- 1State Key Laboratory of Biogeology and Environmental Geology, China University of Geosciences Beijing, Beijing, China
- 2State Key Laboratory of Alpine Ecology, Institute of Tibetan Plateau Research, China Academy of Sciences, Beijing, China
- 3University of Chinese Academy of Sciences, Beijing, China
- 4Institute of Sedimentary Geology, Chengdu University of Technology, Chengdu, China
- 5CAS Center for Excellence in Tibetan Plateau Earth Sciences and Institute of Tibetan Plateau Research, Chinese Academy of Sciences, Beijing, China
Under the scenario of global warming, the response of greenhouse gas emissions from alpine wetlands remains unclear. In this study, fluxes of CO2 and CH4 were measured during daytime for the microtopographic features of hollows and hummocks in a wetland in the Tibetan Plateau under two elevated temperatures, increments of ∼1°C (T1 treatment) and ∼2°C (T2 treatment), during the growing season in 2019. The results showed that warming significantly increased the cumulative net ecosystem CO2 exchanges (NEE) for both microtopographic features in the wetland compared to the control due to a combination of the increased gross primary production (GPP) with an increase in ecosystem respiration (ER). Similarly, warming also increased cumulative CH4 emission significantly. The effect was stronger for T2 than that for T1 for all component fluxes (GPP, ER, NEE, and CH4). Generally, NEE and CH4 fluxes both rose at first and then decreased. NEE peaked at the end of July for both hollows and hummocks, while CH4 emissions peaked in the middle of August. The cumulative CH4 emissions from the hummocks were significantly higher than those of the hollows, and CH4 emissions under illumination were significantly higher than those in darkness, which may be caused by the irradiation-sensitive vegetable internal convective gas transport system which diffuses CH4 from the pedosphere. This study revealed that warming strengthened the function of the CO2 sink but also increased CH4 emissions from the alpine wetlands on the Qinghai–Tibet Plateau.
1 Introduction
Global warming has drawn increasing attention due to the frequency of climatic events, such as hurricanes, flooding, drought/heat waves, and wildfires (Matthews et al., 2017). Greenhouse gases (GHGs) largely contribute to global warming (Cookson et al., 2008; Hou et al., 2011; Tiemeyer et al., 2016; Wu et al., 2019; Günther et al., 2020). Carbon dioxide (CO2) and methane (CH4) are the most important greenhouse gases related to human activities, accounting for 76 and 16% of total anthropogenic GHG emissions, respectively (IPCC, 2014). Generally, wetlands act as important CO2 sinks (Mitra et al., 2005). Meanwhile, they are also a significant source of CH4, contributing 20%–39% of the annual global emissions (Laanbroek, 2009; Köhn et al., 2021; Yuan et al., 2022). Wetlands are estimated to store 15% of the total organic carbon in global terrestrial ecosystems (Liu and Zhou, 2012) and are sensitive to global changes in terms of warming, flooding, drying, and other consequences, which may facilitate the decomposition of organic carbon in wetlands (Tarnocai, 2006; Sun et al., 2022). For example, temperate forested wetlands in Xiaoxing’anling were generally carbon sinks, but there was an obvious spatial variation in the carbon storage of the ecosystems along a moisture gradient (Wang et al., 2021a). Numerous studies on CH4 and CO2 fluxes have been conducted on wetlands from temperate, boreal, and Arctic zones (Sullivan et al., 2008; Merbold et al., 2013; He et al., 2014; Kang et al., 2020). In arid-zone wetlands, a lack of precipitation and extreme temperature results in low nutrient inputs to the soil and low GHG emission (Carnell et al., 2018; Pasut et al., 2021). In addition, land management and atmospheric deposition can impact GHG emission (Cookson et al., 2008; Günther et al., 2020; Jurasinski et al., 2020). Northern boreal–Arctic ecosystems may particularly be sensitive to climate warming referring to methane emissions because thawing the permafrost promotes emission from gas hydrates and biological production of methane in soils with high carbon stocks (Isaksen et al., 2011; Van Huissteden et al., 2011). However, the effects of warming on the fluxes of CO2 and CH4 in alpine wetlands are not well understood, especially on the Qinghai–Tibet Plateau, where the climate conditions are complex and unique.
The Qinghai–Tibet Plateau is dubbed the “third pole,” and the rate of temperature increase is more than double that of other low-altitude areas (Zhou and Zhang, 2021). Thus, the wetlands on the Qinghai–Tibet Plateau have received extensive attention in terms of carbon cycling under global warming scenarios (Chen et al., 2008; Gao et al., 2011; Tian et al., 2015; Wei et al., 2015; Zhang et al., 2019). Additionally, wetlands are composed of two microtopographic features, hollows and hummocks (Shen et al., 2006). Hollows are flattened and usually below the water table during the growing season, while hummocks extend above the water table. Hummocks often have higher light availability, higher temperature, and lower soil moisture content and are thus higher above-ground plant biomass than in the hollows (Shen et al., 2006). The soil organic carbon concentration in hummocks is significantly higher than that in hollows (Wang et al., 2021b). Some researchers have studied the difference in GHG emissions from these two microtopographic features (Sullivan et al., 2008; Dinsmore et al., 2009; Lai, 2009). However, their responses to warming remain unknown. Here, we investigated the changes in CO2 and CH4 fluxes of hollows and hummocks under warming conditions in the alpine wetlands on the Qinghai–Tibet Plateau.
2 Methods
2.1 Study site
The study was carried out in a wetland at the Naqu Ecological Environment Observation Station (E91°12ʹ∼93°02ʹ, N30°31ʹ∼31°55ʹ) of the Tibetan Plateau Research Institute, Chinese Academy of Science. The type of the wetland is an alpine swamp meadow, with an altitude of 4,480 m and a soil type of inceptisol (Lin et al., 2020). Hollows comprise approximately 10∼20% of the surface area with 5–15 cm of standing water or ice and are dominated by the Carex community. Hummocks, elevated above the water table throughout the snow-free seasons, comprise approximately 80∼90% of the surface area and are dominated by the Tibetan Kobresia community. The above-ground biomass in hollows and in hummocks was estimated by using the empirical formula: biomass (g dry weight/m2) = 24.038 * height (cm) * coverage +0.1261 deduced from a bunch of height and weight measurements in 1 m * 1 m patches in the Qinghai–Tibet Plateau (Wang, 2011).
The site has a cold, alpine mountain climate with an average annual precipitation of 406.2 mm and a mean annual temperature of −2.1°C. The growing season is between the end of May and early October with more than 80% of the annual precipitation. The freezing season is between the end of October and early May, but the specific dates vary from year to year. July–August and January feature the highest and lowest mean temperatures, respectively. Observations were carried out through a vegetation period from May to October in 2019.
2.2 Setup of warming devices
IPCC suggested a global warming threshold of 1.5°C above pre-industrial levels. In order to evaluate the effect of global warming of 1.5°C on the GHG emission from the Tibetan wetlands, two warming treatments, designed to warm 1°C (T1) and 2°C (T2) for soil temperature according to our pilot study, as well as a warming control (with no warming device), were conducted for hollows and hummocks in the study area (Figure 1). The warming treatments were permanently set up with passive solar hexagon open-top chamber (OTC) warming devices. The OTC devices were constructed with polymeric methyl methacrylate beads with a light transmittance of 95% according to previous publications (Godfree et al., 2011; Li et al., 2020). Specifically, the bottom edges of the T1 and T2 devices were 75.1 and 86.7 cm, respectively. The top edges of both devices were the same, 57.7 cm. The heights of the T1 and T2 devices were 60 and 100 cm, respectively. The bottom edges were tightly embedded in the soil. Two small fans were installed in the chambers to mix the air. All treatments were repeated at four different sites for both hollows and hummocks. In order to reduce the effects of different patches on the GHG emission, warming devices were adjacently distributed for hollows and hummocks. At some sites, PVC pipes were set up to observe the dynamic changes of the water table. A portable soil temperature and soil-moisture recorder (RHD-13, Handan Ruihua Electronics Co., Ltd., Handan, China) was used to observe the soil temperature and moisture at 5-cm depth when measuring GHGs.
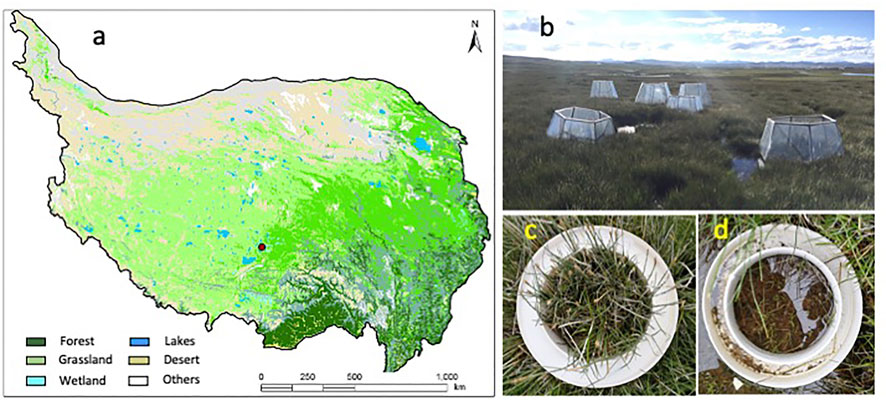
FIGURE 1. Study site on the Qinghai–Tibet Plateau and setup of OTC on hummocks and hollows. (A) Map of the Qinghai–Tibet Plateau (dot denotes the study site). (B) Setup of the OTC. (C) Hummock with the base for gas measurement. (D) Hollow with the base for gas measurement.
2.3 Gas flux measurements
During the growing season (May to October) in 2019, CO2 and CH4 fluxes were measured with a portable greenhouse gas analyzer (LGR-UGGA 915-0011, ABB Inc., Quebec, Canada) every 1–2 weeks, when the weather was sunny with few clouds. The measurements were conducted between 09:00 a.m. and 11:00 a.m. to better represent a daily mean value (Xu and Qi, 2001). The measuring duration lasted for 2 min each time, and the instrument automatically recorded data every 1 s. The CO2 and CH4 concentrations, as well as the air chamber temperature and atmospheric pressure, were recorded as air passed through the analyzer. The flux of CO2 under light conditions represented the net ecosystem carbon exchange (NEE). The flux of CO2 in darkness, as measured with a black cloth covering the OTC device, represented ecosystem respiration (ER), which was measured directly after the NEE measurements for each treatment. To avoid the influence of operation, the data recorded in the first 25 s and the last 25 s were excluded. NEE and ER were calculated with CO2 concentrations under light and darkness, respectively, with the following formula.
Fc: flux of CH4, NEE, or ER (μmol·m−2·s−1); V: volume of the assimilation chamber (m3); Wav: partial pressure of water in the chamber during the measurement (mmol·mol−1); Pav: the average atmospheric pressure (KPa) in the chamber during the measurement; R: the atmospheric constant (8.314 J mol−1·K−1); A: the area of the base (m2); Tav: the average temperature in the chamber during the measurement (°C); and dC/dt: the rate of change of dry CO2 and CH4 concentrations in the chamber with time.
A positive value indicates that the ecosystem is in carbon release (carbon source), and a negative value indicates that the ecosystem is in carbon absorption (carbon sink). Gross primary production (GPP) was calculated by subtracting ER with NEE with a black cloth covering the outsides of the chambers. CH4 fluxes were also measured simultaneously.
2.4 Statistical analysis
The experimental data were statistically analyzed by SPSS 16.0 statistical software. The paired sample t-test was used to analyze the significance of carbon flux differences among various warming treatments. Pearson correlation was used to analyze the correlation between the carbon flux and environmental parameters. Correlations and differences were considered to be significant at a level of p < 0.05.
3 Results
3.1 Variation in microclimate
During the growing season, soil temperatures at the 5-cm depth in hollows averaged 7.32°C in control treatments and 7.94 and 8.40°C in T1 and T2, which were 0.62 and 1.08°C higher than the control treatments, respectively (Figure 2A). In hummocks, soil temperatures at the 5-cm depth averaged 8.54°C in control treatments and 9.38°C and 10.85°C over the growing season for T1 and T2, which were 0.84 and 2.31°C higher than the control treatments, respectively (Figure 2B). Naturally, the soil temperature of the hummocks was warmer than that of the hollows. In contrast, the changes of the temperature in warming chambers recorded from 10:30 a.m. to 1:00 p.m. were largely dependent on the measuring time, other than the different treatments (Supplementary Figure S1).
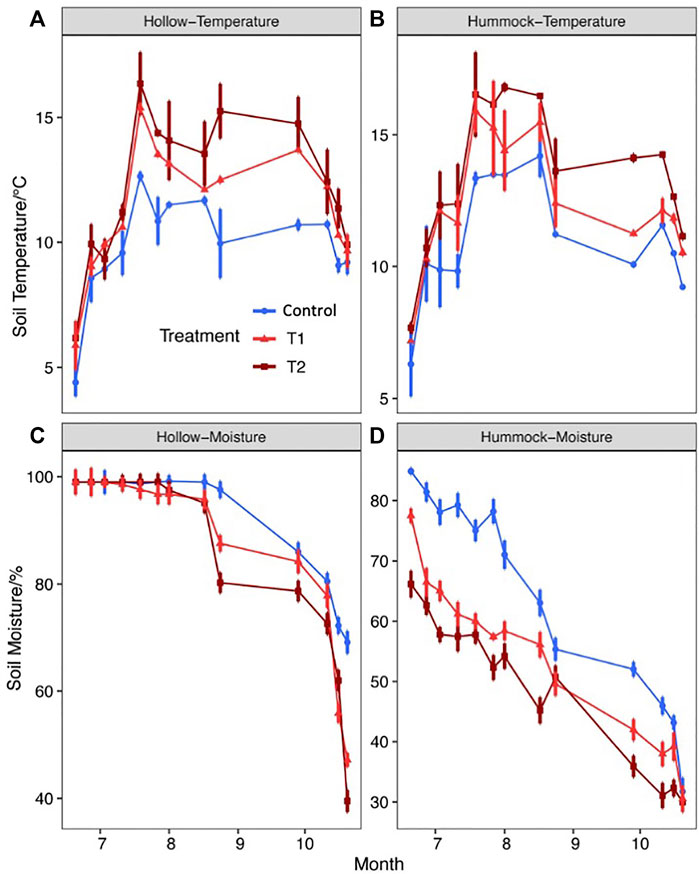
FIGURE 2. Effects of global warming on the soil temperature and soil moisture during the growing season. (A) Variation in the soil temperature of hollows. (B) Variation in soil moisture of hollows. (C) Variation in the soil temperature of hummocks. (D) Variation in soil moisture of hummocks.
For most cases, the hummocks elevated above the water table for 0–25 cm, and the hollows were logged in water (Supplementary Figure S2). The water table obviously declined in October. Warming treatments did not change the water table under the chambers significantly. However, soil moisture at the 5-cm depth decreased significantly with warming treatment (Figures 2C,D). During the peak growing season (from mid-June to mid-August), the hollows were flooded, and the soil moisture was saturated (Figure 2C). For hummocks, the warming treatments significantly reduced the soil moisture throughout the growing season (p < 0.05). The average soil moisture under the control treatment was 58.7%, but it decreased to 54.1 and 50.8% under warming treatments in T1 and T2, respectively (Figure 2D). On the whole, the average values of the above-ground biomass from hummocks were higher than those from the hollows (Supplementary Figure S3). The average values of the above-ground biomass under warming treatments (H1 and H2) were higher than those under control conditions. However, there were no significant differences among the treatments for both hummocks and hollows across the growing season.
3.2 CO2 fluxes in response to warming
NEE refers to the net flux of CO2 between the ecosystem and atmosphere. Since we follow the atmospheric sign convention, negative NEE fluxes denote an uptake by the ecosystem whereas positive fluxes denote emissions. The average values of NEE in the daytime were all negative and significantly different from each other among the different warming treatments (including control, T1, and T2 treatments) for both hollows and hummocks (Figure 3A). Among them, the respective average NEE values of hollows under the control, T1, and T2 treatments were −1.82, −3.11, and −6.05 μmol m−2·s−1, respectively. The respective average NEE values of hummocks under the control, T1, and T2 treatments were −18.92, −26.26, and −28.61 μmol m−2·s−1, respectively (Figure 3A). CO2 fluxes in darkness represent ecosystem respiration (ER). Within the same microtopographic features, ER values under warming treatments were significantly higher than those from control treatments, but no significant differences were observed between the two warming treatments (Figure 3B). Specifically, the average ER values of the hollows under the control, T1, and T2 treatments were 5.42, 6.39, and 7.13 μmol m−2·s−1, respectively, while for hummocks, they were 12.80, 13.98, and 14.73 μmol m−2·s−1, respectively (Figure 3C). Like NEE, ER values from hummocks were also significantly higher than those from hollows. The differences between NEE and ER fluxes represent the uptake by the ecosystem, i.e., the gross primary production (GPP). The average GPP values of hollows under the control, T1, and T2 treatments were 7.24, 9.51, and 13.18 μmol m−2·s−1, respectively. The respective average GPP values of hummocks under the control, T1, and T2 treatments were 31.72, 40.23, and 43.34 μmol m−2·s−1, respectively. Generally, NEE, ER, and GPP values from hummocks were all significantly higher than those from hollows.
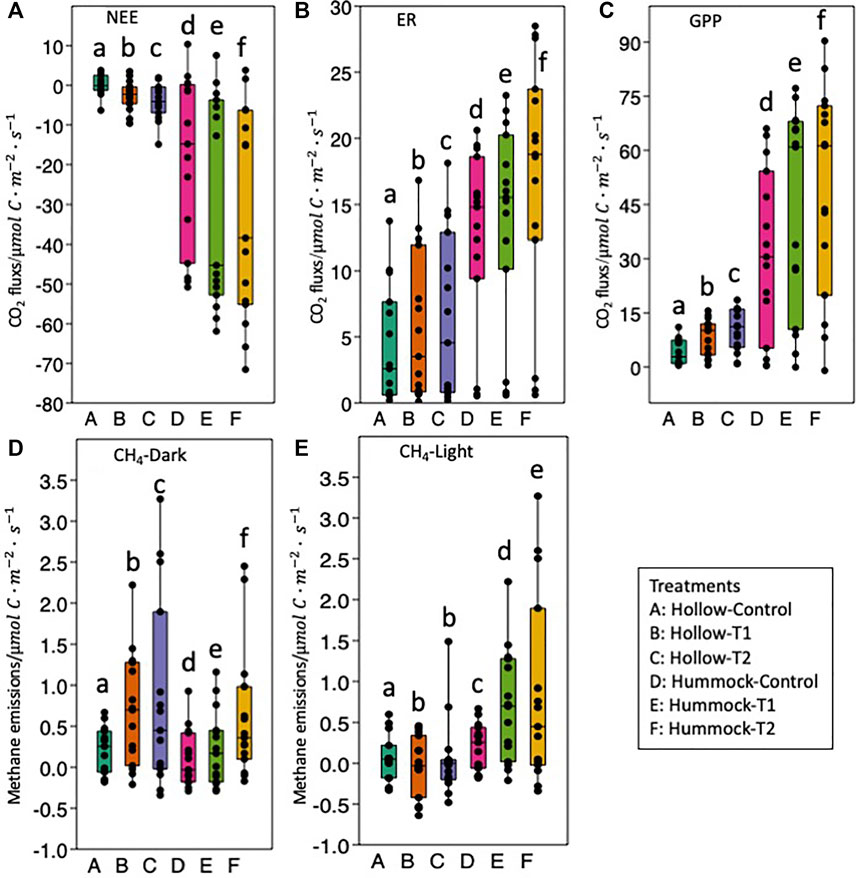
FIGURE 3. Variation in carbon fluxes from hollows and hummocks in the alpine wetlands during the growing season. (A) Net ecosystem carbon exchange (NEE). (B) Ecosystem respiration (ER). (C) Gross ecosystem photosynthesis (GPP). (D) CH4 fluxes measured under the dark condition. (E) CH4 fluxes measured under the light condition. For each analyte, bars with the same letters were not significantly different (p > 0.05, paired sample t-test).
Over the course of time, the varying patterns of NEE, ER, and GPP differed greatly (Figure 4). NEE reached their largest fluxes in late July for both hummocks and hollows (Figures 4A,B). Positive values of NEE from hollows during May and June suggested the sites were CO2 sources in this period. ER values in hummocks and hollows were the greatest during the first half of the growing season from May to July (Figures 4C,D). The fluxes of GPP reached their greatest values during late June in the hollows (Figure 4A) and during late July in the hummocks (Figure 4B). According to the average time course of CO2 fluxes (Figures 3, 4), the warming treatments greatly increased CO2 and its component fluxes of both hummocks and hollows.
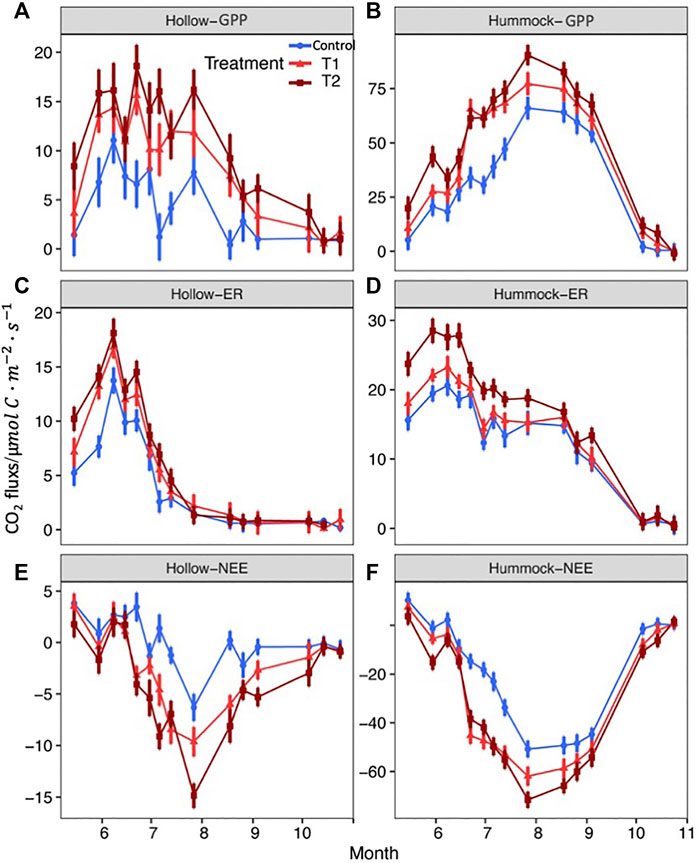
FIGURE 4. Seasonal dynamic changes in the carbon fluxes from hollows and hummocks. GPP of hollows (A) and hummocks (B). ER of hollows (C) and hummocks (D). NEE of hollows (E) and hummocks (F).
3.3 CH4 fluxes in response to warming
Warming also significantly increased the average CH4 emissions throughout the growing season relative to the control treatment for both hollows and hummocks under both light and dark conditions (Figures 3D,E). Generally, the fluxes of CH4 under the light condition were higher than those under the dark condition. The fluxes of CH4 from hummocks were higher than those of hollows. With warming treatments, the average fluxes during the growing season increased from 0.76 μmol m−2·s−1 (control treatment) to 1.33 μmol m−2·s−1 (T2 treatment) for the hollows with light and from 0.11 μmol m−2·s−1 (control treatment) to 0.62 μmol m−2·s−1 (T2 treatment) for the hollows in darkness. For the hummocks, it increased from 1.38 μmol m−2·s−1 (control treatment) to 3.65 μmol m−2·s−1 (T2 treatment) with light and from 0.20 μmol m−2·s−1 (control treatment) to 0.85 μmol m−2·s−1 (T2 treatment) in darkness.
Interestingly, the CH4 emissions exhibited different temporal variation patterns (Figure 5). At the first stage, before the end of June, CH4 emissions from hollows were all around 0 μmol m−2·s−1 for the different warming treatments, regardless of the lighting condition. Then, fluxes increased rapidly for the warming treatments with light but increased only moderately over the same time period for the warming treatments when measuring in darkness (Figures 5A,C). The fluxes reached the highest for the hollows at the end of August then decreased rapidly for the measurements with light and decreased more moderately for the dark measurements. For the hummocks, the variation patterns of the CH4 fluxes in darkness were similar to those for the hollows in darkness. The CH4 fluxes for the hummocks in light increased with fluctuations from early May to the end of August and then decreased gradually (Figures 5B,D).
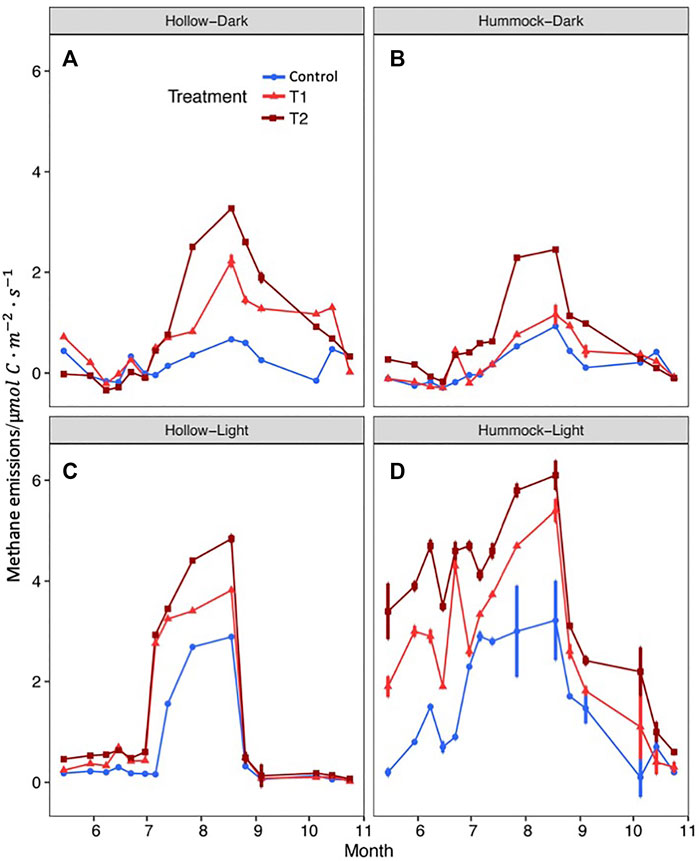
FIGURE 5. Seasonal dynamic changes in the CH4 flux from hollows and hummocks. (A) CH4 flux of hollows measured under the dark condition. (B) CH4 flux of hummocks measured under the dark condition. (C) CH4 flux of hollows measured under the light condition. (D) CH4 flux of hummocks measured under the light condition.
3.4 Correlations of fluxes of CH4 and CO2 with environmental factors
The correlations of fluxes of CH4 and CO2 and environmental factors were evaluated (Table 1). During the growing season, GPP, ER, and NEE of hollows were all significantly correlated with the soil temperature and soil moisture. GPP, ER, and NEE of hummocks were all significantly correlated with the soil temperature and above-ground biomass. Additionally, the ER of hummocks was also significantly correlated with soil moisture. CH4 emissions from both hollows and hummocks were significantly correlated with soil temperature and above-ground biomass, regardless of the lighting condition. However, the CH4 emissions from hummocks in light were also significantly correlated with soil moisture (Table 1).
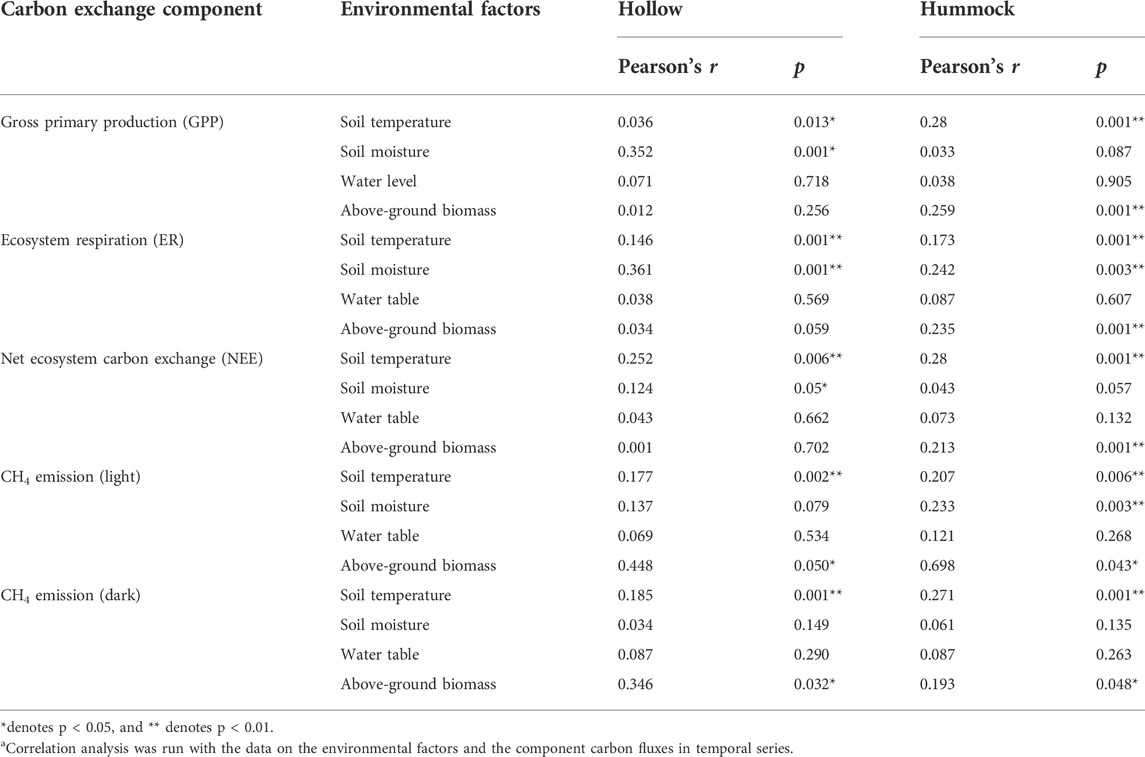
TABLE 1. Correlation analysis between soil environmental factors and carbon fluxes of hollows and hummocksa.
4 Discussion
4.1 Effects of warming on the increase of CO2 fluxes
Our observations of CO2 fluxes demonstrate that all the components of these CO2 fluxes in the wetland were highly sensitive to changes in temperature. GPP is realized by plants. It is well-known that global warming has a positive effect on GPP, especially for alpine environments where temperature is a limiting factor for GPP (Xia et al., 2009; Peng et al., 2014). ER is mainly contributed by both plants and microbes. Higher temperature also has a positive effect on ER from plants and microbes (Atkin et al., 2000; Wang et al., 2014). The size of NEE is jointly determined by the size of GPP and ER. In our study, NEE increased with global warming which suggested that the increase in GPP was greater than that in ER under global warming. A previous study in the alpine meadows of the Qinghai–Tibet Plateau also found the similar results, i.e., NEE increased under warming conditions (Peng et al., 2014).
Our study also showed increases in the soil temperature accelerated the loss of soil moisture (Figure 2). The decrease in soil moisture will inevitably affect root respiration, microbial community composition, and plant physiology and thus affect the global carbon cycle (Selsted et al., 2012; Xu et al., 2014). Specifically, moisture determines the soil’s redox potential, i.e., decrease in soil moisture will increase O2 concentrations and redox potential of the soil (Dinsmore et al., 2009; Rubol et al., 2012; Esch et al., 2016). In wetlands where water is not a limiting factor for microbes and plants, an increase in O2 concentration induced by a decrease in soil moisture might increase both GPP and ER. A previous study showed that insignificant changes in GPP and ER resulted in a consequently small NEE response under global warming in a semi-arid temperate steppe in northern China (Xia et al., 2009). In the arid environment, warming reduced soil moisture to a certain extent, resulting in weakening of the plant and soil microbial respiration, which inhibited plant stomatal conductance, reduced plant photosynthesis, and affected NEE (Guo et al., 2011). These contrasting findings with the results from this study highlight the importance of soil moisture in mediating the response of NEE to global warming.
Distinct temporal variations were also obvious in NEE, ER, and GPP fluxes along with the soil moisture and soil temperature. During the early growing season, the snow did not totally melt and soil temperature was a little above 0oC (Figure 2). Low temperature inhibits the activity of microorganisms (Liu et al., 2009) and affects vegetation respiration (Hicks Pries et al., 2015). During this period, soil temperature was the main factor affecting GPP and ER. Consequently, warming promoted GPP and ER, as well as NEE. From July to early September, the weather of the Tibetan Plateau is controlled by the Indian Ocean monsoon with optimal temperature and precipitation (Hou et al., 2014). The amount of precipitation and cloudy days increase in comparison with those of the former period. Vigorous vegetation growth contributed to high GPP and NEE under warm air temperature (Supplementary Figure S1). During this period, soil temperature still tends to be the most important environmental factor that affects ER. In September and October, plants enter the wilting period (Supplementary Figure S3), and photosynthesis weakens, resulting in a decrease of NEE for both hollows and hummocks.
4.2 Effect of warming and seasonal change on CH4 fluxes
Our study showed that warming significantly increased CH4 emission from the wetlands for both hollows and hummocks, regardless of the lighting condition. Increase in CH4 emission was also observed from microcosms with soil from the Zoige wetland in the Tibetan Plateau under warming conditions (Cui et al., 2015). CH4 emission is determined by the balance between methanogenesis and methanotrophy, which is affected by various environmental conditions (Serrano-Silva et al., 2014). A previous study showed that high temperature increased methanogenesis and decreased CH4 oxidation in rice soils (Das and Adhya, 2012). Rise in temperature potentially leads to decrease in the soil redox potential, which favors methanogenesis but inhibits the activity of CH4-oxidizing bacteria (Das and Adhya, 2012). Such contrasting effects on methanogenesis and methanotrophy necessarily resulted in the fact that warming increases CH4 emission, which was consistent with our observations. The significant correlation between the CH4 flux and soil temperature (Table 1) suggested that temperature was one of the controlling factors affecting the CH4 flux in the alpine wetlands on the Tibetan Plateau.
The higher flux of CH4 emissions with light than those in darkness may be caused by vegetable internal convective gas transport which was reported to quickly respond to changes in irradiation (Günther et al., 2014). Many plants, such as Typha, Phragmites, Kobresia, and Carex dominating the wetland in this study, have been found to transport CH4 from the pedosphere to the air through aerenchyma (Morrissey et al., 1993; Yavitt and Knapp, 1998; Beckett et al., 2001; Cao et al., 2008). By this way, CH4 escapes from oxidation in aerobic peat layers (Günther et al., 2014). Notably, the internal convective gas transport is sensitive to irradiation through stomatal control (Morrissey et al., 1993; Günther et al., 2014). So, our findings about the differences of CH4 emission in darkness and light were largely due to the stomatal control under different light conditions.
Our study also showed that the fluxes of CH4 exhibited a temporal pattern. The peak CH4 emission in the two microtopographic features of the wetland in this study appeared in summer, consistent with previous research results in other wetlands (Dise, 1993; Chen et al., 2008; He et al., 2014; Wei et al., 2015). Beyond the fact that the temperature is optimal for CH4 emission in summer as discussed previously, summer is also the optimal period for vegetation growth. A high input of fresh organic carbon to the soil resulting from vigorous vegetation growth will fuel methanogenesis (Serrano-Silva et al., 2014). The significant correlation between the CH4 flux and above-ground biomass also explained such seasonal changes in the flux of CH4 emission.
Microtopography also influenced CH4 fluxes, i.e., the CH4 fluxes of hummocks were higher than those of hollows, which may be attributed to two reasons. First, hummocks grow greater amounts of above-ground biomass than hollows, which will allocate more fresh organic carbon to the pedosphere and facilitate methanogenesis (Serrano-Silva et al., 2014). Second, high moisture or the water table affects CH4 fluxes. The wetland of this study was recharged with melt water, which was dynamically oxic. As a result, oxic water may inhibit methanogenesis and promote CH4 oxidation.
According to our study, global warming strengthens the CO2-sinking effect in the daytime and also increases CH4 emission from the Tibetan wetlands. Warming for 1–2 oC increased NEE by an average of 7.3–9.7 μmol m−2·s−1 under sunlight during the growing season for hummocks contrary to the control conditions and 1.3–4.2 μmol m−2·s−1 for hollows. The values for CH4 emission were lower than these NEE values, which were 1.3–2.3 μmol m−2·s−1 and 0.35–0.57 μmol m−2·s−1, respectively. However, CH4 possesses a higher global warming potential (GWP), which is about 28 times higher than that of CO2 (IPCC, 2014). From this aspect, an increase in CH4 emissions would bring 3.8 to 7.5 times the warming effect contrast to NEE in the daytime during the growing season.
5 Conclusion
Our study showed that warming significantly increased the CO2 and CH4 fluxes of hollows and hummocks in the alpine wetlands on the Qinghai–Tibet Plateau. The CO2 and CH4 fluxes of hummocks were always higher than those of hollows under the two warming treatments. During the course of the growing season, the GPP of hollows and hummocks first increased and then declined. NEE showed a U-shaped trend, and ER generally showed a downward trend. The difference between hollows and hummocks was that the peaks of GPP and NEE appeared at different times. The GPP, ER, and NEE of hollows and hummocks were positively correlated with the soil temperature, which may be the result of the optimal temperature promoting the growth of vegetation. According to CO2 fluxes, warming effectively strengthens the carbon sink function in the alpine wetland. However, warming also significantly increased methane emissions in the wetland. Taking the warming potential into account, the GWP brought by the increase of CH4 emissions was 3.8–7.5 times that of the GWP mitigated by CO2 uptake under sunlight in the growing season. Therefore, the study of the differential response of microtopographic carbon fluxes to warming in alpine wetland areas will help accurately assess and predict the carbon flux of alpine wetlands on the Qinghai–Tibet Plateau.
Data availability statement
The original contributions presented in the study are included in the article/Supplementary Material; further inquiries can be directed to the corresponding authors.
Author contributions
GZ, WH, and FL conceived the concept. AY and BN conducted data acquisition and analyses. AY, WH, and GZ developed the manuscript with contributions from all other co-authors.
Acknowledgments
This research was supported by grants from the Second Tibetan Plateau Scientific Expedition and Research Program (STEP) (2019QZKK0503) and the National Natural Science Foundation of China (41871066, 41471055, and 91851116). We thank the Naqu Alpine Grassland Ecosystem National Field Scientific Observation and Research Station for the field study.
Conflict of interest
The authors declare that the research was conducted in the absence of any commercial or financial relationships that could be construed as a potential conflict of interest.
Publisher’s note
All claims expressed in this article are solely those of the authors and do not necessarily represent those of their affiliated organizations, or those of the publisher, the editors, and the reviewers. Any product that may be evaluated in this article, or claim that may be made by its manufacturer, is not guaranteed or endorsed by the publisher.
Supplementary material
The Supplementary Material for this article can be found online at: https://www.frontiersin.org/articles/10.3389/feart.2022.935641/full#supplementary-material
References
Atkin, O. K., Edwards, E. J., and Loveys, B. R. (2000). Response of root respiration to changes in temperature and its relevance to global warming. New Phytol. 147 (1), 141–154. doi:10.1046/j.1469-8137.2000.00683.x
Beckett, P. M., Armstrong, W., and Armstrong, J. (2001). Mathematical modelling of methane transport by Phragmites: The potential for diffusion within the roots and rhizosphere. Aquat. Bot. 69 (2), 293–312. doi:10.1016/s0304-3770(01)00144-9
Cao, G., Xu, X., Long, R., Wang, Q., Wang, C., Du, Y., et al. (2008). Methane emissions by alpine plant communities in the Qinghai-Tibet Plateau. Biol. Lett. 4 (6), 681–684. doi:10.1098/rsbl.2008.0373
Carnell, P. E., Windecker, S. M., Brenker, M., Baldock, J., Masque, P., Brunt, K., et al. (2018). Carbon stocks, sequestration, and emissions of wetlands in south eastern Australia. Glob. Chang. Biol. 24 (9), 4173–4184. doi:10.1111/gcb.14319
Chen, H., Yao, S., Wu, N., Wang, Y., Luo, P., Tian, J., et al. (2008). Determinants influencing seasonal variations of methane emissions from alpine wetlands in Zoige Plateau and their implications. J. Geophys. Res. 113, D12303. doi:10.1029/2006jd008072
Cookson, W. R., Murphy, D. V., and Roper, M. M. (2008). Characterizing the relationships between soil organic matter components and microbial function and composition along a tillage disturbance gradient. Soil Biol. Biochem. 40 (3), 763–777. doi:10.1016/j.soilbio.2007.10.011
Cui, M., Ma, A., Qi, H., Zhuang, X., Zhuang, G., and Zhao, G. (2015). Warmer temperature accelerates methane emissions from the Zoige wetland on the Tibetan Plateau without changing methanogenic community composition. Sci. Rep. 5 (1), 11616. doi:10.1038/srep11616
Das, S., and Adhya, T. K. (2012). Dynamics of methanogenesis and methanotrophy in tropical paddy soils as influenced by elevated CO2 and temperature interaction. Soil Biol. Biochem. 47, 36–45. doi:10.1016/j.soilbio.2011.11.020
Dinsmore, K. J., Skiba, U. M., Billett, M. F., and Rees, R. M. (2009). Effect of water table on greenhouse gas emissions from peatland mesocosms. Plant Soil 318 (1-2), 229–242. doi:10.1007/s11104-008-9832-9
Dise, N. B. (1993). Methane emission from Minnesota peatlands: Spatial and seasonal variability. Glob. Biogeochem. Cycles 7 (1), 123–142. doi:10.1029/92gb02299
Esch, E. H., Lipson, D., and Cleland, E. E. (2016). Direct and indirect effects of shifting rainfall on soil microbial respiration and enzyme activity in a semi-arid system. Plant Soil 411 (1-2), 333–346. doi:10.1007/s11104-016-3027-6
Gao, J., Ouyang, H., Lei, G., Xu, X., and Zhang, M. (2011). Effects of temperature, soil moisture, soil type and their interactions on soil carbon mineralization in Zoigê alpine wetland, Qinghai-Tibet Plateau. Chin. Geogr. Sci. 21 (1), 27–35. doi:10.1007/s11769-011-0439-3
Godfree, R., Robertson, B., Bolger, T., Carnegie, M., and Young, A. (2011). An improved hexagon open-top chamber system for stable diurnal and nocturnal warming and atmospheric carbon dioxide enrichment. Glob. Change Biol. 17 (1), 439–451. doi:10.1111/j.1365-2486.2010.02276.x
Günther, A., Barthelmes, A., Huth, V., Joosten, H., Jurasinski, G., Koebsch, F., et al. (2020). Prompt rewetting of drained peatlands reduces climate warming despite methane emissions. Nat. Commun. 11, 1644. doi:10.1038/s41467-020-15499-z
Günther, A., Jurasinski, G., Huth, V., and Glatzel, S. (2014). Opaque closed chambers underestimate methane fluxes of Phragmites australis (Cav.) Trin. ex Steud. Environ. Monit. Assess. 186 (4), 2151–2158. doi:10.1007/s10661-013-3524-5
Guo, Y.-Q., Borjigidai, A., Gao, Q.-Z., Duan, M.-J., Ganzhu, Z., Wan, Y.-F., et al. (2011). Photosynthetic characteristics of Stipa purpurea under irrigation in northern Tibet and its short-term response to temperature and CO2 concentration. Chin. J. Plant Ecol. 35 (3), 311–321. doi:10.3724/sp.j.1258.2011.00311
He, G. X., Li, K. H., Liu, X. J., Gong, Y. M., and Hu, Y. K. (2014). Fluxes of methane, carbon dioxide and nitrous oxide in an alpine wetland and an alpine grassland of the Tianshan Mountains, China. J. Arid. Land 6 (6), 717–724. doi:10.1007/s40333-014-0070-0
Hicks Pries, C. E., van Logtestijn, R. S., Schuur, E. A., Natali, S. M., Cornelissen, J. H., Aerts, R., et al. (2015). Decadal warming causes a consistent and persistent shift from heterotrophic to autotrophic respiration in contrasting permafrost ecosystems. Glob. Chang. Biol. 21 (12), 4508–4519. doi:10.1111/gcb.13032
Hou, W., Dong, H., Li, G., Yang, J., Coolen, M. J., Liu, X., et al. (2014). Identification of photosynthetic plankton communities using sedimentary ancient DNA and their response to late-Holocene climate change on the Tibetan Plateau. Sci. Rep. 4, 6648. doi:10.1038/srep06648
Hou, W. G., Lian, B., and Zhang, X. Q. (2011). CO2 mineralization induced by fungal nitrate assimilation. Bioresour. Technol. 102 (2), 1562–1566. doi:10.1016/j.biortech.2010.08.080
IPCC (2014). Limate change 2014: Mitigation of climate change. New York, USA: Intergovernmental Panel on Climate Change.
Isaksen, I. S., Gauss, M., Myhre, G., Walter Anthony, K. M., and Ruppel, C. (2011). Strong atmospheric chemistry feedback to climate warming from Arctic methane emissions. Glob. Biogeochem. Cycles 25 (2), GB2002. doi:10.1029/2010gb003845
Jurasinski, G., Ahmad, S., Anadon-Rosell, A., Berendt, J., Beyer, F., Bill, R., et al. (2020). From understanding to sustainable use of peatlands: The WETSCAPES approach. Soil Syst. 4 (1), 14. doi:10.3390/soilsystems4010014
Kang, X., Li, Y., Wang, J., Yan, L., Zhang, X., Wu, H., et al. (2020). Precipitation and temperature regulate the carbon allocation process in alpine wetlands: Quantitative simulation. J. Soils Sediments 20 (9), 3300–3315. doi:10.1007/s11368-020-02643-x
Köhn, D., Welpelo, C., Günther, A., and Jurasinski, G. (2021). Drainage ditches contribute considerably to the CH4 budget of a drained and a rewetted temperate fen. Wetlands 41, 71. doi:10.1007/s13157-021-01465-y
Laanbroek, H. J. (2009). Methane emission from natural wetlands: Interplay between emergent macrophytes and soil microbial processes. A mini-review. Ann. Bot. 105 (1), 141–153. doi:10.1093/aob/mcp201
Lai, D. Y. F. (2009). Methane dynamics in northern peatlands: A review. Pedosphere 19 (4), 409–421. doi:10.1016/s1002-0160(09)00003-4
Li, C., Yang, Y., Li, X., Chen, Q., and Zhou, H. (2020). Effects of simulated climate warming and grazing on photosynthesis and respiration of permafrost meadow plant community. Russ. J. Ecol. 51 (3), 224–232. doi:10.1134/s1067413620030042
Lin, X., Dong, Q., Wang, P., Jiang, L., Hu, J., Wang, S., et al. (2020). Effects of nitrogen and phosphorus additions on arbuscular mycorrhizal fungi community in the alpine meadow in Qinghai-Tibetan plateau. J. South China Agric. Univ. 41 (2), 95–103. doi:10.7671/j.issn.1001-411X.201903017
Liu, C., and Zhou, W. (2012). Progress of research on carbon cycle of wetlands in China. Chin. J. Soil Sci. 43, 1264–1270. doi:10.1007/s11783-011-0280-z
Liu, J., Du, J., and Xie, X. (2009). Effect of drought and heat stress and rewatering on photosynthetic physioecological characteristics of Kentuckyturf grass. Acta Ecol. Sin. 29 (5), 2694–2700. doi:10.3321/j.issn:1000-0933.2009.05.061
Matthews, T. K., Wilby, R. L., and Murphy, C. (2017). Communicating the deadly consequences of global warming for human heat stress. Proc. Natl. Acad. Sci. U. S. A. 114 (15), 3861–3866. doi:10.1073/pnas.1617526114
Merbold, L., Steinlin, C., and Hagedorn, F. (2013). Winter greenhouse gas fluxes (CO<sub>2</sub>, CH<sub>4</sub> and N<sub>2</sub>O) from a subalpine grassland. Biogeosciences 10 (5), 3185–3203. doi:10.5194/bg-10-3185-2013
Mitra, S., Wassmann, R., and Vlek, P. L. G. (2005). An appraisal of global wetland area and its organic carbon stock. Curr. Sci. India 88 (1), 25–35.
Morrissey, L. A., Zobel, D. B., and Livingston, G. P. (1993). Significance of stomatal control on methane release from -dominated wetlands. Chemosphere 26 (1), 339–355. doi:10.1016/0045-6535(93)90430-d
Pasut, C., Tang, F. H. M., Hamilton, D. P., and Maggi, F. (2021). Carbon, nitrogen, and sulfur elemental fluxes in the soil and exchanges with the atmosphere in Australian tropical, temperate, and arid wetlands. Atmosphere 12 (1), 42. doi:10.3390/atmos12010042
Peng, F., You, Q. G., Xu, M. H., Guo, J., Wang, T., and Xue, X. (2014). Effects of warming and clipping on ecosystem carbon fluxes across two hydrologically contrasting years in an alpine meadow of the Qinghai-Tibet plateau. Plos One 9 (10), e109319. doi:10.1371/journal.pone.0109319
Rubol, S., Silver, W. L., and Bellin, A. (2012). Hydrologic control on redox and nitrogen dynamics in a peatland soil. Sci. Total Environ. 432, 37–46. doi:10.1016/j.scitotenv.2012.05.073
Selsted, M. B., van der Linden, L., Ibrom, A., Michelsen, A., Larsen, K. S., Pedersen, J. K., et al. (2012). Soil respiration is stimulated by elevated CO2 and reduced by summer drought: Three years of measurements in a multifactor ecosystem manipulation experiment in a temperate heathland (CLIMAITE). Glob. Chang. Biol. 18 (4), 1216–1230. doi:10.1111/j.1365-2486.2011.02634.x
Serrano-Silva, N., Sarria-GuzmÁN, Y., Dendooven, L., and Luna-Guido, M. (2014). Methanogenesis and methanotrophy in soil: A review. Pedosphere 24 (3), 291–307. doi:10.1016/s1002-0160(14)60016-3
Shen, H., Tang, Y., and Washitani, I. (2006). Morphological plasticity of Primula nutans to hummock-and-hollow microsites in an alpine wetland. J. Plant Res. 119 (3), 257–264. doi:10.1007/s10265-006-0269-z
Sullivan, P. F., Arens, S. J. T., Chimner, R. A., and Welker, J. M. (2008). Temperature and microtopography interact to control carbon cycling in a high arctic fen. Ecosystems 11 (1), 61–76. doi:10.1007/s10021-007-9107-y
Sun, H., Chen, Y., Xiong, J., Ye, C., Yong, Z., Wang, Y., et al. (2022). Relationships between climate change, phenology, edaphic factors, and net primary productivity across the Tibetan Plateau. Int. J. Appl. Earth Observation Geoinformation 107, 102708. doi:10.1016/j.jag.2022.102708
Tarnocai, C. (2006). The effect of climate change on carbon in Canadian peatlands. Glob. Planet. Change 53 (4), 222–232. doi:10.1016/j.gloplacha.2006.03.012
Tian, J. Q., Shu, C., Chen, H., Qiao, Y. C., Yang, G., Xiong, W., et al. (2015). Response of archaeal communities to water regimes under simulated warming and drought conditions in Tibetan Plateau wetlands. J. Soils Sediments 15 (1), 179–188. doi:10.1007/s11368-014-0978-1
Tiemeyer, B., Albiac Borraz, E., Augustin, J., Bechtold, M., Beetz, S., Beyer, C., et al. (2016). High emissions of greenhouse gases from grasslands on peat and other organic soils. Glob. Chang. Biol. 22, 4134–4149. doi:10.1111/gcb.13303
Van Huissteden, J., Berrittella, C., Parmentier, F., Mi, Y., Maximov, T., and Dolman, A. (2011). Methane emissions from permafrost thaw lakes limited by lake drainage. Nat. Clim. Chang. 1 (2), 119–123. doi:10.1038/nclimate1101
Wang, B., Mu, C., Lu, H., Li, N., Zhang, Y., and Ma, L. (2021a). Ecosystem carbon storage and sink/source of temperate forested wetlands in Xiaoxing’anling, northeast China. J. For. Res. (Harbin). 33, 839–849. doi:10.1007/s11676-021-01366-0
Wang, M., Wang, S., Cao, Y., Jiang, M., Wang, G., and Dong, Y. (2021b). The effects of hummock-hollow microtopography on soil organic carbon stocks and soil labile organic carbon fractions in a sedge peatland in Changbai Mountain, China. Catena 201, 105204. doi:10.1016/j.catena.2021.105204
Wang, X., Liu, L., Piao, S., Janssens, I. A., Tang, J., Liu, W., et al. (2014). Soil respiration under climate warming: Differential response of heterotrophic and autotrophic respiration. Glob. Chang. Biol. 20 (10), 3229–3237. doi:10.1111/gcb.12620
Wang, Z. (2011). Mechanisms for altitudinal variations in net primary productivity of alpine meadow in central Tibetan Plateau. Beijing: University of Chinese Academy of Sciences.
Wei, D., Tarchen, T., Dai, D., Wang, Y., and Wang, Y. (2015). Revisiting the role of CH4 emissions from alpine wetlands on the Tibetan plateau: Evidence from two in situ measurements at 4758 and 4320 m above sea level. J. Geophys. Res. Biogeosci. 120 (9), 1741–1750. doi:10.1002/2015jg002974
Wu, X. W., Zang, S. Y., Ma, D. L., Ren, J. H., Chen, Q., and Dong, X. F. (2019). Emissions of CO2, CH4, and N2O fluxes from forest soil in permafrost region of daxing'an mountains, northeast China. Int. J. Environ. Res. Public Health 16 (16), 2999. doi:10.3390/ijerph16162999
Xia, J., Niu, S., and Wan, S. (2009). Response of ecosystem carbon exchange to warming and nitrogen addition during two hydrologically contrasting growing seasons in a temperate steppe. Glob. Change Biol. 15 (6), 1544–1556. doi:10.1111/j.1365-2486.2008.01807.x
Xu, B.-X., Hu, Y.-G., Zhang, Z.-S., Chen, Y.-L., Zhang, P., and Li, G. (2014). Effects of experimental warming on CO2, CH4 and N2O fluxes of biological soil crust and soil system in a desert region. Chin. J. Plant Ecol. 38 (8), 809–820. doi:10.3724/sp.j.1258.2014.00076
Xu, M., and Qi, Y. (2001). Soil-surface CO2 efflux and its spatial and temporal variations in a young ponderosa pine plantation in northern California. Glob. Change Biol. 7 (6), 667–677. doi:10.1046/j.1354-1013.2001.00435.x
Yavitt, J. B., and Knapp, A. K. (1998). Aspects of methane flow from sediment through emergent cattail (Typha latifolia) plants. New Phytol. 139 (3), 495–503. doi:10.1046/j.1469-8137.1998.00210.x
Zhang, H., Yao, Z., Ma, L., Zheng, X., Wang, R., Wang, K., et al. (2019). Annual methane emissions from degraded alpine wetlands in the eastern Tibetan Plateau. Sci. Total Environ. 657, 1323–1333. doi:10.1016/j.scitotenv.2018.11.443
Keywords: warming, CO2, CH4, alpine wetland, microtopographic features
Citation: Yasin A, Niu B, Chen Z, Hu Y, Yang X, Li Y, Zhang G, Li F and Hou W (2022) Effect of warming on the carbon flux of the alpine wetland on the Qinghai–Tibet Plateau. Front. Earth Sci. 10:935641. doi: 10.3389/feart.2022.935641
Received: 04 May 2022; Accepted: 16 September 2022;
Published: 27 October 2022.
Edited by:
Evan S. Kane, Michigan Technological University, United StatesReviewed by:
Nina Welti, Commonwealth Scientific and Industrial Research Organisation (CSIRO), AustraliaGerald Jurasinski, University of Rostock, Germany
Copyright © 2022 Yasin, Niu, Chen, Hu, Yang, Li, Zhang, Li and Hou. This is an open-access article distributed under the terms of the Creative Commons Attribution License (CC BY). The use, distribution or reproduction in other forums is permitted, provided the original author(s) and the copyright owner(s) are credited and that the original publication in this journal is cited, in accordance with accepted academic practice. No use, distribution or reproduction is permitted which does not comply with these terms.
*Correspondence: Weiguo Hou, d2VpZ3VvaG91QGN1Z2IuZWR1LmNu; Fengjie Li, bGlmZW5namllNzJAMTYzLmNvbQ==