- 1Guangxi Key Laboratory of Marine Environment Change and Disaster in Beibu Gulf (Beibu Gulf University), Qinzhou, China
- 2School of Geography and Planning, Nanning Normal University, Nanning, China
Suspended sediment in the water body of rivers flowing into the sea is of great significance to the accumulation process in river basins and change pattern of landforms. In particular, small and medium rivers entering the sea in mountainous areas exhibit the characteristics of both mountains and streams, and the suspended sediment concentration (SSC) greatly contributes to the formation and evolution of deltas. However, scholars rarely give attention to the factors influencing changes in the SSC in small- and medium-sized mountainous rivers, and few studies have examined SSC changes in small- and medium-sized mountainous rivers. Here, based on daily SSC and flow data obtained at the Bobai Station and Changle Station, the percentile method and regression analysis method are employed to analyse the changes in SSC from the Nanliu River to the Beibu Gulf and possible influencing factors. The main research results indicate that 1) the SSC in river water bodies from 1965 to 2020 generally reveals a downwards trend, with significant annual variations. Specifically, the overall trend can be divided into three stages: 1) the SSC is the highest from 1965 to 1971, and the mean yearly SSC reaches 0.25 kg/m3; 2) the SSC is relatively high from 1972 to 2006, and the mean yearly SSC reaches 0.16 kg/m3; and 3) the SSC is the lowest from 2007 to 2020, and the mean yearly SSC reaches 0.11 kg/m3 2) High values of the SSC are mainly concentrated in the flood season, and low SSC values mostly occur in the dry season. The monthly average SSC in the flood season from April to September and the dry season from October to March exhibits the characteristics of a decrease in the flood season and an increase in the dry season. Moreover, the peak SSC value in the watershed obviously occurs out of sync with the peak flow value. Generally, the former precedes the latter. 3) The curve of the flow rate-sediment ratio is an irregular clockwise rhombus. Moreover, the impact of tropical cyclones, land cover changes, regional GDP per capita, and engineering construction are crucial reasons for the observed variations in the SSC in the Nanliu River Basin. In this paper, the obtained research results provide an important guiding significance for the planning and management of water and sediment resources in the Nanliu River and offer a reference for hydrological planning of other river basins discharging into the sea.
1 Introduction
The sediment transport process is highly important to socioeconomic development and the ecological environment in river basins. Suspended sediment in river water is transported to the ocean through rivers, and the main driving force is river flow (Matos et al., 2018; Wei et al., 2021). Sediment transport is very important among the processes on the Earth’s surface, and sediment transport exerts a vital influence on the regulation of erosion-accretion phenomena and shaping of the landform pattern (Matos et al., 2018; Wei et al., 2021). Suspended sediment is the main component of the total sediment load, and sediment constitutes a natural part of the river environment and an indispensable part of the river system. Long-term deposition of suspended sediment plays a key role in the formation of the shallow sea continental shelf, evolution of the seabed topography, and estuary environment (Zuo et al., 2012; Zhang et al., 2014; Tang et al., 2019). However, in recent decades, horizontal transport of suspended sediment between the river and ocean has been greatly affected by human activities and climate change, which has caused changes in the transport volume and spatial distribution of sediment (Farnsworth and Milliman, 2003; Li et al., 2012; Wu et al., 2019; Huang et al., 2022a). Because of the continuous reduction of suspended sediment entering the sea, the ecological environment of the estuary has undergone tremendous changes, such as the retreat of coastlines, the reduction of wetland areas, and the impact of biological habitats (Coleman et al., 1998; Dai et al., 2013). Studies have demonstrated that tropical cyclones affect the spatial variation in the amount of suspended sediment (Huang et al., 2022b), leading to changes in the suspended sediment amount reaching the delta (Darby et al., 2016; Tang R. et al., 2021). Therefore, in recent years, researchers have given increasing attention to the long-term change trend and temporal and spatial changes in SSC from rivers to the ocean.
To date, a large number of studies have indicated that runoff and the SSC in many large rivers are regulated by human activities, especially the reconstruction of navigation channels, construction of dams and reservoirs, and soil and water conservation projects, which have caused the amount of suspended sediment in certain large rivers to drastically decrease (Yang et al., 2002; Walling and Fang, 2003; Yang S. L. et al., 2015; Dai et al., 2016). It has been confirmed that human activities are the main factor leading to a reduction in the SSC in rivers flowing to the sea (Dai et al., 2009; Wu et al., 2012). For example, after the completion of the Three Gorges Dam in the Yangtze River, the SSC in the estuary immediately dropped by 40.3%, and the sediment load in the Yangtze River estuary was drastically reduced, resulting in a sharp decline in the wetland area (Yang et al., 2005; Wang et al., 2015; Wei et al., 2021). An early study determined that the construction of the Xiaolangdi Reservoir in the middle and lower reaches of the Yellow River reduced the flow of sediment into the sea by more than 90% (Wang et al., 2010). Moreover, water and soil conservation projects and the Grain for Green project were initiated on the Loess Plateau in the 1970s, and water discharge and the daily SSC consequently declined significantly (Zhang et al., 2017). Not only are the variations in the SSC influenced by human activities (Yang et al., 2002; Chu et al., 2009), but also influenced by natural factors, such as tropical cyclones, rainfall seasonality, soil erosion, watershed size and topography, etc. (Montanher et al., 2018; Shams et al., 2020; Huang et al., 2022b). Therefore, scholars in the SSC-related research field have also studied other possible factors influencing the long-term variations in the SSC, and found that the changes of SSC is controlled by factors such as water flow direction, peak flow, rainfall intensity, and duration of extreme events (Nadal et al., 2007; Dan et al., 2020; Tang R. G. et al., 2021). In summary, researchers have conducted fruitful explorations of the possible factors influencing the variations in the SSC. However, most studies above have mainly concentrated on the impact of natural factors and human activities on the variations in the SSC in large rivers. Therefore, more attention should be given to SSC changes in small and medium mountain rivers flowing into the sea.
However, variations in the SSC influence the composition of local species, survival rate of organisms, estuarine delta marshes, and contents of nitrogen and phosphorus elements (Müller and Förstner, 1968; Yang et al., 2020; Li et al., 2021). Studies have demonstrated that the SSC is a significant nonbiological variable, and its changes can affect the life of benthic organisms (Tramblay et al., 2008; Shi et al., 2017). The SSC is related to the water temperature and salinity (Ramalingam and Chandra, 2019) and land-use change (Ferreira et al., 2020). Additionally, the SSC is also related to land reclamation (van Maren et al., 2016), reservoir storage (Yang Y. P. et al., 2015), and soil erosion (Syvitski et al., 2005). To date, although the study of the SSC has received extensive attention, the long-term and annual variations in the SSC should be further studied, especially in the mountainous Nanliu River.
The Nanliu River is located in the northern part of the Beibu Gulf. This river originates in the Darong Mountain and flows into Lianzhou Bay in Hepu County. The Nanliu is the single largest river in Guangxi flowing into the Beibu Gulf (Figure 1). The total drainage area reaches 9,700 km2, and the river length is 287 km. This river is located in the southern subtropical maritime monsoon climate zone, with a mild climate and abundant rainfall, and the average annual rainfall reaches approximately 1736 mm (Li et al., 2016). The upper and middle reaches of the Nanliu River Basin are the Yulin Basin and Bobai Basin respectively, and the lower reaches include the Hepu Alluvial Plain and the Nanliu River Delta. The Nanliu River flows into the sea in a mountainous area. This river exhibits the highest long-term SSC among the various rivers flowing into the sea surrounding the Beibu Gulf. However, previous studies on the SSC have focused on large rivers, such as the Yellow River (Wang et al., 2010), Amazon River (Montanher et al., 2018), Yangtze River (Chen et al., 2006), Rhine River (Asselman, 1999), and Mississippi River (Meade and Moody, 2010), in regard to the research area, but little attention has been given to the long-term sequential variations in the SSC in small and medium rivers and possible influencing factors. The variability and dynamics of the SSC in small and medium rivers thus remain unclear. Studying the change in the SSC in medium and small rivers can provide important information regarding engineering issues such as regional water conservancy design and river sediment control (Hsu and Cai, 2010). Therefore, it is of great practical significance to study small and medium rivers and provide them with hydrological management strategies. In this paper, the Nanliu River, a typical mountainous small to medium river flowing into the sea, is selected as the research object to study the variations in the SSC at various scales. The objectives of this paper are 1) to examine the overall features of the variations in the SSC in the Nanliu River in the past 60 years; 2) to analyse the main driving factors influencing changes in the SSC in the past 60 years.
2 Materials and Methods
2.1 Materials
The Bobai Station is located in Chengxiang town, Bobai County, Yulin city, Guangxi, in the upper reaches of the Nanliu River. This station mainly controls the variations in water and sediment from the upstream region. The Changle Station is located in Changle town, Hepu County, Beihai city, Guangxi, in the lower reaches of the Nanliu River. This station mainly controls the variations in river flow and sediment volume in the middle and upper reaches of the river. These two stations are the most important hydrological stations in the Nanliu River. The data collected at these two stations can represent the flow and SSC in the Nanliu River. River flow and SSC data between 1965 and 2020 were obtained from the Pearl River Water Conservancy Commission of the Ministry of Water Resources of China, but some SSC data were missing, including data from 1967 to 1975 and 1985 to 2000. Precipitation data for the Nanliu River Basin between 1970 and 2020 were obtained from the China Meteorological Administration. The per capita GDP from 1989 to 2019 was retrieved from the Guangxi Statistical Yearbook of the various years. Additionally, the remote sensing image data mainly comes from the official website of the United States Geological Survey (https://earthexplorer.usgs.gov/bulk).
2.2 Methods
The percentile method is an important method to analyse the characteristics of long-series data. In this study, the percentile method was employed to analyse the changes in the SSC in the Nanliu River. With the use of percentiles, the daily SSC was divided into seven levels: 5, 10, 25, 50, 75, and 95%. The third level (25%) and fifth level (75%) of the daily SSC were selected as thresholds. The third level of 25% represents low-SSC events, while the fifth level of 75% represents high-SSC events. Moreover, to further analyse the changes in the daily SSC throughout the study year, the hydrological coefficient of variation (Cv) was calculated.
To analyse the variations in the daily SSC, a combination of linear regression and power functions was applied to perform power regression of the daily water discharge and daily SSC data to obtain the sediment grade curve and constants a and b in the following form:
where QS is the daily SSC, kg/m3; QW is the water discharge rate, m3/s; a is the slope of the equation; and b is the intercept of the curve (Guzman et al., 2013; Mouri et al., 2014; Dai et al., 2016).
To analyse the changes of building area in the Nanliu River Basin from 1988 to 2018, ENVI 5.3 was used to supervise and classify the remote sensing images, and the building area of the Nanliu River Basin was extracted, and then the building area data of each year was obtained by calculation.
3 Results
3.1 Annual Variation Characteristics of the SSC
Events with an annual average SSC higher than the fifth level at the Bobai Station mainly occurred before 1970, while events with a yearly average SSC lower than the third level mainly occurred after 2006 (Figure 2A). Events in which the yearly average SSC at the Changle Station was higher than the fifth level and lower than the third level occurred in 1971 and 2006, respectively (Figure 3A). Therefore, the annual average SSC in the Nanliu River Basin could be divided into three periods based on these two specific years of 1971 and 2006, namely, 1965–1971, 1972–2006, and 2007–2020. At the first stage, from 1965 to 1971, the annual average SSC was the highest, while at the second stage, from 1972 to 2006, the yearly average SSC was relatively high. Moreover, at the third stage, from 2007 to 2020, the annual average SSC was relatively low. The yearly mean SSC values during these three periods reached 0.25 kg/m3, 0.16 kg/m3 and 0.11 kg/m3, respectively. The annual average SSC in the Nanliu River from 1965 to 2020 exhibited a downward trend in general.
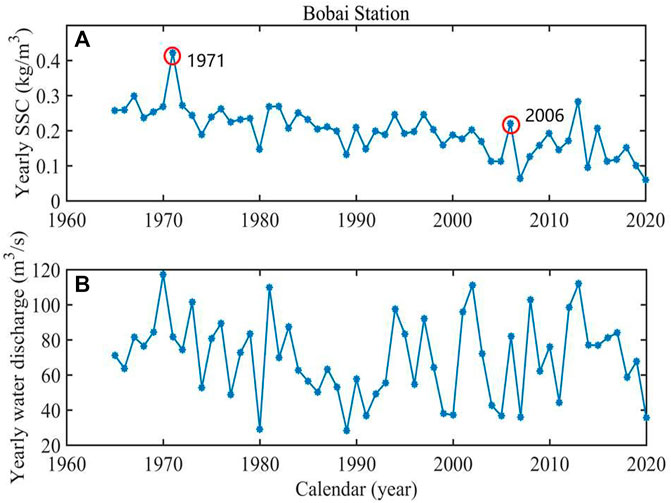
FIGURE 2. Variation trends of the yearly SSC and yearly water discharge at the Bobai Station from 1965 to 2020 (A) variation in the average SSC; (B) variation in the water discharge.
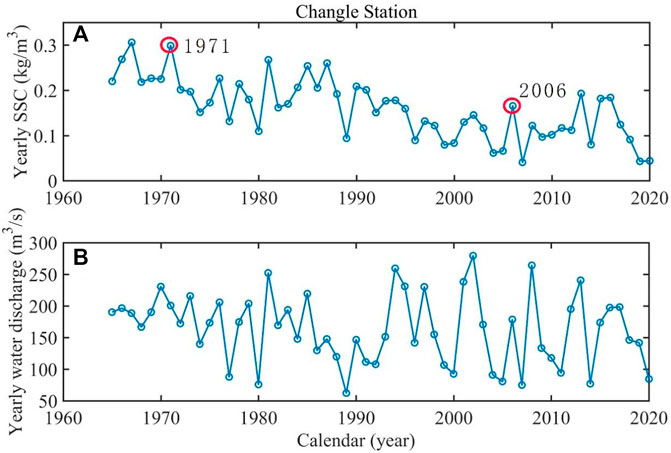
FIGURE 3. Variation trend of the SSC and water discharge at the Changle Station from 1965 to 2020 (A) variation in the yearly SSC; (B) variation in the yearly water discharge.
The third-level change trend of the daily average SSC was basically consistent with the fifth-level change trend (Figures 4A, B). The third level of the daily SSC maintained a low average SSC from 1965 to 2010, which suggests that the proportion of low-to average-SSC events was high. Between 2011 and 2016, there occurred an upwards trend (Figure 4A). After 2016, a clear decreasing trend was observed. Furthermore, the hydrological coefficient and maximum average SSC exhibited a significant downwards trend (Figures 4C, D), indicating that the daily average SSC decreased in the past 60 years.
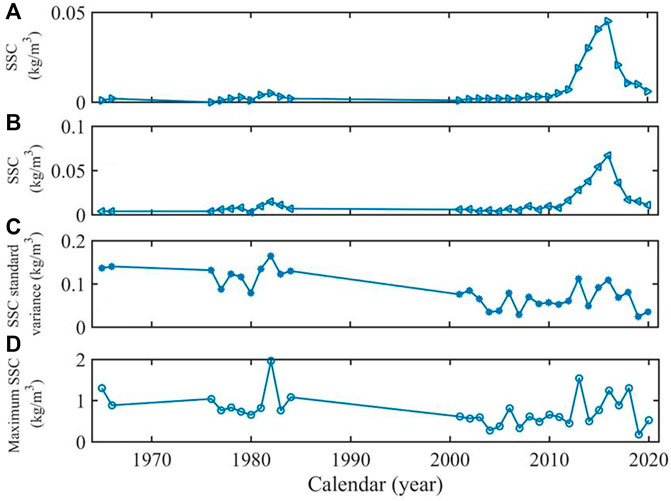
FIGURE 4. Variation trend of the SSC in the Nanliu River water body from 1965 to 2020: SSC at the (A) third level and (B) fifth level (C) coefficient of variation, CV; (D) maximum SSC.
3.2 Frequency Distribution Characteristics of the SSC
Due to the lack of SSC data in certain years, the analysis was divided into three periods from 1965 to 1966, 1976 to 1984, and 2001 to 2020 based on the existing data. There occurred obvious differences in the monthly maximum SSC at the three stages, and the overall state fluctuated to varying degrees. According to the seasonal difference in precipitation in the Nanliu River, April to September was categorized as the flood season, and October to December and January to March were categorized as the dry season. During the above three periods, low-value areas of the SSC mostly occurred in the dry season, and high-value sections were mainly distributed in the flood season. Figure 5 shows that the mean monthly maximum SSC at the two stages of 1965–1966 and 1976–1984 indicated fluctuations of 0.04–0.56 kg/m3 and 0.08–0.46 kg/m3, respectively, and varied with the season. From 2001 to 2020, the mean monthly maximum SSC varied irregularly, fluctuating between 0.05 and 0.49 kg/m3, with peaks in April, July, and November. During the above three periods, the mean monthly maximum SSC difference reached 0.53 kg/m3, 0.36 kg/m3, and 0.41 kg/m3. The mean monthly maximum SSC from 1965 to 1966 varied the most, at 1.5 and 1.3 times that from 1976 to 1984 and 2001–2020, respectively (Figure 5). It should be noted that the maximum SSC in the dry season exhibited an upwards trend during the three periods, following 1965–1966<1976–1984<2001–2020, especially in January, March, November, and December. In contrast, the flood season exhibited a growth trend, following 1965–1966> 1976–1984> 2001–2020, especially in June and August.
Figure 6 shows that the monthly average SSC and monthly average flow significantly changed at the three stages. The monthly average SSC reached 0.24 kg/m3 in the flood season between 1965 and 1966, and in the dry season, it reached 0.05 kg/m3. The monthly average SSC was 0.17 kg/m3 in the flood season from 1976 to 1984, and in the dry season, it reached 0.04 kg/m3. The average monthly SSC values in the flood and dry seasons from 2001 to 2020 were 0.1 kg/m3 and 0.06 kg/m3, respectively. Among the three stages, the average monthly SSC was the highest in the dry season from 2001 to 2020, which was 0.01 kg/m3 and 0.02 kg/m3 higher than the monthly average SSC in the dry season from 1965 to 1966 and 1976 to 1984, respectively. From 1965 to 1966, the peak average SSC value reached 0.4 kg/m3, which occurred in April, and the peak water discharge value occurred in August. Notably, the peak water discharge lagged behind the peak SSC by 4 months. The peak values of the monthly average SSC of 0.2 kg/m3 and 0.15 kg/m3 from 1976 to 1984 and 2001 to 2020, respectively, occurred in May. Therefore, the peak monthly average SSC sequentially decreased from 1965 to 1966, 1976 to 1984, and 2001 to 2020 (Figure 6A). The monthly average SSC reached 0.51 kg/m3 in the flood season during these three periods, which is 0.36 kg/m3 higher than the value of 0.15 kg/m3 in the dry season. Moreover, the monthly average water discharge in the flood season reached 285 m3/s, which is 212 m3/s higher than that in the dry season. In addition, the monthly average SSC and monthly average flow during these three periods exhibited the characteristics of decreasing in the flood season and increasing in the dry season. Notably, the performance of the SSC in the flood season adhered to 1965–1966<1976–1984<2001–2020, and the performance of the SSC in the dry season followed 1965–1966>1976–1984>2001–2020, especially the flood season performance (Figure 6B). This phenomenon is similar to the change characteristics of the monthly maximum SSC (Figure 5).
At 0.01-kg/m3 intervals, the frequency of the average daily SSC was analysed during the three periods. The results indicated that the frequency of daily average SSC values higher than 0.4 kg/m3 exceeded 5% from 1965 to 1966, approached 5% from 1976 to 1984, and decreased to 2% from 2001 to 2002 (Figure 7). The frequency of values ≤0.1 kg/m3 during these three periods accounted for 78, 85, and 92%, respectively, of the overall frequency. The daily average SSC within the interval of 0.2–0.4 kg/m3 was very low, and there occurred almost no value from 2001 to 2020. The maximum frequency of the daily mean SSC was observed at 0.01 kg/m3 from 1965 to 1966, and the frequency approached 40%. From 1976 to 1984 and 2001–2020, the maximum frequency occurred at 0.02 kg/m3, and this frequency accounted for approximately 25% of the overall frequency. Furthermore, the proportion of 0.01 kg/m3 successively decreased over the three periods, while the proportions of 0.02 and 0.03 kg/m3 successively increased. From the above, the single-peak features of the daily average SSC became increasingly distinct and tended to move backwards during the three periods.
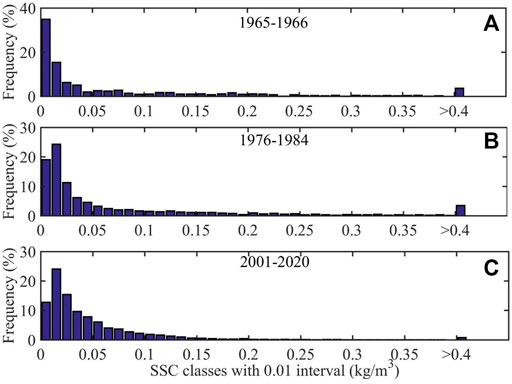
FIGURE 7. Frequency of the daily SSC at the three stages (A) 1965–1966; (B) 1976–1984; (C) 2001–2020.
3.3 Flow-Sediment Ratio Curve
The flow-sediment ratio curve reflects the different temporal and spatial variations in water and sediment (Figure 8). The SSC in the Nanliu River from 1965 to 1966 exhibited an increasing trend from January to April, with the highest peak in April and irregular fluctuations from April to August. Nevertheless, from August to December, the monthly average SSC gradually dropped to its lowest value. The area enclosed by the entire flow-sediment ratio curve resembled a clockwise irregular rhombus, which was the opposite to the counterclockwise rhombus revealed by the Yangtze River flow-sediment ratio curve (Dai et al., 2016). During the period from 1976 to 1984, the peak of the flow-sediment ratio curve was significantly reduced from 1965 to 1966, and the lowest peak occurred in May. The entire curve became relatively narrow, and the area enclosed by the curve was significantly smaller than that enclosed by the curve for the period from 1965 to 1966. Moreover, compared to the periods from 1965 to 1966 and 1976 to 1984, the flow-sediment curve was the narrowest from 2001 to 2020, indicating that the monthly average SSC changes were relatively limited. However, the flow-sediment curve for the two periods from 1976 to 1984 and 2001 to 2020 exhibited the same characteristics: the average SSC increased with increasing flow and time, and the average SSC occurred at the rising stage from January to May. This parameter reached its highest peak throughout the month (Figure 8). Therefore, there existed a positive correlation between the SSC and water discharge (Chu et al., 2009).
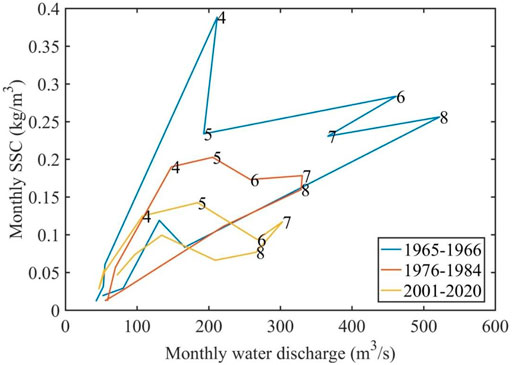
FIGURE 8. Ratio curve of the monthly SSC and monthly water discharge (the number indicates the month).
4 Discussion
4.1 Relationship Between Rainfall and Flow
Rainfall is an important factor affecting river flow and SSC (Tang R. et al., 2021). Figure 9 shows that the mean annual rainfall in the Nanliu River from 1960 to 2020 and the flow at the Bobai Station and Changle Station tended to change simultaneously, Changes in rainfall reflect trends in river flow. At the same time, it can be found from Figure 9 that the rainfall of the Nanliu River during 1965–2020 reached a large value in 1981, 2002 and 2006, and the water discharge of Changle Station and Bobai Station also reached a large value. Rainfall is closely related to flow, rainfall and flow have a great impact on SSC (Moskalski and Torre, 2012). Furthermore, Comparing Figure 9 with Figure 2 and Figure 3, it can be found that the variation trends of rainfall, flow and SSC of Nanliu River are consistent, indicating that rainfall, flow and SSC are closely related. Further discussion on the relationship between rainfall and flow. In 1981, due to the superimposition effect of two typhoons, i.e., Kaili and Linen, heavy rainfall occurred in the Nanliu River Basin, with a mean annual rainfall of approximately 2,452 mm. The Nanliu River experienced a significant increase in water discharge, and a once-in-a-year flood occurred. The peak water level reached as high as 18.18 m, and the peak discharge rate reached 3,630 m3/s. In 2002, the average annual rainfall in the Nanliu River Basin reached 2,373 mm. There occurred a once-in-five-year flood in the Nanliu River, and the flood peak exhibited multipeak features. At this time, the flood peak water level reached 17.75 m, and the peak discharge reached 2,780 m3/s, which accounted for 77% of that in 1981. Furthermore, in 2008, the basin was affected by Typhoon Hagupit, which occurred as a heavy rainstorm in southeastern Guangxi. The average annual rainfall in the Nanliu River Basin reached 2,400 mm, and an over-alert flood occurred in the upper reaches of the Nanliu River. Therefore, in the moderate-flood years of 1981, 2002, and 2008, the high precipitation intensity resulted in high water discharge at the Bobai Station and Changle Station (Figures 9A–C), indicating that precipitation was the principal source of water flow in the Nanliu River Basin.
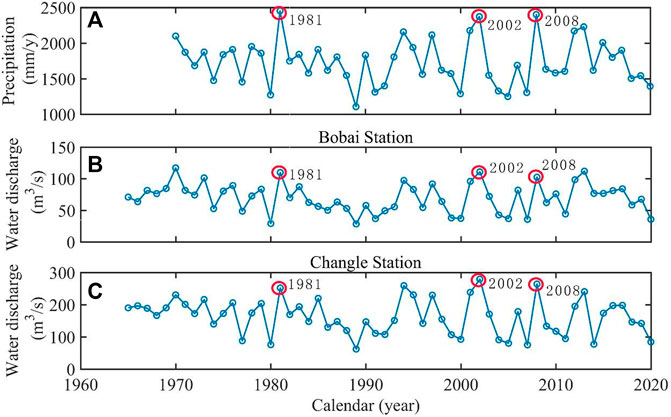
FIGURE 9. Change trend of the precipitation and water discharge from 1965 to 2020 (A) yearly average precipitation; (B) yearly average water discharge at the Bobai station; (C) yearly average water discharge at the Changle station.
4.2 Relationship Between the SSC and Flow
Rainfall and water discharge are the key factors influencing the changes in the sediment volume, and there exists a strong correlation among these three factors (Shams et al., 2020). The analysis revealed that there exists a significant positive correlation between the annual average water discharge and the annual average rainfall in the Nanliu River Basin (Figure 10A), indicating that the river basin flow mainly originates from precipitation, and the flow increases with the increase of rainfall (Figure 9). Studies have shown that rainfall erosion intensity affects the change of sediment volume, and there is a positive correlation between sediment volume and rainfall (Zhang et al., 2015; Shams et al., 2020). Then, correlation analysis between the daily average SSC and daily average water discharge during the three periods from 1965 to 1966, 1976 to 1984, and 2001 to 2020 demonstrated that there exists a positive correlation between these two parameters (Figures 10B–D), SSC changes with the water discharge (Figures 2, 3), and the SSC and water discharge change synchronously. Flow is one of a primary controlling factors for the change of SSC, and SSC increases with the increase of flow (Figure 8) (Gong et al., 2011; Moskalski and Torre, 2012; Dai et al., 2016). To sum up, rainfall affects flow, which further affects SSC. It can be seen that rainfall is an indirect factor affecting the SSC, and flow is the most direct factor affecting the change of SSC.
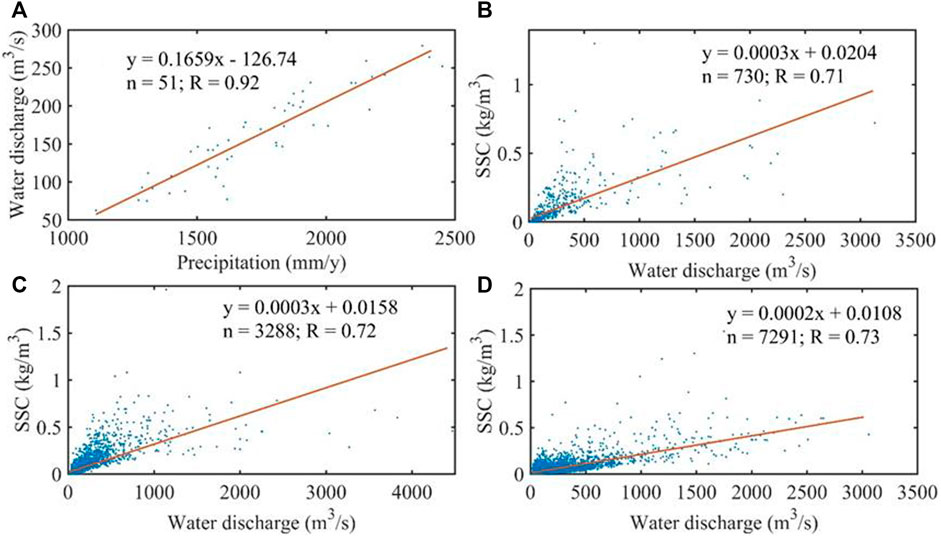
FIGURE 10. Water discharge and precipitation from 1970 to 2020 (A): SSC and water discharge (B–D) indicate 1965–1966, 1976–1984, and 2001–2020).
The peak values of the SSC and water discharge in the watershed occurred obviously out of sync. Generally, the former preceded the latter. Figures 2, 3 show that the average annual precipitation was relatively low in 1980, and 1980 was a dry year, with a low average flow but high SSC value. The average annual rainfall in 2002 and 2006 was relatively high, which were flood years, with a relatively high average water discharge, but the SSC was low. Further analysis demonstrated that the peak values of the SSC and water discharge in 1980, 2002, and 2006 were delayed (Figures 11A–C, respectively). In 1980, the maximum daily average SSC value of 0.65 kg/m3 occurred on July 20, and the maximum daily average water discharge occurred on August 23 (Figure 11A). In 2002, the highest daily average SSC (0.56 kg/m3) occurred on June 4, and the highest daily average water discharge occurred on September 29 (Figure 11B). In 2006, the highest daily mean SSC (0.81 kg/m3) occurred on July 11, and the highest daily mean water discharge occurred on July 18 (Figure 11C).
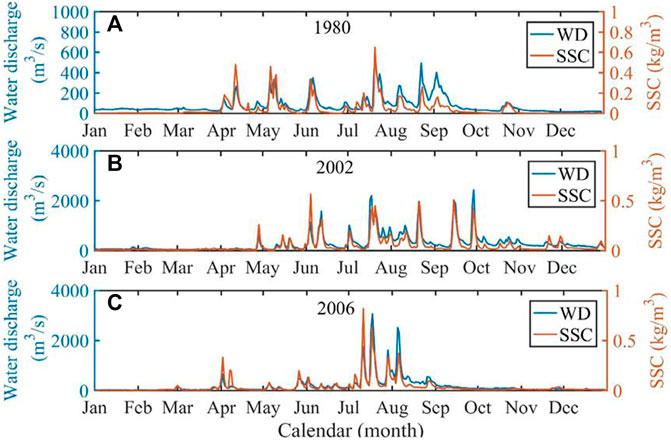
FIGURE 11. Relationship between the SSC and water discharge (A) dry year of 1980; (B) flood year of 2002; (C) flood year of 2006.
4.3 Relationship Between the SSC and Tropical Cyclones
Based on statistics, extreme weather events such as tropical cyclones exert an enormous impact on changes in the SSC in the Nanliu River. In years with more tropical cyclones, the yearly mean SSC was maintained at a relatively high level at the Changle and Bobai stations. (Figure 12). For example, from 1971 to 1974, the average SSC reached 0.21 kg/m3 at the Changle station and reached up to 0.28 kg/m3 at the Bobai station, which was higher than the multiyear average levels of 0.05 kg/m3 and 0.08 kg/m3, respectively. From 1995 to 1996, the average SSC reached 0.17 kg/m3 at the Changle station and reached 0.22 kg/m3 at the Bobai station, which remained higher than the mean level. In contrast, in 2004, without the influence of tropical cyclones, the average online concentration at these two stations remained low. Considering the above statistics, the Nanliu River Basin has been impacted by 260 tropical cyclones over the past 50 years, with an average of 4.6 cyclones per year. Tropical cyclones have become a vital factor impacting the changes in the SSC in the Nanliu River.
4.4 Impacts of Human Activities
Human activities can significantly affect the hydrological characteristics of rivers and changes along deltaic coasts (Yang et al., 2002; Dai et al., 2011; Dai et al., 2014). The Nanliu River is located in the Beibu Gulf Economic Area, and the city is clustered around the Beibu Gulf. This region is affected by human activities such as urbanization and engineering construction. This paper analysed the impact on the SSC from the aspects of land cover change, per capita GDP, and water consumption.
4.4.1 Land Cover Change
Sediment transport depends on land cover and land use (Guzman et al., 2013). Based on the above aspects, the SSC in the Nanliu River exhibited a clear downwards trend, and the SSC revealed the characteristics of a phased decline during these three periods. This may be related to changes in land use, a significant factor reflecting the degree of anthropogenic activities. Deforestation in the basin is severe, and the forest coverage rate has declined, leading to substantial soil erosion. Choosing Lingshan County in the upper reaches of the Nanliu River as an example, the soil erosion area reached 3,120 hm2 in 1974, increased to 5,500 hm2 in 1980, and further increased to 2.141×105 hm2 in 1987 (Li et al., 2016). Therefore, severe soil erosion caused relatively high SSC levels from 1972 to 2006, which is the second period.
Since 2000, the vegetation coverage in the Nanliu River Basin has started to increase, thanks to the large-scale artificial planting of eucalyptus forests. Simultaneously, In response to the problem of soil erosion, relevant departments have implemented corresponding measures. Governments at all levels in the basin area prioritized soil erosion control, extensively executed water and soil conservation laws and regulations, mobilized the masses to plant trees and build terraces, and achieved effective results. Governments at all levels increased funding to encourage people to engage in afforestation and fruit cultivation activities and prevent afforestation in mountains. Since 2016, the Yulin Municipal Government has carried out comprehensive work to improve the environment of the Nanliu River Basin. Therefore, effective results have been achieved in terms of forest protection, soil erosion has been effectively controlled, the river sediment load has been reduced, and the SSC has decreased, which represents the third period.
4.4.2 Economic and Social Development
By analysing the per capita GDP of the three cities of Qinzhou, Beihai, and Yulin in the Nanliu River Basin from 1989 to 2019, it was found that the per capita GDP of these three cities in the basin rapidly increased, revealing an upwards trend. From 1989 to 2006, the per capita GDP slowly rose. From 2007 to 2019, the per capita GDP rapidly increased (Figure 13). The GDP per capita of Qinzhou, Beihai, and Yulin in 2019 increased by 25, 24, and 14 times, respectively, over the 1993 levels, indicating that the economic development level in the Nanliu River Basin is continuously improving. With the improvement of economic level, the demand for construction land increases, which promotes the increase of building scale. It can be seen from Figure 14 that the construction land area of Nanliu River increased significantly from 1988 to 2018. According to statistics, the construction area of Nanliu River Basin was 159 km2 in 1988, and the construction area increased to 526 km2 in 2018. In the past 30 years, the construction area increased by 367 km2. River sediment and gravel are the main materials for construction (Chen et al., 2005), indicating that under the influence of the increase in demand for construction materials, sand mining activities increased to a certain extent, resulting in a decrease in river sediment. If the sediment transport is blocked by sand mining activities, the sediment carried by the water discharge will be reduced (Kondolf, 1997). To sum up, The economic development level and construction land of Nanliu River showed an upward trend in 1989–2019, But the SSC showed an overall downward trend (Figure 2; Figure 3A), indicating that there exists a connection among them. Therefore, sand mining activities caused by the improvement of economic development level may affect the reduction of river sediment to a certain extent, resulting in the decline of SSC.
4.4.3 Hydraulic Engineering
Studies have demonstrated that water conservancy projects such as dam regulation and reservoir construction projects are the main factors leading to reduction in the SSC in rivers flowing into the sea (Yang et al., 2005; Chu et al., 2009; Dai et al., 2016). The Nanliu River Basin contains two typical dams: Shahe Dam and Zongjiang Gate. Similarly, there are the Hongchaojiang Reservoir and Hepu Reservoir (Li et al., 2016). In 2002, Yulin city in the upper reaches and Hepu County in the lower reaches implemented embankments on both banks of the Nanliu River. Reconstruction and construction projects all control changes in the SSC along the Nanliu River. However, because most of these dams and reservoirs were built before 1965 in the basin, water and sediment observation data were lacking before 1965. At present, it is impossible to quantitatively evaluate the impact of dams and other hydraulic projects on the SSC. However, there is no doubt that the SSC has always been affected by human activities such as dam construction and reservoir repair activities.
5 Conclusion
As the largest river flowing into the Beibu Gulf along the coast of Guangxi, the Nanliu River plays a vital role in transporting suspended sediment from the river to the ocean. Here, analysis of the changes in the SSC provides a significant theoretical and practical significance in the Nanliu River. Therefore, we analysed SSC data for the Nanliu River in the past 60 years and obtained the following conclusions:
(1) The average SSC in the Nanliu River exhibited a downward trend from 1965 to 2020, and this period could be divided into three stages. The average SSC reached 0.25 kg/m3 from 1965 to 1971, which was the highest average SSC value. From 1972 to 2006, the average value reached 0.16 kg/m3, and the average SSC was relatively high. The average SSC was low from 2007 to 2020, with an average value of 0.11 kg/m3.
(2) The average SSC in the Nanliu River revealed significant seasonal changes, and the observed seasonal oscillations also varied. High values of the SSC were mainly concentrated in the flood season, while low values were mostly distributed in the dry season. Moreover, the SCC during these three periods exhibited the characteristics of decreasing in the flood season and increasing in the dry season. Furthermore, the peak values of the SSC and water discharge in the watershed occurred obviously out of sync, and the former generally preceded the latter.
(3) In the past 60 years, the gradual and continuous decline in the SSC in the Nanliu River has been caused by human activities and climate change. This basin is relatively heavily affected by soil and water conservation projects, dam construction, reservoir water storage projects, and tropical cyclones. Between 1965 and 2020, the SSC was higher due to soil erosion. However, with the implementation of forest protection policies and construction of water conservancy projects, the SSC gradually decreased. Therefore, under the influence of human activities, the SSC in the Nanliu River may continue to decline in the future.
Data Availability Statement
The data analyzed in this study is subject to the following licenses/restrictions: The data set is confidential. Requests to access these datasets should be directed to the corresponding author.
Author Contributions
SL: Data Curation, Formal analysis, Project administration. XY: Writing—Original Draft, HH: Writing—Review and Editing. XL: Formal analysis, Visualization. RW: Field investigation and simulation test. BF: Field monitoring.
Funding
This study was supported by the National Science Foundation of Guangxi (2018JJD150005, 2019AC20109) and the National Science Foundation of China (NSFC) (41866001 and 41930537).
Conflict of Interest
The authors declare that the research was conducted in the absence of any commercial or financial relationships that could be construed as a potential conflict of interest.
Publisher’s Note
All claims expressed in this article are solely those of the authors and do not necessarily represent those of their affiliated organizations, or those of the publisher, the editors and the reviewers. Any product that may be evaluated in this article, or claim that may be made by its manufacturer, is not guaranteed or endorsed by the publisher.
References
Asselman, N. E. M. (1999). Suspended Sediment Dynamics in a Large Drainage Basin: the River Rhine. Hydrol. Process. 13, 1437–1450. doi:10.1002/(sici)1099-1085(199907)13:10<1437:aid-hyp821>3.0.co;2-j
Chen, X. Q., Zhang, E. F., Mu, H. Q., and Y, Z. (2005). A Preliminary Analysis of Human Impacts on Sediment Discharges from the Yangtze, China, into the Sea. J. Coast. Res. 21 (3), 515–521. doi:10.2112/03-0034.1
Chen, S.-L., Zhang, G.-A., Yang, S.-L., and Shi, J. Z. (2006). Temporal Variations of Fine Suspended Sediment Concentration in the Changjiang River Estuary and Adjacent Coastal Waters, China. J. Hydrol. 331, 137–145. doi:10.1016/j.jhydrol.2006.05.013
Chu, Z., Zhai, S., Zhang, J., and Ding, D. (2009). Filling of the Three Gorges Reservoir to the 135-m Level: Instant Effects on the Yangtze Discharge and Suspended Sediment Concentration Entering the Estuary. J. Ocean. Univ. China 8, 291–295. doi:10.1007/s11802-009-0291-6
Coleman, J. M., Roberts, H. H., and Stone, G. W. (1998). Mississippi River Delta: an Overview. J. Coast. Res. 14 (3), 698–716.
Dai, S. B., Yang, S. L., and Li, M. (2009). The Sharp Decrease in Suspended Sediment Supply from China's Rivers to the Sea: Anthropogenic and Natural Causes. Hydrol. Sci. J. 54, 135–146. doi:10.1623/hysj.54.1.135
Dai, Z., Du, J., Zhang, X., Su, N., and Li, J. (2011). Variation of Riverine Material Loads and Environmental Consequences on the Changjiang (Yangtze) Estuary in Recent Decades (1955-2008). Environ. Sci. Technol. 45, 223–227. doi:10.1021/es103026a
Dai, Z.-J., Chu, A., Li, W.-H., Li, J.-F., and Wu, H.-L. (2013). Has Suspended Sediment Concentration Near the Mouth Bar of the Yangtze (Changjiang) Estuary Been Declining in Recent Years? J. Coast. Res. 289 (4), 809–818. doi:10.2112/jcoastres-d-11-00200.1
Dai, Z., Liu, J. T., Wei, W., and Chen, J. (2014). Detection of the Three Gorges Dam Influence on the Changjiang (Yangtze River) Submerged Delta. Sci. Rep. 4, 6600. doi:10.1038/srep06600
Dai, Z., Fagherazzi, S., Mei, X., and Gao, J. (2016). Decline in Suspended Sediment Concentration Delivered by the Changjiang (Yangtze) River into the East China Sea between 1956 and 2013. Geomorphology 268, 123–132. doi:10.1016/j.geomorph.2016.06.009
Dan, S., Vandenabeele, S., Verwaest, T., and Montreuil, A.-L. (2020). Hydrodynamics versus Sediment Concentration at the Belgian Coast. J. Coast. Res. 95, 632–636. doi:10.2112/si95-123.1
Darby, S. E., Hackney, C. R., Leyland, J., Kummu, M., Lauri, H., Parsons, D. R., et al. (2016). Fluvial Sediment Supply to a Mega-Delta Reduced by Shifting Tropical-Cyclone Activity. Nature 539, 276–279. doi:10.1038/nature19809
Farnsworth, K. L., and Milliman, J. D. (2003). Effects of Climatic and Anthropogenic Change on Small Mountainous Rivers: the Salinas River Example. Glob. Planet. Change 39, 53–64. doi:10.1016/s0921-8181(03)00017-1
Ferreira, C. S. S., Walsh, R. P. D., Kalantari, Z., and Ferreira, A. J. D. (2020). Impact of Land-Use Changes on Spatiotemporal Suspended Sediment Dynamics within a Peri-Urban Catchment. Water 12, 665. doi:10.3390/w12030665
Gong, Z., Zhang, C. C., Zuo, C. B., and Wu, W. D. (2011). Sediment Transport Following Water Transfer from Yangtze River to Taihu Basin. Water Sci. Eng. 4 (04), 431–444. doi:10.3882/j.issn.1674-2370.2011.01.007
Guzman, C. D., Tilahun, S. A., Zegeye, A. D., and Steenhuis, T. S. (2013). Suspended Sediment Concentration-Discharge Relationships in the (Sub-) Humid Ethiopian Highlands. Hydrol. Earth Syst. Sci. 17, 1067–1077. doi:10.5194/hess-17-1067-2013
Hsu, Y.-S., and Cai, J.-F. (2010). Densimetric Monitoring Technique for Suspended-Sediment Concentrations. J. Hydraul. Eng. 136, 67–73. doi:10.1061/(asce)hy.1943-7900.0000132
Huang, Y.-G., Yang, H.-F., Jia, J.-J., Li, P., Zhang, W.-X., Wang, Y. P., et al. (2022). Declines in Suspended Sediment Concentration and Their Geomorphological and Biological Impacts in the Yangtze River Estuary and Adjacent Sea. Estuar. Coast. Shelf Sci. 265, 107708. doi:10.1016/j.ecss.2021.107708
Huang, Y.-G., Yang, H.-F., Wang, Y. P., Jia, J.-J., Wang, Z.-H., Zhu, Q., et al. (2022). Swell-driven Sediment Resuspension in the Yangtze Estuary during Tropical Cyclone Events. Estuar. Coast. Shelf Sci. 267, 107765. doi:10.1016/j.ecss.2022.107765
Kondolf, G. M. (1997). PROFILE: Hungry Water: Effects of Dams and Gravel Mining on River Channels. Environ. Manag. 21 (4), 533–551. doi:10.1007/s002679900048
Li, P., Yang, S. L., Milliman, J. D., Xu, K. H., Qin, W. H., Wu, C. S., et al. (2012). Spatial, Temporal, and Human-Induced Variations in Suspended Sediment Concentration in the Surface Waters of the Yangtze Estuary and Adjacent Coastal Areas. Estuaries Coasts 35 (5), 1316–1327. doi:10.1007/s12237-012-9523-x
Li, S. S., Dai, Z. J., Mei, X. F., Hu, H., Wei, W., and Gao, J. J. (2016). Dramatic Variations in Water Discharge and Sediment Load from Nanliu River (China) to the Beibu Gulf during 1960s–2013. J. Quat. Int. 440, 12–13. doi:10.1016/j.quaint.2016.02.065
Li, J., Chen, X., Townend, I., Shi, B., Du, J., Gao, J., et al. (2021). A Comparison Study on the Sediment Flocculation Process between a Bare Tidal Flat and a Clam Aquaculture Mudflat: The Important Role of Sediment Concentration and Biological Processes. Mar. Geol. 434, 106443. doi:10.1016/j.margeo.2021.106443
Matos, J. P., Hassan, M. A., Lu, X. X., and Franca, M. J. (2018). Probabilistic Prediction and Forecast of Daily Suspended Sediment Concentration on the Upper Yangtze River. J. Geophys. Res. Earth Surf. 123, 1982–2003. doi:10.1029/2017jf004240
Meade, R. H., and Moody, J. A. (2010). Causes for the Decline of Suspended Sediment Discharge in the Mississippi River System, 1940-2007. Hydrol. Process. 24, 35–49. doi:10.1002/hyp.7477
Montanher, O. C., Novo, E. M. L. d. M., and Souza Filho, E. E. d. (2018). Temporal Trend of the Suspended Sediment Transport of the Amazon River (1984-2016). Hydrol. Sci. J. 63, 1901–1912. doi:10.1080/02626667.2018.1546387
Moskalski, S., and Torre, R. (2012). Influences of Tides, Weather, and Discharge on Suspended Sediment Concentration. Cont. Shelf Res. 37, 36–45. doi:10.1016/j.csr.2012.01.015
Mouri, G., Ros, F. C., and Chalov, S. (2014). Characteristics of Suspended Sediment and River Discharge during the Beginning of Snowmelt in Volcanically Active Mountainous Environments. Geomorphology 213, 266–276. doi:10.1016/j.geomorph.2014.02.001
Müller, G., and Förstner, U. (1968). General Relationship between Suspended Sediment Concentration and Water Discharge in the Alpenrhein and Some Other Rivers. Nature 217 (5125), 244–245.
Nadal, E. R., Latron, J., Martí, B. C., and Regüés, D. (2007). Temporal Distribution of Suspended Sediment Transport in a Humid Mediterranean Badland Area: The Araguás Catchment, Central Pyrenees. J. Geomorphol. 97, 601–616. doi:10.1016/j.geomorph.2007.09.009
Ramalingam, S., and Chandra, V. (2019). Experimental Investigation of Water Temperature Influence on Suspended Sediment Concentration. Environ. Process. 6, 511–523. doi:10.1007/s40710-019-00371-0
Shams, S., Ratnayake, U., Abdul Rahman, E. K., and Alimin, A. A. (2020). Analysis of Sediment Load under Combined Effect of Rainfall and Flow. IOP Conf. Ser. Earth Environ. Sci. 476, 012111. doi:10.1088/1755-1315/476/1/012111
Shi, B. W., Yang, S. L., Wang, Y. P., Li, G. C., Li, M. L., Li, P., et al. (2017). Role of Wind in Erosion-Accretion Cycles on an Estuarine Mudflat. J. Geophys. Res. Oceans 122 (1), 193–206. doi:10.1002/2016jc011902
Syvitski, J. P. M., Vörösmarty, C. J., kettner, A. J., and Green, P. (2005). Impact of Humans on the Flux of Terrestrial Sediment to the Global Coastal Ocean. Science 308, 376–380. doi:10.1126/science.1109454
Tang, J., Wang, Y. P., Zhu, Q., Jia, J., Xiong, J., Cheng, P., et al. (2019). Winter Storms Induced High Suspended Sediment Concentration along the North Offshore Seabed of the Changjiang Estuary. Estuar. Coast. Shelf Sci. 228, 106351. doi:10.1016/j.ecss.2019.106351
Tang, R., Dai, Z., Zhou, X., and Li, S. (2021). Tropical Cyclone-Induced Water and Suspended Sediment Discharge Delivered by Mountainous Rivers into the Beibu Gulf, South china. Geomorphology 389, 107844. doi:10.1016/j.geomorph.2021.107844
Tang, R. G., Shen, F., Ge, J. Z., Yang, S. L., and Gao, W. L. (2021). Investigating Typhoon Impact on SSC through Hourly Satellite and Real-Time Field Observations: A Case Study of the Yangtze Estuary. J. Cont. Shelf Res. 224, 104475. doi:10.1016/j.csr.2021.104475
Tramblay, Y., St-Hilaire, A., and Ouarda, T. B. M. J. (2008). Frequency Analysis of Maximum Annual Suspended Sediment Concentrations in North America. J. Water Energy Abstr. 18, 12–13. doi:10.1623/hysj.53.1.236
van Maren, D. S., Oost, A. P., Wang, Z. B., and Vos, P. C. (2016). The Effect of Land Reclamations and Sediment Extraction on the Suspended Sediment Concentration in the Ems Estuary. Mar. Geol. 376, 147–157. doi:10.1016/j.margeo.2016.03.007
Walling, D. E., and Fang, D. (2003). Recent Trends in the Suspended Sediment Loads of the World's Rivers. Glob. Planet. Change 39, 111–126. doi:10.1016/s0921-8181(03)00020-1
Wang, H., Bi, N., Saito, Y., Wang, Y., Sun, X., Zhang, J., et al. (2010). Recent Changes in Sediment Delivery by the Huanghe (Yellow River) to the Sea: Causes and Environmental Implications in its Estuary. J. Hydrol. 391, 302–313. doi:10.1016/j.jhydrol.2010.07.030
Wang, C., Dai, S. B., Ran, L. S., Jiang, L., Li, W. T., and Mischke, S. (2015). Contribution of River Mouth Reach to Sediment Load of the Yangtze River. Adv. Meteorol. 2015, 1–9. doi:10.1155/2015/415058
Wei, L., Shen, F., He, Q., Cao, F., Zhao, H., and Li, M. (2021). Changes in Suspended Sediments in the Yangtze River Estuary from 1984 to 2020: Responses to Basin and Estuarine Engineering Constructions. J. Sci. Total Environ. 805, 150381. doi:10.1016/j.scitotenv.2021.150381
Wu, C. S., Yang, S. L., and Lei, Y.-p. (2012). Quantifying the Anthropogenic and Climatic Impacts on Water Discharge and Sediment Load in the Pearl River (Zhujiang),China (1954-2009). J. Hydrol. 452-453, 190–204. doi:10.1016/j.jhydrol.2012.05.064
Wu, C., Ji, C., Shi, B., Wang, Y., Gao, J., Yang, Y., et al. (2019). The Impact of Climate Change and Human Activities on Streamflow and Sediment Load in the Pearl River Basin. Int. J. Sedim. Res. 34, 307–321. doi:10.1016/j.ijsrc.2019.01.002
Yang, S.-l., Zhao, Q.-y., and Belkin, I. M. (2002). Temporal Variation in the Sediment Load of the Yangtze River and the Influences of Human Activities. J. Hydrol. 263, 56–71. doi:10.1016/s0022-1694(02)00028-8
Yang, S. L., Zhang, J., Zhu, J., Smith, J. P., Dai, S. B., Gao, A., et al. (2005). Impact of Dams on Yangtze River Sediment Supply to the Sea and Delta Intertidal Wetland Response. J. Geophys. Res. 110 (1-12), F03006. doi:10.1029/2004jf000271
Yang, S. L., Luo, X., Temmerman, S., Kirwan, M., Bouma, T., Xu, K., et al. (2020). Role of Delta‐front Erosion in Sustaining Salt Marshes under Sea‐level Rise and Fluvial Sediment Decline. Limnol. Oceanogr. 65 (9), 1990–2009. doi:10.1002/lno.11432
Yang, S. L., Xu, K. H., Milliman, J. D., Yang, H. F., and Wu, C. S. (2015). Decline of Yangtze River Water and Sediment Discharge: Impact from Natural and Anthropogenic Changes. Sci. Rep. 5, 12581. doi:10.1038/srep12581
Yang, Y.-P., Zhang, M.-J., Li, Y.-T., and Zhang, W. (2015). The Variations of Suspended Sediment Concentration in Yangtze River Estuary. J. Hydrodyn. 27, 845–856. doi:10.1016/s1001-6058(15)60547-9
Zhang, X., Hu, F., Zhang, J., and Tang, D. (2014). Northward Drift of Suspended Sediments in the Yangtze Estuary in Spring. Int. J. Remote Sens. 35, 4114–4126. doi:10.1080/01431161.2014.916455
Zhang, Y., Wang, P., Wu, B., and Hou, S. (2015). An Experimental Study of Fluvial Processes at Asymmetrical River Confluences with Hyperconcentrated Tributary Flows. Geomorphology 230, 26–36. doi:10.1016/j.geomorph.2014.11.001
Zhang, J., Zhang, X., Li, R., Chen, L., and Lin, P. (2017). Did Streamflow or Suspended Sediment Concentration Changes Reduce Sediment Load in the Middle Reaches of the Yellow River? J. Hydrol. 546, 357–369. doi:10.1016/j.jhydrol.2017.01.002
Keywords: suspended sediment concentration, tropical cyclone, human activities, nanliu river, beibu gulf
Citation: Li S, Yang X, Huang H, Liang X, Wang R and Feng B (2022) Variations in the Suspended Sediment Concentration in Mountain-Type Rivers Flowing Into the Sea in the Past 60 years—Taking Nanliu River in Beibu Gulf as an Example. Front. Earth Sci. 10:913022. doi: 10.3389/feart.2022.913022
Received: 06 April 2022; Accepted: 08 June 2022;
Published: 30 June 2022.
Edited by:
Xiaolei Liu, Ocean University of China, ChinaReviewed by:
Benwei Shi, East China Normal University, ChinaXiao Wu, Ocean University of China, China
Copyright © 2022 Li, Yang, Huang, Liang, Wang and Feng. This is an open-access article distributed under the terms of the Creative Commons Attribution License (CC BY). The use, distribution or reproduction in other forums is permitted, provided the original author(s) and the copyright owner(s) are credited and that the original publication in this journal is cited, in accordance with accepted academic practice. No use, distribution or reproduction is permitted which does not comply with these terms.
*Correspondence: Hu Huang, mrhuanghu@126.com