- 1State Key Laboratory of Marine Geology, Tongji University, Shanghai, China
- 2Center for Marine Resources, Tongji University, Shanghai, China
- 3Research Institute of Petroleum Exploration and Development, Shengli Oilfield Company, SINOPEC, Dongying, Shandong, China
- 4State Key Laboratory of Oil and Gas Reservoir Geology and Exploitation (Southwest Petroleum University), Chengdu, China
- 5CNOOC China Ltd. Shanghai Branch, Shanghai, China
- 6Key Laboratory of Surficial Geochemistry, Ministry of Education, Nanjing University, Nanjing, China
Lacustrine mudrocks are important hydrocarbon source rocks due to their high contents of organic matter (OM). An accurate determination of the origins of OM in the rocks can contribute greatly to the identification of the sedimentary environment and the evaluation of the hydrocarbon generation capacity of the mudrocks. In this study, the lacustrine mudrocks of the Eocene Shahejie Formation in the Dongying Sag of China were sampled for further discussion of the types and origins of OM and the hydrocarbon generation capacity of the mudrocks. On that basis, the classical palynofacies classification scheme was improved, and five palynofacies types (PF1 to PF5) were classified to accurately reflect different lacustrine environments. Among them, PF1 to PF3 are rich in various amorphous organic matter (AOM), including benthic microbial mats, organic aggregates and algal aggregates, which are part of the lake environment in which aquatic organisms bloom. The hydrocarbon generation abilities of the three palynofacies are different but generally strong. PF5 and PF4 have abundant terrestrial plant fragments and macrophyte fragments, respectively, indicating a shallow, near-provenance lake environment and a shallow, macrophyte-flourishing lake environment. These two types of palynofacies have weaker hydrocarbon generation capacities than the former three. Due to the enrichment of different types of palynofacies, each formation of the Dongying Sag shows distinct hydrocarbon generation potentials. Therefore, it is important to pay special attention to the origin of OM and its influences on the hydrocarbon generation capacity of lacustrine mudrocks for a better understanding of the deposition and hydrocarbon generation potential of source rocks.
Introduction
Lacustrine mudrocks that are rich in organic matter (OM) are important source rocks in eastern China. As the material basis of hydrocarbon generation, OM is a significant component of lacustrine mudrocks. The OM in the rocks are various types and they have different sources and large variation in their abundances, which are all influenced by sedimentary environment factors (Li et al., 2017; Deng et al., 2021). These affect the hydrocarbon generation potential of the source rocks (Zhu et al., 2018). Therefore, it is significant to determine the origin and characteristics of the OM in the mudrocks that formed in different sedimentary environments.
Palynofacies analysis is a useful tool for identifying the origin of OM preserved in rocks based on their morphological characteristics (Tyson 1995; Batten 1996). There are three main OM categories: the structured OM group, amorphous OM group and palynomorphs group. Each group contains OM from various sources, and these OM types are deposited in different sedimentary environments. Therefore, many studies have reconstructed the paleoenvironment based on the OM composition in sediments (El Beialy et al., 2010; Suárez-Ruiz et al., 2012; El Atfy et al., 2014): sediments that have abundant structured groups likely originated in proximal and shallow environments, while amorphous OM often accumulates in relatively distal and reducing conditions (Tyson 1995; Emmings et al., 2019). This environmental reconstruction method is widely used in sediment studies and is considered effective in large-scale depositional environment identification. However, in a relatively stable and continuously changing water environment, such as a stable lake system, sedimentary OM shows only slight changes in the type, so it is of great importance to distinguish the types of OM in different environments.
In a lake basin, varying sedimentary environments cause differences in the deposited OM, and in turn, differences in the hydrocarbon generation capacities of the rocks (Cai et al., 2019; Li et al., 2021). The quality of OM in source rock determines its hydrocarbon generation capacity. Quantitative pyrolysis parameters and palynological organic debris parameters that can indicate the morphology of OM are often used to describe the quality of OM (Singh and Mahesh 2015; Zhang et al., 2015; Mathews et al., 2020). According to differences in hydrocarbon generation characteristics, OM is often divided into oil-prone and gas-prone materials (Zargari et al., 2015). In recent studies, many scholars have also emphasized the concept of “hydrocarbon-generating OM”, believing that different OM types have different contributions to the hydrocarbon generation of rocks. For example, macroscopic algae, such as red algae, are considered major contributors to hydrocarbon generation (Zhang et al., 2007; Zhang et al., 2015). Therefore, analyzing the hydrocarbon generation capacity of OM from different sources is an important basis for accurately assessing the hydrocarbon generation capacity of lacustrine shale.
The Dongying Sag is one of the most oil- and gas-enriched sags in China. The thick dark lacustrine shale in the middle Es3 submember, the lower Es3 member and the upper Es4 submember of the Shahejie Formation is the main source rock of the sag (Zhang et al., 2012; Zeng et al., 2017). Many previous studies have confirmed the formation process and mineral composition of this hydrocarbon source rock (Zeng et al., 2017; Tian Yang et al., 2018), but the source and features of OM in the rock have not been identified. To clarify the characteristics and hydrocarbon generation capacity of sedimentary OM in different sedimentary environments, we systematically collected mudrocks from the middle Es3 submember to the upper Es4 submember in the Dongying Sag. We analyzed the origin and sedimentary depositional environment of OM in source rocks and determined the formation of OM and its influence on the hydrocarbon generation of source rocks to improve the understanding of the formation and hydrocarbon generation of source rocks.
Geological and lithofacies settings
The Dongying Sag is located in the southeastern region of the Jiyang Depression in China and covers an area of more than 5,800 km2 (Figure 1). This sag has a half-graben shape that is faulted in its southern region and overlapping in its northern region.
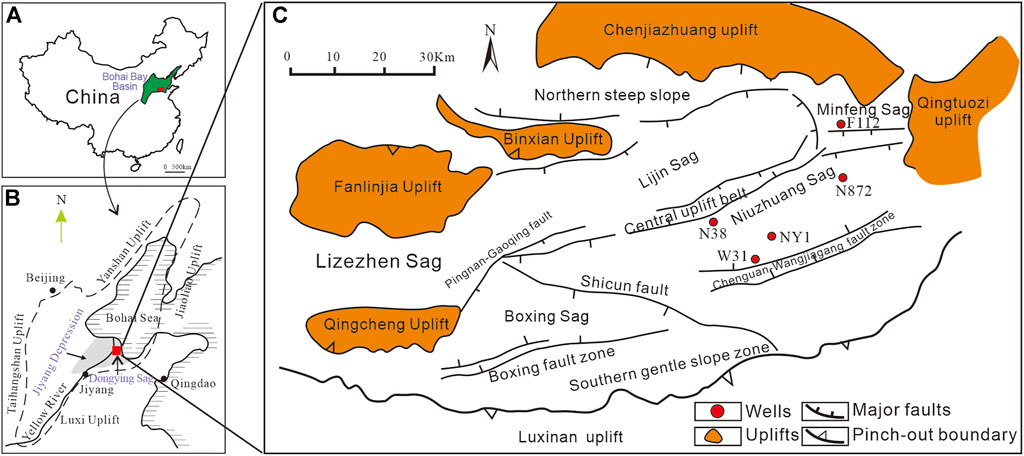
FIGURE 1. Location map. (A,B) Location of the Dongying Sag. (C) Structural features of the Dongying Sag and the sampling well locations in the study area.
The Eocene Shahejie Formation contains the main source rocks of the Dongying Sag. There are three submembers in the Eocene Shahejie Formation: the middle Es3, lower Es3, and upper Es4 submembers (Figure 2).
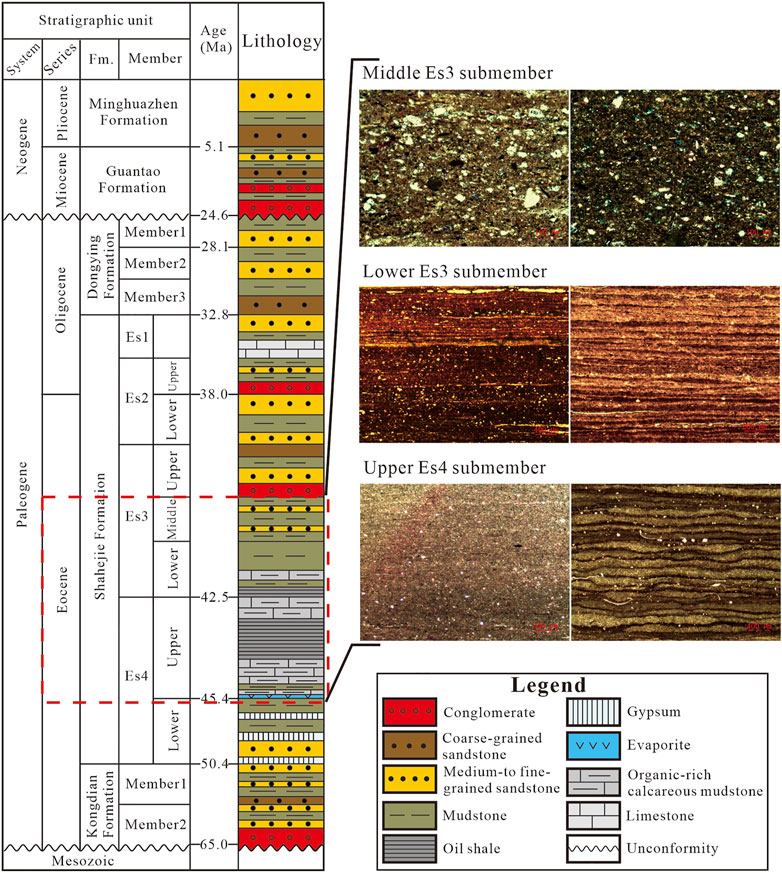
FIGURE 2. Stratigraphic column of the Dongying Sag (Modified from Ma et al., 2017).
During the sedimentary period from the upper Es4 to middle Es3 submember, the water column changed from shallow to deep and then gradually to shallow, the salinity also decreased constantly, and the environment evolved from salty lake to semi-salty-fresh water lake (Wang et al., 2018). As the sedimentary environment represented by each section changes, different mudrock types are present, and the components of OM and minerals vary obviously (Zeng et al., 2017; Liu et al., 2020).
Calcareous shale, oil shale and mudstone are enriched in the upper Es4 submember, with high content of carbonate minerals in the rocks. Microfossils such as Deflandrea and Pediastrum are discovered in the stratum, both paleontological and geochemical parameters reflect the high salinity of the lake (Xu et al., 1997). The salinity of the lake decreases in the lower Es3 submember. Oil shale and mudstone were developed in this formation. The fine-grained rocks were deposit in deep lake environment. The lake kept expanding, and water salinity further decreased during the period of the middle Es3 submember. Silty mudstone enriched in the formation, and silty exogenous minerals (quartz and feldspar) are rich in the rocks (Figure 2), which reflected typical shore shallow lake sedimentary facies.
In general, between the deposition of the upper Es4 and middle Es3 submembers, the ancient lake evolved from a deep and saline lake to a shallow and freshwater lake (Figure 2). During the sedimentary period of the Eocene Shahejie Formation, the ancient lacustrine environment was in an anoxic-reducing environment, and the sedimentary OM is well preserved (Li et al., 2003; Wang et al., 2015; He et al., 2017).
Methods
A total of 198 samples were collected from 5 wells, including NY1, N38, W31, N872, and F112, in the Eocene Shahejie Formation (the upper Es4 to middle Es3 submembers) (Figure 3). All samples were collected for thin section observations and palynofacies and pyrolysis analysis.
Palynofacies analysis
These mudrocks samples were processed for palynofacies analysis. Approximately 50 g of sediments was sampled for organic matter analyses. These samples were first treated with HCl (10%) to remove carbonate minerals, and then treated with HF (35%) to remove silicate minerals. After the treatment, the samples were cleaned to neutral by filtered water treatment. This method ensured that most of the OM in the sample was not lost.
The relative percentage of organic matter components is based upon counting of at least 400 organic particles per sample, which was performed using an OLYMPUS BX51-P microscope with transmitted light and fluorescence light. The morphological characteristics of various OM types were detailedly identified in the study. Differences between terrigenous and aquatic organic matter are the focus of observation. Since amorphous organic matter (AOM) was dominant in the mudrocks, the identification of various AOM was also performed. Quantitative analysis of the composition of OM assemblages in each sample was conducted in this study.
Rock-Eval VI pyrolysis
A total of 198 samples were pyrolyzed using a Rock-Eval 6 pyrolysis apparatus (RE6, Vinci Technologies®). A series of successive stages were performed. First, 50 mg of each crushed sample was subjected to a temperature of 300°C, and a free hydrocarbon (peak S1, mg/g of rock) was qualified. Then, programmed pyrolysis was performed at temperatures increasing from 300 to 650°C to qualify the potential hydrocarbons (peak S2, mg/g of rock). Simultaneously, oxygenated products, including CO and CO2, were measured at temperatures between 300°C and 390°C; these products were referred to as peak S3 (mg CO2/g of rock), and residual carbon at 600°C (which was referred to as peak S4 (mg CO2/g of rock)) was recorded. A number of parameters, including total organic carbon (TOC), the hydrogen index (HI), and the oxygen index (OI), were assessed at peaks S1, S2, S3, and S4. The value of Tmax corresponded to the temperature at the maximum S2.
Thin section observations
The rock samples were ground into thin sections showing the vertical bedding and parallel bedding to observe the preservation of OM in the rocks. Petrographical examination was performed using a Nikon microscope. The thin sections were analyzed under optical plane-polarized and fluorescent light at magnifications ranging from ×50 to 500x.
Scanning electron microscopy observations
Gold-coated sample chips were prepared for observation of the microscopic characteristics using an FEI Quanta Model 200 F scanning electron microscope with a working current of 20 kV.
Results
Organic matter characteristics of rocks
Classification of organic matter in rocks
According to the currently widely used classification scheme (Tyson 1995; Carvalho et al., 2006; Garcia et al., 2011), OM is divided into three main types: structured OM, amorphous OM (AOM) and palynomorphs:
(1) Structured OM includes land plant fragments and macrophytes.
According to microscope observations, land plant fragments retained relatively intact cell structures. The larger, thick-walled cells are regularly arranged (Figures 4A–C). Stomatal structures are visible on the surfaces of some higher plant fragments (Figure 4A). These morphological features are typical differences between land plants and aquatic plants (Martín-Closas et al., 2005). The land plant fragments rarely exhibit fluorescence.
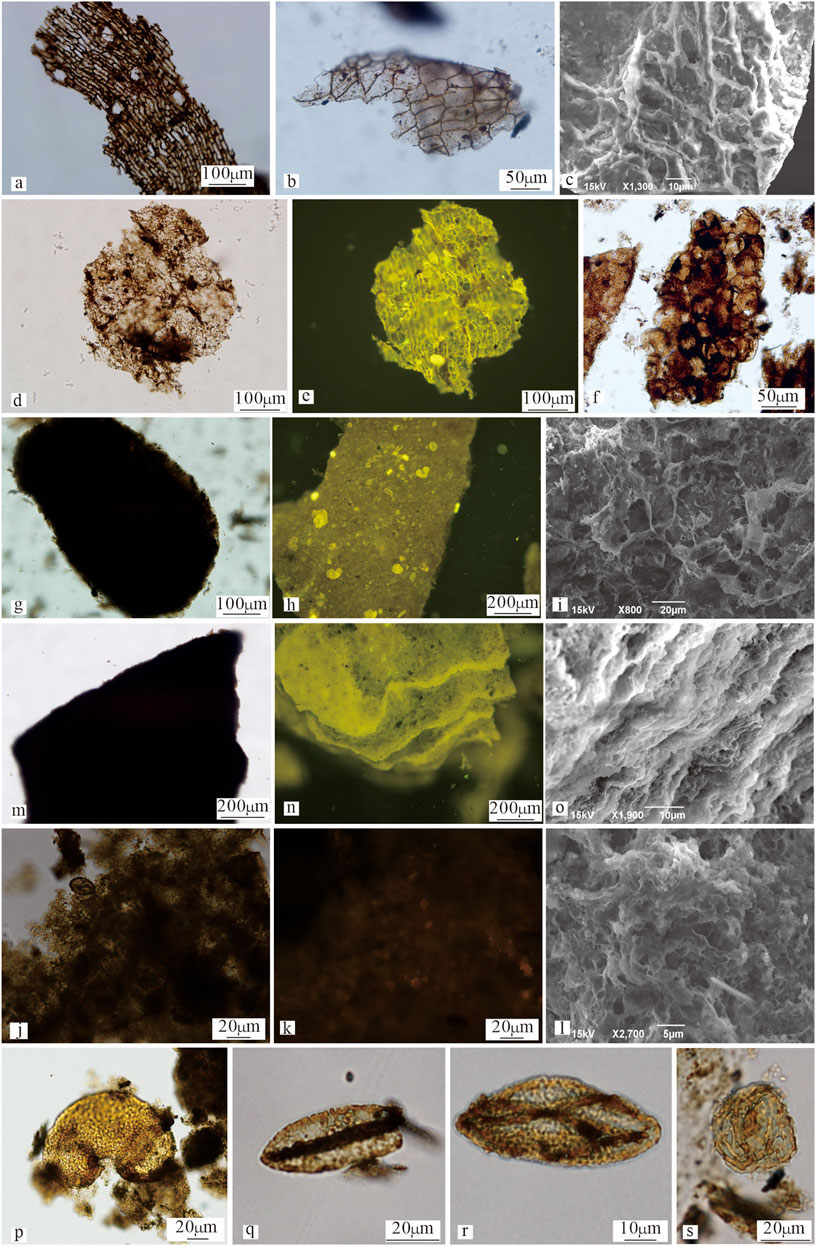
FIGURE 4. Morphological characteristics of each kind of OM. (A,B). Land plant fragments under transmitted light; (C). cell structure of land plant tissues under SEM; (D). macrophyte fragments under transmitted light; (E). macrophyte fragments with good fluorescence intensity; (F). tetrasporangia of macrophytes; (G).; granular AOM under transmitted light; (H). granular AOM under fluorescence; (I). surface of granular OM; (J). spongy AOM under transmitted light; (K). spongy AOM with weak fluorescence intensity; (L). vesicular extracellular polymers on the surfaces of spongy AOM under SEM; (M). laminated AOM under transmitted light; (N). laminated AOM under fluorescent light; (O). edges of laminated AOM under SEM; (P). A. microalatus; (Q). Ephedripites fushunensis; (R). Cupuliferoipollenites sp; (S). Dictyotidium sp.
The original remaining sieve cells are visible in some macrophyte fragments under transmitted light (Figure 4D), and the cell structure is more obvious under fluorescent light (Figure 4E). These sieve cells are elongated and branched and interweave with each other to form the “parenchymatous” thallus (Figure 4D). These particles have moderate fluorescence intensities. Although the preservation potential of macrophytes is not as good as that of land plants, and they are sometimes preserved in a “structureless” state in rocks, the remaining tetrasporangia can occasionally be observed, which is one of the important identification signals of macrophytes (Figure 4F) (Bellinger and Sigee 2011).
(2) Amorphous OM (AOM) group includes granular AOM, laminated AOM and spongy AOM.
Granular OM is yellow to dark brown, with particle sizes ranging from 50 to 400 μm. The particles are regular and structureless, with clear edges and homogeneous surfaces with uneven structures under transmitted light (Figure 4G). The fluorescence intensity of this kind of OM is weak to moderate, and a variety of OM particles, including spores and algae fragments with good fluorescence, are often adhered to the surface (Figure 4H). Furthermore, fossil hyphae can often be found on the particle surfaces in SEM observations (Figure 4I). OM with these morphological characteristics likely formed by the aggregation of phytoplankton blooms (Batten 1996).
Laminated AOM are flake-like particles with sizes of 100–400 μm, and the particles have clear edges (Figure 4M). Although there are no remaining structures on the surfaces of this OM under transmitted light, tiny layers on the edges of OM particles are clearly visible under fluorescent light (Figure 4N). The laminated structure can be further magnified under SEM (Figure 4O). OM with this morphological characteristic is often considered to be lamalginite (Khan et al., 2020).
Spongy AOM is named from the fact that it has no fixed morphology. Spongy AOM is generally dark brown under transmitted light. The particles are amorphous and have no clear edges (Figure 4J). This kind of OM exhibits weak to moderate fluorescence (Figure 4K). Fossil extracellular polymeric substances (EPS) are visible on the surfaces of the spongy AOM under SEM (Figure 4L). Spongy AOM consists predominantly of various OM particles, such as fecal pellets and other particles in the water column (Turner 2015).
The formation and depositional processes of these kinds of AOM is further confirmed in the following text.
(3) Palynomorphs
The palynomorph group includes pollen and spores in the study area. These palynomorph particles are small in size (usually less than 40 μm) with well-preserved forms in transmitted light (Figures 4P–S). Under fluorescent light observations, the particles show distinct cellular structures and obvious yellowish fluorescence. The palynomorphs include A. microalatus, Ephedripites fushunensis, Cupuliferoipollenites sp., and Dictyotidium sp. (Figures 4p–s).
Quantitative analysis of organic matter
The quantitative analytical results show that AOM is the main component of OM in the mudrocks in the study area, with an average content reaching 78.0%; the structured OM content is the second highest, with an average of 19.2%; the palynomorph content is the lowest, with an average of 2.8%; and the palynomorph content in all samples is less than 3%.
Characteristics of pyrolysis
The results of pyrolytic analysis reveal that the TOC values of the rocks in the study area range from 0.35% to 12.22% (Table 1; Figure 5), and the average TOC content is 3.06%. A comparison of the pyrolytic parameters of each submember of the formation reveals that each member also has obvious differences. The TOC of the middle Es3 submember is the lowest, with an average of 1.40%; the TOC of lower Es3 submember is the highest, with an average of 4.56%; and the TOC of the upper Es4 submember is between these two values, with an average of 3.01%.
A comparison of the hydrogen index (HI) and oxygen index (OI) of each member reveals that middle Es3 submember has a low HI and high OI. The HI and OI are 273 mg HC/g TOC and 57 mg HC/g TOC, respectively. Rocks of the upper Es4 submember and lower Es3 submember are characterized by high HI and low OI values; the former has an average HI of 528 mg HC/g TOC and an average OI of 32 mg HC/g TOC, and the latter has an average HI of 624 mg HC/g TOC and an average OI of 19 mg HC/g TOC.
The organic characteristics of mudrocks in each member of the Dongying Sag are reflected not only in the TOC, HI and OI but also in other pyrolytic parameters, such as the hydrocarbon generation indices S1 and S2. Both S1 and S2 are lower in middle Es3 submember and are 0.23 mg/g and 4.47 mg/g, respectively. The lower Es3 submember has the highest S2, averaging 30.59 mg/g, and the average S1 is 3.10 mg/g, while lower Es4 submember has the highest S1, averaging 3.59 mg/g, and the average S2 is 16.35 mg/g.
The Tmax values of all kinds of rocks are between 430 and 441°C, showing similar organic matter maturity.
Discussion
Depositional processes of organic matter and palynofacies
Origin and depositional process of organic matter
The sedimentary process of OM can be inferred based on the preservation of OM in rocks and the structural characteristics of rocks. In this study, we selected separate samples rich in each kind of OM to analyze the preservation and depositional process of each type of sedimentary OM mentioned above.
Samples with many land plant fragments are characterized by abundant silt-size detrital particles (mainly quartz) (Figures 6A–C). These land plant fragments are dispersed in the silt-rich matrix under thin section observation (Figures 6B,C). The coexistence of land plants and silt-size particles reflects exogenous inputs in a shallow lake environment (Zeng et al., 2018). Due to the weaker surface activity (Burdige 2007; Xuan Yang et al., 2018), these organic particles may have been deposited as single grains. The deposition of land plant fragments is a physical process in shallow lakes (Furota et al., 2021).
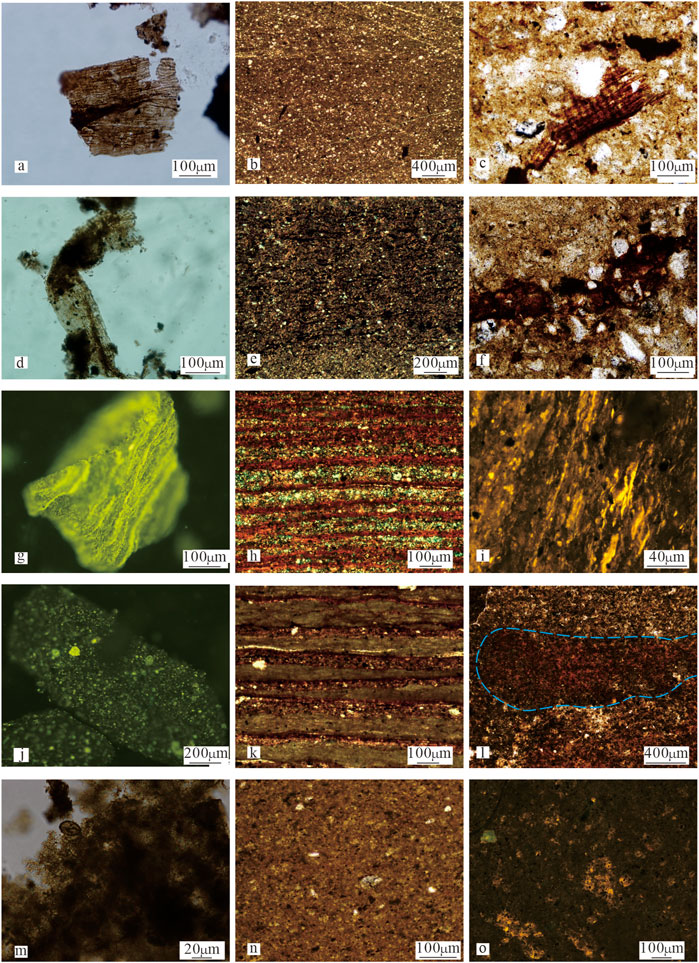
FIGURE 6. OM preserved in rocks. (A). Land plant tissue fragments; (B). vertical section of mudstone rich in land plant fragments; (C). land plant fragments visible in a cross-section of mudstone (A–B). N38, 2951.5 m, middle Es3 submember); (D). macrophyte fragments; (E). discontinuous laminae in mudstone rich in macrophytes (vertical section); (F). preserved macrophyte fragments in a cross-section of mudstones (d-f. N38, 3336.6 m, lower Es3 submember); (G). laminated AOM; (H). shales rich in laminated AOM that contain abundant OM-rich laminae; (I). granular AOM visible in a cross-section of shales under fluorescent light (G–I. NY1, 3375.3 m, upper Es4 submember); (J). granular AOM; (K). shales rich in granular AOM characterized by a laminated structure; (J). granular AOM particles in a mudrock cross-section (within the dotted blue line) (J–L. NY1, 3357.3 m, upper Es4 submember); m. spongy AOM; (N). vertical section of rocks rich in spongy AOM; (O). spongy AOM visible in a mudrock cross-section under fluorescent light (M–O, NY1, 3448.5 m, upper Es4 submember).
Mudrocks, which have abundant macrophyte fragments, are characterized by discontinuous OM laminae and fewer silt-size mineral particles (Figure 6E). The OM laminae were derived from macrophytes (Figure 6D,F). The fabrics of the shales indicate that this rock was deposited in a shallow water environment with relatively less allochthonous inputs (Tyson 1995; Schieber et al., 2010).
Rocks that enriched in laminated AOM, consisted of alternating couplets of dark organic-rich layer and light mud layer (Figure 6H). This indicates that the rocks formed in a standing water environment with periodic changes. Observations of cross-sectional thin section under fluorescent light reveal that the OM in rocks show obvious lamalginite (Figures 6G,I). This kind of structure has been found in source rocks in many areas (Khan et al., 2020; Xie et al., 2020; Froidl et al., 2021), and it has been confirmed that the organic-rich laminae originate from microbial mats (Schieber et al., 2007; Batchelor 2016; Froidl et al., 2021). The microbial mats likely grew in the clear photic zone of the lake bottom and eventually formed rich organic laminae in rocks (Prieto-Barajas et al., 2018). The laminated AOM were finally preserved by autochthonous deposition.
Granular AOM-enriched rocks are usually combinations of multiple laminae (Figure 6K). In vertical thin sections, such OM is preserved in the rock in the form of organic laminae. In cross-section, some forms of granular OM are still visible (Figures 6J,L). Previous studies on this lamina suggest that it is origin from algae blooms (Alam et al., 2016; Zhang et al., 2018). In nutrient-rich water, algae can flourish in large numbers and gather together to form algae clumps, and OM can spontaneously gather and eventually deposit to form organic-rich laminae (Goth et al., 1988).
Rocks with higher contents of spongy AOM show that this kind of OM is only visible under fluorescent light (Figures 6M–O). It is believed that this OM was closely related to clay minerals (Cai et al., 2020). In water column, during algal bloom intermission, OM deposited as ‘organic aggregates’ rather than as individual particles (Romankevich 1984; Tyson 1995). These organic aggregates are deposited with clay minerals via flocculation to form dark laminae or mud matrices (Passow 2002; Turner 2002; Wingender et al., 2012; Turner 2015; Zeng et al., 2017; Zeng et al., 2018).
The palynomorph particles are tiny and can be carried into water column by wind forces and transported by hydrodynamic forces (Behling et al., 2001; Sebag et al., 2006; Guler et al., 2013). However, the palynomorph content in the shales in the study area is so low (less than 2% in most samples) that its contribution to the hydrocarbon generation of the source rocks is likely very limited. Therefore, it is not discussed exclusively in this article.
In conclusion, the sources and sedimentary environments of all kinds of OM are quite different, including benthic OM and flocculation-generated OM. These OM types were deposited via different sedimentary processes and eventually preserved in rocks.
Classification of palynofacies in lacustrine environments
The AOM-phytoclast-palynomorph (APP) ternary diagram of Tyson (1995) is widely used to characterize palynofacies assemblages of various paleoenvironment. Quantitative palynofacies data of the study area distribute in four regions of the ternary diagram: I, II, VI and IX (Figure 7). More than 75% of the shale OM assemblages of upper Es4 and lower Es3 sumembers are concentrated in Zone IX, indicating a distal suboxic-anoxic basin with deeper water environment. Most of the OM assemblages of middle Es3 are distributed in I and II regions. This indicates highly proximal basin and marginal dysoxic-anoxic basins with shallow water. It can be seen that mudrocks of each submember in the study area were deposited in a anoxic water environment, and the water depth changed significantly. Therefore, Tyson’s OM assemblage classification scheme can be used to identify the changes in lake basins in large-scale sedimentary environments.
However, the classic Tyson’s classification scheme also has some limitations; this classification simplifies various OM types into AOM group and structured OM group. As discussed above, the deposition and preservation of these OM are obviously different, and they are the products of different environments. Dividing these OMs into the same component inevitably affects the accurate assessment of the lacustrine environment. Based on the above reasons, this study combined the natural and sedimentary modes of all kinds of OM and further divided the OM assemblage according to Tyson’s classical classification to analyze the formation environment of OM in a more detailed way.
In the new classification, the samples from Zone IX that are rich in amorphous OM were further divided into three OM combinations:
1 PF1: The content of laminated AOM is higher than 40%, which reflects the lake environment in which benthic microbial mats thrive in large numbers.
2 PF2: The content of spongy AOM aggregates is higher than 50%, which reflects the sedimentary environment where a large amount of OM accumulated and extensive flocculated deposition occurred.
3 PF3: The granular AOM is relatively abundant, and the laminated AOM and spongy AOM are lower than 40% and 50%, respectively, which reflects the water environment in which phytoplankton aggregated.
Samples with less than 60% AOM content and relatively high structured OM content were further divided into two categories:
4 PF4: Samples enriched in benthic algae indicate a lakefront environment, in which abundant benthic algae grew; the lake was relatively shallow and the exogenous input was lower.
5 PF5: The presence of higher plants and submerged plants indicates a shallow water environment with exogenous inputs.
Palynofacies and hydrocarbon potential
Palynofacies and total organic carbon
Due to the different sources and depositional processes of OM in rocks, this study focused on the relationship between OM types and TOC. Figure 8A shows the TOC characteristics of each palynofacies. The TOC values of PF1 range from 1.86% to 12.22%, with an average of 5.05%; the average TOC values of PF2 and PF3 are 2.78% and 3.16%, respectively; the values of PF4 vary between 0.52% and 4.16%; and PF5 has the lowest TOC values, with an average of 1.21%. We further analyzed the organic composition in each TOC interval. As shown by Figure 8B, while the TOC values are less than 1.0%, PF4 and PF5 are dominant. As the TOC values increase to 1.0%–3.0%, the proportions of PF2 and PF3 also increase. When the TOC values exceed 5%, the samples are mainly PF1, and there are no PF4 or PF5.
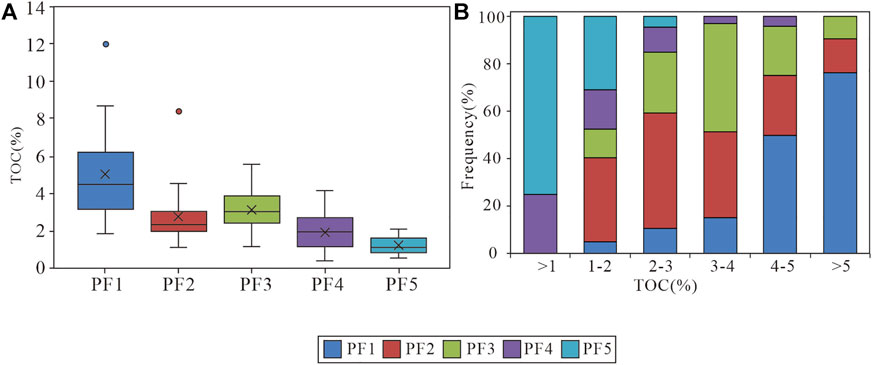
FIGURE 8. Relationship between the palynofacies and TOC of mudrocks. (A). TOC values of each palynofacies; (B). palynofacies composition in each TOC interval.
Both figures (Figures 8A,B) show that there is a close relationship between OM types and TOC in the rocks of the study area. Mudrocks that are rich in terrigenous OM and macrophytes have relatively low TOC values. In contrast, mudrocks, which have abundant AOM, have higher TOC values. As a result, in the low TOC range of the study area, the exogenous OM is the main contributor to the total TOC, while in the high TOC range, the autogenous OM is the main TOC contributor. The amount of exogenous OM input in the lake basin is far less than that of autogenous OM. This is consistent with a previous study (Cai et al., 2020).
In summary, the plankton bloom of the lake during deposition resulted in OM abundance in lacustrine mudrocks. In shallow and inshore lake environments, authigenic OM is diluted by terrigenous inputs (Li et al., 2021; Xie et al., 2021). This results in a lower OM abundance in the mudrocks that formed in the environment.
Hydrocarbon potential of each palynofacies type
Different sources, properties and depositional patterns of OM not only affect the abundance of OM in rocks but also may cause changes in hydrocarbon generation. In this study, the HI, OI, S1, and S2 were used to compare the pyrolytic parameter characteristics of various palynofacies assemblages to clarify the influence of different types of OM assemblages on the hydrocarbon generation process.
From the results of the HI and OI parameters (Table 2; Figure 9), PF1 has the highest HI, reaching an average of 655 mg/g, and the HI values of PF2 and PF3 are similar, averaging 514 mg/g and 543 mg/g, respectively. The HI values of PF4 and PF5 are 372 mg/g and 242 mg/g, respectively, which are significantly lower than those of the former three types of palynofacies. However, the OI values of PF4 and PF5 are significantly higher. According to the kerogen type indicated by the HI-OI values (Figure 9B), the kerogen types of PF1-PF3 are mainly I-II, and this type of kerogen has high HI and low OI values, which are significantly better than those of PF4 and PF5 (in which the kerogen types are mainly II-III). This indicates that rocks formed by the autochthonous OM and organic aggregates are oil-prone kerogen types, while rocks formed by the evolution of macrophytes grown in shallow water environments have relatively poor kerogen types.
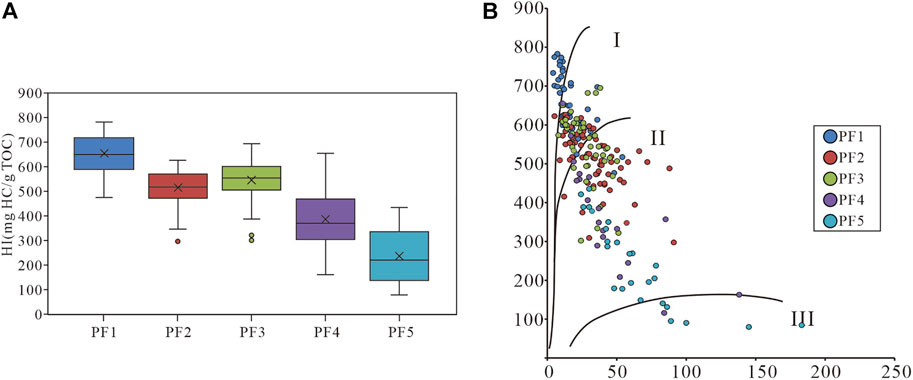
FIGURE 9. HI and kerogen type of each palynofacies. (A). HI values of each palynofacies; (B). kerogen type of each palynofacies.
The average Tmax values of all the palynofacies assemblages concentrated in a interval of 435∼445°C (Table. 2). This indicates early mature stage of OM, and this is consistent with the Ro results of previous tests (Li, 2011; Zhang et al., 2012). Geochemical parameters also reflect that there is little difference in maturity among various palynofacies.
Figure 10 lists TOC-S1+S2 charts of various palynofacies to analyze their hydrocarbon generation capacity (Peters 1986): PF1 has the best hydrocarbon generation capacity among all palynofacies assemblages. PF2 and PF3 are similar, and both are in good-very good stages. Compared with the first three, PF4 has a weak hydrocarbon generation capacity and is in medium-good stages. PF5 is the weakest and is mostly in medium-poor stages. This indicates that authigenic aquatic OM is an important component of hydrocarbon generation. The weak hydrocarbon generation capability of PF4 is probably related to the poor preservation ability of macrophytes (Tyson 1995). The weak hydrocarbon generation capacity of the PF5 is due to the terrigenous land plants and dilution of OM in shallow environment.
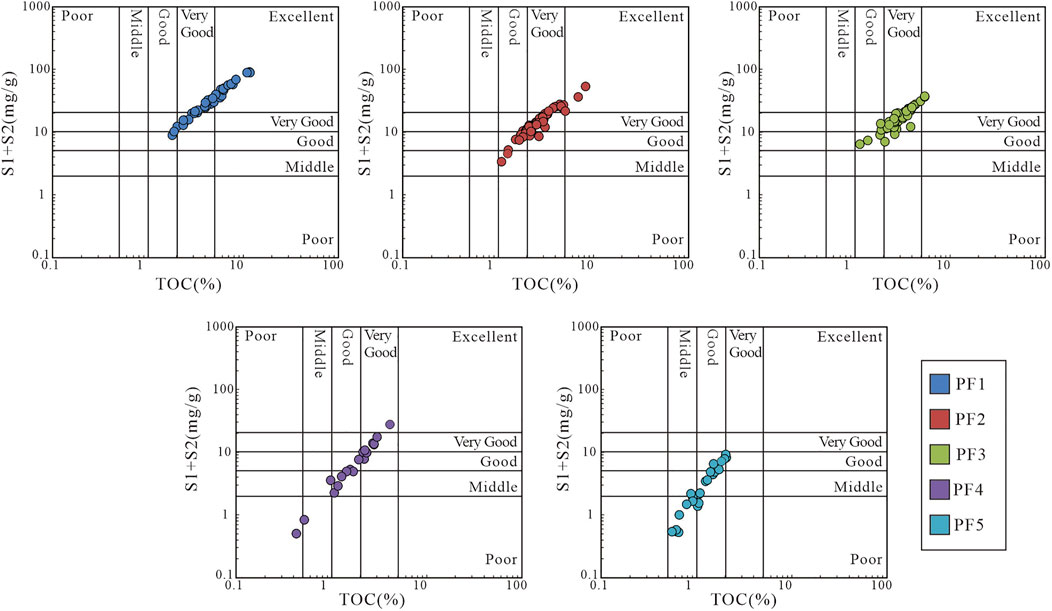
FIGURE 10. Hydrocarbon generation potential of palynofacies (according to Peters, 1986).
Lacustrine sedimentary environment and hydrocarbon generation of mudrocks
Based on the comparison between the deposition process and hydrocarbon generation differences of the palynofacies assemblages, a model of OM deposition and hydrocarbon generation in the study area was established to discuss the determination of environmental factors on the OM characteristics in rocks (Figure 11).
In a shore-shallow lake environment, land plants fragments are carried into water column with silt-size detrital minerals. The OM is deposited into the water bottom mainly through physical deposition which is obviously affected by hydrodynamic action. The abundance of OM is low (TOC is about 1%) in the sediments due to the dilution of exogenous minerals (Tyson, 1995; Xie et al., 2021). The land plants exhibit a low mean hydrogen index (about 242 mg HC/g TOC), and are mainly types II-III kerogen. The hydrocarbon generation capacity of the rock is relatively weak (S1: 0.18 mg/g, S2: 3.49 mg/g). As exogenous components gradually decreased, the autochthonous macrophytes became more concentrated. TOC of rocks deposited at this stage gradually increased to 2%. The kerogen is mainly type II with the HI of 372 mg HC/g TOC. The hydrocarbon generation capacity of the rock is moderate (S1 of 0.62 mg/g ;S2 of 8.73 mg/g).
There are a variety of conditions in the deep lake- semi-deep lake environment. When the water column is eutrophicated, plankton and other microorganisms flourish, and these tiny organic particles deposit as aggregates (Turner, 2015). Mudrocks formed in this environment are often rich in OM and their TOC can reach more than 3.2%. The kerogen type is mainly type I with average HI of 543 mg HC/g TOC, and the rock has good hydrocarbon generation capacity (average S1 and S2 are 2.76 mg/g and 17.40 mg/g).
In the intermission of the algal bloom period, the water column is mainly composed of organic aggregates, which are deposited by flocculation (Turner 2015). There is a close relationship between minerals and OM during deposition (Cai et al., 2019). The hydrogen rich Type I kerogens (average HI of 514 mg HC/g TOC) formed in this environment. Mudrocks with average TOC of 2.8% and Type I kerogen (average HI of 514 mg HC/g TOC) are formed in this environment. The mudrocks show good hydrocarbon generation capacity (S1+S2 of 14.11 mg/g).
In the euphotic zone of the lake, microbial mats flourish at the bottom of the lake, and these mats can be deposited in situ and preserved. The microbial mats exhibit high average HI values of 655 mg HC/g TOC. In this environment, the abundance of OM in mudrocks are the highest (average TOC of 5.0%). Mudrocks have high average S1 and S2 (3.95 mg/g and 34.37 mg/g), show very good hydrocarbon generation potential.
An overview of the hydrocarbon generation capacity of OM in various lake basin environments shows that the capacity gradually changes as the lake changes from shallow to deep. In shallow water environments, exogenous OM is deposited by physical deposition, and the hydrocarbon generation capacity of such OM is generally weak. As the water deepens, the amount of exogenous input decreases, benthic macrophytes dominate the deposition and accumulation of OM, and the hydrocarbon generation capacity of rocks increases. When the water deepens further, algae blooms promote the further hydrocarbon generation of rocks. At the same time, when microbial mats at the bottom of the lake basin, a large amount of OM enrichment places the rocks in a higher hydrocarbon generation stage.
In summary, from shore-shallow lake to deep lake environments, sedimentary environmental factors not only control the difference in OM types but also significantly control the OM abundance and hydrocarbon generation capacity of mudrocks.
Hydrocarbon potential of each formation in the Dongying Sag
Based on the deposition and hydrocarbon generation capacity of various palynofacies, the palynofacies assemblage patterns of the Shahejie Formation in the Dongying Sag were analyzed in this study. The composite chart of each layer is shown in Figure 12.
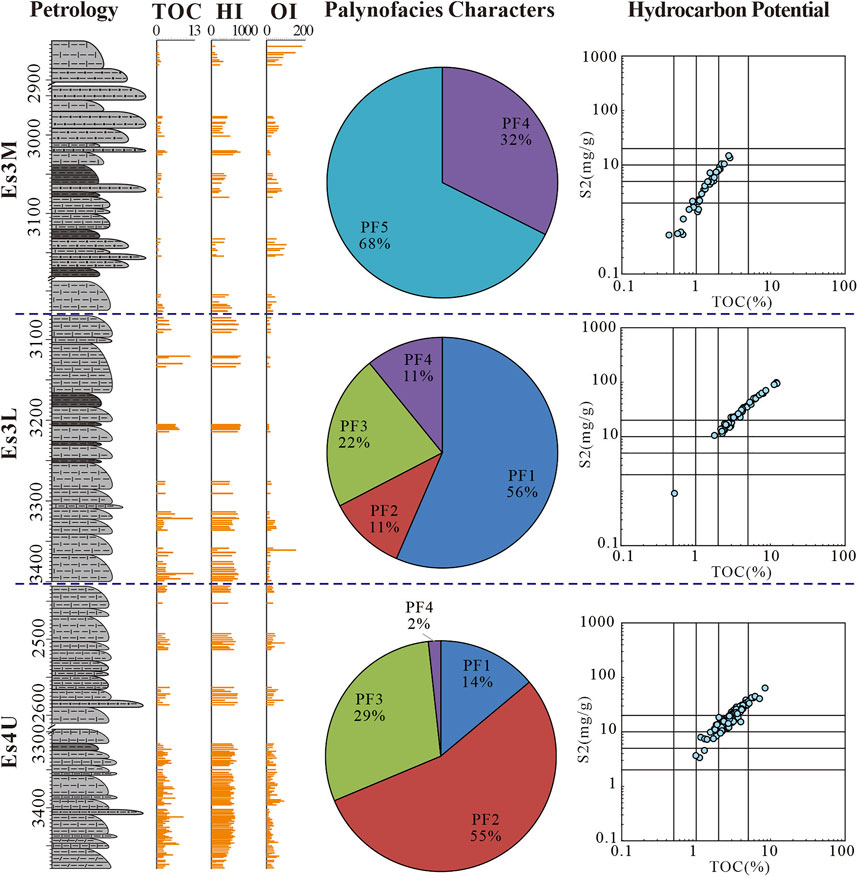
FIGURE 12. Composite histogram of pyrolysis parameter, palynofacies composition and hydrocarbon generation of the middle Es3- upper Es4 submeber in the Dongying Sag.
Among the 37 samples from middle Es3 submember, 68% are PF5-type OM assemblages, and 38% are PF4-type palynofacies. The average TOC of the rocks is 1.40%, the HI and OI values are 273 mg/g and 57 mg/g, respectively, and the kerogen is mainly types II-III.
The PF1 palynofacies assemblage (56%) is found in the 46 rock samples from lower Es3 submember, while the PF3 palynofacies assemblage accounts for 22%, and the PF2 and PF4 palynofacies assemblages are both 11%. The average TOC of OM is 4.6%; the average HI and OI values are 624 mg/g and 19 mg/g, respectively; and the kerogen is types I-II.
Among the 115 samples from the upper Es4 submember, more than 55% are the PF2 palynofacies assemblage; PF3 and PF1 assemblages are 29% and 14%, respectively; and the PF4 palynofacies assemblage is 4%. The average TOC of OM is 3.01%; the HI and OI values are 528 mg/g and 32 mg/g, respectively; and the kerogen type is types I-II, but predominantly type II.
According to the analyses of the palynofacies assemblage and hydrocarbon generation potential, the types and genesis of middle Es3 submember to upper Es4 submember palynofacies in the Dongying Sag are obviously different. The palynofacies assemblages of middle Es3 submember are mainly PF4 and PF5, indicating that the lake was relatively shallow and contained abundant exogenous inputs during the depositional period of this layer. OM from land sources and macrophytes growing in shallow water were gathered. Mechanical deposition played a leading role in the deposition of OM, leading to the weak hydrocarbon generation capacity of the rock strata. The lower Es3submember is rich in the PF1 and PF3 palynofacies assemblages, indicating that the water in this layer was stable and that there were a large number of self-growing organisms in the water. These OMs were deposited by biological action, and the hydrocarbon generation capacity of the layer was very good. The number of palynofacies assemblages in the upper Es4 submember is significantly larger than that in the other subsections, reflecting the stable water environment where organic aggregates were concentrated. In this depositional period, the primary productivity of water bodies was lower than that of the lower Es3 submember depositional period, and OM mainly reached the bottom by chemical flocculation. The pyrolysis parameters show that the hydrocarbon generation capacity of the section is slightly lower than that of lower submember, but it still shows good source rock characteristics. This is consistent with the previous understanding of each section and the evolutionary characteristics of the sedimentary environment. Thus, the types of OM in different lake environments are very different; these OM types reach the water bottom through mechanical, biological and chemical sedimentation, and the difference in these OM types also affects the formation and hydrocarbon generation capacity of mudrocks. Therefore, the accurate analysis of the source and deposition process of sedimentary OM in different lake environments is extremely important for a deep understanding of the formation process of mudrocks and can also provide a reliable basis for the evaluation of source rocks.
Conclusion
The lacustrine mudrocks of the Shahejie Formation in the Dongying Sag have abundant OM types. According to the source and deposition mechanism of OM, we delineate five palynofacies assemblages. PF1 and PF3 indicate a lake environment in which benthic microbial mats grew in abundance and a lake environment in which phytoplankton blooms occurred, respectively. PF2 reflects the deep-water lake environment in which all kinds of nonliving OM were enriched. PF4 represents an organic assemblage in a shallow water macroalgae-enriched lake environment. PF5 represents an OM assemblage in a shallow lacustrine environment near the provenance.
The pyrolysis analysis results indicate that the hydrocarbon generation capacity of the mudrocks is the strongest when the microbial mats grew abundantly and the planktonic algae bloomed. The hydrocarbon generation capacity of mudrocks deposited in lacustrine environments dominated by organic polymers is weaker. In the nearshore environment facies, the overall hydrocarbon generation capacity is weak, and the weakest hydrocarbon generation capacity occurs in the environment with abundant terrigenous clastic OM.
A comparison of the characteristics of palynofacies assemblages in each layer of the Dongying Sag revealed that during the depositional period of the upper Es4 submember- middle Es3 submember, the water depth transitioned from deep to shallow. During the sedimentary period of the upper Es4 and middle Es3 submembers, the aquatic OM in the lake was enriched, and the latter had higher lake productivity and better hydrocarbon generation capacity. During the sedimentary period of the middle Es3 submember, the lake was shallow, and the hydrocarbon generation capacity of the mudrocks was weak due to the influence of exogenous input. Therefore, it is of great significance to understand the formation process and hydrocarbon generation potential of lacustrine shale by paying attention to the OM source and hydrocarbon generation capacity.
Data availability statement
The original contributions presented in the study are included in the article/Supplementary Material, further inquiries can be directed to the corresponding author.
Author contributions
XZ: conceptualization, methodology, investigation, data curation, writing—original draft, writing—review and editing, visualization; JC: conceptualization, writing—review and editing, project administration, funding acquisition; XW: resources, data curation; XZ: resources, data curation; WZ: supervision, project administration; ZD: formal analysis, validation, investigation, visualization; LB: formal analysis, validation, investigation.
Funding
This work was financially supported by the National Natural Science Foundation of China (Grant number: 41972126), the National Oil and Gas Special Fund (Grant number. 2016ZX05006001-003) and Open Fund of Natural Gas Geology Key Laboratory of Sichuan Province (Grant number: 2021trqdz01).
Conflict of interest
Author Zhe Dong was employed by CNOOC China Ltd. Shanghai Branch.
The remaining authors declare that the research was conducted in the absence of any commercial or financial relationships that could be construed as a potential conflict of interest.
Publisher’s note
All claims expressed in this article are solely those of the authors and do not necessarily represent those of their affiliated organizations, or those of the publisher, the editors and the reviewers. Any product that may be evaluated in this article, or claim that may be made by its manufacturer, is not guaranteed or endorsed by the publisher.
Supplementary material
The Supplementary Material for this article can be found online at: https://www.frontiersin.org/articles/10.3389/feart.2022.936262/full#supplementary-material
References
Alam, M. A., Vandamme, D., Chun, W., Zhao, X., Foubert, I., Wang, Z., et al. (2016). Bioflocculation as an innovative harvesting strategy for microalgae. Rev. Environ. Sci. Biotechnol. 15, 573–583. doi:10.1007/s11157-016-9408-8
Batchelor, R. A. (2016). Microbial wrinkle structures in lower devonian shales, wormit, fife. Scott. J. Geol. 52, 65–67. 2015-006. doi:10.1144/sjg2015-006
Batten, D. (1996). “Palynofacies and palaeoenvironmental interpretation,” in Palynology: Principles and applications. Editors J. Jansonius, and D. C. McGregor (New York: AASP Fundation), 1011–1064.
Behling, H., Cohen, M. C. L., and Lara, R. J. (2001). Studies on holocene mangrove ecosystem dynamics of the bragança peninsula in north-eastern pará, Brazil. Palaeogeogr. Palaeoclimatol. Palaeoecol. 167, 225–242. doi:10.1016/s0031-0182(00)00239-x
Bellinger, E. G., and Sigee, D. C. (2011). “A key to the more frequently occurring freshwater algae,” in Freshwater algae: Identification and use as bioindicators. Editors E. G. Bellinger, and D. C. Sigee (Chichester: Wiley), 141–253.
Burdige, D. J. (2007). Preservation of organic matter in marine sediments: Controls, mechanisms, and an imbalance in sediment organic carbon budgets? Chem. Rev. 107, 467–485. doi:10.1021/cr050347q
Cai, J., Zeng, X., Wei, H., Song, M., Wang, X., and Liu, Q. (2019). From water body to sediments: Exploring the depositional processes of organic matter and their implications. J.Palaeogeog Chin. Ed. 21, 55–72. doi:10.7605/gdlxb.2019.01.003
Cai, J., Zhu, X., Zhang, J., Song, M., and Wang, Y. (2020). Heterogeneities of organic matter and its occurrence forms in mudrocks: Evidence from comparisons of palynofacies. Mar. Pet. Geol. 111, 21–32. doi:10.1016/j.marpetgeo.2019.08.004
Carvalho, M. d. A., Mendonça Filho, J. G., and Menezes, T. R. (2006). Palynofacies and sequence stratigraphy of the aptian–albian of the sergipe basin, Brazil. Sediment. Geol. 192, 57–74. doi:10.1016/j.sedgeo.2006.03.017
Deng, Q., Wang, H., Wei, Z., Li, S., Zhang, H., Liu, H., et al. (2021). Different accumulation mechanisms of organic matter in Cambrian sedimentary successions in the Western and northeastern margins of the Tarim Basin, NW China. J. Asian Earth Sci. 207, 104660. doi:10.1016/j.jseaes.2020.104660
El Atfy, H., Brocke, R., Uhl, D., Ghassal, B., Stock, A. T., and Littke, R. (2014). Source rock potential and paleoenvironment of the Miocene Rudeis and Kareem formations, Gulf of Suez, Egypt: An integrated palynofacies and organic geochemical approach. Int. J. Coal Geol. 131, 326–343. doi:10.1016/j.coal.2014.06.022
El Beialy, S. Y., El Atfy, H. S., Zavada, M. S., El Khoriby, E. M., and Abu-Zied, R. H. (2010). Palynological, palynofacies, paleoenvironmental and organic geochemical studies on the Upper Cretaceous succession of the GPTSW-7 well, North Western Desert, Egypt. Mar. Pet. Geol. 27, 370–385. doi:10.1016/j.marpetgeo.2009.10.006
Emmings, J. F., Hennissen, J. A., Stephenson, M. H., Poulton, S. W., Vane, C. H., Davies, S. J., et al. (2019). Controls on amorphous organic matter type and sulphurization in a Mississippian black shale. Rev. Palaeobot. Palynol. 268, 1–18. doi:10.1016/j.revpalbo.2019.04.004
Froidl, F., Littke, R., Baniasad, A., Zheng, T., Röth, J., Böcker, J., et al. (2021). Peculiar Berriasian “Wealden” Shales of northwest Germany: Organic facies, depositional environment, thermal maturity and kinetics of petroleum generation. Mar. Pet. Geol. 124, 104819. doi:10.1016/j.marpetgeo.2020.104819
Furota, S., Sawada, K., and Kawakami, G. (2021). Depositional processes of plant fragment-concentrated sandstones in turbiditic sequences recorded by plant biomarkers (Miocene Kawabata Formation, Japan). Int. J. Coal Geol. 233, 103643. doi:10.1016/j.coal.2020.103643
Garcia, Y. C., Martinez, J. I., Velez, M. I., Yokoyama, Y., Battarbee, R. W., and Suter, F. D. (2011). Palynofacies analysis of the late Holocene San Nicolás terrace of the Cauca paleolake and paleohydrology of northern South America. Palaeogeogr. Palaeoclimatol. Palaeoecol. 299, 298–308. doi:10.1016/j.palaeo.2010.11.010
Goth, K., De Leeuw, J., Püttmann, W., and Tegelaar, E. (1988). Origin of Messel oil shale kerogen. Nature 336, 759–761. doi:10.1038/336759a0
Guler, M. V., Lazo, D. G., Pazos, P. J., Borel, C. M., Ottone, E. G., Tyson, R. V., et al. (2013). Palynofacies analysis and palynology of the agua de la Mula member (agrio formation) in a sequence stratigraphy framework, lower cretaceous, neuquén basin, Argentina. Cretac. Res. 41, 65–81. doi:10.1016/j.cretres.2012.10.006
He, J., Ding, W., Jiang, Z., Jiu, K., Li, A., and Sun, Y. (2017). Mineralogical and chemical distribution of the Es3L oil shale in the Jiyang Depression, Bohai Bay Basin (E China): Implications for paleoenvironmental reconstruction and organic matter accumulation. Mar. Pet. Geol. 81, 196–219. doi:10.1016/j.marpetgeo.2017.01.007
Khan, I., Zhong, N., Luo, Q., Ai, J., Yao, L., and Luo, P. (2020). Maceral composition and origin of organic matter input in neoproterozoic–lower cambrian organic-rich shales of salt range formation, upper indus basin, Pakistan. Int. J. Coal Geol. 217, 103319. doi:10.1016/j.coal.2019.103319
Li, S., Pang, X., Li, M., and Jin, Z. (2003). Geochemistry of petroleum systems in the niuzhuang south slope of bohai bay basin—part 1: Source rock characterization. Org. Geochem. 34, 389–412. doi:10.1016/s0146-6380(02)00210-3
Li, D., Li, R., Zhu, Z., Wu, X., Cheng, J., Liu, F., et al. (2017). Origin of organic matter and paleo-sedimentary environment reconstruction of the Triassic oil shale in Tongchuan City, southern Ordos Basin (China). Fuel 208, 223–235. doi:10.1016/j.fuel.2017.07.008
Li, Y., Zeng, X., Cai, J., Wang, X., Mu, X., and Zhang, Y. (2021). Mudrocks lithofacies characteristics and north-south hydrocarbon generation difference of the Shahejie Formation in the dongpu sag. Miner. (Basel). 11, 535. doi:10.3390/min11050535
Li, Z. (2011). Exploration potential analyses of the shale gas in Dongying Depression. Chengdu: Chengdu University of Technology, 49–66.
Liu, H., Wang, Yong., Yang, Y., and Zhang, S. (2020). Sedimentary environment and lithofacies of fine-grained hybrid sedimentary in dongying sag: A case of fine-grained sedimentary system of the Es4. Earth Sci. 45 (10), 3543–3555. doi:10.3799/dqkx.2020.156
Ma, Y., Fan, M., Lu, Y., Liu, H., Hao, Y., Xie, Z., et al. (2017). Middle Eocene paleohydrology of the Dongying Depression in eastern China from sedimentological and geochemical signatures of lacustrine mudstone. Palaeogeogr. Palaeoclimatol. Palaeoecol. 479, 16–33. doi:10.1016/j.palaeo.2017.04.011
Martín-Closas, C., Permanyer, A., and Vila, M.-J. (2005). Palynofacies distribution in a lacustrine basin. Geobios 38, 197–210. doi:10.1016/j.geobios.2003.09.007
Mathews, R. P., Singh, B. D., Singh, V. P., Singh, A., Singh, H., Shivanna, M., et al. (2020). Organo-petrographic and geochemical characteristics of Gurha lignite deposits, Rajasthan, India: Insights into the palaeovegetation, palaeoenvironment and hydrocarbon source rock potential. Geosci. Front. 11, 965–988. doi:10.1016/j.gsf.2019.10.002
Passow, U. (2002). Transparent exopolymer particles (TEP) in aquatic environments. Prog. Oceanogr. 55, 287–333. doi:10.1016/s0079-6611(02)00138-6
Peters, K. (1986). Guidelines for evaluating petroleum source rock using programmed pyrolysis. AAPG Bull. 70 (3), 318–329. doi:10.1306/94885688-1704-11D7-8645000102C1865D
Prieto-Barajas, C. M., Valencia-Cantero, E., and Santoyo, G. (2018). Microbial mat ecosystems: Structure types, functional diversity, and biotechnological application. Electron. J. Biotechnol. 31, 48–56. doi:10.1016/j.ejbt.2017.11.001
Romankevich, E. A. (1984). Geochemistry of organic matter in the ocean Berlin: Springer-Verlag, 334.
Schieber, J., Southard, J. B., and Schimmelmann, A. (2010). Lenticular shale fabrics resulting from intermittent erosion of water-rich muds—interpreting the rock record in the light of recent flume experiments. J. Sediment. Res. 80, 119–128. doi:10.2110/jsr.2010.005
Schieber, J., Bose, P. K., Eriksson, P., Banerjee, S., Sarkar, S., Altermann, W., et al. (2007). Atlas of microbial mat features preserved within the siliciclastic rock record. Amsterdam, The Netherlands: Elsevier, 117–135.
Sebag, D., Copard, Y., Di-Giovanni, C., Durand, A., Laignel, B., Ogier, S., et al. (2006). Palynofacies as useful tool to study origins and transfers of particulate organic matter in recent terrestrial environments: Synopsis and prospects. Earth. Sci. Rev. 79, 241–259. doi:10.1016/j.earscirev.2006.07.005
Singh, H., and Mahesh, S. (2015). Palynofacies characterization for evaluation of hydrocarbon source rock potential of Lower Paleogene (Thanetian-Ypresian) sub-surface sediments of Barmer Basin, Western Rajasthan, India. Mar. Pet. Geol. 59, 442–450. doi:10.1016/j.marpetgeo.2014.10.001
Suárez-Ruiz, I., Flores, D., Mendonça Filho, J. G., and Hackley, P. C. (2012). Review and update of the applications of organic petrology: Part 1, geological applications. Int. J. Coal Geol. 99, 54–112. doi:10.1016/j.coal.2012.02.004
Tian Yang, T., Cao, Y., Friis, H., Wang, Y., and Zhou, L. (2018). Diagenetic evolution and chemical changes of deep-water mudstones of Shahejie Formation in the dongying sag, jiyang depression, eastern China. Mar. Pet. Geol. 93, 14–32. doi:10.1016/j.marpetgeo.2018.02.005
Turner, J. T. (2002). Zooplankton fecal pellets, marine snow and sinking phytoplankton blooms. Aquat. Microb. Ecol. 27, 57–102. doi:10.3354/ame027057
Turner, J. T. (2015). Zooplankton fecal pellets, marine snow, phytodetritus and the ocean’s biological pump. Prog. Oceanogr. 130, 205–248. doi:10.1016/j.pocean.2014.08.005
Tyson, R. V. (1995). Sedimentary organic matter: Organic facies and palynofacies. Berlin/Heidelberg, Germany: Springer, 431–462.
Wang, G., Chang, X., Wang, T. G., and Simoneit, B. R. T. (2015). Pregnanes as molecular indicators for depositional environments of sediments and petroleum source rocks. Org. Geochem. 78, 110–120. doi:10.1016/j.orggeochem.2014.11.004
Wang, Y., Liu, H., Song, G., Hao, X., Zhu, D., and Zhu, D. (2018). Formation mechanism of carbonates in the lacustrine muddy shale and it implication for shale oil and gas: A case study of source rocks in member 4 and member 3 of Shahejie Formation, dongying sag. Petroleum Res. 3, 248–258. doi:10.1016/j.ptlrs.2018.06.005
Wingender, J., Neu, T. R., and Flemming, H. C. (2012). “What are bacterial extracellular polymeric substances?,” in Microbial extracellular polymeric substances: Characterization, structure and function. Editors J. Wingender, T. R. Neu, and H. C. Flemming (Springer Berlin Heidelberg), 1–15.
Xie, X., Snowdon, L. R., Volkman, J. K., Li, M., Xu, J., and Qin, J. (2020). Inter-maceral effects on hydrocarbon generation as determined using artificial mixtures of purified macerals. Org. Geochem. 144, 104036. doi:10.1016/j.orggeochem.2020.104036
Xie, X., Zhu, G., and Wang, Y. (2021). The influence of syngenetic hydrothermal silica fluid on organic matter preservation in lower Cambrian Niutitang Formation, South China. Mar. Pet. Geol. 129, 105098. doi:10.1016/j.marpetgeo.2021.105098
Xu, J., Pan, Z., Yang, Y., Zhu, Y., and Fan, N. (1997). On the paleogene microphytoplankton from the Shengli oil-bearing region, Shandong, China. Dongying: University of petroleum Press, 31–40.
Xuan Yang, X., Berthold, F., and Berglund, L. A. (2018). Preserving cellulose structure: Delignified wood fibers for paper structures of high strength and transparency. Biomacromolecules 19, 3020–3029. doi:10.1021/acs.biomac.8b00585
Zargari, S., Canter, K. L., and Prasad, M. (2015). Porosity evolution in oil-prone source rocks. Fuel 153, 110–117. doi:10.1016/j.fuel.2015.02.072
Zeng, X., Cai, J., Dong, Z., Wang, X., and Hao, Y. (2017). Sedimentary characteristics and hydrocarbon generation potential of mudstone and shale: A case study of middle submember of member 3 and upper submember of member 4 in Shahejie Formation in dongying sag. Acta Pet. Sin. 38, 31–43. doi:10.7623/syxb201701004
Zeng, X., Cai, J., Dong, Z., Bian, L., and Li, Y. (2018). Relationship between mineral and organic matter in shales: The case of Shahejie Formation, dongying sag, China. Miner. (Basel). 8, 222. doi:10.3390/min8060222
Zhang, S., Zhang, B., Bian, L., Jin, Z., and Chen, J. (2007). Xiamaling Formation oil shale deposits from red algae in 800 million years ago. Sci. China Earth Sci. 37, 636–643. doi:10.3969/j.issn.1674-7240.2007.05.007
Zhang, S., Zhang, L., Li, Z., and Hao, Y. (2012). Formation conditions of paleogene shale oil and gas in jiyang depression. Pet. Geol. Recovery Effic. 19, 1–5+111. doi:10.13673/j.cnki.cn37-1359/te.2012.06.001
Zhang, M., Ji, L., Wu, Y., and He, C. (2015). Palynofacies and geochemical analysis of the Triassic Yanchang Formation, Ordos Basin: Implications for hydrocarbon generation potential and the paleoenvironment of continental source rocks. Int. J. Coal Geol. 152, 159–176. doi:10.1016/j.coal.2015.11.005
Zhang, W., Cao, Q., Xu, G., and Wang, D. (2018). Flocculation–dewatering behavior of microalgae at different growth stages under inorganic polymeric flocculant treatment: The relationships between algal organic matter and floc dewaterability. ACS Sustain. Chem. Eng. 6, 11087–11096. doi:10.1021/acssuschemeng.8b02551
Keywords: lacustrine mudrocks, palynofacies, hydrocarbon generation, OM deposition process, Dongying Sag
Citation: Zeng X, Cai J, Wang X, Zhang X, Yan J, Zhu W, Dong Z and Bian L (2022) Research on the sedimentary characteristics of organic matter in lacustrine mudrocks and their hydrocarbon generation potential based on palynofacies analysis: Eocene shahejie formation, dongying sag. Front. Earth Sci. 10:936262. doi: 10.3389/feart.2022.936262
Received: 05 May 2022; Accepted: 22 July 2022;
Published: 26 August 2022.
Edited by:
Yu Song, China University of Geosciences Wuhan, ChinaReviewed by:
Sun Pingchang, Jilin University, ChinaSiding Jin, Chengdu University of Technology, China
Copyright © 2022 Zeng, Cai, Wang, Zhang, Yan, Zhu, Dong and Bian. This is an open-access article distributed under the terms of the Creative Commons Attribution License (CC BY). The use, distribution or reproduction in other forums is permitted, provided the original author(s) and the copyright owner(s) are credited and that the original publication in this journal is cited, in accordance with accepted academic practice. No use, distribution or reproduction is permitted which does not comply with these terms.
*Correspondence: Jingong Cai, amdjYWlAdG9uZ2ppLmVkdS5jbg==