- 1Fujian Provincial Key Laboratory of Water Cycling and Eco-Geological Processes, Xiamen, China
- 2Institute of Hydrogeology and Environmental Geology, Chinese Academy of Geological Sciences, Shijiazhuang, China
- 3Key Laboratory of Groundwater Contamination and Remediation of Hebei Province and China Geological Survey, Shijiazhuang, China
- 4Faculty of Geosciences and Environmental Engineering, Southwest Jiaotong University, Chengdu, China
In recent years, to alleviate the decline in groundwater levels, extensive restrictions on groundwater pumping have been implemented in the North China Plain (NCP). In September 2018, a large-scale ecological water replenishment project was executed involving 22 rivers and lakes. How to adjust the layout of reduction on groundwater pumping within the context of ecological water replenishment is a key issue to be addressed in the study of groundwater level recovery in the NCP. This study adopted the Juma River Plain in Baoding city as a case study, established a numerical model of river replenishment of groundwater, predicted groundwater level changes over the next 15 years (2021–2035) and quantitatively calculated the impact of river replenishment on groundwater levels. To achieve the goal of an overall groundwater balance by 2035, a suitable groundwater pumping restriction scenario was defined based on the impact of river replenishment on groundwater levels. The results indicated that by 2035, the relative rise in groundwater levels attributed to river replenishment and restrictions on groundwater pumping could reach 3.51 and 2.28 m, respectively. River replenishment significantly impacts groundwater levels, especially those near the river. Under the current groundwater exploitation conditions, river replenishment could ensure groundwater level recovery near the river, which accounts for 15% of the total study area. The goal of an overall groundwater balance by 2035 could be achieved if restrictions on groundwater pumping were superimposed, with an average annual reduction of 56 million m3. This study provides valuable insights into groundwater management across the NCP. The proposed methods are useful for the management of other depleted aquifers recharged via ecological water replenishment.
1 Introduction
Groundwater is an essential source of water for agricultural, industrial, and environmental uses and the drinking water supply due to its generally good quality and widespread occurrence (Cao et al., 2013). However, groundwater depletion has extensively occurred and become a global hotspot due to the unremitting and unreasonable overexploitation in many regions (Aeschbach-Hertig and Gleeson, 2012; Gleeson et al., 2012; Feng et al., 2013; Huang et al., 2015; Alin et al., 2017), such as North Africa (Shah et al., 2003), Northwest India (Rodell et al., 2018), the Middle East (Voss et al., 2013), and the North China Plain (NCP) (Xiao et al., 2022a; Xiao et al., 2022b). The NCP occurs among the most severe regions, with groundwater levels declining as fast as 1 m/a between 1974 and 2000 (Qiu, 2010). This region has begun to experience adverse water shortages, and groundwater overdraft has led to environmental problems such as groundwater drawdown cones, seawater intrusion, groundwater quality deterioration and subsidence, as the water demand has exceeded the natural renewable supply (Zheng et al., 2010; Nakayama, 2011; Zhu et al., 2013; Xiao et al., 2021).
The implementation of the South-to-North Water Diversion Project (SNWD), where water is diverted from the humid south to the arid north, has provided an opportunity for groundwater management in the NCP. To alleviate groundwater depletion, a groundwater extraction restriction policy has been adopted in SNWD water-receiving areas across the NCP since 2014, and groundwater withdrawal has been reduced via the adoption of water conservation in various fields, water source replacement, and adjustment of crop cultivation structures (Chen et al., 2021). By the end of 2018, the average annual groundwater overexploitation level in the NCP decreased to 3.19 km3, a 33% reduction below 2011–2013 levels, and several issues including groundwater drawdown cones and subsidence were alleviated (Li et al., 2020). To restore river ecosystems and further increase the intensity of restrictions on groundwater pumping, the Hebei government initiated the adoption of ecological water replenishment in August 2018, which constitutes the largest ecological replenishment project in China. Three typical rivers in the NCP, the Hutuo River, Fuyang River and Juma River, are replenished by the SNWD and water diversion from the Yellow River, Luan River and local reservoirs (Chen et al., 2020). Based on groundwater level monitoring data, the average groundwater level recovery within 10 km on both sides of these rivers in February 2019 reached 1.62 m compared to pre-replenishment period levels (Chen et al., 2021). Several rivers and lakes were recharged in 2020, and river replenishment has become an important method to restore groundwater levels in the NCP.
Many scholars have conducted studies on groundwater level recovery in the NCP. Cao et al. (2013) applied a groundwater model to evaluate the impact of several alternatives, including restrictions on groundwater pumping, aquifer recharge management, water use efficiency enhancement, and brackish water use, on groundwater in the NCP and indicated that the combination of these strategies could be employed to recover groundwater storage by 50 km3 over a 15-year period. Li et al. (2017) combined climate and water-diversion scenarios to predict groundwater levels in the Beijing–Tianjin–Hebei Plain and estimated that shallow groundwater levels could rise by a maximum value of 17 m over the next 40 years (2011–2050) when considering scenarios combining climate change and restrictions on groundwater pumping. Shu et al. (2012) integrated dynamic and distributed hydrological data to evaluate the spatiotemporal implications of various water use and management options in the NCP and concluded that deficit irrigation of winter wheat could reverse groundwater decline. Sun et al. (2021) adopted machine learning models to describe dynamic changes in streamflow and groundwater levels in response to ecological water replenishment of the Yongding River in the Beijing Plain and estimated that the river could recharge the aquifer by 170 million m3.
In 2019, the Ministry of Water Resources of China and Hebei Province promulgated an action plan and implementation opinions for comprehensive management of groundwater overexploitation. It was clearly stated that the Beijing–Tianjin–Hebei region in the NCP is the focus of treatment, through river replenishment and restrictions on groundwater pumping, to achieve a provincial exploitation–replenishment balance by 2022, meaning that the total exploitation equals the total replenishment in the region, and to accomplish a complete exploitation–replenishment balance by 2035, that is, exploitation equals replenishment overall in the region (The Ministry of Water Resources of the People’s Republic of China, 2019; The People’s Government of Hebei Province, 2019). Previous studies on groundwater recovery in the NCP could help to achieve groundwater balance. However, these studies mainly focused on the impact on groundwater levels of water diversion (Liu et al., 2008; Zhang et al., 2019), climate change (Kang and Eltahir, 2018; Long et al., 2020), restrictions on extraction (Cao et al., 2013; Li and Ren, 2019) and crop water consumption (Sun et al., 2006; Li and Ren, 2019) but did not consider the impact of the implementation of the above river ecological replenishment project, especially on groundwater levels near the river. There are a few studies on the relationship between ecological replenishment and the groundwater regime in the NCP. However, these studies basically applied certain methods, including the genetic algorithm (Hao et al., 2018), water resource allocation (Yan et al., 2018) and machine learning (Sun et al., 2021), to propose an optimal river replenishment plan but did not elucidate in detail the impact of river replenishment on groundwater levels. In addition, previous studies based only on changes in the total groundwater storage and average groundwater level across the region compared the groundwater recovery between different simulated scenarios and thus selected the optimal scenario facilitating a groundwater balance. Obviously, these studies could only help to achieve a provincial exploitation–replenishment balance, that is, these studies could contribute to the achievement of the groundwater balance goal by 2022 but could not ensure that a complete exploitation–replenishment balance will be achieved by 2035.
River replenishment and groundwater pumping restriction are currently the main measures to restore groundwater storage in the NCP. The implementation of river replenishment not only impacts the overall groundwater balance but also impacts groundwater level rise near the river. These impacts of river replenishment should be considered in the development of restriction policies on groundwater pumping to more effectively achieve groundwater level recovery. The groundwater numerical simulation method is clearly appropriate for the assessment of sustainable water use given the complexity and heterogeneity of aquifer systems and can assist in the formulation of groundwater regulation and storage management policies (Wolfgang et al., 2003; Hao et al., 2012). Several numerical models have been constructed for sustainable groundwater management in the NCP (Hu et al., 2010; Zhang and Li, 2014; Zhang et al., 2018). General groundwater models often have difficulty in precisely simulating local groundwater level changes near rivers with large groundwater level changes. In this study, we used local grid refinement to finely characterise groundwater level changes near the river, and Cui and Hao (2020) have successfully applied this method to numerical simulations with ecological water replenishment of rivers. This study was conducted in the Juma River Plain, which flows through the Juma River, one of three ecological replenishment rivers. A numerical groundwater model was applied to simulate and predict the impact of river replenishment on groundwater levels, and a groundwater pumping restriction scenario was subsequently established based on the impact of river replenishment to achieve the goal of an overall groundwater balance by 2035.
The novelty of this study is that a numerical model of river replenishment of groundwater was developed to analyze the impact of river replenishment on groundwater levels, and an appropriate groundwater pumping restriction plan was determined to achieve an overall groundwater balance by 2035. The objective of the study was to quantify the impact of river replenishment on groundwater levels and to propose an optimal groundwater pumping restriction scenario that could minimize the extraction restriction volume while meeting the goal of groundwater balance by 2035.
2 Study area and hydrogeological setting
The Juma River is the only perennial river in Hebei Province constantly flowing throughout the year, with a total length of 69 km and an average bed width ranging from 100 to 200 m (Yao and Lu, 2017). Due to many dam constructions upstream, the runoff volume decreased to an extremely low level. The Juma River Plain occurs in the piedmont plain of the Taihang Mountains, covering an area of approximately 3,300 km2 and ranging in elevation from 15 to 85 m (Figure 1A). The climate in the Juma River Plain is a continental semiarid climate, with a mean annual temperature of 10.7°C and an annual average precipitation of approximately 517–581 mm (Ding et al., 2013). In addition, precipitation is unevenly distributed throughout the year, and rainfall from June to September accounts for 70%–80% of the total annual precipitation amount (Yu et al., 2012).
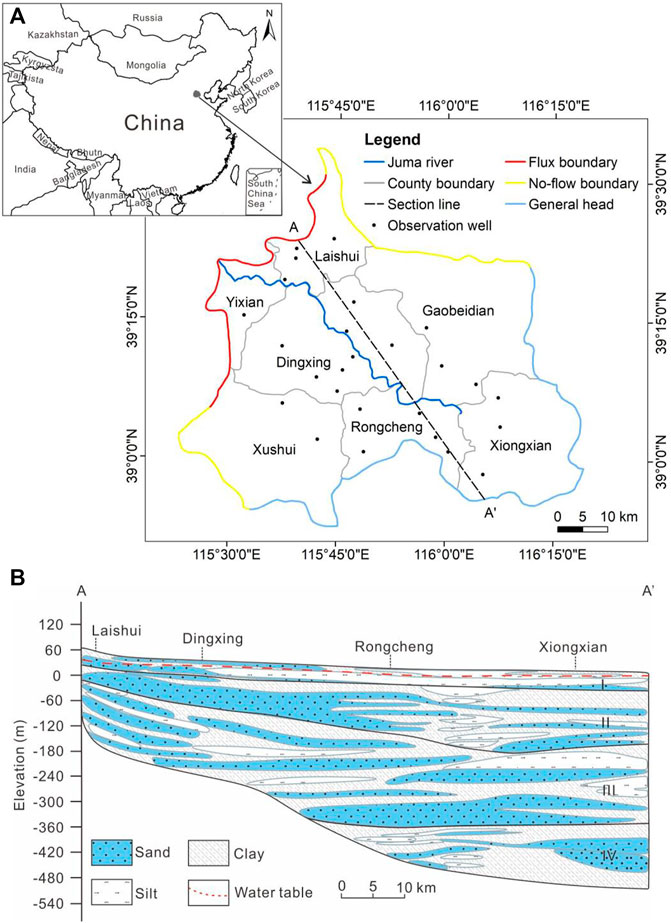
FIGURE 1. (A) Location and model boundaries of the study area; (B) hydrogeological section of the study area.
The stratigraphy in the study area mainly comprises unconsolidated Quaternary sediments where most of the groundwater occurs. The aquifer structure varies from a single aquifer comprising gravel particles and pebbles in the upper parts of the piedmont fan in the northwest to multiple aquifers composed of sand, silt and clay in the southeast. The groundwater system can be divided into four aquifer groups (I, II, III, and IV) based on the stratigraphy (Figure 1B). Aquifer groups I and II represent shallow aquifers, whereas groups III and IV represent deep aquifers.
Recharge primarily occurs through precipitation infiltration, lateral flow in the mountains and return flow from irrigation. Groundwater pumping is currently the main discharge mode from the aquifer system. The water table is relatively deep, and there essentially occurs no phreatic evaporation. Groundwater flows from northwest to southeast under natural conditions.
3 Methods
3.1 Groundwater flow model
A three-dimensional heterogeneous anisotropic transient flow model was developed comprising two aquifers: the top aquifer is a phreatic aquifer, and another aquifer is a confined aquifer. The phreatic aquifer comprised aquifer groups I and II, and the confined aquifer comprised aquifer groups III and IV.
The model domain was discretized with the quadtree refinement method in MODFLOW–USG, which achieves a high simulation precision in modeling river recharge to aquifers (Cui and Hao, 2020). The finest grid was 250 m × 250 m, and the cell size gradually increased to 500 m × 500 m away from both sides of the river. The simulation period was 20 years (2001–2020), and the prediction period was 15 years (2021–2035), with a stress period of 15 days. During each stress period, constant average hydrological conditions were assumed.
The mountain front in the northwest was defined as an inflow boundary because the region experiences significant water exchange with the outside region of the study area, and the flux rate from the northwest boundary was determined based on the profiling method of previous studies (Shao et al., 2013). The northern and southwestern boundaries were defined as no-flow boundaries because the flow lines are generally parallel to these boundaries under present conditions (Figure 1A). The eastern and southern boundaries were treated as general head boundaries, and groundwater heads were obtained from measured data.
The main recharge source of groundwater in the study area includes rainfall infiltration, irrigation infiltration, and river replenishment, whereas the main discharge mode of groundwater is exploitation. The amount of river replenishment was provided by the Ministry of Water Resources of the People’s Republic of China, and other recharge and discharge volumes of aquifers were collected from previous studies and investigations (Zhang and Che, 2017; Zhang et al., 2017). The amount of river replenishment is the average amount over the replenishment period (September 2018–December 2020) during the prediction period, and the annual replenishment volume reaches 101 million m3. Due to the implementation of restriction policies on groundwater pumping, the amount of groundwater extracted in Hebei province has been reduced by 4.35 billion m3 by 2021. Therefore, the exploitation amount during the projection period was set to the same amount as that in 2020. The values of the other source–sink items, such as rainfall and irrigation, are multiyear averages of the corresponding items over the simulation period.
The model was calibrated against data retrieved from 26 observation wells in the vicinity of the river (Figure 1A). Among all the observation wells, a comparison of the simulated and observed water levels in six observation wells is shown in Figure 2. The results demonstrated that the model simulations suitably reflected the actual features of the groundwater regime. The initial aquifer parameter values, including hydraulic conductivity and storage parameters pertaining to groundwater flow, were zoned and assigned based on sediment features and results obtained from a previous investigation (Zhang et al., 2012). These parameters were then manually adjusted in the calibration process. The hydraulic conductivity values ranged from 2 to 130 m/d for the phreatic aquifer and 2–50 m/d for the confined aquifer.
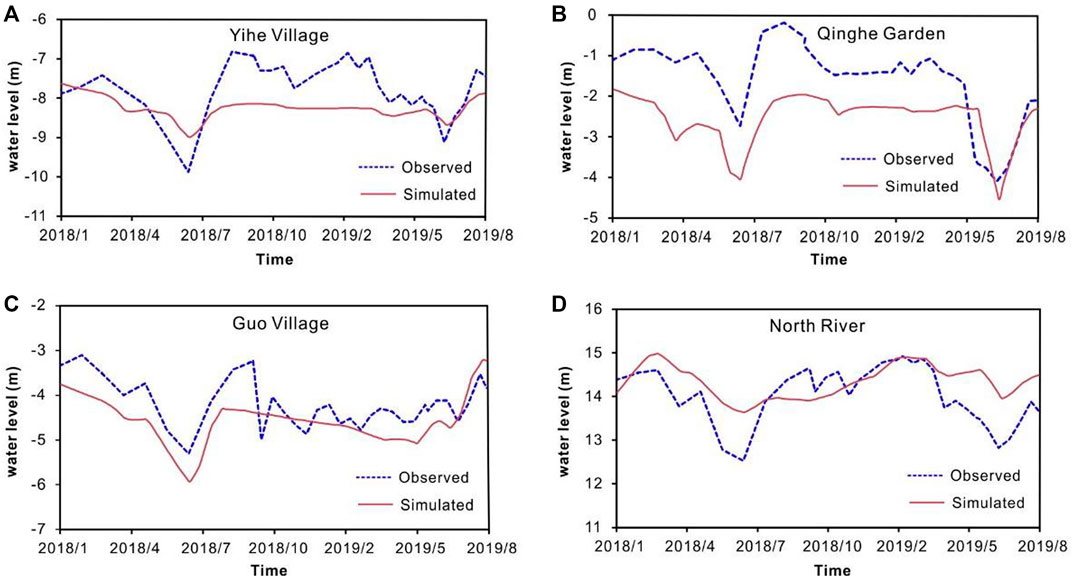
FIGURE 2. Comparison of the observed and simulated groundwater levels in observation wells. (A) Observation well in Yihe Village, (B) Observation well in Qinghe Garden, (C) Observation well in Guo Village, (D) Observation well in North River.
3.2 Relative and absolute groundwater level changes
The relative groundwater level change (RGLC) refers to the difference between groundwater levels altered as a result of a change in certain conditions and groundwater levels under the original conditions. This quantity can be employed to indicate the extent to which changes in conditions affect groundwater levels. In this study, the RGLC with river and non–river replenishment, that is, the groundwater level with river replenishment minus that without river replenishment over the same period, was applied to represent the degree of the impact of river replenishment on groundwater levels. The parameters of two models describing river and non–river replenishment remained constant, including recharge and discharge items and hydrogeological parameters, except for the differences with and without river replenishment.
The absolute groundwater level change (AGLC) refers to the difference in groundwater levels at different times in the same model. This quantity can be employed to indicate the magnitude of groundwater level change over a given time interval. In this study, the AGLC was represented as the current water level minus the previous level, e.g., the AGLC between the end of 2034 and the end of 2035 is the groundwater level in December 2035 minus that in December 2034. The water level in 2035 increases overall if the AGLC values are greater than zero at every location in the study area. The method of the AGLC can therefore be applied to determine the overall state of groundwater balance within a given time interval.
3.3 Zoning for restriction on groundwater pumping
3.3.1 Restriction zone
Prior to the implementation of the ecological water replenishment project, the overall groundwater levels in the study area decreased at a rate of 0–1 m/a. After project implementation, there was a considerable impact on groundwater levels near the river, with some rebound in groundwater levels in the vicinity of the river according to the obtained monitoring data. Considering that water levels recovered in certain areas near the river under the influence of river replenishment, it is only necessary to manage those areas where groundwater levels decreased to minimize the amount of groundwater pumping restriction. In this study, we characterised the achievement of overall groundwater balance by the fact that groundwater levels in each small district (the smallest district is a town in the study area) did not decline any further. In order to achieve the goal of overall groundwater balance in 2035, the AGLC values between 2034 and 2035 required to be greater than 0 in each town in the study area. Therefore, the areas where the AGLC value between 2034 and 2035 is less than zero were set as the groundwater pumping restriction zone, in which the extraction amount should be reduced to achieve the goal of overall groundwater balance in 2035.
The SNWD allows most of the urban areas in Hebei Province to use diverted water as a replacement of groundwater for industrial and domestic purposes, thereby reducing groundwater withdrawal. Given this situation, the urban areas in the study area were identified, and the groundwater pumping restriction volume could be concentrated more in these areas if necessary to ensure that groundwater restrictions in adjacent rural areas (town areas) remained within reasonable limits.
3.3.2 Restriction volume
The total groundwater pumping restriction volume can be preliminarily calculated with the equation of the relationship between the changes in groundwater level, area and specific yield.
Subscript i denotes the zone where the groundwater level declines, n is the number of zones where the groundwater level drops, H is the average value of the groundwater level decline, S is the area of the groundwater level decline, and μ is the specific yield.
Agricultural water use in the NCP accounts for 74.5%–76.6% of the groundwater extraction volume (Zhang et al., 2009), and restrictions on groundwater pumping could lead to a decrease in agricultural irrigation, which in turn could result in a reduction in crop yields. Wheat is the major crop in the NCP and is also a highly water-intensive crop, accounting for 70% of the total agricultural water use among all irrigated crops (Zhang et al., 2016). Given the impact of groundwater extraction restriction on crops, the groundwater pumping restriction scenario developed in this study was designed to ensure that the maximum reduction in crop yield did not exceed 10%. Sun et al. (2006) demonstrated that a 29.5% reduction in agricultural irrigation in the NCP was associated with a 19.5% reduction in the wheat yield. Based on this research, it was assumed that the reduction in wheat yield is linearly related to the reduction in irrigation within this interval. Notably, a 1% reduction in irrigation reduced the wheat yield by 0.66% when the reduction in the irrigation amount remained below 29.5%. It was also assumed that in rural (town) areas, all restrictions on groundwater pumping occurred in the agricultural sector, while other water uses, such as domestic water, experienced no restrictions, and all agricultural water was employed for wheat irrigation. Therefore, the degree of groundwater pumping restriction (the ratio of restriction to exploitation) in rural areas should be lower than 15% to ensure that the reduction in wheat yield remained below 10%. In addition, the replacement of irrigation equipment with water-saving equipment has been strongly promoted in town areas in Hebei Province, and the degree of groundwater pumping restriction could increase. In urban areas, diverted water can serve as a replacement of groundwater for the water supply as a result of SNWD development, therefore assuming that groundwater within these areas could be considered subject to unrestricted extraction reduction, that is, the maximum restriction amount could match the amount of groundwater pumping set in the model.
4 Results and discussion
4.1 Effect of river replenishment on groundwater levels
4.1.1 Flow field change
Because shallow aquifers are more sensitive to river replenishment than deep aquifers, only shallow water levels were analyzed. A comparison of the shallow groundwater flow fields with and without river replenishment in 2020 and 2035 is shown in Figure 3. In terms of the groundwater contours of flow field, river replenishment created water concentration areas along the river, resulting in a significant increase in groundwater levels near the river. In 2020, the impact of river replenishment was less extensive, causing only a rise in groundwater levels very close to the river, whereas the water levels (with and without river replenishment) overlapped in other areas away from the river. With the operation of the developed model, the morphological differences in groundwater contours between the two flow fields (with and without river replenishment) obviously increased. The water concentration areas along the river expanded, and the morphological differences gradually spread from the center of the river to both sides. By 2035, the morphological differences were manifested at the northern boundary, where groundwater levels with river replenishment were higher than those without river replenishment in most of the study area, and the impact of river replenishment on the overall groundwater levels increased.
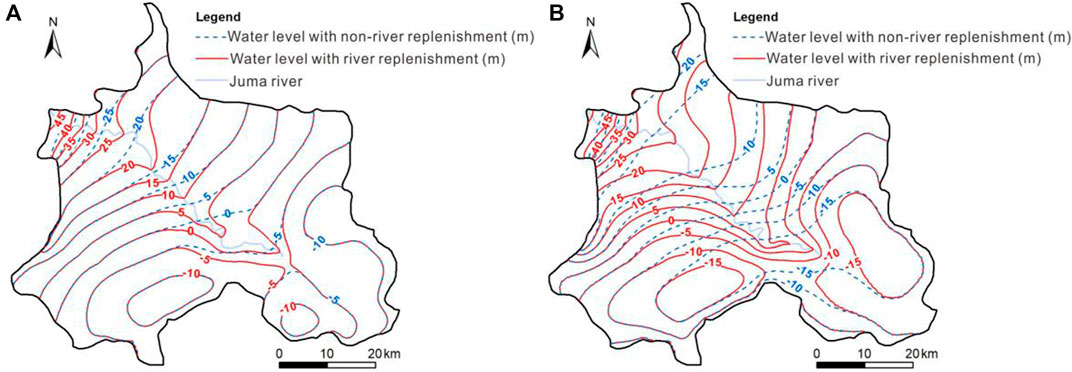
FIGURE 3. Comparison of the flow fields with and without river replenishment: (A) at the end of 2020 and (B) at the end of 2035.
4.1.2 RGLC and changes in the average groundwater level
The RGLC was calculated at the end of 2020 and at the end of 2035, and areas were identified where the RGLC value exceeded 1 m, as shown in Figure 4. In 2020, the maximum RGLC value was 8 m, and the area with a relative rise of more than 1 m reached 563.36 km2. By 2035, the RGLC value could reach a maximum value of 23 m, and this value could exceed 1 m in an area of 1,722.68 km2, which accounts for more than half the total study area (53%). In addition, Figure 4 shows that the lower reaches of the river were more affected by river replenishment, with a relatively greater increase in groundwater levels, whereas the effect decreased toward the upper reaches. This occurs because the lithology in the downstream area mostly includes medium and fine sandy, which is finer grained than the lithology in the upstream area and generates a relatively lower specific yield, thus causing groundwater levels to be more sensitive to river replenishment.
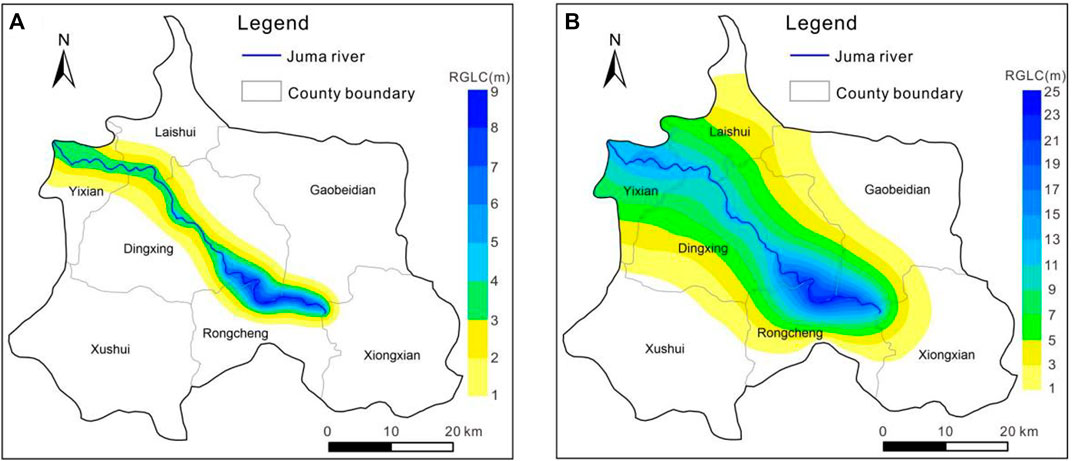
FIGURE 4. Comparison of the RGLC with and without river replenishment: (A) at the end of 2020 and (B) at the end of 2035.
The average groundwater level visually reflects the total groundwater storage in the area. The impact of river replenishment on groundwater recovery was investigated by comparing changes in the average groundwater level with and without river replenishment during different periods. Table 1 provides changes in average groundwater level across the shallow aquifer with and without river replenishment in 2020 and 2035. Under the realistic simulation scenario, after more than 2 years of river replenishment (from September 2018 to December 2020), the average relative rise in groundwater level due to river replenishment was 0.55 m. Under the predicted scenario, all source and sink terms, such as the amount of river replenishment, extraction, and rainfall, remained constant every year, and the average relative rise in the water level due to river replenishment could reach 3.51 m over 15 years (from January 2021 to December 2035), with an average relative rise of 0.23 m per year.
At the prediction stage, the average groundwater levels decreased at a rate of 0.44 m/a assuming no further river replenishment after 2020, whereas the levels could decrease at a rate of 0.21 m/a within the context of river replenishment. This indicates that river replenishment decreased the rate of groundwater level decline, which is conducive to groundwater level recovery. However, the total groundwater storage could still decrease, even under consistent river replenishment, indicating that the magnitude of river replenishment attained under current projection conditions could not achieve complete groundwater recovery over the next 15 years. It was estimated that if the amount of river replenishment during the prediction period was doubled, the regional exploitation–replenishment balance could basically be achieved, that is, the total groundwater storage volume would no longer decrease. As such, only a provincial extraction–replenishment balance (the groundwater balance goal by 2022) could be achieved, not a complete extraction–replenishment balance (the groundwater balance goal by 2035). Therefore, reasonable groundwater pumping restrictions will be required to meet the goal of an overall groundwater balance by 2035.
4.2 Scenario and effect of groundwater pumping restriction
4.2.1 Restriction scenario
The AGLC between the end of 2035 and the end of 2034 under river replenishment conditions is shown in Figure 5, with districts where the value was less than zero identified as the groundwater pumping restriction zone. Groundwater levels declined in most districts of the study area, whereas levels rose within a small area near the river. In these districts with rising groundwater levels, the increase in groundwater level due to river replenishment was greater than the decrease due to other source–sink items (mainly groundwater pumping). The area with the most significant area of water level decrease was is located in the west of Xushui, with a maximum decrease of 0.55 m. While the area with the greatest rise in groundwater levels was located in Dingxing, with a maximum rise of 0.05 m. The area with rising groundwater levels reached 484 km2, accounting for approximately 15% of the total study area. Areas where groundwater levels rised as a result of river replenishment have achieved an overall groundwater balance and there is no need to reduce groundwater extraction in these areas. However, in districts with decreasing groundwater levels, the increase in groundwater levels due to river replenishment no longer offset the decrease due to source–sink items such as groundwater pumping. In these areas, that is, the restriction zone, groundwater extraction reduction is necessary if the goal of an overall groundwater balance by 2035 is to be reached.
The districts of the greatest groundwater level decline were concentrated in or near the urban areas of Xiongxian and Xushui, with a maximum decrease of 0.55 m. The flow fields in Figure 3 also indicate that two groundwater depression cones were located approximately near these two areas. Therefore, more intensive groundwater pumping restrictions are required in the urban areas of Xiongxian and Xushui to restore groundwater levels. When setting the restriction volume, it is possible to allocate a larger volume to these two urban areas, considering the availability of water replacement within urban areas, to ensure that the restriction degree remains lower than 15% in other rural areas (town areas) by the time the groundwater balance goal of 2035 is reached. The groundwater pumping restriction volume was calculated according to the equation in section 3.2.2, with the restriction amount increasing by an equal proportion each year over the prediction period (2021–2035), that is, the amount of groundwater pumping was reduced by an equal proportion each year.
If the restriction volume calculated with the equation in section 3.2.2 was directly applied in the prediction model, the restrictions in the rural areas adjacent to the urban areas of Xushui and Xiongxian could exceed the maximum limit. Therefore, a larger restriction amount was allocated to these two urban areas, considering that urban areas could be supplied by diverted water as a replacement of groundwater to ensure that the restriction degree in the town areas remained lower than 15%. It was finally determined that when the minimum total volume of groundwater pumping restriction reached 0.84 km3, that is, the average annual reduction volume reached 56 million m3, the goal of an overall groundwater balance could be achieved by 2035.
However, if the effect of river replenishment was not considered, that is, no river replenishment of groundwater occurs in the prediction model (2021–2035), both the area and amount of restriction could increase. It was calculated that to achieve the goal of an overall groundwater balance by 2035 without river replenishment, groundwater extraction would have to be reduced across almost the entire study area, with a total volume of 1.22 km3 and an average annual volume of 81 million m3, which is 25 million m3 greater than the volume under river replenishment conditions.
4.2.2 Restriction effect
The AGLC between December 2034 and December 2035 after the implementation of groundwater pumping restrictions is shown in Figure 6. The groundwater levels in the study area rose overall, but the rise was not significant. The greatest rise in groundwater levels was near the lower reaches of the river, with the largest rise reaching 0.15 m. While other areas away from the river showed rises of less than 0.1 m, with the smallest rise of 0.01 m, located within Xiongxian. This indicates that the groundwater levels in 2035 could no longer decline anywhere in the study area under this restriction scenario, which suggests that the groundwater balance goal by 2035 could be achieved. The overall average groundwater level in 2035 reached 3.23 m when groundwater pumping restrictions were superimposed, which is 2.28 m greater than that without restriction during the same period.
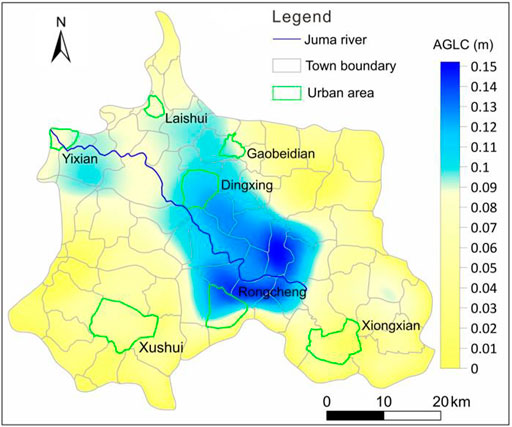
FIGURE 6. AGLC between the end of 2035 and the end of 2034 under the condition of groundwater pumping restriction.
The maximum annual degree of groundwater pumping restriction in the rural and urban areas of the study area is shown in Figure 7. In the urban areas, Xushui and Xiongxian exhibited the highest degree of restriction at 55% and 50%, respectively, while Dingxing and Yixian did not require restriction due to river replenishment, and the other three urban areas remained consistent with the original calculated values. In the rural (town) areas, the greatest restriction on groundwater extraction occurred near the urban area of Xushui, with a reduction of 13.4%, which is below the maximum restriction limit (15%). In addition, only three towns near the urban area of Xushui and one town near the urban area of Xiongxian exhibited a restriction degree higher than 10%, while that in the remaining areas occurred within 10%. These results indicate that under this groundwater pumping restriction scenario, extraction reduction slightly affected the crop yield, with the resulting yield reduction not exceeding a maximum value of 8.9%.
Overall, the two counties of Xushui and Rongcheng required the highest degree of groundwater pumping restriction, which in turn reflects the relatively serious groundwater overexploitation issue in both counties and the need for strict groundwater management. In addition to the influence of groundwater extraction in these two counties, another reason is that these counties are farther away from the river and thus less affected by river replenishment, requiring greater groundwater pumping reductions to achieve groundwater balance.
Groundwater pumping restrictions not only facilitated the recovery of the groundwater levels but also facilitated the overall groundwater balance, meeting the government’s groundwater management goal of 2035. The implementation of the SNWD plays a key role in restoring groundwater levels across the NCP by providing additional water supply sources, which in turn reduces groundwater exploitation. Many studies have predicted the impact of groundwater extraction reduction in the NCP or parts of the interior region. With a decrease of 6.0 km3/a in groundwater pumping in the NCP, groundwater levels in piedmont areas, especially in Beijing, could notably recover by 2030, and the rate of groundwater level decrease could decline in the central and coastal plains by 2030 (Cao et al., 2013). In Beijing, the depletion of groundwater storage is decreasing at a rate of 2.8 km3/a after reducing water use for agricultural purposes (Long et al., 2020). The shallow groundwater levels in the NCP could significantly increase due to climate change and groundwater pumping restriction, and the maximum increase in the absolute rise in groundwater levels could reach 6.71 m after 40 years, in which the relative rise in groundwater levels due to groundwater pumping restrictions could reach approximately 7.94 m (Li et al., 2017). In this study, the relative rise in shallow groundwater levels due to 15 consecutive years of restrictions was 2.28 m, which is generally consistent with the above research results and can provide a certain guiding significance for the development of groundwater pumping restriction policies in the future.
5 Conclusion
The impact of river replenishment on groundwater levels over the next 15 years was simulated and calculated with a numerical model and calculation of the RGLC to achieve the goal of groundwater balance by 2035, considering the policies of river replenishment and restrictions on groundwater pumping currently implemented and to be implemented in the long term. Then, based on the influence of river replenishment, other factors were comprehensively considered, including the impact of extraction reduction on crop yields in rural (town) areas and replacement of groundwater withdrawal with diverted water in urban areas, and a suitable groundwater pumping restriction scenario was proposed, combined with calculation of the AGLC. The objective was to achieve a complete exploitation–replenishment balance by 2035 while minimizing the volume of groundwater pumping reduction and thus minimize the impact on human production and livelihood.
River replenishment facilitates groundwater level recovery, particularly in the vicinity of the river, ensuring that groundwater levels in 15% of the total study area could be recovered without extraction reduction. However, under the current replenishment and extraction conditions, the groundwater level only gradually increased in specific areas near the river, while the overall average groundwater water level still decreased at a rate of 0.21 m/a. Therefore, extraction reduction is necessary to achieve the goal of an overall groundwater balance by 2035. Considering that the groundwater levels near the river already increased due to river replenishment, only in those areas with falling water levels should exploitation be reduced. It was determined that with an average annual reduction in the groundwater pumping volume of 56 million m3, a complete exploitation–replenishment balance could be achieved by 2035. The maximum annual degree of groundwater pumping restriction in town areas under this restriction plan was 13.4%, with a maximum reduction in crop yields of 8.9%.
In the study of sustainable groundwater management with ecological water replenishment and extraction restrictions, it is necessary to fully consider the impact of river replenishment on groundwater levels, and appropriate restrictions on groundwater pumping should subsequently be implemented in specific areas to accomplish more effective restriction. This study did not consider the impact of climate change on groundwater levels. The direct impact of climate change on rainfall and the indirect impact on other sources and sinks require further research to be integrated into the groundwater model.
Data availability statement
The original contributions presented in the study are included in the article/supplementary material, further inquiries can be directed to the corresponding author.
Author contributions
WC and QH designed the conceptualization, methodology, data curation, and original draft preparation. YX performed data curation and formal analysis. YZ was responsible for data curation and language. JL and YZ designed the methodology.
Funding
This study was financially sponsored by the Natural Science Foundation of China (Grant Nos. 41702282), and the China Geological Survey Project (Grant Nos. DD20160238 and DD20190303).
Conflict of interest
The authors declare that the research was conducted in the absence of any commercial or financial relationships that could be construed as a potential conflict of interest.
Publisher’s note
All claims expressed in this article are solely those of the authors and do not necessarily represent those of their affiliated organizations, or those of the publisher, the editors and the reviewers. Any product that may be evaluated in this article, or claim that may be made by its manufacturer, is not guaranteed or endorsed by the publisher.
References
Aeschbach-Hertig, W., and Gleeson, T. (2012). Regional strategies for the accelerating global problem of groundwater depletion. Nat. Geosci. 5 (12), 853–861. doi:10.1038/ngeo1617
Alin, C. D., Wada, Y., Kastner, T., and Puma, M. J. (2017). Erratum: Corrigendum: Groundwater depletion embedded in international food trade. Nature 543 (7647), 366. doi:10.1038/nature24664
Cao, G., Zheng, C., Scanlon, B. R., Jie, L., and Li, W. (2013). Use of flow modeling to assess sustainability of groundwater resources in the North China Plain. Water Resour. Res. 49 (1), 159–175. doi:10.1029/2012WR011899
Chen, F., Ding, Y., Li, Y., Li, W., Tang, S., Yu, l., et al. (2020). Thinking of groundwater overdraft restoration in north plain China. s. N. Water Transf. Water Sci. Technol. 018 (002), 191–198. doi:10.13476/j.cnki.nsbdqk.2020.0042
Chen, F., Ding, Y., Tang, S., Ding, L., and Yang, Y. (2021). Practice and effect analysis of river-lake ecological water supplement and groundwater recharge in the North China region. China Water Resour. 7, 36–39.
Cui, W., and Hao, Q. (2020). Comparing Q-tree with nested grids for simulating managed river recharge of groundwater. Water 12 (12), 3516. doi:10.3390/w12123516
Ding, Z., Li, W., and Zhao, Y. (2013). Study on land use change and its driving forces in the Juma River Basin in recent 20 years. Haihe Water Resour. 5 (05), 27–29.
Feng, W., Zhong, M., Lemoine, J.-M. L., Biancale, R., Hsu, H.-T., Xia, J., et al. (2013). Evaluation of groundwater depletion in North China using the Gravity Recovery and Climate Experiment (GRACE) data and ground-based measurements. Water Resour. Res. 49 (4), 2110–2118. doi:10.1002/wrcr.20192
Gleeson, T., Wada, Y., Bierkens, M., and Beek, L. (2012). Water balance of global aquifers revealed by groundwater footprint. Nature 488 (7410), 197–200. doi:10.1038/nature11295
Hao, Q. C., Shao, J. L., Xie, Z. H., and Xing, G. Z. (2012). A Study of the artificial adjustment of groundwater storage of the Yongding River alluvial fan in Beijing. Hydrogeol. Eng. Geol. 39, 12–18. doi:10.1007/s11783-011-0280-z
Hao, Q., Shao, J., Cui, Y., Zhang, Q., and Huang, L. (2018). Optimization of groundwater artificial recharge systems using a genetic algorithm: A case study in beijing, China. Hydrogeol. J. 26, 1749–1761. doi:10.1007/s10040-018-1781-7
Hu, Y., Moiwo, J. P., Yang, Y., Han, S., and Yang, Y. (2010). Agricultural water-saving and sustainable groundwater management in shijiazhuang irrigation district, north China plain. J. Hydrol. X. 393 (3-4), 219–232. doi:10.1016/j.jhydrol.2010.08.017
Huang, Z., Pan, Y., Gong, H., Yeh, J. F., Li, X., Zhou, D., et al. (2015). Subregional‐scale groundwater depletion detected by GRACE for both shallow and deep aquifers in North China Plain. Geophys. Res. Lett. 42 (6), 1791–1799. doi:10.1002/2014GL062498
Kang, S., and Eltahir, E. A. B. (2018). North China Plain threatened by deadly heatwaves due to climate change and irrigation. Nat. Commun. 9, 2894. doi:10.1038/s41467-018-05252-y
Li, P., and Ren, L. (2019). Evaluating the effects of limited irrigation on crop water productivity and reducing deep groundwater exploitation in the north China plain using an agro-hydrological model: I. Parameter sensitivity analysis, calibration and model validation. J. Hydrol. X. 574, 497–516. doi:10.1016/j.jhydrol.2019.04.053
Li, W., Wang, L., Yang, H., Deng, Y., Cao, W., and Liu, K. (2020). The groundwater overexploitation status and countermeasure suggestions of the North China Plain. China Water Resour. 13 (13), 26–30.
Li, X., Ye, S. Y., Wei, A. H., Zhou, P. P., and Wang, L. H. (2017). Modelling the response of shallow groundwater levels to combined climate and water-diversion scenarios in Beijing-Tianjin-Hebei Plain, China. Hydrogeol. J. 25, 1733–1744. doi:10.1007/s10040-017-1574-4
Liu, J., Zheng, C., Li, Z., and Lei, Y. (2008). Ground water sustainability: Methodology and application to the north China plain. Ground Water 46 (6), 897–909. doi:10.1111/j.1745-6584.2008.00486.x
Long, D., Yang, W., Scanlon, B. R., Zhao, J., Liu, D., Burek, P., et al. (2020). South-to-North Water Diversion stabilizing Beijing's groundwater levels. Nat. Commun. 11, 3665. doi:10.1038/s41467-020-17428-6
Nakayama, T. (2011). Simulation of complicated and diverse water system accompanied by human intervention in the North China Plain. Hydrol. Process. 25 (17), 2679–2693. doi:10.1002/hyp.8009
Rodell, M., Famiglietti, J. S., Wiese, D. N., Reager, J. T., Beaudoing, H. K., Landerer, F. W., et al. (2018). Emerging trends in global freshwater availability. Nature 557 (7707), 651–659. doi:10.1038/s41586-018-0123-1
Shah, T., Roy, A. D., Qureshi, A. S., and Wang, J. (2003). Sustaining asia's groundwater boom: An overview of issues and evidence. Nat. Resour. Forum 27 (2), 130–141. doi:10.1111/1477-8947.00048
Shao, J., Li, L., Cui, Y., and Zhang, Z. (2013). Groundwater flow simulation and its application in groundwater resource evaluation in the north China plain, China. Acta Geol. Sin. - Engl. Ed. 87 (1), 243–253. doi:10.1111/1755-6724.12045
Shu, Y., Villholth, K. G., Jensen, K. H., Stisen, S., and Lei, Y. (2012). Integrated hydrological modeling of the North China Plain: Options for sustainable groundwater use in the alluvial plain of Mt. Taihang. J. Hydrol. X. 464–465 (5), 79–93. doi:10.1016/j.jhydrol.2012.06.048
Sun, H.-Y., Liu, C.-M., Zhang, X.-Y., Shen, Y.-J., and Zhang, Y.-Q. (2006). Effects of irrigation on water balance, yield and WUE of winter wheat in the North China Plain. Agric. Water Manag. 85, 211–218. doi:10.1016/j.agwat.2006.04.008
Sun, K., Hu, L., Guo, J., Yang, Z., Zhai, Y., Zhang, S., et al. (2021). Enhancing the understanding of hydrological responses induced by ecological water replenishment using improved machine learning models: A case study in Yongding River. Sci. Total Environ. 768, 145489. doi:10.1016/j.scitotenv.2021.145489
The Ministry of Water Resources of the People’s Republic of China (2019). Groundwater overexploitation comprehensive management action plan [Online]. Available: http://szy.mwr.gov.cn/dtxx/201904/t20190429_1132485.html.[Accessed].
The People’s Government of Hebei Province (2019). Groundwater overexploitation comprehensive management implementation of the views [Online]. Available: http://info.hebei.gov.cn//eportal/ui?pageId=6806152&articleKey=6862328&columnId=6807806 ([Accessed].
Voss, K. A., Famiglietti, J. S., Lo, M. H., Linage, C. D., Rodell, M., Swenson, S. C., et al. (2013). Groundwater depletion in the Middle East from GRACE with implications for transboundary water management in the Tigris-Euphrates-Western Iran region. Water Resour. Res. 49 (2), 904–914. doi:10.1002/wrcr.20078
Wolfgang, K., Peter, B., Tobias, S., and Philip, B. (2003). Sustainable groundwater management–problems and scientific tools. Episodes 26, 279–284. doi:10.18814/epiiugs/2003/v26i4/002
Xiao, Y., Hao, Q., Zhang, Y., Zhu, Y., Yin, S., Qin, L., et al. (2021). Investigating sources, driving forces and potential health risks of nitrate and fluoride in groundwater of a typical alluvial fan plain. Sci. Total Environ. 802, 149909. doi:10.1016/j.scitotenv.2021.149909
Xiao, Y., Liu, K., Hao, Q., Li, Y., Xiao, D., Zhang, Y., et al. (2022a). Occurrence, controlling factors and health hazards of fluoride-enriched groundwater in the lower flood plain of Yellow River, northern China. Expo. Health, 345–358. doi:10.1007/s12403-021-00452-2
Xiao, Y., Liu, K., Hao, Q., Xiao, D., Zhu, Y., Yin, S., et al. (2022b). Hydrogeochemical insights into the signatures, Genesis and sustainable perspective of nitrate enriched groundwater in the piedmont of Hutuo watershed, China. Catena 212, 106020. doi:10.1016/j.catena.2022.106020
Yan, Z., Zhou, Z., Sang, X., and Wang, H. (2018). Water replenishment for ecological flow with an improved water resources allocation model. Sci. Total Environ. 643, 1152–1165. doi:10.1016/j.scitotenv.2018.06.085
Yao, P., and Lu, G. (2017). Hydrochemical and isotopic characteristics of the Juma River and their implications. Environ. Chem. 36 (7), 1525–1536.
Yu, Y., Wang, Y., and Liu, Y. (2012). Analysis of "12.7" rainstorm flood in Juma River. Haihe Water Resour. 5 (05), 22–24.
Zhang, C., Duan, Q., Yeh, P., Pan, Y., Gong, H., Gong, W., et al. (2019). The effectiveness of the South‐to‐North Water diversion Middle route project on water delivery and groundwater recovery in north China plain. Water Resour. Res. 56, e2019WR026759. doi:10.1029/2019WR026759
Zhang, F., Cheng, W., and Ran, H. (2017). Variation of groundwater level and it’s influencing factors analysis in plain areaof baoding city. Water Resour. Power 35 (8), 128–132.
Zhang, G., Fei, Y., Wang, H., Yan, M., and Liu, Z. (2009). Effects of grain increase and irrigation water saving on groundwater extraction in plain area of Hebei Province. Geol. Bull. China 28, 645–650.
Zhang, J., Wang, D., and Pan, S. (2012). Calculation of ecological water requirements based on groundwater model in baoding. Earth Sci. 37 (002), 370–374. doi:10.3799/dqkx.2012.044
Zhang, K., Zeng, S., Zhao, J., Wang, X., Zhou, J., Xu, H., et al. (2016). Effects of groundwater pressure extraction on wheat production in North China Plain. J. Agr. Sci. Technol. 18, 111–117.
Zhang, M., Hu, L., Yao, L., and Yin, W. (2018). Numerical studies on the influences of the South-to-North water transfer project on groundwater level changes in the Beijing Plain, China. Hydrol. Process. 32, 1858–1873. doi:10.1002/hyp.13125
Zhang, Y., and Che, Y. (2017). Analysis on the status quo and countermeasures of shallow groundwater overexploitation in Baoding Plain area. Groundwater 39 (03), 60–61.
Zhang, Y., and Li, G. M. (2014). Influence of south-to-north water diversion on major cones of depression in North China Plain. Environ. Earth Sci. 71 (9), 3845–3853. doi:10.1007/s12665-013-2771-7
Zheng, C., Jie, L., Cao, G., Kendy, E., and Jia, Y. (2010). Can China cope with its water crisis?-Perspectives from the north China plain. Ground Water 48 (3), 350–354. doi:10.1111/j.1745-6584.2010.00695_3.x
Keywords: river replenishment, restrictions on groundwater pumping, groundwater balance, groundwater model, China
Citation: Cui W, Hao Q, Xiao Y, Zhu Y, Li J and Yuanjing Zhang (2022) Combining river replenishment and restrictions on groundwater pumping to achieve groundwater balance in the Juma River Plain, North China Plain. Front. Earth Sci. 10:902034. doi: 10.3389/feart.2022.902034
Received: 22 March 2022; Accepted: 29 June 2022;
Published: 18 July 2022.
Edited by:
Xiangjun Pei, Chengdu University of Technology, ChinaReviewed by:
Sreekanth J,Commonwealth Scientific and Industrial Research Organisation (CSIRO), AustraliaPingping Luo, Chang’an University, China
Copyright © 2022 Cui, Hao, Xiao, Zhu, Li and Yuanjing Zhang. This is an open-access article distributed under the terms of the Creative Commons Attribution License (CC BY). The use, distribution or reproduction in other forums is permitted, provided the original author(s) and the copyright owner(s) are credited and that the original publication in this journal is cited, in accordance with accepted academic practice. No use, distribution or reproduction is permitted which does not comply with these terms.
*Correspondence: Qichen Hao, aGFvcWljaGVuX2loZWdAMTYzLmNvbQ==