- Department of Atmospheric Sciences, School of Ocean and Earth Science and Technology, University of Hawaiʻi at Mānoa, Honolulu, HI, United States
The El Niño Southern Oscillation (ENSO) phenomenon, manifested by the great swings of large-scale sea surface temperature (SST) anomalies over the equatorial central to eastern Pacific oceans, is a major source of interannual global shifts in climate patterns and weather activities. ENSO’s SST anomalies exhibit remarkable spatiotemporal pattern diversity (STPD), with their spatial pattern diversity dominated by Central Pacific (CP) and Eastern Pacific (EP) El Niño events and their temporal diversity marked by different timescales and intermittency in these types of events. By affecting various Earth system components, ENSO and its STPD yield significant environmental, ecological, economic, and societal impacts over the globe. The basic dynamics of ENSO as a canonical oscillator generated by coupled ocean–atmosphere interactions in the tropical Pacific have been largely understood. A minimal simple conceptual model such as the recharge oscillator paradigm provides means for quantifying the linear and nonlinear seasonally modulated growth rate and frequency together with ENSO’s state-dependent noise forcing for understanding ENSO’s amplitude and periodicity, boreal winter-time phase locking, and warm/cold phase asymmetry. However, the dynamical mechanisms explaining the key features of ENSO STPD associated with CP and EP events remain to be better understood. This article provides a summary of the recent active research on the dynamics of ENSO STPD together with discussions on challenges and outlooks for theoretical, diagnostic, and numerical modeling approaches to advance our understanding and modeling of ENSO, its STPD, and their broad impacts.
Introduction of ENSO STPD
ENSO is the most prominent phenomenon of year-to-year fluctuations in the global climate system. It interacts strongly with the rest of the weather–climate continuum involving timescales ranging from synoptic through multidecadal to centennial, affecting not only the global climate system but also marine and terrestrial ecosystems, fisheries, human health, and other societal and economic aspects of the Earth system (cf. McPhaden et al., 2006; Cashin et al., 2017; Timmermann et al., 2018; Boucharel et al., 2021). The primary dynamical mechanisms for ENSO’s basic features as a canonical oscillator have been extensively investigated in terms of a leading coupled ocean–atmosphere model of the tropical Pacific essentially described by the simple conceptual delayed oscillator/recharge oscillator paradigm (Cane and Zebiak, 1985; Suarez and Schopf, 1988; Battisti and Hirst, 1989; Philander, 1990; Jin and Neelin, 1993; Neelin and Jin, 1993; Jin, 1997a, 1997b; Neelin et al., 1998; Wang and Picaut, 2004; Jin et al., 2020). Different ENSO-like behaviors observed in nature and simulated in various coupled models were viewed qualitatively as the result of the sensitive dependence of the leading coupled linear mode to variations in the climate background state (Jin and Neelin, 1993; An and Jin, 2000; Fedorov and Philander, 2000, 2001).
In the past 2 decades, new layers of complexity of the ENSO phenomenon were identified with important implications for ENSO’s impacts and predictability (Timmermann et al., 2018). It has been widely recognized that the observed ENSO pattern diversity and temporal complexity tend to manifest roughly in two dominant ENSO types known as central Pacific (CP) and eastern Pacific (EP) El Niño events (Larkin and Harrison, 2005a, 2005b; Ashok et al., 2007; Weng et al., 2007; Kug et al., 2009; Kao and Yu, 2009), as exemplified by two typical EP and CP El Niño events shown in Figures 1A, B. Associated with the sea surface temperature (SST) anomaly patterns, there is a westward shift of the slanted boundary marking the transition region between positive and negative equatorial ocean subsurface temperature anomalies (Figures 1C, D), suggesting that different upper thermocline thermal stratification responses may contribute to different dynamical feedback strengths during CP and EP El Niño events (Zhao et al., 2021a). The pacing or periodicity is different as well, with CP events tending to occur every ∼2–3 years (during the epoch of the 2000s, for example), whereas EP events tend to exhibit slower pacing with occurrences every ∼4–5 years, as shown in Figure 1E. Moreover, there is a modulated intermittency with active/less-active epochs with relatively more/fewer CP over EP events. A detailed and informative overview of the key characteristics of ENSO STPD can be found in the work of Capotondi et al. (2020).
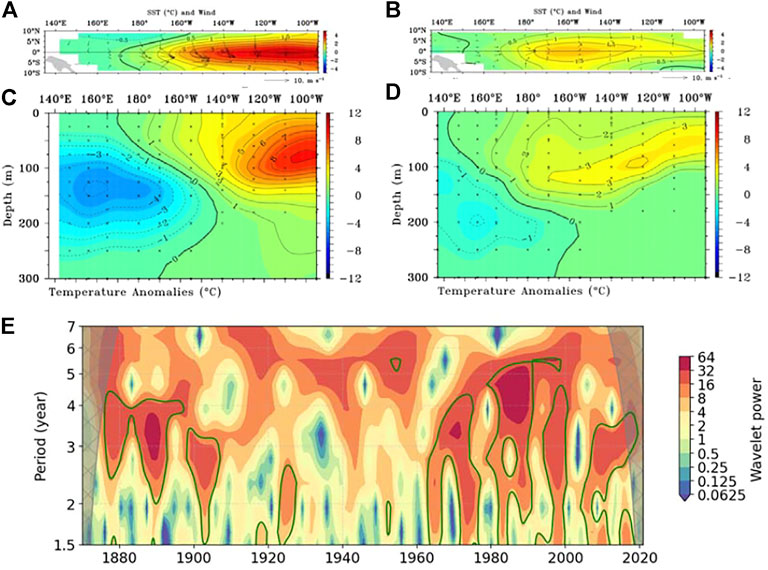
FIGURE 1. November–December–January mean SST anomalies (A,B) and subsurface temperature anomalies at the equator (2°S-2°N) (C,D) during the 1997/98 EP (A,C) and 2009/10 CP (B,D) El Niño events from NOAA/PMEL (https://www.pmel.noaa.gov/tao/drupal/disdel/). (E) Wavelet power spectrum of the normalized time series of Niño-3.4 SST anomalies from HadISST (Rayner et al., 2003). The green contour encloses regions of greater than 95% confidence level tested against red noise. Cross-hatched regions on either end indicate the “cone of influence” where edge effects become important.
The observed key features of diversity in patterns and temporal evolution of ENSO, referred to ENSO STPD hereafter, are still inadequately captured by state-of-the-art climate models, despite the increasing successes achieved in simulating the ENSO phenomenon due to better process representations and increased in model resolutions (Planton et al., 2021). This is in part due to the fundamentally sensitive nonlinear dynamics of ENSO STPD and the fact that ENSO STPD involves multiscale interactions between ENSO and other major modes of variability, including the Madden Julian Oscillation (MJO) and Westerly Wind Bursts (WWBs), Tropical Instability Waves (TIWs), Pacific Meridional Modes (PMMs), the Interdecadal Pacific Oscillation (IPO) and/or Pacific Decadal Oscillation (PDO), and modes in other tropical ocean basins (see reviews by Timmermann et al., 2018; Wang, 2018; Yang et al., 2018; Cai et al., 2019). As a result, various biases in the climate mean state, seasonal cycle, the composition of key coupled feedback processes of ENSO, and ENSO’s interactions with other modes of variability can all play important roles in hindering the state-of-the-art climate model’s capabilities of simulating ENSO and its STPD, which shall affect the performances of these models in projecting changes of ENSO and their associated global impacts under global warming. Thus, advancing our understanding of ENSO STPD and its broad impacts on various aspects of the Earth system has been the subject of active multi-disciplinary research.
Key Sources for ENSO STPD
A great amount of work has been directed to understanding the implications of this ENSO STPD in all aspects of ENSO’s impacts. At the same time, a surge of research activities aimed at understanding the dynamics of ENSO STPD has led to some significant progress. Much of it has been recently reviewed by several authors, including Capotondi et al. (2015, 2020), Timmermann et al. (2018), and Taschetto et al. (2020). A few hypotheses have been put forth as potential mechanisms for observed ENSO STPD. First, based on linear eigen-analyses of modified versions of the Cane-Zebiak (CZ) model and subsequent nonlinear simulations of CP and EP pattern diversity performed by Bejarano and Jin (2008) and Xie and Jin (2018), the coexistence of CP and EP ENSO-like linear eigenmodes under a given climate basic state and parameter setting were proposed as a promising linear pathway for understanding the observed ENSO STPD (Timmermann et al., 2018). Second, ENSO’s interactions with other modes of variability, either in the Pacific Ocean sector or other tropical basins, have been suggested to play some viable roles in generating ENSO STPD (Chiang and Vimont, 2004; Yu et al., 2010; Yu and Kim, 2011; Ham et al., 2013; Lian et al., 2014; Vimont et al., 2014; Zhang et al., 2014; Chen et al., 2015; Fedorov et al., 2015; Jadhav et al., 2015; Hu and Fedorov, 2018). However, the underlying fundamental deterministic dynamics that allow various other modes of variability to be effective in either exciting and/or interacting with ENSO and thereby generating ENSO STPD remains to be addressed. Third, the two types of El Niño were noted to develop under two different combinations of the thermocline feedback and zonal advective feedback, with EP El Niño having relatively stronger thermocline feedback and CP El Niño a relatively stronger zonal advective feedback (Kug et al., 2009; Capotondi et al., 2015, 2020). This notion adds a partial understanding of ENSO STPD but again does not address the key controlling dynamical mechanism that sustains these contrasting ENSO behaviors. Fourth, key nonlinearities residing in 1) the threshold dependence of convective heating on SST (Choi et al., 2013; Takahashi et al., 2019) and 2) the nonlinear zonal advection of SST (Capotondi, 2013; Chen and Majda, 2017) have been suggested important for ENSO nonlinear growth, although how the different nonlinearities effectively alter the linear dynamical processes to generate ENSO STPD remains to be better understood.
A Potential Unifying Paradigm for ENSO STPD
Recently, important progress was made in a newly completed Ph.D. thesis study (Geng, 2021). By uncovering an error in the method of solving the eigen solutions by Bejarano and Jin (2008) and Xie and Jin (2018), this new study dismissed the linear pathway for ENSO STPD proposed by Xie and Jin (2018) and also advocated by Timmermann et al. (2018). This is not only because the proposed linear pathway therein was based on spurious coexistence of unstable linear CP and EP modes but also due to the fact that ENSO STPD simulated by these earlier versions of the CZ-type model and a recently revised CZ model (RCZ hereafter; Geng and Jin, 2022) are all due to a general nonlinearity/noise-induced regime transitions (NIRT) mechanism. To be more specific, when conditions are right, a solo leading linear ENSO mode in the CZ-type model can undergo strong nonlinear modifications owing to advective and/or convective nonlinearities. As a result, it is the nonlinear pathway via the NIRT mechanism that is responsible for the simulated ENSO STPD in the CZ-type model with transitions of EP- and CP-like El Niño events taking place deterministically or stochastically. For this NIRT mechanism to take effect, however, it requires the leading linear ENSO mode to satisfy two stingy constraints: 1) its linear growth rate to be near criticality or supercritical so that nonlinearity becomes effective and 2) its interannual frequency and SST pattern both being highly sensitive to modest changes in the basic state and parameters. Under these strongly double constraints, the leading ENSO mode can lead to irregular transitions between CP and EP El Niño events via deterministic chaos and/or stochastic excitations.
With the RCZ model that underwent careful validations using observational data, Geng (2021) showed that ENSO STPD can be captured only within a narrow range near the best-estimated parameter setting and observed basic state conditions owing to the strongly double constraints. The RCZ model simulated ENSO exhibits basic features reasonably reminiscent of what is observed: intermittency of CP and EP El Niño events with a strong bimodality in the probability density distribution for the SST centroid longitude and some modest decadal to multidecadal modulations of the mean state. In the case without noise forcing and with no annual cycle in the basic state, the leading CP-like ENSO mode will give rise to self-sustained ENSO oscillation whose amplitude increases with stronger supercriticality following the normal manner of Hopf-bifurcation as delineated in the weakly nonlinear regime as in appendix of Jin (1997a). However, under weak to moderate supercritical conditions slightly away from the very limited weakly nonlinear regime, this simple ENSO oscillator itself becomes unstable owing to the sensitive interaction between the ENSO oscillator and the mean state, which generates a regime for ENSO STPD featuring deterministic chaos with CP and EP ENSO regime transitions and quasiperiodic orbits with CP and EP events alternations. When the observed annual cycle in the basic state is included, the parameter range for ENSO STPD is significantly expanded as the route to chaos via the so-called overlap subharmonic resonance (Chang et al., 1994; Jin et al., 1994; Tziperman et al., 1994) becomes effectively activated due to the nonlinear interaction between ENSO and the annual cycle. With stochastic forcing, the ENSO STPD regime broadens further, especially towards the subcritical regime where the leading mode is not self-sustained. It is worthy to emphasize that ENSO chaos generated by ENSO-annual cycle interaction noted by Jin et al. (1994) only features weak temporal irregularity in simulated ENSO behaviors without exhibiting CP and EP pattern diversity. Similarly, in the slightly stronger subcritical regime where the ENSO linear mode is more damped or in the regimes where the leading mode is insensitive to nonlinear modifications, noise forcing can generate temporal irregularity but does not lead to significant CP and EP diversity. In other words, ENSO STPD can be generated via nonlinear deterministic and stochastic dynamics-induced CP and EP ENSO transitions, but only when the aforementioned doubly constrained conditions are satisfied. This general mechanism can be considered as a potential unifying paradigm because it incorporates the keynoted sources for ENSO STPD, including 1) deterministic nonlinear dynamics, 2) stochastic forcing (which can be largely related to various excitations from other modes of variability), and 3) CP and EP’s different mechanisms in terms of relative strengths of the zonal advective feedback and thermocline feedback processes. The doubly constrained conditions for ENSO STPD portrayed by this paradigm make ENSO STPD fundamentally sensitive to modest biases or changes in the climate mean state, seasonal cycle, the composition of feedback processes, and ENSO’s interaction with other modes of variability. This sensitivity poses a great challenge for simulating ENSO STPD and projecting ENSO’s changes through comprehensive climate models. Further active research of the dynamics of STPD may lead to a better understanding of the observed and climate model simulated ENSO and its STPD and associated broad impacts.
Challenges and Outlooks
The advance in our understanding of ENSO in the past 4 decades was achieved in multiple areas, including observations, theory and dynamics, modeling and prediction, past and future changes, and impacts, which all have been comprehensively reviewed in a recent AGU monograph by McPhaden et al. (2020). Hierarchical modeling approaches, comprising conceptual models, intermediate complexity models, and comprehensive climate models, have been instrumental in these remarkable advancements. In fact, ENSO’s basic features (for example, its amplitude, growth, frequency, noise forcing, and asymmetry) and how they may be affected by a model’s compositions of coupled processes, mean state, and annual cycle, have been meaningfully assessed and analyzed in many recent studies with this approach (c.f, Jin et al., 2020; Yu et al., 2016; Chen and Jin, 2020, 2021; Vijayeta and Dommenget, 2018; Zhao et al., 2021b; Levine and Jin, 2010; Levine et al., 2016; Levine and Jin, 2017; Levine et al., 2017; Wengel et al., 2018). For example, using a data-fitted recharge oscillator model, Wengel et al. (2018) demonstrated that the ENSO amplitude simulated by state-of-the-art climate models depends on ENSO’s growth rate and its noise excitation. This ENSO growth rate metric can be proven to be nearly the same as the total linear and nonlinear combined growth rate using a Bjerknes Instability index (a measure of the linear ENSO growth rate resulting from the net effect of positive and negative feedbacks) formulation (Jin et al., 2020). Thus, one may gain further insights into ENSO amplitude in terms of linear and nonlinear growth rate and stochastic forcing (Jin et al., 2020). Chen and Jin (2021) demonstrated that the biases of seasonal modulations of the ENSO growth rate are responsible for pronounced biases of ENSO phase locking in climate models. The broad inter-model spread of simulated ENSO phase locking was shown to be understood by the conceptual RO model. Even for models with relatively “good” ENSO simulation capabilities, such as the E3SM-1-0, CESM2, and GFDL-CM4 models, Chen et al. (2021) noted pronounced common biases in the zonal advective feedback in the central to western Pacific and associated ENSO SST pattern biases near the warm pool edge. These common biases may hinder models’ ability to simulate EP and CP diversity, which is strongly sensitive to the relative strengths of the zonal advective and thermocline feedbacks according to the NIRT mechanism.
The previous success of the hierarchical modeling approaches and the latest advancements in ENSO theory for understanding ENSO and its STPD pointed to promising ways to address and resolve several main challenging issues concerning ENSO STPD and its implications. For example, can we achieve a conceptual understanding of ENSO STPD through a systematic investigation of various contributing sources in a similar way to the simple recharge oscillator paradigm for our understanding of the basic dynamics of ENSO? Can we achieve a better understanding of the roles of model biases in the climate mean state and its seasonal cycle and in the composition of its main feedback processes in model simulated ENSO STPD and thus identify practical pathways for improving climate models’ capability in simulating ENSO and its STPD? Can we achieve a better understanding of ENSO STPD’s predictability for ENSO regime behavior as noted by Timmermann and Jin (2005)? Can we achieve a better understanding of the projections of ENSO changes relative to the current observed ENSO STPD regime, which can be fundamentally sensitive to climate change? Can we achieve a better understanding of ENSO STPD’s direct and indirect impacts from other modes of variability influenced by ENSO on various aspects of the Earth system?
To further discuss the outlooks to address these questions, a synthesized framework focusing on ENSO STPD is illustrated in Figure 2, which is extended from the frameworks schematically depicted in Timmermann et al. (2018) and Wittenberg (2018). This framework highlights three foci in addition to the basic coupled dynamics understood in existing conceptual models and ICMs for ENSO. First, it highlights the newly uncovered NIRT mechanism that is essential for ENSO STPD. Second, it highlights two key physical processes, the convective-radiative feedback in atmospheric moist dynamics (Adames and Kim, 2016) and wind-driven thermal stratification adjustment in the central equatorial Pacific (Zhao et al., 2021a), both of which may have strong effects on the composition of ENSO feedbacks in both nature and comprehensive climate models. Third, it highlights ENSO’s interactions with various other modes of variability and the roles of deterministic nonlinearities and stochastic forcing, which all have been suggested to be of importance for ENSO STPD. I will briefly discuss a few areas for potential significant advances with this guiding framework.
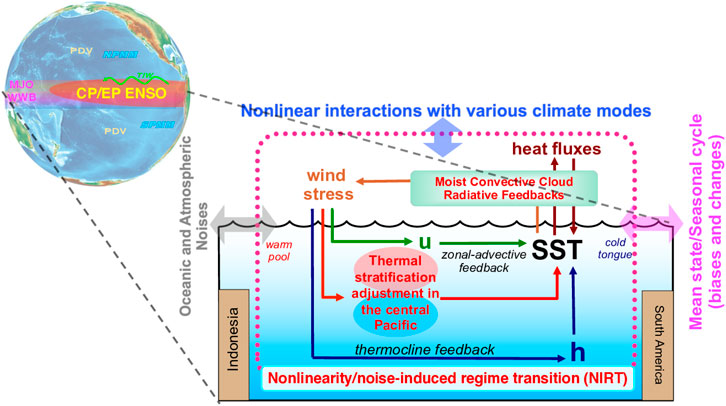
FIGURE 2. Schematic diagram (modified based on Whittenberg 2018) of major modes of variability in the Pacific and key ENSO atmospheric and oceanic processes.
On low-order conceptual models for ENSO STPD: Simple conceptual models such as the recharge oscillator model have played an important role in understanding ENSO’s basic dynamics and features. To address the need of developing new conceptual models that can serve as useful tools for understanding key features of ENSO STPD, a working group under the CLIVAR Pacific Panel was established in 2020 under the leadership of Dr. Jerome Vialard to review the progress and to discuss prospects for developing such a kind of models. Two SST variables for central and eastern Pacific SST anomalies,
On Intermediate Coupled Models (ICM) for ENSO STPD: CZ-type models have served as the core framework for the advances of ENSO theory during the past 4 decades. Our recent study uncovered the NIRT mechanism for ENSO STPD and again used a CZ-type model. Nevertheless, some essential processes, such as 1) convective cloud radiative feedback associated with moisture dynamics and 2) a key oceanic process involving wind-driven upper thermocline ocean stratification adjustment of the equatorial ocean is highlighted in Figure 2, are currently omitted in CZ-type models. The convective cloud radiative feedback associated with moisture dynamics of ENSO affects both the dynamic air-sea coupling through ENSO’s wind-SST relationship and the thermodynamic feedback through surface heat flux sensitivity as noted in our study using new versions of a moist linear baroclinic model (mLBM) for the atmosphere (Hayashi and Jin, 2022; Chen et al., 2022, personal communication). These key feedbacks are controlled by the dynamic air-sea coupling parameter and SST thermal damping parameter, both of which can be assessed using climate model outputs and reanalysis data. However, unlike in a CZ-type model, they cannot be arbitrarily changed in climate models. Thus, using the mLBM as an alternative to the Gill-type atmospheric component to form new versions of ICM models will be useful to examine the role of the convective cloud radiative feedback associated with moisture dynamics in ENSO and its STPD. Similarly, the ocean component of the CZ-type model may be replaced by a linear continuously stratified (LCS) tropical Pacific Ocean model (McCreary, 1981; McPhaden, 1981; Keenlyside and Kleeman, 2002) to capture wind-driven equatorial upper thermocline thermal stratification adjustment, which contributes to ENSO’s zonal advective feedback and thermocline feedback differently between EP and CP ENSO events and thus may affect ENSO and its STPD (Zhao et al., 2021a). Clearly, this kind of extension of the CZ-type model will be helpful for better advancing processes-level understanding of the dynamics of ENSO and its STPD.
On the role of ENSO-Annual Cycle Interactions in ENSO STPD: Although ENSO chaos through nonlinear subharmonic overlap resonance as found by Jin et al. (1994), Tziperman et al. (1994), and Chang et al. (1994) was noted as an important deterministic source of ENSO irregularity, it has not gained much further attention because ENSO as part of the climate-weather continuum has been suggested to be more of a noise-driven irregular oscillator. However, some new evidence indicates that the annual cycle can effectively expand the extent of the ENSO STPD regime in the RCZ model (Geng 2021) because the NIRT mechanism provides a natural nonlinearity-induced ENSO frequency sensitivity which allows the nonlinear subharmonic overlap resonance route to chaos to become effective, not only creating temporal irregularity but also spatial pattern diversity of ENSO. Moreover, ENSO-annual cycle interaction with the NIRT mechanism at work may effectively create a frequency cascade toward ENSO decadal timescale variability through readily exciting nonlinear transitions of frequency-locked CP and EP ENSO cycles. For example, if there were pronounced frequency-locked 3-years and 4-year periodic orbit transitions, one would expect a potential 12-years spectral peak. This kind of ENSO frequency cascade can generate low-frequency variability and provides a potentially important driver for both ENSO modulation and Pacific decadal variability. This role of ENSO-annual cycle interactions in ENSO STPD may have implications for paleo and future changes of both ENSO and the tropical climate mean state.
On ENSO’s nonlinear interactions with other modes for ENSO STPD: There is a large body of studies suggesting that ENSO may be excited or affected by various other modes of variability both within and outside the tropical Pacific while ENSO, as one of the strongest climatic modes, can affect those modes as well. Depending on the natures of these modes, ENSO’s interaction with them may not only affect ENSO’s basic features but also serve as sources for ENSO’s “noise” forcing and modulations, which all may play important roles in ENSO STPD. For example, one of the most prominent features that are closely associated with ENSO is the enhanced/reduced TIW activity during La Niño/El Niño events. An and Jin (2004) demonstrated that nonlinear ENSO-TIW interaction can affect ENSO asymmetry. Boucharel and Jin (2020) and Xue et al. (2020) built a conceptual model to demonstrate that this nonlinear interaction is highly seasonally modulated. This asymmetric nonlinear interaction may have strong implications for ENSO’s rectification on the basic state, which in turn can affect the NIRT pathway to ENSO STPD. Moreover, the strong seasonality in this interaction can serve as another source of seasonal modulation that may affect the extent of the ENSO STPD regime. The work of Boucharel and Jin (2020) and Xue et al. (2020) has paved the way for developing nonlinear and seasonally modulated parameterizations for TIW-induced nonlinear feedback on ENSO, which can be useful for assessing TIW’s feedback on ENSO simulated in climate models and can be incorporated into the CZ-type model or other ICMs for studying the impacts of ENSO-TIW interaction on ENSO and its STPD.
There is a large body of studies investigating the roles of the MJO and WWBs in exciting ENSO’s complex behaviors and even altering ENSO’s growth rate and frequency and predictability using conceptual models, CZ-type models, and coupled climate models. However, simple conceptual and CZ-type ICMs mostly describe MJO/WWB activity and its modulation by ENSO in ad hoc manners, often in terms of state-dependent noise. Developments of conceptual models and ICMs for understanding the dynamics of ENSO’s modulation on MJO/WWB will be essential for better understanding ENSO’s interaction with MJO/WWB activity, especially for its roles in ENSO STPD.
Several studies suggested that Pacific Meridional Modes (PMMs) may play a potentially important role in ENSO STPD through stochastically exciting ENSO cycles and providing distinct optimal precursors for the two types of ENSO (Amaya, 2019, and references therein; Vimont et al., 2014, 2022; Capotondi and Ricchiardulli, 2021). However, the notion that ENSO serves as a strong driver of PMM makes the PMM’s contribution to ENSO and its STPD an open question needing further exploration (Stuecker, 2018). ICMs explicitly resolving the relevant physical processes, particularly the wind-evaporation and cloud-radiation to SST feedbacks that are missing out in most ICMs, may be helpful to advance our understanding of how these PMMs may interact with ENSO to affect the extent of ENSO STPD regimes. Moreover, the wind stress curl in subtropical regions may excite slow ocean adjustment and potentially yield decadal coupled modes once this slow process is connected to coupled dynamics in the equatorial Pacific (Wang et al., 2003a; 2003b). Thus, according to the NIRT mechanism, the presence of PMMs and their associated wind-driven slow ocean adjustment may enhance ENSO’s internal low-frequency modulation as the result of nonlinear interaction between ENSO and the mean state. This ENSO low-frequency variability associated with ENSO STPD and its potential enhancement through ENSO-PMM interaction may serve as important sources for the so-called Pacific Decadal Oscillation (PDO) and Interdecadal Pacific Oscillation (IPO). Moreover, ENSO STPD may affect and also be affected by ENSO’s interactions with the modes of variability outside the Pacific. Further investigation of inter-basin interactions among ENSO and the modes of variability in other ocean basins shall also shed light on ENSO STPD.
The advances in understanding the dynamics of ENSO STPD will also lead to better assessment and understanding of ENSO STPD simulated in state-of-the-art climate models, which are mostly still falling short in realistically simulating ENSO STPD. Such models’ deficiency is likely attributed to the high sensitivity of ENSO STPD to even modest biases in climate models in terms of simulated climate mean state, its seasonal cycle, and the composition of key feedback processes of ENSO. Nevertheless, better assessing and understanding how these biases or potential reductions of them may affect the capabilities of climate models to more realistically capture ENSO and its STPD may lead to potential pathways for model improvements. Moreover, ENSO STPD, together with ENSO’s nonlinearly and seasonally modulated interactions with other modes of variability, make ENSO’s influences on various aspects of the Earth system much more complex. That is, ENSO can have not only direct impacts but also indirect impacts through its influences on the other modes of variability in both nonlinear and nonstationary manners. Better assessing, understanding, simulating ENSO and its STPD may provide better understanding and predictive skills for ENSO and its impacts even on the regional but highly destructive and thus hazardous phenomena as demonstrated, for example, in recent research on ENSO’s impact on worldwide coastal ocean wave activity and coastal shorelines (Boucharel et al., 2021; Almar et al., 2022, personal communication).
Data Availability Statement
Publicly available datasets were analyzed in this study. These data can be found at: https://www.metoffice.gov.uk/hadobs/hadisst/.
Author Contributions
F-FJ designed the study and wrote the manuscript.
Funding
The work was supported by the U.S. National Science Foundation (AGS-1813611) and the U.S. Department of Energy (DESC0005110).
Conflict of Interest
The author declares that the research was conducted in the absence of any commercial or financial relationships that could be construed as a potential conflict of interest.
Publisher’s Note
All claims expressed in this article are solely those of the authors and do not necessarily represent those of their affiliated organizations, or those of the publisher, the editors and the reviewers. Any product that may be evaluated in this article, or claim that may be made by its manufacturer, is not guaranteed or endorsed by the publisher.
Acknowledgments
The author is grateful to Dr. Sen Zhao who produced the two figures and also to Drs. Licheng Geng, Sen Zhao, Malte Stuecker, and Andrew Wittenberg for valuable comments and edits.
References
Adames, Á. F., and Kim, D. (2016). The MJO as a Dispersive, Convectively Coupled Moisture Wave: Theory and Observations. J. Atmos. Sci. 73, 913–941. doi:10.1175/JAS-D-15-0170.1
Almar, R., Graffin, M., Boucharel, J., Abessolo, G. O., Thoumyre, G., Papa, F., et al. (2022). El Niño Controls the Evolution of Shorelines Worldwide. Preprint Research Square. doi:10.21203/rs.3.rs-1283693/v1
Amaya, D. J. (2019). The Pacific Meridional Mode and ENSO: a Review. Curr. Clim. Change Rep. 5, 296–307. doi:10.1007/s40641-019-00142-x
An, S.-I., and Jin, F.-F. (2000). An Eigen Analysis of the Interdecadal Changes in the Structure and Frequency of ENSO Mode. Geophys. Res. Lett. 27, 2573–2576. doi:10.1029/1999gl011090
An, S.-I., and Jin, F.-F. (2004). Nonlinearity and Asymmetry of ENSO*. J. Clim. 17, 2399–2412. doi:10.1175/1520-0442(2004)017<2399:naaoe>2.0.co;2
Ashok, K., Behera, S. K., Rao, S. A., Weng, H., and Yamagata, T. (2007). El Niño Modoki and its Possible Teleconnection. J. Geophys. Res. 112. doi:10.1029/2006jc003798
Battisti, D. S., and Hirst, A. C. (1989). Interannual Variability in a Tropical Atmosphere-Ocean Model: Influence of the Basic State, Ocean Geometry and Nonlinearity. J. Atmos. Sci. 46, 1687–1712. doi:10.1175/1520-0469(1989)046<1687:iviata>2.0.co;2
Bejarano, L., and Jin, F.-F. (2008). Coexistence of Equatorial Coupled Modes of ENSO*. J. Clim. 21, 3051–3067. doi:10.1175/2007JCLI1679.1
Boucharel, J., Almar, R., Kestenare, E., and Jin, F.-F. (2021). On the Influence of ENSO Complexity on Pan-Pacific Coastal Wave Extremes. Proc. Natl. Acad. Sci. U.S.A. 118, 9118. doi:10.1073/pnas.2115599118
Boucharel, J., and Jin, F.-F. (2020). A Simple Theory for the Modulation of Tropical Instability Waves by ENSO and the Annual Cycle. Tellus A Dyn. Meteorology Oceanogr. 72, 1–14. doi:10.1080/16000870.2019.1700087
Brunner, A. D. (2000). El Nino and World Primary Commodity Prices: Warm Water or Hot Air? IMF Work. Pap. doi:10.5089/9781451874440.001.A001
Cai, W., Wu, L., Lengaigne, M., Li, T., McGregor, S., Kug, J.-S., et al. (2019). Pantropical Climate Interactions. Science 363, eaav4236. doi:10.1126/science.aav4236
Cane, M. A., and Moore, D. W. (1981). A Note on Low-Frequency Equatorial Basin Modes. J. Phys. Oceanogr. 11, 1578–1584. doi:10.1175/1520-0485(1981)011<1578:anolfe>2.0.co
Cane, M. A., Münnich, M., and Zebiak, S. F. (1990). A Study of Self-Excited Oscillations of the Tropical Ocean-Atmosphere System. Part I: Linear Analysis. J. Atmos. Sci. 47, 1562–1577. doi:10.1175/1520-0469(1990)047<1562:asoseo>2.0.co;2
Cane, M. A., and Zebiak, S. E. (1985). A Theory for El Niño and the Southern Oscillation. Science 228, 1085–1087. doi:10.1126/science.228.4703.1085
Capotondi, A. (2013). ENSO Diversity in the NCAR CCSM4 Climate Model. J. Geophys. Res. Oceans 118, 4755–4770. doi:10.1002/jgrc.20335
Capotondi, A., and Ricciardulli, L. (2021). The Influence of Pacific Winds on ENSO Diversity. Sci. Rep. 11, 18672. doi:10.1038/s41598-021-97963-4
Capotondi, A., Wittenberg, A. T., Kug, J. S., Takahashi, K., and McPhaden, M. J. (2020). “ENSO Diversity,” in El Niño Southern Oscillation in a Changing Climate (American Geophysical Union (AGU)), 65–86. doi:10.1002/9781119548164.ch4
Capotondi, A., Wittenberg, A. T., Newman, M., Di Lorenzo, E., Yu, J.-Y., Braconnot, P., et al. (2015). Understanding ENSO Diversity. Bull. Amer. Meteor. Soc. 96, 921–938. doi:10.1175/BAMS-D-13-00117.1
Cashin, P., Mohaddes, K., and Raissi, M. (2017). Fair Weather or Foul? the Macroeconomic Effects of El Niño. J. Int. Econ. 106, 37–54. doi:10.1016/j.jinteco.2017.01.010
Chang, P., Wang, B., Li, T., and Ji, L. (1994). Interactions between the Seasonal Cycle and the Southern Oscillation - Frequency Entrainment and Chaos in a Coupled Ocean-Atmosphere Model. Geophys. Res. Lett. 21, 2817–2820. doi:10.1029/94GL02759
Chen, D., Lian, T., Fu, C., Cane, M. A., Tang, Y., Murtugudde, R., et al. (2015). Strong Influence of Westerly Wind Bursts on El Niño Diversity. Nat. Geosci. 8, 339–345. doi:10.1038/ngeo2399
Chen, H.-C., and Jin, F.-F. (2020). Fundamental Behavior of ENSO Phase Locking. J. Clim. 33, 1953–1968. doi:10.1175/JCLI-D-19-0264.1
Chen, H.-C., and Jin, F.-F. (2021). Simulations of ENSO Phase-Locking in CMIP5 and CMIP6. J. Clim. 34, 1–42. doi:10.1175/JCLI-D-20-0874.1
Chen, H.-C., Jin, F.-F., Zhao, S., Wittenberg, A. T., and Xie, S. (2021). ENSO Dynamics in the E3SM-1-0, CESM2, and GFDL-CM4 Climate Models. J. Clim. 34, 1–59. doi:10.1175/JCLI-D-21-0355.1
Chen, N., and Majda, A. J. (2017). Simple Stochastic Dynamical Models Capturing the Statistical Diversity of El Niño Southern Oscillation. Proc. Natl. Acad. Sci. U.S.A. 114, 1468–1473. doi:10.1073/pnas.1620766114
Chiang, J. C. H., and Vimont, D. J. (2004). Analogous Pacific and Atlantic Meridional Modes of Tropical Atmosphere-Ocean Variability*. J. Clim. 17, 4143–4158. doi:10.1175/JCLI4953.1
Choi, J., and An, S.-I. (2013). Quantifying the Residual Effects of ENSO on Low-Frequency Variability in the Tropical Pacific. Int. J. Climatol. 33, 1047–1052. doi:10.1002/joc.3470
Choi, K.-Y., Vecchi, G. A., and Wittenberg, A. T. (2013). ENSO Transition, Duration, and Amplitude Asymmetries: Role of the Nonlinear Wind Stress Coupling in a Conceptual Model. J. Clim. 26, 9462–9476. doi:10.1175/jcli-d-13-00045.1
Fang, X.-H., and Mu, M. (2018). A Three-Region Conceptual Model for Central Pacific El Niño Including Zonal Advective Feedback. J. Clim. 31, 4965–4979. doi:10.1175/JCLI-D-17-0633.1
Fedorov, A. V., Hu, S., Lengaigne, M., and Guilyardi, E. (2015). The Impact of Westerly Wind Bursts and Ocean Initial State on the Development, and Diversity of El Niño Events. Clim. Dyn. 44, 1381–1401. doi:10.1007/s00382-014-2126-4
Fedorov, A. V., and Philander, S. G. (2001). A Stability Analysis of Tropical Ocean-Atmosphere Interactions: Bridging Measurements and Theory for El Niño. J. Clim. 14, 3086–3101. doi:10.1175/1520-0442(2001)014<3086:asaoto>2.0.co;2
Fedorov, A. V., and Philander, S. G. (20002002). Is El Niño Changing? Science 288, 1997–2002. doi:10.1126/science.288.5473.1997
Geng, L. (2021). Dynamics of El Niño-Southern Oscillation Diversity in an Intermediate Coupled Model (Ph.D. Dissertation). Honolulu: University of Hawai’i at Manoa.
Geng, L., and Jin, F.-F. (2022). ENSO Diversity Simulated in a Revised Cane-Zebiak Model. Front. Earth Sci. 10, 899323. doi:10.3389/feart.2022.899323
Ham, Y.-G., Kug, J.-S., Park, J.-Y., and Jin, F.-F. (2013). Sea Surface Temperature in the North Tropical Atlantic as a Trigger for El Niño/Southern Oscillation Events. Nat. Geosci. 6, 112–116. doi:10.1038/NGEO1686
Hu, S., and Fedorov, A. V. (2018). Cross-equatorial Winds Control El Niño Diversity and Change. Nat. Clim. Change 8, 798–802. doi:10.1038/s41558-018-0248-0
Jadhav, J., Panickal, S., Marathe, S., and Ashok, K. (2015). On the Possible Cause of Distinct El Niño Types in the Recent Decades. Sci. Rep. 5, 17009. doi:10.1038/srep17009
Jin, F.-F. (1997a). An Equatorial Ocean Recharge Paradigm for ENSO. Part I: Conceptual Model. J. Atmos. Sci. 54, 811–829. doi:10.1175/1520-0469(1997)054<0811:aeorpf>2.0.co;2
Jin, F.-F. (1997b). An Equatorial Ocean Recharge Paradigm for ENSO. Part II: a Stripped-Down Coupled Model. J. Atmos. Sci. 54, 8302–8847. doi:10.1175/1520-0469(1997)054<0830:aeorpf>2.0.co
Jin, F.-F. (2001). Low-Frequency Modes of Tropical Ocean Dynamics*. J. Clim. 14, 3874–3881. doi:10.1175/1520-0442(2001)014<3874:lfmoto>2.0.co;2
Jin, F.-F., Neelin, J. D., and Ghil, M. (1994). El Niño on the Devil's Staircase: Annual Subharmonic Steps to Chaos. Science 264, 70–72. doi:10.1126/science.264.5155.70
Jin, F.-F., and Neelin, J. D. (1993). Modes of Interannual Tropical Ocean-Atmosphere Interaction-A Unified View. Part I: Numerical Results. J. Atmos. Sci. 50, 3477–3503. doi:10.1175/1520-0469(1993)050<3477:MOITOI>2.0.CO10.1175/1520-0469(1993)050<3477:moitoi>2.0.co;2
Jin, F. F., Chen, H. C., Zhao, S., Hayashi, M., Karamperidou, C., Stuecker, M. F., et al. (2020). “Simple ENSO Models,” in El Niño Southern Oscillation in a Changing Climate. Editors M. J. McPhaden, A. Santoso, and W. Cai (Hoboken, NJ: American Geophysical Union Geophysical Monograph Series) 253, 119–151. doi:10.1002/9781119548164
Kao, H.-Y., and Yu, J.-Y. (2009). Contrasting Eastern-Pacific and Central-Pacific Types of ENSO. J. Clim. 22, 615–632. doi:10.1175/2008jcli2309.1
Keenlyside, N., and Kleeman, R. (2002). Annual Cycle of Equatorial Zonal Currents in the Pacific. J. Geophys. Res. 107, 8–18. doi:10.1029/2000JC000711
Kug, J.-S., Jin, F.-F., and An, S.-I. (2009). Two Types of El Niño Events: Cold Tongue El Niño and Warm Pool El Niño. J. Clim. 22, 1499–1515. doi:10.1175/2008JCLI2624.1
Larkin, N. K., and Harrison, D. E. (2005a). Global Seasonal Temperature and Precipitation Anomalies during El Niño Autumn and Winter. Geophys. Res. Lett. 32, 2860. doi:10.1029/2005GL022860
Larkin, N. K., and Harrison, D. E. (2005b). On the Definition of El Niño and Associated Seasonal Average U.S. Weather Anomalies. Geophys. Res. Lett. 32, 2738. doi:10.1029/2005GL022738
Levine, A. F. Z., and Jin, F.-F. (2010). Noise-Induced Instability in the ENSO Recharge Oscillator. J. Atmos. Sci. 67, 529–542. doi:10.1175/2009JAS3213.1
Levine, A. F. Z., and Jin, F. F. (2017). A Simple Approach to Quantifying the Noise-ENSO Interaction. Part I: Deducing the State-Dependency of the Windstress Forcing Using Monthly Mean Data. Clim. Dyn. 48, 1–18. doi:10.1007/s00382-015-2748-1
Levine, A. F. Z., Jin, F. F., and Stuecker, M. F. (2017). A Simple Approach to Quantifying the Noise-ENSO Interaction. Part II: The Role of Coupling between the Warm Pool and Equatorial Zonal Wind Anomalies. Clim. Dyn. 48, 19–37. doi:10.1007/s00382-016-3268-3
Levine, A., Jin, F. F., and McPhaden, M. J. (2016). Extreme Noise-Extreme El Niño: How State-dependent Noise Forcing Creates El Niño-La Niña Asymmetry. J. Clim. 29, 5483–5499. doi:10.1175/JCLI-D-16-0091.1
Lian, T., Chen, D., Tang, Y., and Wu, Q. (2014). Effects of Westerly Wind Bursts on El Niño: A New Perspective. Geophys. Res. Lett. 41, 3522–3527. doi:10.1002/2014GL059989
McCreary, J. P. (1981). A Linear Stratified Ocean Model of the Equatorial Undercurrent. Phil. Trans. R. Soc. Lond. A 298, 603–635. doi:10.1098/rsta.1981.0002
McPhaden, M. J. (1981). Continuously Stratified Models of the Steady-State Equatorial Ocean. J. Phys. Oceanogr. 11, 337–354. doi:10.1175/1520-0485(1981)011<0337:csmots>2.0.co;2
McPhaden, M. J., Santoso, A., and Cai, W. (2020). El Niño Southern Oscillation in a Changing Climate. John Wiley & Sons.
McPhaden, M. J., Zebiak, S. E., and Glantz, M. H. (2006). ENSO as an Integrating Concept in Earth Science. Science 314, 1740–1745. doi:10.1126/science.1132588
Neelin, J. D., Battisti, D. S., Hirst, A. C., Jin, F.-F., Wakata, Y., Yamagata, T., et al. (1998). ENSO Theory. J. Geophys. Res. 103, 14261–14290. doi:10.1029/97JC03424
Neelin, J. D., and Jin, F.-F. (1993). Modes of Interannual Tropical Ocean-Atmosphere Interaction-A Unified View. Part II: Analytical Results in the Weak-Coupling Limit. J. Atmos. Sci. 50, 3504–3522. doi:10.1175/1520-0469(1993)050<3504:moitoi>2.0.co;2
Picaut, J., Masia, F., and du Penhoat, Y. (1997). An Advective-Reflective Conceptual Model for the Oscillatory Nature of the ENSO. Science 277, 663–666. doi:10.1126/science.277.5326.663
Planton, Y. Y., Guilyardi, E., Wittenberg, A. T., Lee, J., Gleckler, P. J., Bayr, T., et al. (2021). Evaluating Climate Models with the CLIVAR 2020 ENSO Metrics Package. Bull. Am. Meteorological Soc. 102, E193–E217. doi:10.1175/BAMS-D-19-0337.1
Rayner, N. A., Parker, D. E., Horton, E. B., Folland, C. K., Alexander, L. V., Rowell, D. P., et al. (2003). Global Analyses of Sea Surface Temperature, Sea Ice, and Night Marine Air Temperature since the Late Nineteenth Century. J. Geophys. Res. 108. doi:10.1029/2002JD002670
Stuecker, M. F. (2018). Revisiting the Pacific Meridional Mode. Sci. Rep. 8, 3216. doi:10.1038/s41598-018-21537-0
Suarez, M. J., and Schopf, P. S. (1988). A Delayed Action Oscillator for ENSO. J. Atmos. Sci. 45, 32832–33287. doi:10.1175/1520-0469(1988)045<3283:adaofe>2.0.co
Takahashi, K., Karamperidou, C., and Dewitte, B. (2019). A Theoretical Model of Strong and Moderate El Niño Regimes. Clim. Dyn. 52, 7477–7493. doi:10.1007/s00382-018-4100-z
Taschetto, A. S., Ummenhofer, C. C., Stuecker, M. F., Dommenget, D., Ashok, K., Rodrigues, R. R., et al. (2020). “ENSO Atmospheric Teleconnections,” in El Niño Southern Oscillation in a Changing Climate (American Geophysical Union (AGU)), 309–335. doi:10.1002/9781119548164.ch14
Timmermann, A., An, S.-I., Kug, J.-S., Jin, F.-F., Cai, W., Capotondi, A., et al. (2018). El Niño-Southern Oscillation Complexity. Nature 559, 535–545. doi:10.1038/s41586-018-0252-6
Timmermann, A., and Jin, F.-F. (2005). in Predictability of Coupled Processes" in Predictability of Weather and Climate. Editors T. Palmer, and R. Hagedorn (Cambridge University Press), 251–274.
Tziperman, E., Stone, L., Cane, M. A., and Jarosh, H. (1994). El Niño Chaos: Overlapping of Resonances between the Seasonal Cycle and the Pacific Ocean-Atmosphere Oscillator. Science 264, 72–74. doi:10.1126/science.264.5155.72
Vijayeta, A., and Dommenget, D. (2018). An Evaluation of ENSO Dynamics in CMIP Simulations in the Framework of the Recharge Oscillator Model. Clim. Dyn. 51, 1753–1771. doi:10.1007/s00382-017-3981-6
Vimont, D. J., Alexander, M. A., and Newman, M. (2014). Optimal Growth of Central and East Pacific ENSO Events. Geophys. Res. Lett. 41, 4027–4034. doi:10.1002/2014gl059997
Vimont, D. J., Newman, M., Battisti, D. S., and Shin, S.-I. (2022). The Role of Seasonality and the ENSO Mode in Central and East Pacific ENSO Growth and Evolution. J. Clim. 1, 1–46. doi:10.1175/jcli-d-21-0599.1
Wang, C., and Picaut, J. (2004). “Understanding ENSO Physics - A Review,” in Earth’s Climate: The Ocean-Atmosphere Interaction, Geophysical Monograph Series. Editors C. Wang, S. -P. Xie, and J. A. Carton (Washington, D. C.: AGU), 21–48.
Wang, X., Jin, F.-F., and Wang, Y. (2003a). A Tropical Ocean Recharge Mechanism for Climate Variability. Part I: Equatorial Heat Content Changes Induced by the Off-Equatorial Wind. J. Clim. 16, 3585–3598. doi:10.1175/1520-0442(2003)016<3585:atormf>2.0.co;2
Wang, X., Jin, F.-F., and Wang, Y. (2003b). A Tropical Ocean Recharge Mechanism for Climate Variability. Part II: A Unified Theory for Decadal and ENSO Modes. J. Clim. 16, 3599–3616. doi:10.1175/1520-0442(2003)016<3599:atormf>2.0.co;2
Weng, H., Ashok, K., Behera, S. K., Rao, S. A., and Yamagata, T. (2007). Impacts of Recent El Niño Modoki on Dry/wet Conditions in the Pacific Rim during Boreal Summer. Clim. Dyn. 29, 113–129. doi:10.1007/s00382-007-0234-0
Wengel, C., Dommenget, D., Latif, M., Bayr, T., and Vijayeta, A. (2018). What Controls ENSO‐Amplitude Diversity in Climate Models? Geophys. Res. Lett. 45, 1989–1996. doi:10.1002/2017gl076849
Wittenberg, A. T. (2018). ENSO in Climate Models: Progress and Opportunities. IV International Conference On El Niño Southern Oscillation: ENSO in a Warmer Climate, CLIVAR Workshop, FIEC, ESPOL, Guayaquil, Ecuador. Available at: https://extranet.gfdl.noaa.gov/∼atw/yr/2018/wittenberg_20181017.ppt (Assessed February 2, 2022).
Xie, R., and Jin, F.-F. (2018). Two Leading ENSO Modes and El Niño Types in the Zebiak-Cane Model. J. Clim. 31, 1943–1962. doi:10.1175/JCLI-D-17-0469.1
Xue, A., Jin, F. F., Zhang, W., Boucharel, J., Zhao, S., and Yuan, X. (2020). Delineating the Seasonally Modulated Nonlinear Feedback onto ENSO from Tropical Instability Waves. Geophys. Res. Lett. 47, e2019GL085863. doi:10.1029/2019GL085863
Yang, S., Li, Z., Yu, J.-Y., Hu, X., Dong, W., and He, S. (2018). El Niño-Southern Oscillation and its Impact in the Changing Climate. Natl. Sci. Rev. 5, 840–857. doi:10.1093/nsr/nwy046
Yu, J.-Y., Kao, H.-Y., and Lee, T. (2010). Subtropics-Related Interannual Sea Surface Temperature Variability in the Central Equatorial Pacific. J. Clim. 23, 2869–2884. doi:10.1175/2010JCLI3171.1
Yu, J.-Y., and Kim, S. T. (2011). Relationships between Extratropical Sea Level Pressure Variations and the Central Pacific and Eastern Pacific Types of ENSO. J. Clim. 24, 708–720. doi:10.1175/2010JCLI3688.1
Yu, Y., Dommenget, D., Frauen, C., Wang, G., and Wales, S. (2016). ENSO Dynamics and Diversity Resulting from the Recharge Oscillator Interacting with the Slab Ocean. Clim. Dyn. 46, 1665–1682. doi:10.1007/s00382-015-2667-1
Zhang, H., Clement, A., and Di Nezio, P. (2014). The South Pacific Meridional Mode: A Mechanism for ENSO-like Variability. J. Clim. 27, 769–783. doi:10.1175/JCLI-D-13-00082.1
Zhao, S., Jin, F. F., Long, X., and Cane, M. A. (2021a). On the Breakdown of ENSO's Relationship with Thermocline Depth in the Central‐Equatorial Pacific. Geophys Res. Lett. 48, e2020GL092335. doi:10.1029/2020GL092335
Keywords: El Niño Southern Oscillation phenomenon, spatiotemporal pattern diversity, phase locking, ENSO asymmetry, recharge oscillator paradigm
Citation: Jin F-F (2022) Toward Understanding El Niño Southern-Oscillation’s Spatiotemporal Pattern Diversity. Front. Earth Sci. 10:899139. doi: 10.3389/feart.2022.899139
Received: 18 March 2022; Accepted: 19 April 2022;
Published: 25 May 2022.
Edited by:
Hong-Li Ren, Chinese Academy of Meteorological Sciences, ChinaReviewed by:
Antonietta Capotondi, University of Colorado Boulder, United StatesYoumin Tang, University of Northern British Columbia Canada, Canada
Copyright © 2022 Jin. This is an open-access article distributed under the terms of the Creative Commons Attribution License (CC BY). The use, distribution or reproduction in other forums is permitted, provided the original author(s) and the copyright owner(s) are credited and that the original publication in this journal is cited, in accordance with accepted academic practice. No use, distribution or reproduction is permitted which does not comply with these terms.
*Correspondence: Fei-Fei Jin, amZmQGhhd2FpaS5lZHU=