- Key Laboratory of Western China’s Environmental Systems (Ministry of Education), College of Earth and Environmental Sciences, Lanzhou University, Lanzhou, China
The loess deposits widely distributed in the eastern Tibetan Plateau (ETP) are important archives for reconstructing the paleoenvironmental changes and dust transportation history of the Tibetan Plateau. A lack of sufficient investigations on the source of the ETP loess has limited our understanding of dust transportation and paleoenvironmental implications. Quartz luminescence sensitivity holds great potential for tracing eolian dust sources. Numerous quartz luminescence sensitivity investigations have provided new insights into the dust sources and transportation of the Chinese loess. However, studies on luminescence sensitivity and its significance for the ETP loess provenance are scarce. In this work, we selected quartz grains in loess from different sites of the ETP to investigate the variation of luminescence sensitivity by testing the 110°C thermoluminescence (TL) peak and optically stimulated luminescence (OSL) sensitivities of quartz grains and discussed the provenance implications for the ETP loess based on quartz luminescence sensitivities. Our results show the quartz luminescence sensitivities of loess at different locations were significantly different in the ETP. The luminescence sensitivity values of the Machang (MC), Xiaojin (XJ), and Wenchuan (WCH) sections were much higher than those of the Xinshi (XS) and Zhouqu (ZQ) sections. The sensitivity values of the 110°C TL peaks and OSL in the paleosol and loess layers show distinct differences. The quartz luminescence sensitivities of the ETP loess are mainly influenced by the dust sources. Our results show that the luminescence sensitivities of quartz grains have great potential for identifying the loess sources in the ETP.
1 Introduction
The loess-paleosol sequence contains abundant information about past environmental changes and is of great significance for past climatic and environmental reconstruction (Liu, 1985; An et al., 1991). Eolian loess is widely distributed in the eastern Tibetan Plateau (ETP), which is one of the most direct and sensitive sedimentary records for studying past environmental changes on the Tibetan Plateau (TP), and has received increasing attention in recent years (Fang et al., 1996; Pan and Wang, 1999; Chen et al., 2022; Yang et al., 2022). The high–altitude loess varies significantly in terms of the origin of the material, transport processes, and depositional environments, and systematic studies of their material sources and transport mechanisms can contribute to an in-depth understanding of the mechanisms and effects of plateau dust cycle processes, atmospheric circulation patterns, and regional environmental changes (Fang et al., 1996; Jiang et al., 1997; Wang et al., 2005; Yang et al., 2021b). However, the loess sources and transportation processes of the ETP have not been fully studied compared to the loess in the Chinese Loess Plateau (CLP) (e.g., Li et al., 2015; Sun et al., 2007; Zhang et al., 2021). Some studies have suggested that the deserts of northwestern China are the potential dust source areas for ETP loess (Tafel, 1914; Ma, 1944; Liu, 1983), and the dust from the Tarim and Qaidam Basins can contribute to the northern TP (Huang et al., 2007; Xu et al., 2015). Whereas, other studies have proposed that the ETP loess is mainly sourced from the inner TP, and local sediments and glacial activities have a great influence on the ETP loess (Fang, 1994; Chen et al., 1997; Wang and Pan, 1997; Yang et al., 2021b; Ling et al., 2022). Thus, the large uncertainty in the source of the Tibetan loess has hindered further understanding of the dust transport dynamics and paleoenvironmental reconstruction in the Tibetan Plateau (Fang, 1994; Sun et al., 2007; Dong et al., 2017).
Luminescence holds unique potential as a sediment tracer and provenance tool (Sawakuchi et al., 2018; Gray et al., 2019). A common approach in source tracing using luminescence is to examine the luminescence sensitivity of quartz grains to source lithology and transport history (Sawakuchi et al., 2012; Zular et al., 2015; Sawakuchi et al., 2018). The luminescence sensitivity is defined as the luminescence intensity per unit dose per unit mass (Gy−1 kg−1) (Wintle and Murray, 1999). In nature, there are noticeable differences in the luminescence sensitivity of quartz grains from various depositional environments, and these differences may contain important information about the source area and transport processes. The luminescence sensitivity of quartz grains from different deserts in northern China has significant regional differences (Li et al., 2007; Zheng et al., 2009; Lü and Sun, 2011), implying different source areas of the eolian deposits (Lü and Sun, 2011). Lai and Wintle (2006) suggested that the optically stimulated luminescence (OSL) sensitivity of quartz grains in paleosols was greater than that of the loess layer during the Pleistocene and Holocene transitions at the Luochuan section, which can be ascribed to different provenances. Further investigation suggested that the variation of quartz origins during the glacial-interglacial cycle has a major influence on the luminescence sensitivity (Lü et al., 2014).
The cited studies show the great potential for tracing loess sources by using luminescence sensitivity. However, studies of the ETP loess luminescence sensitivity are lacking. Therefore, we first conduct a detailed investigation of the quartz luminescence sensitivity to examine the temporal and spatial variations of the ETP loess, discuss the factors affecting the change in luminescence sensitivity of quartz grains, and, finally, explore the dust source implications in the ETP based on the luminescence sensitivity of quartz. Our results contribute new insights into the dust source of the ETP loess.
2 Material and methods
2.1 Sampling
The ETP is the transition zone from the Tibetan Plateau to the Sichuan Basin and the CLP, located at roughly 97–103°E and 25–37°N, with an average elevation between 2,500 m and 4,000 m above sea level (Figure 1). The aeolian loess of the ETP is mainly deposited in piedmonts, faulted basins, and river terraces, with varying thicknesses from several to tens of meters (Fang et al., 1996). The climate system is mainly influenced by the Westerlies, the Indian monsoon, and the Tibetan Plateau monsoon (Pan and Wang, 1999; Fang et al., 2004), with distinct dry and wet season differences.
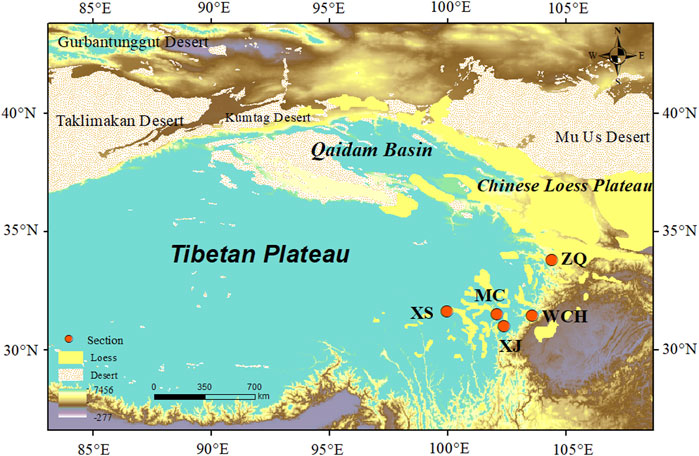
FIGURE 1. Loess distribution and the locations of the study site and sampling profiles (XS, Xinshi; MC, Machang; WCH, Wenchuan; XJ, Xiaojin; ZQ, Zhouqu). (Loess distribution after Liu, 1985; Yang et al., 2010; Muhs, 2013).
A total of 20 luminescence sensitivity samples were collected from five typical loess-paleosol sequences in the ETP (Figure 1), including the Xinshi (XS) (Yang et al., 2022), Machang (MC), Xiaojin (XJ), Wenchuan (WCH) (Liu et al., 2021), and Zhouqu (ZQ) profiles (Yang et al., 2020). The lithologies, magnetic susceptibility (χlf) curves, and OSL dating data for all profiles studied are shown in Figure 2. The XS section (31.62°N, 99.98°E, 3,400 m) is located in the western part of the ETP, near Ganzi County. It is situated on the third terrace of the Yalong River (Yang et al., 2022). Field investigations, magnetic susceptibility (χlf) curves, and luminescence dating results show that the sequence has been deposited since the Last Interglacial, including three paleosols S1, L1S1, and S0 (Yang et al., 2021a). The MC and XJ sections are in the center of the ETP. The MC section (31.50°N, 102.08°E, 2,480 m) is situated on the sixth terrace on the east bank of the Dadu River, near Jinchuan County. In this study, the upper 5 m is selected for the luminescence sensitivity study. Based on the OSL ages, magnetic susceptibilities (χlf), and the field investigations, this section can be divided into S1, L1, and S0. The XJ section (30.99°N, 102.39°E, 3,191 m) is located on a high terrace of the Dadu River, near Xiaojin County, with a thickness of 6.6 m. According to the field observations, this profile has similar characteristics to the MC profile and can be divided into three units: S1, L1, and S0. The WCH section (31.45°N, 103.57°E, 1,630 m) and ZQ section (33.78° N, 104.40° E, 2,047 m) is located in the east and northeast of the ETP, respectively. Previous reports showed that WCH and ZQ sections have accumulated since approximately 60 ka (Yang et al., 2020; Liu et al., 2021). The stratigraphic units of WCH and ZQ sections can be identified by field investigations, OSL dating, and environmental proxies (Yang et al., 2020; Liu et al., 2021).
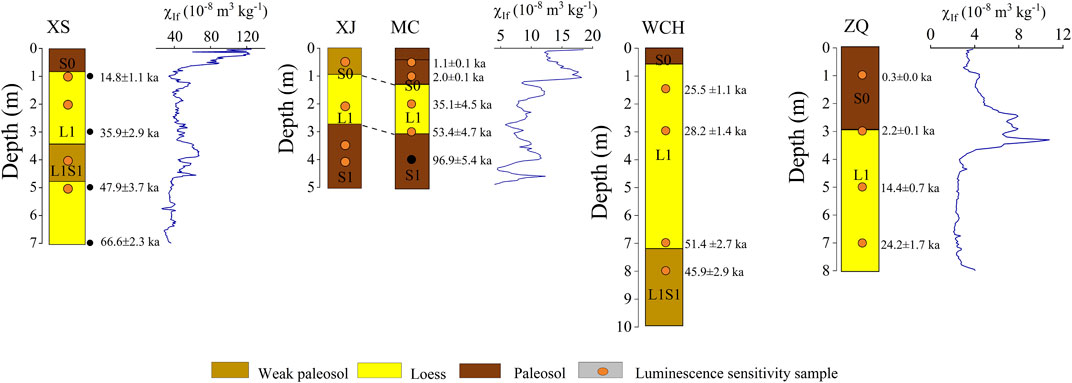
FIGURE 2. Lithology, optically stimulated luminescence (OSL) dating, and magnetic susceptibility (χlf) of the sampling profiles (L1, Malan loess; S, paleosol). The OSL dating results of the XS, MC, WCH and ZQ sections are taken from Yang et al. (2021a), Li (2021), Liu et al. (2021), and Yang et al. (2020), respectively. (XS, Xinshi, MC, Machang; WCH, Wenchuan; XJ, Xiaojin; ZQ, Zhouqu). The orange dots indicate the samples used in this study for the luminescence sensitivity study, and the black dots indicate the results for all OSL ages but not used in the luminescence sensitivity study.
2.2 Sample pretreatment
The luminescence sensitivity of quartz varies significantly with the grain size (Lü and Sun, 2011). For the same mass of quartz with different grain sizes, the finer grains will have a larger total surface area with greater luminescence sensitivities (Lü and Sun, 2011). To avoid the difference in luminescence sensitivities of quartz caused by different grain sizes, we selected 38–63 μm quartz grains for the luminescence sensitivity experiment.
The quartz purifications were performed under a subdued red lighting condition at a wavelength of ∼655 ± 30 nm. The outer 3–5 cm ends of the tube samples were first removed, and the remaining middle part was wet-sieved to obtain the 38–63 μm grain size fraction. Then the organic matter and carbonates were removed with 30% hydrogen peroxide (H2O2) and 10% dilute hydrochloric acid (HCl), followed by soaking in 35% fluorosilicic acid (H2SiF6) for approximately 2 weeks to remove the feldspar, then adding 1 mol/L HCl to remove the fluoride precipitate, until finally the purified quartz sample was obtained. The quartz purity is then tested by using the infrared stimulation (IRSL)/OSL ratio (Lai and Murray, 2006).
2.3 Luminescence sensitivities experiment
All the samples were analyzed for luminescence sensitivities at the Luminescence Dating Laboratory at the Key Laboratory of Western China’s Environmental Systems (Ministry of Education), Lanzhou University, using an automated Risø thermoluminescence (TL)/OSL DA-20 reader installed with a 90Sr/90Y β-radiation source. Blue LEDs (470 nm, ∼80 mW/cm2) on the TL/OSL reader stimulated the quartz OSL signal, which is detected using a photomultiplier tube and a corresponding 7.5 mm Hoya U-340 filter. To avoid the effect of differences in photomultiplier tubes between instruments on the luminescence sensitivity results, all samples were measured in the same instrument. In the quartz luminescence sensitivity experiment, the quartz grains adhered to an approximately 1-cm-diameter stainless steel disc with silicone oil, and all aliquots were weighed with a high-precision (readability of 0.1 mg) analytical electronic balance before and after the grains were placed on the disc for weighted normalization of the luminescence sensitivity signals.
The luminescence sensitivity experimental procedure is shown in Table 1. Ten aliquots of each sample were measured. The aliquots were first bleached at 125°C for 40 s to remove the natural OSL signals, then known laboratory beta irradiation doses (0 Gy, 2.58 Gy, 5.16 Gy, 10.32 Gy) were sequentially applied. Finally, the quartz grains are preheated at 260°C to test the TL signal and stimulated at 125°C for 40 s to obtain the OSL signal.
Data analysis: The dose-response curves were established with the four regenerative doses and the corresponding luminescence signal. The purpose of the regenerative dose of 0 Gy was to check the recuperation. The OSL signal integration of the first 0.32 s in the quartz decay curve minus the last 8 s of background for calculations employing a net OSL signal. The 110°C TL peak areas are derived from integration performed between 100 and 120°C with the dark count deducted. The luminescence sensitivity was calculated according to the method of Lü et al. (2014), and all OSL/TL signals were normalized by the weight of quartz gains and the irradiation dose (Figure 3). In our study, all dose-response curves were fitted with an exponential function, and the slope of the tangent line corresponding to the regenerative dose at 5.16 Gy on the growth curve was taken as the corresponding TL or optically stimulated luminescence (OSL) sensitivity.
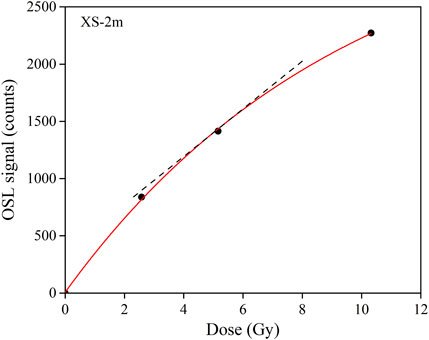
FIGURE 3. Response of regenerative doses (0 Gy, 2.58 Gy, 5.16 Gy, and 10.32 Gy) to the optically stimulated luminescence (OSL) signal for sample XS-2m. All dose-response curves were fitted with an exponential function, and the slope of the tangent line corresponding to the regenerative dose at 5.16 Gy on the growth curve was taken as the corresponding OSL sensitivity.
3 Results
3.1 Characteristics of quartz OSL and TL signals of the eastern Tibetan Plateau loess
Figure 4 illustrates that the OSL signal and 110°C TL peak signal intensity of different aliquots of the same sample vary significantly, showing a discrete distribution of the total data for each sample. The difference in signal intensity is even greater between samples. The OSL signal intensity of XS-1m in the XS profile is the weakest, with OSL signal distribution ranging between 500 counts and 1,000 counts; while the OSL signal intensity of XS-4m is obviously the highest (1,000–16000 counts). The internal difference of OSL signal intensity of XS-4m is the largest in comparison with other samples. The highest OSL intensity occurs in WCH-0.6m and WCH-3m, with the OSL signal varying between 3 × 104 and 8 × 104 counts. The OSL signal in WCH-7m is relatively weak, with overall values of <800 counts. The intensity of the OSL signal in the ZQ profile is lower than that in other profiles, ranging from 100 counts to 6,000 counts, and a lower quartz 110°C TL peak is presented compared to the other sections. The intensity of the 110°C TL peak also varies largely between aliquots of the same sample and for different samples. The 110°C TL peaks in the ZQ and XS profiles have the weakest intensities among all samples. This discrepancy can be ascribed to the number of quartz grains contributing to the luminescence signal in each measurement aliquot and the different sizes of the luminescence sensitivities of the quartz grains (Pietsch et al., 2008).
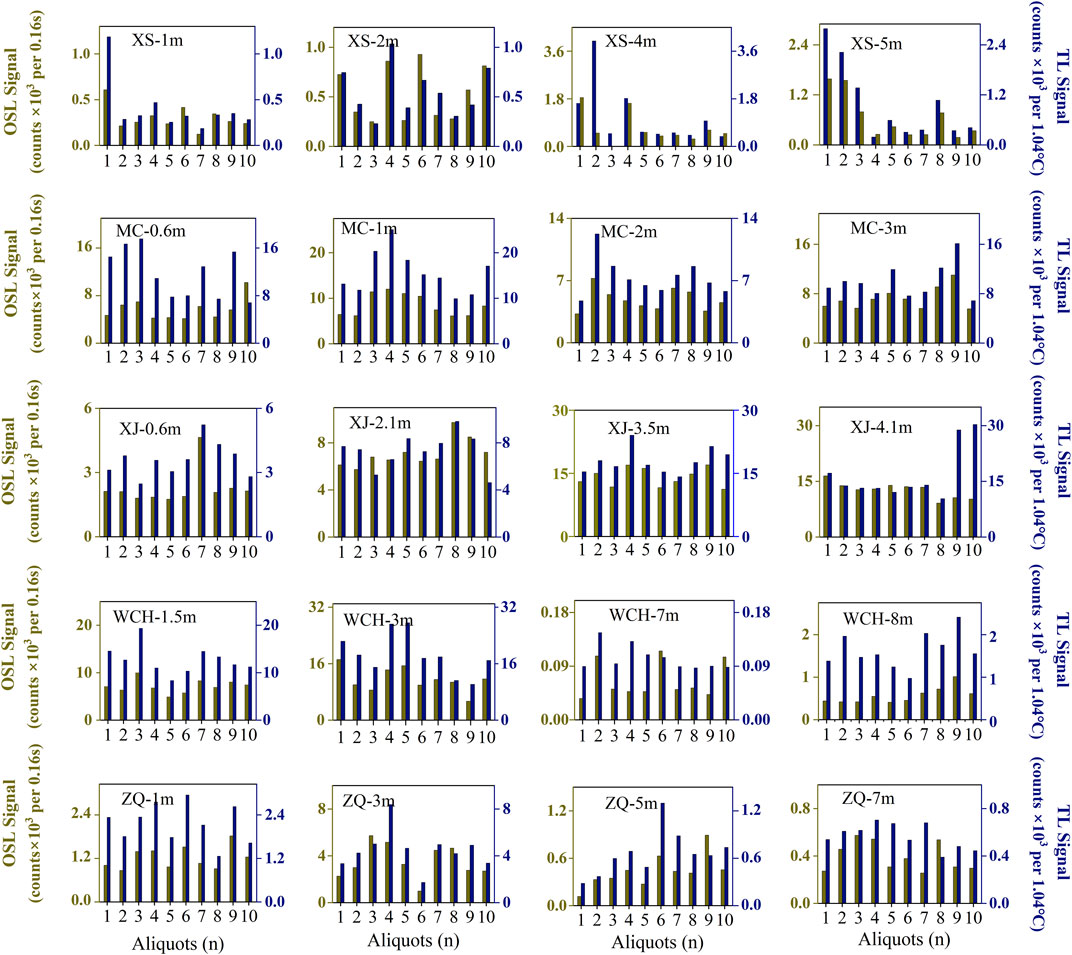
FIGURE 4. Histogram of optically stimulated luminescence (OSL) and 110°C thermoluminescence (TL) signal characteristics of all samples from the XS, MC, XJ, WCH, and ZQ sections. (XS, Xinshi; MC, Machang; WCH, Wenchuan; XJ, Xiaojin; ZQ, Zhouqu).
3.2 Spatial and temporal characteristics of luminescence sensitivity
The quartz grains from the ETP loess-paleosol sequence show a good characteristic of luminescence signals and strong luminescence sensitivity. Spatial variations of the quartz luminescence sensitivity for all L1 samples are plotted in Figure 7, which shows the significant spatial differences in the luminescence sensitivity for loess at different locations in the ETP. The highest OSL and TL sensitivity occurs in the XJ and WCH loess, especially the WCH loess (falling into a distant region at the upper right in Figure 7), with TL sensitivity values above 8×103 counts/Gy/mg, and OSL sensitivity values above 6 × 103 counts/Gy/mg, followed by those of the XJ and MC loess, while the quartz grains of loess from XS and ZQ has the relatively weakest luminescence sensitivity. The OSL and TL sensitivity values of the XS loess are concentrated around 600 counts/Gy/mg and 530 counts/Gy/mg, respectively. And the OSL and TL sensitivity values of ZQ are distributed around 80 counts/Gy/mg and 200 counts/Gy/mg, respectively. Moreover, some overlap can be seen between the sensitivity values of XS samples and those of the MC and XJ sections (Figure 7).
Our results show a distinctive difference in the TL and OSL sensitivities of quartz from paleosol and loess layers at different sites in the ETP (Figure 5). The temporal variations of luminescence sensitivities in the XJ and WCH profiles are obviously higher than those of the other profiles. The higher sensitivity values in the XS and MC sections occurred in the S0 and L1S1 paleosol layers, and the Malan loess (L1) displays a lower luminescence sensitivity; whereas, the Malan loess of the XJ and WCH sections has significantly higher values than the paleosol layer. In addition, the differences between the loess and the Holocene soils (S0) are large compared with the sensitivity values of the weak paleosol (L1S1) in the ETP. The luminescence sensitivity values of the Holocene soil are slightly higher than that of the L1 in the MC and ZQ sections.
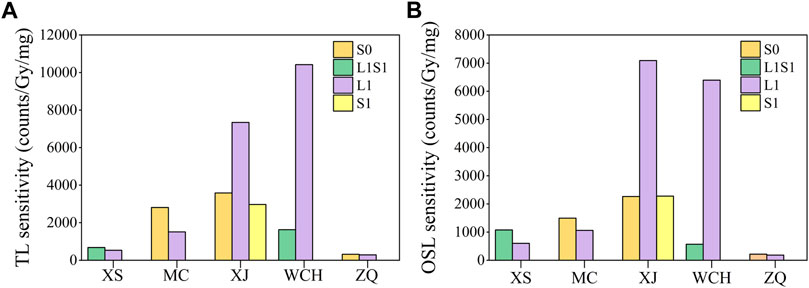
FIGURE 5. Variation of 110°C thermoluminescence (TL) peak (A) and optically stimulated luminescence (OSL) sensitivity (B) of quartz grains from loess-paleosol layers at different sites (locations shown in Figure 1). (XS, Xinshi; MC, Machang; WCH, Wenchuan,; XJ, Xiaojin; ZQ, Zhouqu).
4 Discussion
4.1 Comparison of luminescence sensitivity with CLP loess
The OSL sensitivity comparison between the CLP and ETP loess is shown in Figure 6. In our study, the lowest OSL sensitivity of the L1 loess (approximately 215 counts/Gy/mg) appears in the ZQ section. The OSL sensitivity values of the XS, MC, and XJ sections are approximately 600 counts/Gy/mg, 1,060 counts/Gy/mg, and 7,000 counts/Gy/mg in the L1 loess accumulation period. The highest sensitivity value of approximately 9,310 counts/Gy/mg appears in the WCH profile in the L1 layer on the ETP. The Shimao section from the northern CLP has an OSL sensitivity for quartz grains (38–63 μm) of less than 100 counts/Gy/mg during the L1 loess accumulation period (Lü et al., 2021), while the OSL sensitivities of the L1 loess layer at Jingyuan (the particle size fraction used is 45–63 μm) and Luochuan (25–64 μm) are approximately 950 counts/Gy/mg and 1,230 counts/Gy/mg, respectively (Zhang, 2018). An OSL sensitivity value of approximately 333 counts/Gy/mg (38–64 μm) for the L1 was measured in the Xifeng profile (Li and Yang, 2022). In summary, the OSL sensitivity values of medium fraction quartz grains (38–63 μm) of TP loess were generally higher than those of quartz grains of the L1 layers of the CLP. The maximum OSL sensitivity of the ETP loess is 90 times the sensitivity values observed in the CLP, which implies that the dust sources may be different between the ETP and the CLP.
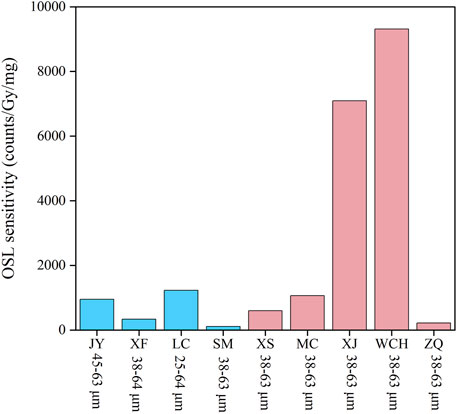
FIGURE 6. Comparison of optically stimulated luminescence (OSL) sensitivity observed for the L1 layer in the Chinese Loess Plateau and eastern Tibetan Plateau (JY, Jingyuan (Zhang, 2018), XF, Xifeng (Li and Yang, 2022); LC, Luochuan (Zhang, 2018), SM, Shimao (Lü et al., 2021); XS, Xinshi; MC, Machang; XJ, Xiaojing, WCH, Wenchuan, and ZQ, Zhouqu).
4.2 Factors affecting spatiotemporal luminescence sensitivity changes
The quartz luminescence sensitivity of sediment grains from different depositional environments is mainly influenced by the parent rock and transportation process (i.e., irradiation/bleaching cycles) (Pietsch et al., 2008; Fitzsimmons, 2011; Sawakuchi et al., 2018). Other factors such as heating histories, regional climate change, and soil-forming process potentially may also contribute to quartz luminescence sensitivity (Juyal et al., 2009; Rengers et al., 2017; Sawakuchi et al., 2018). In addition, variations in quartz grain size have significant effects on luminescence sensitivity. In general, the sensitivity values increase with smaller grain sizes (Li and Zhou, 2021; Li and Yang, 2022).
4.2.1 The effect of material source on luminescence sensitivity changes
Quartz grains are stable and weather resistant, so they retain significant information about their source rocks. Thus, they have the potential to identify the dust source regions (Sun and An, 2000; Rink et al., 2001; Qiao and Yang, 2006). In this study, the loess samples we selected are relatively young and have undergone relatively weak weathering processes. Generally, only silty to fine grains (<63 μm) can be transported long distances by wind suspension; sands or gravels can only be transported by saltation or creep (Pye, 1995). And the silt fraction greater than ∼40 μm is unaffected by the pedogenesis process (Wang et al., 2002). Therefore, the 38–63 μm quartz grains from the Malan loess samples were investigated because they are the main dust component of long-distance wind transmission, unaffected by pedogenesis, and carry information about the dust source (Fang, 1994; Yang et al., 2021b).
Our results show that the quartz luminescence sensitivities of loess samples from different locations in the ETP vary noticeably (Figure 7), suggesting that the quartz luminescence sensitivity may be used as a tool to track dust provenance. The dust in the ETP came from various deposits within the TP interior, including glacial–periglacial, alluvial/fluvial, lacustrine, aeolian, and slope deposits (Fang, 1994; Qiao et al., 2006; Ou et al., 2012; Yang et al., 2021b). The XS loess from the Ganzi Basin has a lower luminescence sensitivity, mainly because the XS loess is more affected by glacial–periglacial sediment content compared with other profiles (Fang, 1994), resulting in more quartz grains with relatively weak luminescence sensitivity. A certain overlap with the luminescence sensitivities of the XS, MC, and XJ loess samples occurs, which indicates that the source areas of these loess sections may be mixed. However, the luminescence sensitivity values of the MC and XJ loess sequences are significantly higher than those of the XS loess, which may indicate different dust sources in these sections compared to the XS profile. The highest luminescence sensitivity of the WCH loess, without overlap with other regions, shows a different source region with less contribution of dust materials produced by glacial activity. The luminescence sensitivity of the ZQ section is obviously different from that of other loess profiles on the Tibetan Plateau, but it is close to the luminescence sensitivity values of the CLP profiles (Zhang, 2018; Lü et al., 2021; Li and Yang, 2022). This may indicate that the ZQ loess has a similar dust source to the CLP loess. And, Fang et al. (1999) suggested that the loess of the West Qinling Mountains may mainly come from the neighboring northeastern Tibetan Plateau, so the “dim” source from North Tibetan Plateau may contribute to the lower luminescence sensitivities of the ZQ loess.
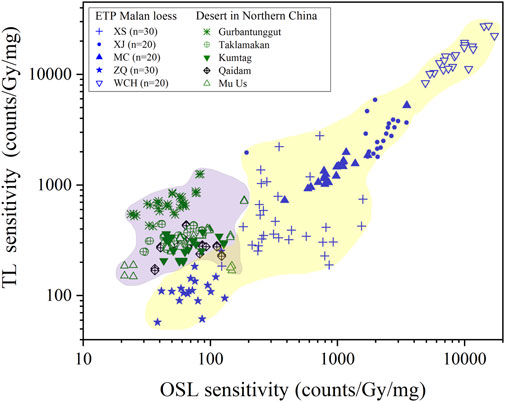
FIGURE 7. The 110°C thermoluminescence (TL) peak versus optically stimulated luminescence (OSL) sensitivity of quartz grains from the Malan loess (L1) in the eastern Tibetan Plateau and for the modern deserts in northwestern China (Lü and Sun, 2011). (XS, Xinshi; MC, Machang; WCH, Wenchuan; XJ, Xiaojin; ZQ, Zhouqu).
Significant temporal variations of luminescence sensitivity of quartz grains from loess-paleosol sequences have also occurred in the ETP. The higher sensitivity values in the XS, MC and ZQ sections occurred in the paleosol layers, and the glacial loess exhibits lower luminescence sensitivity; whereas, the Malan loess layers at XJ and WCH have significantly higher luminescence values than the paleosol layers (Figure 5), which can be mainly attributed to changes in the dust source area. Loess–paleosol sequences in the CLP have shown high luminescence sensitivity in paleosols and low luminescence sensitivity in loess layers, which is considered to be closely related to the sources of the quartz grains (Lai and Wintle, 2006; Lü et al., 2014; Lü et al., 2021).
4.2.2 Other factors affecting the luminescence sensitivity changes
Except for the material sources, other factors such as preburial transport history, heating history, and climate change also are suggested to have important influences on the quartz luminescence sensitivity changes.
The prior transport history, including the natural radiation/bleaching cycles from the source to the sink area, must also be considered as one of the factors affecting the luminescence sensitivity of quartz particles (Moska and Murray, 2006; Pietsch et al., 2008). In general, the more repeated irradiation–burial–exposure cycles, the higher the luminescence sensitivity of quartz grains (Pietsch et al., 2008). However, Liu et al. (2018) found that the luminescence sensitivities of quartz grains from Yellow River sediments were almost the same as those of quartz in the CLP, which indicates the limited impact of the transport–deposition process on luminescence sensitivity. The relationship between the total dose rate of quartz grains and the luminescence sensitivity is shown in Supplementary Figure S1 to investigate the effect of the natural irradiation process on luminescence sensitivity. The results showed a consistent trend between OSL and TL sensitivity, but no consistent change trend between the dose rate and luminescence sensitivity. The trends of OSL and TL sensitivity and total dose rate for the XS section were opposite to that of the luminescence sensitivity (Supplementary Figure S1A). Similar inconsistent variation patterns between luminescence sensitivity and dose rate also occur in the MC, XJ, WCH, and ZQ profiles. This pattern of irregular variations of the luminescence sensitivity and the total dose rate may suggest that luminescence sensitivity is less influenced by irradiation (Lü et al., 2021). In addition, dose rate does not show significant differences with depth in the ETP loess or the CLP loess (e.g., Yang et al., 2020; Lü et al., 2021; Li and Yang, 2022), therefore, we infer that irradiation is not the main factor influencing the quartz luminescence sensitivity of the ETP loess.
Wildfire heating also is suggested as a factor to change the luminescence sensitivity of quartz grains (Rengers et al., 2017). A significant increase in sensitivity values in the S1 layer of the Luochuan section is speculated to be related to wildfire events (Zhang, 2018; Zhang et al., 2022). Climate change can cause changes in the frequency and intensity of fires (Bowman et al., 2009). Microcharcoal preserved in soils is an ideal index and plays an irreplaceable role in understanding past fire histories (Patterson et al., 1987). The microcharcoal records of the XS section show that the large microcharcoal (>100 µm) contents are slightly lower in the L1S1 than in the loess layer (L1), indicating that local natural fire events are not important in the L1S1 stage (Tang et al., 2022). However, the higher sensitivity values in the XS section occurred in the L1S1 paleosol layer, and the lower sensitivity values occurred in the last glacial loess (L1). Therefore, we infer that wildfire heating was not the main factor in the increase of sensitivity values for the XS loess.
In our study, the differences in quartz luminescence sensitivities of the ETP loess and the CLP loess can be mainly attributed to different dust sources. The relatively high luminescence sensitivity of the XJ and WCH profiles can be ascribed to the brighter quartz grains from the source region, while the relatively low sensitivity values of the XS and ZQ loess profiles may be related to a different source area with more “dim” quartz grains that originated in glacial deposits. However, the transport process may have a relatively minor effect on the luminescence sensitivity of quartz grains (Liu et al., 2018). Recent studies on the loess-paleosol sequence in the CLP, such as at Xifeng (Li and Yang, 2022), Luochuan (Lü et al., 2014), and Shimao (Lü et al., 2021), showed that the OSL and TL sensitivities are consistent with glacial-interglacial variations. The similar variations between the quartz OSL/TL sensitivities and the climatic records (such as magnetic susceptibility and grain sizes) may imply luminescence sensitivity has a close relationship to climate changes (Lü et al., 2014; Lü et al., 2021; Li and Yang, 2022), but the causes and mechanisms of these similarities need further investigations.
4.3 Provenance implications for the eastern Tibetan Plateau loess based on quartz luminescence sensitivities
Numerous investigations have shown that the quartz luminescence sensitivity holds great potential for tracing eolian dust sources (Lü and Sun, 2011; Sawakuchi et al., 2018; Lü et al., 2021). From the analysis in Section 4.2, the difference in the luminescence sensitivities of ETP loess samples is thought to be a response to variations in the material sources. Figure 7 shows the significant spatial differences in the luminescence sensitivities for loess at different locations in the ETP. The quartz grains of XJ and WCH profiles exhibit the highest OSL and TL sensitivities, especially the XJ loess, followed by that of the MC loess, while the quartz grains from XS and ZQ have the relatively weakest luminescence sensitivity. In addition to the obvious spatial differences in luminescence sensitivities, there are also obvious vertical variations in the loess-paleosol profile, which are manifested as large differences in the sensitivity values between the loess and paleosol layers in different regions of the ETP (Figure 5). We infer that the spatial and temporal variations in quartz luminescence sensitivity may indicate internal differences in sources of the ETP loess.
The broad deserts in northwest China and the adjacent regions have been regarded as important sources of Chinese loess (Liu and Ding, 1998; Engelbrecht and Derbyshire, 2010), which may also potentially contribute to the ETP loess. However, our results show significant discrepancies in quartz luminescence sensitivities between the ETP loess and the deserts in northern China (including the Gurbantunggut, Taklimakan, Kumtag, Qaidam, and Mu Us) (Figure 7). The OSL sensitivities of quartz grains (90–125 μm fraction) in these deserts of northern China range from 40 counts/Gy/mg to 140 counts/Gy/mg, and the TL sensitivities range from 150 counts/Gy/mg to 1,260 counts/Gy/mg (Lü and Sun, 2011). The sensitivity values of the northern China deserts are close to those of the ZQ loess near the CLP, while they are significantly lower than those of the other Malan loess samples in the ETP (Figure 7). The significant differences in the luminescence sensitivity values may suggest that the northwestern deserts in China were not the main dust source of the ETP loess. It should be noted that due to limited data, we cannot compare the luminescence sensitivity with the same grain size for these two regions, which may introduce some uncertainties. Previous studies have shown that the ETP loess is mainly from the interior of the TP; the northwestern arid zone of the TP is not its main source (Fang, 1994; Yang et al., 2010; Yang et al., 2021b). Our results also show that the ETP loess was mainly sourced from the interior of the Tibetan Plateau, indicating the Tibetan Plateau is an important dust source area. The abundant dust materials derived from the vast west and central Tibetan Plateau can be transported by the westerlies and the plateau winter monsoon, and finally deposited in the ETP (Fang et al., 2004; Yang et al., 2021b). Due to the vast area of the Tibetan Plateau, with significant differences in topography and environment, more research is needed to precisely identify the dust source region of the ETP loess.
5 Conclusion
Based on the analysis and comparison of the luminescence sensitivities of sedimentary quartz from loess at different sites in the eastern Tibetan Plateau, the main conclusions are drawn as follows: The 110°C TL peaks and OSL sensitivities of quartz from different regions of the ETP loess vary widely, especially in the XJ, MC, and WCH samples, which are significantly higher than those of ZQ and XS. The luminescence sensitivity values of quartz are obviously different in loess and paleosol from different areas of the eastern Tibetan Plateau. The luminescence sensitivity of quartz in the ETP loess mainly reflects the variation of dust sources, and the luminescence sensitivity has the potential as an important tool for dust source tracing.
Data availability statement
The raw data supporting the conclusions of this article will be made available by the authors, without undue reservation.
Author contributions
SY and LL designed the study. SY, LL, and HW, carried out fieldwork and collected samples. XJ, LL, and HW performed laboratory analysis. XL, JZ, and PL performed data interpretations. S.Y. and LL wrote the manuscript. All authors discussed interpretations and commented on the manuscript.
Funding
This work was co-supported by the Second Tibetan Plateau Scientific Expedition and Research Program (2019QZKK0602), the National Natural Science Foundation of China (41877447 and 41472147), and the Strategic Priority Research Program of the Chinese Academy of Sciences (XDA20090000).
Acknowledgments
We are grateful for the helpful comments of the reviewers. We also thank Ting Cheng, Shuai Li, Minhao Liang, Dongxue Li, Yuanlong Luo, and Zixuan Chen for their assistance in field and laboratory work.
Conflict of interest
The authors declare that the research was conducted in the absence of any commercial or financial relationships that could be construed as a potential conflict of interest.
Publisher’s note
All claims expressed in this article are solely those of the authors and do not necessarily represent those of their affiliated organizations, or those of the publisher, the editors, and the reviewers. Any product that may be evaluated in this article, or claim that may be made by its manufacturer, is not guaranteed or endorsed by the publisher.
Supplementary material
The Supplementary Material for this article can be found online at: https://www.frontiersin.org/articles/10.3389/feart.2022.939638/full#supplementary-material
References
An, Z., Kukla, G., Porter, S. C., and Xiao, J. (1991). Late quaternary dust flow on the Chinese Loess Plateau. Catena 18, 125–132. doi:10.1016/0341-8162(91)90012-m
Bailey, R. M., Smith, B. W., and Rhodes, E. J. (1997). Partial bleaching and the decay form characteristics of quartz OSL. Radiat. Meas. 27, 123–136. doi:10.1016/s1350-4487(96)00157-6
Bowman, D. M. J. S., Balch, J. K., Artaxo, P., Bond, W. J., Carlson, J. M., Cochrane, M. A., et al. (2009). Fire in the Earth system. Sci. Am. Assoc. Adv. Sci. 324 (5926), 481–484. doi:10.1126/science.1163886
Cao, Y., and Han, J. (2009). Wildfire activity and ecological environment change during Holocene in the Yuncheng Basin. J. Arid Land Resour. Environ. 23 (2), 125
Chen, G., Sun, S., Fang, X., and Kang, S. (1997). Heavy minerals and provenance of the Malan loess on the Qinghai-Xizang (Tibet) Plateau and its adjacent area. Acta Sedimentol. Sin. 15 (4), 134
Chen, Z., Yang, S., Luo, Y., Chen, H., Liu, L., Liu, X., et al. (2022). HIRM variation in the Ganzi loess of the eastern Tibetan Plateau since the last interglacial period and its paleotemperature implications for the source region. Gondwana Res. 101, 233–242. doi:10.1016/j.gr.2021.08.008
Dong, Z., Hu, G., Qian, G., Lu, J., Zhang, Z., Luo, W., et al. (2017). High‐altitude aeolian research on the Tibetan plateau. Rev. Geophys. 55, 864–901. doi:10.1002/2017rg000585
Engelbrecht, J. P., and Derbyshire, E. (2010). Airborne mineral dust. Elements 6 (4), 241–246. doi:10.2113/gselements.6.4.241
Fang, X., Cheng, F., Shi, Y., and Li, J. (1996). Garze loess and the evolution of the cryosphere on the Tibetan Plateau. Chin. Sci. Bull. 41, 336
Fang, X., Han, Y., Ma, J., Song, L., Yang, S., and Zhang, X. (2004). Dust storms and loess accumulation on the Tibetan plateau: A case study of dust event on 4 march 2003 in lhasa. Chin. Sci. Bull. 49, 953–960. doi:10.1360/03wd0180
Fang, X., Li, J., and Voo, R. V. d. (1999). Age and provenance of loess in West Qinling. Chin. Sci. Bull. 44, 2188–2192. doi:10.1007/bf03182706
Fang, X. (1994). The origin and provenance of Malan loess along the eastern margin of Qinghai-Xizang (Tibetan) Plateau and its adjacent area. Sci. China (serise B) 24, 539
Fitzsimmons, K. E. (2011). An assessment of the luminescence sensitivity of Australian quartz with respect to sediment history. Geochronometria 38 (3), 199–208. doi:10.2478/s13386-011-0030-9
Gong, Z., Sun, J., and Lü, T. (2015). Investigating the components of the optically stimulated luminescence signals of quartz grains from sand dunes in China. Quat. Geochronol. 29, 48–57. doi:10.1016/j.quageo.2015.06.004
Gray, H. J., Jain, M., Sawakuchi, A. O., Mahan, S. A., and Tucker, G. E. (2019). Luminescence as a sediment tracer and provenance tool. Rev. Geophys. 57 (3), 987–1017. doi:10.1029/2019rg000646
Huang, J., Minnis, P., Yi, Y., Tang, Q., Wang, X., Hu, Y., et al. (2007). Summer dust aerosols detected from CALIPSO over the Tibetan Plateau. Geophys. Res. Lett. 34 (18), L18805. doi:10.1029/2007gl029938
Jeong, G., and Choi, J. H. (2012). Variations in quartz OSL components with lithology, weathering and transportation. Quat. Geochronol. 10, 320–326. doi:10.1016/j.quageo.2012.02.023
Jiang, F., Wu, X., Xiao, H., Zhao, Z., Tian, G., and Liu, K. (1997). The Ganzi loess stratigraphy in the west Sichuan plateau. Acta Geol. Sin. 18 (4), 414–420.
Juyal, N., Pant, R. K., Basavaiah, N., Bhushan, R., Jain, M., Saini, N. K., et al. (2009). Reconstruction of last glacial to early Holocene monsoon variability from relict lake sediments of the higher central himalaya, uttrakhand, India. J. Asian earth Sci. 34 (3), 437–449. doi:10.1016/j.jseaes.2008.07.007
Lai, Z., and Murray, A. S. (2006). Red TL of quartz extracted from Chinese loess: Bleachability and saturation dose. Radiat. Meas. 41, 836–840. doi:10.1016/j.radmeas.2006.04.017
Lai, Z., and Wintle, A. G. (2006). Locating the boundary between the Pleistocene and the Holocene in Chinese loess using luminescence. Holocene (Sevenoaks) 16, 893–899. doi:10.1191/0959683606hol980rr
Li, D. (2021). A comparative study on luminescence dating methods of loess in the eastern margin of Tibetan Plateau. Lanzhou, China: Lanzhou University.
Li, G., Wen, L., Xia, D., Duan, Y., Rao, Z., Madsen, D. B., et al. (2015). Quartz OSL and K-feldspar pIRIR dating of a loess/paleosol sequence from arid central Asia, Tianshan Mountains, NW China. Quat. Geochronol. 28, 40–53. doi:10.1016/j.quageo.2015.03.011
Li, J., and Yang, L. (2022). Variations in the sensitivity of the optically stimulated luminescence from quartz in the Xifeng section: A spatiotemporal comparison across the Chinese Loess Plateau since the last interglaciation. Quat. Geochronol. 72, 101353. doi:10.1016/j.quageo.2022.101353
Li, S., Chen, Y., Li, B., Sun, J., and Yang, L. (2007). OSL dating of sediments from deserts in northern China. Quat. Geochronol. 2, 23–28. doi:10.1016/j.quageo.2006.05.034
Li, S. (2002). Luminescence sensitivity changes of quartz by bleaching, annealing and UV exposure. Radiat. Eff. defects solids 157, 357–364. doi:10.1080/10420150212998
Li, Y., and Zhou, L. (2021). Variations of thermally and optically stimulated luminescence sensitivity of loess and pedocomplex samples from southern Tajikistan, central asia. Geochronometria 48 (1), 242. doi:10.1515/geochr-2015-0118
Ling, Z., Yang, S., Xia, D., Wang, X., and Chen, F. (2022). Source of the aeolian sediments in the Yarlung Tsangpo valley and its potential dust contribution to adjacent oceans. Earth Surf. Process. Landf. 47 (7), 1860–1871. doi:10.1002/esp.5351
Liu, J., Jiang, F., Chen, J., Sun, Q., Chen, J., and Chen, Z. (2018). Luminescence properties of quartz in the sediments from TypicalChinese rivers and their implications for provenance. Geol. J. China Univ. 24, 88
Liu, L., Yang, S., Cheng, T., Liu, X., Luo, Y., Liu, N., et al. (2021). Chronology and dust mass accumulation history of the Wenchuan loess on eastern Tibetan Plateau since the last glacial. Aeolian Res. 53, 100748–100810. doi:10.1016/j.aeolia.2021.100748
Liu, T., and Ding, Z. (1998). Chinese loess and the palemonsoon. Annu. Rev. Earth Planet. Sci. 26, 111–145. doi:10.1146/annurev.earth.26.1.111
Liu, X. (1983). The quarternary in Sichuan Basin sichuan. Chengdu: Sichuan Sci.& Tech. Pulishers, 88
Lü, T., Sun, J., Feathers, J. K., and Sun, D. (2021). Spatiotemporal variations and implications of luminescence sensitivity of quartz grains on the Chinese Loess Plateau since the last interglaciation. Quat. Res. 99, 190–203. doi:10.1017/qua.2020.53
Lü, T., Sun, J., Li, S., Gong, Z., and Xue, L. (2014). Vertical variations of luminescence sensitivity of quartz grains from loess/paleosol of Luochuan section in the central Chinese Loess Plateau since the last interglacial. Quat. Geochronol. 22, 107–115. doi:10.1016/j.quageo.2014.04.004
Lü, T., and Sun, J. (2011). Luminescence sensitivities of quartz grains from eolian deposits in northern China and their implications for provenance. Quat. Res. 76, 181–189. doi:10.1016/j.yqres.2011.06.015
Moska, P., and Murray, A. S. (2006). Stability of the quartz fast-component in insensitive samples. Radiat. Meas. 41 (7), 878–885. doi:10.1016/j.radmeas.2006.06.005
Muhs, D. R. (2013). The geologic records of dust in the Quaternary. Aeolian Res. 9, 3–48. doi:10.1016/j.aeolia.2012.08.001
Ou, X., Zeng, S., Zhou, S., and Lai, Z. (2012). A review on research of loess and environmental change in west Sichuan Plateau of the eastern Qinghai-Tibetan Plateau. J. Earth Environ. 3, 692
Pan, B., and Wang, J. (1999). Loess record of Qinghai-Xizang Plateau monsoon variations in the eastern part of the plateau since the last interglacial. Quat. Sci., 330
Patterson, W. A., Edwards, K. J., and Maguire, D. J. (1987). Microscopic charcoal as a fossil indicator of fire. Quat. Sci. Rev. 6 (1), 3–23. doi:10.1016/0277-3791(87)90012-6
Pietsch, T. J., Olley, J. M., and Nanson, G. C. (2008). Fluvial transport as a natural luminescence sensitiser of quartz. Quat. Geochronol. 3, 365–376. doi:10.1016/j.quageo.2007.12.005
Pye, K. (1995). The nature, origin and accumulation of loess. Quat. Sci. Rev. 14 (7-8), 653–667. doi:10.1016/0277-3791(95)00047-x
Qiao, S., and Yang, Z. (2006). Advances in a study on quartz as a tracer for material source. Adv. Mar. Sci. 24, 266–274.
Qiao, Y., Zhao, Z., Wang, Y., Wang, S., Fu, J., Li, C., et al. (2006). Magneto stratigraphy and its palepclimatic significance of a loess-soli sequence from Ganzi area, West Sichuan Platrean. Quat. Sci. 26, 250–256.
Rengers, F. K., Pagonis, V., and Mahan, S. A. (2017). Can thermoluminescence be used to determine soil heating from a wildfire? Radiat. Meas. 107, 119–127. doi:10.1016/j.radmeas.2017.09.002
Rink, W. J., Schwarcz, H. P., Lee, H. K., Rees-Jones, J., Rabinovich, R., and Hovers, E. (2001). Electron spin resonance (ESR) and thermal ionization mass spectrometric (TIMS) 230Th/234U dating of teeth in Middle Paleolithic layers at Amud Cave, Israel. Geoarchaeology 16, 701–717. doi:10.1002/gea.1017
Sawakuchi, A. O., Guedes, C. C. F., DeWitt, R., Giannini, P. C. F., Blair, M. W., Nascimento, D. R., et al. (2012). Quartz OSL sensitivity as a proxy for storm activity on the southern Brazilian coast during the Late Holocene. Quat. Geochronol. 13, 92–102. doi:10.1016/j.quageo.2012.07.002
Sawakuchi, A. O., Jain, M., Mineli, T. D., Nogueira, L., Bertassoli, D. J., Häggi, C., et al. (2018). Luminescence of quartz and feldspar fingerprints provenance and correlates with the source area denudation in the Amazon River basin. Earth Planet. Sci. Lett. 492, 152–162. doi:10.1016/j.epsl.2018.04.006
Sun, J., Li, S., Muhs, D. R., and Li, B. (2007). Loess sedimentation in tibet: Provenance, processes, and link with quaternary glaciations. Quat. Sci. Rev. 26 (17), 2265–2280. doi:10.1016/j.quascirev.2007.05.003
Sun, Y., and An, Z. (2000). Sedimentary interpretation of surface textures of quartz grains from the eolian deposits. Acta Sedimentol. Sin. 18, 506
Tafel, A. (1914). My trip to Tibet. A study trip through northwest China and Inner Mongolia to eastern Tibet Stuttgart. Leipzig, Berlin, 698p.
Tang, G., Yang, S., Miao, Y., Luo, Y., Chen, Z., Liu, L., et al. (2022). Grain size characteristics of microfossil charcoal and the environmental implications in loess deposits from Ganzi, Western Sichuan Plateau. J. Lanzhou Univ. Nat. Sci. 58 (3), 289. 10.13885/j.issn.0455-2059.2022.03.002
Wang, H., Chen, F., and Zhang, J. (2002). Environmental significance of grain size of loess-paleosol sequence in Western part of Chinese Loess Plateau. J. Desert Res. 22, 21.
Wang, J., and Pan, B. (1997). Loess deposits in eastern part of qinghai-xizang plateau: Its characteristics and environment. J. Desert Res. 17 (4), 395.
Wang, S., Jiang, F., Tian, G., Fu, J., Liu, K., and Zhao, Z. (2005). The jinchuan loess stratigraghy in Western Sichuan Plateau. Acta Geol. Sin. 26 (4), 355. doi:10.3321/j.issn:1006-3021.2005.04.011
Wen, X., Tang, Y., Huang, C., and Gongbo, S. (2014). Multi-material source of loess deposits from the Jiuzhaigou natonal nature reserve on the Eastern margin of the Tibetan Pleteau. Mt. Res. 32 (5), 603
Wen, X., Wu, Y., Huang, C., Luo, M., and Chen, K. (2019). Grain size & elements composition characteristics and their implications for the late Pleistocene loess in the upper reaches of the Minjiang River,China. Mountain Research
Wintle, A. G., and Murray, A. S. (1999). Luminescence sensitivity changes in quartz. Radiat. Meas. 30, 107–118. doi:10.1016/s1350-4487(98)00096-1
Xu, C., Ma, Y. M., You, C., and Zhu, Z. K. (2015). The regional distribution characteristics of aerosol optical depth over the Tibetan Plateau. Atmos. Chem. Phys. 15 (20), 12065–12078. doi:10.5194/acp-15-12065-2015
Yang, S., Chen, Z., Chen, H., Luo, Y., Liu, L., Liu, X., et al. (2022). Magnetic properties of the Ganzi loess and their implications for precipitation history in the eastern Tibetan plateau since the last interglacial. Paleoceanogr. Paleoclimatol. 37, 1–17. doi:10.1029/2021pa004322
Yang, S., Fang, X., Shi, Z., Lehmkuhl, F., Song, C., Han, Y., et al. (2010). Timing and provenance of loess in the Sichuan Basin, southwestern China. Palaeogeogr. Palaeoclimatol. Palaeoecol. 292 (1), 144–154. doi:10.1016/j.palaeo.2010.03.039
Yang, S., Liu, L., Chen, H., Tang, G., Luo, Y., Liu, N., et al. (2021a). Variability and environmental significance of organic carbon isotopes in Ganzi loess since the last interglacial on the eastern Tibetan Plateau. Catena 196, 104866. doi:10.1016/j.catena.2020.104866
Yang, S., Liu, N., Li, D., Cheng, T., Liu, W., Li, S., et al. (2020). Quartz OSL chronology of the loess deposits in the Western Qinling Mountains, China, and their palaeoenvironmental implications since the Last Glacial period. Boreas 50, 294–307. doi:10.1111/bor.12473
Yang, S., Luo, Y., Li, Q., Liu, W., Chen, Z., Liu, L., et al. (2021b). Comparisons of topsoil geochemical elements from Northwest China and eastern Tibetan Plateau identify the plateau interior as Tibetan dust source. Sci. Total Environ. 798, 149240. doi:10.1016/j.scitotenv.2021.149240
Zhang, H., Nie, J., Liu, X., Pullen, A., Li, G., Peng, W., et al. (2021). Spatially variable provenance of the Chinese Loess Plateau. Geol. (Boulder) 49 (10), 1155–1159. doi:10.1130/g48867.1
Zhang, J. (2018). Applications of optically stimulated luminescence dating in the Chinese Loess Plateau. PhD thesis.
Zhang, J., Li, S., Sun, J., Lü, T., Zhou, X., and Hao, Q. (2022). Quartz luminescence sensitivity variation in the Chinese loess deposits: The potential role of wildfires. J. Quat. Sci., 1–12. doi:10.1002/jqs.3462
Zheng, C., Zhou, L., and Qin, J. (2009). Difference in luminescence sensitivity of coarse-grained quartz from deserts of northern China. Radiat. Meas. 44, 534–537. doi:10.1016/j.radmeas.2009.02.013
Keywords: quartz, luminescence sensitivity, loess, eastern Tibetan Plateau, provenance
Citation: Liu L, Yang S, Liu X, Li P, Wang H and Zhou J (2022) Variation of luminescence sensitivity of quartz grains from loess in eastern Tibetan Plateau and its provenance significance. Front. Earth Sci. 10:939638. doi: 10.3389/feart.2022.939638
Received: 09 May 2022; Accepted: 11 August 2022;
Published: 06 September 2022.
Edited by:
Hui Zhao, Chinese Academy of Sciences (CAS), ChinaReviewed by:
Jianhui Jin, Fujian Normal University, ChinaLinhai Yang, Shaanxi Normal University, China
Copyright © 2022 Liu, Yang, Liu, Li, Wang and Zhou. This is an open-access article distributed under the terms of the Creative Commons Attribution License (CC BY). The use, distribution or reproduction in other forums is permitted, provided the original author(s) and the copyright owner(s) are credited and that the original publication in this journal is cited, in accordance with accepted academic practice. No use, distribution or reproduction is permitted which does not comply with these terms.
*Correspondence: Shengli Yang, shlyang@lzu.edu.cn