- 1Chongqing University of Science and Technology, Chongqing, China
- 2Chongqing Key Laboratory of Complex Oil and Gas Exploration and Development, Chongqing, China
- 312th Oil Production Plant, Changqing Oil Field Company of PetroChina, Gansu, China
- 4College of Geosciences and Engineering, Xi’an Shiyou University, Xi’an, Shaanxi, China
The carbonate successions from the Chihsia Formation play an important role for hydrocarbon exploration in the South China. However, the dolomites occurring in the upper section of the Chihsia Formation have been enigmatic, which confined further understanding of the depositional and/or diagenetic fluids and hydrocarbon prediction. In this study, the Pingdingshan section was investigated as a typical outcrop of Chihsian carbonates. On the basis of lithofacies/mineralogic observation of hand specimens and thin-sections, three types of dolomites were classified according to their crystal sizes, that is, micritic dolomite, sucrosic dolomite, and saddle dolomite. Comprehensive, micro-area geochemical proxies, including homogenization temperatures of fluid-inclusions, carbon and oxygen isotopes, and trace and rare-earth elements, were analyzed on different types of dolomites and matrix calcites. The results revealed that the selected samples were representative of temporal seawater and were not contaminated by terrestrial input. The temperatures and salinities of fluid-inclusions, combined with carbon and oxygen isotopic values, revealed that sucrosic dolomites originated from penecontemporaneous micritic dolomites, which had been crystallized and altered into fine- to medium-sized dolomite clusters during the later-stage burying process. In contrast, the saddle dolomites occurring in carbonate matrix or fractures were formed in a hydrothermal environment with a dolomitizing fluid temperature higher than 170°C. This inference is consistent with evidence from rare-earth elements and carbon and oxygen isotopes. Our findings provide new clues for the origin elucidation of dolomitizing fluids in the Chihsia Formation, South China.
1 Introduction
The dolomite origins and dolomitizing processes have been extensively studied by petroleum geologists because the dolostones provide approximately 50% of the carbonate hydrocarbon reservoirs (Zenger et al., 1980; Sun, 1995; Ma et al., 2007). This percentage increases to more than 80% at deep-burial formations (i.e., depths greater than 4,000 m) as dolostones are commonly more porous and permeable than limestones because of mineralogical alterations during burying diagenesis (Warren, 2000; Machel, 2004). More importantly, the evolution of reservoir porosities related to dolomitizing process has a great influence on the reservoir quality of carbonates. Hence, deciphering dolomitization is significant for high-quality reservoir prediction in carbonates (e.g., Liu et al., 2016, 2017). In addition, carbonate properties are sensitive to diagenetic alterations (particularly dolomitizing process) because mineralogical and/or chemical modifications occurred easily in ancient carbonates due to their unstable chemical properties (Azmy et al., 2001; Azomani et al., 2013). Previous studies have revealed that dolomitization could exert both direct and indirect controls on the reservoir porosity of carbonates, indicating that the dolomitization-induced porosities have both syn- and post-dolomitization origins (e.g., Machel and Burton, 1994; Machel, 2004; Davies and Smith, 2006; Eren et al., 2007). The dolomitizing process may increase, sustain, or suppress porosity development in ancient carbonates, depending on sedimentary and/or diagenetic controls, such as the textures and fabrics of precursor aragonites/calcites, depositional belts, marine environments, and dolomitizing mechanisms (Warren, 2000; Liu et al., 2016). Particularly, dolomitization has been enigmatic and a plethora of mechanisms have been proposed to describe this process, including seepage-reflux, sabkha-style, meteoric-mixing zone, organic-origin, burial, and hydrothermal fluid dolomitizations (Adams and Rhodes, 1960; Friedman and Sanders, 1967; Badiozamani, 1973; Hsu and Schneider, 1973; Baker and Kastner, 1981; Braithwaite and Rizzi, 1997; Huang et al., 2008). Nonetheless, these dolomitizing patterns are usually subjects of debate when being applied to a certain region. For instance, Land (1985) and Hardie (1987) have cast doubts on Baker and Kastner’s (1981) previous experiments by questioning its extremely high temperatures (greater than 200°C) that might not be applicable to wide dolomitization at seafloor environments featured by deep-water depths and low temperatures. Currently, geochemical methods are widely applied to determine dolomite origins, coupled with traditional depositional, petrographic, and diagenetic elucidation. For instance, the oxygen isotope (δ18O) is widely used as an effective proxy to reveal the flowing directions and temperatures of dolomitizing fluids because oxygen isotopic composition is sensitive to salinity and temperature. Moreover, other trace elements (Fe, Mn, and Sr) and stable/radioactive isotopes (δ13C and 87Sr/86Sr) provide effective tools to trace dolomitizing fluids (Kaufman et al., 1991; Ronchi et al., 2011; Liu et al., 2016, 2017).
The Pingdingshan section, outcropped at South China, highlighted its significance in the following aspects: 1) The western Pingdingshan interval is recognized as a potential profile of the “Global Stratotype Section and Point” recording the Induan-Olenekian boundary. Insightful evidence revealing the paleo-environment of the Permian Ocean is required to validate this boundary and further understand the Permian–Triassic transition (Tong et al., 2003). 2) The Permian Chihsian biomicrites contribute to regional hydrocarbon source rocks and provide potential reservoirs in the upper part of South China. Deciphering dolomite origins and related transporting fluids has significant implications for hydrocarbon evaluation (Liu et al., 2011).
In this article, we provide newly collected core samples from the Chihsia Formation in the Pingdingshan profile, Chaohu area. Dolomite occurrence is the focus in lithologic and mineralogic studies. Coupled with field study and regular petrological investigation, integrated geochemical proxies were presented and analyzed to reveal the dolomite origins of the carbonate successions from the Chihsia Formation. The current study has the significance of elucidating petroleum systems related to the Permian–Triassic transition in the South China.
2 Geological Setting
The Chaohu area is located on the Lower Yangtze Plate tectonically. The deposition of this area was controlled by the Jiangnan Faulting event, developing marine sedimentation from the Sinian to the Triassic with total strata thickness of ∼14,000 m. The Pingdingshan section is outcropped at a syncline structure which was formed due to an ancient continuous folding movement characterized by a northeastern trend. From northeast to southwest, the Yufudacun syncline, the Ma’anshan monocline, the Fenghuangshan anticline, the Pingdingshan syncline, and the Majiashan monocline are sequentially outcropped (Figure 1A; Liu et al., 2022). The Chihsia Formation, characterized by wide occurrence and stable distribution with a thickness of 100∼200 m, is an important regional marine hydrocarbon source rock in South China and the most representative carbonate source rock in the Lower Yangtze region. The Chihsia Formation is partly developed at the Pingdingshan section (thickness of ∼35 m), Chaohu area, disconformably in contact with the underlying Chuanshan Formation and overlying Gufeng Formation, respectively. At the studied interval, the Chihsia Formation can be divided into several lithology sets from bottom to top, that is, the bottom coal seam, the lower-part bituminous carbonates, the middle-part siliceous limestones, the upper-part bioclastic carbonates, and the top dolomitized limestones (Figure 1B). During this geological period, the sedimentary environment experienced a constant transgression of seawater, thus accommodating sediments’ transition from platform of shoal clastic with coal to slope marine carbonates.
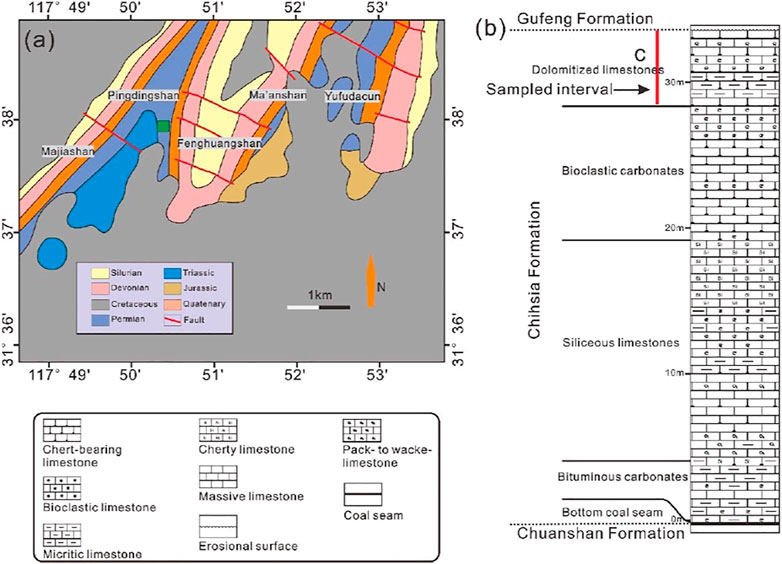
FIGURE 1. (A) Local structural map showing tectonic characteristics of the Chaohu area. (B) Lithologic column showing petrofacies and sample location at the studied interval (modified after Liu et al., 2022).
3 Materials and Methods
Hand specimens were collected from the Chihsia Formation outcropped at the Pingdingshan section of the Chaohu City, Anhui Province. The sampled interval is shown in Figure 1B. Micro-area sampling method was applied using a dental drill (diameter of 20 μm) coupled with microscopic observation to obtain pure dolomite or calcite components. Meanwhile, thin sections without coverslips were prepared for conducting the laser ablation process.
The prepared powder and thin-section samples were sent for mineralogic determination, minor/trace and rare-earth element measurement, fluid-inclusion homogenization thermometer analysis, and oxygen and carbon isotopic measurement. Liquid-vapor fluid-inclusions were observed from double-polished, 0.8-mm–thick thin sections. Ice melting and homogenization temperatures (Th) were measured on inclusions using the Linkam MDS-600 heating-freezing stage linked with microthermometric-analysis software. The temperature accuracy measured is better than ±1°C. Salinities were calculated from Th values using the equation NaCl (wt%) = 1.78Tm + 0.0442Tm2 + 0.000557Tm3 proposed by Hall et al. (1988) in the H2O–NaCl system. Inclusion populations were described by linking UV fluorescent sources, including their morphologies, distribution, sizes, and presence of hydrocarbons. The specific analyzing procedure was performed referring to Shepherd et al. (1985).
Micro-area element analysis was conducted to determine trace and rare-earth element contents for different types of dolomites and calcite matrices. A traditional acidic dissolving method was applied to obtain element concentrations using the ICP-MS method with Finnigan-Mat Element-3 of radio-frequency power of 1,200 kW and vaporized sample flow velocities of 0.660 L/min. The detection limits range from 0.001 to 1 ppm with respect to different elements, and the accuracy is better than ±5%. The specific workflow was conducted referring to Zhang et al. (2019).
For carbon and oxygen isotope determination, the laser ablation method was applied. The LSX-200 UV laser ablation system was equipped with a NdYAG laser emitter. A laser beam with a wave length of 1,046 was focused on the thin sections in a vacuum environment. The agitated carbon dioxide was purified and imported into an isotope mass spectrometer (Type MAT 252) for δ18O and δ13C testing. The detailed parameter settings and workflow were referred from Liu et al. (2017).
All the above experiments were conducted at the State Key Laboratory of Petroleum Resources and Prospecting in China University of Petroleum, Beijing.
4 Lithofacies and Mineralogy
The matrix composition of the sampled interval at the Chihsia Formation is mainly composed of micritic calcites, with crystal sizes commonly less than 20 μm (Figure 2A). Stylolites are developed in micritic calcites (Figure 2B). The dolomites developing in the cored interval can be classified into three categories according to crystal sizes, that is, the micritic to fine-sized dolomites, the sucrosic dolomites of medium- to coarse-sized crystals, and the saddle dolomites. The micritic to fine-sized dolomites are characterized by laminar distribution with crystal sizes commonly smaller than 20 μm (Figure 2C). This type of dolomite usually displays tight lithology. Gypsum and/or halite molds are widely developed in micritic dolomites (Figure 2D), reflecting an evaporative environment. The micritic dolomites are closely associated with matrix calcites and show dark red light under CL observation (cathode-luminescence). This type of dolomite mainly occurs in the upper part of the Chihsia Formation as interbeds of matrix calcite. The sucrosic dolomites are featured by their typical sucrosic texture, developing medium- to coarse-sized crystal sizes (ranging from 50 to 300 μm). The crystal textures are planar-s and planar-e, with the latter being dominant (Figure 2E). Meanwhile, fluid-inclusions aligned to the growth direction are widely developed in crystals (Figure 2F). The saddle dolomites are mainly developed in interparticle or vuggy porosities of the matrix carbonates as cements (Figure 2G), particularly in karst breccias as assemblages (Figure 2H). This type of dolomite is featured by large crystal sizes (i.e., coarse than 500 μm) and curved crystal surfaces. The large crystal is dominantly planar-e type.
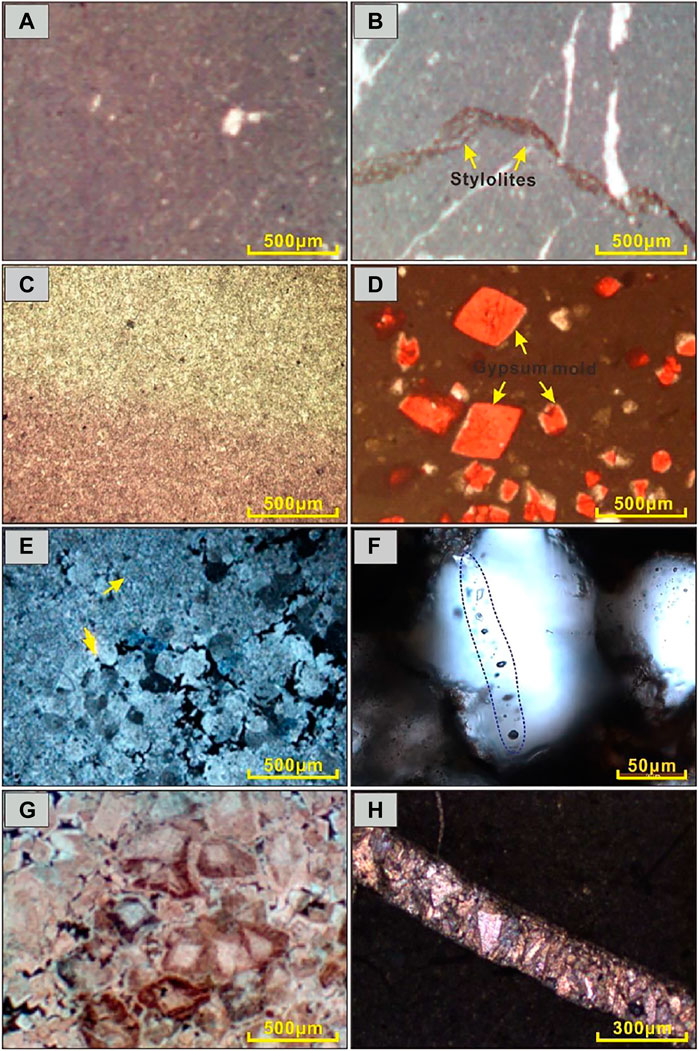
FIGURE 2. Photomicrographs showing the mineralogy of the studied samples under cross-polarized light. (A) Micritic calcites showing very fine-sized crystals. (B) Stylolites developing in the matrix calcite. (C) Micritic dolomites showing invisible crystal sizes. (D) Gypsum mold occurring in the micritic dolomite. (E) Sucrosic dolomites exhibiting medium-sized crystal accumulation. (F) Linear distribution of fluid-inclusion in sucrosic dolomite. (G) Saddle dolomites displaying large crystal size. (H) Saddle dolomites occurring in the fractures.
5 Results and Discussion
5.1 Representativeness Evaluation of Carbonate Samples
5.1.1 Assessment of Diagenetic Alterations
It would cause deviated results for geochemical proxies (such as carbon and oxygen isotopes) when marine carbonates (especially porous carbonates) undergo intensive diagenetic modifications because geochemical signatures inherited from source rocks should be altered due to long-term rock-fluid (meteoric water) interactions. Previous studies verified that it is reliable to assess degrees of diagenetic modification using Mn/Sr ratios because the diagenesis of marine carbonates is a process of Mn accumulation and Sr depletion (Brand and Veizer, 1980; Huang, 1990; Liu et al., 2016). It is commonly recognized that an Mn/Sr ratio of 2 is a threshold value for the representativeness of carbonate samples to geochemical signatures of ancient seawater. Therefore, Mn/Sr ratios lower than 2 usually indicate that weak diagenetic alterations caused by meteoric water occurred and the present-day carbonate samples inherited the geochemical signatures of temporal seawater (Vahrenkamp and Swart, 1990). In the studied interval, all examined dolomite samples exclusively displayed low Mn/Sr ratios with a mean value of 0.63 (Figure 3), indicating that these dolomites did not experience intensive meteoric-water alterations after deposition. Furthermore, intensive diagenetic alterations caused by meteoric water can result in synchronous variations of carbon and oxygen isotopes. However, the δ18O and δ13C values are not correlated in the studied interval of the Chihsia Formation (Figure 4), reflecting that the dolomite proxies inherited the geochemical signatures of ancient seawater.
5.1.2 Assessment of Heterochthonous Contamination
Marine carbonates are usually featured by relatively low rare-earth element content, with their values being less than 100 ppm. A very minor content of terrigenous input can change the rare-earth elemental composition significantly due to its extremely high abundance of rare-earth elements (REE). Thus, the contamination from terrigenous detritus (especially clay minerals) should be quantitatively evaluated (Webb and Kamber, 2000; Nothdurft et al., 2004). In the studied interval, whether the carbonate samples are contaminated by terrigenous input or not can be identified with the following methods. 1) The Y/Ho ratio can be an effective indicator for terrigenous input because of low Y/Ho ratios in terrestrial detritus and high Y/Ho values in marine sediments, with their mean values being 27.500 and 45.500, respectively (Webb and Kamber, 2000). The measured Y/Ho ratios range from 37.88 to 46.84 with a mean value of 43.82 in the studied interval (Table 1), suggesting that these dolomites were closely associated with detritus-free marine products, but not linked to terrestrial input. This indicates that our analyzed samples are not contaminated by terrestrial clays. 2) Ferrum and manganese content in meteoric water are much higher than in seawater. Thus, a minor amount of terrestrial input should cause an increase in both rare-earth and Fe–Mn elements simultaneously (Bau et al., 1996). Our study shows that the total REE abundance of dolomites is randomly correlated with Fe or Mn contents (Figure 5), reflecting that terrestrial contamination was very limited. 3) High field-strength metals, that is, Th, Sc, and Hf, are indications of terrigenous influx because their concentrations are much more abundant than in marine carbonates (Taylor et al., 1981). The dolomite samples from the studied interval of the Chihsia Formation exhibit Th, Sc, and Hf average concentrations of 0.445, 4.033, and 1.321 ppm, respectively (Table 1), which are pronouncedly lower than their mean values in the upper crust, that is, Th = 2.30 ppm, Sc = 14.90 ppm, and Hf = 5.80 ppm (Taylor et al., 1981). Collectively, the dolomite samples in the studied interval are free from terrestrial contamination and reliable for geochemical analysis.
5.2 Origin of Sucrosic Dolomites
Dolomitization of grain calcites and biogenic mechanism are recommended origins of sucrosic dolomites in recent studies. Precursor calcites forming sucrosic dolomites are commonly deposited in subtidal high-energy zones in the open seas, while biogenic dolomites are formed in a restricted marine environment with a temperature of 30∼45°C, salinity of 3.5∼10%, and pH value greater than 8.5 (Zhang et al., 2019). In the studied interval, discernable gypsum and halite molds are observed (Figure 2D), which are typical characteristics of the exposure zone in the supratidal environment. Meanwhile, gypsum or halite and their associated minerals are not developed in biogenic dolomites. Therefore, the sucrosic dolomites in the studied area were not derived from the above mechanisms.
Fluid inclusions developing in fine- to medium-sized grain clusters in sucrosic dolomites are measured with an average homogenization temperature and salinity of 123.5°C and 26.55%, respectively (Figure 6). Assuming that the average geothermal gradient and maximum bury depth of the Chihsia Formation are 2.5°C/100 and 2,200 m, respectively, the calculated formation temperature of the sucrosic dolomite is ∼72°C. Therefore, fine- to medium-grained dolomites derived from sucrosic dolomites were formed at high temperatures. Presumably, the sucrosic dolomites were formed during the burying process and were crystallized from micritic dolomites because the two categories of dolomites exhibit similar salinities. Meanwhile, magnesium ions composing sucrosic dolomites were derived from seawater because of its high salinity, probably by means of reflux seepage.
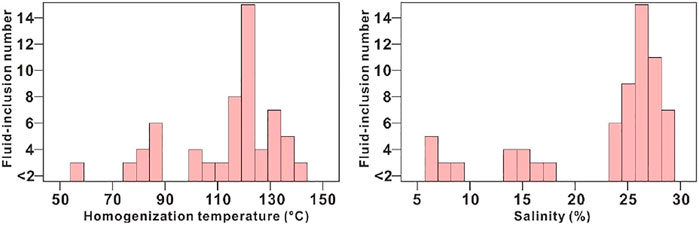
FIGURE 6. Histograms showing homogenization temperatures (left) and salinities of the sucrosic dolomites in the studied interval.
Furthermore, the carbon and oxygen isotope compositions reveal that micritic dolomites from sucrosic clusters and carbonate matrix exhibit similar δ18O and δ13C values, also reflecting their inheriting correlation (Figure 4). It is noteworthy that δ18O and δ13C values show negative excursions with increasing crystal sizes ranging from micritic to fine-/medium-sized (Figure 7). This may result from diagenetic recrystallization with increasing temperatures (Liu et al., 2016, 2017). This inference is also consistent with the result of similar REE characteristics of sucrosic dolomites and micritic dolomite/calcite matrices (Figure 8).
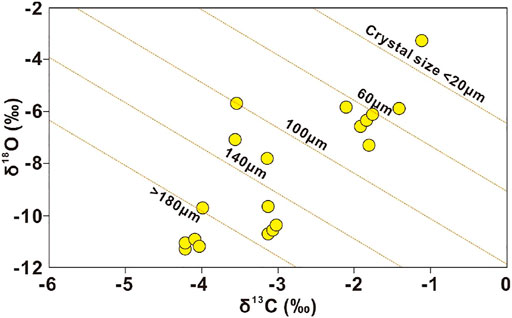
FIGURE 7. δ18O and δ13C values display negative excursions with increasing crystal sizes from micritic to fine-/medium-sized dolomite samples.
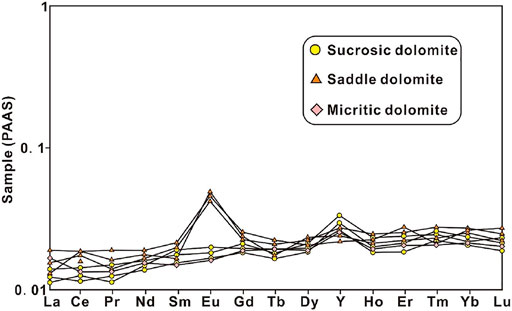
FIGURE 8. REE patterns of dolomites discussed in the article. The concentrations are normalized to PAAS standard (ref. Nothdurft et al., 2004).
To conclude, the sucrosic dolomites originated from penecontemporaneous micritic dolomites formed in an evaporative environment on a restricted platform. During later-stage burying, these micritic dolomites were partly or completely crystallized and altered into fine- or medium-sized dolomites.
5.3 Origin of Saddle Dolomites
Different from the REE characteristics of sucrosic dolomites, the saddle dolomites display slightly positive Ce anomaly and pronouncedly positive Eu anomaly (Figure 8), revealing that the dolomitizing fluids were derived from hydrothermal activities in a closed environment (Alibo and Nozaki, 1999; Bau and Dulski, 1996). Considering that the temperature rising during the penecontemporaneous period is insufficient to break through the kinetic barrier to form coarse-sized dolomites (i.e., saddle dolomite in this study), the heat resulting from hydrothermal activities probably plays an important role in forming saddle dolomites under the background with marine-sourced fluids being dominant. The calcite matrices display multiple peaks, reflecting that different stages of thermal activities occurred, and multiple fluid inclusions were captured (Figure 9). Nonetheless, only the period exhibiting the main peak of 152°C overlapped with that of saddle dolomites (Figure 9). This probably indicates that dolomitizing fluids featured by extremely high temperatures modified the precursor limestone layers. The highest temperature peak reaches >170°C, which obviously exceeds geothermal conditions during the burying process. Meanwhile, it reveals that the formation of this type of dolomite (i.e., saddle dolomite) has experienced an anomalously high temperature. Furthermore, with the temperature increasing to 125°C, the δ13C values will decrease constantly. In contrast, when the temperature is greater than 125°C, the δ13C values exhibit an increasing trend (Figure 10). This feature is determined by the fractionation mechanism of carbon isotopes (Horita, 2014). Presumably, the dolomitizing fluid originated from high-temperature brine water.
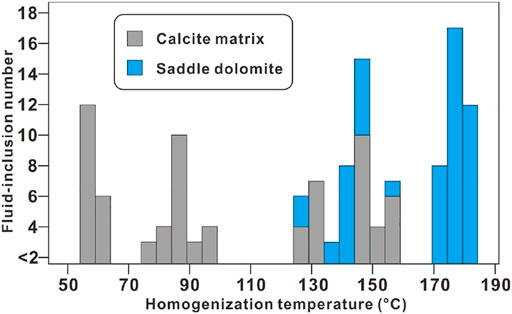
FIGURE 9. Histograms showing homogenization temperatures of the calcite matrix and saddle dolomite in the studied interval.
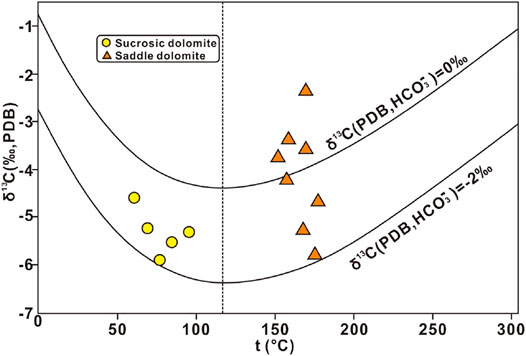
FIGURE 10. Evolution of carbon isotopes with increasing temperature (Horita, 2014) in the studied interval of the Chihsia Formation.
6 Conclusion
According to our study, the following conclusions can be addressed.
1) Sample validity is feasibly examined through the Y/Ho ratio, trace element and carbon-oxygen isotopic correlation, and high field-strength metal contents.
2) The sucrosic dolomites occurring in the Chihsia Formation of the Pingdingshan section originated from the penecontemporaneous micritic dolomites in a restricted platform environment. These micritic dolomites were crystallized and modified into fine- or medium-sized crystals, thus forming sucrosic dolomites during later-stage burying.
3) The saddle dolomites occurring in the Chihsia Formation of the Pingdingshan section are formed in an environment characterized by high temperature (greater than 170°C) and high salinity (brine water).
Data Availability Statement
The original contributions presented in the study are included in the article/Supplementary Material; further inquiries can be directed to the corresponding author.
Author Contributions
HK: Conceptualization, methodology, writing—review and editing, methodology, and project administration. XZ: Formal analysis and writing—original draft. ML: Formal analysis and writing—original draft. ZM: Formal analysis and writing—original draft. CL: Methodology and project administration.
Funding
This study is jointly funded by the National Natural Science Foundation of China (Grant number: 42002159) and the Natural Science Foundation of Chongqing City (Grant number: cstc2019jcyj-msxmX0008).
Conflict of Interest
ZM, XZ and ML were employed by Changqing Oil Field Company of PetroChina.
The remaining authors declare that the research was conducted in the absence of any commercial or financial relationships that could be construed as a potential conflict of interest.
Publisher’s Note
All claims expressed in this article are solely those of the authors and do not necessarily represent those of their affiliated organizations, or those of the publisher, the editors, and the reviewers. Any product that may be evaluated in this article, or claim that may be made by its manufacturer, is not guaranteed or endorsed by the publisher.
References
Adams, J. E., and Rhodes, M. L. (1960). Dolomitization by Seepage Refluxion. AAPG Bull. 44 (12), 1912–1920. doi:10.1306/0bda6263-16bd-11d7-8645000102c1865d
Alibo, D. S., and Nozaki, Y. (1999). Rare Earth Elements in Seawater: Particle Association, Shale-Normalization, and Ce Oxidation. Geochim. Cosmochim. Acta 63 (3-4), 363–372. doi:10.1016/s0016-7037(98)00279-8
Azmy, K., Veizer, J., Misi, A., Oliveira, T. F. D., Sanches, A. L., Dardenne, M. A., et al. (2001). Dolomitization and Isotope Stratigraphy of the Vazante Formation, Sao Francisco Basin. Braz. Precambrian Res. 112 (3-4), 303–329. doi:10.1016/s0301-9268(01)00194-2
Azomani, E., Azmy, K., Blamey, N., Brand, U., and Al-Aasm, I. (2013). Origin of Lower Ordovician Dolomites in Eastern Laurentia: Controls on Porosity and Implications from Geochemistry. Mar. Petroleum Geol. 40 (1), 99–114. doi:10.1016/j.marpetgeo.2012.10.007
Badiozamani, K. (1973). The Dorag Dolomitization Modeldapplication to the Middle Ordovician of Wisconsin. J. Sediment. Pet. 43 (4), 965–984.
Baker, P. A., and Kastner, M. (1981). Constraints on the Formation of Sedimentary Dolomite. Science 213 (4504), 214–216. doi:10.1126/science.213.4504.214
Bau, M., and Dulski, P. (1996). Distribution of Yttrium and Rare-Earth Elements in the Enge and Kuruman Iron-Formations, Transvaal Supergroup, South Africa. Precambrian Res. 79 (1-2), 37–55. doi:10.1016/0301-9268(95)00087-9
Bau, M., Koschinsky, A., Dulski, P., and Hein, J. R. (1996). Comparison of the Partitioning Behaviours of Yttrium, Rare Earth Elements, and Titanium between Hydrogenetic Marine Ferromanganese Crusts and Seawater. Geochimica Cosmochimica Acta 60, 1709–1725. doi:10.1016/0016-7037(96)00063-4
Braithwaite, C. J. R., and Rizzi, G. (1997). The Geometry and Petrogenesis of Hydrothermal Dolomites at Navan, Ireland. Sedimentology 44, 421–440. doi:10.1046/j.1365-3091.1997.d01-30.x
Brand, U., and Veizer, J. (1980). Chemical Diagenesis of Multicomponent Carbonate System-1: Trace Elements. J. Sediment. Pet. 50, 1219–1236.
Davies, G. R., and Smith, L. B. (2006). Structurally Controlled Hydrothermal Dolomite Reservoir Facies: an Overview. Bulletin 90 (11), 1641–1690. doi:10.1306/05220605164
Eren, M., Kaplan, M. Y., and Kadir, S. (2007). Petrography, Geochemistry and Origin of Lower Liassic Dolomites in the Aydincik Area, Mersin, Southern Turkey. Turk. J. Earth Sci. 16 (3), 339–362.
Friedman, G. M., and Sanders, J. E. (1967). “Chapter 6 Origin and Occurrence of Dolostones,” in Carbonate Rocks. Editors G. V. Chilingar, H. J. Bissel, and R. W. Fairbridge Amsterdam: (Elsevier), 267–348. doi:10.1016/s0070-4571(08)71114-2
Hall, D. L., Sterner, S. M., and Bodnar, R. J. (1988). Freezing Point Depression of NaCl-KCl-H 2 O Solutions. Econ. Geol. 83, 197–202. doi:10.2113/gsecongeo.83.1.197
Hardie, L. A. (1987). Dolomitization; a Critical View of Some Current Views. J. Sediment. Res. 57 (1), 166–183. doi:10.1306/212f8ad5-2b24-11d7-8648000102c1865d
Horita, J. (2014). Oxygen and Carbon Isotope Fractionation in the System dolomite-water-CO2 to Elevated Temperatures. Geochimica Cosmochimica Acta 129 (8), 111–124. doi:10.1016/j.gca.2013.12.027
Hsü, K. J., and Schneider, J. (1973). Progress Report on Dolomitization Hydrology of AbuDhabi Sabkhas, Arabian Gulf. The Persian Gulf. New York: Springer, 409–422. doi:10.1007/978-3-642-65545-6_20
Huang, S. (1990). Cathodoluminscence and Diagenetic Alternation of Marine Carbonate Minerals. Sediment. Geol. Teth. Geol. 10 (4), 9–15. (In Chinese with English abstract).
Huang, S., Qing, H., Huang, P., Hu, Z., Wang, Q., Zou, M., et al. (2008). Evolution of Strontium Isotopic Composition of Seawater from Late Permian to Early Triassic Based on Study of Marine Carbonates, Zhongliang Mountain, Chongqing, China. Sci. China Ser. D-Earth Sci. 51 (4), 528–539. doi:10.1007/s11430-008-0034-3
Kaufman, J., Hanson, G. N., and Meyers, W. J. (1991). Dolomitization of the Devonian Swan Hills Formation, Rosevear Field, Alberta, Canada. Sedimentology 38 (1), 41–66. doi:10.1111/j.1365-3091.1991.tb01854.x
Land, L. S. (1985). The Origin of Massive Dolomite. J. Geol. Educ. 33, 112–125. doi:10.5408/0022-1368-33.2.112
Liu, C., Ma, J., Zhang, L., Wang, C., and Liu, J. (2022). Protracted Formation of Nodular Cherts in Marine Platform: New Insights from the Middle Permian Chihsian Carbonate Successions, South China. Carbonate Evaporite 37 (15), 1–19. doi:10.1007/s13146-022-00757-6
Liu, C., Xie, Q., Wang, G., He, W., Song, Y., Tang, Y., et al. (2017). Rare Earth Element Characteristics of the Carboniferous Huanglong Formation Dolomites in Eastern Sichuan Basin, Southwest China: Implications for Origins of Dolomitizing and Diagenetic Fluids. Mar. Petroleum Geol. 81, 33–49. doi:10.1016/j.marpetgeo.2016.12.030
Liu, C., Xie, Q., Wang, G., Song, Y., and Qi, K. (2016). Dolomite Origin and its Implication for Porosity Development of the Carbonate Gas Reservoirs in the Upper Permian Changxing Formation of the Eastern Sichuan Basin, Southwest China. J. Nat. Gas Sci. Eng. 35, 775–797. doi:10.1016/j.jngse.2016.09.027
Liu, F., Cai, J., Lyu, B., and Xu, J. (2011). Formation and Influencing Factors of Carbonate Source Rock of the Lower Permian Chihsia Formation in Chaohu Region, Anhui Province. Sci. China (Earth Sci. 54 (12), 1926–1939. doi:10.1007/s11430-011-4242-x
Ma, Y., Guo, X., Guo, T., Huang, R., Cai, X., and Li, G. (2007). The Puguang Gas Field: New Giant Discovery in the Mature Sichuan Basin, Southwest China. Bulletin 91 (5), 627–643. doi:10.1306/11030606062
Machel, H. G., and Burton, E. A. (1994). Golden Grove Dolomite, Barbados: Origin from Modified Seawater. J. Sediment. Res. Sect. A 64 (4), 741–751. doi:10.1306/d4267eab-2b26-11d7-8648000102c1865d
Machel, H. G. (2004). “Concepts and Models of Dolomitization: a Critical Reappraisal. Geol. Soc. Lond. Spec. Publ. 235, 7–63. Editors C. J. R. Braithwaite, G. Rizzi, and G. Drake (London: Geological Society. Special Publication). doi:10.1144/gsl.sp.2004.235.01.02
Nothdurft, L. D., Webb, G. E., and Kamber, B. S. (2004). Rare Earth Element Geochemistry of Late Devonian Reefal Carbonates, Canning Basin, Western Australia: Confirmation of a Seawater REE Proxy in Ancient Limestones. Geochimica Cosmochimica Acta 68 (2), 263–283. doi:10.1016/s0016-7037(03)00422-8
Ronchi, P., Jadoul, F., Ceriani, A., Di Giulio, A., Scotti, P., Ortenzi, A., et al. (2011). Multistage Dolomitization and Distribution of Dolomitized Bodies in Early Jurassic Carbonate Platforms (Southern Alps, Italy). Sedimentology 58 (2), 532–565. doi:10.1111/j.1365-3091.2010.01174.x
Shepherd, T. J., Rankin, A. H., and Alderton, D. H. M. (1985). A Practical Guide to Fluid Inclusion Studies. London: Blackie.
Sun, S. Q. (1995). Dolomite Reservoirs: Porosity Evolution and Reservoir Characteristics. AAPG Bull. 79 (2), 186–204. doi:10.1306/8d2b14ee-171e-11d7-8645000102c1865d
Taylor, S. R., Mclennan, S. M., Armstrong, R. L., and Tarney, J. (1981). The Composition and Evolution of the Continental Crust: Rare Earth Element Evidence from Sedimentary Rocks [and Discussion]. Philos. Trans. R. Soc. B Biol. Sci. 301 (4), 398–399. doi:10.1098/rsta.1981.0119
Tong, J., Zakharov, Y. D., Orchard, M. J., Yin, H., and Hansen, H. J. (2003). A Candidate of the Induan-Olenekian Boundary Stratotype in the Tethyan Region. Sci. China Ser. D-Earth Sci. 46 (11), 1182–1200. doi:10.1360/03yd0295
Vahrenkamp, V. C., and Swart, P. K. (1990). New Distribution Coefficient for the Incorporation of Strontium into Dolomite and its Implications for the Formation of Ancient Dolomites. Geol 18 (5), 387–391. doi:10.1130/0091-7613(1990)018<0387:ndcfti>2.3.co;2
Warren, J. (2000). Dolomite: Occurrence, Evolution and Economically Important Associations. Earth Sci. Rev. 52 (1), 1–81. doi:10.1016/s0012-8252(00)00022-2
Webb, G. E., and Kamber, B. S. (2000). Rare Earth Elements in Holocene Reefal Microbialites: a New Shallow Seawater Proxy. Geochimica Cosmochimica Acta 64 (9), 1557–1565. doi:10.1016/s0016-7037(99)00400-7
Zenger, D. H., Dunham, J. B., and Ethington, R. L. (1980). Concepts and Models of Dolomitization-An Introduction. SEPM Spec. Publ. 28, 1–9. doi:10.2110/pec.80.28.0001
Keywords: dolomite origin, diagenesis, carbonate reservoir, South China, Chihsia Formation
Citation: Kuang H, Mao Z, Zhuo X, Li M and Liu C (2022) Dolomite Origins of the Carbonate Successions From the Chihsia Formation, South China: A Case Study From the Pingdingshan Section. Front. Earth Sci. 10:930918. doi: 10.3389/feart.2022.930918
Received: 29 April 2022; Accepted: 16 May 2022;
Published: 21 June 2022.
Edited by:
Chen Zhang, Chengdu University of Technology, ChinaReviewed by:
Qingbin Xie, China University of Petroleum, Beijing, ChinaYannick Tepinhi, Schlumberger, United States
Copyright © 2022 Kuang, Mao, Zhuo, Li and Liu. This is an open-access article distributed under the terms of the Creative Commons Attribution License (CC BY). The use, distribution or reproduction in other forums is permitted, provided the original author(s) and the copyright owner(s) are credited and that the original publication in this journal is cited, in accordance with accepted academic practice. No use, distribution or reproduction is permitted which does not comply with these terms.
*Correspondence: Hao Kuang, kwanghow@foxmail.com