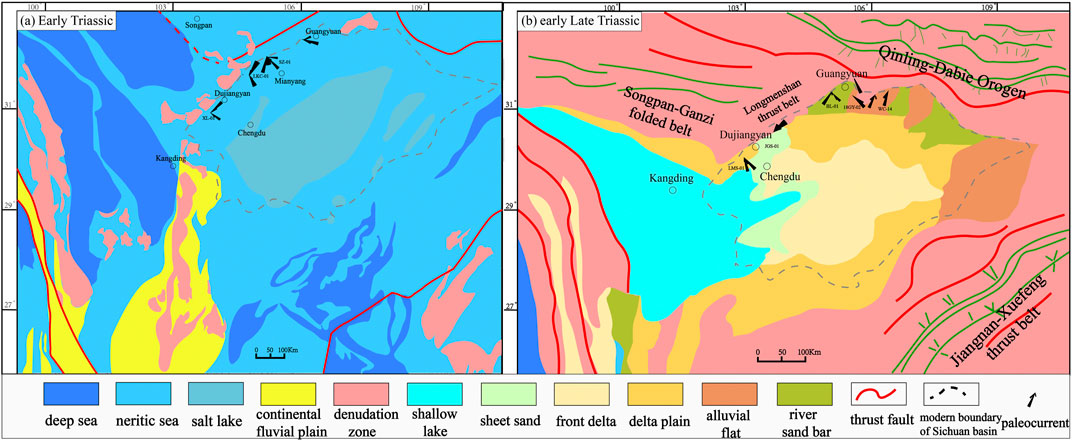
FIGURE 10. Paleographic and paleocurrent direction distribution maps of the Early Triassic (A) and Late Triassic (B) (refer to Wang, 1985; Cui, 2004; Ma et al., 2009; Zhu et al., 2017).
5.2 Possible Provenance of Upper Triassic Succession in NWSB
The biggest zircon age spectrum difference between the Upper Triassic and the Lower Triassic is the abundant ∼1.8 Ga age population and lack of apparent ∼650–500 Ma population. Among all the Upper Triassic samples, 18GY-02 (T3x1), BL-01 (T3x2), and WC-14 (T3x3) from the northern part of NWSB displayed similar detritus age spectrum features with major peaks at ∼250–214 Ma, ∼475–423 Ma, and ∼896–730 Ma and a prominent peak at ∼1940–1739 Ma. This is in accordance with that of the Upper Triassic sandstones from the Central Songpan-Ganzi terrane and inner Sichuan Basin (Figures 9G,H) (Weislogel 2006; Deng et al., 2008; Luo et al., 2014; Li et al., 2016). While the samples from the southern part of NWSB showed slightly different age spectrum features with sample JGS-01 from the southernmost part of NWSB displaying a significant decreasing probability of ∼896–730 Ma zircons, sample LMS-01 at the middle part of NWSB exhibited a unique feature with one single major age peak ranging from ∼880 to 751 Ma. Such difference implied not only the enormous provenance change from the Early Triassic to the Late Triassic but also the multiple source input during the Late Triassic.
Based on the possible provenance analysis of the Lower Triassic, the major age population of ∼1.8 Ga is most likely correlated with the 1.9–1.8 Ga granitic intrusions of the Kongling complex from the northern Yangtze Craton and even part of the southern NCB (Kusky and Li, 2003; Gao et al., 2011; Zhang and Zheng, 2013). However, the very limited distribution of 2.5 Ga zircon group may suggest that the southern part of North China Block (Shi et al., 2013; Zhang et al., 2015) was probably not the major source due to the uplift of the Qinling orogenic belt during the Late Triassic. With the blocking of the source from the southern part of North China Block, the SQB and northern Yangtze Block were most likely responsible for the Late Triassic sediments of NWSB.
The relatively small population of ∼896–730 Ma is in coincidence with the breakup of the supercontinent Rodinia (Li et al., 2008b), which resulted in widespread synchronous plutons, dyke swarms, and volcanics in the South China Block, Qinling orogen, uplifted Longmenshan structural belt, and Khamdian Paleoland. The ∼475–423 Ma age peak is consistent with the Caledonian Orogeny event that occurred at the Qinling orogen (Figure 9D; Lerch et al., 1995; Shi et al., 2013; Zhu et al., 2018) and southeastern South China Block (Ren et al., 1997; Li et al., 2010b), that is, synchronous metamorphic and magmatic rocks were widely developed.
For the major population of ∼250–214 Ma zircons, the Qinling belt to the north and the Emeishan large igneous province (ELIP) to the southwest could be the possible sources. Considering the collision between South Qinling and Yangtze Craton that mainly occurred during the Late Permian to the Early Triassic (Jin et al., 2005; Xiao et al., 2011) with massive synorogenic and postorogenic magmatic rocks of ∼240–210 Ma widely exposed (Dong and Zhao, 2002), the Qinling belt must have been responsible for the detritus of the Upper Triassic. This is also in coincidence with the southward or southwestward paleo-flow direction of this and previous studies (Meng et al., 2005; Xiao et al., 2011). Moreover, the Qm-F-L and Qm-F-Lt triangular diagram (Dickinson, 1985) showed that all the Upper Triassic sandstone samples plot into the field of recycled orogeny. This also indicates the major source of the Qinling orogeny for the NWSB.
The prominent single age peak of ∼880–751 Ma occurred at LMS-01 implying one dominant source with local depositions. The intensely euhedral zircon grains and high lithic fragment portion (45%) also indicated a short transportation of the detritus. Thus, the adjacent Pengguan Complex in the Longmenshan belt which has the age of ∼859–700 Ma (Ma et al., 1996; Yan et al., 2008) is most likely to be the major source. This is also consistent with the northeastward paleo-flow direction and the Qm-F-Lt triangular diagram results (Figures 4, 6). The significant decreasing probability of ∼896–730 Ma zircons of sample JGS-01 also supports the limited uplift of the Pengguan Complex.
5.3 Tectonic–Paleogeographic Evolution From Early to Late Triassic of Northwestern Sichuan Basin
It is widely accepted that the Indosinian event played a major role in the Mesozoic evolution of the Sichuan Basin, especially driving the NWSB into a foreland basin (Meng et al., 2005), and fully changed the deep to shallow-marine carbonate sediments (BGMRSP, 1991; Ma et al., 2009) into continental coal-bearing deposits (Li et al., 2014) from the Middle to the Late Triassic. Based on sandstone petrology, detrital zircon geochronology, and paleocurrent indicator analysis data, this study tries to unravel the provenance change from the Early Triassic to the Late Triassic and reveal its connection to the tectonic evolution of NWSB.
According to all the discussion above, the uplifted Khamdian Paleoland may have provided most of the detritus for the Lower Triassic strata of NWSB, for it widely developed the ELIP massive clastic and basaltic rocks and Rodinia-related magmatism as well as a few tuffaceous beds and metavolcanic strata in Paleozoic strata. The Qiangtang Block and other potential western source were ruled out due to the presence of the Paleo-Tethys Ocean which served as a natural physical barrier (Figure 11A), while the Qinling belt and the southern North China Block at the north may provide a small amount of the detritus. Such tectonic–paleogeographic configuration may last till the end of Middle Triassic (Zhu et al., 2017; Yan et al., 2018).
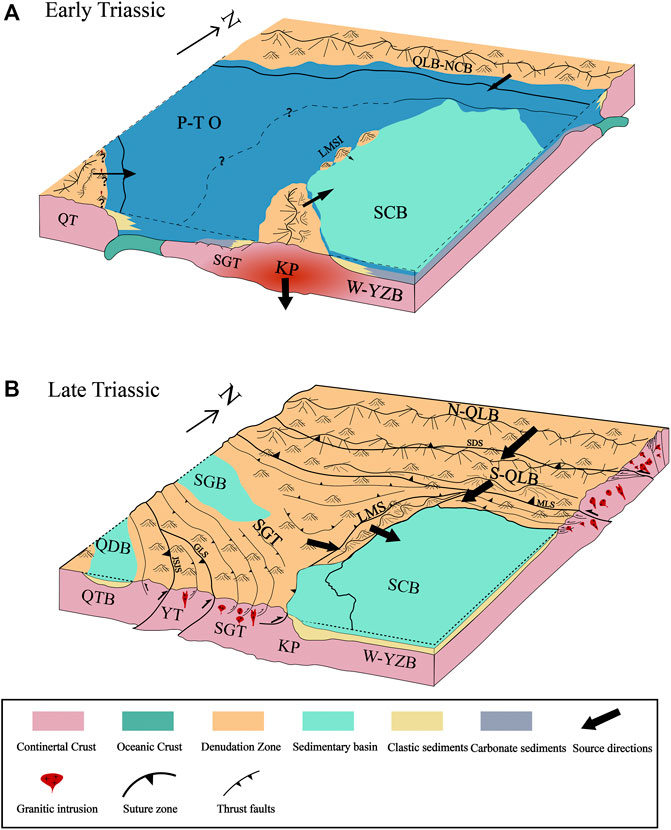
FIGURE 11. Schematic diagram showing the Early Triassic (A) and Late Triassic (B) tectonic evolution of the Northwestern Sichuan Basin (modified from Yan (2018)). SCB, Sichuan Basin; SGT, Songpan-Ganzi terrane; QLB-NCB, Qinling belt-North China Block; S-QLB, South Qinling belt; N-QLB, North Qinling belt; LMS, Longmenshan thrust belt; LMSI, Longmenshan island; P-T O, Paleo-Tethys Ocean; QTB, Qiangtang Block; YT, Yidun terrane; KP, Khamdian Paleoland; W-YZB, Western Yangtze Block; SDS, Shangdan suture; MLS, Mianlue suture; GLS, Ganzi–Litang suture; JSJS, Jinshajiang suture.
As the magmatic events of ELIP caused the uplift and erosion of the southwestern Yangtze before and during the eruption (Yu et al., 2014), the Khamdian Paleoland served as a major source region for the Lower Triassic deposits of the Sichuan Basin. The shrank detritus transportation during the Middle Triassic as the detrital rocks rarely distributed in south-southwest Sichuan Basin adjacent to the Khamdian Paleoland (Zhu et al., 2017; Yan et al., 2018) indicated that it may undergo a gradually thermal subsidence process as a response of the ELIP (Saunders et al., 2005). The gravitational loading caused by the significant shortening of eastern Songpan-Ganze terrane and collision of the Yangtze Block, Yidun arc, and Qiangtang terrane (Yan et al., 2018) may also contribute to the subsidence of the paleoland (Figure 11). The muscovite 40Ar/39Ar age of 235–226 Ma of Pengguan Complex at the Longmenshan belt also recorded such significant crustal shortening (Yan et al., 2011; Zheng et al., 2016).
During the Late Triassic, the intensely activation of Sanjiang, Qinling, Songpan Ganzi and Longmenshan structural Belts resulted in the formation of similar asymmetircal wedge shaped regions adjacent to those belts and received abundant sediments that became thicker as it closer to the structural belts (BGMRSP, 1991; Zhu et al., 2017). The Late Triassic significant shortening of eastern Songpan-Ganze terrane and uplifting of Longmenshan belt are supported by the abundant Pengguan Complex zircons occurring in LMS-01 (T3x2) as well as the southeastward paleocurrent and similar age spectrum of JGS-01 (T3x4). As indicated by our detrital zircons, paleocurrent data, and results of previous studies (Shao et al., 2016), during the Late Triassic, the South Qinling belt and Yangtze Block collided along the Mianlue suture resulting in the limited clastic material transportation to the northern part of Sichuan Basin, and they may not play a major role in the middle to the south part of Northwestern Sichuan Basin. Overall, the convergence of the Qiangtang Block, Yangtze Block, and North China Block during the Late Triassic that lead to the development of the Sanjiang orogen, Songpan-Ganzi complex and Longmenshan thrust belt, and the Qinling orogen have profoundly changed the Sichuan Basin including the provenance configuration (Figure 11B), and resulted in the NWSB evolving from the passive continental margin type basin into a foreland basin.
6 Conclusion
(1) 196 and 441 new U–Pb ages of detrital zircons of Lower Triassic and Upper Triassic sandstones from the NWSB, respectively, display different age populations. The U–Pb age for the Lower Triassic detrital zircons generally shows populations at ∼1,850 Ma, 980–705 Ma, 680–510 Ma, and 290–230 Ma and a minor cluster at ca 2.4 Ga. In contrast, the Upper Triassic shows major peaks at 855–730 Ma, 455–415 Ma, and 290–215 Ma and a prominent age group of 1.9–1.7 Ga.
(2) Combined with the paleocurrent and sandstone composition analysis, a major change in provenance from the Early Triassic to the Late Triassic can be demonstrated. The uplifted Khamdian Paleoland probably supplied most materials for the NWSB during the Early Triassic, with small part from the southern North China Block and Qinling orogenic belt. During the Late Triassic, the Qinling orogenic belt served as the major source region for the north part of the Sichuan Basin and the uplifted Longmenshan thrust belt and Songpan-Ganzi terrane provided the majority sediment for the center and south parts of the Northwestern Sichuan Basin.
(3) The transition of the provenance probably resulted in the combined effect of gradually thermal subsidence and the gravitational loading of the Khamdian Paleoland correlated with the ELIP and the eastward shortening of the Songpan-Ganzi terrane, respectively. Together with the ongoing convergence between Yangtze Block and North China Block, the Late Triassic provenance of the Northwestern Sichuan Basin was dominated by the uplifted Longmenshan thrust belt and the Qinling orogen.
Data Availability Statement
The original contributions presented in the study are included in the article/Supplementary Material, and further inquiries can be directed to the corresponding author.
Author Contributions
All authors listed have made a substantial, direct, and intellectual contribution to the work and approved it for publication.
Funding
This study was supported by funds of the Key Laboratory of Tectonic Controlled Mineralization and Oil Reservoir, Ministry of Land and Resources (gzck201903), School-Enterprise Cooperation Project of Zhejiang University of Technology (KYY-HX-20190113), National Major Science and Technology Projects of China (2017ZX05005001-002) and National Natural Science Foundation of China (Grant No. 41302165).
Acknowledgments
We thank C. M. Fang and B. H. Huang for their assistance during the field trip, H. Z. Wang for the assistance with cathodoluminescence analyses, and H. F. Chen for the assistance with zircon U–Pb analyses.
Conflict of Interest
The authors declare that the research was conducted in the absence of any commercial or financial relationships that could be construed as a potential conflict of interest.
Publisher’s Note
All claims expressed in this article are solely those of the authors and do not necessarily represent those of their affiliated organizations, or those of the publisher, the editors, and the reviewers. Any product that may be evaluated in this article, or claim that may be made by its manufacturer, is not guaranteed or endorsed by the publisher.
Supplementary Material
The Supplementary Material for this article can be found online at: https://www.frontiersin.org/articles/10.3389/feart.2022.940301/full#supplementary-material
References
Amidon, W. H., Burbank, D. W., and Gehrels, G. E. (2005). U–Pb Zircon Ages as a Sediment Mixing Tracer in the Nepal Himalaya. Earth Planet. Sci. Lett. 235, 244–260. doi:10.1016/j.epsl.2005.03.019
Bruguier, O., Lancelot, J. R., and Malavieille, J. (1997). U-pb Dating on Single Detrital Zircon Grains from the Triassic Songpan-Ganze Flysch (Central China): Provenance and Tectonic Correlations. Earth Planet. Sci. Lett. 152, 217–231. doi:10.1016/s0012-821x(97)00138-6
Burrett, C., Khin Zaw, K., Meffre, S., Lai, C. K., Khositanont, S., Chaodumrong, P., et al. (2014). The Configuration of Greater Gondwana-Evidence from LA ICPMS, U-Pb Geochronology of Detrital Zircons from the Palaeozoic and Mesozoic of Southeast Asia and China. Gondwana Res. 26, 31–51. doi:10.1016/j.gr.2013.05.020
Chang, E. Z. (2000). Geology and Tectonics of the Songpan-Ganzi Fold Belt, Southwestern China. Int. Geol. Rev. 42, 813–831. doi:10.1080/00206810009465113
Chen, Q., Sun, M., Long, X., Zhao, G., and Yuan, C. (2016). U-pb Ages and Hf Isotopic Record of Zircons from the Late Neoproterozoic and Silurian-Devonian Sedimentary Rocks of the Western Yangtze Block: Implications for its Tectonic Evolution and Continental Affinity. Gondwana Res. 31, 184–199. doi:10.1016/j.gr.2015.01.009
Chen, S. F., and Wilson, C. J. L. (1996). Emplacement of the Longmen Shan Thrust-Nappe Belt along the Eastern Margin of the Tibetan Plateau. J. Struct. Geol. 18, 413–430. doi:10.1016/0191-8141(95)00096-v
Chen, Y., Liu, S. G., Li, Z. W., Deng, B., Zeng, X. L., and Lin, J. (2011). LA-ICP-MS detritaI Zircon U-Pb Geochronology Approaches to the Sediment Provenance of the Western Sichuan Foreland Basin and Limited Uplift of the Longmen Mountain during the Early Stage of Late Triassic. Geotectionica Metallogenia 35, 315–323. doi:10.16539/j.ddgzyckx.2011.02.017
Corfu, F., Hanchar, J. M., Paul, W. O. H., and Kinny, P. (2003). Atlas of Zircon Textures. Rev. Mineralogy Geochem. 53, 469–500. doi:10.2113/0530469
Cui, B. Q., Long, X. M., and Li, Y. L. (1991). The Subsidence of Western Sichuan Depression and the Rise of Longmenshan Mountains. J. Chengdu Coll. Geol. 18, 39–44.
Cui, K. X. (2004). Regional Palaeogrography and its Evolution Atlas of Southwestern China. Beijing: Seismological Press, 25–130.
Deng, F., Jia, D., Luo, L., Li, H. B., Li, Y. Q., and Wu, L. (2008). The Contrast between Provenances of Songpan-Garze and Western Sichuan Foreland Basin in the Late Triassic: Cluesto the Tectonics and Paleogeography. Geol. Rev. 54, 561–573. doi:10.1016/S1872-5791(08)60056-1
Dickinson, W. R., Beard, L. S., Brakenridge, G. R., Erjavec, J. L., Ferguson, R. C., Inman, K. F., et al. (1983). Provenance of North American Phanerozoic Sandstones in Relation to Tectonic Setting. Geol. Soc. Am. Bull. 94, 222–235. doi:10.1130/0016-7606(1983)94<222:ponaps>2.0.co;2
Dickinson, W. R. (1982). Compositions of Sandstones in Circum-Pacific Subduction Complexes and Fore-Arc Basins. Aapg Bull. 66, 121–137. doi:10.1306/03b59a4e-16d1-11d7-8645000102c1865d
Dickinson, W. R. (1985). Provenance and Sediment Dispersal in Relation to Paleotectonics and Paleogeography of Sedimentary Basins. New Perspectives in Basin Analysis. New York: Springer, 3–25.
Ding, L., Yang, D., Cai, F. L., Pullen, A., Kapp, P., Gehrels, G. E., et al. (2013). Provenance Analysis of the Mesozoic Hoh-Xil-Songpan-Ganzi Turbidites in Northern Tibet: Implications for the Tectonic Evolution of the Eastern Paleo-Tethys Ocean. Tectonics 32, 34–48. doi:10.1002/tect.20013
Dong, Y. P., and Zhao, X. (2002). The Magmatism and Tectonic Events in South Qinling Belt: its Implications for the Crust Growth. J. Northwest Univ. Nat. Sci. Ed. 2, 172–176. doi:10.1080/12265080208422884
Duan, L., Meng, Q.-R., Zhang, C.-L., and Liu, X.-M. (2011). Tracing the Position of the South China Block in Gondwana: U-Pb Ages and Hf Isotopes of Devonian Detrital Zircons. Gondwana Res. 19, 141–149. doi:10.1016/j.gr.2010.05.005
Federico, L., Capponi, G., Crispini, L., and Scambelluri, M. (2004). Exhumation of Alpine High-Pressure Rocks: Insights from Petrology of Eclogite Clasts in the Tertiary Piedmontese Basin (Ligurian Alps, Italy). Lithos 74, 21–40. doi:10.1016/j.lithos.2003.12.001
Gao, S., Yang, J., Zhou, L., Li, M., Hu, Z., Guo, J., et al. (2011). Age and Growth of the Archean Kongling Terrain, South China, with Emphasis on 3.3 Ga Granitoid Gneisses. Am. J. Sci. 311, 153–182. doi:10.2475/02.2011.03
Gehrels, G., Kapp, P., and DeCelles, P. (2011). Detrital Zircon Geochronology of Pre-tertiary Strata in the Tibetan-Himalayan Orogen. Tectonics 30, 1–27. doi:10.1029/2011tc002868
Gehrels, G., and Ross, G.(1998). Detrital Zircon Geochronology of Neoproterozoic to Permian Miogeoclinal Strata in British Columbia and Alberta. Canad. J. Earth Sci., 1998, 35(12):1380-1401. doi:10.1139/cjes-35-12-1380
Guo, Z. W., Deng, K. L., and Han, Y. F. (1996). Formation and Evolution of the Sichuan Basin. Beijing: Geologic Publishing House, 1–52.
Guynn, J. H., Kapp, P., Pullen, A., Heizler, M., Gehrels, G., and Ding, L. (2006). Tibetan Basement Rocks Near Amdo Reveal “Missing” Mesozoic Tectonism along the Bangong Suture, Central Tibet. Geol 34, 505–508. doi:10.1130/g22453.1
Guynn, J., Kapp, P., Gehrels, G. E., and Ding, L. (2012). U-pb Geochronology of Basement Rocks in Central Tibet and Paleogeographic Implications. J. Asian Earth Sci. 43, 23–50. doi:10.1016/j.jseaes.2011.09.003
Hawkesworth, C. J., and Kemp, A. I. S., 2006. Using Hafnium and Oxygen Isotopes in Zircons to Unravel the Record of Crustal Evolution. Chem. Geol. 226, 144–162. doi:10.1016/j.chemgeo.2005.09.018
Hawkesworth, C. J., Dhuime, B., Pietranik, A. B., Cawood, P. A., Kemp, A. I. S., and Storey, C.D. (2010). The Generation and Evolution of the Continental Crust. J. Geol. Soc. London 167, 229–248. doi:10.1144/0016-76492009-072
He, B., Xu, Y. G., Huang, X. L., Luo, Z. Y., Shi, Y. R., Yang, Q. J., et al. (2007). Age and Duration of the Emeishan Flood Volcanism, SW China: Geochemistry and Shrimp Zircon u–pb Dating of Silicic Ignimbrites, Post-Volcanic Xuanwei Formation and Clay Tuff at the Chaotian Section. Earth Planetary Sci. Lett., 255(3-4), 306-323. doi:10.1016/j.epsl.2006.12.021
Hu, L., Du, Y., Cawood, P. A., Xu, Y., Yu, W., Zhu, Y., et al. (2014). Drivers for Late Paleozoic to Early Mesozoic Orogenesis in South China: Constraints from the Sedimentary Record. Tectonophysics 618, 107–120. doi:10.1016/j.tecto.2014.01.037
Hu, Z., Zhang, W., Liu, Y., Gao, S., Li, M., Zong, K., et al. (2015). "Wave" Signal-Smoothing and Mercury-Removing Device for Laser Ablation Quadrupole and Multiple Collector ICPMS Analysis: Application to Lead Isotope Analysis. Anal. Chem. 87, 1152–1157. doi:10.1021/ac503749k
Kemp, A.I.S., Hawkesworth, C.J., Paterson, B.A., and Kinny, P.D.(2006). Episodic Growth of the Gondwana Supercontinent From Hafnium and Oxygen Isotopes in Zircon.Nature 439, 580–583. doi:10.1038/nature04505
Kusky, T., and Li, J. (2003).Paleoproterozoic Tectonic Evolution of the North China Craton. J. Asia. Earth Sci.. 22(4):0-397. doi:10.1016/s1367-9120(03)00071-3
Jin, W. J., Zhang, Q., He, D. F., and Jia, X. Q. (2005). SHRIMP Dating of Adakites in Western Qinling and Their Implications. Acta Petrol. Sin. 21, 959–966. doi:10.3321/j.issn:1000-0569.2005.03.033
Jin, W. Z., Tang, L. J., Yang, K. M., Wan, G. M., Lyu, Z. Z., and Yu, Y. X. (2007). Deformation and Zonation of the Longmenshan Fold and Thrust Zone in the Western Sichuan Basin. Acta Geol. Sin. 81, 1072–1080. doi:10.1016/0248-4900(96)81468-7
Lee, J., Williams, I., and Ellis, D.(1997). Pb, U and Th Diffusion in Nature Zircon.Nature 390, 159–162. doi:10.1038/36554
Leng, C.-B., Huang, Q.-Y., Zhang, X.-C., Wang, S.-X., Zhong, H., Hu, R.-Z., et al. (2014). Petrogenesis of the Late Triassic Volcanic Rocks in the Southern Yidun Arc, SW China: Constraints from the Geochronology, Geochemistry, and Sr-Nd-Pb-Hf Isotopes. Lithos 190-191, 363–382. doi:10.1016/j.lithos.2013.12.018
Lerch, M. F., Xue, F., Kröner, A., Zhang, G. W., and Tod, W. (1995). A Middle Silurian-Early Devonian Magmatic Arc in the Qinling Mountains of Central China. J. Geol. 103, 437–449. doi:10.1086/629762
Li, Y.J., Shao, L.Y., and Eriksson, K.A.(2014). Linked Sequence Stratigraphy and Tectonics in the Sichuan Continental Foreland Basin, Upper Triassic Xujiahe Formation, Southwest China. J. Asian Earth Sci. 88, 116–136. doi:10.1016/j.jseaes.2014.02.025
Li, R. B., Pei, X. Z., Liu, Z. Q., and Li, Z. C. (2010a). Basin-mountain Coupling Relationship of Foreland Basins between Dabashan and Northeastern Sichuan: the Evidence from LAICP-MS UPb Dating of Detrital Zircons. Acta Geol. Sinica-English Ed. 84, 1118–1134. doi:10.1017/S0004972710001772
Li, S. G., Li, Q. L., Hou, Z. H., Yang, W., and Wang, Y. (2005). Cooling History and Exhumation Mechanism of the Ultrahigh-Pressure Metamorphic Rocks in the Dabie Mountains. Acta Petrol. Sin. 21, 1117–1124. doi:10.1016/j.sedgeo.2005.05.009
Li, W., and Li, X. H. (2003). Adakitic Granites within the NE Jiangxi Ophiolites, South China: Geochemical and Nd Isotopic Evidence. Precambrian Res. 122, 29–44. doi:10.1016/s0301-9268(02)00206-1
Li, X.-H. (1999). U-pb Zircon Ages of Granites from the Southern Margin of the Yangtze Block: Timing of Neoproterozoic Jinning: Orogeny in SE China and Implications for Rodinia Assembly. Precambrian Res. 97, 43–57. doi:10.1016/s0301-9268(99)00020-0
Li, Y., He, D., Li, D., Wen, Z., Mei, Q., Li, C., et al. (20162016). Detrital Zircon U-Pb Geochronology and Provenance of Lower Cretaceous Sediments: Constraints for the Northwestern Sichuan Pro-foreland Basin. Palaeogeogr. Palaeoclimatol. Palaeoecol. 453, 52–72. doi:10.1016/j.palaeo.2016.03.030
Li, Z., Li, X. H., Kinny, P. D., Wang, J., Zhang, S., and Zhou, H. (2003). Geochronology of Neoproterozoic Syn-Rift Magmatism in the Yangtze Craton, South China and Correlations with Other Continents: Evidence for a Mantle Superplume that Broke up Rodinia. Precambrian Res. 122, 85–109. doi:10.1016/s0301-9268(02)00208-5
Li, Z. W., Liu, S. G., Chen, H. D., Liu, S., Guo, B., and Tian, X. B. (2008a). Structural Segmentation and Zonation and Differential Deformation across and along the Lomgmen Thrust Belt, West Sichuan, China. J. Chengdu Univ. Technol. Sci. Technol. Ed. 35, 440–454
Li, Z. X., Bogdanova, S. V., Collins, A. S., Davidson, A., De Waele, B., Ernst, R. E., et al. (2008b). Assembly, Configuration, and Break-Up History of Rodinia: a Synthesis. Precambrian Res. 160, 179–210. doi:10.1016/j.precamres.2007.04.021
Li, Z. X., Li, X. H., Wartho, J. A., Clark, C., Li, W. X., Zhang, C. L., et al. (2010b). Magmatic and Metamorphic Events during the Early Paleozoic Wuyi-Yunkai Orogeny, Southeastern South China: New Age Constraints and Pressure-Temperature Conditions. Geol. Soc. Am. Bull. 122, 772–793. doi:10.1130/b30021.1
Lin, G., Li, X., and Li, W. (2007). SHRIMP U-Pb Zircon Age, Geochemistry and Nd-Hf Isotope of Neoproterozoic Mafic Dyke Swarms in Western Sichuan: Petrogenesis and Tectonic Significance. Sci. China Ser. D. 50, 1–16. doi:10.1007/s11430-007-2018-0
Lin, L. B., Chen, H. D., Zhai, C. B., Hu, X. Q., and Li, Q. W. (2006). Sandstone Compositions and Paleogeographic Evolution of the Upper Triassic Xujiahe Formation in the Western Sichuan Basin, China. Petroleum Geol. Expeximent 28, 511–517. doi:10.11781/sysydz200606511
Liu, H. F. (1995). Classification of Foreland Basins and Fold Thrust Style. Front. Earth Sci. 2, 59–68.
Liu, Y., Hu, Z., Gao, S., Günther, D., Xu, J., Gao, C., et al. (2008). In Situ analysis of Major and Trace Elements of Anhydrous Minerals by LA-ICP-MS without Applying an Internal Standard. Chem. Geol. 257, 34–43. doi:10.1016/j.chemgeo.2008.08.004
Ludwig, K. R. (2003). ISOPLOT 3.00: A Geochronological Toolkit for Microsoft Excel. Berkeley: Berkeley Geochronology CenterCalifornia, 39.
Luo, L., Qi, J.-F., Zhang, M.-Z., Wang, K., and Han, Y.-Z. (2014). Detrital Zircon U-Pb Ages of Late Triassic-Late Jurassic Deposits in the Western and Northern Sichuan Basin Margin: Constraints on the Foreland Basin Provenance and Tectonic Implications. Int. J. Earth Sci. Geol. Rundsch) 103, 1553–1568. doi:10.1007/s00531-014-1032-7
Ma, Y. W., Wang, G.Z., and Hu, X.W.(1996).Tectonic Deformation of Pengguan Complex as a Nappe.2, 110–114.
Ma, Y. S., Chen, H. D., and Wang, G. L. (2009). Sequence Stratigraphy and Palaeo Geography of South China. Beijing: Science Press, 116–152.
Meng, E., Liu, F.-L., Du, L.-L., Liu, P.-H., and Liu, J.-H. (2015). Petrogenesis and Tectonic Significance of the Baoxing Granitic and Mafic Intrusions, Southwestern China: Evidence from Zircon U-Pb Dating and Lu-Hf Isotopes, and Whole-Rock Geochemistry. Gondwana Res. 28, 800–815. doi:10.1016/j.gr.2014.07.003
Meng, Q.-R., Wang, E., and Hu, J.-M. (2005). Mesozoic Sedimentary Evolution of the Northwest Sichuan Basin: Implication for Continued Clockwise Rotation of the South China Block. Geol. Soc. Am. Bull. 117, 396–410. doi:10.1130/b25407.1
Pan, G. T., Wang, L. Q., and Li, Z. X. (2001). The Tectonic Framework and Spatial Allocation of the Archipelagic Arc-Basin Systems on the Qinghai-Xizang Plateau. Sediment. Geol. Tethyan Geol. 21, 1–26.
Paul, W. O. H., and Urs, S. (2003). The Composition of Zircon and Igneous and Metamorphic Petrogenesis. Rev. Mineralogy Geochem. 53, 27–62. doi:10.2113/0530027
Peter-Jon, A., Waddell Nicholas, E., Timms Catherine, V., Spaggiari Christopher, L., Kirkland Michael, T. D., et al. (2015). Analysis of the Ragged Basin, Western Australia: Insights into Syn-Orogenic Basin Evolution within the Albany-Fraser Orogen. Precambrian Res. 261, 166–187. doi:10.1016/j.precamres.2015.02.010
Pullen, A., Kapp, P., Gehrels, G. E., Ding, L., and Zhang, Q. (2010). Metamorphic Rocks in Central Tibet: Lateral Variations and Implications for Crustal Structure. Geol. Soc. Am. Bull. 123, 585–600. doi:10.1130/b30154.1
Ren, J., Wang, Z., Chen, B., Jiang, C. F., and Niu, B. G. (1997). Tectonic Map of China and Adjacent Regions. Beijing: Geological Publishing House.
Roger, F., Jolivet, M., and Malavieille, J. (2010). The Tectonic Evolution of the Songpan-Garzê (North Tibet) and Adjacent Areas from Proterozoic to Present: A Synthesis. J. Asian Earth Sci. 39, 254–269. doi:10.1016/j.jseaes.2010.03.008
Rubatto, D., and Gebauer, D. (2000). Use of Cathodoluminescence for U-Pb Zircon Dating by Ion Microprobe: Some Examples from the Western Alps. Cathodoluminescence Geosciences 978, 373–400. doi:10.1007/978-3-662-04086-7_15
Saunders, A. D., England, R. W., Reichow, M. K., and White, R. V. (2005). A Mantle Plume Origin for the Siberian Traps: Uplift and Extension in the West Siberian Basin, Russia. Lithos 79, 407–424. doi:10.1016/j.lithos.2004.09.010
Shao, T., Cheng, N., and Song, M. (2016). Provenance and Tectonic-Paleogeographic Evolution: Constraints from Detrital Zircon U-Pb Ages of Late Triassic-Early Jurassic Deposits in the Northern Sichuan Basin, Central China. J. Asian Earth Sci. 127, 12–31. doi:10.1016/j.jseaes.2016.05.027
Shi, Y., Yu, J.-H., and Santosh, M. (2013). Tectonic Evolution of the Qinling Orogenic Belt, Central China: New Evidence from Geochemical, Zircon U-Pb Geochronology and Hf Isotopes. Precambrian Res. 231, 19–60. doi:10.1016/j.precamres.2013.03.001
Sun, W.-H., Zhou, M.-F., Gao, J.-F., Yang, Y.-H., Zhao, X.-F., and Zhao, J.-H. (2009). Detrital Zircon U-Pb Geochronological and Lu-Hf Isotopic Constraints on the Precambrian Magmatic and Crustal Evolution of the Western Yangtze Block, SW China. Precambrian Res. 172, 99–126. doi:10.1016/j.precamres.2009.03.010
Tang, Q., Li, C., Zhang, M., and Lin, Y. (2014). U-pb Age and Hf Isotopes of Zircon from Basaltic Andesite and Geochemical Fingerprinting of the Associated Picrites in the Emeishan Large Igneous Province, SW China. Min. Pet. 109, 103–114. doi:10.1007/s00710-014-0349-z
Tao, H. X. (1993). Historical Evolution of Tectonics in North Rim of Yangzi Plate. J. Earch Sci. Environ. 3, 75–76.
Veevers, J. J., Saeed, A., Belousova, E. A., and Griffin, W. L. (2005). U-pb Ages and Source Composition by Hf-Isotope and Trace-Element Analysis of Detrital Zircons in Permian Sandstone and Modern Sand from Southwestern Australia and a Review of the Paleogeographical and Denudational History of the Yilgarn Craton. Earth-Science Rev. 68, 245–279. doi:10.1016/j.earscirev.2004.05.005
Wang, B.-Q., Wang, W., Chen, W. T., Gao, J.-F., Zhao, X.-F., Yan, D.-P., et al. (2013). Constraints of Detrital Zircon U-Pb Ages and Hf Isotopes on the Provenance of the Triassic Yidun Group and Tectonic Evolution of the Yidun Terrane, Eastern Tibet. Sediment. Geol. 289, 74–98. doi:10.1016/j.sedgeo.2013.02.005
Wang, H. L., Xiao, S. W., Xu, X. Y., Li, W. Z., He, S. P., Chen, J. L., et al. (2008). Geochronology and Significance of the Early Mesoproterozoic Tectono-Magmatic Event in the Western Segment of the North Qinling Mountains, China. Geol. Bulletion China 10, 1728–1738.
Weislogel, A. L. (2006). The Sedimentary Record of Mesozoic Collisional Orogenesis, Central China: Geochemical, Geochronometry and Paleoenvironmental Constraints. Stanford: Stanford University, 362.
Weislogel, A. L., Graham, S. A., and Chang, E. Z. (2010). Detrital Zircon Provenance Fromthree Turbidite Depocenters of the Middle-Upper Triassic Songpan-Ganzi Complex, Central China: Record of Collisional Tectonics, Erosional Exhumation, and Sediment Production. Geol. Soc. Am. Bull. 122, 2041–2062. doi:10.1130/B26606.1
Wu, C., Yin, A., Zuza, A. V., Zhang, J., Liu, W., and Ding, L. (2016). Pre-Cenozoic Geologic History of the Central and Northern Tibetan Plateau and the Role of Wilson Cycles in Constructing the Tethyan Orogenic System. Lithosphere 8, 254–292. doi:10.1130/l494.1
Xiao, A. C., Wei, G. Q., Shen, Z. Y., Wang, L., Yang, W., and Qian, J, F. (2011). Basin-Mountain System and Tectonic Coupling between Yangtze Block and South Qinling Orogen. Acta Petrol. Sin. 3, 601–611. doi:10.2110/jsr.2011.25
Xu, C., Zhou, Z., Chang, Y., and Guillot, F. (2010). Genesis of Daba Arcuate Structural Belt Related to Adjacent Basement Upheavals: Constraints from Fission-Track and (U-Th)/He Thermochronology. Sci. China Earth Sci. 53, 1634–1646. doi:10.1007/s11430-010-4112-y
Xu, Y., Chung, S.-L., Jahn, B.-m., and Wu, G. (2001). Petrologic and Geochemical Constraints on the Petrogenesis of Permian-Triassic Emeishan Flood Basalts in Southwestern China. Lithos 58, 145–168. doi:10.1016/s0024-4937(01)00055-x
Xu, Z. Q., Hou, l. W., and Wang, Z. X. (1992). Orogenic Processes of the Songpan-Garze Orogenic Belt of China. Beijing: Geological Publishing House, 1–190.
Xu, Z. Q., Yang, J. S., Li, W. C., Li, H. Q., Cai, Z. H., Yan, Z., et al. (2013). Paleo-Tethys Systemand Accretionary Orogen in the Tibet Plateau. Acta Petrol. Sin. 29, 1847–1860. doi:10.3724/sp.j.1260.2013.20161
Xu, Y. G., Luo, Z. Y., Huang, X. L., He, B., Xiao, L., Xie, L. W., et al. (2008). Zircon u–pb and hf Isotope Constraints on Crustal Melting Associated With the Emeishan Mantle Plume. Geochimica Et Cosmochimica Acta, 72(13), 3084–3104. doi:10.1016/j.gca.2008.04.019
Yan, D., Zhou, M., Li, S., and Wei, G. (2011). Structural and Geochronological Constraints on the Mesozoic‐Cenozoic Tectonic Evolution of the Longmen Shan Thrust Belt, Eastern Tibetan Plateau. Tectonics 30. doi:10.1029/2011tc002867
Yan, D., Zhou, M., Wei, G., Liu, H., Dong, T., Zhang, W., and Jin, Z. (2008). Collapse of Songpan-Garzê Orogenic Belt Resulted from Mesozoic Middle-crustal Ductile Channel Flow: Evidences from Deformation and Metamorphism Within Sinian-Paleozoic Strata in Hinterland of Longmenshan Foreland Thrust Belt. Earth Science Frontiers, 15, 186–198. doi:10.1016/S1872-5791(08)60066-4
Yan, Z., Dong, S., Li, Y., Chen, A., Shao, C., and Zhao, S. (2019).Detrital Zircon U-Pb Geochronology of the Triassic Sandstones from the Yanyuan Basin of Southwestern Sichuan, China.Acta Geologica Sinica (English Edition), 93(6), 1974–1975. doi:10.1111/1755-6724.13844
Yan, Z., Tian, Y., Li, R., Vermeesch, P., Sun, X., Li, Y., et al. (2018). Late Triassic Tectonic Inversion in the Upper Yangtze Block: Insights from Detrital Zircon U-Pb Geochronology from South-Western Sichuan Basin. Basin Res. 31, 92–113. doi:10.1111/bre.12310
Yin, A., and Nie, S. (1993). An Indentation Model for the North and South China Collision and the Development of the Tan-Lu and Honam Fault Systems, Eastern Asia. Tectonics 12, 801–813. doi:10.1029/93tc00313
Yu, S.-Y., Song, X.-Y., Chen, L.-M., and Li, X.-B. (2014). Postdated Melting of Subcontinental Lithospheric Mantle by the Emeishan Mantle Plume: Evidence from the Anyi Intrusion, Yunnan, SW China. Ore Geol. Rev. 57, 560–573. doi:10.1016/j.oregeorev.2013.08.006
Yu, S. H., and Liang, X. Q. (2016). Provenance and its Geological Implication for the Upper Triassic Xujiahe Formation in Western Sichuan Basin. Univ. Chin. Acad. Sci. Inst. Geochem. 55, 1–92. doi:10.1139/cjes-2017-0092
Yuan, C., Zhou, M.-F., Sun, M., Zhao, Y., Wilde, S., Long, X., et al. (2010a). Triassic Granitoids in the Eastern Songpan Ganzi Fold Belt, SW China: Magmatic Response to Geodynamics of the Deep Lithosphere. Earth Planet. Sci. Lett. 290, 481–492. doi:10.1016/j.epsl.2010.01.005
Yuan, Q. D., Li, B. L., Liu, H. T., Liu, S. J., and Wang, L. (2010b). The Tectonics Evolution and Lithofacies Palaeogeography in the Northwest of the Sichuan Basin. J. Daqing Petroleum Inst. 34, 37–41. doi:10.1016/S1876-3804(19)60225-9
Zhang, F.-Q., Chen, H.-L., Batt, G. E., Dilek, Y., A, M. N., A, M.-D., et al. (2015). Detrital Zircon U-Pb Geochronology and Stratigraphy of the Cretaceous Sanjiang Basin in NE China: Provenance Record of an Abrupt Tectonic Switch in the Mode and Nature of the NE Asian Continental Margin Evolution. Tectonophysics 665, 58–78. doi:10.1016/j.tecto.2015.09.028
Zhang, G. W., Zhang, B. R., Yuan, X. C., and Xiao, Q. H. (2001). Qinling Orogenic Belt and Continental Dynamics. Beijing: Science Press, 1–855.
Zhang, J., Li, G. H., Xie, J. R., Qiu, J., Wei, X. W., and Tang, D. H. (2006). Stratigraphic Division and Correlation of Upper Triassic in Sichuan Basin. Nat. Gas. Ind. 26, 12–15. doi:10.1016/S1876-3804(12)60097-4
Zhang, L.-Y., Ding, L., Pullen, A., Xu, Q., Liu, D.-L., Cai, F.-L., et al. (2014). Age and Geochemistry of Western Hoh-Xil-Songpan-Ganzi Granitoids, Northern Tibet: Implications for the Mesozoic Closure of the Paleo-Tethys Ocean. Lithos 190-191, 328–348. doi:10.1016/j.lithos.2013.12.019
Zhang, Y.-X., Tang, X.-C., Zhang, K.-J., Zeng, L., Zeng, L., and Gao, C.-L. (2014). U-pb and Lu-Hf Isotope Systematics of Detrital Zircons from the Songpan-Ganzi Triassic Flysch, NE Tibetan Plateau: Implications for Provenance and Crustal Growth. Int. Geol. Rev. 56, 29–56. doi:10.1080/00206814.2013.818754
Zhang, Y., Jia, D., Shen, L., Yin, H., Chen, Z., Li, H., et al. (2015). Provenance of Detrital Zircons in the Late Triassic Sichuan Foreland Basin: Constraints on the Evolution of the Qinling Orogen and Longmen Shan Thrust-fold Belt in Central China. Int. Geol. Rev. 57, 1806–1824. doi:10.1080/00206814.2015.1027967
Zhang, Y. K. (2013). Study on Provenance Analysis. Ground water 35, 174–187. doi:10.3724/sp.j.1218.2013.00657
Zhang, Y. L., Wang, Z. Q., Wang, G., Li, Q., and Lin, J. F. (2016). Chromian Spinel, Zircon Age Constraints on the Provenance of Early Triassic Feixianguan Formation Sandstones from Huize Area, Upper Yangtze Region. Geol. Rev. 62 (1), 54–68. doi:10.16509/j.georeview.2016.01.005
Zhang, Y. P., Chen, X. H., Shao, Z. G., Zhang, J., Zuza, A. V., Li, B., et al. (2022). Ocean–continent Transition of the Northeastern Paleotethys during the Triassic: Constraints from Triassic Sedimentary Successions across the Qinling Orogen, Central China. J. Asian Earth Sci. 232, 1–18. doi:10.1016/j.jseaes.2022.105264
Zhao, X., and Coe, R. S. (1987). Palaeomagnetic Constraints on the Collision and Rotation of North and South China. Nature 327, 141–144. doi:10.1038/327141a0
Zheng, Y., Li, H., Sun, Z., Wang, H., Zhang, J., Li, C., et al. (2016). New Geochronology Constraints on Timing and Depth of the Ancient Earthquakes along the Longmen Shan Fault Belt, Eastern Tibet. Tectonics 35, 2781–2806. doi:10.1002/2016tc004210
Zhang, S., and Zheng, Y. (2013). Formation and Evolution of Precambrian Continental Lithosphere in South China. Gondwana Res., 23, 1241–1260. doi:10.1016/J.GR.2012.09.005
Zhong, Y.-T., He, B., Mundil, R., and Xu, Y.-G. (2014). CA-TIMS Zircon U-Pb Dating of Felsic Ignimbrite from the Binchuan Section: Implications for the Termination Age of Emeishan Large Igneous Province. Lithos 204, 14–19. doi:10.1016/j.lithos.2014.03.005
Zhou, M.-F., Yan, D.-P., Kennedy, A. K., Li, Y., and Ding, J. (2002). SHRIMP U-Pb Zircon Geochronological and Geochemical Evidence for Neoproterozoic Arc-Magmatism along the Western Margin of the Yangtze Block, South China. Earth Planet. Sci. Lett. 196, 51–67. doi:10.1016/s0012-821x(01)00595-7
Zhou, M. F., Yan, D. P., Kennedy, A. K., Li, Y. Q., and Ding, J. (2002). SHRIMP Zircon U-Pb Geochronological and Whole-Rock Geochemical Evidence for an Early Neoproterozoic Sibaoan Magmatic Arc along the Southeastern Margin of the Yangtze Block, South China. Gondwana Res. 12, 144–156. doi:10.1016/j.gr.2006.09.001
Zhou, M., Ma, Y., Yan, D., Xia, X., Zhao, J., and Sun, M. (2006). The Yanbian Terrane (Southern Sichuan Province, SW China): A Neoproterozoic Arc Assemblage in the Western Margin of the Yangtze Block. Precambrian Res. 144, 19–38. doi:10.1016/j.precamres.2005.11.002
Zhu, M., Chen, H. L., Zhou, J., and Yang, S. F. (2016). Provenance of Early Triassic in Yanyuan Basin, Upper Yangtze and its Implication for the Tectonic Evolution. J. Earth Sci. 41 (8), 1309–1321. doi:10.3799/dqkx.2016.106
Zhu, M., Chen, H., Yu, L., Zhou, J., and Yang, S. (2018). Provenance of the Early Triassic in the Southwestern Sichuan Basin, Upper Yangtze, and its Implications for Tectonic Evolution. Can. J. Earth Sci. 55 (1), 70–83. doi:10.1139/cjes-2017-0092
Zhu, M., Chen, H., Zhou, J., and Yang, S. (2017). Provenance Change from the Middle to Late Triassic of the Southwestern Sichuan Basin, Southwest China: Constraints from the Sedimentary Record and its Tectonic Significance. Tectonophysics 700-701, 92–107. doi:10.1016/j.tecto.2017.02.006
Zhu, X.-Y., Chen, F., Li, S.-Q., Yang, Y.-Z., Nie, H., Siebel, W., et al. (2011). Crustal Evolution of the North Qinling Terrain of the Qinling Orogen, China: Evidence from Detrital Zircon U-Pb Ages and Hf Isotopic Composition. Gondwana Res. 20, 194–204. doi:10.1016/j.gr.2010.12.009
Zhu, X. Y. (2010). Evolution and Provenance of the Basement in the Eastern Qinling Orogen, China: Evidence from Geochemistry and Zircon U‒Pb Geochronology Ph.D. Thesis. Beijing: Graduate University of Chinese Academy of Science, 1–138. (in Chinese with English abstract).
Zong, K., Klemd, R., Yuan, Y., He, Z., Guo, J., Shi, X., et al. (2017). The Assembly of Rodinia: The Correlation of Early Neoproterozoic (Ca. 900 Ma) High-Grade Metamorphism and Continental Arc Formation in the Southern Beishan Orogen, Southern Central Asian Orogenic Belt (CAOB). Precambrian Res. 290, 32–48. doi:10.1016/j.precamres.2016.12.010
Keywords: Northwestern Sichuan Basin, detrital zircon, provenance, U–Pb dating, sandstone petrology, tectonic–paleogeographic evolution
Citation: Meng L, Chen W, Shen T and Cai J (2022) A Study on the Provenance of Early to Late Triassic Clastic Rocks From the Northwestern Sichuan Basin, Southwestern China: Constraints on the Early Mesozoic Tectonic Evolution of the Western Yangtze Block. Front. Earth Sci. 10:940301. doi: 10.3389/feart.2022.940301
Received: 10 May 2022; Accepted: 17 June 2022;
Published: 05 August 2022.
Edited by:
Hanlin Chen, Zhejiang University, ChinaReviewed by:
Wei Yang, China University of Petroleum, Beijing, ChinaDong Jia, Nanjing University, China
Copyright © 2022 Meng, Chen, Shen and Cai. This is an open-access article distributed under the terms of the Creative Commons Attribution License (CC BY). The use, distribution or reproduction in other forums is permitted, provided the original author(s) and the copyright owner(s) are credited and that the original publication in this journal is cited, in accordance with accepted academic practice. No use, distribution or reproduction is permitted which does not comply with these terms.
*Correspondence: Jinfa Cai, hzcaijf@zjut.edu.cn