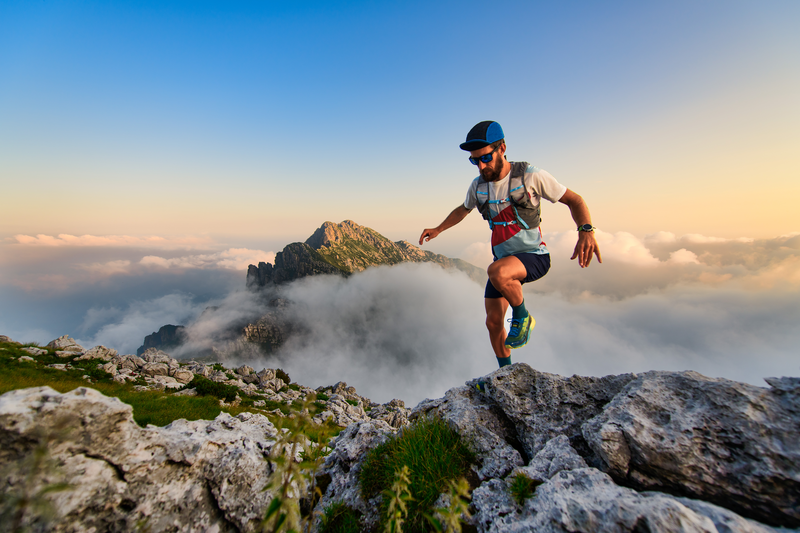
94% of researchers rate our articles as excellent or good
Learn more about the work of our research integrity team to safeguard the quality of each article we publish.
Find out more
ORIGINAL RESEARCH article
Front. Earth Sci. , 19 August 2022
Sec. Quaternary Science, Geomorphology and Paleoenvironment
Volume 10 - 2022 | https://doi.org/10.3389/feart.2022.897183
This article is part of the Research Topic Ancient Starch Remains and Prehistoric Human Subsistence View all 19 articles
Starch-rich plants have played an important role in human evolution and societal development. Collected, grown, and consumed to support ever-increasing populations, such plants are integral to understanding past human diets. With the advent of starch granule analysis, plant resources that were invisible in the archaeological record can now be revealed in the cracks and crevices of artifacts. Widespread application of this technique, however, has stalled due to a lack of rigorous and standardized protocols. For example, taxonomic identification of starch granules using consistent diagnostic characteristics is still a challenge as there are no comprehensive surveys across important (i.e., dietary) plant taxa, especially at the levels of families, genera, and species. This study provides characteristics for identifying starch granules of seven major North American plant families (Amaranthaceae, Apiaceae, Fagaceae, Liliaceae, Pinaceae, Poaceae, and Solanaceae) based on systematic, morphometric studies of modern reference materials. A dichotomous key to starch granules of the seven families was also generated to aid in identification of those from archaeological contexts. Although we have focused on plants from western North America, these families occur across the globe and have had dietary significance throughout prehistory.
The study of starch granules preserved in archaeological contexts, such as from dental calculus and crevices on stone tools, can indicate, often to species-level, the identity of plant foods that had been consumed. Starch granules are the energy storage structures of plants, abundantly found within seeds, fruits, and tubers. They are produced by most vascular plants and the morphology of granules is genetically controlled. Thus, the size, shape and surface features of the granules can indicate which plant taxon (e.g., family, genus, and species) produced them (Nägeli, 1858; Reichert, 1913; Shannon et al., 2009). Although this technique has shed light on the importance of plants in ancient human diets, starch granule research is still underdeveloped and faces several significant issues (Mercader et al., 2018), including rigorous methods for taxonomic identification.
The analysis of starch granules relies on careful measurements of dimensions and accurate descriptions of morphological characteristics, while taxonomic identification is based on comparison to modern reference material. Diagnostic keys for identification are especially useful and have been produced for plant taxa from China (Yang and Perry 2013), eastern North America (Messner 2011), and eastern Mediterranean (Ahituv and Henry 2022). Developing standards that increase the quality and replicability of measurements, descriptions, keys, and documentation is the next necessary step in advancing this technique. This study provides a standardized, systematic approach to defining characteristics that identify the granules of seven major plant families (Amaranthaceae, Apiaceae, Fagaceae, Liliaceae, Pinaceae, Poaceae, and Solanaceae) using reference materials of genera from western North America. A dichotomous key to starch granules of the seven plant families is also presented.
Reference materials for this study includes eighteen species from thirteen genera and seven major plant families having regional and global dietary significance (Table 1). Not only have these taxa been documented in ethnographic literature, the majority have also been recovered from archaeological sites across western North America. Some of these materials were collected from preserved herbarium specimens while others were collected from live plants in the field. To capture variation within a species, starch granules were extracted from three geographically dispersed individuals (replicate samples) and described using an array of morphometric characteristics (e.g., size, shape, surface features, response to polarized light). Source and collection data for can be found in Supplementary Table S1. Plant nomenclature follows USDA GRIN http://www.ars-grin.gov/∼sbmljw/johnindex.html.
Different plant parts that most likely contain starch (seeds, fruits, nuts, endosperm, bulbs, tubers, caryopses, and taproots) were processed according to standard protocols (e.g., Torrence and Barton 2006) in the Natural History Museum of Utah (NHMU) Archaeobotany Lab. Material was ground using a sterile mortar and pestle and was sieved through a 125 µm mesh Endecott screen into a beaker using DH20. Sample material <125 µm was transferred to a sterile 50 ml test tube and each sample was centrifuged for 3 minutes at 3000 RPM. The supernatant was discarded, and the sample pellet was transferred to a sterile 15 ml test tube. Each test tube was re-suspended with a vortex mixer, adding 7 ml of lithium heteropolytungstate (LST; specific gravity 2.00–2.35), and then centrifuged for 15 min at 1000 RPM. The sample was extracted from the heavy liquid using a pipette, carefully removing the top 1–2 mm layer of organics containing starch and placed into new 15 ml test tubes. Each sample was rinsed three times until all residual heavy liquid was removed. Samples were then rinsed with acetone, mixed with a vortex, and centrifuged for 3 min at 3000 RPM. The acetone was decanted, and samples were covered and left to dry overnight. Once dried, the samples were mixed with 50% DH20 and 50% glycerol solution and then mounted on microscope slides.
Each slide was scanned using a transmitted brightfield microscope fitted with polarizing filters and Nomarski optics (Zeiss Axioscope 2, Zeiss International, Göttingen, Germany). A digital camera (Zeiss HRc) with imaging and measurement software (Zeiss Zen) were used to capture images of and measure starch granules. For each reference sample, randomly generated X-Y coordinates were used to measure and photograph approximately 100 granules from each individual plant (n=∼300 from each species). All starch granules present in these photographs were measured and examined for morphological characteristics.
Under ×400 magnification, the sizes, shapes, surface features and responses to polarized light of the starch granules were observed. Once identified and photographed, starch granules were described according to an established set of structural and surface characteristics (Reichert, 1913; ICSN, 2011) (Table 2). The size of each starch granule was measured as the maximum length through the hilum. Granules were also examined for the presence of morphological characteristics including 2-D shape, hilum position, central cavity, extinction cross, fissures, lamellae, pressure facets, and depressions along the margins (Reichert, 1913; ICSN, 2011). Starch granule size tends to be non-normally distributed and, therefore, relying on mean granule size is not appropriate for identification purposes (Louderback et al., 2017). Boxplots showing the distribution of starch granule size for each species was generated using the boxplot function in the “graphics” package for R v. 3.6.2 (Chambers et al., 1983; Becker et al., 1988; Murrell, 2005; R Core Team, 2019). To overlay individual granule size data, we used the jitter method within the stripchart function in the “graphics” package for R v.3.6.2.
TABLE 2. Description of starch granule morphological characteristics adapted from Reichert (1913) and ICSN (2011).
Size distributions among all possible pairs of the study species were statistically compared using the non-parametric Kolmogorov-Smirnov (K-S) test. Applying a statistical analysis to starch granule identification promotes reproducibility and an overall increase in confidence (Louderback et al., 2017; Gao et al., 2021). It is becoming standard practice to measure the size, shape and morphological characteristics of reference starch granules from multiple populations so that variation and statistical significance can be assessed (Liu et al., 2014a; Brown and Louderback, 2020; Wilks et al., 2021).
Starch granule measurements from individual species were pooled for overall size and morphological analyses (Supplementary Data S1). Smaller granules tend to exhibit few diagnostic characteristics, therefore, relying on the top 20% granule lengths allowed for greater differentiation between taxa because morphological characteristics occur more frequently in larger granules (Liu et al., 2014a; Louderback et al., 2017). Granule size distributions of all plant taxa are plotted in Figure 1. All comparisons were significantly different (p < 0.05), except between Cymopterus bulbosus and Lomatium triternatum (p = 0.08), Lomatium donnellii and Quercus agrifolia (p = 0.70), Lomatium donnellii and Quercus douglasii (p = 0.35), Quercus douglasii and Quercus agrifolia (p = 0.28), and Calochortus nuttallii and Erythronium grandiflorum (p = 0.38).
FIGURE 1. Starch granule size distributions for the upper 20% granule lengths. All comparisons were significantly different (p < 0.05) except between Cymopterus bulbosus and Lomatium triternatum, Lomatium donnellii and Quercus agrifolia, Lomatium donnellii and Quercus douglasii, Quercus douglasii and Quercus agrifolia, and Calochortus nuttallii and Erythronium grandiflorum.
Morphological characteristics, including 2D shape, hilum position, central cavity, extinction cross, fissures, lamellae, pressure facets, and depressions were documented for each granule for all 18 taxa (Supplementary Data S1). The frequency of those characteristics occurring on granules was calculated and expressed as a number between 0.0 and 1.0. (Figures 2A,B). Definitions for these characteristics were compiled primarily from ICSN (2011) and Reichert (1913), but some definitions were refined for this particular study based on previous work (Piperno et al., 2004, 2009; Holst et al., 2007; Perry and Quigg 2011; Musaubach et al., 2013; Yang and Perry 2013; Louderback et al., 2017; Brown and Louderback 2020; Joyce et al., 2021). All characteristics described above occur more frequently in the top 20% size range of starch granules and, therefore, we report frequencies for those granules (Figures 2A,B).
FIGURE 2. (A,B) Frequency of morphological characteristics observed and documented on the upper 20% sizes of starch granules from each species.
A dichotomous key for identifying starch granules of the seven plant families analyzed in this study was generated (Box 1). Because it is based only on a few species from each family, the key will undoubtedly be revised as more families, genera, and species are systematically analyzed using the characteristics presented herein. Furthermore, new techniques will lead to new diagnostic characteristics resulting in further revision and greater application of new diagnostic keys.
BOX 1
FEART_feart-2022-897183_wc_tfx1
Different characteristics dominate the array of plant families that we have examined (Figure 3). For example, Amaranthaceae produces miniscule granules (<5 µm) with centric hila and a circular/oval shape. Because they are so small, it is difficult to visually discern additional morphological characteristics. Furthermore, Amaranthaceae starch are often formed in dense clusters of amyloplasts (sheets) bounded by cell walls, so it is rare to see isolated granules (even in reference material, Figure 3) (Reichert, 1913; Louderback, 2014; Capparelli et al., 2015; López et al., 2015). Apiaceae also produces starch granules with centric hila, circular/oval shape, and are frequently observed with transverse or stellate fissures, visible lamellae, and pressure facets (Herzog, 2014; Joyce et al., 2021). Another family that produces circular/oval granules with centric hila is Pinaceae. What distinguishes these granules from those in other families is the obvious presence of a round central cavity (Tinsley et al., 2021). Fagaceae, on the other hand, have starch granules with slightly eccentric hila and are characterized by triangular-trapezoidal shape with confused crosses, mesial longitudinal clefts, and visible lamellae (Messner 2011; Liu et al., 2014b; Brown and Louderback 2020). Liliaceae and Solanaceae produce starch granules with clearly eccentric hila, longitudinal fissures, and visible lamellae, but Liliaceae granules tend to be more circular/oval while Solanaceae are almost always elongated (Messner 2011; Louderback et al., 2017; Ahituv and Henry 2022).
FIGURE 3. Images of starch granules from each study species (n=18), shown in both differential interference contrast (DIC) (Nomarski) (top rows) and polarized (bottom rows) views.
Of the plant families examined by this study, Poaceae exhibits the most variation in starch granule size, shape, surface structures, and responses to polarized light. For example, Achnatherum hymenoides and Sporobolus airoides produce very small granules (<10 µm) with centric hila, but their shape is often angular, thus distinguishing them from Amaranthaceae. Elymus elymoides (and most Triticeae grasses—barley, wheat, rye) produce distinctive starch granules that can be quite large (up to ∼30 µm), are circular/oval with oblong central cavities, indistinct crosses, and very visible lamellae. Additional characteristics (e.g., pits) have also been observed on these granules (Perry and Quigg, 2011; Yang and Perry 2013; Brown and Louderback, 2020). Finally, Zea mays is another grass genus that produces unique granules. These have a significantly different size range from Elymus, Achnatherum, and Sporobolus (Figure 1) and they also have an irregular shape with transeverse/stellate fissures that are not observed in other Poaceae taxa (Musaubach et al., 2013; Wilks et al., 2021).
The current study provides a standardized, systematic approach to defining characteristics that identify starch granules of seven major North American plant families (Amaranthaceae, Apiaceae, Fagaceae, Liliaceae, Pinaceae, Poaceae, and Solanaceae) that have dietary importance to humans. This approach was based on large numbers of reference starch granules from multiple plant populations of different genera. Our results revealed distinctive and, therefore, diagnostic characteristics of starch granules among the different families. A dichotomous key was developed based on these characteristics to aid in the identification of archaeological starches. Although the focus was on plant taxa from western North America, we find that our descriptions for size and morphological characteristics of starch granules are generally consistent with other studies at the genus and family levels. This includes plants from regions across the globe, such as Central and South America (e.g., Holst et al., 2007; Piperno et al., 2009; Musaubach et al., 2013; Capparelli et al., 2015; Lopez et al., 2015), China (e.g., Yang et al., 2012; Yang and Perry, 2013; Wang et al., 2019), the Mediterrean (Ahituv and Henry 2022), and eastern North America (Messner 2011).
Characteristics highlighted in our diagnostic key, however, will probably require revision once more genera are systematically analyzed. For example, the size, shape, and surface features of Poaceae starch are known to vary significantly among genera (e.g., Yang and Perry, 2013). It may be more important, therefore, to focus systematic surveys and identifications at the level of subfamilies or tribes in particularly diverse plant families.
Other intrinsic (genetic) and extrinsic (environmental) variables need to be analyzed with respect to starch granule characteristics and dynamics. Greater attention should be paid to sources of variation, particularly at the population level, and within large and diverse taxa, such as Poaceae. Furthermore, physiological variables, such as phenology, plant developmental stages, and seed maturation and fruit ripening processes should be addressed to understand how granule characteristics are affected. The taphonomy of starch granules and how that affects preservation, and ultimately the reliability of the identifications based upon subtle and microscopic characteristics, should be further assessed. However, this and many other studies to date continue to improve reliability of starch granule analysis in the understanding of plant foods in ancient human diets.
The original contributions presented in the study are included in the article/Supplementary Material, further inquiries can be directed to the corresponding author.
LL: conceptualization, formal analysis, methodology, writing and editing of original draft; SW: data analysis, figures, review and editing; NH: data analysis, figures, review and editing; GB: data analysis, review and editing; KJ: data analysis, review and editing; BP: botanical expertise, methodology, writing and editing of original draft.
Funding for this project came from a variety of sources over many years. These include National Science Foundation (award no. BCS-1827414), Lincoln County Archaeological Initiative (Nevada Bureau of Land Management), Oregon Bureau of Land Management (Award L20AS00005), Range Creek Archaeological Field Station, Department of Anthropology, University of Utah, and the Natural History Museum of Utah.
Author GHB is currently employed by Pacific Legacy, Inc., but was a graduate student at California State University, Sacramento when research was conducted for this study.
The remaining authors declare that the research was conducted in the absence of any commercial or financial relationships that could be construed as a potential conflict of interest.
All claims expressed in this article are solely those of the authors and do not necessarily represent those of their affiliated organizations, or those of the publisher, the editors and the reviewers. Any product that may be evaluated in this article, or claim that may be made by its manufacturer, is not guaranteed or endorsed by the publisher.
Many students participated in this study and helped collect data, including Lauren Lewis and Haden Kingrey. We wish to thank Daniel Calderon Sanchez for his help with microscopy and imaging. Also thank you to Ying Guan, Li Liu, and Xiaoyan Yang for putting together the special issue on starch analysis and to the Submissions Team at Frontiers in Earth Science.
The Supplementary Material for this article can be found online at: https://www.frontiersin.org/articles/10.3389/feart.2022.897183/full#supplementary-material
Ahituv, H., and Henry, A. G. (2022). An Initial Key of Starch Grains from Edible Plants of the Eastern Mediterranean for Use in Identifying Archaeological Starches. J. Archaeol. Sci. Rep. 42, 103396. doi:10.1016/j.jasrep.2022.103396
Becker, R. A., Wilks, A. R., and Chambers, J. M. (1988). The New S Language: A Programming Environment for Data Analysis and Graphics. Belmont, California: Taylor Francis.
Brown, G. H., and Louderback, L. A. (2020). Identification of Starch Granules from Oak and Grass Species in the Central Coast of California. J. Archaeol. Sci. Rep. 33, 102549. doi:10.1016/j.jasrep.2020.102549
Capparelli, A., Pochettino, M. L., Lema, V., López, M. L., Andreoni, D., Ciampagna, M. L., et al. (2015). The Contribution of Ethnobotany and Experimental Archaeology to Interpretation of Ancient Food Processing: Methodological Proposals Based on the Discussion of Several Case Studies on Prosopis Spp., Chenopodium Spp. and Cucurbita Spp. from Argentina. Veget. Hist. Archaeobot. 24, 151–163. doi:10.1007/s00334-014-0497-4
Chambers, J. M., Cleveland, W. S., Kleiner, B., and Tukey, P. A. (1983). Graphical Methods for Data Analysis. United Kingdom: Wadsworth Brooks/Cole Advanced Books Software.
Gao, M., Moussavi, M., and Myers, D. (2021). Analysis and Specification of Starch Granule Size Distributions. J. Vis. Exp. 169, 61586. doi:10.3791/61586
Herzog, N. M. (2014). Starch Grain Analysis in California and the Great Basin. Calif. Archaeol. 6, 171–189. doi:10.1179/1947461x14z.00000000039
Holst, I., Moreno, J. E., and Piperno, D. R. (2007). Identification of Teosinte, Maize, and Tripsacum in Mesoamerica by using Pollen, Starch Grains, and Phytoliths. Proc. Natl. Acad. Sci. U.S.A. 104, 17608–17613. doi:10.1073/pnas.0708736104
ICSN (2011). The International Code of Starch Nomenclature. Available at: http://www.fossilfarm.org/ICSN/Code.html (Accessed 10 12, 2021).
Joyce, K. T., Louderback, L. A., and Robinson, E. (2021). Direct Evidence for Geophyte Exploitation in the Wyoming Basin. Am. Antiq. 64, 659–674. doi:10.1017/aaq.2021.115
Liu, L., Kealhofer, L., Chen, X., and Ji, P. (2014b). A Broad-Spectrum Subsistence Economy in Neolithic Inner Mongolia, China: Evidence from Grinding Stones. Holocene 24 (6), 726–742. doi:10.1177/0959683614526938
Liu, L., Ma, S., and Cui, J. (2014a). Identification of Starch Granules Using a Two-Step Identification Method. J. Archaeol. Sci. 52, 421–427. doi:10.1016/j.jas.2014.09.008
López, M. L., Medina, M. E., and Rivero, D. E. (2015). First Records of Chenopodium spp./Amaranthus spp. Starch Grains and their Relevance to the Study of the late Holocene Human Subsistence in Central Argentina. Holocene 25 (2), 288–295. doi:10.1177/0959683614558652
Louderback, L. A., Herzog, N. M., and Pavlik, B. M. (2017). A new approach for identifying starch granules of wild food plants from arid western North America. Starch - Stärke 69 (5–6), 1600167. doi:10.1002/star.201600167
Louderback, L. A. (2014). The Ecology of Human Diets during the Holocene at North Creek Shelter, Utah. PhD dissertation. Seattle, WA: University of Washington. https://digital.lib.washington.edu/researchworks/handle/1773/25980.
Mercader, J., Akeju, T., Brown, M., Bundala, M., Collins, M. J., Copeland, L., et al. (2018). Exaggerated expectations in ancient starch research and the need for new taphonomic and authenticity criteria. Facets 3, 777–798. doi:10.1139/facets-2017-0126
Messner, T. C. (2011). Acorns and Bitter Roots: Starch Grain Research in the Prehistoric Eastern Woodlands. Tuscaloosa: University Alabama Press.
Musaubach, M. G., Plos, A., and Babot, M. D. P. (2013). Differentiation of Archaeological Maize (Zea Mays L.) from Native Wild Grasses Based on Starch Grain Morphology. Cases from the Central Pampas of Argentina. J. Archaeol. Sci. 40, 1186–1193. doi:10.1016/j.jas.2012.09.026
Nägeli, C. (1858). Die Stärkekörner. Morphologishe, Physiologishe, Chemisch-Physicalisch und Systematisch-Botansiche. Zurich: Verlag F. Schulthess.
Perry, L., and Michael Quigg, J. (2011). Starch Remains and Stone Boiling in the Texas Panhandle Part II: Identifying Wildrye (Elymusspp.). Plains Anthropol. 56, 109–119. doi:10.1179/pan.2011.011
Piperno, D. R., Ranere, A. J., Holst, I., Iriarte, J., and Dickau, R. (2009). Starch Grain and Phytolith Evidence for Early Ninth Millennium B.P. Maize from the Central Balsas River Valley, Mexico. Proc. Natl. Acad. Sci. U.S.A. 106, 5019–5024. doi:10.1073/pnas.0812525106
Piperno, D. R., Weiss, E., Holst, I., and Nadel, D. (2004). Processing of Wild Cereal Grains in the Upper Palaeolithic Revealed by Starch Grain Analysis. Nature 430, 670–673. doi:10.1038/nature02734
R Core Team (2019). R: A Language and Environment for Statistical Computing. Vienna, Austria: R Foundation for Statistical Computing.
Reichert, E. T. (1913). The Differentiation and Specificity of Starches in Relation to Genera, Species, Etc.: Stereochemistry Applied to Protoplasmic Processes and Products, and as a Strictly Scientific Basis for the Classification of Plants and Animals. Washington, DC: Carnegie Institution of Washington.
Shannon, J. C., Garwood, D. L., and Boyer, C. D. (2009). “Genetics and Physiology of Starch Development,” in Genetics and Physiology of Starch Development” in: Starch: Chemistry and Technology. Editors J. BeMiller, and R. Whistler (New York: Academic Press), 23–82. doi:10.1016/b978-0-12-746275-2.00003-3
Tinsley, D., Louderback, L., Pavlik, B., Baker, M., Townsend, T., Tucker, K., et al. (2021). Testing the Pinyon Premise: Archaeobotanical Analyses of Sediments, Bedrock Milling Features, and Ground Stone Artifacts from West-Central Lincoln County, Nevada. Ely, Caliente, NV: Prepared for BLM Ely District and Caliente Field Offices.
Wang, J., Zhao, X., Wang, H., and Liu, L. (2019). Plant Exploitation of the First Farmers in Northwest China: Microbotanical Evidence from Dadiwan. Quat. Int. 529, 3–9. doi:10.1016/j.quaint.2018.10.019
Wilks, S., Louderback, L. A., and Boomgarden, S. (2021). Starch Granule Size and Morphology as a Proxy for Water Regime Influence on Zea mays. EblEthnobio. Lett. 12 (1), 35–43. doi:10.14237/ebl.12.1.2021.1725
Yang, X., and Perry, L. (2013). Identification of Ancient Starch Grains from the Tribe Triticeae in the North China Plain. J. Archaeol. Sci. 40 (8), 3170–3177. doi:10.1016/j.jas.2013.04.004
Keywords: starch granule analysis, taxonomic identification, western North America, human evolution, starch-rich foods
Citation: Louderback LA, Wilks S, Herzog NM, Brown GH, Joyce K and Pavlik BM (2022) Morphometric Identification of Starch Granules From Archaeological Contexts: Diagnostic Characteristics of Seven Major North American Plant Families. Front. Earth Sci. 10:897183. doi: 10.3389/feart.2022.897183
Received: 15 March 2022; Accepted: 20 June 2022;
Published: 19 August 2022.
Edited by:
Xiaoyan Yang, Institute of Tibetan Plateau Research (CAS), ChinaReviewed by:
Thomas Hart, Franklin & Marshall College, United StatesCopyright © 2022 Louderback, Wilks, Herzog, Brown, Joyce and Pavlik. This is an open-access article distributed under the terms of the Creative Commons Attribution License (CC BY). The use, distribution or reproduction in other forums is permitted, provided the original author(s) and the copyright owner(s) are credited and that the original publication in this journal is cited, in accordance with accepted academic practice. No use, distribution or reproduction is permitted which does not comply with these terms.
*Correspondence: Lisbeth A. Louderback, bGlzYmV0aC5sb3VkZXJiYWNrQGFudGhyby51dGFoLmVkdQ==
Disclaimer: All claims expressed in this article are solely those of the authors and do not necessarily represent those of their affiliated organizations, or those of the publisher, the editors and the reviewers. Any product that may be evaluated in this article or claim that may be made by its manufacturer is not guaranteed or endorsed by the publisher.
Research integrity at Frontiers
Learn more about the work of our research integrity team to safeguard the quality of each article we publish.