- 1Research Institute of Exploration and Development, PetroChina Xinjiang Oilfield Company, Karamay, China
- 2Institute of Energy, Peking University, Beijing, China
- 3Operation Area of Fengcheng Oilfield, PetroChina Xinjiang Oilfield Company, Karamay, China
- 4Research Institute of Petroleum Exploration and Development, PetroChina, Beijing, China
- 5Institute of Geology and Geophysics, Beijing, China
High organic matter abundance is necessary for large-scale hydrocarbon accumulation and enrichment in shale oil. Comparing conventional marine basins with lacustrine ones, the formation of organic matter in the latter usually changes rapidly with strong heterogeneity, which brings great challenges to identify sweet spot prediction and resource evaluation. In addition, water columns with different redox conditions also affect the burial and preservation of organic matter during the deposition of lacustrine shales, especially under the impact of ephemeral critical geological events such as volcanic activities. Therefore, determining the properties and the influencing factors on the water columns is one of the key scientific issues in revealing the differential enrichment of organic matter in such basins. By comparing the petrological and geochemical characteristics of organic-rich shales between a typical freshwater and a saline lacustrine basin, this study analyzes the depositional environment and water column properties during the burial and preservation of organic matter in the Ordos and Junggar basins. The results demonstrate that volcanic activity intensifies the degree of hypoxia during the formation of organic-rich matter, which in turn affects organic matter preservation. The sulfate reduction index (SRI) indicates that the organic-rich shale of the Chang seven Member (Ch7) of Yanchang Formation in the Ordos Basin and the Lucaogou Formation (P2l) in the Jimsar Sag of Junggar Basin in freshwater-saline environments have a certain intensity of sulfate reduction (BSR) (SRI <1.375). The organic matter consumed by BSR is lower than the preserved organic matter, resulting a higher TOC content. However, excessive volcanic activity or the input of hydrothermal fluids caused strong BSR (SRI >1.375) of the Fengcheng Formation (P2f) in saline lake of the Mahu Sag in Junggar Basin. This indicates that a large amount of organic matter was degraded and consumed, causing more iron oxides (and other iron-containing minerals) dissolve gradually to form pyrite, reducing the TOC content. This study enabled us to understand the shale oil enrichment, sweet spot prediction and evaluation, and propose a better theoretical and practical model for hydrocarbon accumulation in shale formations, to guide future exploration efforts.
Highlights
1) The geological and geochemical characteristics of the organic-rich shales in the Ordos and the Junggar basins were analyzed.
2) The volcanic impacts on the formation of organic-rich shales from the freshwater to saline lakes was compared.
3) Volcanic activity is more favorable to the formation and preservation of organic matter in freshwater-saline lacustrine basins.
Introduction
The formation of organic-rich shale is resulting interaction between global and regional key geological events in the lithosphere, hydrosphere, atmosphere, and biosphere (Aller, 1994; MacKenzie, 2004; Ridgwell and Zeebe, 2005; Ridgwell and Hargreaves, 2007; Kim et al., 2015; LaRowe et al., 2020; Makeen et al., 2020). In this regard, considering the size of the basin in relation to such events, lacustrine depositional basins are much smaller than marine ones. This means organic matter preservation would be more sensitive to variations in this basin compared to marine basins. Therefore, it can be said deposition, burial and preservation of organic matter in lacustrine basins should be more complicated. This process becomes more challenging when volcanism is involved. This is due to the fact that major source rocks in the world are deposited with volcanic ash layers, i.e., the Bazhenov Formation in the West Siberia in Russia, the Bakken Formation in the Williston Basin, the Eagle Ford Formation in the Gulf Coast Basin, the Wufeng-Longmaxi Formation in the Sichuan Basin, the Ch7 Formation in the Ordos Basin, the P2l Formation in Jimsar Sag of Junggar Basin in China (Zhang et al., 2008; Zhang et al., 2009; Zhi et al., 2021; Dawson, 2000; Daviesg, 2004; Zhang et al., 2015; Yu et al., 2016; Zhang et al., 2011; Shen et al., 2019a; Shen et al., 2019b; Shen et al., 2019c; Yang et al., 2019; Ji et al., 2021; Liang et al., 2021; Liang et al., 2019). Volcanic activity is accompanied by high temperature and high-pressure mantle hydrothermal fluid flow, which changes the physical characteristics of the layers during the deposition of organic matter. The volcanic activity carries a large amount of CO2, CH4, and other gases, providing carbon-rich sources that will eventually move upward to the ground or the surface of the ocean. In addition, the volcanic activity releases huge amounts of nutritious elements such as N, Si, P, and other important trace metal elements such as Fe, Zn, Mn, Ni, V, etc., which will alter the surface and the water of the ocean’s chemical properties. The nutritious elements will enter the water in the basin and cause algae and bacteria to bloom, which will change the biological conditions of the water column. Therefore, it can be concluded that the formation of organic-rich shale is the result of various physical, chemical, and biological forces that come together as geological events.
It is relatively achieved for the understanding formation conditions and controlling factors of the organic matter preservation in marine environments. Most studies show that geological events such as volcanism can accelerate the formation of organic-rich matter by changing the reducing properties of water column (Berner, 1982; Hedges, 1987; Hedges, 1992; Hedges and Keil, 1995; Cui et al., 2016). Several studies have shown that from Archean to Neoproterozoic and even Phanerozoic, anoxic and ferruginous or euxinic water conditions for a long time has been dominant in the oceans globally (Chang et al., 2010; Yuan et al., 2014; Canfield et al., 2018; Bekker et al., 2010; Li et al., 2010; Konhauser et al., 2017; Li et al., 2018). Under such conditions, the degradation of organic matter by bacteria is inhibited, which is more conducive to the burial and preservation of organic carbon (Raiswell and Berner, 1986; Raiswell and Canfield, 1998; Shen et al., 2002; Shen, Knoll and Walter, 2003; Poulton, Fralick and Canfield, 2004; Poulton and Canfield, 2005; Canfield et al., 2008; Raiswell et al., 2008; Planavsky et al., 2011; Shen et al., 2011; Clarkson et al., 2014; Guilbaud et al., 2015; Clarkson et al., 2016; Thomas and Li, 2020). During the formation of organic-rich shales in lacustrine basins in China, the water column has been also anoxic in a wide range of time and space (Huang and Wang, 2015; Liu et al., 2018, 2020; Liu et al., 2021; Yuan et al., 2021), especially when there has been volcanic or hydrothermal input. However, it needs to be further studied with the relationship between petrological and geochemical characteristics of the organic matter with variations in minerals, elements and isotopes in the basin.
Therefore, in this research paper, we take the typical organic-rich shale in the Ordos Basin and the Junggar Basin as the research objects (Figure 1.), explore the synergistic mechanism between the anoxic environment and organic matter enrichment (deposition, burial and preservation), in order to provide a theoretical basis for more economical exploration and development of shale oil in lacustrine basins.
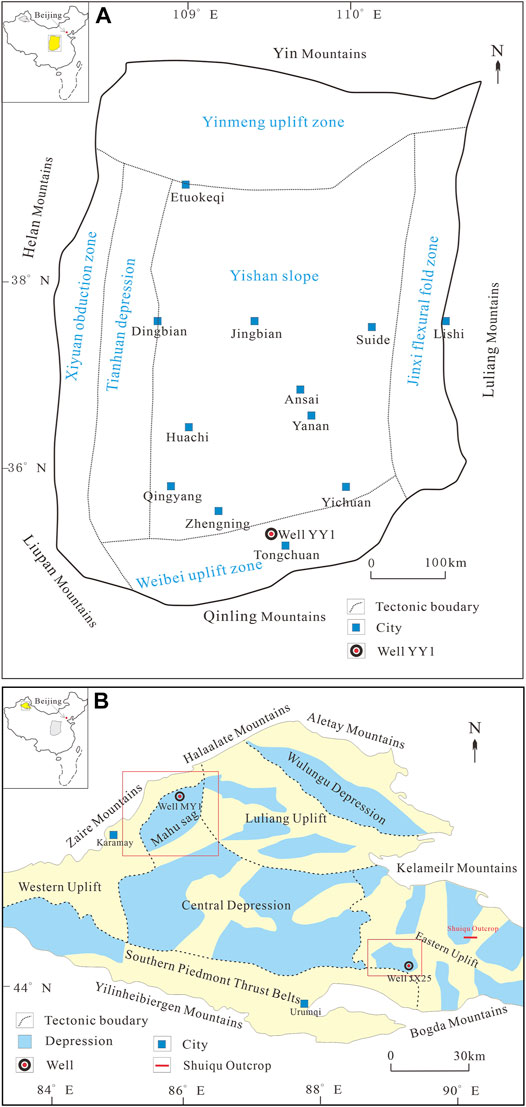
FIGURE 1. Location of the study wells: (A) The Well YY1 in the Ordos Basin; (B) The Well JX25 in Jimsar Sag, the Well MY1 in Mahu Sag, and Shuiqu outcrop, Junggar Basin (Modified from Zhang et al., 2008 and Zhi et al., 2019).
Geological Setting
The organic-rich shales of the Chang seven Member of Yanchang Formation (Ch7) in the Ordos Basin was formed in a freshwater lacustrine basin (Zhang et al., 2008; Zhang et al., 2009; Qiu et al., 2014). The Ordos Basin in the Late Triassic underwent a fundamental transition from neritic seas to lake deposits. During the depositional period of the Ch7, the basement of the Ordos Basin sank altogether, and the development of the lacustrine basin reached its peak. The water column of the lacustrine basin was significantly deepened, with a maximum water depth of 60 m. The depositional environment is quiet, the water was stagnant, and the deposition rate was low (Zhang et al., 2008; Zhang et al., 2009; Qiu et al., 2014; Andrew, Bradley, and Moldowan, 2007; Zhao et al., 1996). The Ch7 shale is about 100 m thick and the biogenic origin of the organic matter is mainly aquatic planktonic algae with rare terrestrial organic components (Yang and Zhang, 2005; Zhang et al., 2015). The Ch7 contains multiple layers of volcanic tuff of varying thickness, and the cores are mainly gray, gray-yellow, gray-white, and occasionally gray-green and gray-brown, indicating that the water column of the Ch7 has been a reducing sedimentary environment (Qiu et al., 2014; Liu et al., 2021). The majority of previous studies have employed a classical approach to model preservation of the organic matter in the study area with little attention to the anoxic conditions of the water and its consequences.
The Lucaogou Formation (P2l) was deposited in a fresh-saline lake with shallow to semi-deep lake and delta facies at the margins of the basin. The burial depth of the formation is about 2500–4500 m (Kuang et al., 2012,2014; Zhi et al., 2019; Wang L. B. et al., 2020). The Lucaogou Formation consists of different lithologies, with a large amount of terrigenous sediments and carbonates and a small amount of pyroclastic rocks, forming a mixed sedimentary system with a thickness of about 90–350 m (Jiang et al., 2015). Most prolific and highest producing layers are known as the “upper sweet spot” (P2l22) and the “lower sweet spot” (P2l12), that are divided by a thick mudstone layer (Kuang et al., 2012,2014). The crude oil that is being produced in the upper and lower sweet spots is mainly heavy oil, with a density of 0.88–0.92 g/cm3.
The Fengcheng Formation (P2f) in the Mahu Sag of the Junggar Basin is a typical organic-rich shale formed in a saltwater lacustrine basin. The depositional period of the P2f was the period of vigorous development of the western foreland basin system. The main lithology of the P2f is a multi-source mixture of fine-grained sedimentary startas that are deposited in a semi-deep-deep alkaline lake setting. In addition, the P2f is characterized by endogenous chemical deposits because of the arid and hot evaporative environments, as well as from volcanic materials provided by peripheral volcanic activities during the development of the foreland basins. Therefore, the formation can be considered as a combination of both conventional and unconventional oil and gas reservoir system (Zhi et al., 2021). Previous studies have confirmed that the source rocks of the P2f had a high degree of alkalization during the formation period (Liu et al., 2020; Zhi et al., 2021). Moreover, the paleoclimate of the P2f experienced a transition from semi-arid to wet, followed by semi-arid and arid, and finally semi-arid conditions. Furthermore, the water column in the basin experienced at least two periods of volcanic/hydrothermal activities (Zhang Y. Y. et al, 2018). Some researchers believe that the hydrothermal currents are the sole reason for the formation of lacustrine basin alkali water environment (He et al., 2018; Zhang et al., 2019), while others speculate the combination of evaporation and hydrothermal currents should be responsible (Chang et al., 2016; Yu et al., 2016; Zhang Z. J. et al, 2018; Wang Y. et al, 2020; Zhi et al., 2021). At the same time, the iron and sulfur elements input by volcanic activity also aggravated the degree of hypoxia during the deposition and preservation of organic matter. However, the investigation of anoxic water conditions in lacustrine basins requires further studies to fully address these ambiguities.
Samples and Methods
We select Well YY1 of the Ch7 of Yanchang Formation in Ordos Basin, and Well JX25 of the P2l in Jimusar Sag, Well MY1 of the P2f in Mahu Sag of Junggar Basin as research objects (Figure 1). The macroscopic sedimentary petrological observation of cores and field geological Shuiqu Profile, thin section analysis, TOC and TS tests were carried out on the research object. 120 samples were obtained from Well YY1 at 200–250 m in the Ordos Basin, 150 sampling points were obtained from the cores at 3461–3818 m of Well JX25 in the P2l of the Jimusar Sag, and 117 sampling points were obtained from cores at 4,585–4,950 m from Well MY1 in the P2f of the Mahu Sag.
The TOC was calculated according to the test results of Rock-Eval, the TS data was tested by the CS 900 high frequency infrared analyzer, the trace element Zr data of the wells JX25 and MY1 was tested by ICP-MS, and the Zr data of the YY1 well was intensively tested sieved by the handheld XRF instrument. Here, the water salinity and redox properties were evaluated in combination with trace element indicators; then we calculated the sulfate reduction index SRI value and evaluated the BSR intensity for judging the relationship between organic matter consumption and preservation, and lastly compared the formation and preservation mechanisms of organic-rich shale in freshwater and saline lacustrine basins by the influence of volcanic activity.
Results
Petrological and Geochemical Characteristics
Shiqu geologic profiles, core observations and thin section review was integrated by mineralogy and elemental analysis of the samples to identify fluid flow in deeper parts of the basin that is believed to be affected by volcanic activity. In the field geological Shuiqu outcrop of the Junggar Basin (Figure 1), the volcanic ash of the syn-sedimentary period was found to be significantly developed with different thicknesses. These volcanic ashes are yellow-brown, dark gray, light gray, gray-white, and purple-red due to different oxidizing or reducing environment depositional environments. However, in the core samples there are often thin interlayers of fused tuff or tuffaceous mud shale and tuffaceous siltstone with a thickness of about 2–3 mm. Tuff structures can be observed in these thin interlayers, but pure tuff layers are usually difficult to distinguish (Figure 2).
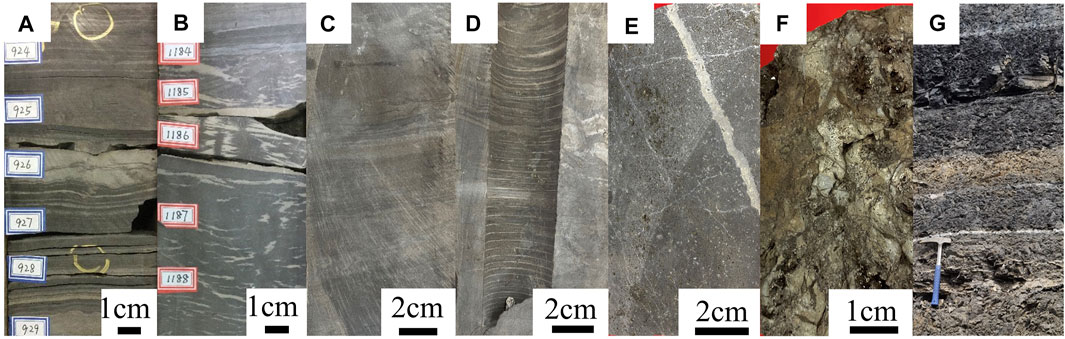
FIGURE 2. Cores samples of volcanic ash from the Ch7 of Yanchang Formation in Well YY1 (A), 230.64 m; (B), 243.48 m, the volcanic ash is mainly grayish white), the P2l in Well JX25 (C), 3680 m; (D), 3686.39 m, the volcanic ash is difficult to identify with the naked eye, mainly yellow-brown centimeter-millimeter scale), the P2f in Well MY1(E), 4,867.97 m; (F), 4,905.81 m, tuff structure), and the Shuiqu outcrop (G), outcrop location see Figure 1, the volcanic ash is layered and mainly yellowish brown or grayish white).
XRD analysis results show that there are different contents of heavy minerals such as zeolite and glauber’s salt vary among the samples. In the P2f and the P2l formations there are abundant tuffaceous silty mudstone, tuffaceous dolomitic mudstone and volcanic dust, where most of them are transported by the water flow. The Ch7 is mostly comprised of pure tuff with the thickness ranging from a few to a dozen centimeters, with a yellow to brown fluorescence under the microscope (Figure 3). These intervals are affected by volcanism that were rich in U, Mo, and other elements. The Zr content of Well YY1 is between 30.06 and 347.30 ppm (an average of 115.26 ppm), of Well JX25 is between 14.46 and 542.38 ppm (an average of 154.23 ppm), of Well MY1 is between 28.21 and 326.43 ppm (an average of 77.11 ppm) (Figure 4). Moreover, the rare earth elements (REE) have an obvious negative Eu anomaly, implying the deposition rate is relatively slow in the semi-deep and deeper parts of water. This condition could have been relatively favorable for the deposition and accumulation of organic matter.
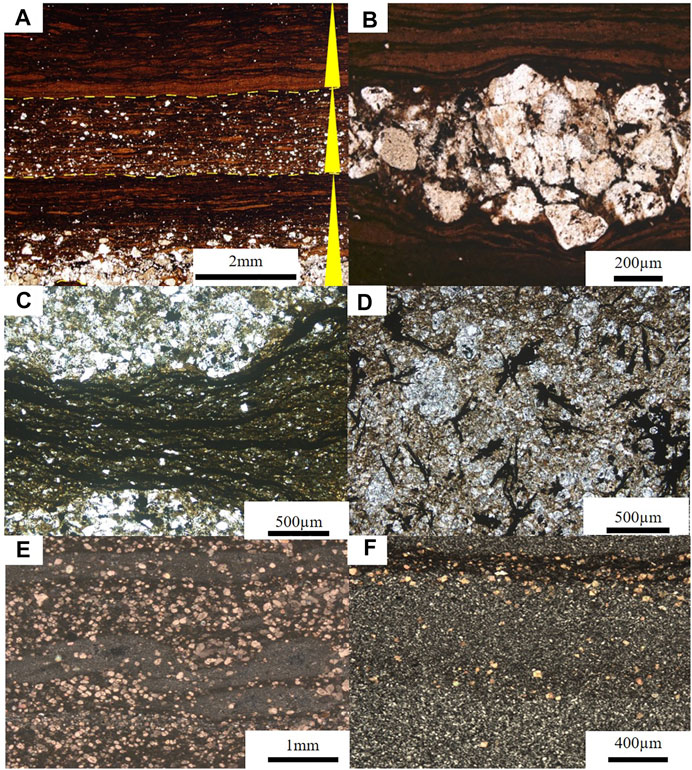
FIGURE 3. Typical volcanic ash thin section in the study area. (A) Two phases of lenticular clay-volcanic crystals developed in horizontally laminated shale, positive grain sequence, the Ch72 sub-member, 226 m, Well YY1; (B) Crystalline volcanic ash layer, the Ch72 sub-member, 228.44 m, Well YY1; (C) Volcanic debris in P2l, 3552.82 m, Well JX25; (D) Acicular pyrite formed by volcanic hydrothermal fluids in P2l, 3639.41 m, Well JX25; (E) Volcanic ash is distributed in silicified strips, tuffaceous dolomitic mudstone, 4,705.51 m, P2f, Well MY1; (F) Volcanic dust tuff, 4,708.69m, P2f, Well MY1.
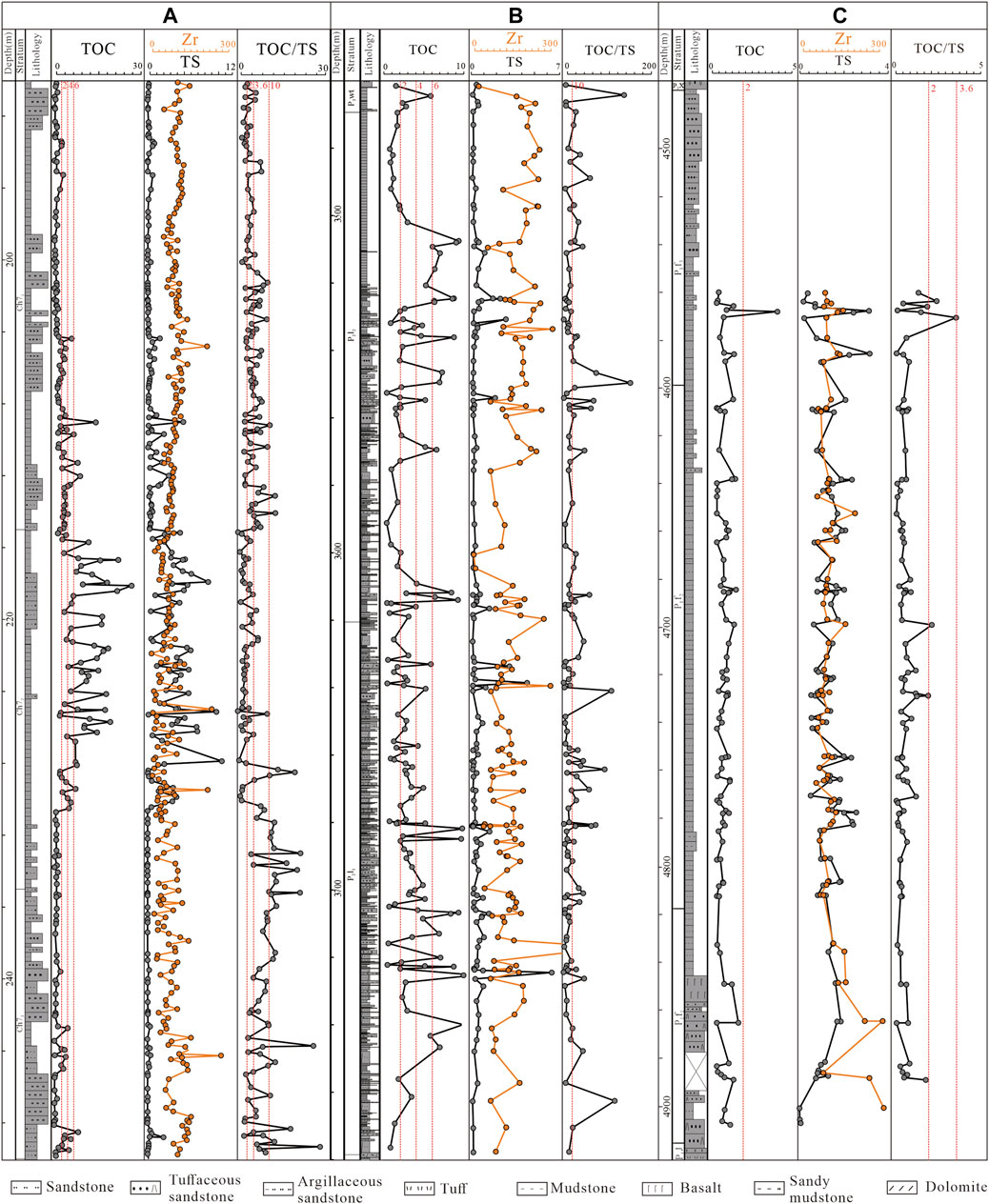
FIGURE 4. Vertical distribution column of TOC-TS and Zr element in the Ch7 of Yanchang Formation in Well YY1 (A), the P2l in Well JX25 (B), and the P2f in Well MY1 (C).
Geochemical Features of TOC-TS
The TOC content of 289 samples obtained from the Ch7 of Well YY1 in the Ordos Basin. ranges from 0.25 to 27.88 wt%, with an average of 4.14 wt%. The TS content is between 0.00 and 10.9 wt% (an average of 1.27 wt%), the TOC/TS ratio ranges from 0.25 to 56.71 (an average of 6.57), and the SRI value ranges from 1.01 to 4.04 (an average of 1.19). The TOC content of 151 samples obtained from the P2l of Well JX25 in the Jimusar Sag ranges from 0.07 to 11.79 wt% (an average of 3.74 wt%). The TS content is between 0.00 and 6.30 wt% (an average of 0.42 wt%), the TOC/TS ratio ranges from 0.24 to 160.00 (an average of 22.33), and the SRI value ranges from 1.00 to 2.90 (an average of 1.10). The TOC content of 117 samples obtained from the P2f of Mahu Sag, Junggar Basin ranges from 0.08 to 4.17 wt%, with an average of 0.81 wt%. The TS content ranges from 0.0068 to 4.56 wt%, (an average of 1.46 wt%), the TOC/TS ratio ranges from 0.02 to 41.01 (an average of 1.53), and the SRI values range from 1.02 to 35.88 (an average of 3.88).
The vertical variation of all samples in the corresponding single well is shown in Figure 4.
Discussion
TOC/TS Variations Influenced by Volcanic Activity
Pyrite formed during early diagenesis because of the reaction of iron-bearing clastic minerals in fine-grained sediments with H2S in the sedimentary environment (Goldhaber and Kaplan, 1974). During this reaction, the recycling of sulfur is an integral part of the formation of pyrite. Earth’s surface carbon and sulfur cycles, together constitute the main controlling factor for atmospheric O2 concentration over geological time (Garrels and Perry, 1974; Holland, 1978). To maintain a relatively stable O2 concentration in the atmosphere, the total storage capacity of sulfur or carbon must be in equilibrium with the oxidation capacity of both elements. This balance can be simply expressed as the following equation (Garrels and Perry, 1974; Lerman, 1981):
Where, CH2O represents sedimentary material (reducing carbon), CaSO4 means deposited gypsum plus anhydrite (sulfur oxide), FeS2 represents sedimentary pyrite (reducing sulfur), and CaCO3 represents sedimentary carbonate minerals (carbon oxides).
According to reaction (1), regardless of how pyrite is formed in the shale, the increase in the amount of pyrite in the reservoir must be achieved by reducing the organic carbon storage. If the content of pyrite in sediments increases, there is a corresponding decrease in organic carbon burial. The amount of pyrite formed in shale is controlled by the amount and activity of organic matter that can be broken down by bacteria, the concentration of sulfate in the water column, and the amount of active iron (Berner, 1984; Boesen et al., 1988; Middelburg, 1991; Morse and Berner, 1995). Therefore, with the same organic carbon content, the C/S ratio of organic-rich sediments in freshwater sedimentary environments should be higher than that in marine sediments. When the organic carbon content is higher, the difference in the C/S ratio of freshwater sediments and marine sediments would be distinct. Thus, the C/S ratio can be used as an indicator of paleosalinity (Berner and Raiswell, 1984).
Berner and Raiswell (1983) and Leventhal (1995) proposed that if the TOC/TS values are between 2.0 and 3.6, the sediments are formed under normal seawater conditions, and if TOC/TS is greater than 10, the sediments are formed under freshwater conditions (Berner and Raiswell, 1984). Therefore, based on the relationship between TOC and TS in modern sediments, four categories can be recognized between these boundries: TOC/TS = 2, TOC/TS = 3.6, TOC/TS = 10, representing freshwater, freshwater-seawater transition, saline wate, and euxinic depositional environments respectively.
The TOC content of the Ch7 and the P2l is mostly greater than 2%, and the samples are mainly distributed in the freshwater-seawater transition and the saline water regions. However, the TOC of the P2f in the Mahu Sag is generally less than 2 wt%, and there is a clear positive correlation between the organic carbon content and the sulfur content in the shale. This positive correlation could have happened by the redox reaction between sulfate and organic matter in the early diagenesis process when the supply of sulfate is sufficient. Therefore, the depositional period of the P2f in the Mahu Sag was euxinic. However, there was no obvious correlation between organic carbon content and sulfur content when the TOC was greater than 2 wt%. The TOC and TS contents of the Ch7 shale in the Ordos Basin vary notably (see Figure 4), but the TOC of the Ch7 shale exhibits a correlation with TS. The Ch7 shale data points are mainly located in the region of the plot that represents the freshwater-brackish water depositional environment. Finally, due to the influence of volcanic activity, the sulfate concentration of the water in the local interval is close to the normal seawater concentration (Figures 5B, C).
Based on Figure 5, the data from the Ch7 Shales (A) in the Ordos Basin and the P2l Shales (B) in the Jimusar Sag are mostly characterizing the freshwater and the freshwater-seawater transitional depositional environment; the shales of the P2f, a majority of the data in Figure 5C is referring to the euxinic sedimentary environment, which means the water column could have been euxinic at the time. This suggests that the intervention of external sulfates during the sedimentary process made the water column to become saline, which could have been affected by the frequent volcanic eruptions in the orogenic belt during the same period. Due to the sufficient sulfate concentration in the P2f, the organic matter burial and preservation (enrichment) process is susceptible to the transformation via bacterial sulfate reduction (BSR). The concentration of sulfate in the lacustrine waters of the P2l in the Jimusar Sag and the Ch7 is limited, which could have been unfavorable for the occurrence of BSR. However, a large amount of sulfate is injected into the water column as long as the volcanic activity was ongoing, which could have increased the sulfate concentration in the paleo-lake and also trigger the occurrence of BSR.
Volcanic Activity and BSR Intensity
Lallier-Verges et al. (1993) expressed the bacterial sulfate reduction (BSR) strength in terms of the sulfate reduction index (SRI). The sulfate reduction index (SRI) refers to the ratio corresponding to the initial organic carbon content to the residual organic carbon. Initial organic carbon refers to the sum of organic carbon lost by sulfate reduction degradation and residual organic carbon. Residual organic carbon is the total organic carbon (TOC wt%) of present-day. The organic carbon lost to degradation is defined as the total sulfur divided by 1.33, calculated from the stoichiometry of the sulfate reduction equation proposed by Berner and Raiswell (1984):
Closs represents the amount of organic carbon lost by degradation in sulfate reduction:
The SRI ratio reflects the strength of the BSR effect. The higher the BSR intensity lead to the higher the SRI values. SRI is based on the assumption that all hydrogen sulfide produced is either fixed in sulfide precipitation in sediments or synthesized in organic compounds. Therefore, SRI should be regarded as the lowest degradation consumption index of organic carbon relative to the consumption of organic matter by BSR. In general, the SRI and TOC of freshwater sediments and sediments in the Sulfur Tranquil Sea (Black Sea) show a good power exponential relationship. With the increase of the SRI index, TOC gradually decreases, denoting that the greater the intensity of the BSR should correspond to the greater consumption of organic matter. The SRI in the Sulfur Tranquil Sea is generally greater than that in the freshwater sediments, which indicates that the Sulfur Tranquil Sea is more prone to BSR than the freshwater sediments. Westrich and Berner (1984) discussed that the sulfate reduction rate is fundamentally dependent on the quantity and quality of organic carbon. Therefore, organic carbon is the major controlling factor for sulfide formation in normal marine sediments. The sulfated Tranquil Sea (Black Sea) has the strongest sulfate reduction intensity, followed by normal seawater deposition and the freshwater depositional environment. The main reason for this condition is that the sulfate concentration in seawater is higher than that in freshwater, so there is enough S source to supply BSR. The high SRI index of the Sulfide Tranquil environment is because the redox interface has elevated from the bottom of the water-rock interface to the top of the water-rock interface in this depositional environment, resulting in an anoxic environment at the bottom of the water column, rich in H2S (Shen et al., 2011). This leads to an increase in the intensity of sulfate reduction, which increases the consumption of organic matter. The study between TOC and TS in modern sediments explains that the boundary between sulfide and normal fresh-salt water deposits is TOC/TS = 2.0, SRI = 1.375 (Figure 6). When SRI <1.375, the TOC value decreases rapidly with the increase of SRI, indicating that BSR consumes organic matter faster. When SRI >1.375, the BSR intensity increases, and TOC is mostly lower than 2%, inferring that the strong BSR process could continue to consume the organic matter, decreasing the TOC content.
Volcanic Activity Affects the Organic-Rich Matter Formation and Preservation
From the cross plot of TOC and TS, the Ch7 of Well YY1 in the Ordos Basin and the P2l in Jimusar Sag are mainly characterized by freshwater, transitional, and saltwater depositional environment; while the P2f is mainly characterized by the sulfide sedimentary environment. This shows that the sulfate concentration of the water column was changed from freshwater to a transition of a saline water environment, which means that the intervention of external sulfate during the deposition process could have increased the salinity of the water column and caused the sulfate concentration to change. Outcrops and cores of organic-rich shale indicate that there are centimeter-micron-scale pozzolanic interlayers in this shale, while such features are more abundant in the thicker high-quality oil shale.
There is not any clear relationship between SRI and TOC content. The SRI index is less than 1.375, signifying that the sulfate reduction intensity is relatively weak, and the TOC is mostly less than 10 wt%, which may represent two different water environments. When the SRI is less than 1.1, the water column represents the freshwater depositional environment however, the SRI is between 1.1 and 1.375, exhibits a brackish water-saline water deposition, with a weak negative correlation between SRI and TOC (Figure 6). The TOC content of freshwater-deposited shale is generally lower than the one formed in a saline environment. This means organic matter in shale is affected by the depositional environment. This change in salinity could be related to the injection of sulfate by volcanic activity. For example, Algae are abundant in the Ch7 shale, and cyanobacteria to green algae and nanofossils are also abundant in tuff-containing layers (Zhang et al., 2016; Ji et al., 2012). These organic-rich laminations have been interpreted as having a short-term “boom-extinction” signature that typically occurs near tuffaceous lamellae. The tuff development section of the Ch7 corresponds to the volcanic eruption period in geological history. A large amount of nutrients brought by volcanic activity is introduced in a relatively short period of time, which induces the formation of blooming aquatic organisms. The flourishing of aquatic organisms leads to the formation of hypertrophic organic matter sedimentation zones within a certain period, resulting in higher conditions of paleoproductivity. At the same time, large amounts of sulfur-containing gases such as SO2 and H2S were emitted by the volcano, that eventually entered the lacustrine basin in the form of acid rain, to greatly increase the SO42- concentration in freshwater lakes. Since the average sulfate concentration in freshwater environments is less than one percent of what is observed in seawater (Berner, 1984), low sulfate concentrations would limit BSR. When the volcanic activity increases the sulfate in the lake water, it provides a sufficient source of sulfur for the BSR action. BSR uses various organic matter as carbon sources and sulfate ion (SO42-) to generate H2S, enhancing the reducibility of water. The SRI index is less than 1.375, indicating that the BSR is weak and has limited consumption of organic matter.
As the biological flourishing and sulfate enrichment caused by volcanic activity, there is a dynamic balance between the consumption of organic matter and the accumulation of organic matter by BSR, which is manifested as the changing relationship between high TOC and high TS. The overall SRI index is less than 1.375, implying that the degree of preservation of organic matter is stronger than its consumption. This situation is conducive to the formation of organic-rich shale. Meanwhile, the layers with relatively high TOC and low TS would characterize that in the freshwater the sulfate concentration is low, the overall SRI index is less than 1.1, and the BSR effect would have been weak. Achieving a balance between a lower consumption rate of organic matter and a relatively high preservation rate of organic matter is generally beneficial to the enrichment of organic matter. Therefore, for freshwater-brackish lacustrine basins, suitable volcanic activity provides nutrients to not only promote biological growth but also to improve paleoproductivity. Moreover, the sulfate injected by volcanic activity triggers the BSR and produces H2S, which enhances the reducibility of the water column, and is beneficial to the preservation of organic matter.
Conclusion
1) By selecting the typical freshwater and saline lacustrine basins - the Ordos and the Junggar, we systematically analyzed the petrological, mineralogical, and organic-inorganic geochemical characteristics of the organic-rich shales, compared the degree of hypoxia, the action mechanism, and the controlling factors of the formation of organic-rich shale at the bottom of the sedimentary water column in lacustrine basins under the influence of volcanic activity. In addition, we clarified the synergistic mechanism that caused a varying degree of organic matter enrichment in freshwater and saline lacustrine basins.
2) Under the influence of volcanic activity, the SRI index of shales in freshwater-saline basins (the Ch7 and the P2l) were found generally less than 1.375, and the TOC is relatively higher than the value of the shales in sulphated basins (P2f), indicating that more organic carbon was preserved but not consumed; the SRI index of the P2f shales in the Mahu Sag was measured generally greater than 1.375, and the TOC is relatively lower, which meant that the BSR overreacted and consumed a large amount of organic matter, reducing the TOC content.
3) The volcanic activity not only carries nutrients to improve paleoproductivity but also forms a higher sulfate concentration that triggered BSR. BSR produces H2S to form a reducing environment, which is favorable to the preservation of organic matter. However, the consumption of organic matter by excess BSR is greater than the effective preservation of organic matter, which resulted in the loss of organic matter.
Data Availability Statement
The raw data supporting the conclusion of this article will be made available by the authors, without undue reservation.
Author Contributions
XL and JG conceived the study, coordinated the writing, and verified the studying results. XW, ZJ, and RZ shared the results and contributed to the discussion and interpretation. All the authors contributed to the article and approved the submitted version.
Conflict of Interest
Authors XW, WH, and LZ are employed by the PetroChina Xinjiang Oilfield Company. RZ is employed by Research Institute of Petroleum Exploration and Development, PetroChina.
The remaining authors declare that the research was conducted in the absence of any commercial or financial relationships that could be construed as a potential conflict of interest.
Publisher’s Note
All claims expressed in this article are solely those of the authors and do not necessarily represent those of their affiliated organizations, or those of the publisher, the editors and the reviewers. Any product that may be evaluated in this article, or claim that may be made by its manufacturer, is not guaranteed or endorsed by the publisher.
Acknowledgments
The authors acknowledge financial support from the Natural Science Foundation of China (42090025 and 42172151), the scientific research project of Sinopec (P20049-1), the CNPC Xinjiang Company and thank the reviewers for constructive comments that have greatly improved this paper.
References
Aller, R. C. (1994). Bioturbation and Remineralization of Sedimentary Organic Matter: Effects of Redox Oscillation. Chem. Geol. 114, 331–345. doi:10.1016/0009-2541(94)90062-0
Andrew, D. H., Bradley, D. R., and Moldowan, J. M. (2007). Organic Geochemistry of Oil and Source Rock Strata of the Ordos Basin, North-Central China. AAPG Bull. 91 (9), 1273–1293. doi:10.1306/05040704131
Bekker, A., Slack, J. F., Planavsky, N., Krapez, B., Hofmann, A., Konhauser, K. O., et al. (2010). Iron Formation: the Sedimentary Product of a Complex Interplay Among Mantle, Tectonic, Oceanic, and Biospheric Processes. Econ. Geol. 105, 467–508. doi:10.2113/gsecongeo.105.3.467
Berner, R. A. (1982). Burial of Organic Carbon and Pyrite Sulfur in the Modern Ocean; its Geochemical and Environmental Significance. Am. J. Sci. 282 (4), 451–473. doi:10.2475/ajs.282.4.451
Berner, R. A., and Raiswell, R. (1983). Burial of Organic Carbon and Pyrite Sulfur in Sediments over Phanerozoic Time: a New Theory. Geochimica Cosmochimica Acta 47 (5), 855–862. doi:10.1016/0016-7037(83)90151-5
Berner, R. A., and Raiswell, R. (1984). C/S Method for Distinguishing Freshwater from Marine Sedimentary Rocks. Geol 12 (6), 365. doi:10.1130/0091-7613(1984)12<365:cmfdff>2.0.co;2
Berner, R. A. (1984). Sedimentary Pyrite Formation: An Update. Geochimica Cosmochimica Acta 48, 605–615. doi:10.1016/0016-7037(84)90089-9
Boesen, C., and Postma, D. (1988). Pyrite Formation in Anoxic Environments of the Baltic. Am. J. Sci. 288 (6), 575–603. doi:10.2475/ajs.288.6.575
Canfield, D. E., Zhang, S., Wang, H., Wang, X., Zhao, W., Su, J., et al. (2018). A Mesoproterozoic Iron Formation. Proc. Natl. Acad. Sci. U. S. A. 115, E3895. doi:10.1073/pnas.1720529115
Canfield, D. E., Poulton, S. W., Knoll, A. H., Narbonne, G. M., Ross, G., Goldberg, T., et al. (2008). Ferruginous Conditions Dominated Later Neoproterozoic Deep-Water Chemistry. Science 321, 949–952. doi:10.2475/ajs.288.6.575
Chang, H. J., Chu, X. L., Feng, L. J., and Huang, J. (2010). The Iron Speciation in the Silica Rock of the Laobao Formation in Northern Guangxi: an Indication of the Deep-Water Paleoenvironment of the Anoxic and Iron-Bearing Basin. Chin. Sci. Bull. 55 (20), 2010. doi:10.1007/s11434-010-4006-6
Chang, H. L., Zheng, R. C., Guo, C. L., and Wen, H. G. (2016). Characteristics of Rare Earth Elements of Exhalative Rock in Fengcheng Formation, Northwestern Margin of Junggar Basin. Geol. Rev. 62 (03), 550–568. doi:10.3389/feart.2021.774501
Clarkson, M. O., Poulton, S. W., Guilbaud, R., and Wood, R. A. (2014). Assessing the Utility of Fe/Al and Fe-Speciation to Record Water Column Redox Conditions in Carbonate-Rich Sediments. Chem. Geol. 382, 111–122. doi:10.1016/j.chemgeo.2014.05.031
Clarkson, M. O., Wood, R. A., Poulton, S. W., Richoz, S., Newton, R. J., Kasemann, S. A., et al. (2016). Dynamic Anoxic Ferruginous Conditions during the End-Permian Mass Extinction and Recovery. Nat. Commun. 7, 12236. doi:10.1038/ncomms12236
Cui, X., Bianchi, T. S., Savage, C., and Smith, R. W. (2016). Organic Carbon Burial in Fjords: Terrestrial versus Marine Inputs. Earth Planet. Sci. Lett. 451, 41–50. doi:10.1016/j.epsl.2016.07.003
Daviesg, B. (2004). Mississippian Banff-Pekisko Reservoir Report, West-Central Alberta. Calgary, Canada: Graham Davies Geological Consultants Ltd.
Dawson, W. C. (2000). Shale Microfacies: Eagle Ford Group (Cenomanian-Turonian) North-Central Texas Outcrops and Subsurface Equivalents. Gulf Coast Assoc. Geol. Soc. Trans. 50, 607–621. doi:10.1306/8626C005-173B-11D7-8645000102C1865D
Garrels, R. M., and Perry, E. A. (1974). Cycling of Carbon Sulfur and Oxygen through Geologic Time [M]. NASA, Washington: The Global Sulfur Cycle. Wiley.
Goldhaber, M. B., and Kaplan, I. R. (1974). The Sulfur Cycle [M]. Science. doi:10.1126/science.175.4022.587
Guilbaud, A., Poulton, S. W., Butterfield, N. J., Zhu, M. Y., and Shields-Zhou, G. A. (2015). Business Partners. Nat. Geosci. 8 (6), 1–5. doi:10.3917/scpo.guilb.2015.01
He, Y. X., Xian, B. Z., Niu, H. P., and Liu, J. P. (2018). Effects of Paleogeographic Environment on Vocano Eruption Style: Example from the Lower Permian Fengcheng Formation in Eastern Mahu Sag, Junggar Basin. J. Palaeogeogr. 20 (02), 245–262. doi:10.1016/j.jseaes.2019.104222
Hedges, J. I. (1992). Global Biogeochemical Cycles: Progress and Problems. Mar. Chem. 39 (1-3), 67–93. doi:10.1016/0304-4203(92)90096-s
Hedges, J. I., and Keil, R. G. (1995). Sedimentary Organic Matter Preservation: an Assessment and Speculative Synthesis. Mar. Chem. 49 (2-3), 123–126. doi:10.1016/0304-4203(95)00013-h
Huang, Y. J., and Wang, C. S. (2015). Research Progress of Marine and Terrestrial Active Iron Burial and its Enlightenment to the Origin of Source Rocks in Songliao Basin. Nat. Mag. 37 (02), 79–85.
Ji, L., Li, J., Zhang, M., Lu, H., He, C., Jin, P., et al. (2021). Effects of Lacustrine Hydrothermal Activity on the Organic Matter Input of Source Rocks during the Yanchang Period in the Ordos Basin. Mar. Petroleum Geol. 125, 104868. doi:10.1016/j.marpetgeo.2020.104868
Ji, L. M., Xu, J. L., and Song, Z. G. (2012). Lacustrine Cyanobacteria from the Yanchang Formation in Ordos Basin and its Implication on Oil Source. Acta Micropalaeontologica Sin. 29 (3), 270–281.
Jiang, Y., Liu, Y., Yang, Z., Nan, Y., Wang, R., Zhou, P., et al. (2015). Characteristics and Origin of Tuff-type Tight Oil in Jimusaer Sag, Junggar Basin, NW China. Petroleum Explor. Dev. 42 (6), 810–818. doi:10.1016/s1876-3804(15)30077-x
Kim, T. Y., North, R. L., Guildford, S. J., Dillon, P., and Smith, R. E. H. (2015). Phytoplankton Productivity and Size Composition in Lake Simcoe: The Nearshore Shunt and the Importance of Autumnal Production. J. Gt. Lakes. Res. 41 (4), 1075–1086. doi:10.1016/j.jglr.2015.09.011
Konhauser, K. O., Planavsky, N. J., Hardisty, D. S., Robbins, L. J., Warchola, T. J., Haugaard, R., et al. (2017). Iron Formations: A Global Record of Neoarchaean to Palaeoproterozoic Environmental History. Earth-Science Rev. 172, 140–177. doi:10.1016/j.earscirev.2017.06.012
Kuang, L. C., Gao, G., Xiang, B. L., Wang, X. L., and Wang, C. Y. (2014). Lowest Limit of Organic Carbon Content Ineffective Source Rocks from P2l in Jimusar Sag. Pet. Geol. Exp. 36 (2), 224–229. doi:10.11781/sysydz201402224
Kuang, L., Tang, Y., Lei, D., Chang, Q., Ouyang, M., Hou, L., et al. (2012). Formation Conditions and Exploration Potential of Tight Oil in the Permian Saline Lacustrine Dolomitic Rock, Junggar Basin, NW China. Petroleum Explor. Dev. 39 (6), 700–711. doi:10.1016/s1876-3804(12)60095-0
Lallier-Verges, E., Bertrand, P., and Desprairies, A. (1993). Organic Matter Composition and Sulfate Reduction Intensity in Oman Margin Sediments. Mar. Geol. 112 (1–4), 57–69. doi:10.1016/0025-3227(93)90161-n
LaRowe, D. E., Arndt, S., Bradley, J. A., Estes, E. R., Hoarfrost, A., Lang, S. Q., et al. (2020). The Fate of Organic Carbon in Marine Sediments - New Insights from Recent Data and Analysis. Earth-Science Rev. 204, 103146. doi:10.1016/j.earscirev.2020.103146
Lerman, G. A. (1981). [Part 1: Physical Sciences] || Phanerozoic Cycles of Sedimentary Carbon and Sulfur. Proc. Natl. Acad. Sci. U. S. A. 78 (8), 4652–4656. doi:10.1073/pnas.78.8.4652
Leventhal, J. S. (1995). Carbon-sulfur Plots to Show Diagenetic and Epigenetic Sulfidation in Sediments. Geochimica Cosmochimica Acta 59 (6), 1207–1211. doi:10.1016/0016-7037(95)00036-y
Li, C., Love, G. D., Lyons, T. W., Fike, D. A., Sessions, A. L., and Chu, X. (2010). A Stratified Redox Model for the Ediacaran Ocean. Science 328 (5974), 80–83. doi:10.1126/science.1182369
Li, Z.-Q., Zhang, L.-C., Xue, C.-J., Zheng, M.-T., Zhu, M.-T., Robbins, L. J., et al. (2018). Earth's Youngest Banded Iron Formation Implies Ferruginous Conditions in the Early Cambrian Ocean. Sci. Rep. 8, 9970. doi:10.1038/s41598-018-28187-2
Liang, X. P., Jin, Z. J., Alexander, S., Li, P., Morozov, V., and Uspensky, B. (2019). Geological Characteristics and Latest Progress in Exploration and Development of Russian Shale Oil. Oil Gas Geol. 40 (03), 478–490. doi:10.11743/ogg20190304
Liang, X. P., Jin, Z. J., Alexander, S., Yin, J. Y., Liu, Q. Y., and Boris, U. (2021). Impact of Volcanic Ash on the Formation of Organic-Rich Shale: A Case Study on the Mesozoic Bazhenov Formation,West Siberian Basi. Oil Gas Geol. 42 (01), 201–211. doi:10.11743/ogg20210117
Liu, B., Shi, J., Fu, X., Lyu, Y., Sun, X., Gong, L., et al. (2018). Petrological Characteristics and Shale Oil Enrichment of Lacustrine Fine-Grained Sedimentary System: A Case Study of Organic-Rich Shale in First Member of Cretaceous Qingshankou Formation in Gulong Sag, Songliao Basin, NE China. Petroleum Explor. Dev. 45 (5), 884–894. doi:10.1016/s1876-3804(18)30091-0
Liu, B., Song, Y., Zhu, K., Su, P., Ye, X., and Zhao, W. (2020). Mineralogy and Element Geochemistry of Salinized Lacustrine Organic-Rich Shale in the Middle Permian Santanghu Basin: Implications for Paleoenvironment, Provenance, Tectonic Setting and Shale Oil Potential. Mar. Petroleum Geol. 120, 104569. doi:10.1016/j.marpetgeo.2020.104569
Liu, M., Sun, P., Them, T. R., Li, Y., Sun, S., Gao, X., et al. (2020b). Organic Geochemistry of a Lacustrine Shale across the Toarcian Oceanic Anoxic Event (Early Jurassic) from NE China. Glob. Planet. Change 191, 103214. doi:10.1016/j.gloplacha.2020.103214
Liu, Q., Li, P., Jin, Z., Liang, X., Zhu, D., Wu, X., et al. (2021). Preservation of Organic Matter in Shale Linked to Bacterial Sulfate Reduction (BSR) and Volcanic Activity under Marine and Lacustrine Depositional Environments. Mar. Petroleum Geol. 127, 104950. doi:10.1016/j.marpetgeo.2021.104950
MacKenzie, I. A. (2004). Middle-atmospheric Response to a Future Increase in Humidity Arising from Increased Methane Abundance. J. Geophys. research-atmospheres 109 (D2), 1–18. doi:10.1029/2003jd003590
Makeen, Y. M., Hakimi, M. H., Abdullah, W. H., Mustapha, K. A., Amir Hassan, M. H., Shushan, I. E., et al. (2020). Basin Modelling and Bulk Kinetics of Heterogeneous Organic-Rich Nyalau Formation Sediments of the Sarawak Basin, Malaysia. J. Petroleum Sci. Eng. 195, 107595. doi:10.1016/j.petrol.2020.107595
Middelburg, J. J. (1991). Organic Carbon, Sulphur, and Iron in Recent Semi-euxinic Sediments of Kau Bay, Indonesia. Geochimica Cosmochimica Acta 55, 815–828. doi:10.1016/0016-7037(91)90344-5
Morse, J. W., and Berner, R. A. (1995). What Determines Sedimentary C/S Ratios? Geochimica Cosmochimica Acta 59 (6), 1073–1077. doi:10.1016/0016-7037(95)00024-t
Planavsky, N. J., McGoldrick, P., Scott, C. T., Li, C., Reinhard, C. T., Kelly, A. E., et al. (2011). Widespread Iron-Rich Conditions in the Mid-proterozoic Ocean. Nature 477, 448–451. doi:10.1038/nature10327
Poulton, S. W., and Canfield, D. E. (2005). Development of a Sequential Extraction Procedure for Iron: Implications for Iron Partitioning in Continentally Derived Particulates. Chem. Geol. 214, 209–221. doi:10.1016/j.chemgeo.2004.09.003
Poulton, S. W., Fralick, P. W., and Canfield, D. E. (2004). The Transition to a Sulphidic Ocean ∼ 1.84 Billion Years Ago. Nature 431, 173–177. doi:10.1038/nature02912
Qiu, X., Liu, C., Mao, G., Deng, Y., Wang, F., and Wang, J. (2014). Late Triassic Tuff Intervals in the Ordos Basin, Central China: Their Depositional, Petrographic, Geochemical Characteristics and Regional Implications. J. Asian Earth Sci. 80, 148–160. doi:10.1016/j.jseaes.2013.11.004
Raiswell, R., and Berner, R. A. (1986). Pyrite and Organic Matter in Phanerozoic Normal Marine Shales. Geochimica Cosmochimica Acta 50 (9), 1967–1976. doi:10.1016/0016-7037(86)90252-8
Raiswell, R., and Canfield, D. E. (1998). Sources of Iron for Pyrite Formation in Marine Sediments. Am. J. Sci. 298 (3), 219–245. doi:10.2475/ajs.298.3.219
Raiswell, R., Newton, R., Bottrell, S. H., Coburn, P. M., Briggs, D. E. G., Bond, D. P. G., et al. (2008). Turbidite Depositional Influences on the Diagenesis of Beecher's Trilobite Bed and the Hunsruck Slate; Sites of Soft Tissue Pyritization. Am. J. Sci. 308 (2), 105–129. doi:10.2475/02.2008.01
Ridgwell, A., and Hargreaves, J. C. (2007). Regulation of Atmospheric CO2 by Deep‐sea Sediments in an Earth System Model. Glob. Biogeochem. Cycles 21 (2), GB2008. doi:10.1029/2006gb002764
Ridgwell, A., and Zeebe, R. (2005). The Role of the Global Carbonate Cycle in the Regulation and Evolution of the Earth System. Earth Planet. Sci. Lett. 234, 299–315. doi:10.1016/j.epsl.2005.03.006
Shen, J., Algeo, T. J., Planavsky, N. J., Yu, J., Feng, Q., Song, H., et al. (2019c). Mercury Enrichments Provide Evidence of Early Triassic Volcanism Following the End-Permian Mass Extinction. Earth-Science Rev. 195, 191–212. doi:10.1016/j.earscirev.2019.05.010
Shen, J., Chen, J., Algeo, T. J., Yuan, S., Feng, Q., Yu, J., et al. (2019a). Evidence for a Prolonged Permian-Triassic Extinction Interval from Global Marine Mercury Records. Nat. Commun. 10, 1563. doi:10.1038/s41467-019-09620-0
Shen, J., Yu, J., Chen, J., Algeo, T. J., Xu, G., Feng, Q., et al. (2019b). Mercury Evidence of Intense Volcanic Effects on Land during the Permian-Triassic Transition. Geology 47, 1117–1121. doi:10.1130/g46679.1
Shen, Y., Canfield, D. E., and Knoll, A. H. (2002). Middle Proterozoic Ocean Chemistry: Evidence from the McArthur Basin, Northern Australia. Am. J. Sci. 302, 81–109. doi:10.2475/ajs.302.2.81
Shen, Y., Farquhar, J., Zhang, H., Masterson, A., Zhang, T., and Wing, B. A. (2011). Multiple S-Isotopic Evidence for Episodic Shoaling of Anoxic Water during Late Permian Mass Extinction. Nat. Commun. 2, 210. doi:10.1038/ncomms1217
Shen, Y., Knoll, A. H., and Walter, M. R. (2003). Evidence for Low Sulphate and Anoxia in a Mid-proterozoic Marine Basin. Nature 423, 632–635. doi:10.1038/nature01651
Thomas, J. A., and Li, C. (2020). Redox Classification and Calibration of Redox Thresholds in Sedimentary System. Geochimica Cosmochimica Acta 287, 8–26. doi:10.1016/j.gca.2020.01.055
Wang, L. B., Hou, G. F., Bian, B. L., Li, Y. Z., Dou, Y., You, X. C., et al. (2020a). The Role of Modern Alkaline Lakes in Explaining the Sedimentary Environment of the Fengcheng Formation, Mahu Depression. Acta Sedimentol. Sin. 38 (05), 913–922. doi:10.14027/j.issn.1000-0550.2020.021
Wang, Y., Cao, J., Tao, K., Li, E., Ma, C., and Shi, C. (2020b). Reevaluating the Source and Accumulation of Tight Oil in the Middle Permian Lucaogou Formation of the Junggar Basin, China. Mar. Petroleum Geol. 117, 104384. doi:10.1016/j.marpetgeo.2020.104384
Westrich, J. T., and Berner, R. A. (1984). The Role of Sedimentary Organic Matter in Bacterial Sulfate Reduction: The G Model Tested1. Limnol. Oceanogr. 29, 236–249. doi:10.4319/lo.1984.29.2.0236
Yang, H., and Zhang, W. Z. (2005). Leading Effect of the Seventh Member High-Quality Source Rock of Yanchang Formation in Ordos Basin during the Enrichment of Low-Penetrating Oil-Gas Accumulation: Geology and Geochemistr. Geochimica 34 (2), 147–154. doi:10.19700/j.0379-1726.2005.02.007
Yang, S., Hu, W., Wang, X., Jiang, B., Yao, S., Sun, F., et al. (2019). Duration, Evolution, and Implications of Volcanic Activity across the Ordovician-Silurian Transition in the Lower Yangtze Region, South China. Earth Planet. Sci. Lett. 518, 13–25. doi:10.1016/j.epsl.2019.04.020
Yu, K. H., Cao, Y. C., Qiu, L. W., Sun, P. P., Yang, Y. Q., Qu, C. S., et al. (2016). Brine Evolution of Ancient Lake and Mechanism of Carbonate Minerals during the Sedimentation of Early Permian Fengcheng Formation in Mahu Depression, Junggar Basin, China. Nat. Gas. Geosci. 27 (7), 1248–1263. doi:10.11764/j.issn.1672-1926.2017.06.1248
Yuan, W., Liu, G. D., Bulseco, A., and Zhou, X. X. (2021). Iron Speciation in Organic-Rich Shales from the Upper Triassic Yanchang Formation, Ordos Basin, Northern China: Implications for Depositional Environment. J. Asian Earth Sci. 220, 104917. doi:10.1016/j.jseaes.2021.104917
Yuan, Y. Y., Cai, C. F., Wang, T. K., Xiang, L., Jia, L. Q., and Chen, Y. (2014). Redox Properties of the Lower Yangtze Deepwater Basin during the Ediacaran-Cambrian Transition Period: Constraints from the Iron Speciation and Organic Carbon Isotopes of the Diben Section in Western Zhejiang. Chin. Sci. Bull. 59 (23), 2278–2290. doi:10.1007/s11434-014-0483-3
Zhang, S., Cao, Y., Liu, K., Jahren, J., Xi, K., Zhu, R., et al. (2019). Characterization of Lacustrine Mixed Fine-Grained Sedimentary Rocks Using Coupled Chemostratigraphic-Petrographic Analysis: a Case Study from a Tight Oil Reservoir in the Jimusar Sag, Junggar Basin. Mar. Petroleum Geol. 99, 453–472. doi:10.1016/j.marpetgeo.2018.10.039
Zhang, W., Yang, H., Xia, X., Xie, L., and Xie, G. (2016). Triassic Chrysophyte Cyst Fossils Discovered in the Ordos Basin, China. Geology 44, 1031–1034. doi:10.1130/g38527.1
Zhang, W. Z., Yang, H., Peng, P. A., Yang, Y. H., Zhang, H., and Shi, X. H. (2009). The Influence of Late Triassic Volcanism on the Development of Chang 7 High Grade Hydrocarbon Source Rock in Ordos Basin. Geochimica 38 (6), 573–582. doi:10.1016/S1874-8651(10)60080-4
Zhang, W. Z., Yang, H., Xie, L. Q., and Xie, G. W. (2011). Discovery of Micro and Nanofossils in High Grade Hydrocarbon Source Rocks of the Triassic Yanchang Formation Chang7 Member in Ordos Basin and its Scientific Significance. Acta Palaeontol. Sin. 50 (1), 109–117. doi:10.1007/s10255-011-0044-3
Zhang, W. Z., Yang, H., Yang, W. W., Wu, K., and Liu, F. (2015). Assessment of Geological Characteristics of Lacustrine Shale Oil Reservoir in Chang 7 Member of Yanchang Formation, Ordos Basin [J]. Geochimica 44 (5), 505–515. doi:10.19700/j.0379-1726.2015.05.010
Zhang, W. Z., Yang, H., Yang, Y. H., Kong, Q. F., and Wu, K. (2008). Petrology and Element Geochemistry and Development Environment of Yanchang Formation Chang-7 High Quality Source Rocks in Ordos Basin. Geochimica 37 (1), 59–64. doi:10.19700/j.0379-1726.2008.01.008
Zhang, Y. Y., Li, W., and Tang, W. B. (2018a). Tectonic Setting and Environment of Alkaline Lacustrine Source Rocks in the Lower Permian Fengcheng Formation of Mahu Sag. Xinjiang Pet. Geol. 39 (1), 48–54. doi:10.7657/XJPG20180106
Zhang, Z. J., Yuan, X. J., Wang, M. S., Zhou, C. M., Tang, Y., ChenLin, X. Y. M. J., et al. (2018b). Alkaline-lacustrine Deposition and Paleoenvironmental Evolution in Permian Fengcheng Formation at the Mahu Sag, Junggar Basin, NW China. Petroleum Explor. Dev. 45 (06), 972–984. doi:10.1016/s1876-3804(18)30107-1
Zhao, M. W., Behr, H. J., Ahrendt, H., Wemmer, K., Ren, Z. L., and Zhao, Z. Y. (1996). Thermal and Tectonic History of the Ordos Basin, China: Evidence from Apatite Fission Track Analysis, Vitrinite Reflectance, and K-Ar Dating. AAPG Bull. 80, 1110–1134. doi:10.1306/64ed8caa-1724-11d7-8645000102c1865d
Zhi, D. M., Tang, Y., He, W. J., Guo, X. G., Zheng, M. L., and Huang, L. L. (2021). Orderly Coexistence and Accumulation Models of Conventional and Unconventional Hydrocarbons in Lower Permian Fengcheng Formation, Mahu Sag, Junggar Basin. Petroleum Explor. Dev. 48 (1), 38–51. doi:10.1016/s1876-3804(21)60004-6
Keywords: volcanic activity, the freshwater and saline lakes, Fengcheng Formation, Lucaogou Formation, Chang 7 Member, organic-rich shale
Citation: Wang X, Gao J, Zhong L, He W, Jin Z, Zhu R, Liang X, Liu K and Zhang W (2022) The Volcanic Impacts on the Formation of Organic-Rich Shales From the Freshwater to Saline Lakes: Cases Study in the Ordos and the Junggar Basins. Front. Earth Sci. 10:918391. doi: 10.3389/feart.2022.918391
Received: 12 April 2022; Accepted: 03 May 2022;
Published: 31 May 2022.
Edited by:
Yu Song, China University of Geosciences Wuhan, ChinaReviewed by:
Hui Pu, University of North Dakota, United StatesMin Wang, China University of Petroleum, China
Lianbo Zeng, China University of Petroleum, China
Copyright © 2022 Wang, Gao, Zhong, He, Jin, Zhu, Liang, Liu and Zhang. This is an open-access article distributed under the terms of the Creative Commons Attribution License (CC BY). The use, distribution or reproduction in other forums is permitted, provided the original author(s) and the copyright owner(s) are credited and that the original publication in this journal is cited, in accordance with accepted academic practice. No use, distribution or reproduction is permitted which does not comply with these terms.
*Correspondence: Zhijun Jin, jinzj1957@pku.edu.cn; Xinping Liang, xinping.liang@pku.edu.cn