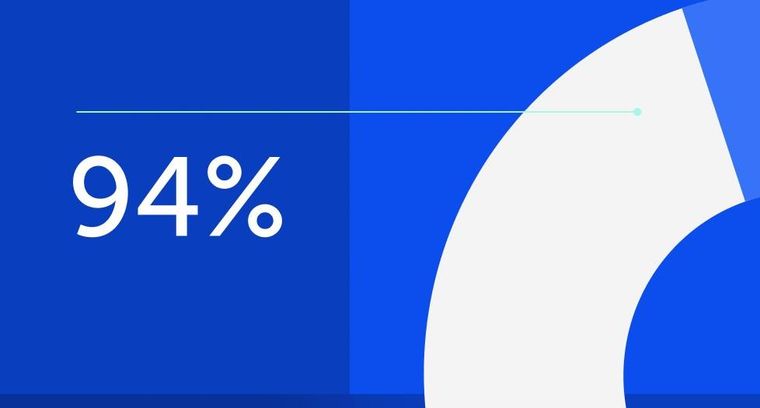
94% of researchers rate our articles as excellent or good
Learn more about the work of our research integrity team to safeguard the quality of each article we publish.
Find out more
ORIGINAL RESEARCH article
Front. Earth Sci., 04 August 2022
Sec. Geochemistry
Volume 10 - 2022 | https://doi.org/10.3389/feart.2022.914432
This article is part of the Research TopicHow Large Igneous Provinces (LIPs) During the Triassic Shaped Modern-Day EcosystemsView all 15 articles
Globally, Late Permian to Early Triassic carbonate rocks record several pronounced positive and negative C-isotope excursions, indicating a dramatic reorganization of the global carbon cycle. These C-isotopic anomalies provide important constraints on environmental changes that occurred during the end-Permian extinction and the subsequent delayed biotic recovery. In this study, we present high-resolution carbonate C-isotopic data (δ13Ccarb) spanning the Permian-Triassic transition at Dajiang, South China. Our results reveal a general decrease in δ13Ccarb of ∼3.3‰ during the microbialite formation which was followed by an increase. C-isotopic chemostratigraphic correlation between the Dajiang section and the Global Boundary Stratotype Section and Point (GSSP) at Meishan suggest a hiatus of several thousands of years between the pre-extinction skeletal limestones and the microbialite deposition in the aftermath of the end-Permian extinction in South China. We suggest that multiple sources of 13C-depleted dissolved carbon are required to explain the negative δ13Ccarb excursions as well as the δ13Ccarb differences among microbialites deposited in various paleogeographic locations. Our study shows that carbon cycles during the formation of microbialite may have been more complex than previously thought, and cessation of microbialite formation may have been controlled by both global and local environmental changes.
The end-Permian mass extinction (∼252 Ma), was the most severe biotic crisis of the Phanerozoic, which eliminated over 90% of marine species and fundamentally altered marine ecosystems (Knoll et al., 2007; Song et al., 2012, 2018). It is generally accepted that environmental deterioration, triggered by the eruption of the Siberian Traps Large Igneous Province, may have led to the end-Permian mass extinction (Kamo et al., 2003; Svensen et al., 2009; Grasby et al., 2011; Shen et al., 2011; Burgess et al., 2017; Zhang et al., 2017; Fielding et al., 2019; Li et al., 2021, 2022). C-isotopic changes in space and time have been instrumental in understanding the environmental changes that occurred during Late Permian–Early Triassic transition (e.g., Grasby and Beauchamp, 2008; Meyer et al., 2011; Grasby et al., 2013; Song et al., 2013). Existing data show large perturbations of global carbon cycles during the end-Permian mass extinction and the delayed Early Triassic biotic recovery (e.g., Payne et al., 2004; Xie et al., 2007). For example, a pronounced negative C-isotopic excursion coincided with the end-Permian extinction worldwide (Holser et al., 1989; Cao et al., 2002, 2009). The sources of the 13C-depleted carbon causing this global negative C-isotopic excursion are argued to have come from volcanic emissions of CO2 (Payne and Kump, 2007; Cao et al., 2009), the oxidation of methane (Ryskin, 2003), the increase of 12C-enriched organic carbon weathering due to eustatic sea-level fall (Holser et al., 1989), the incursion of 12C-enriched dissolved carbon in anoxic/euxinic deep water into the shallow marine environment (Riccardi et al., 2007; Zhang et al., 2021), amongst others. However, δ13C data from the Permian-Triassic transition indicate that δ13Ccarb compositions continued to decrease following the end-Permian mass extinction level, when microbialites were deposited (Krull et al., 2004; Mu et al., 2009; Wang et al., 2009; Liao et al., 2010; Liu et al., 2010; Luo et al., 2010, 2011; Yang et al., 2011; Wu et al., 2017).
Permian-Triassic microbialites are carbonates rich in microbial structures and cements of various forms including stromatolites, thrombolites and dendrolites, that were deposited in the aftermath of the end-Permian mass extinction (Lehrmann 1999; Wang et al., 2005; Baud et al., 2007; Kershaw et al., 2007, 2012; Yang et al., 2011; Wang et al., 2019). Most of the Permian-Triassic microbialites were developed in the low-latitude Tethys Ocean, especially in South China where many excellent sections are exposed. Extensively multidisciplinary studies have greatly improved our knowledge about the age, microbial structure, genesis, and environmental implications of these microbialites (Wang et al., 2005; Luo et al., 2010; Wang et al., 2016; Deng et al., 2017; Pei et al., 2019). For example, all of the C-isotopic profiles from South China have shown that the δ13Ccarb compositions continue to decrease from pre-extinction bioclastic limestones enriched in benthic fossils to the overlying microbialites (e.g., Yang et al., 2011).
In this study, we report high-resolution δ13Ccarb data of microbialites from the Dajiang section in South China. The microbialite interval is about 14.5 m in the Dajiang section and might be the thickest in South China, which could reveal more details of C-isotopic variations during this period. We aim to better understand environmental significance of C-isotopic variations in microbialites and their possible link to the delayed biotic recovery from the end-Permian mass extinction.
During the Permian-Triassic transition, the South China craton, including the Yangtze and Cathaysia blocks, was situated north of the paleo-equator in the eastern part of the Paleotethys Ocean (Figure 1A). The Nanpanjiang Basin is a deep-marine embayment in the Yangtze Platform, which opened south-eastward to the Panthalassa Ocean (Lehrmann, 1999). The Great Bank of Guizhou, which evolved as an isolated carbonate platform within the Nanpanjiang Basin, preserved continuous shallow marine deposition from the late Permian to the middle Triassic (Lehrmann et al., 2003). There are many outstanding sections that preserved microbialites in the Nanpanjiang Basin (Luo et al., 2011, 2014). In part because of its thickness and excellent exposure, the Dajiang microbialites were the focus of this study.
FIGURE 1. Permian-Triassic paleogeographic sketch map of the world (A) and the South China Craton (B). Inset red rectangle in (A) shows the area of the South China Craton. The red star in (B) shows the location of the Dajiang section. Paleogeography of the world is revised from Shen et al. (2015), and the map of the South China Craton is modified from Xie et al. (2010) and Yin et al. (2014). DJ: Dajiang section; NDB: Northern Deepwater Basin; NPJB: Nanpanjiang Basin.
The Dajiang section (106°39′49″E, 25°33′47″N) is located in Bianyang town, Luodian County, Guizhou Province. Paleogeographically, the study section was situated on the Great Bank of Guizhou and was deposited at a water depth possibly less than 100 m (Song et al., 2013) (Figure 1B). The Dajiang section comprises, in ascending order, the upper Permian Wujiaping Formation and the lower Triassic Daye Formation (Figure 2). The uppermost Wujiaping Formation is characterized by skeletal limestone, and contains abundant shallow-marine fossils such as fusulinids, foraminifera, brachiopods, and calcareous algae (Lehrmann et al., 2003). At the base of the Daye Formation, a 14.5 m thick microbialite was deposited, which is mainly composed of laminar or spotted thrombolites (Figure 2). The microbialite deposit is overlain by grainstone and packstone (Jiang et al., 2014).
FIGURE 2. Profiles of δ13Ccarb and δ18Ocarb from the Dajiang section. Conodont zones are from Jiang et al. (2014). Abbreviations: H. parvus = Hindeodus parvus zone; I. L. = Isarcicella lobate zone; I. I. = Isarcicella isarcica zone; H. sosioensis = Hindeodus sosioensis zone; Fm. = Formation; Con. = Conodont zone; Lith. = Lithology.
It is widely accepted that the end-Permian extinction level is correlated with bed 25 in the GSSP at Meishan, South China and the Permian-Triassic boundary (PTB) is correlated with bed 27c at Meishan, indicated by the first appearance datum (FAD) of the conodont species Hindeodus parvus (Yin et al., 2001). However, the position of the FAD of H. parvus in the Dajiang section was placed at different levels, and therefore the exact placement of the PTB remains debated (Jiang et al., 2014; Lehrmann et al., 2015; Zhang et al., 2020). The PTB has been placed either at the uppermost of the Wujiaping Formation (Jiang et al., 2014) or within the microbialite (Lehrmann et al., 2015; Zhang et al., 2020). In this study, we follow the scheme of Jiang et al. (2014) (Figure 2). Regardless of the precise PTB level, there is little doubt that the microbialites at Dajiang were deposited in the aftermath of the end-Permian mass extinction.
A total of 100 bulk samples were collected continuously over ∼23.4-m of strata across the PTB in the Dajiang section. All sample depths are measured relative to the PTB. Among them, 16 samples are from the skeletal limestone interval (−3.1–0 m), 66 samples are from the microbialite interval (0–14.5 m), and 18 samples are from the grainstone and packstone (14.5–20.3 m) (Figure 2). Prior to isotopic analysis, any weathered surfaces and visible calcite veins were trimmed off. Pieces of the crushed samples were then ground to fine powders (<200 mesh) using an automated agate mortar and pestle device. Sample preparation and analyses were performed in the Biogeochemical Laboratory at University of Science and Technology of China.
For δ13Ccarb and δ18Ocarb analysis, approximately 150 μg of sample powder was reacted with 102–105% anhydrous phosphoric acid at 70°C in a Kiel IV carbonate device to generate CO2. The evolved CO2 gas was purified in a cryogenic cleaning device and then measured on a ThermoFinnigan MAT 253 Mass Spectrometer. The carbon and oxygen isotope compositions are expressed in the conventional delta notation as per mil (‰) deviations relative to the V-PDB standard. Analytical reproducibility was monitored by replicate analysis of a Chinese national standard GBW04416 (δ13Ccarb = +1.61‰, δ18Ocarb = −11.59‰), and was better than ±0.05‰ for δ13Ccarb and ±0.06‰ for δ18Ocarb.
The δ13Ccarb data show large variations during the Permian–Triassic transition in the Dajiang section, ranging from −0.44‰ to +2.87‰ (Supplementary Table S1; Figure 2). Post-depositional diagenetic processes can alter the primary C-isotopic compositions of sedimentary carbonates (Kaufman and Knoll, 1995). Therefore, diagenetic effects must be evaluated prior to the interpretation of the isotope data. It has been shown that diagenetic fluids enriched in organic-derived carbon can simultaneously alter the carbonate δ13C and δ18O towards more negative values, and as such, the positive correlation between δ13Ccarb and δ18Ocarb may be indicative of significant diagenetic alteration (Kaufman and Knoll, 1995). Extreme negative δ18Ocarb values (<−10‰) have also been suggested to be an additional indicator of diagenetic alteration (Kaufman and Knoll, 1995). However, the δ18Ocarb compositions from the Dajiang section range from −2.7‰ to −7.5‰, implying the primary nature for δ13Ccarb.
The plot of δ13Ccarb and δ18Ocarb show moderate correlation (R2 = 0.4653) for the pre-extinction skeletal limestone (Figure 3A). This could suggest diagenetic alteration of the primary isotopic compositions. However, the narrow δ13Ccarb range from +2.35‰ to +2.87‰ suggest that the δ13Ccarb compositions were not significantly changed and they are of primary isotopic significance, although the δ18Ocarb compositions could be altered. There is little correlation between δ13Ccarb and δ18Ocarb for the microbialites and overlying grainstones and packstones, suggesting that the primary δ13Ccarb compositions were well preserved (Figures 3B,C). Similarly, the plot between δ13Ccarb and δ18Ocarb for all samples from Dajiang shows little correlation, reinforcing the primary nature for δ13Ccarb (Figure 3D).
FIGURE 3. Cross-plot of δ13Ccarb and δ18Ocarb for pre-extinction skeletal limestone (A), microbialites (B), overlying grainstones and packstones (C), and all samples from the Dajiang section (D).
It is worthy to note that a preliminary δ13Ccarb study on the Dajiang section was carried out by Krull et al. (2004) (Figure 4). In general, the two datasets are consistent. However, our high-resolution sampling and precise measurements reveal more details about C-isotopic changes. As shown in Figure 2, the δ13Ccarb values of the pre-extinction skeletal limestone range from +2.35‰ to +2.87‰, with an average of +2.55‰. There is not much change in δ13Ccarb in the pre-extinction interval, although there is a slight δ13Ccarb increase from +2.41‰ to +2.78‰ (Figure 4).
FIGURE 4. Comparison of δ13Ccarb records of the Dajiang section from this study and Krull et al. (2004).
After the end-Permian extinction event, the δ13Ccarb data display a broad decrease through the microbialite interval, with δ13Ccarb decreasing from +2.78‰ to −0.44‰ (Figure 2). Following this the δ13Ccarb data recover from the nadir of −0.44‰ to +0.29‰ in the uppermost part of the microbialite interval, and continue to rise to +2.29‰ in the overlying grainstones and packstones (Figure 2).
Our high-resolution C-isotope data reveal that the broad δ13Ccarb decrease in the microbialite interval consists of three consecutive episodes (Figure 2). During episode I, δ13Ccarb slightly decreases from +2.78‰ to +1.98‰ followed by a recovery to +2.39‰ at 4.92 m (Figure 2). The δ13Ccarb continues to decrease from +2.39‰ to +0.84‰ at the height of 6.68 m during episode II, followed by an increase to +1.61‰ at 8.76 m (Figure 2). Episode III shows a δ13Ccarb decrease from +1.61‰ to the minimal value of −0.44‰ at 11.35 m in the microbialite interval (Figure 2). The δ13Ccarb increases from −0.44‰ to +0.30‰ at the uppermost of the microbialite interval and continues to increase to +2.29‰ in the overlying grainstones and packstones though a sharp negative shift from +1.40‰ at 15.4 m to +0.26‰ at 15.65 m is shown (Figure 2).
Microbialites were deposited immediately following the end-Permian extinction in a shallow marine carbonate environment. Like Dajiang, observations from many sections in South China indicate an erosional surface between the underlying skeletal packstone and overlying microbialite (Jiang et al., 2014) (Figure 2). This has been interpreted as submarine chemical dissolution due to ocean acidification (Payne et al., 2007; Lehrmann et al., 2015) or subaerial erosion due to the major global regression near the extinction event (e.g., Wignall et al., 2009). However, both models could work depending on the paleotopography and accommodation space of the studied section (Yin et al., 2014). It was estimated that a hiatus between the skeletal limestone and the basal microbialite may be 50–100 ky (Yin et al., 2014). The δ13C stratigraphic correlation between Dajiang and GSSP Meishan section suggest that the deposition from the skeletal packstone to the overlying microbialite at Dajiang may be not continuous (Figures 2, 5).
FIGURE 5. Comparison of δ13Ccarb records from the Dajiang (this study) and Meishan section (Cao et al., 2002).
At Dajiang, there is minor δ13Ccarb increase from +2.37‰ to +2.78‰ from the top of the packstone to the basal microbialite (Figure 5). In contrast, a gradual δ13Ccarb decrease from the bed 23 to bed 24e, followed by another δ13Ccarb decrease of about 4‰, occurs at the extinction level at bed 25 at Meishan (Cao et al., 2002) (Figure 5). After the extinction, the δ13Ccarb at Meishan increases about 3‰, gradually from bed 25 to beds 28–29 (Cao et al., 2002) (Figure 5). However, the δ13Ccarb continues to decrease from the packstone to microbialite at Dajiang (Figures 2, 5). The Meishan section may be a condensed section which may have not recorded all of the C-isotopic changes during Permian-Triassic transition. However, the δ13Ccarb from Dajiang missed several significant δ13Ccarb changes in Meishan and elsewhere. Therefore, our δ13Ccarb data from Dajiang support that the microbialites were deposited in the aftermath of the end-Permian extinction and that the deposition of several thousands of years was probably missing prior to the microbialite formation.
The correlations between the Dajiang section and Zuodeng and Taiping sections in the same Nanpanjiang Basin show similarities as well as differences in δ13C variations (Figure 6). The δ13Ccarb from all three sections show decreasing values in the microbialite interval and increasing values after the deposition of microbialites (Figure 6). The minimal δ13Ccarb values at Dajiang, Zuodeng, and Taiping are −0.44‰, +0.3‰, and −0.7‰, respectively (Figure 6). Unlike Dajiang, the δ13Ccarb profiles of the microbialites from Zuodeng and Taiping remain relatively stable after the δ13Ccarb decrease from the pre-extinction skeletal limestones, reflecting complexities of carbon cycling in the basin (Figure 6). The correlations between Dajiang and Cili and Chongyang sections add more complexities of carbon cycling as the late two sections were also deposited in a carbonate platform close to the Northern Deepwater Basin (Luo et al., 2014). Like the Dajiang section, the δ13Ccarb profile at Cili shows a general decrease to −0.4‰ and consists of three minor negative δ13Ccarb excursions (Figure 6). However, the Chongyang section, which was deposited in a similar environment as the Cili section (Luo et al., 2014), shows relatively stable δ13Ccarb values around −0.3‰, after the decrease of about 2‰ from the pre-extinction skeletal limestones (Figure 6).
FIGURE 6. Comparison of δ13Ccarb records during the Permian-Triassic transition in South China. The data are from the Dajiang (this study), Zuodeng (Luo et al., 2011), Taiping (Luo et al., 2011), Cili (Luo et al., 2011), and Chongyang (Luo et al., 2011) section. The red lines indicate the onset and termination of the microbialite interval.
It appears that there is a general δ13Ccarb decrease from the pre-extinction skeletal limestones to the microbialites in all studied sections, although the absolute δ13Ccarb decrease varies between each section (Figure 6). After this general δ13Ccarb decrease, the δ13Ccarb profile of the microbialite either remains relatively stable or continues to decrease (Figure 6). The δ13Ccarb value then increases starting from either within the microbialite or after the microbialite deposition (Figure 6). However, this δ13Ccarb increase was not shown in the Chongyang section (Figure 6).
Regardless, the significant δ13Ccarb decrease from the pre-extinction skeletal limestones to the microbialites in all studied sections requires inputs of 13C-depleted dissolved carbon. Continental weathering of 13C-depleted carbon was unlikely a major source as a large amount of clastic inputs would have inhibited the growth of microbialite. Likewise, dissociation of methane hydrates was unlikely a major source considering the continuous decreasing δ13Ccarb compositions as at Dajiang, as well as the very shallow water environment for precipitation of microbialites. There are possible 13C-depleted sources that could help to explain the decreasing δ13Ccarb values for the microbialites. Emission of CO2 and possibly methane as well by the Siberian Traps magmatism could provide 13C-depleted carbon, producing the negative δ13Ccarb excursion (Burgess and Bowring, 2015). Upwelling of anoxic deep water into the photic zone could also contribute 13C-depleted carbon during the microbialite formation (e.g., Kershaw et al., 2007). As well, low productivity could possibly lead to 13C-depleted isotopic values in surface waters. However, evidence for either of the mechanisms may be circumstantial and neither of them can independently explain the negative δ13Ccarb excursion from the pre-extinction skeletal limestone to the microbialite level, as well as the differences in δ13Ccarb minimums amongst the microbialites deposited in various paleogeographic locations (Figure 6).
The general increasing δ13Ccarb profiles within the microbialite interval at Dajiang or after the deposition of the microbialtes elsewhere (Figure 6) suggest changes of carbon cycling in the post-microbialite oceans. Increasing primary productivity and/or anoxia could facilitate enhanced burial of organic carbon, resulting in the increasing δ13Ccarb compositions. We speculate that the cessation of microbialites in South China may be linked to the changes of carbon cycling which may have controlled by global and local environmental changes.
The δ13Ccarb data from the Dajiang section reveal a general decrease of ∼3.2‰ from pre-extinction skeletal limestone to the microbialite deposition. Unlike the δ13Ccarb data from many sections in South China, the decreasing δ13Ccarb at Dajiang is followed by an increasing δ13Ccarb profile. We suggest that multiple sources of 13C-depleted dissolved carbon may be possibly required to explain the decreasing δ13Ccarb during the microbialite formation. There is little doubt that carbon cycling during the microbialite precipitation are more complex than previously thought. Though great details about morphology and mineralogical compositions have been studied, local environments for microbialite formation have not been well reconstructed. Future studies should focus on environmental reconstruction for microbialite formation and their possible link to the delayed recovery from the end-Permian mass extinction.
The original contributions presented in the study are included in the article/Supplementary Material, further inquiries can be directed to the corresponding authors.
YS conceived the study. XZ and YS collected samples. XH, XZ, and ML wrote the manuscript with inputs from DL, YX, LS, and YS.
This study was supported by the National Natural Science Foundation of China (41721002 and 41890842) and the 111 project (B14026).
We dedicated this paper to the late Yongbiao Wang for his pioneering work on the microbialite in South China and his help in collecting samples from Dajiang. We thank Steve Grasby, Haishui Jiang and the reviewers for their constructive comments.
The authors declare that the research was conducted in the absence of any commercial or financial relationships that could be construed as a potential conflict of interest.
All claims expressed in this article are solely those of the authors and do not necessarily represent those of their affiliated organizations, or those of the publisher, the editors and the reviewers. Any product that may be evaluated in this article, or claim that may be made by its manufacturer, is not guaranteed or endorsed by the publisher.
The Supplementary Material for this article can be found online at: https://www.frontiersin.org/articles/10.3389/feart.2022.914432/full#supplementary-material.
Baud, A., Richoz, S., and Pruss, S. (2007). The lower Triassic anachronistic carbonate facies in space and time. Glob. Planet. Change 55, 81–89. doi:10.1016/j.gloplacha.2006.06.008
Burgess, S. D., and Bowring, S. A. (2015). High-precision geochronology confirms voluminous magmatism before, during, and after Earth’s most severe extinction. Sci. Adv. 1, e1500470. doi:10.1126/sciadv.1500470
Burgess, S. D., Muirhead, J. D., and Bowring, S. A. (2017). Initial pulse of Siberian Traps sills as the trigger of the end-Permian mass extinction. Nat. Commun. 8, 164. doi:10.1038/s41467-017-00083-9
Cao, C., Love, G. D., Hays, L. E., Wang, W., Shen, S., Summons, R. E., et al. (2009). Biogeochemical evidence for euxinic oceans and ecological disturbance presaging the end-Permian mass extinction event. Earth Planet. Sci. Lett. 281, 188–201. doi:10.1016/j.epsl.2009.02.012
Cao, C., Wang, W., and Jin, Y. (2002). Carbon isotope excursions across the permian-triassic boundary in the meishan section, zhejiang Province, China. Chin. Sci. Bull. 47, 1125–1129. doi:10.1360/02tb9252
Deng, B., Wang, Y., Woods, A., Li, S., Li, G., Chen, W., et al. (2017). Evidence for rapid precipitation of calcium carbonate in South China at the beginning of Early Triassic. Palaeogeogr. Palaeoclimatol. Palaeoecol. 474, 187–197. doi:10.1016/j.palaeo.2016.06.007
Fielding, C. R., Frank, T. D., McLoughlin, S., Vajda, V., Mays, C., Tevyaw, A. P., et al. (2019). Age and pattern of the southern high-latitude continental end-Permian extinction constrained by multiproxy analysis. Nat. Commun. 10, 385. doi:10.1038/s41467-018-07934-z
Grasby, S. E., Beauchamp, B., Embry, A., and Sanei, H. (2013). Recurrent early triassic ocean anoxia. Geology 41, 175–178. doi:10.1130/G33599.1
Grasby, S. E., and Beauchamp, B. (2008). Intrabasin variability of the carbon-isotope record across the permian-triassic transition, sverdrup basin, arctic Canada. Chem. Geol. 253, 141–150. doi:10.1016/j.chemgeo.2008.05.005
Grasby, S. E., Sanei, H., and Beauchamp, B. (2011). Catastrophic dispersion of coal fly ash into oceans during the latest Permian extinction. Nat. Geosci. 4, 104–107. doi:10.1038/ngeo1069
Holser, W. T., Schonlaub, H. P., Attrep, M., Boeckelmann, K., Klein, P., Magaritz, M., et al. (1989). A unique geochemical record at the Permian-Triassic boundary. Nature 337, 39–44. doi:10.1038/337039a0
Jiang, H., Lai, X., Sun, Y., Wignall, P. B., Liu, J., Yan, C., et al. (2014). Permian-Triassic conodonts from Dajiang (Guizhou, South China) and their implication for the age of microbialite deposition in the aftermath of the end-Permian mass extinction. J. Earth Sci. 25, 413–430. doi:10.1007/s12583-014-0444-4
Kamo, S. L., Czamanske, G. K., Amelin, Y., Fedorenko, V. A., Davis, D., Trofimov, V., et al. (2003). Rapid eruption of Siberian flood-volcanic rocks and evidence for coincidence with the Permian-Triassic boundary and mass extinction at 251 Ma. Earth Planet. Sci. Lett. 214, 75–91. doi:10.1016/S0012-821X(03)00347-9
Kaufman, A. J., and Knoll, A. H. (1995). Neoproterozoic variations in the C-isotopic composition of seawater: Stratigraphic and biogeochemical implications. Precambrian Res. 73, 27–49. doi:10.1016/0301-9268(94)00070-8
Kershaw, S., Crasquin-soleau, S., Li, Y., Collin, P-Y., Forel, M-B., Mu, X., et al. (2012). Microbialites and global environmental change across the permian-triassic boundary: A synthesis. Geobiology 10, 25–47. doi:10.1111/j.1472-4669.2011.00302.x
Kershaw, S., Li, Y., Crasquin-soleau, S., Feng, Q., Mu, X., Collin, P-Y., et al. (2007). Earliest triassic microbialites in the South China block and other areas: Controls on their growth and distribution. Facies 53, 409–425. doi:10.1007/s10347-007-0105-5
Knoll, A. H., Bambach, R. K., Payne, J. L., Pruss, S., and Fischer, W. W. (2007). Paleophysiology and end-Permian mass extinction. Earth Planet. Sci. Lett. 256, 295–313. doi:10.1016/j.epsl.2007.02.018
Krull, E. S., Lehrmann, D. J., Druke, D., Kessel, B., Yu, Y., Li, R., et al. (2004). Stable carbon isotope stratigraphy across the Permian-Triassic boundary in shallow marine carbonate platforms, Nanpanjiang Basin, south China. Palaeogeogr. Palaeoclimatol. Palaeoecol. 204, 297–315. doi:10.1016/S0031-0182(03)00732-6
Lehrmann, D. J., Bentz, J. M., Wood, T., Goers, A., Dhillon, R., Akin, S., et al. (2015). Environmental controls on the Genesis of marine microbialites and dissolution surface associated with the end-permian mass extinction: New sections and observations from the Nanpanjiang Basin, south China. Palaios 30, 529–552. doi:10.2110/palo.2014.088
Lehrmann, D. J. (1999). Early triassic calcimicrobial mounds and biostromes of the Nanpanjiang Basin, south China. Geology 27, 359–362. doi:10.1130/0091-7613(1999)027<0359:etcmab>2.3.co;2
Lehrmann, D. J., Payne, J. L., Felix, S. V., Dillett, P. M., Wang, H., Yu, Y., et al. (2003). Permian-triassic boundary sections from shallow-marine carbonate platforms of the Nanpanjiang Basin, south China: Implications for oceanic conditions associated with the end-permian extinction and its aftermath. Palaios 18, 138–152. doi:10.1669/0883-1351(2003)18<138:pbsfsc>2.0.co;2
Li, M., Frank, T. D., Xu, Y., Fielding, C. R., Gong, Y., Shen, Y., et al. (2022). Sulfur isotopes link atmospheric sulfate aerosols from the Siberian Traps outgassing to the end-Permian extinction on land. Earth Planet. Sci. Lett. 592, 117634. doi:10.1016/j.epsl.2022.117634
Li, M., Grasby, S. E., Wang, S., Zhang, X., Wasylenki, L. E., Xu, Y., et al. (2021). Nickel isotopes link Siberian Traps aerosol particles to the end-Permian mass extinction. Nat. Commun. 12, 2024. doi:10.1038/s41467-021-22066-7
Liao, W., Wang, Y., Kershaw, S., Weng, Z., and Yang, H. (2010). Shallow-marine dysoxia across the permian-triassic boundary: Evidence from pyrite framboids in the microbialite in South China. Sediment. Geol. 232, 77–83. doi:10.1016/j.sedgeo.2010.09.019
Liu, H., Wang, Y., Yuan, A., Yang, H., Song, H., Zhang, S., et al. (2010). Ostracod fauna across the Permian-Triassic boundary at Chongyang, Hubei Province, and its implication for the process of the mass extinction. Sci. China Earth Sci. 53, 810–817. doi:10.1007/s11430-010-0045-8
Luo, G., Algeo, T. J., Huang, J., Zhou, W., Wang, Y., Yang, H., et al. (2014). Vertical δ13Corg gradients record changes in planktonic microbial community composition during the end-Permian mass extinction. Palaeogeogr. Palaeoclimatol. Palaeoecol. 396, 119–131. doi:10.1016/j.palaeo.2014.01.006
Luo, G., Kump, L. R., Wang, Y., Tong, J., Arthur, M. A., Yang, H., et al. (2010). Isotopic evidence for an anomalously low oceanic sulfate concentration following end-Permian mass extinction. Earth Planet. Sci. Lett. 300, 101–111. doi:10.1016/j.epsl.2010.09.041
Luo, G., Wang, Y., Yang, H., Algeo, T., Kump, L., and Huang, J. (2011). Stepwise and large-magnitude negative shift in δ13Ccarb preceded the main marine mass extinction of the Permian-Triassic crisis interval. Palaeogeogr. Palaeoclimatol. Palaeoecol. 299, 70–82. doi:10.1016/j.palaeo.2010.10.035
Meyer, K. M., Yu, M., Jost, A. B., Kelley, B. M., and Payne, J. L. (2011). δ13C evidence that high primary productivity delayed recovery from end-Permian mass extinction. Earth Planet. Sci. Lett. 302, 378–384. doi:10.1016/j.epsl.2010.12.033
Mu, X., Kershaw, S., Li, Y., Guo, L., Qi, Y., Reynolds, A., et al. (2009). High-resolution carbon isotope changes in the Permian-Triassic boundary interval, Chongqing, South China; implications for control and growth of earliest Triassic microbialites. J. Asian Earth Sci. 36, 434–441. doi:10.1016/j.jseaes.2007.08.004
Payne, J. L., and Kump, L. R. (2007). Evidence for recurrent Early Triassic massive volcanism from quantitative interpretation of carbon isotope fluctuations. Earth Planet. Sci. Lett. 256, 264–277. doi:10.1016/j.epsl.2007.01.034
Payne, J. L., Lehrmann, D. J., Follet, D., Seibel, M., Kump, L. R., Riccardi, A., et al. (2007). Erosional truncation of uppermost Permian shallow-marine carbonates and implications for Permian Triassic boundary events. Geol. Soc. Am. Bull. 119, 771–784. doi:10.1130/B26091.1
Payne, J. L., Lehrmann, D. J., Wei, J. Y., Orchard, M. J., Schrag, D. P., Knoll, A. H., et al. (2004). Large perturbations of the carbon cycle during recovery from the end-Permian extinction. Science 305, 506–509. doi:10.1126/science.1097023
Pei, Y., Chen, Z., Fang, Y., Kershaw, S., Wu, S., Luo, M., et al. (2019). Volcanism, redox conditions, and microbialite growth linked with the end-Permian mass extinction: Evidence from the Xiajiacao section (Western Hubei Province), South China. Palaeogeogr. Palaeoclimatol. Palaeoecol. 519, 194–208. doi:10.1016/j.palaeo.2017.07.020
Riccardi, A., Kump, L. R., Arthur, M. A., and D’Hondt, S. (2007). Carbon isotopic evidence for chemocline upward excursions during the end-Permian event. Palaeogeogr. Palaeoclimatol. Palaeoecol. 248, 73–81. doi:10.1016/j.palaeo.2006.11.010
Ryskin, G. (2003). Methane-driven oceanic eruptions and mass extinctions. Geology 31, 741–744. doi:10.1130/G19518.1
Shen, J., Schoepfer, S. D., Feng, Q., Zhou, L., Yu, J., Song, H., et al. (2015). Marine productivity changes during the end-Permian crisis and Early Triassic recovery. Earth. Sci. Rev. 149, 136–162. doi:10.1016/j.earscirev.2014.11.002
Shen, Y., Farquhar, J., Zhang, H., Masterson, A., Zhang, T., Wing, B. A., et al. (2011). Multiple S-isotopic evidence for episodic shoaling of anoxic water during Late Permian mass extinction. Nat. Commun. 2, 210. doi:10.1038/ncomms1217
Song, H., Tong, J., Algeo, T. J., Horacek, M., Qiu, H., Song, H., et al. (2013). Large vertical δ13CDIC gradients in Early Triassic seas of the South China craton: Implications for oceanographic changes related to Siberian Traps volcanism. Glob. Planet. Change 105, 7–20. doi:10.1016/j.gloplacha.2012.10.023
Song, H., Wignall, P. B., and Dunhill, A. M. (2018). Decoupled taxonomic and ecological recoveries from the Permo-Triassic extinction. Sci. Adv. 4, eaat5091. doi:10.1126/sciadv.aat5091
Song, H., Wignall, P. B., Tong, J., and Yin, H. (2012). Two pulses of extinction during the Permian-Triassic crisis. Nat. Geosci. 6, 52–56. doi:10.1038/ngeo1649
Svensen, H., Planke, S., Polozov, A. G., Schmidbauer, N., Corfu, F., Podladchikov, Y. Y., et al. (2009). Siberian gas venting and the end-Permian environmental crisis. Earth Planet. Sci. Lett. 277, 490–500. doi:10.1016/j.epsl.2008.11.015
Wang, L., Wignall, P. B., Wang, Y., Jiang, H., Sun, Y., Li, G., et al. (2016). Depositional conditions and revised age of the permo-triassic microbialites at gaohua section, Cili county (hunan Province, south China). Palaeogeogr. Palaeoclimatol. Palaeoecol. 443, 156–166. doi:10.1016/j.palaeo.2015.11.032
Wang, Q., Tong, J., Song, H., and Yang, H. (2009). Ecological evolution across the permian/triassic boundary at the kangjiaping section in Cili county, hunan Province, China. Sci. China Ser. D-Earth. Sci. 52, 797–806. doi:10.1007/s11430-009-0077-0
Wang, T., Burne, R. V., Yuan, A., Wang, Y., and Yi, Z. (2019). The evolution of microbialite forms during the early triassic transgression: A case study in Chongyang of hubei Province, south China. Palaeogeogr. Palaeoclimatol. Palaeoecol. 519, 209–220. doi:10.1016/j.palaeo.2018.01.043
Wang, Y., Tong, J., Wang, J., and Zhou, X. (2005). Calcimicrobialite after end-Permian mass extinction in South China and its palaeoenvironmental significance. Chin. Sci. Bull. 50, 665–671. doi:10.1360/982004-323
Wignall, P. B., Kershaw, S., Collin, P. Y., and Crasquin, S. S. (2009). Erosional truncation of uppermost permian shallow-marine carbonates and implications for permian-triassic boundary events: Comment. Geol. Soc. Am. Bull. 121, 954–956. doi:10.1130/B26424.1
Wu, S., Chen, Z., Fang, Y., Pei, Y., Yang, H., Ogg, J., et al. (2017). A Permian-Triassic boundary microbialite deposit from the eastern Yangtze Platform (Jiangxi Province, South China): Geobiologic features, ecosystem composition and redox conditions. Palaeogeogr. Palaeoclimatol. Palaeoecol. 486, 58–73. doi:10.1016/j.palaeo.2017.05.015
Xie, S., Pancost, R. D., Huang, J., Wignall, P. B., Yu, J., Tang, X., et al. (2007). Changes in the global carbon cycle occurred as two episodes during the Permian-Triassic crisis. Geology 35, 1083–1086. doi:10.1130/G24224A.1
Xie, S., Pancost, R. D., Wang, Y., Yang, H., Wignall, P. B., Luo, G., et al. (2010). Cyanobacterial blooms tied to volcanism during the 5 m.y. Permo-Triassic biotic crisis. Geology 38, 447–450. doi:10.1130/g30769.1
Yang, H., Chen, Z., Wang, Y., Tong, J., Song, H., Chen, J., et al. (2011). Composition and structure of microbialite ecosystems following the end-Permian mass extinction in South China. Palaeogeogr. Palaeoclimatol. Palaeoecol. 308, 111–128. doi:10.1016/j.palaeo.2010.05.029
Yin, H., Jiang, H., Xia, W., Feng, Q., Zhang, N., Shen, J., et al. (2014). The end-Permian regression in South China and its implication on mass extinction. Earth. Sci. Rev. 137, 19–33. doi:10.1016/j.earscirev.2013.06.003
Yin, H., Zhang, K., Tong, J., Yang, Z., and Wu, S. (2001). The global stratotype section and point (GSSP) of the Permian-Triassic boundary. Episodes 24, 102–114. doi:10.18814/epiiugs/2001/v24i2/004
Zhang, G., Zhang, X., Hu, D., Li, D., Algeo, T. J., Farquhar, J., et al. (2017). Redox chemistry changes in the Panthalassic Ocean linked to the end-Permian mass extinction and delayed Early Triassic biotic recovery. Proc. Natl. Acad. Sci. U. S. A. 114, 1806–1810. doi:10.1073/pnas.1610931114
Zhang, G., Zhang, X., and Shen, Y. (2021). Quantitative constraints on carbon cycling and temporal changes in episodic euxinia during the end-Permian mass extinction in South China. Chem. Geol. 562, 120036. doi:10.1016/j.chemgeo.2020.120036
Keywords: Carbon isotopes, End-Permian mass extinction, Microbialite, 13C-depleted carbon, South China
Citation: Huang X, Li D, Zhang X, Xu Y, Sun L, Li M and Shen Y (2022) High resolution C-isotopic data from microbialites in the aftermath of the end-Permian mass extinction in South China. Front. Earth Sci. 10:914432. doi: 10.3389/feart.2022.914432
Received: 06 April 2022; Accepted: 11 July 2022;
Published: 04 August 2022.
Edited by:
Haijun Song, China University of Geosciences Wuhan, ChinaReviewed by:
Mao Luo, Nanjing Institute of Geology and Paleontology (CAS), ChinaCopyright © 2022 Huang, Li, Zhang, Xu, Sun, Li and Shen. This is an open-access article distributed under the terms of the Creative Commons Attribution License (CC BY). The use, distribution or reproduction in other forums is permitted, provided the original author(s) and the copyright owner(s) are credited and that the original publication in this journal is cited, in accordance with accepted academic practice. No use, distribution or reproduction is permitted which does not comply with these terms.
*Correspondence: Xiaolin Zhang, emh4bDIwMTJAdXN0Yy5lZHUuY24=; Menghan Li, bGltaEB1c3RjLmVkdS5jbg==
Disclaimer: All claims expressed in this article are solely those of the authors and do not necessarily represent those of their affiliated organizations, or those of the publisher, the editors and the reviewers. Any product that may be evaluated in this article or claim that may be made by its manufacturer is not guaranteed or endorsed by the publisher.
Research integrity at Frontiers
Learn more about the work of our research integrity team to safeguard the quality of each article we publish.