- 1Sichuan Metallurgical Geological Survey and Design Group Co. Ltd, Chengdu, China
- 2Department of Earth Sciences, University of the Western Cape, Cape Town, South Africa
- 3College of Environment and Civil Engineering, Chengdu University of Technology, Chengdu, China
Heavy-metal pollution of soils has become a major environmental concern around the world presently. Soil washing provides an effective measure of removing contaminants from soil permanently, of which washing reagent plays a vital role in the process. This article reviews the current knowledge acquired on the main aspects concerning washing reagents of soil washing for remediation of heavy-metal-contaminated soil based on more than 150 published studies over a period from 1990 to 2021. The review identifies and discusses the types of washing reagents with their associated characteristics. Based on the factors influencing washing remediation, multi-criteria decision-analysis, together with an integrated four-step procedure, is put forward to manage the selection and prioritizing of washing reagents. It crops out from the literature survey that the selection of the washing reagents is a balanced process by considering washing effect, environmental impact, and cost-effectiveness. On the basis of such observation and evaluation, it is recommended that further study should be focused on developing new washing reagents or compound washing reagents that possess the advantage over heavy-metal removal ability, eco-friendliness, and cost-effectiveness as well. Selecting washing agents that are capable of being recovered from waste such as dissolved organic matter would be a promising trend in washing remediation. Artificial intelligence is expected to assist in the selection of washing reagents.
1 Introduction
Heavy-metal pollution of water and soils has become a major environmental concern around the world for the last few decades due to anthropogenic activities that lead to pollution, such as mining, smelting, electroplating, and wastewater irrigating (Jez and Lestan, 2016; Wang et al., 2020; Zhang et al., 2021a; Zhang et al., 2021b). Globally, there are over five million sites covering 20 million ha of land in which the soils are contaminated by various heavy metals (He et al., 2015; Liu et al., 2018). Soil contamination with heavy metals poses serious environmental hazards owing to the fact that heavy metals are non-biodegradable and they can accumulate in living organisms and cause long term harmful effects to human health. Therefore, there is an urgent need to remediate heavy-metal contaminated soils and protect environmental integrity of soils.
Various remediation techniques based on physical, chemical, and even biological processes have been proposed to remove heavy metals from contaminated soils. Among these techniques, soil washing is considered an effective measure that can permanently remove heavy metals from soil with high-remediation efficiency despite the concern of losing some nutrients and causing secondary pollution (Wei et al., 2016; Feng et al., 2020). Soil washing employs physical processes to separate the most contaminated soil particles (Figure 1), which in turn undergo chemical extraction with specific washing reagents to transfer heavy metals from the soil to solution (Saponaro et al., 2002; Dermont et al., 2008). The remediation effectiveness of soil washing is closely related to the extracting reagents. Several studies in Superfund programmes of the United States have shown that a wrong selection of washing reagents may lead to the failure of washing remediation (USEPA, 2004; 2005). Thus, the research on washing reagents is a hot button of washing remediation.
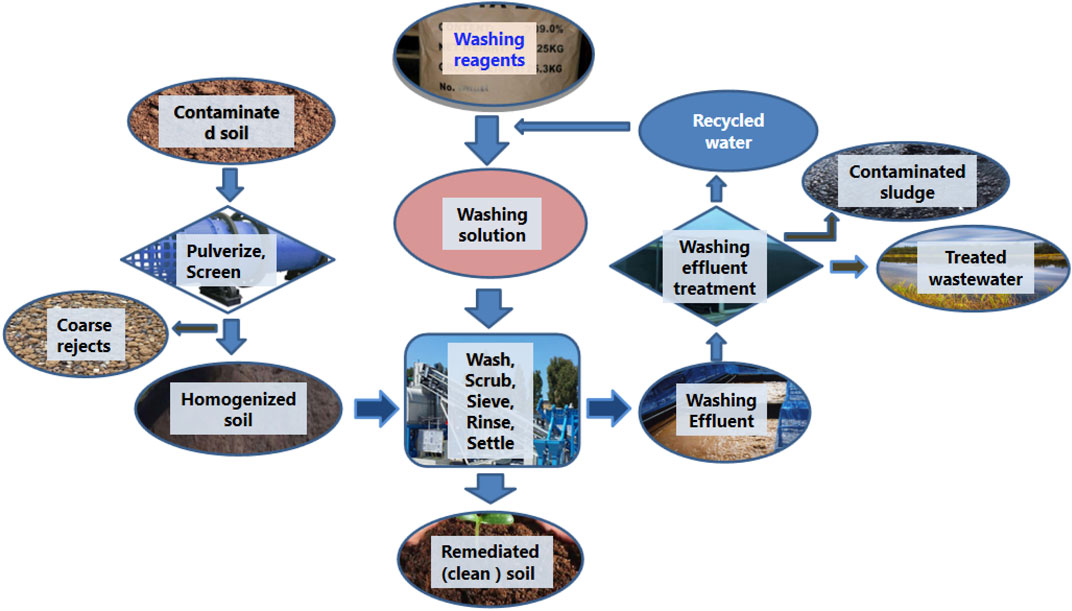
FIGURE 1. Typical technological process of soil washing (after Liu L et al., 2018).
Due to the extremely important role of washing reagents in soil washing remediation, an array of chemicals has been tested to formulate effective washing solutions (Moutsatsou et al., 2005; Bilgin and Tulun, 2015). Nevertheless, most of these washing extracts are developed on a case-by-case basis depending on the specific contaminant type at a particular site. Different washing reagents exhibit different effects and mechanisms under varying conditions (Liu et al., 2021). To the authors’ knowledge, there are few systematic studies on washing reagents of soil washing. The objective of the present article was to review current knowledge acquired on the main aspects concerning washing reagents of soil washing for remediation of heavy metals, which help to provide a significant reference for the study of soil washing. The review covered a brief description on washing reagents’ types with their associated characteristics. It also discussed factors that influence washing remediation and summarized the selection and prioritizing method of washing reagents. Finally, based on the current challenges, perspectives on washing reagents were provided for future research.
2 Types and Characteristics of Washing Reagents
Most chemicals have been investigated as washing reagents for remediating the heavy-metal contaminated soils (Yang et al., 2009; Fedje et al., 2013; Alghanmi et al., 2015). These washing reagents are generally divided into four categories, namely inorganic reagents, chelating reagents, surfactants, and compound chemical reagents.
2.1 Inorganic Reagents
The commonly used inorganic solutions include water, inorganic acids, alkali, inorganic compounds, and reducing or oxidizing (redox) reagents. The mechanism of soil washing with inorganic reagents is mainly to destroy the functional groups of the soil surface and form complex formation with heavy metals through washing, acidolysis, and complexation or ion exchange, thus exchanging and desorbing the heavy metals from the soil to the leachates (Ke, et al., 2004).
2.1.1 Water
Water, which is economic and easy to access, is able to flush away contaminants that are of soluble state. In a pilot study, which was carried out by Xiong et al. (2016), deionized water was reported to remove over 70% of hexavalent chromium. However, heavy metals are sparingly soluble and predominantly in the sorbed state; thus, washing the soils with water alone would not be expected to remove significant amount of heavy metals (Dikinya and Arela, 2010).
2.1.2 Acids
The most commonly reported acids used as washing reagents include hydrochloric acid (HCl), sulfuric acid (H2SO4), nitric acid (HNO3), and phosphoric acid (H3PO4). Oh et al. (2015) conducted a risk assessment of two different heavy-metal-contaminated soils that were collected from a former refinery site in Korea. As a result, it was found that 1 M HCl and 1 M HNO3 were effective for the removal of all heavy metals including Cd, Pb, Cu, Zn, Ni, and As, but HNO3 was considered the most efficient extract for Pb because the maximum removal efficiency of which reached 96.9%. Lin et al. (2012) performed laboratory- and pilot-scale washing of soils contaminated with Cr, Cu, Ni, and Zn using HCl, H2SO4, EDTA, and citric acid. H2SO4 with the concentrations between 0.3 M and 1 M was reported to have the best performance, and the removal efficiencies achieved 41%–42%, 38%–68%, 23%–25%, 47%–53%, and 42%–55%, respectively, when targeting Cr, Cu, Ni, Zn, and the total metal content.
Acids generally remove heavy metals from the soil relying on ion exchange and dissolution of soil components/discrete metal compounds, and the removal efficiency of inorganic acids varied in different studies. However, the high removal efficiency of heavy metals is usually achieved at the acid concentration of >1 mol/L, whereas a high concentration of acids causes a high increase in acidity of the treated soil, thus resulting in massive nutrient loss, soil structure change, and causing harm to microorganisms. Kwak et al. (2019) assessed the soil before and after washing remediation with sulfuric acid and phosphoric acid and found that the adverse effects observed in soil algae and earthworms after remediation were related to changes in soil pH, EC, total nitrogen, and total phosphorus. Owing to the changes in physical and chemical properties of soil, treated soil might need time to recover from the remediation before habitat quality and function are restored. Furthermore, wastewater and treated soils need to be neutralized, and the strong acidic conditions also have high requirements on treatment equipment.
2.1.3 Alkali
Alkali used as a washing reagent is not as popular as acids. The commonly used alkali for soil washing is sodium hydroxide. Yang et al. (2013) made use of 0.05 M H2SO4 and 0.05 M NaOH to wash soil contaminated by As and Pb. The result showed that sodium hydroxide solution had better performance on the removal of As than sulfuric acid. Sodium hydroxide is, especially effective in removing arsenic from soils, which is due to the ligand displacement reaction of hydroxyl ions with arsenic species and high pH conditions that can prevent reabsorption of arsenic. Similar to strong acids, alkali also causes serious damage to the physical and chemical properties of soil.
2.1.4 Inorganic Salts
The use of salt solutions may be an effective alternative to acid or alkali washing since these inorganic salt reagents have less damage to the physical and chemical properties of treated soils. Chloride salt solutions are the most investigated because metal ions can form soluble metal-Cl complexes with chloride, which is conducive to metal ions to dissolve into the solution (Dermont et al., 2008; Wahla and Kirkham, 2008). Ferric trichloride was reported to be effective in the removal of heavy metals, including Cd, Pb, Zn, and Cu (Zhai et al., 2018; Guo et al., 2019). Elements Fe and Cl are important constituents of soils; however, the research of Chen and Wu (2018) indicated that FeCl3 would also acidify the soil. Furthermore, the high concentration of FeCl3 can make the solution sticky, and therefore it is hard for the heavy-metal ions to spread in the solution, which can affect the extraction efficiency (Guo et al., 2016). ferric trichloride, according to Nedwed and Clifford (2000), the acidified NaCl and CaCl2 solutions were comparable and efficient with conventional extractants (EDTA and HCl) in removing Pb from fine-grained soils but with minimized destruction of soils’ physico-chemistry and microbiology. Alam et al. (2001) tried to apply several salts (including potassium phosphate, potassium chloride, potassium nitrate, potassium sulfate, or sodium perchlorate) to extract arsenic from a modeled soil and found that potassium phosphate was the most effective in the pH range of 6–8 (more than 40% extraction).
2.1.5 Reducing or Oxidizing (Redox) Reagents
Reducing and oxidizing reagents provide another option to enhance solubilization of heavy metals since chemical oxidation/reduction can convert metals to more soluble or less toxic forms through valence change. The United States Environmental Protection Agency (USEPA) has conducted laboratory research on Pb extraction involving redox manipulations and valence changes to promote the removal of various Pb compounds from synthetic contaminated soils since the 1990s (USEPA, 1994). Wang et al. (2014) investigated the removal of Cr (VI) from contaminated soil using sodium carboxymethyl cellulose stabilized nanoscale zero-valent iron that was prepared from steel pickling waste liquor and found that 80% of the loaded Cr (VI) was reduced to less toxic Cr (III) when the soil was treated with 0.3 g/L of Fe nanoparticles for 72 h. Several oxidant reagents have also been used to enhance the removal of heavy metals. Lin et al. (2001) used sodium hypochlorite as an oxidizer reagent in the chloride-based leaching process (2 M NaCl at pH 2) to extract metallic Pb-particles (smaller than 0.15 mm) and other Pb-species from highly contaminated soils. Based on the removal mechanism, the use of reducing or oxidizing reagents is usually limited to the heavy metals, which are varied in solubility and toxicity under different valences.
2.2 Chelating Reagents
Chelating reagents are capable of forming stable water-soluble complexes with a variety of metal ions through chelating and desorption, thus creating favorable conditions for soil washing. Various chelating reagents have been tested for soil washing, and their roles are becoming increasingly important. There are generally two kinds of chelating reagents that are commonly used in the study and practice, namely aminopolycarboxylic acids (also called synthetic chelating reagents) and natural low molecular organic acids (NLMOAs).
2.2.1 Aminopolycarboxylic Acids
Aminopolycarboxylic acids such as ethylenediamine tetraacetic acid (EDTA), diethylenetriamine five acetic acid (DTPA), hydroxyethyl ethylenediamine triacetate (HEDTA), ethylene glycol double tetraacetic acid (EGTA), nitrilotriacetic acid (NTA), N-(2-acetamide) iminodiacetic acid (ADA), ethylenediaminedisuccinic acid (EDDS), and iminodisuccinic acid (IDSA), have strong ability to activate metals and thus are commonly utilized in the soil washing processes due to their ability to interact with the majority of heavy metals (Leˇstan et al., 2008).
EDTA and its homologs form stable complexes with several heavy metals over a wide pH range, consequently becoming one of the most commonly investigated chelating reagents. Voglar and Lestan (2012) washed garden soil from a chemical extraction plant with 0.06 M EDTA on a pilot-scale and on average removed 79%, 38%, 70%, and 80% of Pb, Zn, Cd, and As, respectively. Jez and Lestan (2016) used H4EDTA, Na2H2EDTA, and CaNa2EDTA to wash twenty soil samples from Pb-contaminated areas in Slovenia, Austria, Czech Republic, in Pribram and United States, and removed more than 70% of Pb from the contaminated soils. EDTA is recognized as the most effective synthetic chelating reagent (especially for Pb, Cd, and Cu) because: 1) EDTA has a strong chelating ability for cationic heavy metals; 2) EDTA can treat a broad range of soil types; and 3) EDTA is recoverable and reusable owing to its low biodegradability. Compared to extraction with acids that changes the soil matrix, EDTA largely preserves soil property as a plant substrate. However, EDTA also presents two main disadvantages: 1) the price of EDTA products is high, and EDTA is a non-selective extracting reagent that are able to form a strong complex with a variety of metals, including both target toxic heavy metals and alkaline-earth cations such as Al3+, Ca2+, Fe2+, and Mg2+, and thus extracts large quantities of soil nutrients and meanwhile increases the cost; 2) EDTA may pose an ecological threat if it is not recycled or destroyed in the washing process because EDTA is resistant to chemical and biological degradation and thus has the potential for remobilizing heavy metals in the environment. The research conducted by Jez and Lestan (2016) showed that 15% and up to 64% of applied EDTA was retained in acidic soils after remediation, while in average 1% and up to 22% of EDTA was retained in calcareous soils.
Since EDTA has poor biodegradability and high persistence in the soil environment, which may result in deterioration of soil functions and groundwater pollution, biodegradable chelators such as ethylenediaminedisuccinic acid (EDDS), iminodisuccinic acid (IDSA), methylglycinediacetic acid (MGDA), iminodisuccinic acid (ISA), glutamate-N,N-diacetic acid (GLDA), glucomonocarbonic acid (GCA), 3-hydroxy-2,2′-iminodisuccinic acid (HIDS), and polyaspartic acid (PASP), have been suggested as alternatives to EDTA (Luo et al., 2015; Satyro, et al., 2016; Yoo et al., 2018). Begum et al. (2012) conducted lab-scale washing treatments of samples from metal-contaminated soils for the removal of the toxic metal ions using EDDS, MGDA, IDSA, GLDA, and HIDS, and as a result, GLDA showed relatively higher removal efficiencies for Cd, Cu, Ni, Pb, and Zn at pH 7 but tended to remove less soil organic matter, total and plant available ammonium, and exchangeable K, Na, Ca, and Mg which support the revitalization of the treated soils. Wang et al. (2018; 2020) also conducted batch washing to evaluate the potential for several biodegradable chelators for removing Cd, Pb, and Zn from polluted soils and found that GLDA, IDSA and ISA were appealing alternatives to EDTA.
Compared to extraction with acids that changes the soil matrix, aminopolycarboxylate chelating reagents largely preserve soil properties as a plant substrate. However, the eco-environmental consequences due to the release of aminopolycarboxylate acids into the surroundings become an issue of concern (Rahman et al., 2010). The lethal exposure resulting from some of the aminopolycarboxylate acids is likely to persist for a long period because of their poor photo-, chemo- and biodegradability, and in most cases, an increase in the threshold values for toxic effects may be observed with metal complexation. Thus, an increasing interest is focused on the development and use of the eco-friendly chelating reagent having better biodegradability and less environmental toxicity. According to the study by Begum et al. (2012) and Wang et al. (2018), GLDA appears to possess the greatest potential to rehabilitate polluted soils with limited toxicity remaining. However, Kaurin et al. (2020) designed a pilot-scale experiment using EDTA and biodegradable GLDA, EDDS, and IDS as chelators and argued about the advantage of EDTA over tested biodegradable chelators in process and remediation efficiency and environmental safety under realistic conditions.
2.2.2 Natural Low-Molecular Organic Acids
Natural low-molecular organic acids including acetic, citric, oxalic, tartaric, formic, malic, lactic, succinic, and fumaric acids are natural products of root exudates and microbial secretions as well as plant and animal residue decomposition in soils. These natural organic acids are considered biodegradable and eco-friendly chelating reagents, which are able to promote the dissolution and transformation of heavy metals from soils by forming complexes. Consequently, a large number of studies are conducted using varied NLMOAs. Acetic acid was used to leach Pb in field demonstrations at Fort Polk in Leesville, LO, but the nuisance of odors and its relative low removal efficiency limited its application (ITRC, 2003). Xiao et al. (2019) also studied the washing remediation of Cd and Zn using NLMOAs including acetic, oxalic, citric, and tartaric acids and concluded that in view of heavy-metal removal efficiencies and less disturbing on soil fertilities and plant growth, NLMOAs, especially citric acid, were more suitable than HCl, EDTA, and NTA.
The washing mechanism of using NLMOAs includes 1) the desorption of soil minerals by organic acids and the displacement of hydrogen ions; 2) organic acids complex with heavy metals to form positively charged metal complexes and exchange ions with soil; 3) after adsorption of organic acids on the soil surface, their functional groups complex with heavy metals to form ternary complexes; 4) coordination occurs between organic acids and heavy metals, and the complexes produced are not adsorbed to the soil, thus reducing the adsorption (Zhou et al., 2002; Zhou et al., 2016). The NLMOAs vary in ligand form, dissociation constant (pKa), and function group, which resulted in different removal efficiency of heavy metals (Li et al., 2011). Usually, the removal ability of the NLMOAs has a positive correlation with its dissociation constant and the number of carboxyl groups (-COOH). Although the NLMOAs are less effective in removing heavy metals from soils than aminopolycarboxylic acids because they are reported only effective to dislodge the exchangeable, carbonate, and reducible fractions of heavy metals (Peters, 1999; Wuana et al., 2010), they are more eco-friendly to the environment.
2.3 Surfactants
Surfactants are surface-active compounds which are able to change the interface state of the solution system significantly. Since surfactants are capable of assisting the solubilization, dispersal, and desorption of hydrophobic compounds and heavy metals due to their hydrophobic and hydrophilic portions, they are considered promising washing solutions to remediate heavy-metal-contaminated soils (Liu et al., 2019). Surfactants with different structures and properties can serve for different decontamination purposes. Generally, surfactants are classified into four categories: anionic (negatively charged), cationic (positively charged), nonionic (uncharged), and amphoteric (presents both positive and negative charges at an intermediate pH). Cationic surfactants are mainly nitrogen-containing organic amine derivatives which can dissociate cations in water. Since cationic surfactants make the surface of the matrix hydrophobic and easily cause secondary pollution in soil by adsorption, consequently, they are rarely used in soil washing. Anionic surfactants are the most productive and most widely used surfactants, which are classified into sulfonate and sulfate salts according to their structure of hydrophilic groups. Nonionic surfactants can be used in combination with other surfactants due to the good compatibility because the main hydrophilic group of nonionic surfactants is an undissociated ether group in an aqueous solution. Therefore, anionic and nonionic surfactants are widely used in soil remediation. The surfactant molecules are found either in nature or as products of laboratory synthesis, corresponding with the natural and synthetic origin; consequently, the current surfactants reviewed in soil remediation research and practice are usually classified into biosurfactants and chemical surfactants. The heavy-metal removal mechanism is that surfactant first adsorbed on the soil surface and interacted with the organic molecules and heavy-metal ions, causing the heavy-metal ions to leave the soil particle surface via ionic exchange and surfactant-associated complexation, and then a complex micelle forms, which would prevent the adsorption of heavy-metal ions to the soil (Figure 2).
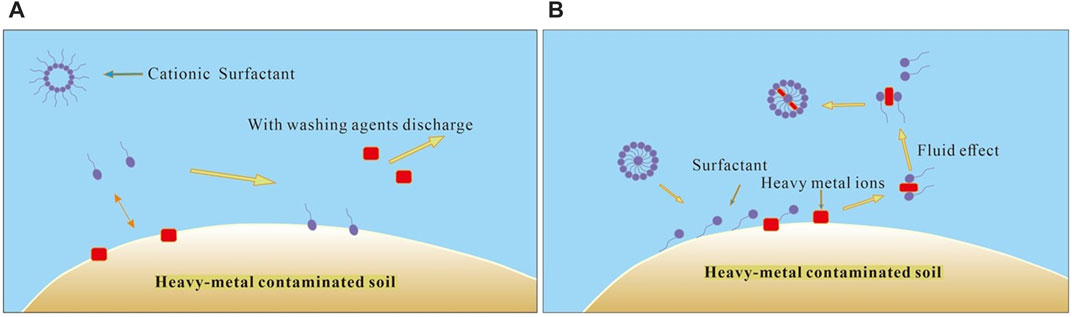
FIGURE 2. Mechanisms of soil washing with surfactants [(A) mechanism of washing with cationic surfactants; (B) mechanism of washing with anionic surfactants and biosurfactants].
2.3.1 Chemical Surfactants
The commonly used chemical surfactants include sodium dodecyl benzene sulfonate (SDBS), sodium dodecyl sulfate (SDS), cetyl trimethyl ammonium bromide (CTAB), Tween, TritonX, and polyoxyethylene laurel ether (Brij-35). Chen and Fu (2012) studied the heavy-metal removal effects of SDBS, SDS, and Tween-80 and found that the removal rates of chromium and cadmium by Tween-80 achieved 37.06% and 61.2%, respectively. Sun et al. (2011) compared the removal effects of SDS, CTAB, and Tween-80 in heavy metals contaminated soils through lab-scale experiments. The result indicated that these three surfactants showed poor performance on the clay soils but good extraction ability for sandy soils, and the removal ability was in the order of SDS > CTAB > Tween-80. Although several studies are conducted on the heavy-metal removal ability of chemical surfactants, the use of chemical surfactants to wash heavy-metal contaminated soils is still unpopular due to the following reasons: 1) the removal efficiency is limited because the polluted soils have a strong adsorption effect on the surfactants, thus causing adverse effect of removing heavy metals from the soil; and 2) the use dosage of surfactants and cost would be high. Therefore, chemical surfactants are always accompanied by other washing reagents to increase the heavy-metal removal ability.
2.3.2 Biosurfactants
Biosurfactants are bio-available surface-active compounds that are mainly generated in the vital movement of bacteria, fungi, and yeast as well as the metabolites of plants and animals (Paria, 2008; Liu et al., 2019). The commonly investigated surfactin, rhamnolipids, cyclodextrin, and sophorolipids are produced by microorganisms, while saponin and tannic acid are produced by plants. Owing to different produced microorganisms, raw matter, and process conditions, biosurfactants have many different types of structures and chemical compositions (e.g., fatty acids, glycolipids, lipopeptides, lipopolysaccharides, and lipoproteins).
Biosurfactants are beneficial for improving the interaction between microorganisms and contaminants in soil and have little negative impact on soil structure and microbial growth; thus, in recent years, biosurfactants have attracted wide attention in soil remediation (Maity et al., 2013; Kholghi et al., 2020). Gusiatin et al. (2019) applied saponin, tannic acid, and rhamnolipids to remove multi-metals from soils. The removal efficiency order of Cu, Ni, and Zn was saponin > tannic acid > rhamnolipids, whereas saponin and tannic acid were the least effective for Pb removal (18%–31% and 11%–35%, respectively). These three biosurfactants effectively removed the heavy metals of the exchangeable and acid soluble fraction and partially removed them from the reducible and oxidizable fraction. A new trend in soil washing with biosurfactants is the use of plant extracts containing saponins instead of commercial powder products. Saponins extracted from soapberry (Sapindus mukorossi L) and soapnut fruit pericarp have a better potential for the removal of heavy metals (Ni, Cr, and Mn) from the soil than commercial saponin (Maity et al., 2013; Wang et al., 2015; Ye et al., 2015). Furthermore, with plant extracts, the operational costs of soil remediation are substantially lower than those with commercial biosurfactants.
Compared to other washing reagents, biosurfactants are important reagents in soil remediation because they are biodegradable, hypotoxic, easily available, and have high selectivity to metal removal. However, concerns on the application of biosurfactants exist in the following aspects: 1) the cost is usually relatively high; 2) there is a risk of secondary pollution caused by the recycle process of leaching waste as well as the parts of toxic reagent; 3) several biosurfactants may be required to remedy the multiple-metal-contaminated soils due to the diverse affinities of biosurfactants to different heavy metals.
2.4 Compound Chemical Reagents
Various metals often coexist at contaminated sites. These coexisting metals, together with the need of soil protection, present difficulties to soil washing due to their dissimilar chemical properties. Under this situation, a single reagent may be insufficient to achieve the remediation goal. For instance, Na2EDTA is recognized as the most effective chelating reagent to remove cationic metals but not in anionic metals (Udovic and Lestan, 2010). By contrast, NLMOAs (e.g., oxalic acid, citric acid, and tartaric acid) and some inorganic acids (e.g., phosphoric acid and nitric acid) attain much higher anionic metal extraction from soils, sediments, and mine wastes (Drahota et al., 2014). Consequently, using multiple solutions as compound chemical reagents to improve the removal efficiency has become a new favorite method in recent years. There are mainly two kinds of compound chemical reagents: 1) mixed reagents that are composed of several solutions; and 2) dissolved organic matter from various wastes or composts.
2.4.1 Mixed Reagents
Since the heavy-metal removal abilities from soils vary among different washing reagents, there is the possibility of enhancing the washing effect by combining different reagents. Plenty of studies were conducted on the development of mixed reagents recently, and most of the studies showed that the combination of multiple reagents achieved higher removal efficiencies than a single reagent did (Table 1).
As can be seen from Table 1, nearly all kinds of common reagents are involved in the combination of mixed reagents: aminopolycarboxylic acids–NLMOAs, aminopolycarboxylic acids–chemical surfactants, aminopolycarboxylic acids–biosurfactants, aminopolycarboxylic acids–inorganic salts, and NLMOAs–biosurfactants. In addition to these commonly used mixed washing reagents, novel combinations of solutions such as combination of EDTA, NLMOAs, saponin, and nanoscale zero-valent iron, deep eutectic solvents were reported to remove heavy metals from contaminated soils (Wang et al., 2014; Mukhopadhyay et al., 2016; Mehrabi et al., 2020; Huang et al., 2021). Mixed reagents were developed due to the following advantages: 1) increase the removal efficiency of target heavy metals when a single reagent is insufficient; 2) decrease the dosage of an expensive reagent and thus decrease the cost; and 3) decrease the dosage of a toxic reagent and thus protect soil from damage. However, not all the combinations of mixed reagents can be expected to have better performance. For instance, Li et al. (2011) reported that the combination of “Citric acid + SDBS + EDTA” got a worse leaching efficiency of chromium than using citric acid alone. Furthermore, despite the aforementioned advantages of mixed reagents, there is still a risk of increasing secondary pollution to the environment which is caused by the combination of different reagents.
2.4.2 Dissolved Organic Matter
Washing reagents should be compounds which have a high number of functional groups that are capable of forming complexes with metal ions and removing them from soil to the washing solution. Such reagents can be dissolved organic matter (DOM) of different origins, for e.g., wastewater treatment, food processing, or wine processing (Klik et al., 2021). DOM possesses multiple binding sites and thus has the ability to form highly stable and soluble organo-metallic complexes with heavy metals, which is a desirable characteristic of washing reagents that can be used in soil washing technology.
The DOM in the forms of soluble humic substances and volatile fatty acids have a high potential to be used as washing reagents to remove heavy metals (Hartley et al., 2014; Kulikowska et al., 2015; Dong et al., 2021). Soluble humic substances are composed of humic acids and fulvic acids, which show good surface-active properties, such as decreasing the surface tension of water, and in this regard, they are similar to plant biosurfactants. The interaction of humic acids with metal ions results in the formation of strong metal–humate complexes. Fulvic acids have a poorly developed aromatic nucleus and numerous side chains with oxygen-containing functional groups (Stevenson, 1994), and they contain more carboxylic groups than humic acids. The lower molecular weight of fulvic acids, due to their low degree of polymerization and their higher content of functional groups, favor the formation of more soluble, bioavailable, and mobile complexes with heavy metals. Volatile fatty acids are also effective in the removal of heavy metals. Zou et al. (2019) applied volatile fatty acids, which were derived from food waste, as soil washing reagent to treat contaminated soil and attained removal rates of 57.09% and 23.55% of extractable fractions of vanadium and chromium, respectively.
In addition to the aforementioned humic substances and volatile fatty acids, there are other sources of DOM. Distillery sludge and alkaline substances, including NaOH, KOH, Ca(OH)2, and Mg(OH)2, were employed to prepare DOM solution to treat Cd-contaminated soil through soil washing. Approximately 80% of Cd was removed from the soil, while other indices of soil fertility were improved after soil washing (Liu and Chen, 2013). Can et al. (2018) employed four wastes including pineapple peel, soybean straw, broad bean straw, and tea residue to remove Cd, Pb, and Zn in contaminated soils and found that pineapple peel has the highest removals for Cd (90.1%), Pb (18.6%), and Zn (15.2%). The relatively high metal removal was mainly attributed to the effective removal of the exchangeable and acid soluble fractions due to the fact that hydroxyl, carboxyl, amine, carbonyl, and amide groups were involved in the interaction with metal ions by complexation or ion exchange. Some liquid waste can be used directly to treat soil without the need of extracting DOM. For example, Zhang et al. (2017) employed a citric acid fermentation broth to wash heavy-metal polluted soil. This solution contained a variety of microbial metabolites that were derived from three carboxylic acid cycles along with other components in the form of organic acids and surface-active substances. Preliminary investigations showed that this type of washing solution was highly applicable for the removal of Cd, Cr, Cu, and Pb from the soil, and the removal efficiency was higher than that of the citric acid solution but had only a small effect on the mineral composition of the soil.
Compared to other commercial washing reagents, DOM costs very low because it can be derived from various wastes or composts. Furthermore, composts and various kinds of wastes are rich not only in soluble organics but also in nutrients, which indicates that DOM as a washing reagent can both remove heavy metals and fertilize the treated soil by increasing the content of organic matter and nutrients. Therefore, the use of DOM as a washing reagent is a recent improvement in soil washing.
2.5 Characteristics of Washing Reagents
Based on the aforementioned, each type of washing reagent has both advantages and limitations (Table 2). Generally, the inorganic acids HCl, H2SO4, HNO3, and H3PO4 are effective in removing heavy metals. Alkali was reported to be especially effective in the removal of As. However, acid or alkali washing strongly affects the pH of the soils, thus causing damage to the soil structure as well as important loss of soil mineral substances and organic matter. Inorganic salts and reducing or oxidizing reagents are reported to have a good performance in the removal of heavy metals, but they are generally less effective in comparison with inorganic acids.
EDTA is reported to be effective in extracting most kinds of heavy metals from soils, but it has poor biodegradability and thus is worried by some researchers to cause secondary pollution to soil and groundwater. EDDS, IDSA, GLDA, and NTA are biodegradable; accordingly, they are usually considered substitutes of EDTA. GLDA appears to possess the greatest potential to rehabilitate polluted soils with limited toxicity remaining among these reagents. However, opinions are divided on this point. EDTA is tested to have better performance on remediation efficiency and environmental safety over the aforementioned biodegradable chelators in a recent pilot-scale study. Another important limitation is that these aminopolycarboxylic acids are too expensive to be widely used. Natural low-molecular organic acids such as citric acid, oxalic acid, tartaric acid, and acetic acid are reported to be less effective for the removal of the heavy metals in comparison with EDTA, but they are biodegradable and thus are considered to be more eco-friendly to the environment. Citric acid is reported to be the most effective washing reagent among these NLMOAs.
Chemical surfactants are seldom reported to have good ability on heavy metal extraction from contaminated soils, but they are able to improve the removal ability of heavy metals when used together with other washing agents. Compared with the chemical surfactants, biosurfactants are much effective in the removal of heavy metals although the removal efficiency is generally not as good as strong acids and EDTA, and the difference is obvious in extracting rates of biosurfactants to different heavy metals. Moreover, biosurfactants are biodegradable and easy to be available. However, the cost of chemical surfactants and biosurfactants is usually high.
Compound chemical reagents are mixed reagents or compounds that have a high number of functional groups which are capable of forming complexes with metal ions and removing them from soil. Compared to single washing reagents, compound chemical reagents usually have a good performance in the removal of heavy metals. DOM is eco-friendly and cost-efficient, thus becoming a promising washing reagent in recent improvements in soil washing.
3 Selection of Washing Reagents for the Remediation of Heavy-Metal-Contaminated Soils
3.1 Affecting Factors of the Selection of Washing Reagents
Although plenty of washing reagents are employed to treat the heavy-metal-contaminated soil, it is important to mention that the selection of the most suitable reagents depends highly on the affecting factors. Therefore, understanding of the affecting factors is fundamental to the choice of reagents for washing remediation. There are various factors that affect the application of washing remediation; however, geochemistry of the soil, metal characteristics, washing reagents together with the processing conditions, and treatment and reuse of the washing effluent influence the environment, and the cost and available budget are considered the primary affecting factors.
3.1.1 Geochemistry of the Contaminated Soil
Soil is a dynamic system, which is a heterogeneous mixture of organic and mineral components with differences in pH values, redox conditions, moisture content, and undergoing gradual alterations in response to changes in the environment. The sub-factors of soil geochemistry that affect the applicability and effectiveness of washing include soil texture, organic matter content, cation exchange capacity, and buffering capacity.
3.1.1.1 Soil Texture
Research works have shown that the solubilization/exchange/extraction of heavy metals by washing differs considerably for different soil types. For example, the extraction of lead using hydrochloric acid differs greatly in the allophanic soil and the smectitic soil (Isoyama and Wada, 2007). Furthermore, particle size plays an important role in soil washing. Fine particles have high surface areas for retaining strongly inorganic contaminants and further partially consist of recalcitrant minerals; thus, contaminants can be enriched in fine particles (Ko et al., 2005). Consequently, it is easier to remove heavy metals from larger size particles than very fine particles such as silt and clay since less molecular attraction is exited between heavy metals and large soil particles than fine particles. Furthermore, heavy metals could readily be readsorbed by iron-manganese oxides that are located on the surface of fine particles than larger size particles, and the combination is too strong to make the metals migrate to the solution (Xia et al., 2009). There is a generally held opinion that soil washing is only effective for sandy and granular soils where the clay and silt content (particles less than 0.063 mm) is less than 25% of the soil because the clay-rich soils pose problems such as difficulties with materials handling, solid-liquid separation, longer contact time, and even reduced extraction efficiency (Tampouris et al., 2001; Wuana and Okieimen, 2011). Recently, Yang et al. (2016) and Rui et al. (2018) reported effective washing processes that have treated soils with high clay/silt content (>50%) using compound chemical reagents.
3.1.1.2 Organic Matter Content
The carboxyl groups (adsorption sites) on organic matter or humic substances have a high affinity for heavy metals; therefore, organic matter usually has strong absorption to the washing reagents. Based on the study of Morin et al. (1999), inner-sphere adsorption complexes are directly observed in soils contaminated with Pb; thus, high rates of Pb removal seldom occur from organic matter and metal oxides. Furthermore, the strong absorption between the organic matter and washing reagents not only reduces the concentration of solution so as to inhibit metal extraction but also has a toxic effect on microorganisms in the soil if a large amount of the reagent is adsorbed for a long time.
3.1.1.3 Cation Exchange Capacity
The exchangeable cations of major elements such as Fe, Ca, and Mg may interfere with the washing process owing to the competition between exchangeable cations and the target heavy metals. For example, the low selectivity of EDTA causes a great consumption of this reagent due to the potential chelation of all the exchangeable cations presented in soil, such as Ca 2+, Fe 3+ (Palma and Ferrantelli, 2005).
3.1.1.4 Buffering Capacity of Soils
Calcite in the contaminated soil forms the buffering capacity of heavy metal removal through soil washing, especially acid leaching. The high calcite content or high buffering capacity may decrease the acid-leaching efficiency; thus, acid leaching may be ineffective in soils that have a high buffering capacity, such as calcareous soils (Tejowulan and Hendershot, 1998). This adverse effect of buffering capacity of soils to heavy-metal removal would also be supported by the fact that the addition of calcium carbonate to soil would strongly reduce metal scavenging by the solution from all soil fractions (Ash et al., 2015).
Based on the aforementioned, sub-factors from the soil geochemistry aspect that may limit the applicability and effectiveness of soil washing include 1) high clay/silt content; 2) high organic matter content; 3) high content of Fe and Ca element; and 4) high calcite content or high buffering capacity.
3.1.2 Characteristics of Metal Contaminants
Toxic metals are present in various chemical forms in soils, which exhibit different physical and chemical behaviors in terms of chemical interactions, mobility, biological availability, and potential toxicity. Consequently, the removal efficiency of various heavy metals differs considerably owing to the varied characteristics of metal contaminants which include metal type, concentration, valence, speciation (distribution of chemical species), and fractionation (fractions according to bonding with specific soil substrates) of heavy metals (Maity et al., 2013; Zhai et al., 2018). For example, chromium exists either as the species of trivalent chromium Cr (III) or as hexavalent chromium Cr (VI). Cr (VI) can be easily reduced to Cr (III), and meanwhile, it can also be transformed from Cr (III) to Cr (VI) by manganese oxide in the soil. Washing with hydrochloric acid is relatively efficient for chromate (CrO42−) removal from non-allophanic soils, but it is inefficient for the removal of trivalent chromium Cr3+, particularly from soil that has high cation exchange capacity (Isoyama and Wada, 2007).
Chemical speciation plays a vital role in the solubility, thus influencing the removal of metals from soils; however, the metal speciation analysis can be complicated (especially when the soil is contaminated with a complex mixture of metal compounds); thus, the metal fraction according to soil substrates is often applied. The partitioning of metals according to their association with the soil substrates is usually determined by the sequential extraction procedure, the methods of which are widely used, include the Tessier procedure and BCR sequential extraction procedure (Fernández et al., 2004). A total of five fractions are involved in the extraction procedure by Tessier et al. (1979), including F1) exchangeable, F2) acid soluble/carbonate bound, F3) reducible/Fe-Mn bound, F4) oxidizable/organic matter and sulfide bound, and F5) residual. BCR procedure is largely similar to that produced by Tessier with the chief difference in the first fraction of the procedure. Instead of evaluating the exchangeable and carbonate bound separately, the BCR procedure combines both in the first fraction (Zimmerman and Weindorf, 2010). The fractionation of heavy metals in soil is closely related to soil geochemistry. The exchangeable fraction is mostly adsorbed on humus and clay which is easy to remove; the carbonate bond is most sensitive to pH value; the Fe-Mn oxide bond is to be released when the redox potential decreases; the organic bound is released under strong oxidation conditions; however, the residue fraction is the most stable. Therefore, the fractions most amenable to removal by chemical washing are 1) exchangeable, 2) associated with carbonates, and 3) associated with reducible Fe-Mn oxides of soils (Peters, 1999), while metals associated with the residual soil fraction, imbedded in the mineral lattices or discrete particle forms, are difficult to be removed. Meanwhile, the extraction of heavy metals that are bound to exchangeable and carbonate fractions is faster compared to the fraction of Fe-Mn oxides (Wasay et al., 2001). Nevertheless, the metal fractionation data does not always clearly explain metal removal efficiency because removal efficiency also depends on other factors such as metal concentration and soil geochemistry. Meanwhile, the research of Ash et al. (2015) indicated that there would be a fraction of redistribution during the washing process.
Metal fractionation is important for the choice consideration of washing reagents. Generally, heavy metals in the water-soluble state can be removed by water; while heavy metals in exchangeable, carbonate and Fe-Mn bound can be removed by ion exchange through salt solutions or through chelating and complexing of chelating agents and surfactants; and the removal of organic matter and sulfide bound as well as residual generally requires high concentration of acids and chelating reagents with strong chelating ability.
3.1.3 Washing Reagents and the Processing Condition
Washing reagents are generally the solutions with the functions of ion exchange, chelation, and complexation, and the removal ability of heavy metals differs considerably among the extracting reagents. The effects of the washing reagents, together with their processing conditions on removing heavy metals from contaminated soil, should be carefully considered in both in situ leaching and ex situ washing despite the fact that quantities of studies have been conducted. Moon et al. (2012) studied the removal effect of Zn using several washing reagents, including inorganic acids, alkali, and NLMOAs. The results showed that using HCl at 3 M achieved the highest removal efficiency of Zn, while the removal effect of residence time (1 h or 2 h) and soil to solution ratio (from 1:10 to 1:20) was not obvious. However, the research of Zhu et al. (2013) indicated that EDTA was the most effective for the removal of Cd and Pb, and the optimal processing conditions were: 0.1 M EDTA, soil-to-solution ratio 1:6, residence time 3 h, and washing two times. Sun et al. (2011) studied the optimum processing condition of removing heavy metals in the sandy and clay soils using surfactants (SDS, CTAB, and Tween 80). The removal efficiency order in sandy soils was SDS > CTAB > Tween 80, and the highest removal rate of heavy metals Cu, Zn, Pb, Ni, and Cd was achieved under the condition: soil-to-solution ratio 1:50, moral ratio (surfactants/heavy metal) 15:1, and pH 4. These case studies reflect that type and dosage of the washing reagent, pH value, temperature, residence time, soil-to-solution ratio, washing times, and the order of sequential washing are important sub-factors of washing reagents that affect the removal of heavy metals.
Generally, washing reagents with a strong chelating ability or strong acidity have a good heavy-metal removal effect on soil (Table 2); however, strong chelating ability and strong acidity may cause adverse effects on the environment. Usually, the higher the concentration of the washing agent, the better the removal ability; however, high concentrations of washing reagents can lead to the loss of large numbers of ions.
The pH of the washing solution plays a significant role in the extractability of heavy metals from soils. The extractability of most cationic heavy metals (e.g., Cd, Cu, Pb, and Zn) increases when the solution pH decreases. At low pH, the protons (H+) added can react with soil surface sites (layer silicate minerals and/or surface functional groups, including Al-OH, Fe-OH, and COOH groups) and enhance the desorption of metal cations, which are transferred into the washing fluid (Isoyama and Wada, 2007). However, for the arsenic-contaminated soil, good removal ability is achieved under both strong acid and strong alkali conditions.
The residence time, which is also called washing time or contact time, is one of the main sub-factors affecting the removal efficiency. It is necessary to ensure that the washing solution is in full contact with the soil particles so as to leach out heavy metals. The residence time is directly related to the consumption of the washing reagent, thus increasing the cost. There is usually an optimal residence time for the washing process, within which the removal efficiency of the heavy metals increases sharply with the increase of the residence time. However, after the optimum residence time, the continued consumption of the washing reagent could not significantly improve the removal efficiency. In general, the residence time of inorganic washing reagents is relatively short, while that of organic reagents is relatively longer so as to ensure a sufficient reaction between reagents and heavy metals in soil (Wang, 2013). Under inorganic acid washing condition, the extraction of exchangeable and residual fractions was less variable above more than 15 min, while in the case of Fe/Mn oxide and organic/sulfides fractions, there are possibly a gradual extraction of As and Zn by an increase of reaction time (Ko et al., 2005).
The soil-to-solution ratio refers to the ratio of the mass or volume of the soil to the washing reagent. The removal efficiency of heavy metals usually increases with the decrease of the soil to solution ratio because the reduction of the soil to solution ratio means an increase in the amount of washing reagent added to the contaminated soil. The choice of soil to solution ratio should be appropriate because it is not conducive to stirring if the ratio is too large (e.g., bigger than 1:2), while too small of the ratio (e.g., smaller than 1:20) will increase the load of the equipment as well as the consumption of washing reagent and waste effluent, thus increasing the cost of treatment.
During the washing process, the number of washing times reflects the change of soil to solution ratio. The study of the washing times can better reflect the removal effect of different washing batches on heavy metals so as to maximize the heavy-metal removal efficiency of the washing reagent.
The optimum processing conditions vary among studies owing to the differences in soil geochemistry, metal contaminants, and washing reagents. However, these sub-factors in terms of washing reagents are related to each other. The times of washing reflect the change of soil to solution ratio, while the soil to solution ratio and concentration reflect the different ratios of the dosage of washing reagent and the amount of soil to some extent.
3.1.4 Influence on Soil and the Environment
Before the implementation of soil washing, the influences of remediation on the soil and environment should be considered carefully because some of the extracting reagents can persist in the environment at unacceptable levels, which may cause adverse influence on surrounding residents, microbial communities, and other organisms in the soil. For example, EDTA is low in degradation; thus, there is a potential to contaminate soil and groundwater. EDDS is considered a degradable chelating reagent; however, in the case of being taken as the only source of carbon and nitrogen, Cr (III)-EDDS, Fe (III)-EDDS, Pb-EDDS, Al-EDDS, and Cd-EDDS have degraded, while Cu-EDDS, Ni-EDDS, and Hg-EDDS have not degraded yet (Vandevivere et al., 2001). Citric acid and tartaric acid are usually considered eco-friendly chelating agents; however, they are also reported to cause an 80% loss of the elements (Wasay et al., 1988).
3.1.5 Treatment and Reuse of the Washing Effluent
The remediation process produces a final effluent which contains washing reagents and extracted metals at concentrations higher than discharge limits; therefore, an important factor in connection with the application of washing reagents to full scale remains; however, the subsequent treatment of the washing effluent before it can be safely discharged into the aquatic environment so as to avoid of secondary pollution or be reused to reduce remediation cost.
Several strategies are proposed for the treatment of the washing effluent. The treatment of soil acidification and effluent caused by washing with inorganic acids is neutralization and precipitation. The commonly used effluent treatment techniques for chelating reagents include trans-complexation, ion exchange, reverse osmosis, advanced oxidation processes, and electrochemical method (Pociecha and Lestan, 2010; Sun et al., 2015; Satyro et al., 2017). Kim and Ong (1999) introduced the trans-complexation method: the Pb in the EDTA complex was replaced with Fe3+ at low pH, and the Pb was subsequently removed using NaOH as a precipitating agent. This process was relatively expensive and proved difficult if the EDTA was complexed with more than one metal. Lim and Kim (2013) used alkaline chemicals to precipitate arsenic and heavy metals in the effluent containing oxalic acid and then reused the decontaminated effluent for the soil washing. Juang and Wang (2000) proposed electrolytic recovery of Pb and Cu from a solution containing EDTA using sensitive and expensive cation-exchange membranes, but the process of which was technically quite complicated. Di Palma et al. (2003) proposed reverse osmosis for the separation of EDTA complexes from the washing solution, while the soil colloidal particles tend to clog the membranes. Finzgar and Lestan (2000) proposed oxidative decomposition of EDTA complexes in washing solution using advanced oxidation processes, which was much less effective when the washing solution was either turbid or colored. The treatment of electrochemical-advanced oxidation processes was more robust but consumed a significant amount of electricity and used an expensive boron-doped diamond anode (Pociecha and Lestan, 2009). Pociecha and Lestan (2010) used electrocoagulation with an Al sacrificial anode to separate the Pb, Zn and Cd from a washing solution. Satyro et al. (2016) applied the combined TiO2− photocatalytic processes to decontaminate the effluents which were produced in the washing process of polluted soil using EDDS as an organic chelate. Despite the fact that various techniques have been studied, the difficulty and high cost of effluent treatment make it an obstacle to the full scale application and commercialization of soil washing technologies.
3.1.6 Cost and Available Budget
The price of the washing reagents and the available budget are key constraints that affect the implementation of soil washing. For example, EDDS is an eco-friendly chelating agent with good heavy-metal removal efficiency, but the relatively high price limits its application (Hong and Jiang, 2005). Zhou et al. (2016) compared the costs of different remediation techniques and concluded that the cost of soil washing is up to seven times more expensive than other available remediation techniques. Due to the high costs, several cases of soil washing in the Superfund program of the United States (Table 3) failed implementation (Dermont et al., 2008). Therefore, soil washing is practical only when the cost and available budget are balanced, and the low cost of the washing reagent is an eternal pursuit in the practice of soil washing.

TABLE 3. Examples of deselected soil-washing projects in the Superfund Program (data extracted from Dermont et al., 2008).
The aforementioned affecting factors have internal relationships among themselves, which interact with each other and work together to determine the removal of heavy metals from soils.
3.2 Preliminary Choice of Washing Reagents Based on the Affecting Factors
The inorganic acids, chloride salts, chelating reagents, and biosurfactants are generally reported effective in the removal of heavy metals (Supplementary Table S1). However, each kind of washing reagent has both advantages and limitations. Strong inorganic acids have strong heavy-metal removal ability and low price, but they strongly affect the pH of the soil. Alkali has strong removal ability to As and medium removal ability to Zn and Hg, which is cheap in price but poor in eco-friendliness. The heavy-metal removal ability, eco-friendliness, and cost-effectiveness of chloride salts are at a medium level. Aminopolycarboxylic acids are poor in cost-effectiveness; EDTA has strong heavy-metal removal ability and is medium in eco-friendliness. Other degradable aminopolycarboxylic acids are medium in heavy-metal removal ability and good in eco-friendliness. Natural low molecular organic acids, which a have medium level of heavy-metal removal ability, are good in eco-friendliness and cost-effectiveness. Synthetic surfactants are poor in heavy-metal removal ability, eco-friendliness, and cost-effectiveness. Biosurfactants which have medium heavy-metal removal ability are eco-friendly but poor in cost-effectiveness. Dissolved organic matters present medium heavy-metal removal ability, but they are good in eco-friendliness and cost-effectiveness. During the preliminary choice process of washing reagents for the common heavy metals, washing effect, environmental impact, and cost-effectiveness should be balanced to get the suitable options. Under the condition of ensuring the removal effect, the impact on the environment and cost should be reduced as far as possible.
Due to technical issues of uncertainties in different soil and contaminant characteristics, together with the relationship of the affecting factors, it is difficult to make decisions on the selection of suitable washing reagents. Moreover, remediation practices are unique (Hou et al., 2014) in that they involve multiple local stakeholders in the decision-making process (Lehigh et al., 2020). To come to a consensus on such complex interdisciplinary problems, different multi-criteria decision-analysis (MCDA) tools, adopted for choosing an optimum site-specific remediation method, have been developed (Hou et al., 2018). A promising MCDA method that is able to assist the choice of washing reagents is the analytical hierarchy process (AHP) (Saaty, 1980), which can be used to combine all the representative criteria into a “criteria tree”, with different levels of priorities accordingly. The application of AHP involves the decomposition of the ultimate goal into a three-level hierarchy consisting of subcriteria of the goal. A suitable washing reagent means that it is able to remove the metals to meet safety standards, meanwhile preserving the natural soil properties to the maximum extent within a reasonable budget. Accordingly, the top of the hierarchy is the goal of the analysis, relating to the “choice of washing reagent”; the middle level contains more specific criteria with regard to the objective, relating to “heavy-metal removal efficiency,” “influence on soil and environment,” and “cost and available budget,” which can take a reference as Supplementary Table S1; and the bottom level refers to the most specific criteria related to the main criteria in the middle level: 1) “types of washing reagents” is chosen to support the “influence on soil and environment,” 2) “soil geochemistry,” “metal characteristics,” and “washing reagents and the processing conditions” are taken into account to support “heavy-metal removal efficiency,” and 3) “price of washing reagents” and “treatment and reuse of washing effluent” are considered to support “cost and available budget”. The AHP hierarchy structure of affecting factors in the choice of washing reagents is shown in Figure 3.
Each subcriterion in the criteria tree is represented by a value supporting the criterion of upper level through pair comparison. Then, the weights reflecting the relative importance order of criterion at each level are assigned based on the opinions of experts or the objective judgment of the decision-maker. The values of supporting objective and relative weights for all levels are calculated and then sorted. The preliminary choice of washing reagents is based on the following principle: highly suitable washing reagents obtain high values, while less suitable reagents obtain lower values.
where SI is the suitability index of washing reagent; n is the total number of subcriteria; vi is the value for subcriterion i; and wi is the weight assigned to subcriterion i.
Since criteria interaction in decision-making problems is complex, therefore, another MCDA method named INfluence-based deciSIon guiDE (INSIDE) which combines the Decision Making Trial and Evaluation Laboratory and Analytic Network Process (ANP) techniques, is introduced as a methodology to prioritize between remediation methods for a contaminated groundwater aquifer (Naseri-Rad et al., 2020). Therefore, taking criteria interaction into account, INSIDE is also useful for the preliminary choice of washing reagents.
3.3 Integrated Procedure of Selecting Washing Reagents
The selection of washing reagents is aimed at assisting the removal of heavy metals but preserving the natural soil properties within reasonable budget limits, which is also considered a balanced process of washing effect, environmental impact, and cost-effectiveness. Therefore, during the process of choosing washing reagents, the main affecting factors of the reagents for washing remediation should be taken into account comprehensively. Four steps are included in the procedure of choosing washing reagents (Figure 4).
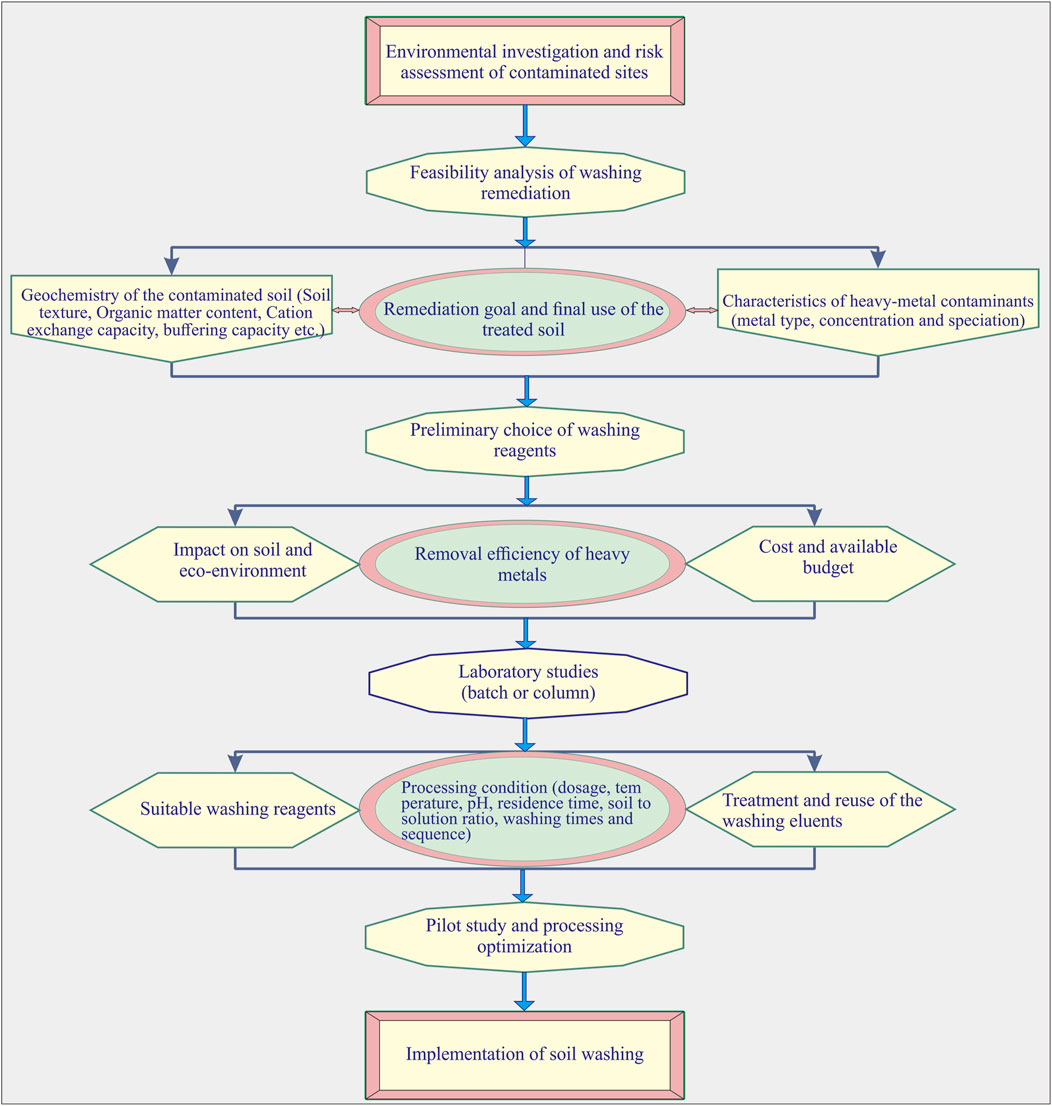
FIGURE 4. Procedure of choosing washing reagents in soil remediation (four steps are included: 1) feasibility analysis of washing remediation; 2) preliminary choice of washing reagents; 3) laboratory study; 4) pilot study and processing optimization).
First, a feasibility analysis of soil washing should be conducted based on the learning of soil geochemistry, the characteristics of heavy-metal contaminates, and the remediation goal which are available through the environmental investigation and risk assessment of contaminated sites. Soil washing is usually supported by limited clay content (usually less than 25%) and low content of humic, as well as low content of Fe, and Ca elements, and calcite from the soil geochemistry aspect. Metal speciation is another important factor that affects soil washing from the aspect of heavy metal characteristics. The fractions most amenable to the removal of metals by washing are associated with exchangeable carbonates and reducible Fe-Mn oxides of soils, while metals associated with organic matter and sulfide bond and residual fractions are difficult to be removed. If the factors of soil geochemistry and heavy-metal characteristics show great negative influence on soil washing, the determination of remediation through washing and the choice of reagents should be cautious. Either auxiliary ways such as heating, ultrasonic, microwave, and electrokinetics are needed to enhance the removal of heavy metals or other remediation techniques should be taken into account to achieve the remediation goal (Zhang et al., 2016; Chang et al., 2020; Choi et al., 2021).
Second, the preliminary choice of washing reagents should be conducted once the washing remediation is determined. During this process, the factors of washing effect, eco-environment impact, and cost are required to be considered a whole to get the suitable options. Multi-criteria decision-analysis (MCDA) based on AHP or ANP can be adopted to assist the preliminary choice of washing reagents.
Third, laboratory studies are required to testify the remediation effect of initially screened reagents, as well as to get the optimum processing conditions such as reagent dosage, temperature, pH, residence time, soil-to-solution ratio, washing times, and washing sequences. Batch tests are usually beneficial to soil washing, while column tests are helpful to soil leaching.
Fourth, a pilot study is necessary so as to optimize the processing conditions which are obtained through laboratory studies before the implementation of soil washing in full scale. Once the washing reagents and the processing conditions are finally determined, soil washing can be implemented to achieve the remediation goals.
To sum up, the selection of washing reagents for the remediation of heavy-metal-contaminated soils is concluded as shown in Figure 5.
4 Summary and Recommendations
Soil washing, which is considered an effective technology that can be used to permanently remove heavy metals from soil, is particularly relevant for the remediation of heavy-metal-contaminated soils. The key point to the success of washing remediation technology is that appropriate washing reagents can be selected. This article identified and discussed the types of washing reagents with their associated characteristics and focused on the selection of washing reagents for extracting heavy metals from soils. The commonly used washing reagents include inorganic reagents, chelating reagents, surfactants, and compound chemical reagents. It is noted that each kind of washing reagent has both advantages and limitations. The review also showed that heavy-metal removal of washing remediation is generally related to the geochemistry of the contaminated soil, metals characteristics, and washing reagents together with the processing conditions, treatment and reuse of the washing effluent, and influence on the environment as well as the cost and available budget. The selection and prioritizing of washing reagents is a balanced process that considers washing effect, environmental impact, and cost-effectiveness. Therefore, the multi-criteria decision-analysis method is suggested to assist the preliminary choice analysis of washing reagents. Furthermore, an integrated four-step procedure for the selection of washing reagents is put forward:
1) Feasibility analysis of soil washing is carried out based on the learning of soil geochemistry, heavy-metal contaminates, and the remediation goal;
2) Preliminary screening of washing reagents is conducted based on the analysis of washing effect, environmental impact, and cost-effectiveness through the MCDA method;
3) Laboratory studies are required to testify the remediation effect of initially selected reagents, as well as to get the optimum processing conditions;
4) A pilot study is necessary so as to optimize the processing conditions before the implementation of soil washing in full scale. Soil washing can then be successfully implemented.
Based on the review, soil washing technology for the removal of heavy metals continues to progress toward using washing reagents that possess the advantage over heavy-metal removal, eco-friendliness, and cost-effectiveness. The perspectives of washing reagents are foreseen as follows:
1) Developing new washing reagents or compound washing reagents which are highly effective on heavy-metal removal but low risk of environmental pollution and low cost. Searching for washing reagents that are capable of being recovered from waste (e.g., dissolved organic matter) would be a promising trend.
2) Research on the improvement of removal ability of the heavy-metal residual fraction is needed since it is difficult to remove the residual fraction from a technical aspect. The research on the combination of washing reagents with other auxiliary ways or other remediation technologies (e.g., bioremediation) may be a solution.
3) Study on the treatment and recycling of washing effluent so as to manage the cost-effectiveness as well as the risk of secondary pollution that might be induced.
4) Artificial intelligence is expected to be applied to assist in the selection of washing reagents and the survey of in situ remediation environments.
Author Contributions
HZ: investigation, data curation, and writing—original draft. YX: conceptualization and writing—review and editing. TK: data curation and methodology. Y-W: software. MS: methodology.
Conflict of Interest
HZ and MS are employed by Sichuan Metallurgical Geological Survey and Design Group Co. Ltd.
The remaining authors declare that the research was conducted in the absence of any commercial or financial relationships that could be construed as a potential conflict of interest.
Publisher’s Note
All claims expressed in this article are solely those of the authors and do not necessarily represent those of their affiliated organizations, or those of the publisher, the editors, and the reviewers. Any product that may be evaluated in this article, or claim that may be made by its manufacturer, is not guaranteed or endorsed by the publisher.
Acknowledgments
The authors wish to thank Sichuan Provincial Bureau of Metallurgical Geological Exploration, China, for the financial support (No. [2018]158). The contributions of all references cited in this article are also acknowledged.
Supplementary Material
The Supplementary Material for this article can be found online at: https://www.frontiersin.org/articles/10.3389/feart.2022.901570/full#supplementary-material
Supplementary Table S1 | Laboratory and field investigations on washing remediation of commonly used washing reagents, together with the recommendation index of heavy-metal removal ability, eco-friendliness, and cost-effectiveness.
References
Alam, M. G. M., Tokunaga, S., and Maekawa, T. (2001). Extraction of Arsenic in a Synthetic Arsenic-Contaminated Soil Using Phosphate. Chemosphere 43, 1035–1041. doi:10.1016/S0045-6535(00)00205-8
Alghanmi, S. I., Al Sulami, A. F., El-Zayat, T. A., Alhogbi, B. G., and Abdel Salam, M. (2015). Acid Leaching of Heavy Metals from Contaminated Soil Collected from Jeddah, Saudi Arabia: Kinetic and Thermodynamics Studies. Int. Soil Water Conservation Res. 3 (3), 196–208. doi:10.1016/j.iswcr.2015.08.002
Arab, F., and Mulligan, C. N. (2018). An Eco-Friendly Method for Heavy Metal Removal from Mine Tailings. Environ. Sci. Pollut. Res. 25, 16202–16216. doi:10.1007/s11356-018-1770-3
Ash, C., Tejnecký, V., Šebek, O., Houška, J., Chala, A. T., Drahota, P., et al. (2015). Redistribution of Cadmium and Lead Fractions in Contaminated Soil Samples Due to Experimental Leaching. Geoderma 241-242, 126–135. doi:10.1016/j.geoderma.2014.11.022
Begum, Z. A., Rahman, I. M. M., Tate, Y., Sawai, H., Maki, T., and Hasegawa, H. (2012). Remediation of Toxic Metal Contaminated Soil by Washing with Biodegradable Aminopolycarboxylate Chelants. Chemosphere 87 (10), 1161–1170. doi:10.1016/j.chemosphere.2012.02.032
Beiyuan, J., Tsang, D. C. W., Valix, M., Baek, K., Ok, Y. S., Zhang, W., et al. (2018). Combined Application of EDDS and EDTA for Removal of Potentially Toxic Elements under Multiple Soil Washing Schemes. Chemosphere 205 (AUG), 178–187. doi:10.1016/j.chemosphere.2018.04.081
Bilgin, M., and Tulun, Ş. (2015). Biodrying for Municipal Solid Waste: Volume and Weight Reduction. Environ. Technol. 36, 1691–1697. doi:10.1080/09593330.2015.1006262
Cao, M., Hu, Y., Sun, Q., Wang, L., Chen, J., and Lu, X. (2013). Enhanced Desorption of PCB and Trace Metal Elements (Pb and Cu) from Contaminated Soils by Saponin and EDDS Mixed Solution. Environ. Pollut. 174, 93–99. doi:10.1016/j.envpol.2012.11.015
Chang, J.-H., Dong, C.-D., Huang, S.-H., and Shen, S.-Y. (20202020). The Study on Lead Desorption from the Real-Field Contaminated Soil by Circulation-Enhanced Electrokinetics (CEEK) with EDTA. J. Hazard. Mater. 383, 121194. doi:10.1016/j.jhazmat.2019.121194
Chen, C., Wang, G., and Wang, J. (2014). Study on the Leaching Effect of HCl and Three Neutral Salt. J. Saf. Environ. (05), 211–216.
Chen, F., and Fu, M. (2012). Study on Remediation of Soils Polluted by Heavy Metals Using Surfactants. Sichuan Environ. 31 (4), 61–64.
Chen, W., Qu, Y., Xu, Z., He, F., Chen, Z., Huang, S., et al. (2017). Heavy Metal (Cu, Cd, Pb, Cr) Washing from River Sediment Using Biosurfactant Rhamnolipid. Environ. Sci. Pollut. Res. Int. 24 (6), 16344–16350. doi:10.1007/s11356-017-9272-2
Chen, X., and Wu, Y. (2018). Remediation Mechanism of Multi-Heavy Metal Contaminated Soil by Using Different Chemical Washing Reagents. Chin. J. Environ. Eng. 12 (10), 2845–2854. doi:10.12030/j.cjee.201804192
Choi, J., Lee, D., and Son, Y. (2021). Ultrasound-assisted Soil Washing Processes for the Remediation of Heavy Metals Contaminated Soils: The Mechanism of the Ultrasonic Desorption. Ultrason. Sonochemistry 74 (2021), 105574. doi:10.1016/j.ultsonch.2021.105574
Cui, J., Xue, W., Yan, Z., Liu, S., and Wang, N. (2013). Repairing of Heavy Metals Contaminated Soil with Different Surfactants. J. Dalian Dalian Polytech. Univ. 32 (04), 279–282. doi:10.19670/j.cnki.dlgydxxb.2013.04.013
Dermont, G., Bergeron, M., Mercier, G., and Richer-Laflèche, M. (2008). Soil Washing for Metal Removal: A Review of Physical/chemical Technologies and Field Applications. J. Hazard. Mater. 152, 1–31. doi:10.1016/j.jhazmat.2007.10.043
Di Palma, L., Ferrantelli, P., Merli, C., and Petrucci, E. (2003). Treatment of the Solution Extracted from Metal Contaminated Soils by Reverse Osmosis and Chemical Precipitation. Ann. Chim. 93, 1005–1011. doi:10.2116/analsci.19.1687
Dikinya, O., and Areola, O. (2010). Comparative Analysis of Heavy Metal Concentration in Secondary Treated Wastewater Irrigated Soils Cultivated by Different Crops. Int. J. Environ. Sci. Technol. 7 (2), 337–346. doi:10.1007/bf03326143
Dong, Y., Lin, H., Zhao, Y., and Gueret Yadiberet Menzembere, E. R. (20212021). Remediation of Vanadium-Contaminated Soils by the Combination of Natural Clay Mineral and Humic Acid. J. Clean. Prod. 279, 123874. doi:10.1016/j.jclepro.2020.123874
Drahota, P., Grösslová, Z., and Kindlová, H. (2014). Selectivity Assessment of an Arsenic Sequential Extraction Procedure for Evaluating Mobility in Mine Wastes. Anal. Chim. Acta 839, 34–43. doi:10.1016/j.aca.2014.06.022
Fedje, K. K., Yillin, L., and Strömvall, A.-M. (2013). Remediation of Metal Polluted Hotspot Areas through Enhanced Soil Washing - Evaluation of Leaching Methods. J. Environ. Manag. 128, 489–496. doi:10.1016/j.jenvman.2013.05.056
Feng, C., Zhang, S., Li, L., Wang, G., Xu, X., Li, T., et al. (2018). Feasibility of Four Wastes to Remove Heavy Metals from Contaminated Soils. J. Environ. Manage 212, 258–265. doi:10.1016/j.jenvman.2018.01.030
Feng, W., Zhang, S., Zhong, Q., Wang, G., Pan, X., Xu, X., et al. (2020). Soil Washing Remediation of Heavy Metal from Contaminated Soil with EDTMP and PAA: Properties, Optimization, and Risk Assessment. J. Hazard. Mater. 381, 120997. doi:10.1016/j.jhazmat.2019.120997
Fernández, E., Jiménez, R., Lallena, A. M., and Aguilar, J. (2004). Evaluation of the Bcr Sequential Extraction Procedure Applied for Two Unpolluted Spanish Soils. Environ. Pollut. 131 (3), 355–364. doi:10.1016/j.envpol.2004.03.013
Finzgar, N., and Lestan, D. (2000). Heap Leaching of Pb and Zn Contaminated Soil Using Ozone/UV Treatment of EDTA Extractants. Chemosphere 63, 1736–1743. doi:10.1016/j.chemosphere.2005.09.015
Gan, W., He, Y., Zhang, X., Shan, Y., Zheng, L., and Lin, Y. (2012). Speciation Analysis of Heavy Metals in Soils Polluted by Electroplating and Effect of Washing to the Removal of the Pollutants. J. Ecol. Rural Environ. 28 (1), 82–87. doi:10.3969/j.issn.1673-4831.2012.01.014
Gitipour, S., Ahmadi, S., Madadian, E., and Ardestani, M. (2016). Soil Washing of Chromium- and Cadmium-Contaminated Sludge Using Acids and Ethylenediaminetetra Acetic Acid Chelating Agent. Environ. Technol. 37 (1), 145–151. doi:10.1080/09593330.2011.597784
Guo, W., Zhang, H., Yin, X., Wang, L., and Wang, Z. (2019). Cadmium Removal from Contaminated Soil inside Non-ferrous Metal Smelter by Washing. Chin. J. Environ. Eng. 13 (2), 389–395. doi:10.1080/15320383.2019.1689919
Guo, X., Wei, Z., Wu, Q., Li, C., Qian, T., and Zheng, W. (2016). Effect of Soil Washing with Only Chelators or Combining with Ferric Chloride on Soil Heavy Metal Removal and Phytoavailability: Field Experiments. Chemosphere 147, 412–419. doi:10.1016/j.chemosphere.2015.12.087
Gusiatin, Z. M., Klik, B., and Kulikowska, D. (2017). Tannic Acid for Remediation of Historically Arsenic-Contaminated Soils. Environ. Technol. 40 (8), 1050–1061. doi:10.1080/09593330.2017.1417490
Gusiatin, Z. M., Radziemska, M., and Żochowska, A. (2019). Sequential Soil Washing with Mixed Biosurfactants Is Suitable for Simultaneous Removal of Multi-Metals from Soils with Different Properties, Pollution Levels and Ages. Environ. Earth Sci. 78, 529. doi:10.1007/s12665-019-8542-3
Gusiatin, Z. M. (2014). Tannic Acid and Saponin for Removing Arsenic from Brownfield Soils: Mobilization, Distribution and Speciation. J. Environ. Sci. 26 (4), 855–864. doi:10.1016/s1001-0742(13)60534-3
Gzar, H. A., Abdul-Hameed, A. S., and Yahya, A. Y. (2014). Extraction of Lead, Cadmium and Nickel from Contaminated Soil Using Acetic Acid. Open J. Soil Sci. 04 (6), 207–214. doi:10.4236/ojss.2014.46023
Hartley, N. R., Tsang, D. C. W., Olds, W. E., and Weber, P. A. (2014). Soil Washing Enhanced by Humic Substances and Biodegradable Chelating Agents. Soil Sediment Contam. Int. J. 23 (6), 599–613. doi:10.1080/15320383.2014.852511
He, J., Tang, J., Liu, T., and Xin, X. (2017). Removal of Heavy Metals in the Presence of the Biodegradable Biosurfactant of Sophorolipid from the Municipal Dewatered Sludge. dwt 89, 225–232. doi:10.5004/dwt.2017.21353
He, Z., Shentu, J., Yang, X., Baligar, V. C., Zhang, T., and Stoffella, P. J. (2015). Heavy Metal Contamination of Soils: Sources, Indicators, and Assessment. J. Environ. Indic. 9, 17–18.
Hong, P. K. A., and Jiang, W. (2005). “Factors in the Selection of Chelating Agents for Extraction of Lead from Contaminated Soil: Effectiveness, Selectivity, and Recoverability,” in Biologechemistry of Chelating Agents. Editors B Nowack, and JM Vanbriesen (Washington DC: American Chemical Society), 421–432. doi:10.1021/bk-2005-0910.ch025
Hou, D., Al-tabbaa, A., Chen, H., and Mamic, I. (2014). Factor Analysis and Structural Equation Modelling of Sustainable Behaviour in Contaminated Land Remediation. J. Clean. Prod. 84, 439–449. doi:10.1016/j.jclepro.2014.01.054
Hou, D., Ding, Z., Li, G., Wu, L., Hu, P., Guo, G., et al. (2018). A Sustainability Assessment Framework for Agricultural Land Remediation in China. Land Degrad. Dev. 29, 1005–1018. doi:10.1002/ldr.2748
Huang, K., Shen, Y., Wang, X., Song, X., Yuan, W., Xie, J., et al. (20212021). Choline-based Deep Eutectic Solvent Combined with EDTA-2Na as Novel Soil Washing Agent for Lead Removal in Contaminated Soil. Chemosphere 279, 130568. doi:10.1016/j.chemosphere.2021.130568
Interstate Technology and Regulatory Council (2003). Characterization and Remediation of Soils at Closed Small Arms Firing Ranges. Washington, DC: Technical/Regulatory Guidelines.
Isoyama, M., and Wada, S. (2007). Remediation of Pb-Contaminated Soils by Washing with Hydrochloric Acid and Subsequent Immobilization with Calcite and Allophanic Soil. J. Hazard. Mater. 143, 636–642. doi:10.1016/j.jhazmat.2007.01.008
Jang, M., Hwang, J. S., Choi, S. I., and Park, J. K. (2005). Remediation of Arsenic-Contaminated Soils and Washing Effluents. Chemosphere 60 (3), 344–354. doi:10.1016/j.chemosphere.2004.12.018
Jang, M., Hwang, J. S., and Choi, S. I. (2007). Sequential Soil Washing Techniques Using Hydrochloric Acid and Sodium Hydroxide for Remediating Arsenic-Contaminated Soils in Abandoned Iron-Ore Mines. Chemosphere 66 (1), 8–17. doi:10.1016/j.chemosphere.2006.05.056
Jez, E., and Lestan, D. (2016). EDTA Retention and Emissions from Remediated Soil. Chemosphere 151, 202–209. doi:10.1016/j.chemosphere.2016.02.088
Jia, J., Huang, Y., Liu, F., Shi, W., Hou, C., and Teng, Y. (2018). Washing Remediation of Mercury Contaminated Soil from Mercury Mining Area. Environ. Prot. Chem. Industry 38 (2), 231–235. doi:10.3969/j.issn.1006-1878.2018.02.020
Jiang, X., Li, X., Zhang, J., Lu, J., Chang, J., and Cui, D. (2012). Study of Citric Acid Extraction and Washing from Different Soil Polluted by Cr. Chin. Agric. Sci. Bull. 28 (02), 278–281. doi:10.1007/s11783-011-0280-z
Jiao, W. (2017). New Biodegradable Chelator GLDA Leaching Restoration of Heavy Metal Contaminated Soil. Changchun: Jilin University.
Juang, R., and Wang, S. W. (2000). Electrolytic Recovery of Binary Metals and EDTA from Strong Complexed Solutions. Water Res. 34, 3179–3185. doi:10.1016/s0043-1354(00)00061-0
Juwarkar, A. A., Nair, A., Dubey, K. V., Singh, S. K., and Devotta, S. (2007). Biosurfactant Technology for Remediation of Cadmium and Lead Contaminated Soils. Chemosphere 68 (10), 1996–2002. doi:10.1016/j.chemosphere.2007.02.027
Kaurin, A., Gluhar, S., Tilikj, N., and Lestan, D. (2020). Soil Washing with Biodegradable Chelating Agents and Edta: Effect on Soil Properties and Plant Growth. Chemosphere 260, 127673. doi:10.1016/j.chemosphere.2020.127673
Ke, X., Li, P., Gong, Z., Yin, W., and Su, D. (2004). Advances in Flushing Agents Used for Remediation of Heavy Metal-Contaminated Soil. Chin. J. Ecol. 23 (5), 145–149. doi:10.13292/J.1000-4890.2004.0171
Kholghi, N., Amani, H., Malekmahmoodi, S., and Amiri, A. (2020). Investigation on Heavy Metal Removal from a Crude Oil Contaminated Soil Using Rhamnolipid Biosurfactant as a New Eco-Friendly Method. Tenside Surfactants Deterg. 57 (6), 515–520. doi:10.3139/113.110710
Kim, C., and Ong, S. K. (1999). Recycling of Lead-Contaminated EDTA Wastewater. J. Hazard. Mat. 69, 273–286. doi:10.1016/s0304-3894(99)00115-6
Klik, B., Gusiatin, Z. M., and Kulikowska, D. (2021). Quality of Heavy Metal-Contaminated Soil before and after Column Flushing with Washing Agents Derived from Municipal Sewage Sludge. Sci. Rep. 11 (1), 15773. doi:10.1038/s41598-021-95441-5
Ko, I., Lee, C. H., Lee, K. P., Lee, S. W., and Kim, K. W. (2005). Remediation of Soil Contaminated with Arsenic, Zinc, and Nickel by Pilot-Scale Soil Washing. Environ. Prog. 25 (1), 39–48. doi:10.1002/ep.10101
Kulikowska, D., Gusiatin, Z. M., Bułkowska, K., and Klik, B. (2015). Feasibility of Using Humic Substances from Compost to Remove Heavy Metals (Cd, Cu, Ni, Pb, Zn) from Contaminated Soil Aged for Different Periods of Time. J. Hazard. Mater. 300, 882–891. doi:10.1016/j.jhazmat.2015.08.022
Kwak, J. I., Nam, S.-H., Kim, S. W., Bajagain, R., Jeong, S.-W., and An, Y.-J. (2019). Changes in Soil Properties after Remediation Influence the Performance and Survival of Soil Algae and Earthworm. Ecotoxicol. Environ. Saf. 174, 189–196. doi:10.1016/j.ecoenv.2019.02.079
Lee, J.-C., Kim, E. J., and Baek, K. (2017). Synergistic Effects of the Combination of Oxalate and Ascorbate on Arsenic Extraction from Contaminated Soils. Chemosphere 168, 1439–1446. doi:10.1016/j.chemosphere.2016.11.155
Lehigh, G. R., Wells, E. C., and Diaz, D. (2020). Evidence-informed Strategies for Promoting Equitability in Brownfields Redevelopment. J. Environ. Manag. 261, 110150. doi:10.1016/j.jenvman.2020.110150
Leˇstan, D., Luo, C., and Li, X. (2008). The Use of Chelating Agents in the Remediation of Metal-Contaminated Soils: A Review. Environ. Pollut. 153, 3–13. doi:10.1016/j.envpol.2007.11.015
Li, D., Hao, X., Zhou, D., and Zhan, X. (2011). Remediation of Chromium Residue Contaminated Soil Using a Washing Technology. J. Agro-Environment Sci. 30 (12), 2451–2457.
Li, H., and Pan, G. (2015). Simultaneous Removal of Harmful Algal Blooms and Microcystins Using Microorganism- and Chitosan-Modified Local Soil. Environ. Sci. Technol. 49 (10), 6249–6256. doi:10.1021/acs.est.5b00840
Lim, M., and Kim, M.-J. (2013). Reuse of Washing Effluent Containing Oxalic Acid by a Combined Precipitation-Acidification Process. Chemosphere 90, 1526–1532. doi:10.1016/j.chemosphere.2012.08.047
Lin, H. K., Man, X. D., and Walsh, D. E. (20012001). Lead Removal via Soil Washing and Leaching. Jom 53, 22–25. doi:10.1007/s11837-001-0007-x
Lin, K. (2009). Research on the Leaching Characteristics of Mercury and its Remediation by Washing of Serious Polluted Soil, a Case in Qingzhen, Guizhou Province (Guiyang, China: Guizhou University). Doctoral dissertation.
Lin, Y.-T., Chien, Y.-C., and Liang, C. (2012). A Laboratory Treatability Study for Pilot-Scale Soil Washing of Cr, Cu, Ni, and Zn Contaminated Soils. Environ. Prog. Sustain. Energy 31 (3), 351–360. doi:10.1002/ep.10555
Liu, C.-C., and Chen, G.-B. (2013). Reclamation of Cadmium-Contaminated Soil Using Dissolved Organic Matter Solution Originating from Wine-Processing Waste Sludge. J. Hazard. Mater. 244-245, 645–653. doi:10.1016/j.jhazmat.2012.10.060
Liu, G. H., Ren, J., Gang, H. U., Qin, S., and Fan, C. W. (2018). Effects of Low Molecular Organic Acids on the Removing of pb,cd in Calcareous Soils. Hubei Agricultural Sciences 057 (006), 43–47.
Liu, J., Xue, J., and Wei, X. (2019). Research Progress of Surfactant Washing for Remediation of Heavy Metal Pollution in Soil. Chin. J. Soil Sci. 50 (1), 240–245.
Liu, J., Zhao, L., Liu, Q., Li, J., Qiao, Z., Sun, P., et al. (2021). A Critical Review on Soil Washing during Soil Remediation for Heavy Metals and Organic Pollutants. Int. J. Environ. Sci. Technol. 19, 601–624. doi:10.1007/s13762-021-03144-1
Liu, L., Hu, S. P., Chen, Y. X., and Li, H. (2010). Feasibility of Washing as a Remediation Technology for the Heavy Metals-Polluted Soils Left by Chemical Plant. Ying Yong Sheng Tai Xue Bao 21 (6), 1537–1541.
Liu, L., Li, W., Song, W., and Guo, M. (2018). Remediation Techniques for Heavy Metal-Contaminated Soils: Principles and Applicability. Sci. Total Environ. 633, 206–219. doi:10.1016/j.scitotenv.2018.03.161
Liu, P. Y., Liu, H., Li, Y. J., and Dong, C. X. (2014). Remediation of Arsenic Contaminated Soils and Treatment of Washing Effluent Using Calcined Mn-Fe Layered Double Hydroxide. Amr 955-959, 2014–2021. doi:10.4028/www.scientific.net/amr.955-959.2014
Liu, R., Liu, J., Zhang, J., Yan, X., and Yuan, C. (2018). Two Carboxylic Ligands Controlled Coordination Polymers Based on Cd II Ion. J. Mol. Struct. 1164 (6), 45–49. doi:10.1016/j.molstruc.2018.03.036
Luo, C., Wang, S., Wang, Y., Yang, R., Zhang, G., and Shen, Z. (2015). Effects of EDDS and Plant-Growth-Promoting Bacteria on Plant Uptake of Trace Metals and PCBs from E-Waste-Contaminated Soil. J. Hazard. Mater. 286, 379–385. doi:10.1016/j.jhazmat.2015.01.010
Maity, J. P., Huang, Y. M., Hsu, C.-M., Wu, C.-I., Chen, C.-C., Li, C.-Y., et al. (2013). Removal of Cu, Pb and Zn by Foam Fractionation and a Soil Washing Process from Contaminated Industrial Soils Using Soapberry-Derived Saponin: a Comparative Effectiveness Assessment. Chemosphere 92 (10), 1286–1293. doi:10.1016/j.chemosphere.2013.04.060
Makino, T., Sugahara, K., Sakurai, Y., Takano, H., Kamiya, T., Sasaki, K., et al. (2006). Remediation of Cadmium Contamination in Paddy Soils by Washing with Chemicals: Selection of Washing Chemicals. Environ. Pollut. 144 (1), 2–10. doi:10.1016/j.envpol.2006.01.017
Mehrabi, N., Abdul Haq, U. F., Reza, M. T., and Aich, N. (2020). Application of Deep Eutectic Solvent for Conjugation of Magnetic Nanoparticles onto Graphene Oxide for Lead(II) and Methylene Blue Removal. J. Environ. Chem. Eng. 8 (2020), 104222. doi:10.1016/j.jece.2020.104222
Mohamed, M. A., Efligenir, A., Husson, J., Persello, J., Fievet, P., and Fatin-Rouge, N. (2013). Extraction of Heavy Metals from a Contaminated Soil by Reusing Chelating Agent Solutions. J. Environ. Chem. Eng. 1 (3), 363–368. doi:10.1016/j.jece.2013.05.015
Moon, D. H., Lee, J.-R., Wazne, M., and Park, J.-H. (2012). Assessment of Soil Washing for Zn Contaminated Soils Using Various Washing Solutions. J. Industrial Eng. Chem. 18 (2), 822–825. doi:10.1016/j.jiec.2011.11.137
Morin, G., Ostergren, J. D., Juillot, F., Ildefonse, P., Calas, G., and Brown, G. E. (1999). XAFS Determination of the Chemical Form of Lead in Smelter-Contaminated Soils and Mine Tailings; Importance of Adsorption Processes. Am. Mineralogist 84, 420–434. doi:10.2138/am-1999-0327
Moutsatsou, A., Gregou, M., Matsas, D., and Protonotarios, V. (2005). Washing as a Remediation Technology Applicable in Soils Heavily Polluted by Mining-Metallurgical Activities. Chemosphere 63 (10), 1632–1640. doi:10.1016/j.chemosphere.2005.10.015
Mukhopadhyay, S., Mukherjee, S., Adnan, N. F., Hayyan, A., Hayyan, M., Hashim, M. A., et al. (2016). Ammonium-based Deep Eutectic Solvents as Novel Soil Washing Agent for Lead Removal. Chem. Eng. J. 294, 316–322. doi:10.1016/j.cej.2016.02.030
Naseri-Rad, M., Berndtsson, R., Persson, K. M., and Nakagawa, K. (2020). INSIDE: An Efficient Guide for Sustainable Remediation Practice in Addressing Contaminated Soil and Groundwater. Sci. Total Environ. 740 (2020), 139879. doi:10.1016/j.scitotenv.2020.139879
Nedwed, T., and Clifford, D. A. (2000). Feasibility of Extracting Lead from Lead Battery Recycling Site Soil Using High-Concentration Chloride Solutions. Environ. Prog. 19, 197–206. doi:10.1002/ep.670190312
Neilson, J. W., Artiola, J. F., and Maier, R. M. (2003). Characterization of Lead Removal from Contaminated Soils by Nontoxic Soil‐Washing Agents. J. Environ. Qual. 32 (3), 899–908. doi:10.2134/jeq2003.8990
Oh, S., Bade, R., Lee, H., Choi, J., and Shin, W. S. (2015). Risk Assessment of Metal(loid)-Contaminated Soils before and after Soil Washing. Environ. Earth Sci. 74 (1), 703–713. doi:10.1007/s12665-015-4075-6
Palma, L. D., and Ferrantelli, P. (2005). Copper Leaching from a Sandy Soil: Mechanism and Parameters affecting EDTA Extraction. J. Hazard. Mater. 122 (1-2), 85–90. doi:10.1016/j.jhazmat.2005.03.010
Paria, S. (2008). Surfactant-enhanced Remediation of Organic Contaminated Soil and Water. Adv. Colloid Interface Sci. 138, 24–58. doi:10.1016/j.cis.2007.11.001
Peters, R. W. (1999). Chelant Extraction of Heavy Metals from Contaminated Soils. J. Hazard. Mater. 66, 151–210. doi:10.1016/s0304-3894(99)00010-2
Pociecha, M., and Lestan, D. (2010). Using Electrocoagulation for Metal and Chelant Separation from Washing Solution after Edta Leaching of Pb, Zn and Cd Contaminated Soil. J. Hazard. Mat. 174 (1-3), 670–678. doi:10.1016/j.jhazmat.2009.09.103
Pociecha, M., and Lestan, D. (2009). EDTA Leaching of Cu Contaminated Soil Using Electrochemical Treatment of the Washing Solution. J. Hazard. Mater. 165, 533–539. doi:10.1016/j.jhazmat.2008.10.006
Qi, X., Xu, X., Zhong, C., Jiang, T., Wei, W., and Song, X. (2018). Removal of Cadmium and Lead from Contaminated Soils Using Sophorolipids from Fermentation Culture of Starmerella Bombicola Cgmcc 1576 Fermentation. Int. J. Environ. Res. Public Health 15 (11), 2334. doi:10.3390/ijerph15112334
Rahman, I. M. M., Hossain, M. M., Begum, Z. A., Rahman, M. A., and Hasegawa, H. (2010). “Eco-environmental Consequences Associated with Chelant-Assisted Phyto-Remediation of Metal-Contaminated Soil,” in Handbook of Phytoremediation. Editor I. A. Golubev (New York: Nova Science Publishers), 709–722.
Ramamurthy, A., and Schalchian, H. (2013). Surfactant Assisted Removal of Cu(II), Cd(II) and Pb(II) from Contaminated Soils. Environ. Prot. Eng. 39 (3), 87–99. doi:10.37190/epe130307
Rashad, M., Assaad, F. F., and Shalaby, E. A. (2013). Effect of Dissolved Organic Matter Derived from Waste Amendments on the Mobility of Inorganic Arsenic (Iii) in the Egyptian Alluvial Soil. Int. J. Energy & Environ. 4 (4), 677–686.
Reddy, K. R., Danda, S., Yukselen-Aksoy, Y., and Al-Hamdan, A. Z. (2010). Sequestration of Heavy Metals in Soils from Two Polluted Industrial Sites: Implications for Remediation. Land Contam. Reclam. 18 (1), 13–23. doi:10.2462/09670513.909
Rui, D., Wu, Z., Wu, Y., Chen, X., Liu, J., and Ding, J. (2018). Synergistic Remediation of Heavy Metal Cd and Pb Contaminated Clay by Freeze-Thaw and Chemical Washing. Trans. Chin. Soc. Agric. Eng. Trans. CSAE) 34 (23), 199–205. doi:10.11975/j.issn.1002-6819.2018.23.025
Saponaro, S., Bonomo, L., Barbafieri, M., and Petruzzelli, G. (2002). Soil Washing Feasibility at a Manufacturing Gas Plant Site. Soil Sediment Contam. An Int. J. 11 (5), 751–767. doi:10.1080/20025891107078
Satyro, S., Race, M., Di Natale, F., Erto, A., Guida, M., and Marotta, R. (2016). Simultaneous Removal of Heavy Metals from Field-Polluted Soils and Treatment of Soil Washing Effluents through Combined Adsorption and Artificial Sunlight-Driven Photocatalytic Processes. Chem. Eng. J. 283, 1484–1493. doi:10.1016/j.cej.2015.08.039
Satyro, S., Race, M., Marotta, R., Dezotti, M., Guida, M., and Clarizia, L. (2017). Photocatalytic Processes Assisted by Artificial Solar Light for Soil Washing Effluent Treatment. Environ. Sci. Pollut. Res. 24, 6353–6360. doi:10.1007/s11356-016-6431-9
Singh, A. K., and Cameotra, S. S. (2013). Efficiency of Lipopeptide Biosurfactants in Removal of Petroleum Hydrocarbons and Heavy Metals from Contaminated Soil. Environ. Sci. Pollut. Res. 20 (10), 7367–7376. doi:10.1007/s11356-013-1752-4
Stevenson, F. J. (1994). Humus Chemistry, Genesis, Composition, Reactions. 2nd edn. Canada, USA: John Wiley & Sons, 512.
Subirés-Muñoz, J. D., García-Rubio, A., Vereda-Alonso, C., Gómez-Lahoz, C., Rodríguez-Maroto, J. M., García-Herruzo, F., et al. (2011). Feasibility Study of the Use of Different Extractant Agents in the Remediation of a Mercury Contaminated Soil from Almaden. Sep. Purif. Technol. 79, 151–156. doi:10.1016/j.seppur.2011.01.032
Sun, H., Wang, H., Qi, J., Shen, L., and Lian, X. (2011). “Study on Surfactants Remediation in Heavy Metals Contaminated Soils,” in 2011 International Symposium on Water Resource and Environmental Protection (Xi'an: IEEE), 1862–1865. doi:10.1109/iswrep.2011.5893616
Sun, T., Lu, K., and Wang, H. (2015). Advance in Washing Technology for Remediation of Heavy Contaminated Soils: Effect of Eluants and Washing Conditions. J. Zhejiang A F Univ. 32 (1), 140–149. doi:10.11833/j.issn.2095-0756.2015.01.021
Tampouris, S., Papassiopi, N., and Paspaliaris, I. (2001). Removal of Contaminant Metals from Fine Grained Soils, Using Agglomeration, Chloride Solutions and Pile Leaching Techniques. J. Hazard. Mater. 84, 297–319. doi:10.1016/s0304-3894(01)00233-3
Tao, Y. (2013). The Selection of Washing Reagents for Soils Polluted by Cadmium and Chromium. Changsha: Central South University.
Tejowulan, R. S., and Hendershot, W. H. (1998). Removal of Trace Metals from Contaminated Soils Using EDTA Incorporating Resin Trapping Techniques. Environ. Pollut. 103, 135–142. doi:10.1016/S0269-7491(98)00080-3
Tessier, A., Campbell, P. G. C., and Bisson, M. (1979). Sequential Extraction Procedure for the Speciation of Particulate Trace Metals. Anal. Chem. 51, 844–851. doi:10.1021/ac50043a017
Udovic, M., and Lestan, D. (2010). Redistribution of Residual Pb, Zn, and Cd in Soil Remediated with Edta Leaching and Exposed to Earthworms (eisenia Fetida). Environ. Technol. 31 (6), 655–669. doi:10.1080/09593331003610907
USEPA (19941994). A Literature Review Summary of Metals Extraction Processes Used to Remove Lead from Soils, Project Summary. Cincinnati, OH: Office of Research and Development. EPA/600/SR-94/006.
USEPA (2004). Summary of Status Report Updates, Changes, and Deletions, Appendix D of Treatment Technologies for Site Clean up: Annual Status Report. 11th ed. Washington, DC: Office of Solid Waste and Emergency Response. EPA 542-R-03-009.
USEPA (2005). Superfund Programs’ Proposed Plan, Myers Property Site. New York, NY: USEPA. Region II. July, 2005.
Vandevivere, P. C., Saveyn, H., and Verstraete, W. (2001). Biodegradation of metal--[s,s]-edds complexes. Environ. Sci. Technol.
Voglar, D., and Lestan, D. (2012). Pilot-scale Washing of Metal Contaminated Garden Soil Using EDTA. J. Hazard. Mater. 215-216, 32–39. doi:10.1016/j.jhazmat.2012.02.022
Wahla, I. H., and Kirkham, M. B. (2008). Heavy Metal Displacement in Salt-Water-Irrigated Soil during Phytoremediation. Environ. Pollut. 155 (2), 271–283. doi:10.1016/j.envpol.2007.11.020
Wang, G., Pan, X., Zhang, S., Zhong, Q., Zhou, W., Zhang, X., et al. (2020). Remediation of Heavy Metal Contaminated Soil by Biodegradable Chelator-Induced Washing: Efficiencies and Mechanisms. Environ. Res. 186, 109554. doi:10.1016/j.envres.2020.109554
Wang, G., Zhang, S., Xu, X., Zhong, Q., Zhang, C., Jia, Y., et al. (2016). Heavy Metal Removal by GLDA Washing: Optimization, Redistribution, Recycling, and Changes in Soil Fertility. Sci. Total Environ. 569-570, 557–568. doi:10.1016/j.scitotenv.2016.06.155
Wang, G., Zhang, S., Zhong, Q., Xu, X., Li, T., Jia, Y., et al. (2018). Effect of Soil Washing with Biodegradable Chelators on the Toxicity of Residual Metals and Soil Biological Properties. Sci. Total Environ. 625, 1021–1029. doi:10.1016/j.scitotenv.2018.01.019
Wang, Q., Liu, X., Wang, C., Zhang, X., Li, H., Chen, T., et al. (2015). Solubilization Effect of Surfactants on Morphological Transformation of Cadmium and Pyrene in Cocontaminated Soils. Water Air Soil Pollut. 226, 1–9. doi:10.1007/s11270-015-2409-3
Wang, S., and Mulligan, C. N. (2004). Rhamnolipid Foam Enhanced Remediation of Cadmium and Nickel Contaminated Soil. Water Air & Soil Pollut. 157 (1-4), 315–330. doi:10.1023/b:wate.0000038904.91977.f0
Wang, W. (2013). Study on Leaching Technology of Zn, Pb, Cu Contaminated Soils. Changsha: Central South University.
Wang, Y., Fang, Z., Liang, B., and Tsang, E. P. (2014). Remediation of Hexavalent Chromium Contaminated Soil by Stabilized Nanoscale Zero-Valent Iron Prepared from Steel Pickling Waste Liquor. Chem. Eng. J. 247, 283–290. doi:10.1016/j.cej.2014.03.011
Wasay, S. A., Barrington, S., and Tokunaga, S. (2001). Organic Acids for the In Situ Remediation of Soils Polluted by Heavy Metals: Soil Flushing in Columns. Water, Air, & Soil Pollut. 127, 301–314. doi:10.1023/a:1005251915165
Wasay, S. A., Barrington, S., and Tokunaga, S. (1988). Remediation of Soils Polluted by Heavy Metals Using Salts of Organic Acids and Chelating Agents. Environ. Technol. 19, 369–380.
Wei, M., Chen, J., and Wang, X. (2016). Removal of Arsenic and Cadmium with Sequential Soil Washing Techniques Using Na2EDTA, Oxalic and Phosphoric Acid: Optimization Conditions, Removal Effectiveness and Ecological Risks. Chemosphere 156, 252–261. doi:10.1016/j.chemosphere.2016.04.106
Wu, L., Xian, S., Kong, D., Ou, M., and Deng, Q. (2016). Remediation of Cd Polluted Soil by Co-leaching of Tannic Acid and Citric Acid. Environ. Eng. (08), 178–181. doi:10.13205/j.hjgc.201608037
Wuana, R. A., and Okieimen, F. E. (2011). Heavy Metals in Contaminated Soils: A Review of Sources, Chemistry, Risks and Best Available Strategies for Remediation. ISRN Ecol. 2011, 1–20. doi:10.5402/2011/402647
Wuana, R. A., Okieimen, F. E., and Imborvungu, J. A. (2010). Removal of Heavy Metals from a Contaminated Soil Using Organic Chelating Acids. Int. J. Environ. Sci. Technol. 7 (3), 485–496. doi:10.1007/bf03326158
Xia, W., Li, X., Gao, H., Huang, B., Zhang, H., Liu, Y., et al. (2009). Influence Factors Analysis of Removing Heavy Metals from Multiple Metal-Contaminated Soils with Different Extractants. J. Cent. South Univ. Technol. 16 (01), 108–111. doi:10.1007/s11771-009-0018-2
Xiao, R., Ali, A., Wang, P., Li, R., Tian, X., and Zhang, Z. (2019). Comparison of the Feasibility of Different Washing Solutions for Combined Soil Washing and Phytoremediation for the Detoxification of Cadmium (Cd) and Zinc (Zn) in Contaminated Soil. Chemosphere 230, 510–518. doi:10.1016/j.chemosphere.2019.05.121
Xiong, H., Wang, X., Ma, J., Xia, F., Han, C., Pei, Y., et al. (2016). Discussion on Available Condition of Ex-Situ Soil Washing for Remediation of Chromiun-Contaminated Soil. Environ. Eng. 34 (11), 155–161. doi:10.13205/j.hjgc.201611033
Xu, F., Liu, N., Liu, J., Liu, H., and Chen, T. (2016). Chromium Leaching by Sulfuric Acid/sodium Sulfate Composite Eluent from Chromium Slag. Environ. Eng. 34 (9), 155–159. doi:10.13205/j.hjgc.201609034
Xu, Z., Xu, D., Guo, S., Qiu, X., and Li, F. (2014). Combined Leaching of Heavy Metals in Soil by Citric Acid and Saponin. J. Agro-Environment Sci. 33 (8), 1519–1525. doi:10.11654/jaes.2014.08.008
Yan, J., Mo, C., Wang, D., and Xie, Y. (2015). Removal of Chromium from Soil by Saponin. J. Guangxi Univ. (Nat Sci Ed) 40 (3), 558–564. doi:10.13624/j.cnki.issn.1001-7445.2015.0558
Yang, J.-S., Hwang, J.-M., Baek, K., and Kwon, M. J. (2013). Soil Washing and Effluent Treatment for Contaminated Soil with Toxic Metals. Korean Chem. Eng. Res. 51 (6), 745–754. doi:10.9713/kcer.2013.51.6.745
Yang, J.-S., Lee, J. Y., Baek, K., Kwon, T.-S., and Choi, J. (2009). Extraction Behavior of as, Pb, and Zn from Mine Tailings with Acid and Base Solutions. J. Hazard. Mater. 171, 443–451. doi:10.1016/j.jhazmat.2009.06.021
Yang, J., and Mosby, D. (2006). Field Assessment of Treatment Efficacy by Three Methods of Phosphoric Acid Application in Lead-Contaminated Urban Soil. Sci. Total Environ. 366 (1), 136–142. doi:10.1016/j.scitotenv.2005.09.050
Yang, J., Wang, J., Li, J., and Li, H. (2016). Discussion on Heavy Metals Removal Efficiency in Clay by Soil Washing. Environ. Sci. Technol. 39 (S2), 294–298.
Yang, X., Bai, L., Deng, L., Chen, T., Pan, C., and Fan, H. (2014). Research on Drip Washing and Rehabilitation of Soil in Polluted Areas of Electroplate. China Environ. Prot. Ind. (9), 50–52. doi:10.3969/j.issn.1006-5377.2014.09.010
Ye, M., Sun, M., Wan, J., Fang, G., Li, H., Hu, F., et al. (2015). Evaluation of Enhanced Soil Washing Process with Tea Saponin in a Peanut Oil-Water Solvent System for the Extraction of PBDEs/PCBs/PAHs and Heavy Metals from an Electronic Waste Site Followed by Vetiver Grass Phytoremediation. J. Chem. Technol. Biotechnol. 90 (11), 2027–2035. doi:10.1002/jctb.4512
Yoo, J. C., Beiyuan, J., Wang, L., Tsang, D. C. W., Baek, K., Bolan, N. S., et al. (2018). A Combination of Ferric nitrate/EDDS-Enhanced Washing and Sludge-Derived Biochar Stabilization of Metal-Contaminated Soils. Sci. Total Environ. 616-617, 572–582. doi:10.1016/j.scitotenv.2017.10.310
Zhai, X., Li, Z., Huang, B., Luo, N., Huang, M., Zhang, Q., et al. (2018). Remediation of Multiple Heavy Metal-Contaminated Soil through the Combination of Soil Washing and In Situ Immobilization. Sci. Total Environ. 635, 92–99. doi:10.1016/j.scitotenv.2018.04.119
Zhan, H., Jiang, Y., Wang, B., Zhu, K., and Zhao, B. (2012). Study on Removal Efficiencies and Mechanism of Heavy Metal from Sewage Irrigated Soils by Saponin Compared with Commonly Used Washing Agents. Int. J. Sci. Eng. Res. 3, 1–9.
Zhang, H., Gao, Y., and Xiong, H. (2017). Removal of Heavy Metals from Polluted Soil Using the Citric Acid Fermentation Broth: a Promising Washing Agent. Environ. Sci. Pollut. Res. 24 (10), 9506–9514. doi:10.1007/s11356-017-8660-y
Zhang, S. (2013). Study on Washing Remediation of Cadmium, Lead Contaminated Calcareous Soil (Changsha, China: Central South University). Doctoral dissertation.
Zhang, S., Wen, J., Hu, Y., Fang, Y., Zhang, H., Xing, L., et al. (2019). Humic Substances from Green Waste Compost: an Effective Washing Agent for Heavy Metal (Cd, Ni) Removal from Contaminated Sediments. J. Hazard. Mater. 366, 210–218. doi:10.1016/j.jhazmat.2018.11.103
Zhang, W., Xu, F., Yang, Y., Huang, H., Jiang, W., Tian, L., et al. (2016). Process Analysis and Design Suggestion on Ex-Situ Washing Technology for Heavy Metal Contaminated Soil. Environ. Eng. 34 (12), 177–182. doi:10.13205/j.hjgc.201612037
Zhang, Y., Dai, Y., Wang, Y., Huang, X., Xiao, Y., and Pei, Q. (2021a). Hydrochemistry, Quality and Potential Health Risk Appraisal of Nitrate Enriched Groundwater in the Nanchong Area, Southwestern China. Sci. Total Environ. 784, 147186. doi:10.1016/j.scitotenv.2021.147186
Zhang, Y., He, Z., Tian, H., Huang, X., Zhang, Z., Liu, Y., et al. (2021b). Hydrochemistry Appraisal, Quality Assessment and Health Risk Evaluation of Shallow Groundwater in the Mianyang Area of Sichuan Basin, Southwestern China. Environ. Earth Sci. 80 (17), 576. doi:10.1007/s12665-021-09894-y
Zhou, D., Zheng, C., and Chen, H. (2002). Interaction of Cadmium and Citric Acid, Edta in Several Kinds of Soil. Acta Pedol. Sin. 39 (1), 29–36. doi:10.11766/trxb200004040105
Zhou, F., Zhong, L., and Yang, S. (2017). Leaching Effects of Compound Eluent on Cadmium Contaminated Soil. J. Anhui Agric. Sci. 45 (23), 52–54. doi:10.3969/j.issn.0517-6611.2017.23.018
Zhou, J., Liang, Z., Cai, X., Mao, Y., and Zhou, M. (2016). Chemical Leaching Remediation of Soil Contaminated by Heavy Metals: a Review. Environ. Prot. Technol. 22 (4), 54–57.
Zhu, G. X., Guo, Q. J., Yang, J. X., Zhang, H. Z., Wei, R. F., Wang, C. Y., et al. (2013). Research on the Effect and Technique of Remediation for Multi-Metal Contaminated Tailing Soils. Huan Jing Ke Xue 34 (9), 3690–3696.
Zhu, Q., Shao, C., Zhang, Z., and Wen, X. (2010). Saponin Biosurfactant-Enhanced Flushing for the Removal of Heavy Metals from Soils. Acta Sci. Circumstantiae 30 (12), 2491–2498. doi:10.1631/jzus.A1000244
Zimmerman, A. J., and Weindorf, D. C. (2010). Heavy Metal and Trace Metal Analysis in Soil by Sequential Extraction: a Review of Procedures. Int. J. Anal. Chem. 2010 (3-4), 387803. doi:10.1155/2010/387803
Keywords: soil washing, heavy metals, affecting factors, multi-criteria decision analysis, selection procedure
Citation: Zhang H, Xu Y, Kanyerere T, Wang Y-s and Sun M (2022) Washing Reagents for Remediating Heavy-Metal-Contaminated Soil: A Review. Front. Earth Sci. 10:901570. doi: 10.3389/feart.2022.901570
Received: 22 March 2022; Accepted: 20 April 2022;
Published: 01 June 2022.
Edited by:
Yunhui Zhang, Southwest Jiaotong University, ChinaReviewed by:
Gang Yang, Sichuan Agricultural University, ChinaLiu Shengyu, Chengdu University of Information Technology, China
Copyright © 2022 Zhang, Xu, Kanyerere, Wang and Sun. This is an open-access article distributed under the terms of the Creative Commons Attribution License (CC BY). The use, distribution or reproduction in other forums is permitted, provided the original author(s) and the copyright owner(s) are credited and that the original publication in this journal is cited, in accordance with accepted academic practice. No use, distribution or reproduction is permitted which does not comply with these terms.
*Correspondence: Heng Zhang, aGVuZ3oxOTgyQDE2My5jb20=, Mzc3Mjg1OEBteXV3Yy5hYy56YQ==