- 1Department of Pharmacognosy, School of Pharmacy, Qingdao University, Qingdao, China
- 2Department of Science and Technology, Qingdao University, Qingdao, China
- 3Shandong Wendeng Jizhen Ginseng Industry Co.Ltd., Weihai, China
American ginseng (Panax quinquefolius L.) has been cultivated in many locations in China, and we have noticed that its phenological performance and quality characteristics varied from location to location. However, the exogenous factors driving this process are still poorly understood, and this reduces the successful introduction of American ginseng for quality ensuring. Here, we conducted field and cabinet experiments to explore the relationship among environmental factors, phenological development, and ginsenoside accumulation in American ginseng. In the field experiments, we found that American ginseng from different original locations showed different phenological rhythms and different ginsenoside accumulation. Especially, those from higher latitude locations (e.g., Wuchang, 44.55°N) presented delayed spring phenology and advanced autumn phenology, but higher ginsenoside contents along with higher gene expression levels of ginsenoside biosynthesis than those from lower latitude locations (e.g., Wendeng, 37.23°N). Data analysis indicated that the phenological rhythm of American ginseng is closely related to the seasonal change in environmental factors, especially winter chilling duration, spring warming, and autumn day-length shortening. In the cabinet experiments, results further proved that temperature interaction with photoperiod plays a decisive role in the phenological development and ginsenoside accumulation of American ginseng in the absence of water and nutrient limitation. Both field and cabinet experiments indicated that sufficient winter cold exposure coupled with spring long day-length photoperiod is a beneficial environmental factor for normal spring phenology development, while shortening autumn day-length coupled with autumn cooling is a beneficial environmental factor for normal autumn phenology development of American ginseng. Results also indicated that insufficient cold exposure and unsuitable day-length photoperiod are detrimental factors to both spring and autumn phenology, and are not good for ginsenoside accumulation in American ginseng. An understanding of the environmental factors influencing phenological development along with ginsenoside accumulation can provide guidance for predicting suitable cultivation locations and improving planting techniques for high yield and good quality of American ginseng.
Introduction
American ginseng (Panax quinquefolius L.), a relative species of Asian ginseng (Panax ginseng C. A. Meyer) in the family of Araliaceae, is widely used in Chinese traditional medicine for its precious roots which have been demonstrated to have beneficial effects on human health (Wei et al., 2019; Huang et al., 2021). American ginseng can improve immunity, slow the aging process, prevent certain cancers, and protect against cardiovascular disease because of the presence of numerous bioactive substances, especially ginsenosides (Qi et al., 2011; Bian et al., 2021). The ginsenoside content differs greatly depending on the environment of the growing region and also determines the quality and corresponding market price of American ginseng (Huang et al., 2013; Zhang et al., 2018; Liao et al., 2019; Sun et al., 2019; Hao et al., 2020).
Wild American ginseng with slow growing rates is mainly distributed in the United States and Canada, and is artificially cultivated over a hundred years ago in the United States due to the shortage of wild resources (Pritts, 1995). This species was introduced successfully into China during the 1970s (Li, 1995). Currently, in China, American ginseng is mainly cultivated in Beijing, Shandong Province, Jilin Province, and Heilongjiang Province (Liao et al., 2019). However, suitable regions for American ginseng are increasingly shrinking because of replant failure and soil degradation in China (Zhang et al., 2018). Therefore, it is vital to hunt new regions for American ginseng cultivation in order to meet the huge market demand. However, American ginseng introduction is very difficult, and some questions should be answered in order to produce high-yielding and good-quality ginseng roots in new locations: Can this species complete its life cycle in a new location? What are the variations in the quality and yield of this species’ products? The yield and quality of many medicinal plants are usually variable when it is introduced into a new area, which is caused by the specific local environments. Meanwhile, the plants usually presented changes in phenological stages due to local adaptation (Huang et al., 2012; Shen et al., 2017; Stucky et al., 2018; Sun et al., 2019; Sheban et al., 2021). Therefore, it is thought that the phenological performance of an introduced medicinal plant is closely related to the variation in the quality and yield of the medicinal plant, which are induced by local environmental factors.
As a high-latitude medicinal plant, American ginseng has adapted to the local climate and other conditions of original wild habitats involving a geographical location. American ginseng probably is a cold-dependent and long day-length plant species according to its original wild habitats (Hao et al., 2020; Sheban et al., 2021). When it is introduced into a new different area, the phenological rhythm of American ginseng often changes significantly (Li, 1995; Pritts, 1995; Huang et al., 2013; Zhang et al., 2018; Sun et al., 2019). The change in American ginseng phenological rhythm just reflects the difference in the environmental factors of its original habitats (Pritts, 1995). The phenological rhythms of American ginseng and Asian ginseng from some locations have been observed and recorded in detail (Proctor et al., 2003; Kim et al., 2020). Moreover, there are many reports about the differences in quality and yield of American ginseng from different cultivated locations (Huang et al., 2013; Zhang et al., 2018; Liao et al., 2019; Sun et al., 2019; Hao et al., 2020). However, few studies have established a relationship between the phenological performance and ginsenoside accumulation in American ginseng. Therefore, we think that connecting the phenological performance and ginsenoside accumulation in American ginseng will help us disclose the mechanism of quality formation in American ginseng.
Research on the phenological dynamics of secondary metabolite accumulation induced by environmental factors in medicinal plants is not rich. It is generally agreed that meteorological factors, among many environmental factors, change the phenological rhythm of plants significantly, and they can also cause variation in secondary metabolite accumulation in medicinal plants (Mishra, 2016; Li et al., 2020; Pant et al., 2021). Many exogenous factors can change the plant phenological performance, such as temperature, sunshine, precipitation, and soil nutrients (Andresen et al., 2018; Shen et al., 2018; Ren et al., 2022). Temperature and photoperiod are the two most important meteorological factors influencing the plant phenology, which modulate plant phenological development (especially the time rhythm of sprouting, flowering, and fruiting) cooperatively and interactively (Weikai and Wallace, 1998; Adams and Langton, 2005; Li et al., 2016; Wu et al., 2020). However, the developmental stages of medicinal plants have a significant influence on the content and composition of secondary metabolites (Li et al., 2020); this is because the developmental factors influence the initiation and subsequent differentiation of particular tissues involved in the biosynthesis of secondary metabolites. Furthermore, particular tissues and the developmental stages influence the expression pattern of genes related to secondary metabolite biosynthesis (Padilla-González et al., 2019; Li et al., 2020). For this reason, a large number of secondary metabolites often occur at a certain stage of plant growth and development (Padilla-González et al., 2019; Hao et al., 2020). We hypothesized that the changes in phenological development induced by temperature and photoperiod when American ginseng is introduced into a new location will finally affect the ginsenoside biosynthesis significantly.
In view of the aforementioned findings and hypothesis, the mechanism of ginsenoside accumulation related to phenological performance in American ginseng induced by temperature and photoperiod should be studied clearly for producing high-yielding and good-quality ginseng roots in a wider range of introduction locations.
Materials and Methods
Plant Material and Experimental Design
Three-year-old seedlings of American ginseng grown in the fields of seven cultivated locations (Wendeng, Wuchang, Ningan, Tonghua, Changbai, Liuba, and Huairou) in China (Figure 1) were used to study the relationship among environmental factors, phenological rhythms, and ginsenoside accumulation. Three-year-old seedlings of American ginseng grown in the growth cabinets were used to study the co-effects of temperature and photoperiod on phenological rhythms and ginsenoside accumulation.
Field Experiments
Field experiments were conducted on 3-year-old American ginseng in seven cultivated locations during 2018 and 2019. Phenological observations of the American ginseng seedlings were performed synchronously in each location according to the method described by Wan and Liu (1979). The annual phenology of American ginseng was observed, including dormancy, initiation, vegetation, flowering, fruiting, and senescence. The seedlings were observed once every 2 days at about 2 p.m. and the date when American ginseng reaches the target phenology was recorded. To be able to compare the timing of phenological events, the dates were transformed into Julian date (1 corresponding to 1 January).
The meteorological data of the seven cultivated locations of American ginseng were collected from the National Meteorological Information Centre (http://data.cma.cn) (Table 1; Figure 1). The meteorological data were converted into raster data by ArcGIS software with spatial interpolation. The meteorological data of each sampling plot were obtained according to their longitude and latitude, which included Tmin Jan, minimum temperature in January; Tave Jan, average temperature in January; Tmax Jul, maximum temperature in July; Tave Jul, average temperature in July; Tcum Ann, annual cumulative temperature; Tave Ann, annual average temperature; Scum Ann, annual cumulative sunshine hours; DL sprouting, average day-length during sprouting; DL flowering, average day-length during flowering; DL Ann, annual average day-length; Scum pre-flowering, cumulative sunshine hours before flowering; RH Ann, annual average air relative humidity; and P Ann, annual average precipitation.
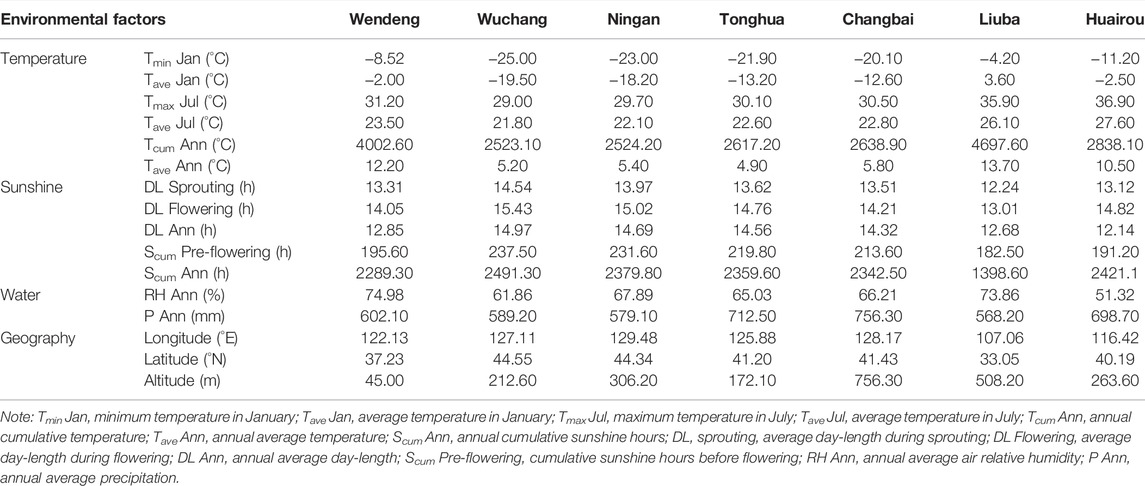
TABLE 1. Overall comparison of environmental factors among seven cultivated locations of American ginseng from 2017 to 2019 in China.
Focusing on two cultivated locations (Wendeng and Wuchang), the seedlings were sampled in triplicate × 2 from fields at the onset dates of each phenological stage in 2018 and 2019. One triplicate of the samples was dried to a constant weight (50°C for 72 h) after washing and cutting them into different parts for determining the biomass and the ginsenoside contents. Another triplicate of samples was stored in a −70°C refrigerator quickly after washing and cutting them into different parts for determining the related gene expression levels of ginsenoside biosynthesis.
Growth Cabinet Experiments
Growth cabinet experiments were conducted in intelligent artificial climate growth cabinets (RXZ-type, Ningbo Jiangnan Instrument Factory, China). In early October 2018, the intact dormant roots of 2-year-old American ginseng of uniform size were collected from Wendeng, and each root was transported in a pot (8 cm × 20 cm) containing vermiculite and sand (1:1). The pots were equipped with an automatic infiltration irrigation system and Hoagland nutrient solution. The pots were arranged within the cabinets and rearranged weekly for minimizing edge effects.
In the growth cabinets, six treatment combinations of temperature and photoperiod were set up (Figure 2): 1) sufficient low temperature and long day-length; 2) sufficient low temperature and short day-length; 3) no low temperature and long day-length; 4) no low temperature and short day-length; 5) semi-low temperature duration and long day-length; and 6) semi-low temperature duration and short day-length. The temperature and photoperiod in control treatment CK1 were set according to the weather forecast from Wuchang and adjusted in time according to the daily changes; CK2 were set according to the weather forecast from Wendeng and adjusted in time according to the daily changes. The air relative humidity and plant root nutrients were consistent for each seedling of American ginseng under different treatments in the growth cabinets.
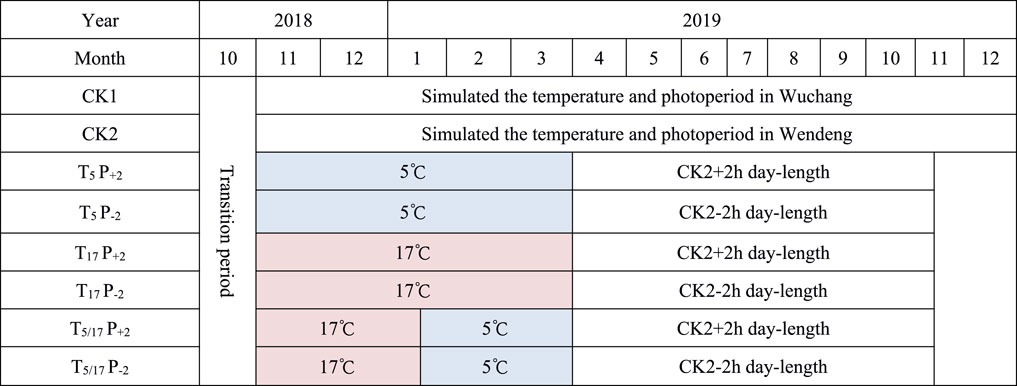
FIGURE 2. Schematic diagram of experimental schedule and treatment timing in cabinet experiments. Note: CK1, simulated the temperature and photoperiod in Wuchang; CK2, simulated the temperature and photoperiod in Wendeng; T5P+2, temperature during dormancy is 5°C, day-length during growth period is natural day-length in Wendeng +2 h; T5P-2, temperature during dormancy is 5°C, day-length during growth period is natural day-length in Wendeng − 2 h; T17P+2, temperature during dormancy is 17°C, day-length during growth period is natural day-length in Wendeng +2 h; T17P-2, temperature during dormancy is 17°C, day-length during growth period is natural day-length in Wendeng − 2 h; T5/17P+2, temperature during dormancy is 5/17°C, day-length during growth period is natural day-length in Wendeng +2 h; T5/17P-2, temperature during dormancy is 5/17°C, day-length during growth period is natural day-length in Wendeng − 2 h.
Phenological observations of seedlings in the growth cabinets were performed daily. The seedlings were sampled in triplicate × 2 at the onset and end dates of each phenological stage. The biomass, the ginsenoside contents in roots, and the related gene expression levels in leaves of ginsenoside biosynthesis were determined as aforementioned.
Ginsenoside Extraction and HPLC Analysis
The determination of ginsenosides was according to the method described by Hao et al. (2020) with some modifications. The samples from different parts of American ginseng plants collected during different phenological stages were ground into powder with a medicine grinder and filtered through a 355-mm sieve. Then, 1 g of the powder was accurately weighed and placed in a corked triangle flask. To the powder, 50 ml of 80% (V/V) methanol solution was added for ultrasonic extraction for 60 min. The flask was placed until it reached room temperature, and methanol was used to make up the reduced weight. The extract was filtered and then evaporated to remove the solvent. After the liquid evaporated, the residue was dissolved in distilled water and extracted three times with water-saturated n-butanol. The n-butanol layer was then evaporated to obtain ginsenosides. Each extracted sample was dissolved in HPLC-grade methanol (1.0 g/5 ml) and then filtered through a 0.45-μm microporous membrane for HPLC analysis.
The HPLC analysis was performed using an Agilent 1260 HPLC system (Agilent Technologies Co. Ltd., United States) equipped with a diode array detector and an OpenLAB services for data processing. The separation was performed on a Zorbax SB-C18 column (5 μm, 250 × 4.6 mm). Authentic standards of ginsenosides Rb1, Re, and Rg1 were obtained from Chengdu DeSiTe Phyto-chemicals Ltd (Chengdu, Sichuan, China). The standards and samples were separated using a gradient mobile phase consisting of acetonitrile (A) and 0.1% phosphoric acid in water (B). The gradient elution program is 0–10 min, 21% A; 10–16 min, 21–24% A; 16–17 min, 24–35% A; 17–20 min, 35% A; 20–25 min, 35–60% A; 25–30 min, 60–70% A, and finally, to recondition the column, 21% A at 33 min for 5 min.
The injection volume was 10 uL, the flow rate was set at 1.0 ml min-1, and the column temperature was 40°C. The detection wavelength was set at 203 nm. Standard curves were constructed by plotting the peak areas (mAU min−1) versus the amount (ug) of each analyte injected. The content in the samples was calculated by the following formula:
where C is the concentration of a ginsenoside (mg/ml) calculated through the standard curve; V is the sample extract volume (ml); and W is the sample dry weight (g).
Gene Expression Level Analysis of Ginsenoside Biosynthesis
Ginsenosides are mainly biosynthesized utilizing the precursor isopentenyl pyrophosphate (IPP) through the mevalonic acid (MVA) pathway and its condensation with dimethylallyl diphosphate (DMAPP) to produce the central intermediate farnesyl pyrophosphate (FPP). Then squalene is synthesized from two FPP catalyzed by squalene synthase (SS). Squalene is the precursor for both protopanaxadiol (PPD) and protopanaxatriol (PPT) types of ginsenosides. Two key reaction steps from squalene to dammarenediol-II are catalyzed by squalene epoxidase (SE) and dammarenediol synthase (DS), respectively. The next key reaction step from dammarenediol to protopanaxadiol (PPD) is catalyzed by dammarenediol-12-hydroxylase (D12H). So we selected the three key genes (PqSE: encoding squalene epoxidase in P. quinquefolius, PqDS: encoding dammarenediol synthase in P. quinquefolius, and PqD12H: encoding dammarenediol-12-hydroxylase in P. quinquefolius) that are involved in ginsenoside biosynthesis in American ginseng (P. quinquefolius) for validation using RT-qPCR. The gene expression level analysis was according to the method described in some literature (Wang et al., 2014; Yang et al., 2017; Wang et al., 2019; Hao et al., 2020) with some modifications. About 50–100 mg of American ginseng leaves samples was ground into powder in liquid nitrogen. Total RNA was extracted using the RNeasy Plant Mini Kit including DNase I digestion following the manufacturer’s instructions (QIAGEN, Hilden, Germany). RNA purity and concentration were measured using a NanoDrop ND-1000 UV–Vis spectrophotometer (Thermo Fisher Scientific, United States). RNA extracts were reverse-transcribed into cDNA with a ReverTra Ace® qPCR RT Master Mix with gDNA remover (Toyobo, Osaka, Japan). The qRT-PCR was performed using cDNA in a reaction volume of 25 μL using SYBR Premix Ex Taq (TaKaRa, Kyoto, Japan) in a 7500 Real-Time PCR System (Applied Biosystems, Foster City, CA, United States). The thermal cycler conditions recommended by the manufacturer were 95°C for 30 s, followed by 35 cycles at 94°C for 30 s, 56°C for 30 s, and 72°C for 30 s. The fluorescent product was detected during the final step of each cycle. Amplification, detection, and data analysis were carried out on a Rotor-Gene 6000 real-time rotary analyzer (Corbett Life Science, Sydney, Australia). The primers used were 5′-CGT GGC TAT CTA TGG CGT TG-3′ and 5′-GGG AGC TCT GTA GTA AGC AG-3′ for PqSE, 5′-TTT GGT AGT CAA CTA TGG GA-3′ and 5′-AAC CAC CTT CTT CAT TTT-3′ for PqDS, and 5′-TTT GGT TCC CGA GCA GTG-3′ and 5′-GAG TCC GGC CTC TAT GT-3′ for PqD12H. To determine the relative fold differences in template abundance for each sample, the Ct values for each of the gene-specific primers were normalized to the Ct value for β-actin (5′-AGA GAT TCC GCT GTC CAG AA-3′ and 5′-ATC AGC GAT ACC AGG GAA CA-3′).
Data Analysis
SPSS 20.0 (Chicago, IL., United States) and Microsoft Excel 2013 were used for statistical evaluations. The results are presented as mean ± standard deviation (SD). One-way analysis of variance (ANOVA) was used to determine the differences between data, and the differences in the means were obtained using Duncan’s multiple comparison test at p < 0.05. Principal component analysis (PCA) was first performed to reduce the number of dimensions in the data set of environmental factors by extracting several factors associated with the phenological events. Pearson’s correlation coefficient (r) was performed to assess the association among the environmental factors, the phenological events, the contents of ginsenosides, and the gene expression levels of ginsenoside biosynthesis. Multiple linear stepwise regression analysis was used to obtain the regression equation of the contents of ginsenosides against the important environmental factor.
Results
Principal Component Analysis
Principal component analysis (PCA) was performed to reduce the number of dimensions in the data set on 16 environmental factors (Table 1; Figure 1) from the seven cultivating locations of American ginseng. According to the PCA results (Table 2), the first four principal components (PC1–4), with eigenvalues >1, explained 93.056% of the total variability among the 16 variables in the original data, where PC1, PC2, PC3, and PC4 contributed 46.777, 30.269, 8.368, and 7.643% of the total variance, respectively. PC1, which described 46.777% of the total variance of the data, was positively correlated to Tmin in January, Tave in January, Tmax in July, Tave in July, Tcum Ann, and Tave Ann; these factors can be classified as temperature factors. PC2, which described 30.269% of the total variance of the data, was positively correlated to DL sprouting, DL flowering, DL Ann, Scum pre-flowering, and Scum Ann; most of these factors are sunshine factors. PC3, which described 8.368% of the total variance of the data, was positively correlated to RH Ann and P Ann, so this can be classified as water factors. PC4, which described 7.643% of the total variance of the data, was positively correlated to latitude, so it can be classified as a geographical factor. It can be inferred from these results that the effect of temperature and sunshine factors is greater than that of water and geographical factors on the phenological events of American ginseng.
Different Climatic Characteristics Between the Two American Ginseng Cultivated Locations
The climatic characteristics of the two cultivated locations (Wendeng and Wuchang) are obviously different based on the climatic data during 2018 and 2019. In Wendeng, temperatures ranged from −11.2 to 35.1°C with an average of 12.2°C during 2018 and −7.2 to 35.8°C with an average of 13.6°C during 2019. In Wuchang, temperatures ranged from −25.3 to 37.2°C with an average of 5.2°C during 2018 and −27.3 to 30.9°C with an average of 5.4°C during 2019. In the two cultivated locations (Wendeng and Wuchang), temperature fluctuates similarly with high in June–August and low in December–February, but the winter temperature in Wuchang is much lower than that in Wendeng. In the two cultivated locations (Wendeng and Wuchang), both annual sunshine hours and monthly sunshine hours always present a constant difference due to the difference in the latitude of the earth, and the daily photoperiods also present a constant difference. The photoperiod in Wendeng varied from 9.35 to 14.86 h per day during a whole year, and maximum and minimum photoperiods were recorded on 21 June and 26 December, respectively. The photoperiod in Wuchang varied from 8.92 to 15.64 h per day during a whole year, and maximum and minimum photoperiods were recorded on 21 June and 21 December, respectively. In particular, we noticed that the day-length photoperiods in Wuchang are always longer than that in Wendeng during the American ginseng growing season (from March to September every year), while that in Wuchang is always shorter than that in Wendeng during the American ginseng dormant season (from September to March in the next year). In addition, annual or monthly precipitation also presented a difference between the two cultivated locations (Wendeng and Wuchang) (Figures 3A,B,C).
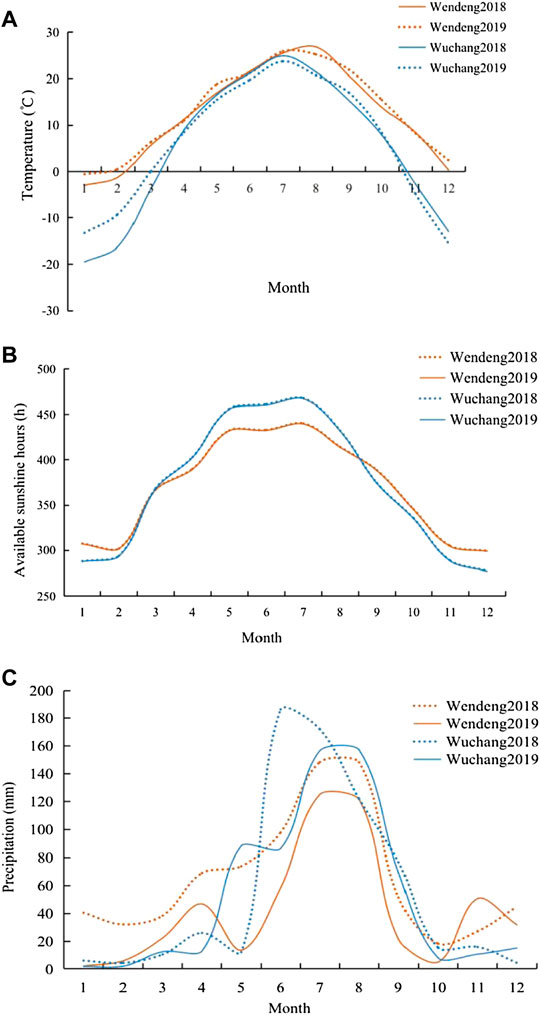
FIGURE 3. Comparison of temperature (A), sunshine (B), and precipitation (C) between Wendeng and Wuchang during 2018 and 2019.
Different Phenological Performance of American Ginseng From Two Cultivated Locations
We observed the phenological events of American ginseng in the field of two cultivated locations (Wendeng and Wuchang) and in the growth cabinets imitating the two locations’ climate to determine the difference in their phenological performance. The phenological performance of American ginseng growing both in the field and in the growth cabinets (CK1 and CK2) was very similar, no matter whether the provenance of American ginseng comes from Wengdeng or Wuchang. Six principal stages were used to describe the phenology of American ginseng (Figure 4).
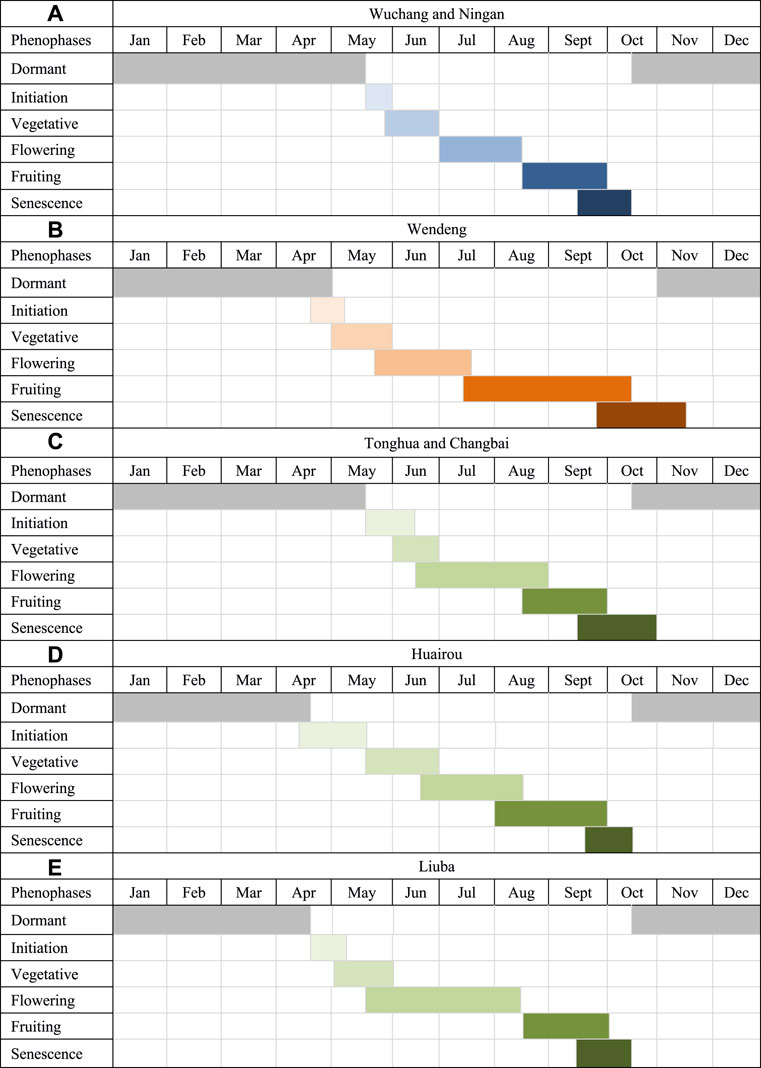
FIGURE 4. Comparison of phenological stages of American ginseng in seven cultivated locations in China.
Initiation: This stage refers to the period that plants emerged from underground roots. At this stage, American ginseng (over 2 years old) stems and inflorescences arise from an apical perennating bud on the underground rhizome on top of the root after an obligatory cold period to satisfy dormancy. Once the soil temperature reaches about 8°C (mid-April in Wendeng; mid-May in Wuchang), the perennating bud swells and becomes active (Figure 4). The membranous bud scales enclosing the shoot primordium separate, exposing the hooked stem. This hooked stem emerges from the bud scales and grows toward the soil surface. Emergence is complete when the hooked stem breaks through the soil surface, exposing folded leaves.
Vegetative: This stage refers to the period when the folded leaves become fully unfolded and the aerial stem has reached maximum height, which usually takes about 4 weeks in Wendeng and 3 weeks in Wuchang. At this stage, the fresh weight of the root decreases slightly (from the late April sprouting period to late May in Wendeng, and from the late May sprouting period to late June in Wuchang) until the crop canopy is completed. Next, the root growth starts to increase linearly from mid-June in Wendeng and from late-June in Wuchang, and it is almost fully grown by mid-October in Wendeng and by late-September in Wuchang.
Flowering: The flowering period (including inflorescence development and flower opening) lasted for about 6 weeks in Wendeng and for about 4 weeks in Wuchang, and each plant usually flowered for 1–3 weeks. Inflorescence growth is rapid during June in Wendeng and during July in Wuchang. After inflorescence diameter is maximized, inflorescence growth is slow for the remainder of the growing season. Flower opening in Wendeng begins in late May and continues to late July, while flower opening in Wuchang begins in early July and continues to mid-August. Flowers continue to open until most petals have fallen. At the end of flowering, most of the fruits, which are green, will have set.
Fruiting: Once flowering had occurred, fruits were set rapidly, but fruit growth is slow. This stage is from early July to early October in Wendeng and from mid-late August to late September in Wuchang. Once the fruits have set, at 6–7 weeks after flowering starts, they are still green. Gradually the fruits start to change color from green to red. As the fruits turn red, they can be easily dislodged from the inflorescences. Seeds disperse when the fruits drop to the ground in September and early October in Wendeng and in mid-late August and September in Wuchang.
Senescence: Following fruit abscission, there is a 3- to 4-week period when the foliage remains green, and then the plants start to turn yellowing during this period. The yellowing of the leaves continues and they droop. Leaf abscission occurs and the stems turn yellow. In commercial practice, this stage is used as the time for root harvest. If the plant canopy is not removed, all leaves abscise and the stems turn brown, but remain erect and persist. The other part of the plant that remains is the root, the harvestable product.
Dormant: Aboveground plant parts died during the period in early November in Wendeng and in mid-October in Wuchang, leaving the rhizome to perennate. This period lasted about 170 days in Wendeng and about 220 days in Wuchang.
Phenological Stages and Temperature
In field experiments, we found that the fluctuation of temperature during the whole phenological stages of American ginseng has a significant impact on its growth and development. When the temperature is above 10°C and the soil temperature is above 7°C at 10 cm depth, the seedlings can sprout. The plant can grow normally only when the temperature is above 20°C and the soil temperature is about 15°C; only when the temperature is about 28°C and the soil temperature is about 20°C, flowers can bloom and then the fruit can be produced; when the temperature is below 12–14°C and the soil temperature is 10°C at a depth of 10 cm, it enters the withering period. Therefore, the suitable temperature for the whole growth period of American ginseng is 10–28°C. Too high and too low temperature has different effects on each growth stage of American ginseng. Pearson correlation analysis (Table 3) indicated that temperature factors are closely related to the 3 important phenological events (sprouting, flowering, and dormancy) of American ginseng. In particular, the cumulative temperature during winter was significantly negatively correlated with the initial time of sprouting and flowering, while the cumulative temperature in March and April before sprouting was negatively significantly correlated with the initial time of sprouting (p < 0.01).
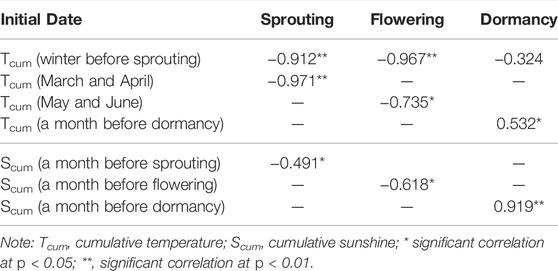
TABLE 3. Pearson correlation analysis between the key phenological stages and the key environmental factors.
In cabinet experiments (Table 4), we found that American ginseng had very little sprouting and no flowering during the whole growth period under two photoperiod treatments of no low temperature treatments (17°C during the whole winter). However, after enough low temperature treatments (5 months), both shortened and prolonged photoperiods could ensure the percentage of sprouting and flowering reached 50%, while only with semi-low temperature duration treatments (2.5 months), the percentage of sprouting and flowering decreased to less than 30%. Under both shortened and prolonged photoperiod treatments, the time of sprouting and flowering was delayed by 13–28 days and 21–23 days, respectively. Therefore, insufficient cold experiences (2.5 months) can shorten the growing season by 26–30 days.
Phenological Stages and Photoperiod
In field experiments, we found that the gradual changing of sunshine duration (photoperiod) during the whole phenological stages of American ginseng also has a significant impact on its growth and development. The photoperiod sensitive stage included the sprout initial stage (plants change from dormant state to active growth state in the spring), the flower initial stage (gradually increasing day length promotes flowering initiation), and the plant senescence stage (gradually decreasing day length accelerates the “die-back” of plants in the fall). We observed that the duration of both the sprouting initial stage and the senescence stage has been greatly shortened in Wuchang than in Wendeng (Figure 4). We also observed that American ginseng flowered more uniformly, the flowering percent is higher, and the time from sprouting to flowering is greatly shortened in Wuchang than in Wendeng though the flowering initial date was delayed in Wuchang. We think that this is related to the faster temperature rise and the longer day-length in Wuchang than in Wendeng. The Pearson correlation analysis (Table 3) indicated that photoperiod factors are also closely related to the 3 important phenological events (sprouting, flowering, and dormancy) of American ginseng, of which the cumulative sunshine during 1 month before dormancy was significantly negatively correlated with the initial time of American ginseng dormancy (p < 0.01).
In cabinet experiments (Table 4), we found that after enough low temperature treatments (5 months), the prolonged photoperiod (natural + 2 h) could advance the time of sprouting and flowering by 4–9 days and increase the percentage of sprouting and flowering by 3–10%, and the growing season is extended by 9 days, while the shortened photoperiod (natural − 2 h) could delay the time of sprouting and flowering by 21–27 days, and reduce the percentage of sprouting and flowering by 26–28%. But under only semi-low temperature duration treatments (2.5 months), sprouting time under the prolonged photoperiod (natural + 2 h) was 40 days earlier than that under the shortened photoperiod (natural − 2 h), and the percentage of flowering increased by 13%. Specifically, we noticed that the shortened photoperiod treatments (natural − 2 h) could advance the time of senescence and dormancy by 46–57 days when compared with the prolonged photoperiod treatments (natural + 2 h). Furthermore, we found that the shortened photoperiod (natural − 2 h) interactive with the semi-low temperature duration (2.5 months) advanced the senescence and dormancy more significantly than the enough low temperature treatments (5 months). Thus, shortening the photoperiod (natural − 2 h) shortens the growing season by 82–86 days. These implied that the photoperiods interactive with temperature could affect the plant growth period significantly.
Changes in Biomass Over Different Phenological Development Stages
The biomass accumulation process of the whole plant, including roots, leaves, stems, flowers, and fruits, is very similar in American ginseng from the two cultivated locations (including the two imitating locations in the growth cabinets) although their growth duration is different due to different climates. As shown in Figure 5, the plant biomass accumulation process presents variable speed in different parts of American ginseng during different growth stages. For the whole plant, the growth was slower from the initiation stage to the vegetative stage (late May in Wendeng while mid-June in Wuchang), then accelerated from the vegetative stage to the full fruiting stage (late-September in Wendeng while mid-September in Wuchang), and finally slowed down to a halt from the fruiting stage to senescence (late October in Wendeng while mid-October in Wuchang). For the roots, the dry weight actually decreased a little from the initiation stage to the vegetative stage and then increased significantly till the senescence stage. For leaves and stems, both presented a continuous increase in dry weight from the initiation stage to the early fruiting stage and then tended to be stable and decreasing. For flowers and fruits, both presented an increase in dry weight only during the flowering and fruiting stages.
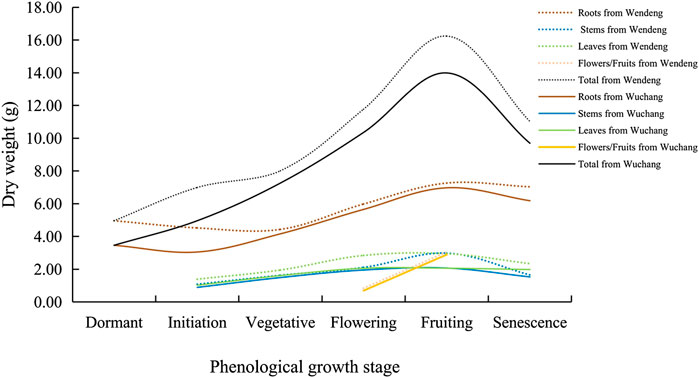
FIGURE 5. Biomass accumulation dynamics at different phenological stages of American ginseng harvested from Wendeng and Wuchang.
The biomass allocation between plants aboveground and underground is also very similar in American ginseng from the two cultivated locations (including the two imitating locations in the growth cabinets). Usually, the aboveground dry weight of American ginseng fluctuates in the range of 25–35%, while the underground dry weight fluctuates in the range of about 65–75%, and the allocation between aboveground and underground changes regularly with different growth stages. From the initiation stage to the vegetative stage, the aboveground dry weight might increase to about 35%, while the underground dry weight might decrease to about 65%, which is due to underground root nutrition supporting the aboveground growth. From the vegetative stage to the fruiting stage, weight gain occurred rapidly in both aboveground and underground parts; while the aboveground dry weight might decrease to about 25%, the underground dry weight might increase to about 75%, which is due to the transport of a large quantity of photosynthate underground. After that, from the late fruit stage to the senescence stage, the aboveground dry weight decreased significantly, while the underground dry weight still increased to a certain extent.
However, we found that the final root yields of American ginseng were significantly different between the two cultivated locations (including the two imitating locations in the growth cabinets): the root yield of American ginseng in Wendeng is almost always greater than that of Wuchang. This probably results from the different growth durations of American ginseng in the two cultivated locations: the growth duration of American ginseng in Wendeng is about 190 days, while that in Wuchang is about 140 days.
Changes in Ginsenosides Over Different Phenological Development Stages
The change patterns in the contents and yields of total ginsenosides (Rg1 + Re + Rb1) over the phenological development stage are very similar in American ginseng from the two cultivated locations (including the two imitating locations in the growth cabinets). As shown in Figure 6, results showed that the contents of ginsenosides in roots presented a trend of decreasing at first and then increasing in the phenological development stage, during which they reached at a minimum during the vegetative stage and a maximum during the fruiting stage (red fruits on the plants). However, when comparing the two cultivated locations (Wendeng and Wuchang), the contents of ginsenosides in Wuchang were found to be significantly higher than that of Wendeng.
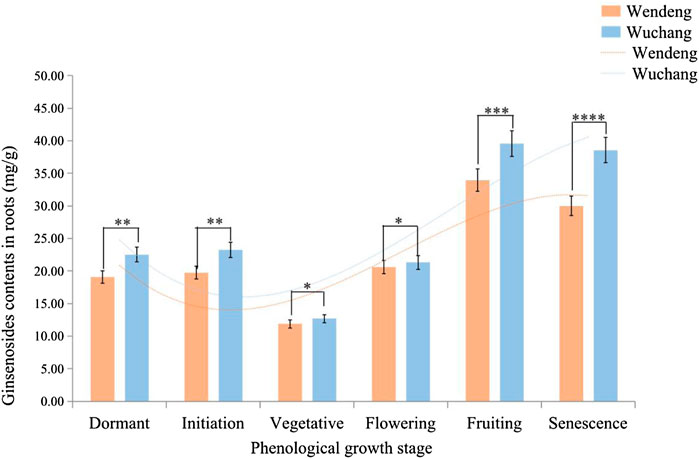
FIGURE 6. Ginsenoside contents in roots at different phenological stages of American ginseng harvested from Wendeng and Wuchang. Note: The results are expressed as mean value ±SD (n = 3). *p < 0.05, **p < 0.01, ***p < 0.001, ****p < 0.0001).
We used stepwise multiple linear regression (SMLR) analysis to establish a regression equation of the ginsenoside contents against the important environmental impact factor as follows:
The 6 main climatic factors included (x1, AATgrowth) active accumulated temperature during plant growth season, (x2, AATdormancy) active accumulated temperature during plant dormancy season, (x3, ASDgrowth) accumulated sunshine duration during plant growth season, (x4, ASDdormancy) accumulated sunshine duration during plant dormancy season, (x5, Pgrowth) precipitation during plant growth season, and (x6, Pdormancy) precipitation during plant dormancy season. Climate factors were averaged over 2 years from 2018 to 2019. The standardized regression coefficient reveals the significance of an individual factor in the regression equation. A high absolute value of this coefficient corresponds to a high weight of variables in the equation. The ginsenoside contents showed a negative linear correlation with AATdormancy, AATgrowth, Pdormancy, Pgrowth, and ASDdormancy, but only exhibited a positive linear correlation with ASDgrowth. The absolute values of the standardized regression coefficients were ranked as follows: AATdormancy > ASDgrowth > Pgrowth > AATgrowth > ASDdormancy > Pdormancy. AATdormancy had the strongest negative influence, while ASDgrowth had the strongest positive influence, followed by Pgrowth which had a significant negative influence on the ginsenoside contents. The influence of AATgrowth, ASDdormancy, and Pdormancy was weaker than the influence of AATdormancy, ASDgrowth, and Pgrowth. Therefore, ginsenoside contents can be considered to be mainly influenced by AATdormancy, ASDgrowth , and Pgrowth.
Expression of Key Enzyme Genes at Different Phenological Development Stages of American Ginseng
The expression levels of PqSE, PqDS, and PqD12H genes in American ginseng leaves at different phenological development stages were detected by RT-PCR. As shown in Figures 7A,B,C, the expression levels of PqSE, PqDS, and PqD12H genes had a consistent trend in the leaves of American ginseng from the two cultivated locations (including the two imitating locations in the growth cabinets). The expression levels of all three genes changed significantly with the change in phenological development stages. All the three genes are highly expressed during the fruiting stage, which is an important period of ginsenoside accumulation. However, when comparing the two cultivated locations, the expression levels of PqSE, PqDS, and PqD12H genes in American ginseng leaves also were found to present significant differences. The expression levels of PqSE, PqDS, and PqD12H genes during the fruiting stage from Wuchang were about 1.1, 1.2, and 1.2 folds higher than those from Wendeng. This indicated that the gene expression levels of ginsenoside biosynthesis in leaves of American ginseng during the same phenological stage were significantly higher in Wuchang than Wendeng.
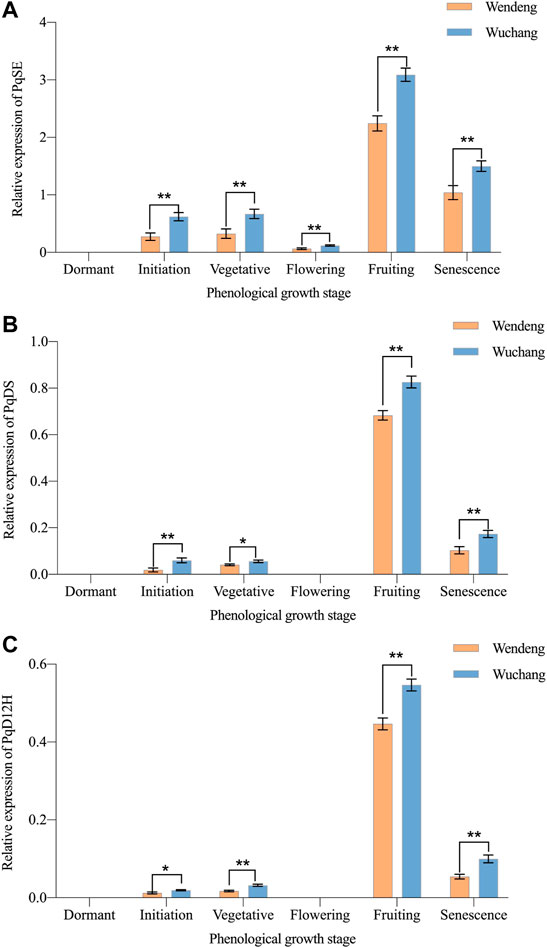
FIGURE 7. Genes expression dynamics of ginsenoside biosynthesis at different phenological stages of American ginseng harvested from Wendeng and Wuchang. Note: PqSE, the gene of squalene epoxidase in P. quinquefolium (A); PqDS, the gene of dammarenediol synthase in P. quinquefolium (B); PqD12H, the gene of protopanaxadiol synthase in P. quinquefolium (C). The results are expressed as mean value ±SD (n = 3) (*p < 0.05, ** p < 0.01).
The Pearson correlation analysis (Table 6) showed that 1) the expression level of PqSE, PqDS and PqD12H genes during fruiting stage correlated positively and significantly with the ginsenosides contents in finally harvested roots (p < 0.01); and 3) the ginsenoside contents in finally harvested roots also correlated positively significantly with ASDgrowth and negatively significantly with AATdormancy and Pgrowth (p < 0.05).
Effects of Photoperiod and Temperature on Biomass, Ginsenoside Accumulation, and Gene Expression
In the cabinet experiments (Tables 4, 5), we found that, under the same photoperiod conditions, American ginseng after enough low-temperature treatments (5 months) presented larger dry weights of the stem, leaf, and root at the harvest stage, higher ginsenoside contents in roots, and higher expression level of PqSE, PqDS, and PqD12H in leaves, when compared with the semi-low temperature duration treatments (2.5 months). Furthermore, without experiencing low-temperature treatment in winter, American ginseng only sprouted sporadically and could not blossom, and the plants were stunted and died early regardless of the photoperiod conditions. Obviously, the low-temperature experience of American ginseng in winter is a necessary condition for its growth and development and ginsenoside accumulation. Results also showed that after enough low-temperature treatments (5 months), the prolonged photoperiod (natural + 2 h) treatments could further increase the dry weights of the stem, leaf, and root at the harvest stage; the ginsenoside contents in roots; and the gene expression level of PqSE, PqDS, and PqD12H in leaves, when compared with the shortened photoperiod (natural − 2 h) treatments. These implied that interactive effects of temperature and photoperiod play an important role in regulating the plant development and ginsenoside accumulation of American ginseng.
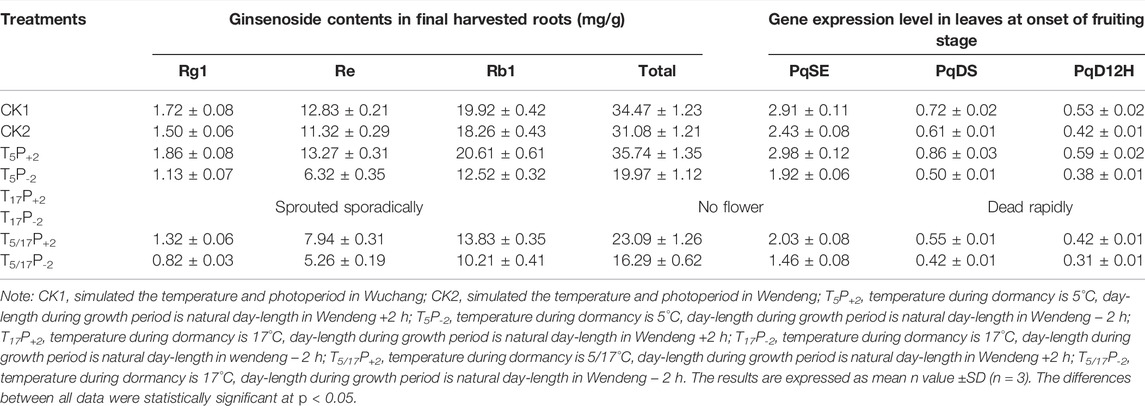
TABLE 5. Effects of temperature and photoperiod on ginsenoside contents and related gene expression level of American ginseng.
Discussion
Different Phenological Performance due to Different Environmental Factors
Plant phenology is the study of the timing of annually recurring plant growth and reproductive phenomena, and the drivers of these events associated with endogenous and exogenous factors (Yang et al., 2016; Chen et al., 2019; Stucky et al., 2018; Liu et al., 2021). Perennial plants can best reflect the fluctuation rhythm of phenology as they transition between growth and dormancy in response to seasonal changes, of which initiation from dormant state, initial flowering dates, and re-entering dormant state are the most critical stages. In this study, American ginseng, as a perennial plant that originated in the cold regions of North America, presented significantly different phenological performance when growing in different cultivated locations in China. Comparing two cultivated locations in China (Wendeng and Wuchang), American ginseng in Wendeng showed early initiation, early flowering, and late dormancy and thus can grow for a longer period, while American ginseng in Wuchang showed late initiation, late flowering, and early dormancy and thus can grow for a shorter period (Figures 4A,B).
Many exogenous factors can change the plant phenological performance, such as temperature, sunshine, precipitation, and soil nutrients (Andresen et al., 2018; Shen et al., 2018; Ren et al., 2022). In the field experiments, the exogenous factors influencing American ginseng phenology can be clustered into four principal components (PC1–4) by PCA, of which two environmental factors (temperature and photoperiod) were clearly distinguished (Table 2). Furthermore, Pearson correlation analysis indicated that the plant phenological performance (especially the initial date of sprouting, flowering, and dormancy) of American ginseng is closely related to the change in temperature and photoperiod (Table 3), In the cabinet experiments, results further proved that temperature along with photoperiod plays a decisive role in the phenological development of American ginseng in the absence of water and nutrient limitation (Table 4). Both field and cabinet experiments confirmed that temperature is more related to the initial date of sprouting in spring, while photoperiod is more related to the initial date of dormancy in American ginseng (Tables 3, 4). Some studies have shown that temperature is a main trigger for initiation, while photoperiod is a main trigger for dormancy in some plants (Wang et al., 2015; Way and Montgomery, 2015; Kiss et al., 2017; Fu et al., 2020). Multiple studies have shown that many dormant plants do require a period of cold temperature before active growth is resumed in response to warming spring temperatures (Hao et al., 2020; Im et al., 2020; Wang et al., 2020). Other studies have shown that plant growth cessation is generally induced by changes in photoperiod or associated changes in the light spectrum (Ford et al., 2017). Both photoperiod and temperature are two pivotal regulatory factors of plant flowering (Yang et al., 2016; Fu et al., 2019; Im et al., 2020; Wang et al., 2020). In the present study, they are closely related to the initial flowering period of American ginseng. Therefore, we believe that temperature and photoperiod play an important role in the phenological performance of American ginseng.
Fu et al. (2020) proposed a theoretical framework for the co-regulation of plant phenology by thermoperiod–photoperiod interactions. Temperature is widely accepted as the dominant controlling factor of plant phenology. Temperature affects the timing of plant phenology in temperate and boreal zones involving two main aspects: winter chilling and spring warming. Spring warming accelerates the spring onset, while insufficient chilling in mild winters delays the spring onset. Therefore, the response of phenological events to temperature changes is nonlinear, and the interaction effect between chilling and warming should be considered. Photoperiod, as another important cue that controls plant phenology, indirectly affects the plant phenology by altering temperature sensitivity. Studies have shown that cold in winter also influenced the photoperiod sensitivity, but the cold treatment did not completely replace long-day photoperiod (Im et al., 2020). In addition, the mechanism of photoperiod primary control of autumn phenology is widely accepted (Way and Montgomery, 2015; Fu et al., 2020). A continually declining day-length during autumn is an essential environmental cue inducing growth cessation and dormancy. Our experiments indicated that temperature was more related to spring phenology while photoperiod was more related to autumn phenology in American ginseng in both field and cabinet experiments (Tables 3, 4). This is consistent with Fu’s viewpoints.
Changes in Biomass and Ginsenoside Accumulation Along With Different Phenological Performance
There were clear differences in the growing dynamics (Figure 5) and the ginsenoside accumulation dynamics (Figure 6) related to the phenological stages of American ginseng from different cultivated locations. Although there is great similarity in the plant biomass accumulation process of the whole plant and in the plant biomass allocation between the plant aboveground and underground, we found that the final harvested root dry weight of American ginseng was significantly different between the different cultivated locations, which is probably due to the different growth durations or growth speeds of American ginseng in different cultivated locations. Meanwhile, the ginsenoside accumulation dynamics and the phenological development stage are very similar in American ginseng from different cultivated locations (Wendeng and Wuchang), but the final ginsenoside contents of whole American ginseng roots collected from Wuchang were significantly higher than those from Wendeng. Our results suggested that different phenological performance must be accompanied by differences in the ginsenoside accumulation in American ginseng.
Key Environmental Factors Influencing Ginsenoside Accumulation of American Ginseng
In our study, ginsenoside accumulation varied between the different cultivated locations (Wendeng and Wuchang) during the 2 years of study. This variation must be caused by the difference in environmental factors in different cultivated locations. Results of the multiple regression analysis showed that the ginsenoside contents negatively significantly correlated with AATdormancy and positively significantly correlated with ASDgrowth, and Pgrowth had a negative weak influence on the ginsenoside contents. Therefore, we believe that temperature and photoperiod, which play a decisive role in the phenological stages, also play a very important role in ginsenoside accumulation.
This study further confirmed that the key genes in ginsenoside biosynthesis (PqSE, PqDS, and PqD12H) are expressed in high levels during the fruiting stage of American ginseng from all cultivated locations (Figures 7A,B,C). In field experiments, Pearson correlation analysis indicated the higher expression levels of key genes during the fruit stage, the higher ginsenoside contents of American ginseng, and that the expression levels of key genes are also negatively correlated with AATdormancy, positively correlated with ASDgrowth, and weakly negatively correlated with Pgrowth (Table 6). In the cabinet experiments, results further proved that temperature along with photoperiod plays a decisive role in the ginsenoside accumulation of American ginseng in the absence of water and nutrient limitation (Table 5). We thus deduced that the interactive effects of temperature and photoperiod play decisive roles in ginsenoside accumulation, as they determined the level of ginsenoside gene expression of American ginseng during the key stage of ginsenoside biosynthesis. Although other factors, especially water and soil nutrients, might influence the ginsenoside accumulation significantly, we think they are not the decisive factors, as they do not play a key role in the plant phenological development of American ginseng.
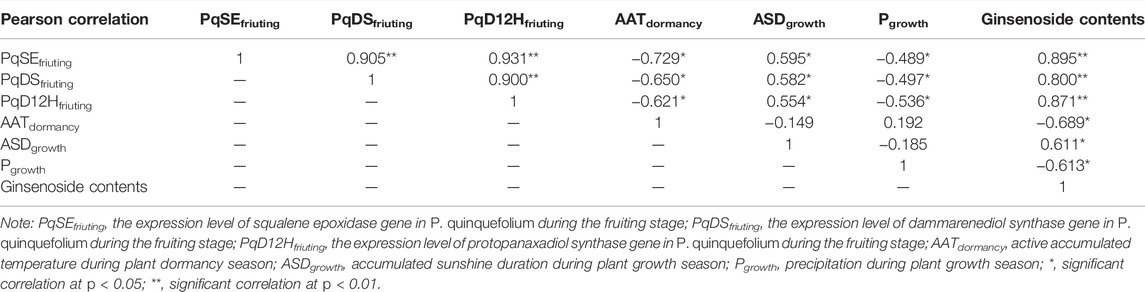
TABLE 6. Pearson correlation analysis of the gene expression level, the key phenological factors, and the ginsenoside contents.
The environmental factors influencing plant phenology are diversity and complexity. In this study, we focused on the effects of temperature and photoperiod on the phenology of American ginseng. However, the interactive effect of temperature and photoperiod on the phenology is a nonlinear relationship, and this limits our interpretation of the phenological change mechanism of American ginseng. In addition, the effects of other environmental factors (such as rainfall and soil nutrients) on the phenology of American ginseng need to be further studied. In future, the research focus on plant phenology should gradually transition from field observation and statistical analysis to revealing the molecular regulation mechanism of environment-induced phenological change, and the research methods should shift from the phenological phenomenon description responding to environmental changes to the comprehensive analysis of multi-scale and multi-factor coupling relationship.
Conclusion
Plants adapt to climate change through various mechanisms, including phenological changes. The challenge for introduction of medicinal plants is to understand how plants respond and adapt to the different environmental factors in a new cultivated location through modulation of the plant phenological stages. We conducted field and cabinet experiments to provide a demonstration that the phenological development rhythm in American ginseng is primarily driven by temperature and photoperiod or their interactions, followed by other factors such as soil moisture and soil nutrient availability. This study is an attempt to disclose the decisive exogenous factors that can affect plant phenological development with the aim of elucidating the underlying mechanisms of quality formation in American ginseng. Our results provide unique evidence supporting the regulation of quality formation by exogenous factors through a phenological development process that can be used to improve the predictions of new cultivated locations for American ginseng. Future studies that determine the molecular mechanism of how external environmental factors regulate phenological development may generate a deeper understanding of quality mechanisms in American ginseng.
Data Availability Statement
The original contributions presented in the study are included in the article/supplementary material. Further inquiries can be directed to the corresponding author.
Author Contributions
Conceptualization: TC and SZ; methodology: TC, LW, and SZ; resources: SJ; data curation: TC and SZ; writing—original draft preparation: TC and LW; writing—review and editing: SZ; supervision: HW; funding acquisition: SZ. All authors have read and agreed to the published version of the manuscript.
Funding
This research was funded by Traditional Chinese medicine research project (Shandong Province, China) under grant number 2020Z23.
Conflict of Interest
Author SJ was employed by the company Shandong Wendeng Jizhen Ginseng Industry Co.Ltd.
The remaining authors declare that the research was conducted in the absence of any commercial or financial relationships that could be construed as a potential conflict of interest.
Publisher’s Note
All claims expressed in this article are solely those of the authors and do not necessarily represent those of their affiliated organizations, or those of the publisher, the editors, and the reviewers. Any product that may be evaluated in this article, or claim that may be made by its manufacturer, is not guaranteed or endorsed by the publisher.
Acknowledgments
The authors would like to acknowledge the phenological observation, the sample collection, and the experimental field offering by Wenshui Wang in Wendeng, Jingliu Guo in Wuchang, Yinyuan Liu in Liuba, Jing Zhang in Huairou, Hong Yang in Tonghua, Ming Zhou in Ningan, and Yanhua Zhang in Changbai.
References
Adams, S. R., and Langton, F. A. (2005). Photoperiod and Plant Growth: a Review. J. Hortic. Sci. Biotechnol. 80, 2–10. doi:10.1080/14620316.2005.11511882
Andresen, C. G., Tweedie, C. E., and Lougheed, V. L. (2018). Climate and Nutrient Effects on Arctic Wetland Plant Phenology Observed from Phenocams. Remote Sens Environ. 205, 46–55. doi:10.1016/j.rse.2017.11.013
Bian, S., Zhao, Y., Li, F., Lu, S., He, Z., Wang, S., et al. (2021). Total Ginsenosides Induce Autophagic Cell Death in Cervical Cancer Cells Accompanied by Downregulation of Bone Marrow Stromal Antigen-2. Exp. Ther. Med. 22 (1), 667. doi:10.3892/etm.2021.10099
Chen, W., Balan, P., and Popovich, D. G. (2019). Comparison of the Ginsenoside Composition of Asian Ginseng (Panax Ginseng) and American Ginseng (Panax Quinquefolius L.) and Their Transformation Pathways. Stud. Nat. Prod. Chem. 63, 161–195. doi:10.1016/B978-0-12-817901-7.00006-X
Ford, K. R., Harrington, C. A., and St. Clair, J. B. (2017). Photoperiod Cues and Patterns of Genetic Variation Limit Phenological Responses to Climate Change in Warm Parts of Species' Range: Modeling Diameter-Growth Cessation in Coast Douglas-fir. Glob. Change Biol. 23 (8), 3348–3362. doi:10.1111/gcb.13690
Fu, Y. H., Piao, S., Zhou, X., Geng, X., Hao, F., Vitasse, Y., et al. (2019). Short Photoperiod Reduces the Temperature Sensitivity of Leaf-Out in Saplings of Fagus Sylvatica but Not in Horse Chestnut. Glob. Chang Biol. 25, 1696–1703. doi:10.1111/gcb.14599
Fu, Y., Li, X., Zhou, X., Geng, X., Guo, Y., and Zhang, Y. (2020). Progress in Plant Phenology Modeling under Global Climate Change. Sci. China Earth Sci. 63, 1237–1247. doi:10.1007/s11430-019-9622-2
Hao, M., Zhou, Y., Zhou, J., Zhang, M., Yan, K., Jiang, S., et al. (2020). Cold-induced Ginsenosides Accumulation Is Associated with the Alteration in DNA Methylation and Relative Gene Expression in Perennial American Ginseng (Panax Quinquefolius L.) along with its Plant Growth and Development Process. J. Ginseng Res. 44 (5), 747–755. doi:10.1016/j.jgr.2019.06.006
Huang, L. F., Suo, F. M., Song, J. Y., Wen, M. J., Jia, G. L., Xie, C. X., et al. (2013). Quality Variation and Ecotype Division of Panax Quinquefolium in China. Yao Xue Xue Bao 48 (4), 580–589. Chinese with English abstract. doi:10.16438/j.0513-4870.2013.04.004
Huang, L. F., Fu, J., and Chen, S. L. (2012). Academic Discussion on Ecological Variation of Chinese Medicinal Materials. Chin. Tradit. Herb. Drugs 43 (07), 1249–1258. Chinese with English abstract. doi:10.7501/j.issn.0253-2670
Huang, L., Ren, C., Li, H.-J., and Wu, Y.-C. (2021). Recent Progress on Processing Technologies, Chemical Components, and Bioactivities of Chinese Red Ginseng, American Red Ginseng, and Korean Red Ginseng. Food Bioproc. Technol 15 (1), 47–71. doi:10.1007/s11947-021-02697-w
Im, N. H., Lim, S. H., Lee, H. B., An, S. K., Lee, S. Y., and Kim, K. S. (2020). Growth and Flowering Responses of Lysimachia Mauritiana Lam. To Cold Treatment and Photoperiod. Scientia Horticulturae 270, 109429. doi:10.1016/j.scienta.2020.109429
Kim, Y. S., Park, C. S., Lee, D. Y., Lee, J. S., Lee, S. H., In, J. G., et al. (2021). Phenological Growth Stages of Korean Ginseng (Panax Ginseng) According to the Extended BBCH Scale. J. Ginseng. Res. 45 (4), 527–534. doi:10.1016/j.jgr.2020.12.006
Kiss, T., Dixon, L. E., Soltész, A., Bányai, J., Mayer, M., Balla, K., et al. (2017). Effects of Ambient Temperature in Association with Photoperiod on Phenology and on the Expressions of Major Plant Developmental Genes in Wheat (Triticum aestivum L.). Plant Cell Environ. 40 (8), 1629–1642. doi:10.1111/pce.12971
Li, L., Li, X., Liu, Y., and Liu, H. (2016). Flowering Responses to Light and Temperature. Sci. China Life Sci. 59 (4), 403–408. doi:10.1007/s11427-015-4910-8
Li, T. S. C. (1995). Asian and American Ginseng—A Review. Horttechnology 5 (1), 27–34. doi:10.21273/HORTTECH.5.1.27
Li, Y., Kong, D., Fu, Y., Sussman, M. R., and Wu, H. (2020). The Effect of Developmental and Environmental Factors on Secondary Metabolites in Medicinal Plants. Plant Physiol. Biochem. 148, 80–89. doi:10.1016/j.plaphy.2020.01.006
Liao, D., Jia, C., Sun, P., Qi, J., and Li, X. e. (2019). Quality Evaluation of Panax Quinquefolium from Different Cultivation Regions Based on Their Ginsenoside Content and Radioprotective Effects on Irradiated Mice. Sci. Rep. 9, 1079. doi:10.1038/s41598-018-37959-9
Liu, Y., Li, G., Wu, X., Niklas, K. J., Yang, Z., and Sun, S. (2021). Linkage between Species Traits and Plant Phenology in an alpine Meadow. Oecologia 195, 409–419. doi:10.1007/s00442-020-04846-y
Mishra, T. (2016). Climate Change and Production of Secondary Metabolites in Medicinal Plants: A Review. Int. J. Heb. Med. 4 (4), 27–30.
Padilla-González, G. F., Frey, M., Gómez-Zeledón, J., Costa, F. B. D., and Spring, O. (2019). Metabolomic and Gene Expression Approaches Reveal the Developmental and Environmental Regulation of the Secondary Metabolism of Yacón (Smallanthus Sonchifolius, Asteraceae). Sci. Rep. 9 (1), 13178. doi:10.1038/s41598-019-49246-2
Pant, P., Pandey, S., and Dall'Acqua, S. (2021). The Influence of Environmental Conditions on Secondary Metabolites in Medicinal Plants: A Literature Review. Chem. Biodivers. 18 (11), e2100345. doi:10.1002/cbdv.202100345
Pritts, K. D. (1995). Ginseng: How to Find, Grow, and Use North America's Forest Gold. Mechanicsburg, PA: Stackpole Books.
Proctor, J. T. A., Dorais, M., Bleiholder, H., Willis, A., Hack, H., and Meier, V. (2003). Phenological Growth Stages of North American Ginseng (Panax Quinquefolius). Ann. Appl. Biol. 143 (3), 311–317. doi:10.1111/j.1744-7348.2003.tb00299.x
Qi, L.-W., Wang, C.-Z., and Yuan, C.-S. (2011). Ginsenosides from American Ginseng: Chemical and Pharmacological Diversity. Phytochemistry 72, 689–699. doi:10.1016/j.phytochem.2011.02.012
Ren, S., Vitasse, Y., Chen, X., Peichl, M., and An, S. (2022). Assessing the Relative Importance of sunshine, Temperature, Precipitation, and spring Phenology in Regulating Leaf Senescence Timing of Herbaceous Species in China. Agric. Meteorol. 313, 108770. doi:10.1016/J.AGRFORMET.2021.108770
Sheban, C. K., Woodbury, J. D., and Duguid, C. M. (2021). Importance of Environmental Factors on Plantings of Wild-Simulated American GinsengAgroforestry Syst. 96, 1–14. doi:10.1007/S10457-021-00705-8
Shen, L., Meng, X. X., Huang, L. F., Xie, C. X., Xu, J., Tang, H., et al. (2017). Research Strategy on Global Eco-Suitability Regionalization for Medicinal Plants. WJTCM 12 (05), 961–968+973. Chinese with English abstract. doi:10.3969/j.issn.1673-7202.2017.05.001
Shen, X., Liu, B., Henderson, M., Wang, L., Wu, Z., Wu, H., et al. (2018). Asymmetric Effects of Daytime and Nighttime Warming on spring Phenology in the Temperate Grasslands of China. Agric. Meteorol. 259, 240–249. doi:10.1016/j.agrformet.2018.05.006
Stucky, B. J., Guralnick, R., Deck, J., Denny, E. G., Bolmgren, K., and Walls, R. (2018). The Plant Phenology Ontology: A New Informatics Resource for Large-Scale Integration of Plant Phenology Data. Front. Plant Sci. 9, 517. doi:10.3389/fpls.2018.00517
Sun, X., Qian, Q. Y., Zheng, S. H., Chen, H. M., and Huang, L. F. (2019). Quality Ecotype of Panax Quinquefolium L. Based on Heredity-Chemistry-Ecology Characteristics. Acta Pharmacol. Sin. 54 (9), 1695–1705. doi:10.16438/j.0513-4870.2019-0394
Wan, M. W., and Liu, X. Z. (1979). Phenological Monitoring Methodology in China. Beijing: Science Press.
Wang, H., Ge, Q., Rutishauser, T., Dai, Y., and Dai, J. (2015). Parameterization of Temperature Sensitivity of spring Phenology and its Application in Explaining Diverse Phenological Responses to Temperature Change. Sci. Rep. 5, 8833. doi:10.1038/srep08833
Wang, H., Wang, H., Ge, Q., and Dai, J. (2020). The Interactive Effects of Chilling, Photoperiod, and Forcing Temperature on Flowering Phenology of Temperate Woody Plants. Front. Plant Sci. 11, 443. doi:10.3389/fpls.2020.00443
Wang, L., Zhao, S.-J., Cao, H.-J., and Sun, Y. (2014). The Isolation and Characterization of Dammarenediol Synthase Gene from Panax Quinquefolius and its Heterologous Co-expression with Cytochrome P450 Gene PqD12H in Yeast. Funct. Integr. Genomics. 14 (3), 545–557. doi:10.1007/s10142-014-0384-1
Wang, S., Liang, W., Yao, L., Wang, J., and Gao, W. (2019). Effect of Temperature on Morphology, Ginsenosides Biosynthesis, Functional Genes, and Transcriptional Factors Expression in Panax Ginseng Adventitious Roots. J. Food Biochem. 43 (4), e12794. doi:10.1111/jfbc.12794
Way, D. A., and Montgomery, R. A. (2015). Photoperiod Constraints on Tree Phenology, Performance and Migration in a Warming World. Plant Cell Environ 38, 1725–1736. doi:10.1111/pce.12431
Weikai, Y., and Wallace, D. H. (1998). Simulation and Prediction of Plant Phenology for Five Crops Based on Photoperiod×temperature Interaction. Ann. Bot. 81 (6), 705–716. doi:10.1006/anbo.1998.0625
Wu, X., Cheng, C., Qiao, C., and Song, C. (2020). Spatio-temporal Differentiation of spring Phenology in China Driven by Temperatures and Photoperiod from 1979 to 2018. Sci. China Earth Sci. 63 (10), 1485–1498. doi:10.1007/s11430-019-9577-5
Yang, L. L., Zhang, T., Yang, L. M., and Han, M. (2017). Effects of Ecological Factors on Ginsenosides Synthesis and its Key Enzyme Genes Expression. Chin. Tradit. Herb. Drugs 48 (20), 4296–4305. doi:10.7501/j.issn.0253-2670.2017.20.026
Yang, Y., Luo, X., Rong-Ping, L. I., Wang, Y., Wen, R. H., Yang, G. J., et al. (2016). A Review of the Effect of Meteorological Factors on Plant Phenology and its Driving Mechanisms. J. Meteorol. Envir. 32 (5), 154–159. doi:10.3969/j.issn.1673-503X.2016.05.021
Keywords: American ginseng, temperature, photoperiod, phenology, ginsenoside accumulation
Citation: Chen T, Wang L, Wang H, Jiang S and Zhou S (2022) Photoperiod and Temperature as Dominant Environmental Drivers Triggering Plant Phenological Development of American Ginseng Along With Its Quality Formation. Front. Earth Sci. 10:894251. doi: 10.3389/feart.2022.894251
Received: 11 March 2022; Accepted: 11 April 2022;
Published: 06 June 2022.
Edited by:
Xiangjin Shen, Northeast Institute of Geography and Agroecology, (CAS), ChinaReviewed by:
Dengpan Xiao, Hebei Academy of Sciences, ChinaJiaqi Zhang, Northeast Institute of Geography and Agroecology, (CAS), China
Copyright © 2022 Chen, Wang, Wang, Jiang and Zhou. This is an open-access article distributed under the terms of the Creative Commons Attribution License (CC BY). The use, distribution or reproduction in other forums is permitted, provided the original author(s) and the copyright owner(s) are credited and that the original publication in this journal is cited, in accordance with accepted academic practice. No use, distribution or reproduction is permitted which does not comply with these terms.
*Correspondence: San Zhou, emhvdXNhbjNAMTYzLmNvbQ==