- 1State Key Laboratory of Oil and Gas Reservoir Geology and Exploitation, Southwest Petroleum University, Chengdu, Sichuan, China
- 2Northwest Oil Company, SINOPEC, Urumqi, China
- 3Key Laboratory of Deep Oil and Gas, China University of Petroleum (East China), Qingdao, China
Light oil is a high-quality crude oil. The formation and chemical composition of light oil is a matter of great concern to petroleum geologists. Yakela Field and Shunbei Field, which are located in Yakela and Shunbei regions, respectively, are the two main light oil fields in the Tabei uplift of the Tarim Basin. In order to identify the causative mechanism of light oils in these two fields, 17 crude oil samples were selected and analyzed by gas chromatography (GC) for saturated hydrocarbons and gas chromatography–mass spectrometry (GCMS) for saturated and aromatic biomarkers. Then, the compounds were measured via the mass spectrometry detector after GC separation. The molecular geochemical characteristics showed that the light oils in the two fields are sourced from similar marine source rocks deposited under weak oxidative and reductive environments. The maturity of crude oil in the Shunbei Field is higher than that in the Yakela Field. Simulation results of hydrocarbon generation history of source rocks in two fields showed that the formation mechanism of the two light oils is different. The light oil in the Yakela Field is directly generated by the source rock in the late oil-generating window. The crude oil in the Shunbei Field is formed by the deep burial and maturation of the crude oil generated in the early stage of the source rock.
1 Introduction
Light oil refers to crude oil with an API higher than 36° (Tissot and Welte, 1978). Compared to normal crude oil, light oil has a higher economic value (Thompson, 1983). Therefore, the formation and composition of light oil is a matter of great concern to petroleum geologists. Previous research suggests that light oils have multiple mechanisms of formation. The formation of light oil is mainly related to the type of parent material, the degree of thermal evolution, and the secondary alteration processes within the reservoir. Humic-type organic matter can produce a small amount of light oil (Snowdon and Powell, 1982; Thompson, 1983; Thompson, 1987). Sapropel-type organic matter can generate a certain amount of light oil in the high evolution stage. High-temperature thermal cracking can break the carbon chain of normal oil to form light oil. Gas invasion can separate short-chain hydrocarbons from normal crude oil to form light oil. Light oils formed by different formation mechanisms are often different (Zhang et al., 2011). In general, light oils formed by high-temperature thermal cracking of crude oil are often larger than those formed by other mechanisms (Zhang et al., 2021). Therefore, the identification of the genetic type of light oil is of great significance for the exploration (Thompson, 1983; Zhang et al., 2011).
The Tarim Basin is the largest petroleum-bearing basin in China. The Tabei uplift is the most abundant oil and gas zone in the Tarim Basin (Jia, 1997; He et al., 2016). There are various types of crude oil in the Tabei uplift, including heavy oil, normal oil, and light oil. Heavy oil accounts for the vast majority of crude oil in the uplift and is thought to be due to biodegradation (Yang et al., 2020; Gu et al., 2020). Light oil mainly exists in the southern Shunbei region and the northern Yakela region. Although previous studies have been carried out on the origin of light oil in the Tarim Basin, most of them are related to secondary alteration processes, such as gas washing and fractionation (Zhang et al., 2021). The genetic mechanism of light oil in the Shunbei and Yakela fields has not been systematically studied. In this study, the geochemical characteristics of light oil in these two fields will be systematically investigated in order to identify its causative mechanism. The study is of great significance for clarifying the genetic mechanism and identifying the source of light oils in the Tarim Basin and the discovery of new large-scale oil and gas fields.
2 Geological setting
The Tarim Basin, with an area of approximately 5.6×105 km2, is the largest petroliferous basin in China (Figure 1A). It is a typical superimposed basin in western China, consisting of Paleozoic cratons in the lower part and Mesozoic–Cenozoic foreland depressions in the upper part (Zhang et al., 2005; Lin et al., 2012; Liu et al., 2019). The basin is bounded by the Tianshan Mountains in the north, the Kunlun Mountains in the southwest, and the Altyn Mountains in the southeast. The first-level tectonic units in the basin include the Tabei uplift, Tazhong uplift, Kuqa depression, Northern depression, Southwest depression, and Southeast fault-uplift belt (Figure 1A) (Zhang et al., 2000; Chang et al., 2013). The Tarim Basin has a complex history of tectonic movements, which has experienced three major tectonic events in the late Caledonian, late Hercynian, and Yanshan–Himalayan periods (Jia, 1997; He et al., 2016). The sedimentary strata of Tarim Basin include the Sinian–Devonian marine sedimentary strata, the Carboniferous–Permian marine-continental transitional strata, and the Triassic–Quaternary terrestrial sedimentary strata (Figure 2) (Jia and Wei, 2002; Zhu et al., 2019). Organic-rich shale of the Lower Cambrian Yuertusi Formation (Є1y) was widely developed in the Tabei uplift, which is the main source rock of the uplift (Cheng et al., 2020; Wang et al., 2021). The source rock was mainly composed of two types of organic facies, namely, planktonic algae in undercompensated basin and planktonic algae in evaporative lagoon. The source rock was widely distributed, with high organic matter abundance and maturity. The organic facies of source rocks in Shunbei Field and Yakela Field were mainly planktonic algae in undercompensated basin (Zhang et al., 2004; Zhu et al., 2018). The middle Ordovician Yijianfang Formation (O2yj) and the upper Yingshan Formation (O1-2y) are the main reservoirs in Shunbei Field (Cai and Li, 2008; Lu et al., 2017). The Lianglitage (O3l) and Sangtamu (O3s) Formations provide excellent regional cap rocks for the preservation of deep oil and gas accumulations (Figure 2) (Jiao, 2018). The lower Cretaceous Yageliemu Formation (K1y) is the main reservoir, and the Xiaokuzibai Formation (E2k) provide the cap rocks in Yakela Field (Dai et al., 2009; Li et al., 2016).
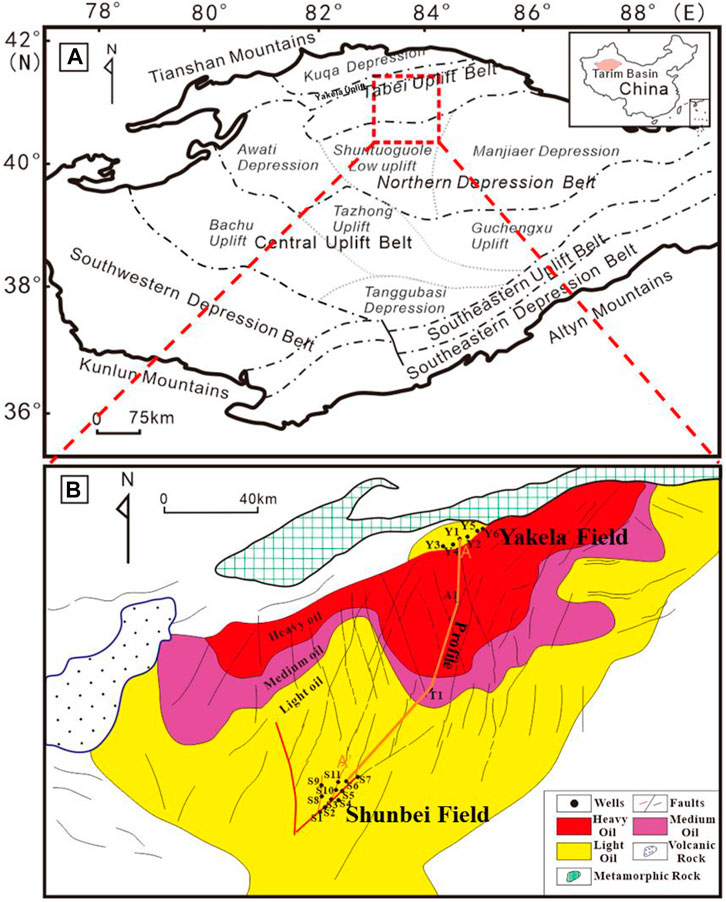
FIGURE 1. (A)Location of the Tarim Basin in western China and major tectonic domains. (B)Close-up view of the Tabei uplift showing the major faults and sampled wells.
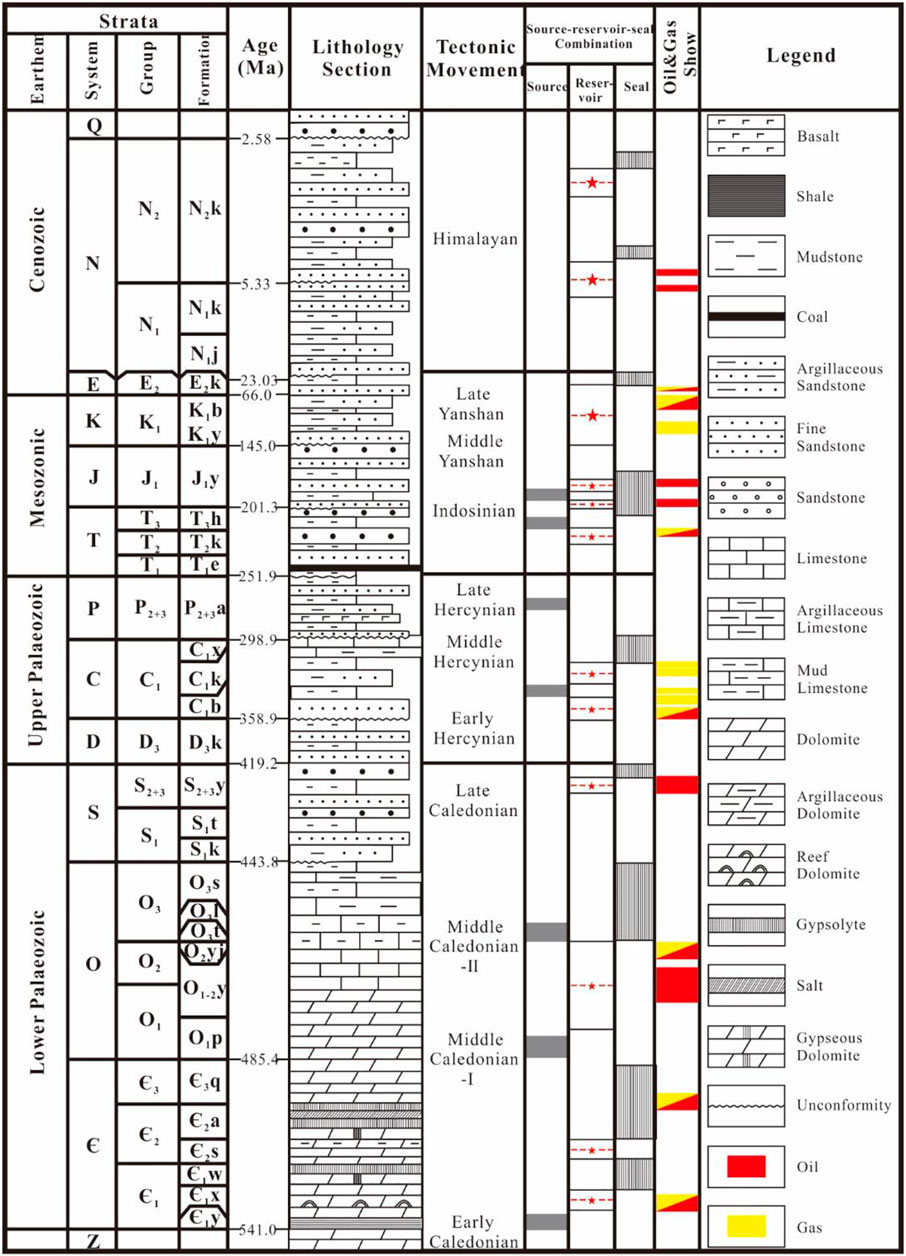
FIGURE 2. Generalized chart showing stratigraphy, lithology, tectonic evolution, and petroleum system elements of the Tarim Basin (modified from Zhu et al., 2019).
After decades of oil and gas exploration and development, several large deep oil and gas fields such as Tahe, Halahatang, and Tazhong oil fields have been discovered in Tabei uplift, Tazhong uplift, and other areas, with total oil and gas reserves of more than 1×1010 tons (7.3×1010 barrels) (Yang et al., 2020). Tabei uplift is one of the most enriched areas of oil and gas resources in Tarim Basin. The oil and gas properties in the Tabei uplift show regular spatial changes and generally show the characteristics of heavy in the north and light in the south (Zhu et al., 2019). Light oil reservoirs in both Shunbei and Yakela oil fields have been discovered in this area. (Figure 1B) (Gu et al., 2020).
Shunbei Field is located in the south of Tabei uplift and at the center of Tarim Basin. It is located between Awati Depression and Manjiaer Depression and is located in the “saddle” of relatively low structure (Ma et al., 2022). Yakela Field is located inside the Yakela uplift, which is a successional fault block uplift formed by Luntai and Yanan faults in the northern Tarim Basin. It was mainly formed in the late Hercynian period. Due to long-term uplift, the pre-Mesozoic strata suffered intense denudation and formed a complex buried-hill system (Dai et al., 2009; Luo et al., 2012).
3 Samples and methods
3.1 Sample selection and pretreatment
A total of 17 representative crude oil samples were selected for this study: 6 from Yakela Field and 11 from Shunbei Field in the Tabei area. (Figure 1B and Table 1). Samples of crude oil were sealed and refrigerated in dark brown glass bottles to prevent the loss of light components due to light and temperature increases. Crude oil samples of about 20 mg were dissolved by adding n-hexane, thoroughly shaken, and kept for 12 h. Asphaltene was obtained by filtering using cotton. Saturated hydrocarbon, aromatic hydrocarbon, and polar hydrocarbon fractions were obtained by filtration of alumina and silica gel using n-hexane, dichloromethane/n-hexane (2:1, v/v), and dichloromethane/methanol (93:7, v/v) as solvents, respectively.
3.2 Gas chromatography and gas chromatography–mass spectrometry analysis
Saturated hydrocarbons from the reservoir oils and reservoir extracts were firstly analyzed via gas chromatography (GC) using an Agilent 7890 A gas chromatograph fitted with a 25 m × 0.20 mm i. d. DB-5 column with a film thickness of 0.33 μm and using helium as carrier gas at a constant flow mode. The GC oven temperature was initially set to 60°C for 1 min, ramped from 60 to 310°C at 7°C/min, and then held at 310°C for 20 min. Separated n-alkane and isoprenoid compounds were then analyzed using the Agilent 5977B MSD mass spectrometry detector. Gas chromatography–mass spectrometry analysis of saturated and aromatic hydrocarbon biomarkers was performed on an Agilent gas chromatography–mass spectrometry 8,860 GC-5977B MSD gas chromatography mass spectrometer. The chromatographic column model was HP-5 quartz elastic capillary (60 m×0.32 mm×0.25 μm). Samples were injected in a pulsed splitless mode, the temperature of the injector was 300°C, the carrier gas was helium, the flow rate was 1 ml/min, the ionization energy was 70 eV, and the detection method was full scan (SCAN)/selected ion monitoring (SIM). The saturated hydrocarbon heating program condition was as follows: initial temperature of 50°C, maintained for 1 min, increased to 120°C at 20°C/min, increased to 310°C at 3°C/min, and maintained for 25 min. The heating program of aromatic hydrocarbon was as follows: the initial temperature was 80°C, increased to 310°C at 3°C/min, and held for 25 min. The separation of crude oil components, gas chromatography, and gas chromatography–mass spectrometry experiments were performed in the experimental research center of Wuxi research institute of petroleum geology of SINOPEC.
3.3 Basin numerical simulation
In this study, the numerical simulation of geological history and hydrocarbon generation history was mainly carried out using Petromod software. Prior to the simulation, parameters such as depth, lithology, deposition and denudation age, denudation thickness, sedimentary palaeowater depth, geochemical indexes of source rocks, and hydrocarbon-generation dynamics model were input. The TⅡ kerogen kinetic model by Dieckmann et al. (2000) was adopted for the hydrocarbon generation kinetics model, and other parameter data such as the stratigraphic, lithologic data, and eroded thickness were obtained from Northwest Oilfield Branch Company Ltd., SINOPEC. The terrestrial heat flow data were obtained from Li et al. (2010), and the thermal conductivity of rocks was obtained from Li et al. (2019).
4 Results and discussion
4.1 Geochemical characteristics of light oils
4.1.1 Sedimentary environment and source of parent material for crude oil
As for isoprenoids, the pristane/phytane (Pr/Ph) ratios of crude oils in Yakela Field range from 1.00 to 1.24, with an average of 1.08. The Ph/nC18 ratio ranges from 0.30 to 0.54, with an average value of 0.43. The Pr/nC17 ratio ranges from 0.28 to 0.47, with an average value of 0.38. However, the Pr/Ph value of crude oils in Shunbei Field is 0.94–0.98, with an average of 0.96. The Ph/nC18 ratio was 0.49–0.55, with an average value of 0.53. The ratio of Pr/nC17 was 0.45–0.49, with an average of 0.46 (Table 1). The Pr/Ph of crude oils in Shunbei Field is lower than those in Yakela Field, but the Ph/nC18 and Pr/nC17 ratios are higher on the whole.
The most abundant sources of Pr and Ph are chlorophyll A in photosynthetic organisms and the phytological side chains of bacterial chlorophyll A and B in purple sulfur bacteria (Brooks et al., 1969; Powell and McKirdy, 1973). Previous studies show that Pr/Ph, Ph/nC18 and Pr/nC17 can be used to indicate the oxidative and reductive environments of source rock deposition, organic matter types, and oil maturities (Connan and Cassau, 1980; Peters et al., 2005; Hanson et al., 2000). In addition to the oxidative and reductive environment, the ratio is partly controlled by thermal maturity and source organic matter input (Peters et al., 2005). The Pr/Ph ratios of crude oils in Shunbei Field are less than 1, indicating an anoxic sedimentary environment. The Pr/Ph ratios of crude oils in Yakela Field are slightly greater than 1, indicating the sedimentary environment of weak oxidation (Peters et al., 2005; Didyk et al., 1978). The cross plot of Pr/nC17 versus Ph/nC18 (Figure 3A) indicates that both the oils in Yakela Field and Shunbei Field originated from the type II marine organic kerogen deposited in a weak reductive environment (Connan and Cassau, 1980).
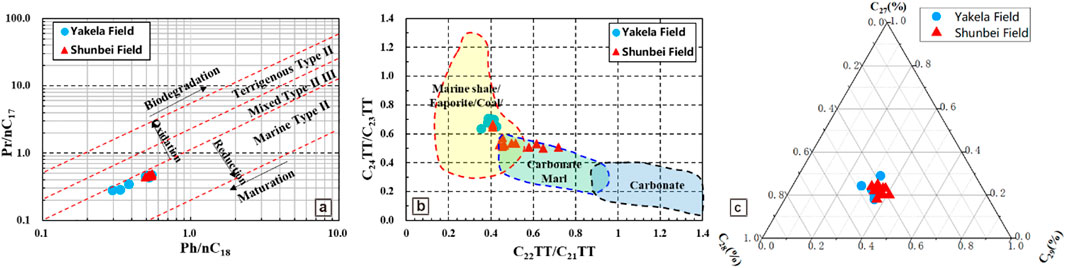
FIGURE 3. Cross plots of biomarker ratios: (A) Pr/nC17 vs. Ph/nC18; (B) C24TT/C23TT vs. C22TT/C21TT; (C) Triangular plot of the normalized relative abundances of C27–C29 ααα 20R steranes (modified from Connan and Cassau, 1980; Peters et al., 2005).
Representative m/z191 chromatograms of the saturated fraction are shown in Figure 4. Terpanes such as tricyclic terpanes (TT), pentacyclic terpanes, and C24 tetracyclic terpanes can be detected in crude oil samples from both regions. The C22TT/C21TT ratios of crude oils in Yakela Field range from 0.35 to 0.41, with an average of 0.39. The C24TT/C23TT ratio range from 0.64 to 0.71, with an average value of 0.68. However, the C22TT/C21TT value of crude oils in Shunbei Field is 0.41–0.72, with an average of 0.52. The C24TT/C23TT ratio is 0.50–0.67, with an average value of 0.55 (Table 1).
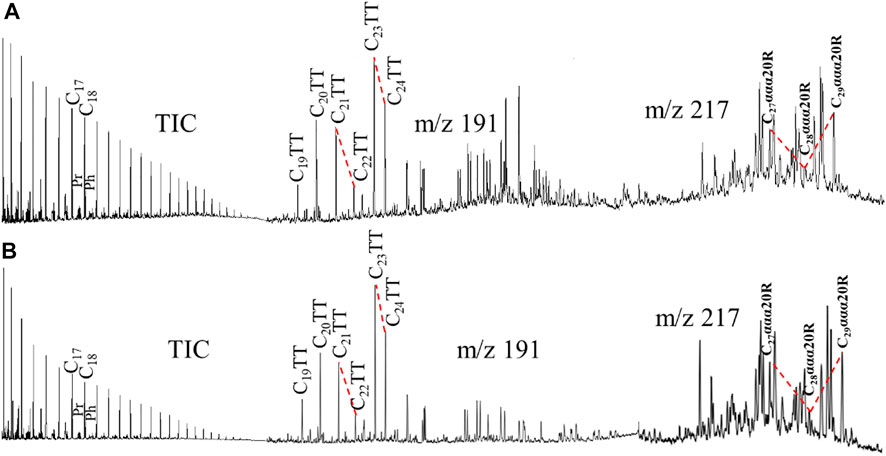
FIGURE 4. Representative gas chromatography (GC) and m/z191 and m/z217 mass chromatograms of the saturated hydrocarbon fraction for oils samples. (A)Y1, K1b; (B) S1, O1-2y.
The ratios of C22TT/C21TT and C24TT/C23TT can be used to identify the type of source rock for crude oils (Peters et al., 2005). Although terpanes with low carbon numbers tend to be formed in the high maturity stage of source rocks, previous studies have shown that these terpanes are more resistant to thermal maturation than hopanes (Peters, 2000; Alberdi et al., 2001). All samples showed that C20 and C23 tricyclic terpanes were dominant, and C19 tricyclic terpanes were low (Figure 4). This is consistent with the traditional perception that C23TT is dominant in marine crude oil as well as C21TT/C23TT<1. The cross plot of C22TT/C21TT vs. C24TT/C23TT (Figure 3B) indicates that both the oils in Yakela Field and Shunbei Field mainly originated from marine shales, although a marl and carbonate source cannot be completely excluded in Shunbei Field. (Peters et al., 2005).
Representative m/z217 chromatograms of the saturated fraction are shown in Figure 4. Steranes, such as regular steranes, can be detected in crude oil samples from both regions. The relative abundance of C27, C28, and C2C9 regular steranes is often used to trace source rocks (Moldowan and Gago, 1986; Li et al., 2016). The C27-29ααα-20R regular steranes of both regions show similar characteristics. Both of them exhibit a V-shape distribution and with the characteristics of the C27ααα-20R and C29ααα-20R dominant set (Figures 3C, 4). It indicated that the organic matter of crude oil in the study area may be mainly marine algae such as green algae in the early Paleozoic marine environment, and the crude oils in both fields were likely from the same set of source rocks (Grantham, 1986; Volkman, 1986; Volkman et al., 1998).
4.1.2 Maturity of crude oil in Shunbei and Yakela fields
The ratio of 17α(H)-22,29,30 trisnorhopane (Tm) and 18α(H)-22,29,30 trisnorhopane (Ts) is a commonly used biomarker parameter to reflect the maturity of crude oil (Peters et al., 2005). Because Ts has greater thermal stability than Tm, the ratio of Ts/(Ts+Tm) increases with the degree of thermal evolution (Peters et al., 2005). The studies show that the parameter has a very reliable effect in evaluating the maturity of crude oil from the same organic facies and is less affected by biodegradation in the process of migration, so it can accurately indicate the maturity change of crude oil from immature to mature stage (Seifert and Moldowan, 1978; Peters et al., 2005). Previous studies have also shown that the source rocks of the two fields have the same organic facies (Zhang et al., 2004; Zhu et al., 2018). The 18α-30-norhopane (C29Ts) followed by C2917α-hopane (C29H) on m/z 191 mass chromatogram was identified using the advanced nuclear magnetic resonance (NMR) method (Moldowan et al., 1991). Similar to parameter Ts/(Ts+Tm), C29Ts/(C29Ts+C29H) ratio is often used as a parameter reflecting the maturity of crude oil from the immature to mature stage (Fowler and Brooks, 1990). The Ts/(Ts+Tm) ratios of crude oils in Yakela Field range from 0.37–0.63, with an average of 0.52. The Ts/(Ts+Tm) value of crude oils in Shunbei Field range from 0.51–0.88, with an average of 0.67 (Table 2). The C29Ts/(C29Ts+C29H) ratios of crude oils in Yakela Field range from 0.25–0.36, with an average of 0.31. However, the C29Ts/(C29Ts+C29H) value of crude oils in Shunbei Field range from 0.31–0.49, with an average of 0.48 (Table 2). The intersection diagram can effectively classify the maturity of crude oil when evaluating oils from common source facies and depositional settings (Peters et al., 2005). Both fields belong to Tabei uplift, and their source rocks have similar lithology and organic facies (Zhang et al., 2004; Zhu et al., 2018). The cross plot of C29Ts/(C29Ts+C29H) versus Ts/(Ts+Tm) indicates that the maturities of crude oils in both fields reach the mature stage, but there are differences between them. The crude oils in Yakela Field are generally in the mature stage, with Ts/Ts+Tm values between 0.3 and 0.7 (About 0.7%Ro-1.0%Ro) (Peters et al., 2005). The crude oils in Shunbei Field are in the mature and high mature stages, with the value of Ts/(Ts+Tm) greater than 0.7 (About 1.0%Ro) in most of the samples, and the max value reached 0.88 (About 1.3%Ro) (Peters et al., 2005) (Figure 5A). It indicates that the maturity of the latter sample is higher than that of the former.
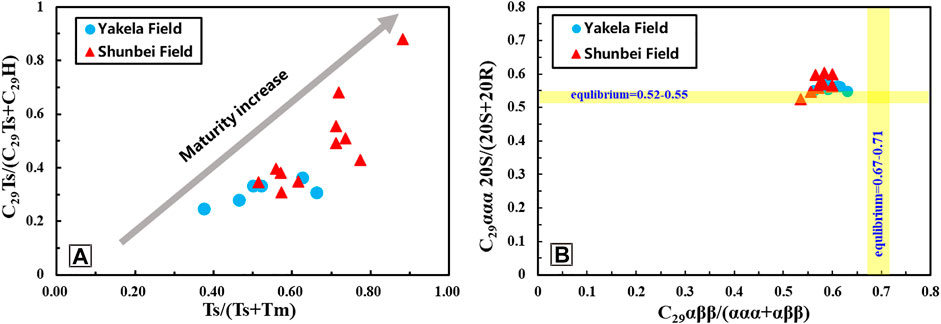
FIGURE 5. Cross plot showing the maturity of the oils in Yakela Field and Shunbei Field, Tarim Basin. (A) Cross plot of C29Ts/(C29Ts+C29H) and Ts/(Ts+Tm); (B) Cross plot of C29ααα 20S/(20S+20R) sterane and C29αββ/(ααα+αββ) sterane.
N-alkanes in all crude oil samples in the study area are completely distributed, without obvious unresolved complex mixture (UCM) hump. Low carbon number n-alkanes (C6-C12) were not eliminated (Figure 4). At the same time, no 25-norhopane could be identified in all crude oil samples; it indicated that the crude oils from Shunbei Field and Yakela Field have not been subjected to biodegradation (Reed, 1977; Peters et al., 2005). The carbon preference index (CPI, the calculation equation is shown in Table 2) and odd–even dominance index (OEP, the calculation equation is shown in Table 2) can be used to make a preliminary assessment of the thermal maturity of crude oil, although the ratios can be affected by source and biodegradation. (Bray and Evans, 1961; Scalan and Smith, 1970). The CPI ratio of crude oils in Yakela Field ranges from 1.14 to 1.3, with an average of 1.18. The OEP ratio ranges from 0.97 to 0.99, with an average of 0.98 (Table 2). The CPI and OEP ratios of crude oils in Shunbei Field range from 1.11 to 1.29 and from 0.97 to 1.01, with an average of 1.20 and 0.98, respectively (Table 2). The CPI and OEP values of crude oils from the two fields are roughly the same. Both are close to 1, suggesting that they are thermal mature. However, Ts/(Ts+Tm) values showed that the maturity of crude oils from Shunbei Field is higher than that from Yakela Field. This result may be due to the fact that the crude oil maturity of the two fields has reached the maturity stage, so the values of the two fields are close to 1, leading to the insignificant difference between the values of CPI and OEP.
The isomerization of C295α,14α,17α(H)-steranes on C-20 increases the 20S/(20S+20R) ratio from 0 to an equilibrium value of about 0.5 (0.52–0.55, 0.9%Ro) with increasing maturity (Seifert and Moldwan., 1986). The parameter is suitable for the maturity evaluation of samples from the immature to mature stage (<0.9%Ro) and may be affected by the difference in organic phase, weathering, and biodegradation (Moldowan and Figo, 1986; Peters et al., 2005). The isomerization of 20S and 20RC29 regular steranes at C-14 and C-17 sites increases the ββ/(αα+ββ) ratio from close to 0 to 0.7 (equilibrium value 0.67 to 0.71, 0.9%Ro) with increasing maturity (Seifert and Moldowan., 1986). The parameter is suitable for maturity evaluation from the immature to mature stage (<0.9%Ro) and is less affected by parent material input (Moldowan and Figo, 1986; Peters et al., 2005). The C29ααα 20S/(20S+20R) ratios of crude oils in Yakela Field range from 0.55 to 0.58, with an average of 0.56. The C29αββ/(ααα+αββ) ratios of crude oils in Yakela Field range from 0.56 to 0.63, with an average of 0.60 (Table 2). The C29ααα20S/(20S+20R) and C29αββ/(ααα+αββ) values of crude oils from Shunbei Field range from 0.52 to 0.60 and from 0.54 to 0.60, with the averages of 0.57 and 0.58 (Table 2). As displayed in Figure 5B, all the samples from two fields have C29ααα20S/(20S+20R) sterane isomerization ratios reaching equilibrium values (0.52–0.55, 0.9%Ro), suggesting that the maturities reached 0.9%Ro according to Justwan et al. (2006). Although the values of all samples reached the equilibrium point, the values of the crude oils from Shunbei Field were slightly higher than those from Yakela Feild, indicating that the maturity of the crude oils from the two fields may be different. According to Peters et al. (2005), in the mature and high maturity stages (>1.0%Ro), the Ts/(Ts+Tm) is considered more reliable than C29ααα20S/(20S+20R) in evaluating the maturity of crude oils when evaluating oils from a common source with a common source facies and depositional setting. Therefore, combined with Ts/(Ts+Tm) values, it is inferred that the maturity of crude oils in Yakela Field was in the mature stage and in the peak stage of hydrocarbon generation (About 0.9%Ro - 1.0%Ro), and the maturity of crude oils in Shunbei Field was in the mature and high mature stage, the present maturities of partial samples are greater than that of the peak oil generation stage (About 1.0%Ro - 1.3%Ro).
Alkylated dibenzothiophenes (DBTs), as heterocyclic compounds, are generally assigned to aromatic sulfur compounds. It mainly contains DBT and its C1-∼ C3-substituted alkyl derivatives, which usually exist in the aromatic fractions of crude oil or sediment (Hughes, 1984; Connan et al., 1986). Previous studies have proved that DBTs molecular parameters can be used to study the maturity of crude oil from the mature to high mature stage when evaluating oils from common source facies and depositional settings (Hughes, 1984; Redke et al., 1986). Especially Methyldibenzothiophene ratio MDR (4-/1-MDBT) is considered to be an effective maturity parameter for the maturity evaluation of samples from the mature to over mature stage (>0.8%Ro) (Connan et al., 1986; Chakhmakhchev et al., 1997; Radke et al., 1986; Luo et al., 2001). Alkylated dibenzothiophenes isomers with substituents show different thermal stability at different carbon sites of benzene ring. The isomer substituted by C-4(β) alkyl group is the most stable. C-1(α) alkyl–substituted isomers are the most unstable; The thermal stability of the isomers substituted at C-2 or C-3 is in the order between the two. Therefore, the descending sequence of thermal stability of alkyl DBTs isomers is C-4>C-2>C-3>C-1 (Budzinski et al., 1991). Therefore, as the maturity of crude oils increases, the value of 4-/1-MDBT will also increase (Chakhmakhchev et al., 1997; Luo et al., 2001). The 4-/1-MDBT ratio ranges from 8.64 to 14.00, with an average value of 11.48 in Yakela Field. However, the 4-/1-MDBT ratio ranges from 18.96 to 26.94, with an average value of 24.24 in Shunbei Field (Table 2). It suggested that the maturity of crude oils in Shunbei Feld and Yakela Feld is different, and the maturity of crude oils in Shunbei Field is higher than that in Yakela Field.
In this study, the values of 4-/1-MDBT of crude oil samples from the Shunbei Field are greater than those from the Yakela Field (Table 2). The equivalent vitrinite reflectance Rc (Rc=0.036×(4-/1-MDBT)+0.56) (Huo et al., 2008) of crude oil samples in the study were calculated (Table 2). The Rc values of crude oils in Yakela Field range from 0.87–1.06, with an average of 0.97. The Rc value of crude oils in Shunbei Field range from 1.23–1.53, with an average of 1.43. According to the data, the maturity of the crude oils from Shunbei Field is higher than that from Yakela Field (Figure 6). The calculated maturity of crude oils from Yakela Field was consistent with the maturity reflected by the other abovementioned parameters, while the maturity of the crude oils from Shunbei Field was slightly higher than that calculated by the other parameters. It may be caused by the low concentration of some biomarker compounds due to the high maturity of crude oil, and some early charging residual crude oil may have an impact on partially saturated hydrocarbon maturity parameters in Shunbei Field (Yang et al., 2021). On the other hand, the maturity calculated by the maturity parameter (4-/1-MDBT) can only be an approximate value (Peters et al., 2005; Huo et al., 2008), but on the whole, it can still reflect that the maturity of the crude oils of Shunbei Field is higher than that of Yakela Field.
To sum up, the crude oils from both Yakela Field and Shunbei Field were in the mature stage, but the parameters reflected the difference in maturity between them to varying degrees. The maturity of crude oils in Yakela Field is in the peak stage of hydrocarbon generation (About 0.9%Ro-1.0%Ro), while that in Shunbei Field has passed the peak stage of hydrocarbon generation (About 1.0%Ro-1.3%Ro, may be up to 1.53%Ro). The maturity of crude oils in Shunbei Field was higher than that in Yakela Field.
4.2 Formation mechanism of light oils
In this study, an approximately NE-SW profile was selected, passing Y1, A1, T1, and S1 Wells from north to south, covering Yakela Field and Shunbei Field (Figure 1). The actual downhole data show that the Lower Cambrian source rocks in Shunbei Field have a high degree of thermal evolution with %Ro over 2.5 at present (Figure 7). However, the thermal evolution degree of the source rocks near Yakela Field in the northern part of the profile is relatively low, with %Ro about 1.50–1.70 (Figure 7).
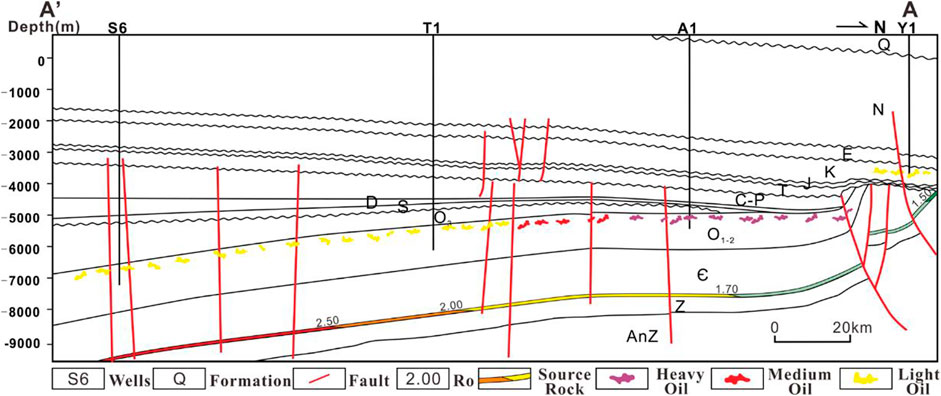
FIGURE 7. The tectonic profile of the study area contains the current thermal evolution of the source rocks of the Lower Cambrian Yuertusi Formation.
The hydrocarbon generation history of Lower Cambrian Yuertusi Formation source rocks widely distributed in the study area was simulated. The thermal evolution history of source rocks and the simulation results of hydrocarbon generation amount are shown in Figure 8. The hydrocarbon generation history results show that the source rocks in Shunbei Field reached the hydrocarbon generation threshold as early as before the late Caledonian (About 0.5%Ro) and reached the peak of hydrocarbon generation at the late Caledonian (About 1.0%Ro). The source rocks were in the main stage of oil generation from the late Caledonian to the early Hercynian, and from the late Hercynian to the Himalayan period were in the high maturity and over-maturity stage (Figure 8A). The simulation results of hydrocarbon generation amount of source rocks showed that the source rocks mainly generated hydrocarbon from before the late Caledonian to the early Hercynian (About 2.5 Mtons), and the amount of hydrocarbon generated from the late Hercynian to the present is small (About 0.3 Mtons) (Figure 8C). The source rocks near Yakela Field reached the threshold of hydrocarbon generation from late Caledonian to the early Hercynian (About 0.5%Ro) and reached the peak of hydrocarbon generation at the Himalayan period (About 1.0%Ro) (Figure 8B). The simulation results of hydrocarbon generation amount of source rocks showed that the source rocks mainly generated hydrocarbon at the late Hercynian (About 1.0 Mtons) and from the Himalayan period to the present (About 2.5 Mtons). (Figure 8D). These results indicated that the main hydrocarbon generation period of the source rocks in Shunbei Field is different from that of Yakela Field. The former mainly generated hydrocarbons from late Caledonian to early Hercynian, while the latter mainly generated hydrocarbons from the late Hercynian and Himalayan period to the present.
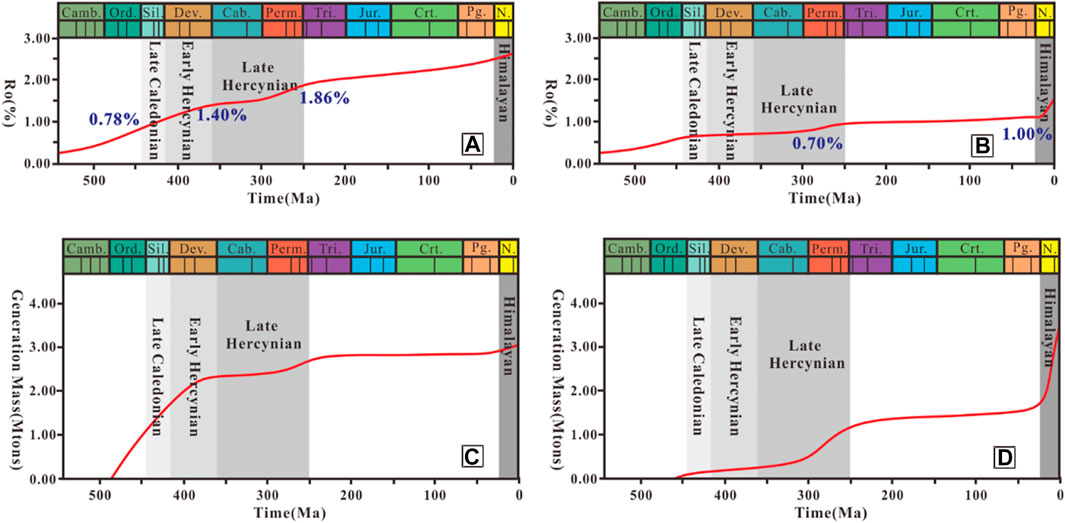
FIGURE 8. Thermal evolution history of source rocks and the amount of hydrocarbon generation in each period. (A) the history of source rocks in Shunbei Field; (B) the history of source rocks in Yakela Field; (C) the amount of hydrocarbon generation in Shunbei Field; (D) the amount of hydrocarbon generation in Yakela Field.
Therefore, the maturity of crude oils in Yakela Field is consistent with the degree of the thermal evolution of source rocks since the Himalayan period (Figures 8B and 5). However, the maturity of crude oils in Shunbei Field is higher than the degree of the thermal evolution of local source rocks during the peak of hydrocarbon generation (Figures 8A and 5). It indicates that the high-maturity crude oils in the two areas have different genetic models.
The genetic model of light oils in Shunbei Field is mainly through early oil generation, early charging, and then deep burial in the reservoir, with subsequent maturation cracking. The maturity of crude oils in the present reservoir (About 1.0%Ro-1.3%Ro, may be up to 1.53%Ro) is higher than the thermal evolution of source rocks in the main hydrocarbon generation stage (About 0.8%Ro-1.0%Ro). When the source rock evolved to the maturity corresponding to the present crude oils, it was already in the mature and high mature stage, and the amount of hydrocarbon generation was very small (About 0.3 Mtons). Therefore, the existing high-maturity crude oils cannot be directly generated by source rocks and should be the result of deep burial and maturation in the reservoirs.
The genetic model of light oils in the Yakela Field is mainly formed by the direct charging of late source rocks into reservoirs. The simulation results of hydrocarbon generation history show that the source rocks are in the window of hydrocarbon generation, and the amount of hydrocarbon generation has been high (About 2.5 Mtons) since the Himalayan period. The maturity of crude oils in the present reservoirs (About 0.9%Ro-1.0%Ro) is also consistent with the thermal evolution stage of source rocks in the main hydrocarbon generation stage. It also indicates that hydrocarbons generated from source rocks in this field were directly charged into the reservoirs during hydrocarbon generation and maintained the thermal evolution characteristics of the source rocks without the interference of subsequent geologic alteration processes such as deep burial and maturation such as the secondary thermal cracking.
5 Conclusion
1) The molecular geochemical characteristics show that the light oils in the two areas are sourced from similar marine source rocks deposited under the weak oxidative and reductive environment. The maturities of crude oils in Yakela Field and Shunbei Field were in the mature stage, but the parameters reflected the difference in maturity between them to varying degrees. On the whole, the maturity of crude oil in Shunbei Field was higher than that in Yakela Field.
2) The formation mechanism of the two light oils is different. The light oil in Yakela Field was directly generated by the source rock in the oil-generating window. The crude oil in Shunbei Field was formed by the deep burial and maturation of the crude oil generated in the early stage of the source rock. This study is of great significance for further clarifying the genesis mechanism of light oil and gas in the deep Tarim Basin, guiding further deep oil and gas exploration and discovering new large-scale condensate and natural gas fields.
Data availability statement
The original contributions presented in the study are included in the article/Supplementary Material; further inquiries can be directed to the corresponding author.
Author contributions
All authors listed have made a substantial, direct, and intellectual contribution to the work and approved it for publication.
Funding
This study was funded by the Strategic Priority Research Program of the Chinese Academy of Sciences (Grant No. XDA14010305) and the National Natural Science Foundation of China (Grants Nos. 41821002 and 41872159).
Conflict of interest
YW was employed by Northwest Oil Company, SINOPEC.
The remaining authors declare that the research was conducted in the absence of any commercial or financial relationships that could be construed as a potential conflict of interest.
Publisher’s note
All claims expressed in this article are solely those of the authors and do not necessarily represent those of their affiliated organizations, or those of the publisher, the editors, and the reviewers. Any product that may be evaluated in this article, or claim that may be made by its manufacturer, is not guaranteed or endorsed by the publisher.
References
Alberdi, M., Moldowan, J. M., Peters, K. E., and Dahl, J. E. (2001). Stereoselective biodegradation of tricyclic terpanes in heavy oils from the Bolivar Coastal Fields, Venezuela. Org. Geochem. 32, 181–191. doi:10.1016/s0146-6380(00)00130-3
Bray, E. E., and Evans, E. D. (1961). Distribution of n-paraffins as a clue to recognition of source beds. Geochim. Cosmochim. Acta 22, 2–15. doi:10.1016/0016-7037(61)90069-2
Brooks, P. W., Gould, K., and Smith, J. W. (1969). Isoprenoid hydrocarbons in coal and petroleum. Nature 222, 257–259. doi:10.1038/222257a0
Budzinski, H., Garrigues, P., and Connan, J. (1991). “Determination of maturity indicators in alkylated aromatic series by gas chromatography-mass spectrometry (GC-MS),” in Organic Geochemistry: Adevances and applications in energy and the natural environment. 15th meeting of the EAOG, poster abstracts. Editor D. A. C. Manning (Manchester: Manchester University Press), 619–623.
Cai, X. Y., and Li, Y. (2008). Ordovician lithofacies and stratigraphic lacunae in the southern part of the Central Tarim, Xinjiang. J. Stratigr. v 32 (4), 353–362. in Chinese with English abstract.
Chakhmakhchev, A., Suzuki, M., and Takayama, K. (1997). Distribution of alkylated dibenzothiophenes in petroleum as a tool for maturity assessments. Org. Geochem. 26 (7-8), 483–489. doi:10.1016/s0146-6380(97)00022-3
Chang, X. C., Wang, T. G., Li, Q. M., and Ou, G. X. (2013). Charging of Ordovician reservoirs in the Halahatang depression (Tarim Basin, NW China) determined by oil geochemistry. J. Petroleum Geol. 36, 383–398. doi:10.1111/jpg.12562
Cheng, B., Liu, H., Cao, Z., Wu, X., and Chen, Z. (2020). Origin of deep oil accumulations in carbonate reservoirs within the north tarim Basin: Insights from molecular and isotopic compositions. Org. Geochem. 139, 103931. doi:10.1016/j.orggeochem.2019.103931
Connan, J., Bouroullec, J., and Dessort, D. (1986). “The microbial input in carbonate-anhydrite facies of sabkha palaeoenvironment from Guatemala: A molecular approach,”. Editors D. Leythhaeuser, and J. Rullkötter, Org. Geochem, 10, 29–50. doi:10.1016/0146-6380(86)90007-0
Connan, J., and Cassau, A. M. (1980). Properties of gases and petroleum liquids derived from terrestrial kerogen at various maturation levels. Geochim. Cosmochim. Acta 44, 1–23. doi:10.1016/0016-7037(80)90173-8
Dai, H. S., Zhao, X. K., Liu, S. G., Sun, W., Li, K., Yu, T. F., et al. (2009). The buried hill tructure and oil–gas accumulation of Pre-Mesozoic in Yakela fault bulge, Tarim Basin. Xinjiang Pet. Geol. 30, 17–20. in Chinese with English abstract.
Didyk, B. M., Simoneit, B. R. T., Brassell, S. C., and Eglinton, G. (1978). Organic geochemical indicators of palaeoenvironmental conditions of sedimentation. Nature 272, 216–222. doi:10.1038/272216a0
Dieckmann, V., Horsfield, B., and Schenk, H. J. (2000). Heating rate dependency of petroleum-forming reactions: Implications for compositional kinetic predictions. Org. Geochem. 31 (12), 1333–1348. doi:10.1016/s0146-6380(00)00105-4
Fowler, M. G., and Brooks, P. W. (1990). Organic geochemistry as an aid in the interpretation of the history of oil migration into different reservoirs at the Hibernia K-18 and Ben Nevis I-45 wells, Jeanne d'Arc Basin, offshore eastern Canada. Org. Geochem. 16, 461–475. doi:10.1016/0146-6380(90)90062-5
Grantham, P. J. (1986). The occurence of unusual C27 and C29 sterane predominances in two types of Oman crude oil. Org. Geochem. 9, 1–10. doi:10.1016/0146-6380(86)90077-x
Gu, Y., Huang, S. W., Jia, C. S., Shao, Z. B., Sun, Y. G., and Lu, Q. H. (2020). Research progress on marine oil and gas accumulation in Tarim Basin. Petroleum Geol. Exp. 42 (01), 1–12. in Chinese with English abstract.
Hanson, A. D., Zhang, S. C., Moldowan, J. M., Liang, D. G., and Zhang, B. M. (2000). Molecular organic geochemistry of tarim Basin, NW China. AAPG Am. Assoc. Pet. Geol.) Bull. 84, 1109–1128.
He, B., Jiao, C., Xu, Z., Cai, Z., Zhang, J., Liu, S., et al. (2016). The paleotectonic and paleogeography reconstructions of the Tarim Basin and its adjacent areas (NW China) during the late Early and Middle Paleozoic. Gondwana Res. 30, 191–206. doi:10.1016/j.gr.2015.09.011
Hughes, W. B. (1984). “Use of thiophenic organosulfur compounds in characterizing crude oils derived from carbonate versus siliclastic sources,” in Petroleum Geochemistry and source rock potential of carbonate rocks. Editor J. B. Palacas (Oklahoma: AAPG Bull), 18, 181–196.
Huo, Q. L., Li, Z. G., Fu, L., and Gao, M. (2008). Study on the correlation of methyldibenzothiophenes distribution and organic maturity. Petroleum Geol. Oilfield Dev. Daqing 2008 (02), 32–35. in Chinese with English abstract.
Jia, C. (1997). Tectonic characteristics and petroleum. Tarim basin, China (in Chinese): Beijing: Petroleum Industry Press, 295.
Jia, C., and Wei, G. (2002). Structural characteristics and petroliferous features of Tarim Basin. Chin. Sci. Bull. 47, 1–11. doi:10.1007/bf02902812
Jiao, F. (2018). Significance and prospect of ultra-deep carbonate fault-karst reservoirs in Shunbei area, Tarim Basin. Oil Gas. Geol. 39, 207–216. in Chinese with English abstract.
Justwan, H., Dahl, B., and Isaksen, G. H. (2006). Geochemical characterisation and genetic origin of oils and condensates in the South Viking Graben, Norway. Mar. Pet. Geol. 23, 213–239. doi:10.1016/j.marpetgeo.2005.07.003
Li, M. J., Wang, T. G., Chen, J. F., He, F. Q., Yun, L., Akbar, S., et al. (2010). Paleo-heat flow evolution of the Tabei uplift in tarim Basin, northwest China. J. Asian Earth Sci. 37 (1), 52–66. doi:10.1016/j.jseaes.2009.07.007
Li, M. J., Wang, T. G., Li, H. B., Fang, R. H., Yang, L., Shi, S. B., et al. (2016). Occurrence and geochemical significance of phenylnaphthalenes and terphenyls in oils and condensates from the Yakela faulted uplift, tarim Basin, northwest China. Energy fuels. 30 (6), 4457–4466. doi:10.1021/acs.energyfuels.5b02697
Li, X. L., Liu, S. W., and Feng, C. G. (2019). Thermal properties of sedimentary rocks in the Tarim Basin, northwestern China. Am. Assoc. Pet. Geol. Bull. 103 (7), 1605–1624. doi:10.1306/11211817179
Lin, C. S., Yang, H. J., Liu, J. Y., Rui, Z. F., Cai, Z. Z., and Zhu, Y. F. (2012). Distribution and erosion of the paleozoic tectonic unconformities in the tarim Basin, northwest China: Significance for the evolution of paleo-uplifts and tectonic geography during deformation. J. Asian Earth Sci. 46, 1–19. doi:10.1016/j.jseaes.2011.10.004
Liu, Y. F., Qiu, N. S., Hu, W. X., Li, H. L., Shen, F. Y., and Yao, Q. Y. (2019). Temperature and pressure characteristics of Ordovician gas condensate reservoirs in the Tazhong area, Tarim Basin, northwestern China. Am. Assoc. Pet. Geol. Bull. 103 (6), 1351–1381. doi:10.1306/1115181617017043
Lu, Z., Chen, H., Qing, H., Chi, G., Chen, Q., You, D., et al. (2017). Petrography, fluid inclusion and isotope studies in Ordovician carbonate reservoirs in the Shunnan area, Tarim basin, NW China: Implications for the nature and timing of silicification. Sediment. Geol. 359, 29–43. doi:10.1016/j.sedgeo.2017.08.002
Luo, J., Cheng, L. M., Fu, L. X., Hu, Y. J., and Jiang, N. H. (2001). Alkylated dibenzothiophene index—a new method to assess thermal maturity of source rocks. Acta Pet. Sin. 22 (3), 27–31. in Chinese with English abstract.
Luo, X. L., Tang, L. J., Xie, D. Q., Qiu, H. J., Jiang, H. S., Yang, Y., et al. (2012). Structural styles and hydrocarbon accumulation in Yakela fault-convex, Tarim Basin. Pet. Geol. Recovery Effic. 19, 38–41. [in Chinese with English abstract].
Ma, Y. S., Cai, X. Y., Yu, L., Li, Z. J., Li, H. L., Deng, S., et al. (2022). Practice and theoretical and technical progress in exploration and development of Shunbei ultra-deep carbonate oil and gas field, Tarim Basin, NW China. Petroleum Explor. Dev. 49 (01), 1–20. in Chinese with English abstract. doi:10.1016/s1876-3804(22)60001-6
Moldowan, J. M., and Fago, F. J. (1986). Structure and significance of a novel rearranged monoaromatic steroid hydrocarbon in petroleum. Geochimica Cosmochimica Acta 50, 343–351. doi:10.1016/0016-7037(86)90188-2
Moldowan, J. M., Lee, C. Y., Watt, D. S., Jeganathan, A., Slougui, N. E., and Gallegos, E. J. (1991). Analysis and occurrence of C26-steranes in petroleum and source rocks. Geochimica Cosmochimica Acta 55, 1065–1081. doi:10.1016/0016-7037(91)90164-z
Peters, K. E. (2000). Petroleum tricyclic terpanes: Predicted physicochemical behavior from molecular mechanics calculations. Org. Geochem. 31, 497–507. doi:10.1016/s0146-6380(00)00029-2
Peters, K. E., Walters, C. C., and Moldowan, J. M. (2005). The biomarker guide: Biomarkers and isotopes in petroleum exploration and Earth history, 2. Cambridge: Cambridge University Press.
Powell, T. G., and McKirdy, D. M. (1973). Relationship between ratio of pristane to phytane, crude oil composition and geological environment in Australia. Nat. Phys. Sci. 243, 37–39. doi:10.1038/physci243037a0
Radke, M., Welte, D. H., and Willsch, H. (1986). Maturity parameters based on aromatic hydrocarbons: Influence of the organic matter type. Org. Geochem. 10, 51–63. doi:10.1016/0146-6380(86)90008-2
Reed, W. E. (1977). Molecular compositions of weathered petroleum and comparison with its possible source. Geochimica Cosmochimica Acta 41, 237–247. doi:10.1016/0016-7037(77)90231-9
Scalan, R. S., and Smith, J. E. (1970). An improved measure of the odd-even predominance in the normal alkanes of sediment extracts and petroleum. Geochimica Cosmochimica Acta 34, 611–620. doi:10.1016/0016-7037(70)90019-0
Seifert, W. K., and Moldowan, J. M. (1978). “Applications of steranes, terpanes and monoaromatics to the maturation, migration and source of crude oils,” in Methods in Geochemistry and Geophysics Vol. 24 (Amsterdam: Elsevier), 261–290.
Seifert, W. K., and Moldowan, J. M. (1986). Use of biological markers in petroleum exploration. Methods Geochem. Geophys. 24, 261–290.
Snowdon, L. P., and Powell, T. G. (1982). Immature oil and condensate modification of hydrocarbon generation model for terrestrial organic matter. AAPG Bull. 69 (6), 946–949. doi:10.1306/03B5A313-16D1-11D7-8645000102C1865D
Thompson, K. (1983). Classification and thermal history of petroleum based on light hydrocarbons. Geochimica Cosmochimica Acta 43 (2), 303–316. doi:10.1016/0016-7037(83)90143-6
Thompson, K. (1987). Fractionated aromatic petroleums and the generation of gas-condensates. Org. Geochem. 11 (6), 573–590. doi:10.1016/0146-6380(87)90011-8
Tissot, B. P., and Welte, D. H. (1978). Petroleum formation and occurrence. New York: Spring Verlag.
Volkman, J. K. (1986). A review of sterol markers for marine and terrigenous organicmatter. Org. Geochem. 9, 83–99. doi:10.1016/0146-6380(86)90089-6
Volkman, J. K., Barrett, S. M., Blackburn, S. I., Mansour, M. P., François, G., and Gelin, F. (1998). Microalgal biomarkers: A review of recent research developments. Org. Geochem. 29, 1163–1179. doi:10.1016/s0146-6380(98)00062-x
Wang, Q., Hao, F., Cao, Z., Tian, J., and Cong, F. (2021). Geochemistry and origin of the ultra-deep ordovician oils in the Shunbei field, tarim Basin, China: Implications on alteration and mixing. Mar. Pet. Geol. 123, 104725. doi:10.1016/j.marpetgeo.2020.104725
Yang, H., Deng, X., Zhang, Y., Xie, Z., Li, Y., Li, S., et al. (2020). Great discovery and its significance of exploration for Ordovician ultra-deep fault-controlled carbonate reservoirs of Well Manshen 1 in Tarim Basin. China Pet. Explor. 25 (3), 13–23.
Yang, P., Liu, K. Y., Liu, J. L., Yu, S., Yu, B., Hou, M. G., et al. (2021). Petroleum charge history of deeply buried carbonate reservoirs in the Shuntuoguole Low Uplift, Tarim Basin, west China. Mar. Pet. Geol. 128, 105063. doi:10.1016/j.marpetgeo.2021.105063
Zhang, S. C., Huang, H. P., Su, J., Liu, M., Wang, X. M., and Hu, J. (2005). Geochemistry of palaeozoic marine petroleum from the tarim Basin, NW China: Part 1. Oil family classification. Org. Geochem. 36 (8), 1204–1214. doi:10.1016/j.orggeochem.2005.01.013
Zhang, S. C., Liang, D. G., and Zhang, B. M. (2004). formation of marine oil and gas in tarim Basin. Beijing: Petroleum Industry Press.
Zhang, S. C., Su, J., Wang, X. M., Zhu, G., Yang, H., Liu, K., et al. (2011). Geochemistry of palaeozoic marine petroleum from the tarim Basin, NW China: Part 3. Thermal cracking of liquid hydrocarbons and gas washing as the major mechanisms for deep gas condensate accumulations. Org. Geochem. 2011 42 (11), 1394–1410. doi:10.1016/j.orggeochem.2011.08.013
Zhang, S. C., Su, J., Zhang, B., and Wang, X. M. (2021). Genetic mechanism and controlling factors of deep Marine light oil and condensate in Tarim Basin. Acta Patroleum Sin. 42 (12), 1566–1580. in Chinese with English abstract.
Zhang, S., Hanson, A. D., Moldowan, J. M., Graham, S. A., Liang, D., Chang, E., et al. (2000). Paleozoic oil–source rock correlations in the Tarim Basin, NW China. Org. Geochem. 31, 273–286. doi:10.1016/s0146-6380(00)00003-6
Zhu, G. Y., Chen, F. R., Wang, M., Zhang, Z. Y., Ren, R., and Wu, L. (2018). Discovery of the lower Cambrian high-quality source rocks and deep oil and gas exploration potential in the Tarim Basin, China. Am. Assoc. Pet. Geol. Bull. 102 (10), 2123–2151. doi:10.1306/03141817183
Keywords: light oil, genesis, Shunbei, Yakela, maturation
Citation: Wang Y, Zhang S, Xie H, Wang Q and Tian J (2023) Characteristics and genesis of light oil in Tabei Area, Tarim Basin, Northwestern China. Front. Earth Sci. 10:922311. doi: 10.3389/feart.2022.922311
Received: 17 April 2022; Accepted: 01 August 2022;
Published: 11 January 2023.
Edited by:
Tao Wen, Syracuse University, United StatesReviewed by:
Palma Botterell, United States Geological Survey (USGS), United StatesYan Li, Jinan University, China
Copyright © 2023 Wang, Zhang, Xie, Wang and Tian. This is an open-access article distributed under the terms of the Creative Commons Attribution License (CC BY). The use, distribution or reproduction in other forums is permitted, provided the original author(s) and the copyright owner(s) are credited and that the original publication in this journal is cited, in accordance with accepted academic practice. No use, distribution or reproduction is permitted which does not comply with these terms.
*Correspondence: Jinqiang Tian, dGlhbmpxQHVwYy5lZHUuY24=