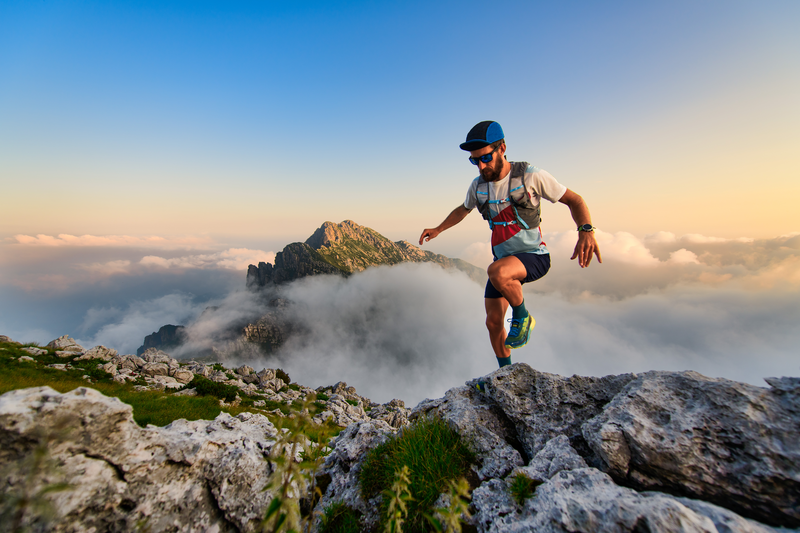
95% of researchers rate our articles as excellent or good
Learn more about the work of our research integrity team to safeguard the quality of each article we publish.
Find out more
ORIGINAL RESEARCH article
Front. Earth Sci. , 02 June 2022
Sec. Geohazards and Georisks
Volume 10 - 2022 | https://doi.org/10.3389/feart.2022.894033
This article is part of the Research Topic Interaction between Human Activities and Geo-environment for Sustainable Development View all 27 articles
Brackish groundwater was widely used in arid areas which may cause soil salinization and groundwater environmental declines. To ensure the sustainable development of agriculture in arid areas, brackish water intermittent infiltration experiments were conducted in Southern Xinjiang, Northwest China between June to September 2018, and Hydrus-2D numerical simulation was used to analyze the underground pipe drainage systems. The field experiments were carried out during cotton growth stages after the first freshwater flood infiltration and salt washing. Two control experiments were, respectively, designed as freshwater (0.68 g/L) and brackish water (1.66 g/L) with water amount of 1.0 Q = 572 mm. Other eight groups (1.05–1.40 Q) were compared to analyze the effect of soil salt leaching by increasing the brackish water amount. The results showed that the soil moisture content was almost less than the field capacity of 0.203 at depth of 0–60 cm before each infiltration due to roots water uptake, and the soil water holding capacity rate was lower than 0.2 after 5 days under 1.20–1.40 Q brackish water treatments. Variation of EC1:5 at depth of 0–30 cm was less than 0.5 dS/m. Salt mainly accumulated at the depth of 40–60 cm whether the water amount was excessive or insufficient even under the fresh water infiltration. The optimal brackish water amount was 1.15 Q = 657.8 mm, and the soil total salinity was less than 0.55 dS/m (EC1:5) and reached mild salinization degree. Numerical simulations were used based on the 2018 field experimental results and extended by another 10 years. The soil salt accumulated to 1.10–2.99 dS/m (EC1:5) at the depth of 40–60 cm during 0–30 days. The no salinization area expanded to depth of 40–45 cm after 120 days and reduced during non-infiltration period due to evaporation effect. The soil salt was gradually leached and less than 0.55 dS/m (EC1:5) after 10 years. The optimized leaching–drainage system could not only provide a low salinity soil condition for cotton growth and realize sustainable cultivation but also greatly protect the soil and groundwater environment.
Due to rapid population growth and significant industrial development, freshwater scarcity has been an urgent problem to be addressed around the world (Zhang et al., 2021a; Zhang et al., 2021b; Li et al., 2021). Brackish groundwater as an alternative source was used to solve the shortage of fresh water and has been widely used in cotton fields in Southern Xinjiang, Northwest China (Qi et al., 2018; Hu et al., 2020). Brackish water under mulched was intermittently infiltrated into the soil to irrigate salt-tolerant crops, which could increase soil nutrients, and the total salts would not be excessively accumulated (Wang et al., 2014; Li et al., 2018; Chen et al., 2018; Wu et al., 2021; Wang Z. et al., 2022). However, long-term salt supply leads to salt accumulation in soil, which not only threatens the cotton growth but also threaten the groundwater environment (Wang H. et al., 2022; Wang J. et al., 2022; Cao et al., 2022). Therefore, it is necessary to develop an optimized leaching–drainage system to effectively improve the soil salinization, ensure the sustainable development of agriculture in arid areas, and protect the groundwater environment.
Many scholars had studied the leaching requirement, influencing factors, and calculation models of different infiltration ways and crops (Šimůnek et al., 2016; Jia et al., 2021; Feng et al., 2022; Ochege et al., 2022). Salt and fresh water rotation infiltration, deficit infiltration, and intermittent infiltration were indicated to be superior (Zeng et al., 2014; Peng et al., 2016; Chen et al., 2022). The effect of intermittent infiltration on leaching salt was better than that of flood infiltration at the depth of 10–30 cm and had little difference at the depth of 30–60 cm. Intermittent point source infiltration could leach salt below 50 cm of soil some years later (Zhang et al., 2010). The leaching fraction (LF) was small in the shallow groundwater table field and more effective near the infiltration tapes (Hanson et al., 2009). Additional brackish water also was considered to leach excess soil salt to avoid soil salinization (Li et al., 2014). So, it is necessary to scientifically determine the infiltration water amount, quality, and frequency based on the salt-tolerant of crops and leaching requirement (Maas and Hoffman 1977; Beltrán 1999; Chu et al., 2016; Min et al., 2017).
The salt leaching effect of the drainage system depended on the local groundwater table. In the shallow water table areas, brackish water infiltration may lead to soil secondary salinization, which was suggested to control the water table through drainage canals or shafts to desalinate and improve soil quality (Sun et al., 2015). Groundwater evaporation had little impact on the salt accumulation content in the vadose zone when the water table was about 3.0 m in sandy regions and 5.0 m in loam regions (Li et al., 2014). An underground pipe drainage system was more suitable for deep water table areas (Sallam 2017; Inosako et al., 2019), which could effectively improve soil salinization and increase crop yield (Heng et al., 2018; Tian et al., 2018). In our experimental field, the water table ranged from 7.0 to 8.0 m in 2018 and had 1.8 m deep drainage channels.
To optimize the intermittent infiltration regimes of the brackish water leaching–drainage system, field leaching experiments were conducted in Southern Xinjiang based on soil physical properties and salt leaching requirements. Specific objectives of the research were to 1) analyze the effect of different leaching fractions (LF) on salt accumulation and cotton yield and determined the optimal infiltration water amount; 2) calibrate the HYDRUS-2D simulations using the experimental data; and 3) use the model to calculate the effects on desalting of underground pipe drainage system for 10 years.
The leaching experiments were carried out in the Tarim Basin of southern Xinjiang, Northwest China, between June to September 2018 in the cotton field. The experimental site was located 901 m above mean sea level (Figure 1). The field belongs to continental desert climate with less rain and strong evaporation. The annual precipitation was 53.3–62.7 mm, and the annual evaporation was 2,273–2,788 mm. Compared with evaporation and crop transpiration, the supply of atmospheric rainfall was very little. The fresh water (FW) was taken from the Peacock River with an average total dissolved solids (TDS) of 0.62–0.72 g/L, and the brackish water (BW) was taken from a well located in the test field with TDS of 1.61–1.71 g/L (Table 1). The sodium adsorption ratio (SAR) of infiltration water ranged from 10 to 14 of FW and 8–9 of BW. The main soil type was loamy sand (Table 2). The electrical conductivity of soil was less than 1.0 dS/m with no salinization (Slavich and Petterson 1993; Chen et al., 2018). The field water capacity was 0.2.
The leaching experiments were conducted in 10 randomly selected field plots, each having a size of 225 m2 (Figure 2). There were set as 17 infiltration lines with the mode of “one mulch, two infiltration lines, and four rows”. In total, 2 infiltration lines were installed for every four rows of cotton. The distance between the infiltration lines was 55 cm and that between two cotton rows was 20 cm. Infiltration points along each line were spaced 30 cm apart, while the non-mulched area of bare soil was 40-cm wide. Before sowing, flood infiltration of fresh water was conducted to leach salt and preserve soil water on 20 April, with amount of 57.3 mm. Then, cotton seeds were sown and mulched with degradable plastic sheeting. Brackish water was conducted one time per 5 days, for totally 14 times. The emitter flow was 2.2 L/h. The amount of applied infiltration water during the growing stages followed traditional irrigation patterns of cotton (Table 3). In total, 2 groups of control experiments were designed for fresh water of 0.68 g/L and brackish water of 1.66 g/L with 1.0 Q = 572 mm. Other eight groups of MDI experiments leached soil salt by increasing the amount of brackish water with 1.05–1.40 Q.
The soil samples were drilled at 8:00 p.m. before each infiltration for gravimetric water content and EC1:5 analysis. The sampling interval of each sample was 10 s at a depth of 0–60 cm and 20 s at depth of 60–100 cm. The soil water content was measured by the drying method. The EC1:5 was referred to the EC of 1–5 soil/water suspensions using a conductivity meter (DDS-307, INESA Scientific Instrument Co., Ltd., Shanghai, China).
A 600 × 100 m section was to present the water–salt transport–based 2D conceptual model (Figure 3). The infiltration point was simplified as line water supply. Under the intermittent infiltration of brackish water, soil salt mainly accumulated in 40–60 cm (Chen et al., 2018; Li et al., 2018; Hu et al., 2020), and the soil was less affected by evaporation below 1.2 m (Heng et al., 2018). The pipe was theoretically suggested to install underground 1.6m, vertical to infiltration line (Figure 3).
The numerical simulation of soil water–salt transport was conducted using HYDRUS (Šimůnek et al., 2016; Katarina et al., 2019). The ground pipe was set as the free drainage boundary, the bare area was the atmospheric boundary, and the dipper was the variable flow boundary (Var.Fl1). The water quantity data of the infiltration point was assigned by Table 3. There was no flow in the Y direction of the model and under the mulch except infiltration points (Var.Fl2).
Flow boundary condition:
Lateral boundaries:
Atmospheric boundaries:
Var.Fl1:
Var.Fl2:
Solute boundary conditions:
where h is the water pressure head, cm; θ is the volumetric water content, cm3/cm3; c is the salinity of the soil water, g/L; t is time, day; x and z are the spatial coordinates, cm; K is the unsaturated hydraulic conductivity, cm/d; D was the dispersion coefficient, cm2/d; qy, qd, and qa are the water flux of lateral boundaries, infiltration points, bare soil surface, cm/day; ca was the salinity of the infiltration water, g/L.
The soil hydraulic properties were described using the standard equations of Van Genuchten (1980). The estimated hydraulic parameters were listed in Table 4. The initial longitudinal dispersion coefficient (DL) and transverse dispersion coefficient (DT) were defined by previous literature (Wang et al., 2014; Singh et al., 2019). The final diffusion coefficient was set to 1.58 cm2/day after verification and adjustment based on the experimental values (Wang et al., 2017; Ranjbar et al., 2019). The linear isotherm adsorption coefficient Kd was set as 0.28 cm3 g−1 (Kadyampakeni et al., 2018).
We simulated the spatial distributions of soil water and salt in the two-direction under mulched intermittent infiltration. Furthermore, the model values of HYDRUS-2D were compared with actual observed values (Figures 4,5). The model could display the dynamic variation of soil moisture and salinity better with infiltration events. A t-test was carried out between the measured and simulated values, and the results showed that the significance level of p > 0.05, indicating that the simulation results of the solute transport model were verified successfully (Table 5).
Soil samples were collected the day before infiltration treatment and obtained soil moisture dates, which could better reflect the water holding capacity of the soil (Figure 6). Within a single infiltration interval of 5 days, the migration distance of soil water in unsaturated zone was more than 40 cm. Due to the water uptake by plant roots, the soil moisture content was almost less than the field capacity of 0.203 at depth of 0–60 cm before each infiltration, and the water mainly existed at the depth of 60–100 cm. Compared two control experiment schemes, the soil water migrated faster under fresh water treatment and the soil reached saturation at the depth of 100 cm with moisture content of 0.35. Brackish water changed the soil structure and reduced the soil permeability because of high sodium ion content and large sodium adsorption ratio. The soil water holding capacity rate was lower than 0.2 after 5 days with 1.20Q–1.40Q brackish water treatments.
The soil salt accumulation at the depth of 0–30 cm was lower under water leaching in the whole growth period (Figure 7). The EC1:5 of soil in the root zone was basically less than 0.55 dS/m, which was no salinized (Table 6). There was massive salt accumulation at the depth of 30–60 cm. The average salinity of the 10 experimental groups was 0.539 ds/m, 0.979 dS/m and 0.684 ds/m respectively at the depth of 30–40, 40–50, 50–60 cm. The maximum salinity was accumulated at the depth of 40–50 cm. When the groundwater level was greater than the limit evaporation depth, the soil in the root zone formed a “low salt zone” at the depth of 0–30 cm. The cumulative increase of soil salt at the depth of 30–50 cm reached largest, and decreased below 50 cm.
Comparing the soil salinity of each infiltration event to the first leaching salt by fresh water (20 April) showed that the variation of EC1:5 at depth of 0–30 cm was less than 0.5 dS/m (Figure 8). The root distribution was measured and time-variable through plant growth which could be accounted for by modifying the ratio between evaporation and transpiration (Wang et al., 2014). Soil salinization mainly occurs in 30–60 cm, and the variation of EC1:5 exceed 4.0 dS/m. Root uptake as a key way to transport soil water into atmosphere contributed mainly to soil salinization. The average variation of EC1:5 showed that the soil salt little changed before and after brackish water infiltration. The brackish water intermittent infiltration under plastic mulch could effectively improve soil salinization and protect the cultivated environment.
FIGURE 8. Variation of soil EC1:5 compared to initial time after leaching salt by fresh water (20 April) at different depths.
Soil salinity was evaluated using the electric conductivity of the saturation extract as ECe, which could be calculated from measured values of EC1:5 using ECe = 3.64 EC1:5 (Slavich and Petterson 1993; Wang et al., 2014). We evaluated the salinization degree of soil based on Table 6 (Fitzpatrick 1980; Akça et al., 2020).
The maximum salinity of 10 experimental treatments at depth of 40–50 cm was 3.07, 2.46, 1.99, 0.274, 0.32, 0.38, 1.64, 1.43, 2.41, 0.31 dS/m respectively. Fresh water infiltration would still lead to soil moderate salinization. The soil salinity decreased first and then increased with increasing infiltration water amount of 1.0–1.35Q under brackish water infiltration. The total salt of soil was the lowest with water treatment of 1.15 Q = 657.8 mm. The EC1:5 of soil was less than 0.55 dS/m, reaching the degree of no salinization. Whether the amount of water was too large or too small, it would lead to salt accumulation. The leaching intensity was not enough with low water amount, resulting in the accumulation of salt in the topsoil. Numerous ions were input into the soil through large amount of water, which could not be absorbed by plants and lead to root salt stress. The optimization of soil environment achieved the best effect with 1.15 Q water treatment.
Long-term infiltration causes the salt of topsoil to penetrate into the deep soil layer and then salinize the groundwater. At present, the drainage channel with the depth of 1.8 m in the experimental field could not meet the salt discharge requirement. Therefore, underground pipe drainage system should be adopted to receive the upper leaching water and salt, collect and recycle it, desalinate soil, and protect groundwater. We simulate water–salt of soil under a leaching–drainage system for 10 years with 1.15 Q = 657.8 mm treatment (Figure 9).
Soil water gradually pushed downward under brackish water intermittent infiltration, and soil salinity is gradually concentrated to the edge of the wetting front. Under the leaching of multiple infiltration points, the wetting front overlaps with each other to make the salt migrate downward, forming a “low salination soil layer”. The salt mainly accumulated to 1.10–2.99 dS/m at the depth of 40–60 cm during 0–30 days, and the soil salinity under mulch area was lower than 0.55 dS/m at depth of 0–10 cm. After 120 days, no salinization soil area expanded to depth of 40–45 cm. During non-infiltration period (121–365 days), the soil salt moved upward under evaporation effect, and the no salinization area was reduced and showed mild salinization. Figures 7E–H showed the prediction results of 2–10 years. The salt was gradually leached and discharged through underground pipe. The soil total salt under mulch was less than 0.55 dS/m, which could not only provide a low salt area for cotton growth but also control soil salt accumulation and protect soil as well as groundwater environment.
Combined field leaching experiments under the brackish water intermittent infiltration in 2018 and the Hydrus-2D soil water transport numerical model under the leaching-drainage system, we analyzed the optimal leaching water amount and predicted the soil salinization degree after 10 years. The main conclusions of this paper are as follows.
Within a single infiltration interval of 5 days, soil water transported to below 40 cm in unsaturated zone, and soil moisture content was almost less than the field capacity of 0.203 at depth of 0–60 cm due to the root water uptake. Soil water holding capacity rate was lower than 0.2 under 1.20Q–1.40Q brackish water treatments. The field leaching experiments showed that whatever the higher or lower amount of infiltration water, the salt all accumulated at depth of 40–50 cm. We recommend that the optimum amount of brackish water for leaching was 1.15 Q = 600 mm, and the soil salinity in the soil was less than 2.0 dS/m as no salinization. The LF = 1.15 could ensure the no salinization environment of soil and realize the sustainable cultivation of cotton.
To prevent the accumulation of salt in the deep soil layer, the salt in the root zone should be drained during the intermittent infiltration period. The 10-year simulation results of the leaching–drainage system showed that salt mainly accumulated to 1.10–2.99 dS/m at the depth of 40–60 cm during 0–30 days. No salinization area expanded to depth 40–45 cm after 120 days and reduced under evaporation effect during non-infiltration period. The soil salt was gradually leached and discharged through underground pipe and was less than 0.55 dS/m after 10 years, which could provide a theoretical basis for the protecting soil and groundwater environment.
The raw data supporting the conclusions of this article will be made available by the authors, without undue reservation.
LG contributed to field experiments, testing, data analysis, and article writing. ZW contributed to fund support, field experiments, data analysis, and article polishing.
This work was supported by the National Natural Science Foundation of China [Grant No.41702259] and Doctoral research start up fund of Technological Innovation R & D project [2021SCSZYD-03].
The authors declare that the research was conducted in the absence of any commercial or financial relationships that could be construed as a potential conflict of interest.
All claims expressed in this article are solely those of the authors and do not necessarily represent those of their affiliated organizations, or those of the publisher, the editors, and the reviewers. Any product that may be evaluated in this article, or claim that may be made by its manufacturer, is not guaranteed or endorsed by the publisher.
Akça, E., Aydin, M., Kapur, S., Kume, T., Nagano, T., Watanabe, T., et al. (2020). Long-term Monitoring of Soil Salinity in a Semi-arid Environment of Turkey. Catena 193, 104614. doi:10.1016/j.catena.2020.104614
Beltrán, J. M. (1999). Irrigation with Saline Water: Benefits and Environmental Impact. Agric. Water Manage. 40 (2), 183–194.
Cao, Y., Cai, H., Sun, S., Gu, X., Mu, Q., Duan, W., et al. (2022). Effects of Drip Irrigation Methods on Yield and Water Productivity of Maize in Northwest China. Agric. Water Manag. 259, 107227. doi:10.1016/j.agwat.2021.107227
Chen, F., Cui, N., Jiang, S., Li, H., Wang, Y., Gong, D., et al. (2022). Effects of Water Deficit at Different Growth Stages under Drip Irrigation on Fruit Quality of Citrus in the Humid Areas of South China. Agric. Water Manag. 262, 107407. doi:10.1016/j.agwat.2021.107407
Chen, W., Jin, M., Ferré, T. P. A., Liu, Y., Xian, Y., Shan, T., et al. (2018). Spatial Distribution of Soil Moisture, Soil Salinity, and Root Density beneath a Cotton Field under Mulched Drip Irrigation with Brackish and Fresh Water. Field Crops Res. 215, 207–221. doi:10.1016/j.fcr.2017.10.019
Chu, L.-l., Kang, Y.-h., and Wan, S.-q. (2016). Effect of Different Water Application Intensity and Irrigation Amount Treatments of Microirrigation on Soil-Leaching Coastal Saline Soils of North China. J. Integr. Agric. 15 (9), 2123–2131. doi:10.1016/s2095-3119(15)61263-1
Feng, D., Li, G., Wang, D., Wulazibieke, M., Cai, M., Kang, J., et al. (2022). Evaluation of AquaCrop Model Performance under Mulched Drip Irrigation for Maize in Northeast China. Agric. Water Manag. 261, 107372. doi:10.1016/j.agwat.2021.107372
Hanson, B. R., May, D. E., Simünek, J., Hopmans, J. W., and Hutmacher, R. B. (2009). Drip Irrigation Provides the Salinity Control Needed for Profitable Irrigation of Tomatoes in the San Joaquin Valley. Cal. Ag. 63 (3), 131–136. doi:10.3733/ca.v063n03p131
Heng, T. H., Liao, R. K., Wang, Z. H., and Wu, W. Y. (2018). Effects of Combined Drip Irrigation and Sub-surface Pipe Drainage on Water and Salt Transport of Saline-Alkali Soil in Xinjiang, China[J]. J. Arid Land 10 (06), 932–945. doi:10.1007/s40333-018-0061-7
Hu, Y., Li, X., Jin, M., Wang, R., Chen, J., and Guo, S. (2020). Reduced Co-occurrence and Ion-specific Preferences of Soil Microbial Hub Species after Ten Years of Irrigation with Brackish Water. Soil Tillage Res. 199, 104599. doi:10.1016/j.still.2020.104599
Inosako, K., Saito, T., and Omachi, K. (2019). Analysis by Numerical Experiments of Desalinization of a Salt-Affected Paddy Field Using a Rice Husk Underdrainage System. Paddy Water Environ. 17, 211–219. doi:10.1007/s10333-019-00713-6
Jia, Q., Shi, H., Li, R., Miao, Q., Feng, Y., Wang, N., et al. (2021). Evaporation of Maize Crop under Mulch Film and Soil Covered Drip Irrigation: Field Assessment and Modelling on West Liaohe Plain, China. Agric. Water Manag. 253, 106894. doi:10.1016/j.agwat.2021.106894
Kadyampakeni, D. M., Morgan, K. T., Nkedi-Kizza, P., Schumann, A. W., and Jawitz, J. W. (2018). Modeling Water and Nutrient Movement in Sandy Soils Using HYDRUS-2D. J. Environ. Qual. 47 (6), 1546–1553. doi:10.2134/jeq2018.02.0056
Katarina, L. G., de Miranda, J. H., Silveira, L. K., and van Genuchtenbc, M. T. (2019). HYDRUS-2D Simulations of Water and Potassium Movement in Drip Irrigated Tropical Soil Container Cultivated with Sugarcane. Agric. Water Manag. 221, 334–347. doi:10.1016/j.agwat.2019.05.010
Li, S., Luo, W., Jia, Z. H., and Pan, Y. X. (2014). Impact of Water Table Depth and Shallow Groundwater Use on Salt Leaching Cycle in Irrigated Areas. J. Hydraulic Eng. 45 (8), 950–957. (in Chinese). doi:10.13243/j.cnki.slxb.2014.08.008
Li, X., Huang, X., and Zhang, Y. (2021). Spatio-temporal Analysis of Groundwater Chemistry, Quality and Potential Human Health Risks in the Pinggu Basin of North China Plain: Evidence from High-Resolution Monitoring Dataset of 2015-2017. Sci. Total Environ. 800, 149568. doi:10.1016/j.scitotenv.2021.149568
Li, X., Jin, M., Zhou, N., Jiang, S., and Hu, Y. (2018). Inter-dripper Variation of Soil Water and Salt in a Mulched Drip Irrigated Cotton Field: Advantages of 3-D Modelling. Soil Tillage Res. 184, 186–194. doi:10.1016/j.still.2018.07.016
Maas, E. V., and Hoffman, G. J. (1977). Crop Salt Tolerance-Current Assessment. J. Irrig. Drain. Div. 103 (2), 115–134. doi:10.1061/jrcea4.0001137
Min, W., Guo, H. J., and Hu, Z. Q. (2017). Cotton Growth and the Fate of N Fertilizer as Affected by Saline Water Irrigation and N Fertigation in a Drip-Irrigated Field. Acta Agric. Scand. Sect. B-Soil Plant Sci. 67 (8), 712–722. doi:10.1080/09064710.2017.1338309
Ochege, F. U., Luo, G., Yuan, X., Owusu, G., Li, C., and Justine, F. M. (2022). Simulated Effects of Plastic Film-Mulched Soil on Surface Energy Fluxes Based on Optimized TSEB Model in a Drip-Irrigated Cotton Field. Agric. Water Manag. 262, 107394. doi:10.1016/j.agwat.2021.107394
Peng, Z. Y., Wu, J. W., and Huang, J. S. (2016). Feasibility of Applying Intermittent Irrigation on Solute Leaching. Adv. Water Sci. 27 (1), 31–39. (in Chinese). doi:10.14042/j.cnki.32.1309.2016.01.004
Qi, Z., Feng, H., Zhao, Y., Zhang, T., Yang, A., and Zhang, Z. (2018). Spatial Distribution and Simulation of Soil Moisture and Salinity under Mulched Drip Irrigation Combined with Tillage in an Arid Saline Irrigation District, Northwest China. Agric. Water Manag. 201, 219–231. doi:10.1016/j.agwat.2017.12.032
Ranjbar, A., Rahimikhoob, A., Ebrahimian, H., and Varavipour, M. (2019). Simulation of Nitrogen Uptake and Distribution under Furrows and Ridges during the Maize Growth Period Using HYDRUS-2D. Irrig. Sci. 37 (4), 495–509. doi:10.1007/s00271-019-00627-5
Sallam, G. A. H. (2017). “The Assessment of Egypt’s Subsurface Drainage System,” in The Handbook of Environmental Chemistry (Berlin, Heidelberg: Springer), 1–32.
Šimůnek, J., Van Genuchten, M. Th., and Sejna, M. (2016). The HYDRUS Software Package for Simulating Two and Three Dimensional Movement of Water, Heat, and Multiple Solutes in Variably-Saturated Porous Media. Technical Manual 2.
Singh, S., Coppi, L., Wang, Z., Tenuta, M., and Holländer, H. M. (2019). Regionalisation of Nitrate Leaching on Pasture Land in Southern Manitoba. Agric. Water Manag. 222, 286–300. doi:10.1016/j.agwat.2019.05.016
Slavich, P., and Petterson, G. (1993). Estimating the Electrical Conductivity of Saturated Paste Extracts from 1:5 Soil, Water Suspensions and Texture. Soil Res. 31, 73. doi:10.1071/sr9930073
Sun, Z. Z., Yue, C. F., and Shi, K. B. (2015). Analysis of Drainage Measures in Xinjiang Water-Saving Irrigation Area. Water Sav. Irrig. 5, 20–25. (in Chinese). doi:10.3969/j.issn.1007-4929.2015.05.006
Tian, F. Q., Wen, J., Hu, H. C., and Ni, G. (2018). Review on Water and Salt Transport and Regulation in Drip Irrigated Fields in Arid Regions. J. Hydraulic Eng. 49 (01), 126–135. (in Chinese). doi:10.13243/j.cnki.slxb.20170909
van Genuchten, M. T. (1980). A Closed-form Equation for Predicting the Hydraulic Conductivity of Unsaturated Soils. Soil Sci. Soc. Am. J. 44, 892–898. doi:10.2136/sssaj1980.03615995004400050002x
Wang, H., Feng, D., Zhang, A. Q., et al. (2022). Effects of Saline Water Mulched Drip Irrigation on Cotton Yield and Soil Quality in the North China Plain. Agric. Water Manag. 262, 107405. doi:10.1016/j.agwat.2021.107405
Wang, J. W., Du, Y. D., Niu, W. Q., et al. (2022). Drip Irrigation Mode Affects Tomato Yield by Regulating Root-Soil-Microbe Interactions. Agric. Water Manag. 260, 107188. doi:10.1016/j.agwat.2021.107188
Wang, J. J., Huang, Y. F., Long, H. Y., et al. (2017). Simulations of Water Movement and Solute Transport through Different Soil Texture Configurations under Negative‐pressure Irrigation. Hydrol. Process. 31 (14), 2599–2612. doi:10.1002/hyp.11209
Wang, Z. M., Li, Z. F., and Zhan, H. B. (2022). Effect of Long-Term Saline Mulched Drip Irrigation on Soil-Groundwater Environment in Arid Northwest China. Sci. Total Environ. 820, 153222. doi:10.1016/j.scitotenv.2022.153222
Wang, Z. M., Jin, M. G., Šimůnek, J., et al. (2014). Evaluation of Mulched Drip Irrigation for Cotton in Arid Northwest China. Irrig. Sci. 32, 15–27. doi:10.1007/s00271-013-0409-x
Wu, Y., Bian, S.-f., Liu, Z.-m., Wang, L.-c., Wang, Y.-j., Xu, W.-h., et al. (2021). Drip Irrigation Incorporating Water Conservation Measures: Effects on Soil Water-Nitrogen Utilization, Root Traits and Grain Production of Spring Maize in Semi-arid Areas. J. Integr. Agric. 20 (12), 3127–3142. doi:10.1016/s2095-3119(20)63314-7
Zeng, W., Xu, C., Wu, J., and Huang, J. (2014). Soil Salt Leaching under Different Irrigation Regimes: HYDRUS-1D Modelling and Analysis. J. Arid. Land 6 (1), 44–58. doi:10.1007/s40333-013-0176-9
Zhang, P., Zhao, X. F., Zhang, T., Zhang, G., Li, B., Xu, H., et al. (2010). Leaching Salinity Effect of Drip Irrigation on Soil of Oasis Shelter Forests in Arid Area. Trans. CSAE 26 (10), 25–30. (in Chinese). doi:10.3969/j.issn.1002-6819.2011.05.005
Zhang, Y., Dai, Y., Wang, Y., Huang, X., Xiao, Y., and Pei, Q. (2021a). Hydrochemistry, Quality and Potential Health Risk Appraisal of Nitrate Enriched Groundwater in the Nanchong Area, Southwestern China. Sci. Total Environ. 784, 147186. doi:10.1016/j.scitotenv.2021.147186
Keywords: brackish water intermittent infiltration, arid area, salt accumulation, numerical simulations, leaching-drainage system, Hydrus-2D
Citation: Leilei G and Zaimin W (2022) Interaction Between Brackish Water Intermittent Infiltration and Cultivated Soil Environment: A Case Study From Arid Piedmont Plain of Northwest China. Front. Earth Sci. 10:894033. doi: 10.3389/feart.2022.894033
Received: 11 March 2022; Accepted: 25 April 2022;
Published: 02 June 2022.
Edited by:
Yunhui Zhang, Southwest Jiaotong University, ChinaReviewed by:
Xianwen Li, Northwest A&F University, ChinaCopyright © 2022 Leilei and Zaimin. This is an open-access article distributed under the terms of the Creative Commons Attribution License (CC BY). The use, distribution or reproduction in other forums is permitted, provided the original author(s) and the copyright owner(s) are credited and that the original publication in this journal is cited, in accordance with accepted academic practice. No use, distribution or reproduction is permitted which does not comply with these terms.
*Correspondence: Wang Zaimin, d2FuZ3phaW1pbjEzQGNkdXQuY24=
Disclaimer: All claims expressed in this article are solely those of the authors and do not necessarily represent those of their affiliated organizations, or those of the publisher, the editors and the reviewers. Any product that may be evaluated in this article or claim that may be made by its manufacturer is not guaranteed or endorsed by the publisher.
Research integrity at Frontiers
Learn more about the work of our research integrity team to safeguard the quality of each article we publish.