- 1Department of Geology, University of Delhi, Delhi, India
- 2Hiroshima Institute of Plate Convergence Region Research, Higashihiroshima, Japan
“Present is the key to the past”—in this all-inclusive uniformitarians’ geological panacea, the Precambrian continental sedimentation system stands out as an odd candidate. The distinctive nature of Precambrian alluvial sedimentation, not analogous to their Phanerozoic counterparts, is highlighted in literature in the absence of rooted vegetation, soil, and an overall greenhouse atmospheric condition. Documentation from Precambrian alluvial deposits around the globe suggests a sedimentation pattern dominated by scree cones, alluvial fans, and braid-plains under the combined effects of aggressive weathering and absence of vegetation; ephemeral braid-plains convincingly outweigh the other alluvial systems in the rock record. It is also surmised that Precambrian fluvial systems, unlike their Phanerozoic-modern analogs, maintained unequivocal ephemeral character across different climatic zones with very less chance of assuming meandering channel planform in absence of buffering effects of rooted plant and adequate mud. Local ponding of muddy sediments, though visualized in some rare cases, was mostly connected with the generation of local paleoslope and unusual riverine hydrology. Recent studies, however, raised a question about the actual paucity of mud, documented meander channel planforms from Precambrian fluvial records, and drew a parallel between the character of the Pre-Silurian alluvial plain on the surface of Earth and meander channel planforms on the Martian surface, interpreted from satellite imagery data. From the collation of data on alluvial deposits from a number of Indian Precambrian basins, augmented by new data from the Bayana basin, a Paleoproterozoic basin in the North Delhi fold belt, the present study attempts to document nuances and intricacies in Precambrian alluvial sedimentation motif that include facies architecture and paleohydrology. From an extensive, though not exhaustive, database, the study intends to claim the establishment of a meander channel-form in Precambrian river systems at a local scale in areas of low discharge and availability of higher silt and mud.
1 Introduction
A possible analog for the pre-Silurian river systems is sought in modern arid climate alluvial systems where rooted vegetation is sparse or absent (Schumm, 1969). In absence of sediment binding, trapping, and baffling by rooted vegetation, Precambrian rivers are modeled with flashy surface runoff, low bank stability, high to very high width: depth ratio, and nearly uniform sandy or gravelly braided character irrespective of climatic and hydrological conditions (Eriksson et al., 1998; Eriksson et al.,2013; Lelpi et al., 2017; Long, 2004; Long, 2006; Long, 2011; McMahon and Davies 2020). Since alluvial sedimentation witnessed a remarkable change with the advent of continental vegetation in Mid-Paleozoic times, it is argued that the hydrology of Pre-Silurian river systems needs special treatment and should not be equated with modern river systems. A distinguishing slope requirement for the alluvial fan (>0.026 m/m) and river (<0.007 m/m) systems and a gap in natural deposition between 0.007 m/m and 0.026m/mof slope values, as proposed by Blair and McPherson (1994), from modern fans and river systems is considered a benchmark for assessment of depositional slope and hydrology for Precambrian river systems (Van der Neut and Eriksson, 1999; Eriksson et al., 2008; Bose et al., 2012 and many others). Working on Precambrian alluvial deposits around the Globe, documentation of a continuous range of variation in river gradients from alluvial fans to the plains (encompassing the “natural depositional gap” of Blair and McPherson, 1994) allowed workers to visualize a general steep gradient for Precambrian river systems (Van der Neut and Eriksson, 1999; Eriksson et al., 2006, 2008; Koykka, 2011a, 2011b; Sarkar et al., 2012; Chakraborty and Paul, 2014). A unique combination involving lack of vegetation, high rate of weathering, and the fault-related steep basin-margin slope is identified as a trigger for such a high alluvial gradient (Van der Neut and Eriksson, 1999), though a bias in the result because of partially preserved channel-forms also may not be overruled (Eriksson et al., 2006). In a parallel study, measurement of slope data from 430 modern alluvial fans (214 from humid climate, Japan, and 216 from arid climate, Death Valley and Owen valley, California) allowed Hasimoto et al. (2008) to document humid fan slope values right within the “natural depositional gap” marked by Blair and McPherson (1994) and hence, question the validity of slope gap conjecture between alluvial fan and river systems in a humid climate. Certainly, the revised understanding prompted workers to raise doubt on the interpretation of Precambrian alluvial strata as a product of fan or fluvial origin since it is well appreciated that 1) enhanced slope failures, landslides and debris flow on hill slopes in absence of vegetation and soil cover may result in misinterpretation of scree cone and alluvial fan deposits as products of coarse-grained braided fluvial systems (Els, 1990; Eriksson et al., 1998; Bose et al., 2012; Barkat et al., 2020), and 2) reworking of a distal alluvial fan by a fluvial system in humid climate may incite misinterpretation of fan deposit as of braid plain origin.
Additionally, increased availability of labile fine materials under greenhouse-gas dominated paleo-atmosphere was cited as a possible trigger behind the high incidence of mass flows and hyper concentrated flows in fluvial systems of Precambrian, but rarely considered in enough volume to generate flood plains in Precambrian fluvial systems (Schumm, 1969; Tirsgaard and Xnevad, 1998; Santos et al., 2014). Nearly all documented Precambrian and early Paleozoic fluvial deposits are dominated by channel or sheet-channel facies with high lateral continuity and a large width-to-depth ratio. Overbank and interfluvial deposits are largely underrepresented (Eriksson et al., 2008; Long, 2011). Going beyond this generalized idea, some recent studies (Owen and Santos, 2014; Santos and Owen, 2016; Barkat et al., 2020) documented the development of point bars and flood plains in Precambrian fluvial systems, particularly of Neoproterozoic time, which raised the question of whether the paucity of flood plain and meandering stream geometry in Precambrian alluvial records is environmentally triggered or more of a bias in preservation record of a fine-grained flood plain. In the absence of any modern-day analog for vegetation-less meandering river systems in humid climates, the extent to which the meandering channel form developed in Precambrian alluvial systems remains uncertain.
In this backdrop, the present article aims at the collation of available understanding from alluvial deposits in Indian Proterozoic basins (traditionally referred to as the “Purana” basins) ranging in age from late Paleoproterozoic to Neoproterozoic, including their paleohydraulic characters and augments it by the description of new data from the Paleoproterozoic Damdama Formation, Alwar Group, Bayana basin, North Delhi fold belt (NDFB). The objective is to highlight the wide variation in scale, architecture, and paleohydraulics of Precambrian alluvial deposits exposed in the Indian subcontinent. An attempt has also been made to provide an idea of paleo flow conditions and possible downstream variation in alluvial style, wherever possible, from a description of architectural elements and empirical hydraulic constraints. Since climate plays a major role in sediment supply and platform development in modern alluvial systems (Vandenberghe, 2003; Kasse et al., 2017), we also tried to assess the possible role of climate in Precambrian fluvial systems from the nature of architectural elements and derived paleohydraulic (discharge) parameters from the alluvial deposits.
2 Tectonic Set Up for the Fluvial Deposits Described Herein
The description of thick and laterally extensive Precambrian fluvial deposits mostly come from the rift, continental margin, foreland, and successor-basin settings. There is no exception in the Indian perspective. This article summarizes fluvial deposits spanning over entire Indian peninsula encompassing Paleoproterozoic Par Formation, Gwalior Group, central India and Damdama Formation, Alwar Group, Bayana basin western India, Mesoproterozoic Rehtikhol Formation, Singhora Group, Chhattisgarh Supergroup, and unnamed basal formation, Ampani Group, central India and Ramdurg Formation, Bagalkot-Badami Group, southern India, and Neoproterozoic Sonia and Girbakhar Formations, Marwar Supergroup, western India and Banganapalli Formation, Kurnool Group, southern India (Figure 1). Detailed facies mapping, documentation of facies stacking motif, “Sequence” boundary tracing in space and time, and tracking (Figures 2A–J) of geochemical tracers (e.g., εNd) from source to sink in the last two decades have allowed workers (Chakraborti et al., 2007; Patranabis-Deb and Chaudhuri 2008; Chakraborty et al., 2020) to propose tectonic models including foreland, rift, passive margin, sag, etc., for Indian Proterozoic basins, although none of these models have found unequivocal acceptance. Ariftogenic origin is proposed for the Gwalior, Bayana, Bagalkot-Badami, and Marwar basins, fringing different craton boundaries (Basu and Bickford, 2015; Meert et al., 2010; Chakraborty et al., 2009, 2020), though signatures supporting fault-control on sedimentation are often not unequivocal. For the Chhattisgarh, basin opinions vary between intracratonic sag (Das, 1992), rift (Patranabis-Deb and Chaudhuri 2008), and foreland (Chakraborty and Paul 2014), and two competing hypotheses, viz. 1) foreland (Singh and Mishra 2002) and 2) deep-seated basin-margin fault systems and mantle-induced thermal perturbation (Chaudhuri et al., 2002), are available for the initiation of the Kurnool basin. Though none of these models satisfy all lines of evidence obtained from geological and geophysical studies, a general consensus is emerging that the initiation and closure of Indian Proterozoic basins may be related to the accretion and breakup of Supercontinents “Columbia” and “Rodinia” through this time period (Chakraborty et al., 2009; Meert et al., 2010; Basu and Bickford, 2015; Meert and Pandit, 2015; Chakraborty et al., 2020; Samanta et al., 2022).
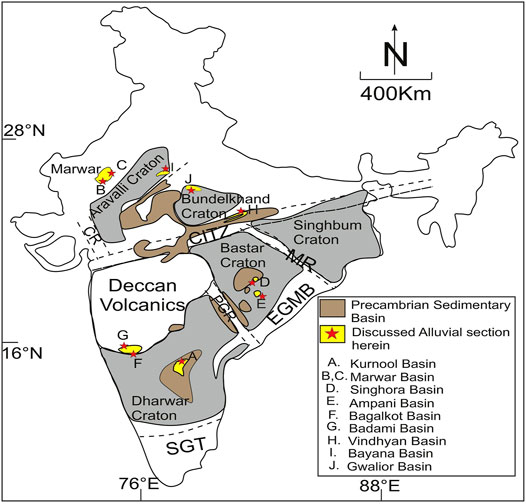
FIGURE 1. Indian peninsula, cratons therein, and Precambrian sedimentary basins. Location of discussed alluvial sections marked by asterisks.
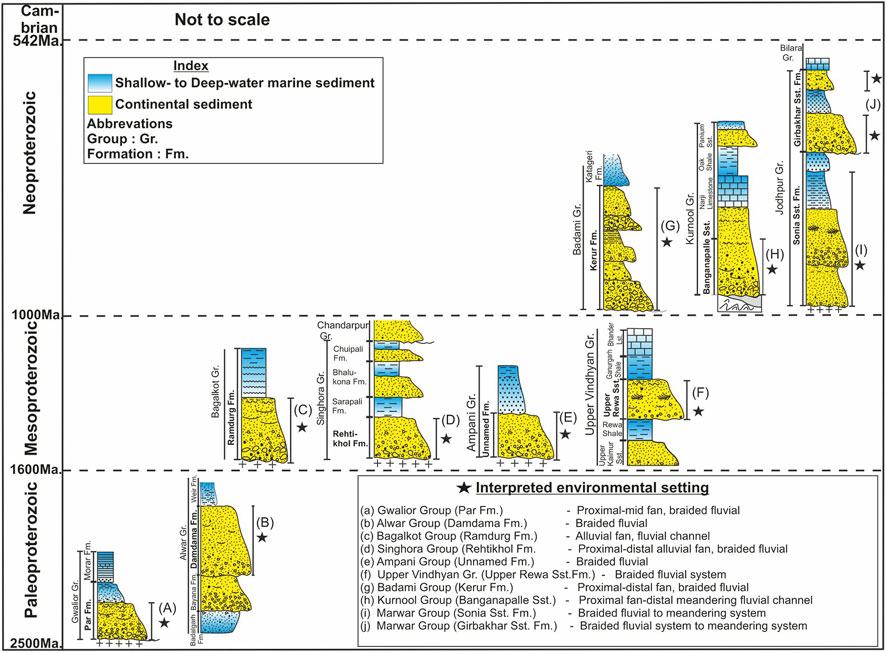
FIGURE 2. Age-wise classification of Indian Precambrian sedimentary basins, their generalized stratigraphic successions, and stratigraphic positions from where alluvial deposits are described (marked by asterisks).
3 Facies and Architectural Element
A wide variation in lithology, including conglomerates, gravelly, pebbly mixed granular/coarse-grained sandstone, coarse- to medium-grained sandstone, fine-grained sandstone, and heterolithic sandstone-shale, represents the alluvial deposits in Indian Proterozoic basins. Studies in the last two decades have attempted to classify these deposits in terms of various lithofacies and then grouped them in terms of architectural elements, following Miall (1983), Miall (1985), Miall (1988), and Allen (1983). The documentation of architectural elements, their characteristics, and mutual relationships allowed the understanding of the evolution of the Precambrian alluvial system (fan and fluvial) and the delineation of local and regional processes involved therein. Architectural elements are defined by their grain size, constituent lithofacies, bounding surfaces, paleocurrents, and vertical and lateral relationships. Table 1 illustrates the architectural element classification used in the present study. Rank designations (hierarchy of depositional units and bounding surfaces), wherever available, are documented here following Miall (1993). Commonly, if the orientation of an accretionary unit including cross-stratifications or any other paleocurrent indicator is within 60° of each other, then it is considered frontal accretion (upstream or downstream), and where the orientation of the accretionary unit and the cross-stratification are more nearly perpendicular (>60°), a lateral accretion interpretation is preferred (cf. Jones et al., 2001).
3.1 Paleoproterozoic Alluvial Deposits
3.1.1 Par Formation, Gwalior Group, Central India
Unconformably overlying Archean granites/gneisses of the Bundelkhand craton, a ∼20 m thick coarse-grained conglomerate/granular sandstone package represents the alluvial product at the basal part in the Par Formation of the two-tiered (Par Formation and overlying Morar Formation) Gwalior Group (Figure 2A). Depending on the relative abundance of conglomerate, granular sandstone, and coarse- to medium-grained sandstone and their depositional architecture, three different continental environmental conditions are deciphered, viz., inner fan, mid fan, and braided fluvial. Conglomerates with 1) unorganized high-concentration angular, bouldery/pebbly clasts, 2) chaotic boulder clasts, 3) inverse- to coarse-tail normal-graded pebbles, 4) crude bed-parallel clasts, and sandstones with 5) coarse grain and stray pebbly clasts and 6) massive/plane-lamination, in combination, constitute the inner fan, whereas 1) sheet conglomerates, 2) pebbly sandy planar couplets and iii) tabular, massive granular sandstones comprise the mid fan part. The braided fluvial product is represented by granular—fine-grained sandstone couplets and multi-storied sheet sandstones. Rock avalanches (Figure 3A) and hyper concentrated flows of widely varying rheology dominate the inner fan part, whereas sheet floods (Figure 3B) and streamlet flows are major depositional components in the mid fan. Multi-story sheet sandstones (SS) of braided fluvial deposit are made up of granular, coarse-grained massive (Gms), horizontal (Gh) and planar cross-stratified (Sp) sandstone, coarse- to medium-grained cross-stratified (St) or plane-laminated (Sh) sandstone and buff-colored fine-grained sandstone/siltstone (Sf). The upward transition from massive/parallel laminated (Gm/Gh) to ripple laminated sandstone (Sr) within the sheets together, with the capping of a thin layer of siltstone/mudstone (Sf), are identified as products of waning flow, commonly observed in pre-Devonian sandy ephemeral river systems (Martins-Neto, 1994; Long, 2002). Sheets with a planar, non-erosional base and with a definite signature of basal incision are identified as CHS and CH elements. In association with SS, CHS, and CH elements, products of upstream (UA) and lateral (LA) accretion, in subordinate volume, constitute the fluvial section. It is surmised that accretionary deposits (LA and UA) formed during events of enhanced rates of sedimentation (cf. Bose et al., 2001; Long, 2011). The dominance of upper-flow-regime elements and unconfined sheet flood deposits prompted the characterization of the channel system as sandy ephemeral in nature.
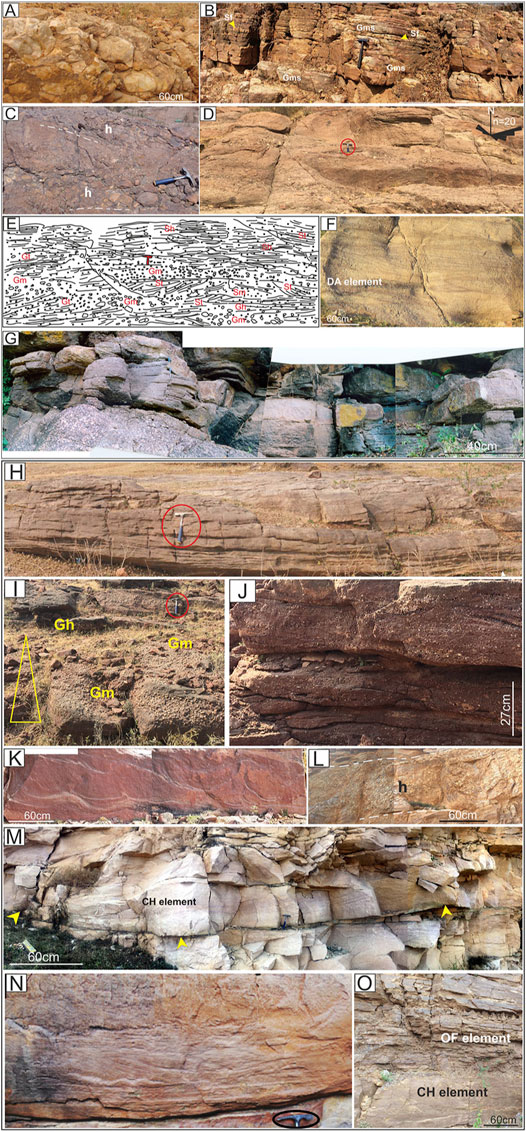
FIGURE 3. Field photographs showing (A) rock avalanche, (B) alternation between massive, pebbly granular sandstone (Gms) and centimeters-thick fine/medium-grained sandstone (Sf) resulting in couplet stratigraphy of sheet flood deposit from alluvial fan of Par Formation, Gwalior basin. (C) hyperconcentrated flow (h) deposit, (D) ephemeral fluvial deposit showing mutual disposition of gravel bar and channel elements (E) sketch thereof and (F) downstream accretion element from the Damdama Formation, Bayana basin. (G) Amalgamated sheet sand (SS) element from the Rehtikhol fluvial deposit, Singhora basin; note sharp contact with underlying conglomerate. (H) Large-scale trough cross-stratifications with superimposed bedforms from the Rewa fluvial deposit, Vindhyan basin. (I) Incipient fining-upward sequence starting with (Gm) at the base and (St) at its top, (J) amalgamated CH elements in coarse-grained granular poorly-sorted sediment and (K) trains of convolute lamination from the Girbakhar fluvial deposit, Jodhpur Group. (L) Hyperconcentrated (h) flow deposit, (M) CH element, sand bar (SB) with superimposed trough cross-stratification (St) filling the channel, (N) lateral accretion and (O) flood plain (OF) from the Banganapalle fluvial deposit, Kurnool basin.
Chakraborty and Paul (2014) identified five different orders of bounding surfaces with the Par fluvial association; whereas surfaces that bound the fluvial association i.e., the contact with the basement at its base and the contact with shallow marine succession at its top are assigned the highest order i.e., the 5th order, surfaces bounding sheet-sandstone units, comprising of channel-overbank fine assemblage, is considered next lower order in the rank. The third-order bounding surfaces are the boundaries that define the contact between different macroforms e.g., channel (CH), subordinate overbank fines (OF), and upstream/lateral accretion (UA, LA) elements, etc.
3.1.2 Damdama Formation, Alwar Group, Western India
Unconformably overlying the Archean basement comprised of meta-sediments and granite, the Paleoproterozoic Alwar Group of rocks, the Delhi Supergroup, includes ∼200 m thick poorly-sorted, coarse-grained, often pebbly and bouldery, sandstone succession of the Damdama Formation that represents the alluvial product in the Bayana basin of the North Delhi fold belt (NDFB) in the northwestern part of the Indian peninsula (Figure 1). As a fine-upward succession, the alluvial section registers two different styles of riverine action. Whereas deposits of a shallow gravel-bed braided system dominate the basal part, deposits of a shallow, sandy braided system constitute the upper part of the Damdama succession (Figure 2B). Stacked decimeter- to meter-thick sheets of gravelly, granular sandstone and very coarse- to coarse-grained sandstone, dominantly constituted of gravel bars and bedforms (GB) and minor sandy bedforms (SB), define the general character of the lower gravel-bed braided system; SB elements are mostly confined as thin wedges in abundant channels. Whereas lenticular units of massive/cross-stratified gravelly/pebbly granular sandstones (Gm, Gt) with erosional base signify shallow ephemeral channels, non-channelized conglomerates (Gm; Figure 3C), sheet-like in geometry, with interbeds of coarse-grained sandstones, constitute couplets formed out of sheet floods. The occurrence of multi-storied sheet flood deposits, traceable up to tens of meters, suggests a lobe of aggravation because of multiple sheet flood events from the feeder channel. Crudely defined cross-stratifications with the randomly strewn pebble at their base, in cases along with forests, suggest deposition from high-velocity flashflood within the channels (Pfluger and Seilacher, 1991; Blair and McPherson, 1994) with down current in the north and30° deviation in both east and west (Figures 3D, E). An increase in the abundance of trough cross-stratifications (Gt, St) upward in the succession bears an indication of active bedform migration in decreased sediment concentration in the flow. Plain laminations at the top of the beds indicate an increase in shear flow with decreasing flow depth.
A sharp contrast in the fluvial character is noticed in the upper part of the Damdama lithopackage. Amalgamated sand sheet (SS) elements, composed of laterally extensive coarse-grained sandstone with tabular or gentle concave-up geometry (100–150 m wide, 0.85–1.6 m thick) and a scalloped/planar base, represent the shallow, sandy ephemeral fluvial deposit. Deposits of SS elements, with co-sets of planar (Sp) and trough (St) cross-bedding, dip gently toward the northeast and form a ∼60 m thick stacked succession. Sf element is recorded between some of the sandstone sheets and represented by a cm-thick fine-grained sandstone/siltstone unit. In contrast, channel (CH) elements, made up of medium-coarse grained sandstone, have a broad lenticular bed geometry and are internally composed of horizontal lamination (Sh), planar cross-stratification (Sp), and trough cross-stratification (St; with an average set thickness of 12 cm), in order of superposition. In addition, decimeter- to-meter-thick downstream accretion (DA) elements are also observed at the upper part of the studied fluvial succession (Figure 3F), with a decrease in the set thickness of cross-strata. Only a minor presence of lateral accretion (LA) elements is noticed in the uppermost part.
In the upper part of the stratigraphic succession of the Damdama Formation, the noteworthy changes include 1) a decrease in the maximum grain size with a complete absence of gravels and pebbles in the upper part; 2) the absence of conglomeratic/granular sandy units; 3) a decrease in the set thickness of cross-strata, and 4) a decline in the incisive character of the channel (CH) element.
3.2 Mesoproterozoic Alluvial Deposits
3.2.1 Rehtikhol Formation, Singhora Group, Chhattisgarh Supergroup
Resting unconformably above phyllites and gneissic rocks of the Bastar craton, the Rehtikhol Formation of the Singhora group, Chhattisgarh Supergroup, demonstrates a transition from alluvial fan to braid plain system (Chakraborty et al., 2009). From the preponderance of granular and sandy sediment gravity flow products with subordinate rock fall deposits (Figure 2D) within the alluvial fan system together with a pervasive west-northwesterly paleocurrent, Chakraborty et al. (2009) suggested a gently sloping beveled source area in the east–southeast of the basin. Depending on the relative dominance of mass-flow conglomerates and channel flow products, two anatomical parts of the alluvial fan, viz., inner fan, and mid fan are delineated. Conglomerates in the inner fan are mostly lenticular and interpreted either as products of rock fall or hyper concentrated flows; free fall of clasts are identified by their penetration of the underlying substrate. A subaerial origin for the cohesive pseudoplastic flows is inferred from their low mud content (cf. Vallence, 2000). Reverse graded, clast-supported conglomerates are identified as sieve deposits formed by transport and segregation of larger clasts; their upward movement is not prevented in a non-cohesive matrix (Todd, 1989; Major, 1997). Sheet sandstones (SS), sheet conglomerates, and channelized (CH) conglomerates, in decreasing order of abundance, represent the mid fan. Whereas the SS units are identified as products of ephemeral fluvial channels, sheet conglomerates are interpreted as products of unconfined flows related to sheet flood events (McPherson et al., 1987; Chakraborty et al., 2009; Barkat et al., 2020). An intergradational relationship between sheet flood and stream channel deposits is a common observation in mid fan and is considered a hallmark character for alluvial fan succession (Rust and Koster, 1984). Surges of coarse-grained, highly concentrated flows generated during high-magnitude flood events either remain confined within channels or spread out beyond the margins of shallow ephemeral channels (Benvenuti, 2003; Deynoux et al., 2005).
Horizontal- and cross-stratified granule-rich, coarse-grained, poorly sorted sheet sandstones, granule-free coarse-grained sandstones with clast-supported conglomerates, in subordinate volume, constitute the Rehtikhol braid-plain association (Figure 3G). Stacked decimeter- to meter-thick fining-upward successions constituted of SS or CH elements (on average 0.85 m in thickness) with erosive, scalloped, or scoured base make up the association and traceable in outcrop for lengths exceeding tens of meters (cf., Miall, 1985; Eriksson et al., 1995; Jones et al., 2001). Internally the SS units are characterized by horizontal lamination (Sh) and lesser low-angle planar cross-bedding (Sp) and suggest a series of sandstone channel-fills, with only small-scale bar development, probably under flood conditions (cf., Miall, 1977, 1978; Dam and Andreasen, 1990; Eriksson et al., 1995). From the occurrence of planar cross-bedded units in lateral and vertical association with horizontally laminated units a variable energy flow condition is inferred in channels with the transition from lower flow regime dune bedforms to upper flow regime plane bed conditions. A weakly dispersed paleocurrent pattern favors this view.
3.2.2 Unnamed Formation, Ampani Group
Resting unconformably above the granitic-gneissic basement of the Bastar craton, the ∼300 m thick Ampani siliciclastic succession (Figure 3E) records products of continental to shallow marine depositional environments. The fluvial lithopackages, which occupy the basal position in the succession, hold the continental sediment record, and are comprised of stacked decimeter- to meter-thick tabular units made up of poorly sorted coarse-grained, granule-rich arkosic sandstone, clast-supported conglomerate, and granule free sandstones in the form of a sandy sheet (SS) and small channel (CHS) elements. CHS elements dominate the lower half of the fluvial succession, show fine upward character, and are internally constituted of conglomerate (Gm, Gh), granular sandstone (Sgm, Sgt), and granule-free coarse- to medium-grained sandstone (Sh and St) lithofacies. Also, Sgt, Sh, and St facies units were arranged in a multi-set manner to assume a “Sandy bedform (SB)” element. Average set and coset thicknesses of trough cross-stratification (St) are ∼8 and 17 cm, respectively, and their paleocurrent is unimodal with moderate dispersion. The upper half of the fluvial succession is devoid of Gm and Gh lithofacies; instead, stacked sandstone lithopackages dominate, internally made up of St, Sh, and Sp facies units. Overbank fine deposits (OF) are absent in the Ampani fluvial deposit (Chakraborty et al., 2017).
3.2.3 Ramdurg Formation, Bagalkot Group
A sedimentary record that includes terrestrial screen and fan giving way downslope to a braided fluvial channel is documented from the Mesoproterozoic Ramdurg Formation, lower Lokhapur subgroup, Bagalkot Group in a riftogenic setting (Bose et al., 2001). Whereas the fan deposit records a coarsening-upward stacking motif, aggradation of channels within the fluvial succession results in a fining-upward stacking motif with a reduction in downslope gradient and grain size fining (Figure 2C). An ensemble composed of scree breccia, conglomerates of debris flow, sheared mass flow, sheet flow, modified grain-flow origin, and granular sandstones of hill wash and streamlet origin constitute the fan deposit. The fluvial deposit is represented by coarse- to medium-grained sandstones representing channel and bar elements with occasional aeolian adhesion laminae and translated strata. Products of SS, SB, CH, DA, LA, and SG elements are documented from the fluvial succession (Bose et al., 2001); incorporation of SG element in local scale is identified as a signature for flow variability, albeit at a large scale and long interval. Taking into consideration sand body geometry and different flow vectors, their stages, and architectural elements, three different types of stratal packaging, each with a fine upward succession, are identified within the fluvial deposit (Bose et al., 2001) and interpreted as products of different fluvial styles. Whereas vertically and laterally juxtaposed small channel elements (CHS) and crudely-stratified flash flood deposits characterize the Type I package, the occurrence of scours (up to 0.5 m depth) within wide channel belts and the presence of master erosion surfaces mark the Type-II and Type III packages, respectively. On the basis of detailed architectural element documentation and their spatial relations, Bose et al. (2001) claimed the Type I package as the product of ephemeral streams, whereas type II and III were semi-perennial and perennial river deposits. In absence of vegetation, tectonics-related slope variation along and across the basin is considered the prime factor for sediment distribution and sequence development motif in the Bagalkot alluvial system.
3.2.4 (Upper) Rewa Sandstone Formation, Vindhyan Supergroup
Constituted of medium- to coarse-grained quartz-arenitic sandstone, the Rewa Formation is represented by an upward transition from marine-tidal to fluvial/eolian deposits (Bose and Chakraborty, 1994) (Figure 2F). Unlike most other fluvial records described herein, the Rewa Formation holds a thick fluvial deposit (∼350 m thick) in its upper part with the presence of large-scale (meters-scale in width) bedforms (Figure 3H). Stacked decimeter- to meter-thick multi-storied poorly sorted, granule-rich arenitic sandstone bodies having large-scale planar cross-stratification, trough cross-stratification, parting lineated parallel lamination represent the fluvial product (Bose and Chakrborty, 1994, Verma and Shukla, 2020). Studies in the last three decades (Bose and Chakraborty, 1994; Verma and Shukla, 2015, 2020) documented six distinct lithofacies types from the Rewa fluvial succession, viz. 1) granule rich trough cross-stratified lensoidal sandstone with concave-up geometry; heavy concentration of coarse-grained sediment (Gm) at their bases with occasional cross-stratified granule filled scours (Gt), 2) solitary sandstone bodies with planar cross-sets; low-dipping forests (6°—9°), commonly separated by granules rich layers (Gm). 3) granule-free, medium- to coarse-grained sandstone with superimposed planar cross-cross-stratified (Sp), and trough cross-stratified (St) units, 4) tabular to lensoidal sandstones constituted of compound cross-stratification (Sp) with very low angle (2°-3°) dip for both cross-strata and their set boundaries in the same direction, 5) fine-grained and well-sorted sandstone with low-angle ripple cross-lamination (Sr, Sl), and 6) Tabular cross-stratified well-sorted sandstone internally composed of alternating cross-strata in two different styles; one lighter and inversely graded and the overlying one finer-grained, dark-colored and upslope wedging. Dominant architectural elements documented from the Upper Rewa fluvial deposit include a channel (CH; comprised of Gm, Gt, and Gp lithofacies), and a Sandy bedform (SB; comprised of Sp, St lithofacies). UA (Sr lithofacies), DA (Sp lithofacies), and DA (St, Sl lithofacies) elements. Mukhopadhay (2012) identified six different orders of sand bodies within the fluvial succession, the largest being the valley-fill and the smallest being small cycles of channels.
3.3 Neoproterozoic Alluvial Deposits
3.3.1 Kerur Formation, Badami Group
Fining-upward alluvial succession ranging from scree cone through the alluvial fan to braided fluvial is described from the Kerur Formation, Badami basin (Samanta et al., 2022) (Figure 2G). Whereas rockfall and debris flow dominate the Kerur scree cone and proximal alluvial fan successions, streamlet, sieve, and sheet flood share major depositional processes in the middle to a distal fan. With the decrease in depositional slope downstream, the braided fluvial system occupied the Kerur alluvial plain. Whereas streams in the middle to distal alluvial plain behave as ephemeral, those in the braided fluvial setting are identified as semi-perennial to perennial. Conglomerates (clast- and matrix-supported, massive/graded), coarse- to medium-grained sandstones (massive, trough or tabular cross-stratified, planar stratified, ripple laminated) in form of different architectural elements such as channels (CH), sediment gravity flow (SG), laminated sand sheet (LSS), downstream accretion (DA), lateral accretion (LA) and transverse bar (TB) elements represent the Kerur alluvial succession. Samanta et al. (2022) have described a wide range of depositional processes including debris flow, modified grain flow, sheet flow, or as channel thalweg deposit from facies architectures of the conglomerate units. From facies building patterns, their spatio-temporal variations, and paleocurrent analysis, it is inferred that the stratigraphic architecture in the Kerur alluvial succession is controlled dominantly by basin margin tectonics in both strike-and dip-parallel directions. In addition, it is also inferred that the water table fluctuations in a semi-arid climatic condition controlled the spatial flow duration within the braided fluvial systems. From the progradational facies stacking pattern in the proximal fan and facies architecture within the fluvial succession, Samanta et al. (2022) identified the Kerur Formation as a product of a low-stand systems tract (LST) with a slow rise in the base profile and inferred a more accelerated rise in the later part of alluvial depositional history.
3.3.2 Sonia Formation, Jodhpur Group, Marwar Supergroup
Overlying the 752 ± 18 Ma (786 Ma-750 Ma; Pradhan et al., 2010; Meert et al., 2013) Malani Igneous suite (MIS), the late Neoproterozoic Jodhpur Group, Marwar supergroup presents continental fluvial record at multiple stratigraphic levels (Figure 2I). Stratigraphically the Jodhpur Group is subdivided into two formations, namely the Sonia and Girbakhar; whereas the Sonia Formation is arenaceous, the Girbakhar Formation is dominantly argillaceous. Products of continental fluvial sedimentation are recorded from the basal part of the Sonia Formation and from two different stratigraphic levels (base and top) of the Girbakhar Formation. Encased between the MIS at its base and shallow-marine sediments at the top (Samanta, 2009; Sarkar et al., 2012), the Sonia fluvial deposit is represented by poorly sorted and profusely cross-stratified sandstone. On the basis of bounding surface geometry, constituent lithofacies, and architectural element analysis, the Sonia fluvial deposit is classified into three distinctive subdivisions (subdivisions I, II, and III). Subdivision I occupies the basal position of fluvial lithopackage with narrow lenticular bed geometry, minimum preservation of flood plain deposit, and is composed of medium to fine-grained quartz arenite, devoid of any extramarital pebbles and interpreted as a result of channel aggradation in response to rising base level (water table). The presence of an intermittent eolian interdune facies deposit suggests the semi-perennial nature of the river system (Sarkar et al., 2012). An overall expansion of drainage area in the fluvial system is documented in the upward transition from Division I to II in connection with either source upliftment or climate-related enhancement in sediment discharge rate. Overall, the subdivision II (55 m in thickness) represents a fining-upward succession with the occurrence of a wide spectrum of water escape structures and patches of adhesion laminae, translated strata, grain flow/grain fall cross strata. A fining-upward stacking in this subdivision reflects sediment aggradation due to a rise in base level. Only in the uppermost part of subdivision II records an increase in grain size registers a fall in base level. The ∼45 m thick uppermost subdivision III comprises mudstone and a silty mudstone unit that rests uncomformably above the rhyolitic basement along the basin margin. It lacks adhesion laminae, translated strata, and grain flow/grain fall cross strata. A sharp increase in mud content marks the transition from subdivision II to subdivision III and records a rapid enhancement in the rate of base-level rise due to basin subsidence. The presence of a soft sedimentary deformation feature at the top of subdivision II favors the tectonic subsidence hypothesis that enhanced the base level profile substantially. The great preservation of aeolian deposits in Sonia fluvial system possibly reflects the dominant role of accommodation variation on the facies distribution rather than the climatic influence. With the help of detailed facies and facies association analysis, various fluvial architectural elements like small channel (CHS), downstream accretion (DA), lateral accretion (LA), laminated sand sheet (LSS), transverse bar (TB), overbank fine (OF), and sediment gravity flow (SG) elements have been delineated within the Sonia fluvial system.
3.3.3 Girbakhar Formation, Jodhpur Group, Marwar Supergroup
Two different styles of fluvial products are documented from the Girbakhar Formation of the Jodhpur Group, viz., 1) ephemeral sandy braided system and 2) ephemeral sandy meandering system. Whereas granular-pebbly sheet sandstones in amalgamation represent the sandy braided system, medium- to coarse-grained ferruginous sheet sandstone with or without siltstone/silty mudstone represents the meandering alluvial planform (Figure 2J). The Garushia Mandir section at the easternmost margin of the basin records deposits of both braided and meandering systems in order of superposition; whereas the dominance of GB, SG, and CHS elements in the basal part of the section demonstrates flashy, ephemeral braided channel character (Figures 3I, J), the preponderance of SS, CH, and OF elements within fining-upward sequences allowed interpretation of distal meandering channel-form in the upper part of the section. Unidirectional cross-stratifications dipping at an oblique angle with basal bounding surfaces is a common observation in the upper part. Additionally, the occurrence of laterally persistent soft-sediment deformations (SSD) layers, including convoluted lamination, flame structure, etc., is noteworthy in the upper part (Figure 3K). Westward, occupying the uppermost part of the Girbakhar Formation, deposits of shallow, sandy perennial braided river deposits can be tracked in most parts of the basin with stacked sequences of sandy bedforms assuming small-scale depositional sequences with high lateral continuity (cf. sheet braided; Cotter, 1978; Long, 2011). Sheet elements (SS) with monotonous planar and trough cross-bedded characteristics and set boundaries parallel to coset boundaries are a common observation. Locally, the occurrence of downstream accretion macroforms (DA) is a result of in-channel languid or complex bar migration (Long and Donaldson, 2005).
3.3.4 Banganapalle Formation, Kurnool Group
Resting unconformably above the granite-migmatite basement of the Peninsular gneiss and the Cuddapah lithopackage (Cumbum Shale), the Banganapalle alluvial deposit is made up of clast-and matrix-supported conglomerate, gravelly mixed coarse- to medium-grain sandstone, and fine-grained sandstone with the subordinate presence of siltstone and mudstone (Figure 2H). From lithofacies and facies association analysis, the alluvial deposit is classified under both alluvial fan and fluvial depositional systems, spanning from mid fan to a distal ephemeral meandering fluvial system with sinuous migratory channels combing over the floodplain (Barkat et al., 2020). Products indicating processes like sheet flow, stream flow, occasional hyper-concentrated and debris flow (cohesive and non-cohesive; Figure 3L), and slope failure (SG with minor CHS elements) are documented from the alluvial fan system. From the relative dominance of mass flow and channel deposition products, two anatomical parts, namely the mid fan and distal fan of the alluvial fan, are delineated. In addition, an overall decrease in clast size and concentration, and bed thickness demarcates the distal fan from the mid fan. Long-term denudation of basement rock along with base-level fall is identified as a major controlling factor for stream paleohydrology and sediment discharge in the Banganapalle alluvial fan system. In the down-current braid, plain and distal ephemeral meandering systems represent the fluvial deposit. A multi-story coarse-grained sandstone unit with a sand sheet (SS) and channel (CH) element (Figure 3M) suggests domination of the dune field and a simple bar in the braid plain. Further down-current, the distal ephemeral meandering system is represented by an ensemble of laminated sandstone sheets (LS), downstream accretion (DA), lateral accretion (LA) (Figure 3N), and small channel elements (CHS) in alternation with overbank fine (OF) element (Figure 3O); small channel elements generally thinner with respect to downstream accretion or lateral accretion element with which they are laterally adjacent to or overlying them. Fining-upward packages composed of an ensemble of laterally adjacent bars and channels represent the stacking motif of a distal meandering deposit. Overall, the fine upward stacking pattern with the presence of heterolithic lateral accretion strata infers the operation of stream meander and helical flow in the channel system. From the U-Pb detrital zircon geochronology, Barkat et al. (2020) identified the TTG suite of peninsular gneiss and volcanic-hosted greenstone belt in central and western Dharwar craton as major provenances those supplied detritus in the Banganapalle alluvial system.
4 Alluvial Hydraulics and Distinction Between Different Continental Sub-Environments
Braided streams often represent a major component of the alluvial fan system and, hence, braided streams and alluvial fans are not separable in space and time (Boothroyd et al., 1978; Nilsen, 1982; Eriksson et al., 1998; Bose et al., 2012). In fact, the distinction between fluvial-dominated alluvial fans (Hartley et al., 2010; Weissmann et al., 2010), braided fluvial, and braid plain systems in Precambrian complex alluvial records often becomes tentative and difficult in the absence of channel paleoslope data (Eriksson et al., 2006). Process-based sedimentology is commonly advocated to overcome the challenge. Since the height of dunes within river channels is reflective of channel flow parameters, empirical relations derived between the set thickness of dune cross-strata and channel parameters based on observations in modern rivers are relied upon for deduction of paleoflow parameters from Precambrian fluvial systems (Van der Nuet and Eriksson, 1999; Leclair and Bridge, 2001; Ito et al., 2006; Sarkar et al., 2012; Chakraborty and Paul, 2014). Though the obtained values may not be precise, they are still considered robust enough for comparative studies. An attempt has been made here to collate paleohydraulic data documented from different Precambrian fan and fluvial deposits present in the Indian peninsula and discussed herein (Figure 4; Table 2). A special mention regarding the paleohydraulic data from the Damdama fluvial section, Bayana basin since it is attempted for the first time. Paleohydraulic estimations were carried out using various methods following Eriksson et al., 2006, Sarkar et al., 2012, and Long 2021 (Figures 5A–C, Appendix A, B, and C) reveal a significant difference in slope estimation. Whereas following Eriksson et al. (2006) and Sarkar et al. (2012) estimated slope values from the Damdama fluvial deposit fall mostly in the natural depositional gap or in the alluvial fan (>0.026 m/m) field, estimations done following Long (2021) fall dominantly in riverine (<0.007 m/m) field. Bankfull discharge values vary between 254.50 m3/s and 840.40 m3/s from section to section.
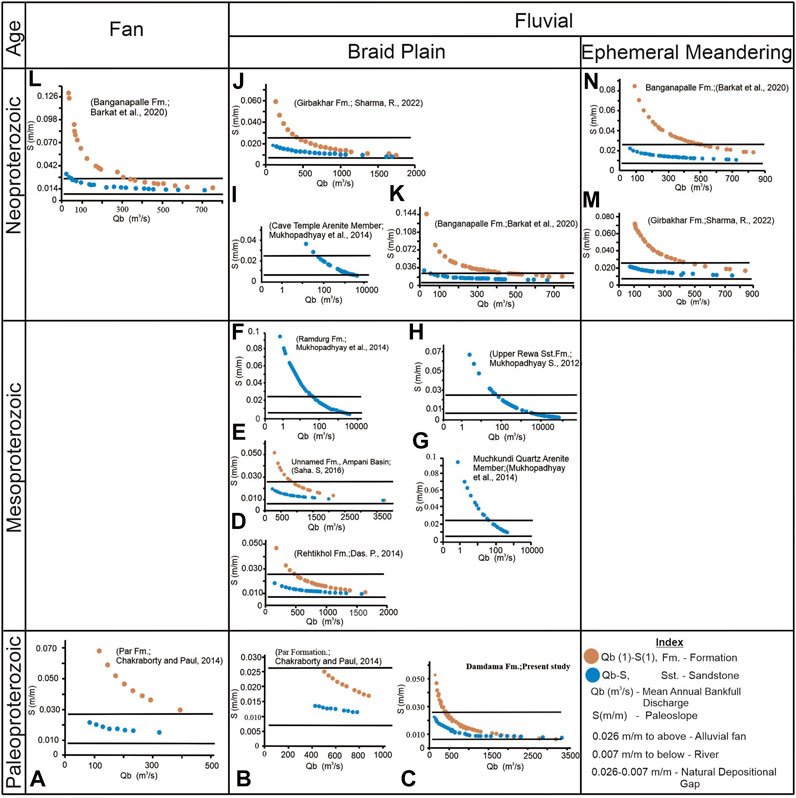
FIGURE 4. Binary plots of palaeoslope (S) and mean annual bankful discharge values (Qb) were calculated from alluvial deposits (fan, braid plain and ephemeral meandering) of Indian Precambrian basins ((A,B) Par Formation Gwalior basin. (C) Damdama Formation, Bayana basin. (D) Rehtikhol Formation, Singhora basin. (E) Unnamed Formation, Ampani basin. (F) Ramdurg Formation, Bagalkot Group. (G) Muchkundi Quartz Arenite Member, Badami Group. (H) Upper Rewa Sst, Formation, Vindhyan Supergroup. (I) Cave Temple Arenite Member, Badami Group. (J,M) Girbakhar Formation, Marwar Supergroup. (K,L,N) Banganapalle Formation, Kurnool Group). Note slope values falling in the Natural Depositional Gap (0.007 m/m to 0.026 m/m) in most of the cases. Also, note low to very low discharge in most of the cases and very high discharge in the Rewa fluvial system.
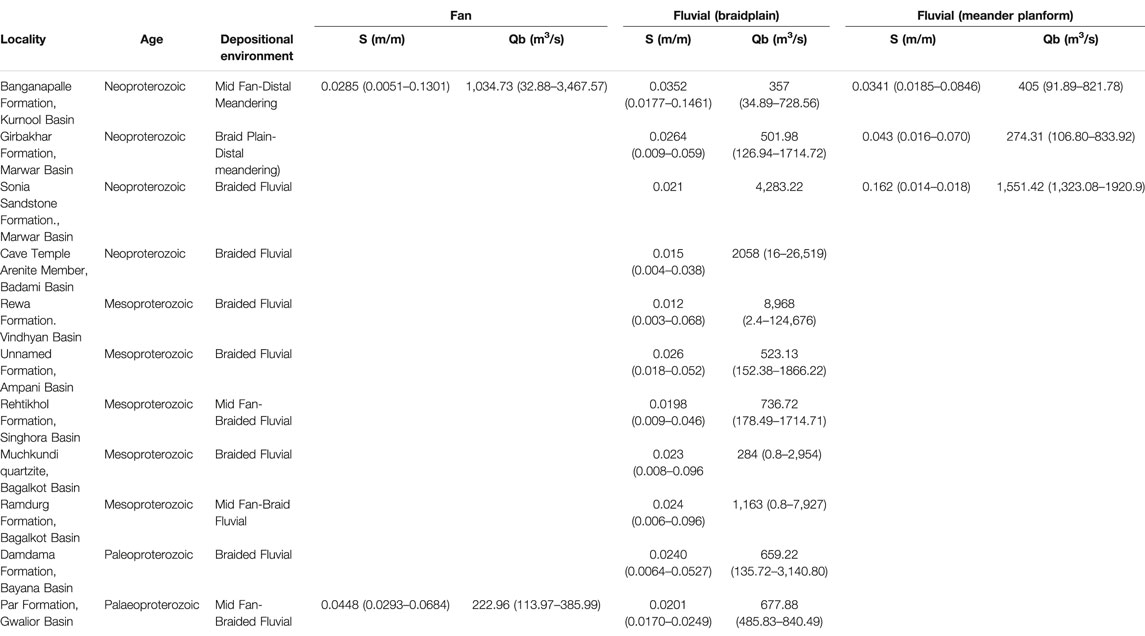
TABLE 2. Alluvial deposits from Indian Precambrian basins, their geological ages, interpreted depositional settings viz. fan, braided fluvial, distal meandering fluvial, and deduced paleohydraulic parameters (slope and discharge).
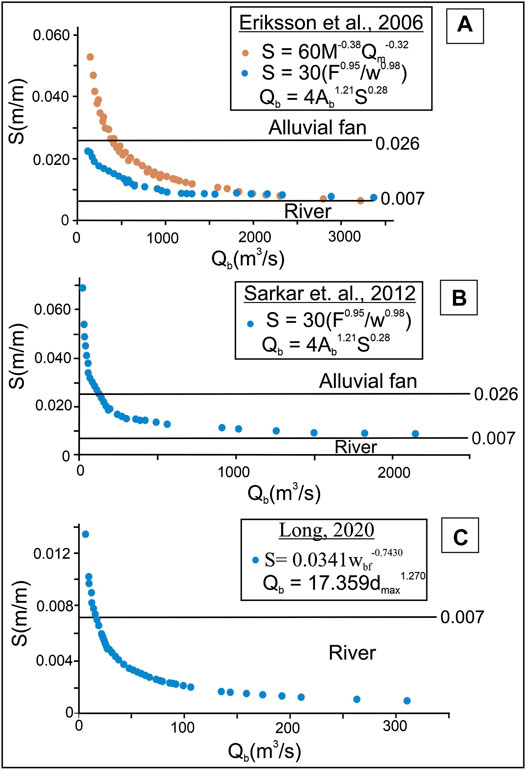
FIGURE 5. Binary plots of palaeoslope (S) and mean annual bankful discharge values (Qb) for Damdama fluvial system following (Eriksson et al., 2006) (A); (Sarkar et al., 2012); (B) and (Long 2021) (C). Note dominant plotting of slope values either in the field of an alluvial fan or in the natural depositional gap in panels A and B, whereas plotting of values mostly in the river field in panel (C)
It is apparent that irrespective of fan or fluvial system, the paleoslope values obtained from Indian Precambrian alluvial deposits always exceed the upper slope limit (0.007 m/m) of fluvial systems earmarked by Blair and McPherson (1994). Most studies related the continuous range of river gradient values from alluvial fan to the plains with higher sediment bypassing in absence of vegetation (Eriksson et al., 2008; Koykka, 2011a, 2011b; Sarkar et al., 2012). Indeed, it hindered the distinction between the fan and fluvial systems in the Precambrian based on paleoflow parameters and thus forced over-dependence on sedimentological criteria, including documentation of genetically related packages, known as architectural elements. Production of argillaceous sediment in aggressive greenhouse paleoenvironment and its local (temporary) accumulation has been cited as the root cause behind the high gradient of river systems (Eriksson et al., 2008; Mukhopadhyay et al., 2014). However, the logic seems not unequivocally tenable in absence of mud in most of the fluvial deposits and it is unlikely to have development of local features in fluvial systems worldwide during the Proterozoic time. In addition, documentation of slope data falling within the “natural depositional gap” from modern humid fans, Japan by Hasimoto et al. (2008) raised more questions on the unequivocal applicability of “depositional gap” hypothesis. Arguably, consideration of slope data from wet fan systems would have pushed down the lower limit of fan slope data to merge with the upper limit of fluvial slope value in the Blair and McPherson (1994) diagram, and a continuous range of slope values between modern alluvial fan and fluvial systems would have resulted. Many of the documented Proterozoic high gradient river systems may be reworked as fan systems in humid climates with enhanced fluvial processes. The Indian Proterozoic alluvial record is no different.
Further, a wide variation in the discharge data can be observed from the Indian record. Whereas some fluvial systems, namely Sonia Sandstone, Marwar Supergroup, Cave Temple arenite, Badami Group, Upper Rewa sandstone, Vindhyan Supergroup, and Ramdurg Formation, Bagalkot Group record high (in excess of 1,000 m3/s) to very high (in excess of 2000 m3/s to as high as ∼8,000 m3/s) discharge values, others, namely Banganapalle Formation, Kurnool basin, fluvial deposit, Ambani Group, Rehtikhol Formation, Singhora Group, Damdama Formation, Alwar Group, and Par Formation, Gwalior Group, record significantly low (∼600 m3/S or much less) discharge values. It may be worth noticing that medium to coarse-grained sandstone with thick, tabular bed geometry, large to very large-scale (meters-scale) bedforms having a near consistent, uniform paleocurrent motif is a common observation in fluvial deposits with high to very high discharge, whereas gravelly, pebbly, granular sandstones with small-scale bedforms (cms scale only) with exposure-scale lenticular bed geometry dominate the fluvial systems with low to very low discharge. It is argued that both perennial (with average high discharge) and ephemeral (low to very low average discharge) fluvial systems coexisted in Proterozoic time, as it is today.
5 Discussion
A general consensus regarding Precambrian fluvial systems is their multi-channel character with high to very high width, depth ratio in large braidplains, high bed-load, lack of bank stability in the absence of mud, enhanced rates of channel abandonment and reoccupation, and high discharge with flashy surface runoff. It is presumed that ephemeral braided rivers dominated a broader climatic range in the Precambrian in contrast to Phanerozoic-modern analogs (Tisgaard and Øxnevad, 1998). Documentation of perennial rivers is rare in the Precambrian record. Documentation of the non-vegetated meandering river system principally came from supposed fining-upward trends in channel deposits. Architectural elements such as small-scale crevasse splays, channels, levees, and flood plains that bear undoubted testimony to meandering planforms are under-represented in the pre-vegetation alluvial record because of poorly-stabilized point bars in the absence of enough mud supply. In this backdrop, documentation of 1) laterally accreted macroforms from heterolithic fluvial strata, and 2) overbank fine-grained deposits in significant thickness in some recent studies (Bose et al., 2012; Owen and Santos, 2014; Ielpi et al., 2015; Santos and Owen, 2016; Santos et al., 2017; Barkat et al., 2020) allowed workers to believe that sinuous, meandering channel systems used to comb floodplains, at least locally, in the Precambrian as well.
Following the general doctrine, most of the Indian Precambrian fluvial systems are described as braidplains with unequivocal ephemeral channels having a high to very high width, depth ratio. Documentation of deposits indicating alluvial fans or meandering streams is rare in comparison. Whereas limited descriptions of fan systems (mid to distal fan) can be traced from the Paleoproterozoic Dhanjori (Mazumdar and Sarkar, 2004), Par (Chakraborty and Paul, 2014), and Neoproterozoic Banganapalle Formations (Barkat et al., 2020), descriptions of distal sandy meandering planforms are confined to the Neoproterozoic Marwar Supergroup (Sonia and Girbakhar Formations) and Banganapalle Formation of the Kurnool Group. Amongst these, the Banganapalle Formation offers a rare opportunity for documentation of an entire range of Precambrian alluvial systems, varying from mid fan to distal meander plain, through a sandy braid plain in between. Though preservation bias may be a reason behind the rarity of fan records, (mis) interpretation of fluvially-reworked distal fans as braided fluvial deposits may be the other factor. A thorough reassessment is warranted in many Indian alluvial deposits in view of the lack of any slope gap in channel gradient between those of alluvial fans and river plains, as claimed by Blair and McPherson (1994). Depending on spatial disposition in the drainage basin (upstream/midstream/downstream), the discharge amount and duration of flow within a channel system depend on tectonics (physiography), climatic variation vis-à-vis water table position, or sea level. Surface runoff and groundwater reservoirs were the main contributors in Precambrian rivers all along their courses in absence of biogenic activity withdrawing groundwater hence, an increase in downstream discharge were inevitable in Precambrian rivers. Additionally, water table fluctuations in varied climatic conditions (dry, semi-arid, or arid) with occasional heavy seasonal rainfall dominated the water discharge pattern in river systems. Indian Precambrian rivers, irrespective of their age, register the same with a decline in ephemerality downstream. Contribution from tributaries, particularly in low-gradient downstream, also cannot be overruled in view of modern alluvial plains where tributaries contribute significant water and sediment for the construction of alluvial plain (Petts and Amoros, 1996; Bridge, 1997; Bruno et al., 2021). Lack of preservation, however, rarely provides the chance for documentation of the tributary's role in Precambrian fluvial systems.
Though most Indian Precambrian braided fluvial records are thin and with low to very low discharge values (≤1,000 m3/s), the thick (∼350 m) Rewa fluvial deposit records are unusually high (>8000 m3/s) discharge value with the presence of large-scale channel bedforms. A definite difference in the scale of the channel is inferred for the Rewa fluvial system that largely remained perennial; this may be due to the dominant role of climate. Since the majority of Indian alluvial records come from the initiation of respective basins, basement erosion vis-à-vis erosional base level falls tend to result in relatively thin alluvial fans and braid plain deposits either concomitant with a falling-stage or a lowstand in relative sea level. In contrast, the Rewa fluvial succession overlies a thick (∼200 m) argillaceous shelf succession of the Rewa shale (Bose et al., 2001) in a progradational motif and is modeled as a product correlative with sea-level highstand. A slow, steady rise in the base level possibly allowed the development and preservation of a thick fluvial succession in the Rewa succession.
Generally fixed meander planforms in Precambrian fluvial records are found confined either in the falling stage systems tract (FSST) and early lowstand systems tract (LST) when valleys are expected to be narrow and incised within the bedrock (Bose et al., 2001) or at the late stage of lowstand when the rise in relative sea-level may trigger stagnation in the lower reaches of the alluvial plain (Barkat et al., 2020). A combination involving low gradient and discharge of alluvial plain, adequate supply of fine detritus from the hinterland, and base profile rise at an enhanced rate provoked meander planform to develop during the Precambrian, but only at cases. Meander planforms described from India records viz. Marwar Group and Banganapalle Formation, Kurnool Group are identified as the result of the increasing tendency of ponding and mud settlement in the downstream end of the river valley with the rising of base profile in response to the rise in relative sea level.
Author Contributions
PPC, RB, and PPP are instrumental for fieldwork, collection of samples, data, and visualization of the manuscript. RB and PPC carried out manuscript writing, PPP, RB, and AS performed paleohydraulic estimations. Conceptualization of figures and drawing was jointly carried out by PPP, RB, and AS.
Conflict of Interest
The authors declare that the research was conducted in the absence of any commercial or financial relationships that could be construed as a potential conflict of interest.
Publisher’s Note
All claims expressed in this article are solely those of the authors and do not necessarily represent those of their affiliated organizations, or those of the publisher, the editors, and the reviewers. Any product that may be evaluated in this article, or claim that may be made by its manufacturer, is not guaranteed or endorsed by the publisher.
Acknowledgments
RB and PPP acknowledge financial help from the Council of Scientific and Industrial Research (CSIR) in form of fellowships. AS acknowledges UGC for fellowship. PPC acknowledges the Institute of Eminence (IOE), University of Delhi, for financial help in carrying out fieldworks.
Supplementary Material
The Supplementary Material for this article can be found online at: https://www.frontiersin.org/articles/10.3389/feart.2022.892717/full#supplementary-material
References
Allen, J. R. L. (1983). Gravel Overpassing on Humpback Bars Supplied with Mixed Sediment: Examples from the Lower Old Red Sandstone, Southern Britain. Sedimentology 30, 285–294. doi:10.1111/j.1365-3091.1983.tb00671.x
Barkat, R., Chakraborty, P. P., Saha, S., and Das, K. (2020). Alluvial Architecture, Paleohydrology and Provenance Tracking from the Neoproterozoic Banganapalle Formation, Kurnool Group, India: An Example of Continental Sedimentation before Land Plants. Precambrian Res. 350, 105930. doi:10.1016/j.precamres.2020.105930
Basu, A., and Bickford, M. E. (2015). An Alternate Perspective on the Opening and Closing of the Intracratonic Purana Basins in Peninsular India.
Benvenuti, M. (2003). Facies Analysis and Tectonic Significance of Lacustrine Fan-Deltaic Successions in the Pliocene-Pleistocene Mugello Basin, Central Italy. Sediment. Geol. 157, 197–234. doi:10.1016/S0037-0738(02)00234-8
Blair, T. C., and McPherson, J. G. (1994). Alluvial Fans and Their Natural Distinction from Rivers Based on Morphology, Hydraulic Processes, Sedimentary Processes, and Facies Assemblages. J. Sediment. Res. 64 (3a), 450–489. doi:10.1306/d4267dde-2b26-11d7-8648000102c1865d
Bose, P. K., and Chakraborty, P. P. (1994). Marine to Fluvial Transition: Proterozoic Upper Rewa Sandstone, Maihar, India. Sediment. Geol. 89, 285–302. doi:10.1016/0037-0738(94)90098-1
Bose, P. K., Eriksson, P. G., Sarkar, S., Wright, D. T., Samanta, P., Mukhopadhyay, S., et al. (2012). Sedimentation Patterns during the Precambrian: A Unique Record? Mar. Petroleum Geol. 33, 34–68. doi:10.1016/j.marpetgeo.2010.11.002
Bose, P. K., Sarkar, S., Chakrabarty, S., and Banerjee, S. (2001). Overview of the Meso- to Neoproterozoic Evolution of the Vindhyan Basin, Central India. Sediment. Geol. 141-142 (141), 395–419. doi:10.1016/s0037-0738(01)00084-7
Bridge, J. S. (1997). Thickness of Sets of Cross Strata and Planar Strata as a Function of Formative Bed-Wave Geometry and Migration, and Aggradation Rate. Geol 25, 971–974. doi:10.1130/0091-7613(1997)025<0971:tosocs>2.3.co;2
Bruno, H., Heilbron, M., Strachan, R., Fowler, M., de Morisson Valeriano, C., Bersan, S., et al. (2021). Earth's New Tectonic Regime at the Dawn of the Paleoproterozoic: Hf Isotope Evidence for Efficient Crustal Growth and Reworking in the São Francisco Craton, Brazil. Geology 49, 1214–1219. doi:10.1130/G49024.1
Chakraborti, R., Basu, A. R., and Chakraborti, A. (2007). Trace Element and Nd-Isotopic Evidence for Sediment Sources in the Mid-proterozoic Vindhyan Basin, Central India. Precambrian Res. 159 (3-4), 260–274.
Chakraborty, P. P., and Paul, P. (2014). Depositional Character of a Dry-Climate Alluvial Fan System from Palaeoproterozoic Rift Setting Using Facies Architecture and Palaeohydraulics: Example from the Par Formation, Gwalior Group, Central India. J. Asian Earth Sci. 91, 298–315. doi:10.1016/j.jseaes.2013.09.019
Chakraborty, P. P., Saha, S., and Das, K. (2017). Record of Continental to Marine Transition from the Mesoproterozoic Ampani Basin, Central India: An Exercise of Process-Based Sedimentology in a Structurally Deformed Basin. J. Asian Earth Sci. 143, 122–140. doi:10.1016/j.jseaes.2017.04.015
Chakraborty, P. P., Sarkar, A., Das, K., and Das, P. (2009). Alluvial Fan to Storm-Dominated Shelf Transition in the Mesoproterozoic Singhora Group, Chattisgarh Supergroup, Central India. Precambrian Res. 170, 88–106. doi:10.1016/j.precamres.2008.12.002
Chakraborty, P. P., Tandon, S. K., Roy, S. B., Saha, S., and Paul, P. P. (2020). Proterozoic Sedimentary Basins of India. Springer GeologySpringer, 145–177. doi:10.1007/978-3-030-15989-4_4Proterozoic Sedimentary Basins of India
Chaudhuri, A. K., Saha, D., Deb, G. K., Patranabis Deb, S., Kanti Mukherjee, M., and Ghosh, G. (2002). The Purana Basins of Southern Cratonic Province of India - A Case for Mesoproterozoic Fossil Rifts. Gondwana Res. 5, 23–33. doi:10.1016/S1342-937X(05)70884-4
Cotter, E. (1978). The Evolution of Fluvial Style, with Special Reference to the Central Appalachian Paleozoic.
Dam, G., and Andreasen, F. (1990). High-energy Ephemeral Stream Deltas; an Example from the Upper Silurian Holmestrand Formation of the Oslo Region, Norway. Sediment. Geol. 66, 197–225. doi:10.1016/0037-0738(90)90060-7
Das, D. P. (1992). Lithostratigraphy and Sedimentation of Chattisgarh Basin. Indian Min. 46, 271–288.
Das, P. (2014). Facies Model, Geochronology and Sequence Analysis of the Singhora Group of Rocks:implications to Age and Basinal Forcings in Early History of the Chhattisgarh Basin, Central India. Higashi-Hiroshima, Japan: Unpublished Ph.D thesis, Hiroshima University, 155pp.
Deynoux, M., Çiner, A., Monod, O., Karabıyıkoglu, M., Manatschal, G., and Tuzcu, S. (2005). Facies Architecture and Depositional Evolution of Alluvial Fan to Fan-Delta Complexes in the Tectonically Active Miocene Köprüçay Basin, Isparta Angle. Turk. Sediment. Geol. 173 (1-4), 315–343. doi:10.1016/j.sedgeo.2003.12.013
Els, B. G. (1990). Determination of Some Palaeohydraulic Parameters for a Fluvial Witwatersrand Succession. South Afr. J. Geol. 93, 531–537.
Eriksson, P. G., Banerjee, S., Catuneanu, O., Corcoran, P. L., Eriksson, K. A., Hiatt, E. E., et al. (2013). Secular Changes in Sedimentation Systems and Sequence Stratigraphy. Gondwana Res. 24, 468–489. doi:10.1016/J.GR.2012.09.008
Eriksson, P. G., Bumby, A. J., Brümer, J. J., and van der Neut, M. (2006). Precambrian Fluvial Deposits: Enigmatic Palaeohydrological Data from the C. 2-1.9 Ga Waterberg Group, South Africa. Sediment. Geol. 190, 25–46. doi:10.1016/j.sedgeo.2006.05.003
Eriksson, P. G., Condie, K. C., Tirsgaard, H., Mueller, W. U., Altermann, W., Miall, A. D., et al. (1998). Precambrian Clastic Sedimentation Systems. Sediment. Geol. 120, 5–53. doi:10.1016/S0037-0738(98)00026-8
Eriksson, P. G., Reczko, B. F. F., Jaco Boshoff, A., Schreiber, U. M., Van der Neut, M., and Snyman, C. P. (1995). Architectural Elements from Lower Proterozoic Braid-Delta and High-Energy Tidal Flat Deposits in the Magaliesberg Formation, Transvaal Supergroup, South Africa. Sediment. Geol. 97, 99–117. doi:10.1016/0037-0738(95)00004-R
Eriksson, P., Long, D., Bumby, A., Eriksson, K., Simpson, E., Catuneanu, O., et al. (2008). Palaeohydrological Data from the C. 2.0 to 1.8 Ga Waterberg Group, South Africa: Discussion of a Possibly Unique Palaeoproterozoic Fluvial Style. South Afr. J. Geol. 111, 281–304. doi:10.2113/gssajg.111.2-3.281
Hartley, A. J., Weissmann, G. S., Nichols, G. J., and Warwick, G. L. (2010). Large Distributive Fluvial Systems: Characteristics, Distribution, and Controls on Development. J. Sediment. Res. 80, 167–183. doi:10.2110/jsr.2010.016
Hashimoto, A., Oguchi, T., Hayakawa, Y., Lin, Z., Saito, K., and Wasklewicz, T. A. (2008). GIS Analysis of Depositional Slope Change at Alluvial-Fan Toes in Japan and the American Southwest. Geomorphology 100, 120–130. doi:10.1016/j.geomorph.2007.10.027
Ielpi, A., Rainbird, R. H., Ventra, D., and Ghinassi, M. (2017). Morphometric Convergence between Proterozoic and Post-vegetation Rivers. Nat. Commun. 8 (1), 15250–15258. doi:10.1038/ncomms15250
Ielpi, A., Gibling, M. R., Bashforth, A. R., and Dennar, C. I. (2015). Impact of Vegetation on Early Pennsylvanian Fluvial Channels: Insight from the Joggins Formation of Atlantic Canada. J. Sedi. Res. 85, 999–1018. doi:10.2110/jsr.2015.50
Ito, M., Matsukawa, M., Saito, T., and Nichols, D. J. (2006). Facies Architecture and Paleohydrology of a Synrift Succession in the Early Cretaceous Choyr Basin, Southeastern Mongolia. Cretac. Res. 27, 226–240. doi:10.1016/j.cretres.2005.11.005
Jones, S. J., Frostick, L. E., and Astin, T. R. (2001). Braided Stream and Flood Plain Architecture: the Rio Vero Formation, Spanish Pyrenees. Sediment. Geol. 139, 229–260. doi:10.1016/S0037-0738(00)00165-2
Kasse, C., Van Balen, R. T., Bohncke, S. J. P., Wallinga, J., and Vreugdenhil, M. (2017). Climate and Base-Level Controlled Fluvial System Change and Incision during the Last Glacial-Interglacial Transition, Roer River, the Netherlands - Western Germany. Neth. J. Geosciences 96, 71–92. doi:10.1017/NJG.2016.50
Köykkä, J. (2011a). Precambrian Alluvial Fan and Braidplain Sedimentation Patterns: Example from the Mesoproterozoic Rjukan Rift Basin, Southern Norway. Sediment. Geol. 234, 89–108. doi:10.1016/j.sedgeo.2010.12.004
Köykkä, J. (2011b). The Sedimentation and Paleohydrology of the Mesoproterozoic Stream Deposits in a Strike-Slip Basin (Svinsaga Formation), Telemark, Southern Norway. Sediment. Geol. 236, 239–255. doi:10.1016/j.sedgeo.2011.01.010
Leclair, S. F., and Bridge, J. S. (2001a). Quantitative Interpretation of Sedimentary Structures Formed by River Dunes. J. Sediment. Res. 71, 713–716. doi:10.1306/2DC40962-0E47-11D7-8643000102C1865D
Leclair, S. F., and Bridge, J. S. (2001b). Quantitative Interpretation of Sedimentary Structures Formed by River Dunes. J. Sediment. Res. 71, 713–716. doi:10.1306/2DC40962-0E47-11D7-8643000102C1865D
Long, D. G. F. (2011). Architecture and Depositional Style of Fluvial Systems before Land PlantsA Comparison of Precambrian, Early Paleozoic, and Modern River Deposits. river rock Rec. Preserv. Fluv. sediments their Subseq. Interpret. 97, 37–61. doi:10.2110/sepmsp.097.037
Long, D. G. F. (2006). Architecture of Pre-vegetation Sandy-Braided Perennial and Ephemeral River Deposits in the Paleoproterozoic Athabasca Group, Northern Saskatchewan, Canada as Indicators of Precambrian Fluvial Style. Sediment. Geol. 190, 71–95. doi:10.1016/j.sedgeo.2006.05.006
Long, D. G. F. (2002). Aspects of Late Palaeoproterozoic Fluvial Style: the Uairén Formation, Roraima Supergroup, Venezuela. Precambrian Sediment. Environ. A Mod. Approach Anc. Depos. Syst., 323–338.
Long, D. G. F., and Donaldson, J. A. (2005). Modern and Ancient Clastic Sedimentary Environments: A Collection of Field Photographs, 1. Ontario, Canada: Geological Association of Canada CSRG-CD. 1-897095-04-X (968. p, html).
Long, D. G. F. (2021). Trickling Down the Paleoslope: an Empirical Approach to Paleohydrology. Earth-Science Rev. 220, 103740. doi:10.1016/J.EARSCIREV.2021.103740
Long, D. (2004). The Tectonostatigraphic Evolution of the Huronian Basement and the Subsequent Basin Fill: Geological Constraints on Impact Models of the Sudbury Event. Precambrian Res. 129, 203–223. doi:10.1016/J.PRECAMRES.2003.10.003
Major, J. J. (1997). Depositional Processes in Large‐Scale Debris‐Flow Experiments. J. Geol. 105, 345–366. doi:10.1086/515930
Martins-Neto, M. A. (1994). Braidplain sedimentation in a Proterozoic rift basin: the São João da Chapada Formation, southeastern Brazil. Sediment. Geol. 89, 219–239. doi:10.1016/0037-0738(94)90095-7
Mazumder, R., and Sarkar, S. (2004). Sedimentation History of the Palaeoproterozoic Dhanjori Formation, Singhbhum, Eastern India. Precambrian Res. 130, 267–287. doi:10.1016/j.precamres.2003.12.005
McMahon, W. J., and Davies, N. S. (2020). Physical and Biological Functioning in Proterozoic Rivers: Evidence from the Archetypal Pre-vegetation Alluvium of the Torridon Group, NW Scotland. Scott. J. Geol. 56, 1–29. doi:10.1144/SJG2019-013
McPherson, J. G., Shanmugam, G., and Moiola, R. J. (1987). Fan-deltas and Braid Deltas: Varieties of Coarse-Grained Deltas. Geol. Soc. Am. Bull. 99, 331–340. doi:10.1130/0016-7606(1987)99<331:fabdvo>2.0.co;2
Meert, J. G., and Pandit, M. K. (2015). Chapter 3 the Archaean and Proterozoic History of Peninsular India: Tectonic Framework for Precambrian Sedimentary Basins in India. Geol. Soc. Lond. Memoirs 43, 29–54. doi:10.1144/M43.3
Meert, J. G., Pandit, M. K., and Kamenov, G. D. (2013). Further Geochronological and Paleomagnetic Constraints on Malani (And Pre-malani) Magmatism in NW India. Tectonophysics 608, 1254–1267. doi:10.1016/j.tecto.2013.06.019
Meert, J. G., Pandit, M. K., Pradhan, V. R., Banks, J., Sirianni, R., Stroud, M., et al. (2010). Precambrian Crustal Evolution of Peninsular India: A 3.0 Billion Year Odyssey. J. Asian Earth Sci. 39, 483–515. doi:10.1016/j.jseaes.2010.04.026
Miall, A. D. (1977). A Review of the Braided-River Depositional Environment. Earth-Science Rev. 13, 1–62. doi:10.1016/0012-8252(77)90055-1
Miall, A. D. (1985). Architectural-element Analysis: A New Method of Facies Analysis Applied to Fluvial Deposits. Earth-Science Rev. 22, 261–308. doi:10.1016/0012-8252(85)90001-7
Miall, A. D. (1983). “Basin Analysis of Fluvial Sediments,” in Modern and Ancient Fluvial Systems (Oxford, UK: Blackwell Publishing Ltd.), 277–286. doi:10.1002/9781444303773.ch22
Miall, A. D. (1988). Facies Architecture in Clastic Sedimentary Basins, 67–81. doi:10.1007/978-1-4612-3788-4_4
Miall, A. D. (1978). Lithofacies Types and Vertical Profile Models in Braided River Deposits: a Summary. Fluv. Sedimentol. 5, 597–600. Available at: http://archives.datapages.com/data/dgs/005/005001/597_cspgsp0050597.htm (Accessed July 16, 2021).
Miall, A. D. (1993). The Architecture of Fluvial-Deltaic Sequences in the Upper Mesaverde Group (Upper Cretaceous), Book Cliffs, Utah. Geol. Soc. Lond. Spec. Publ. 75, 305–332. doi:10.1144/gsl.sp.1993.075.01.19
Mukhopadhyay, S., Choudhuri, A., Samanta, P., Sarkar, S., and Bose, P. K. (2014). Were the Hydraulic Parameters of Precambrian Rivers Different? J. Asian Earth Sci. 91, 289–297. doi:10.1016/j.jseaes.2013.07.042
Mukhopadhyay, S. (2012). Evolutionary History of Proterozoic Fluvial Basins within Bagalkot Group and Rewa Formation: Facies, Palaeogeography and Stratigraphic Architecture. Kolkata, India: Jadavpur Univ. Unpubl. Ph. D. Thesis, 255p.
Owen, G., and Santos, M. G. M. (2014). Soft-sediment Deformation in a Pre-vegetation River System: The Neoproterozoic Torridonian of NW Scotland. Proc. Geologists' Assoc. 125, 511–523. doi:10.1016/j.pgeola.2014.08.005
Patranabis-Deb, S., and Chaudhuri, A. K. (2008). Sequence Evolution in the Eastern Chhattisgarh Basin: Constraints on Correlation and Stratigraphic Analysis.
Petts, G. E., and Amoros, C. (1996). “The Fluvial Hydrosystem,” in The Fluvial Hydrosystems (Springer), 1–12. doi:10.1007/978-94-009-1491-9_1
Pradhan, V. R., Meert, J. G., Pandit, M. K., Kamenov, G., Gregory, L. C., and Malone, S. (2010). India’s Changing Place in Global Proterozoic Reconstructions: New Geochronologic Constraints on Key Paleomagnetic Poles from the Dharwar and Aravalli/Bundelkhand Cratons. J. Geodyn. doi:10.1016/j.jog/2009.11.08
Rust, B. R., and Koster, E. H. (1984). Coarse Clastic Deposits. Facies Model. Geosci. Can. Repr. Ser. 1, 53–69.
Samanta, P., Mukhopadhyay, S., Sen, A., Ghosh, N., and Bumby, A. (2022). Precambrian Fans on Opposite Margins of an Intracratonic Rift Basin; Palaeogeography, Palaeoclimate and Provenance: Neoproterozoic Badami Group, Karnataka, India. Sediment. Geol. 428, 106050. doi:10.1016/J.SEDGEO.2021.106050
Samanta, P. (2009). Proterozoic Siliciclastic Sedimentation and Sequence Building: Possible Role of Microbial Mat: Sonia Sandstone. Rajasthan, IndiaIndia: Jadavpur UniversityUnpublished Ph.D. thesis, 303.
Santos, M. G. M., Almeida, R. P., Godinho, L. P. S., Marconato, A., and Mountney, N. P. (2014). Distinct Styles of Fluvial Deposition in a Cambrian Rift Basin. Sedimentology 61, 881–914. doi:10.1111/SED.12074
Santos, M. G. M., Mountney, N. P., and Peakall, J. (2017). Tectonic and Environmental Controls on Palaeozoic Fluvial Environments: Reassessing the Impacts of Early Land Plants on Sedimentation. J. Geol. Soc. 1174 (3), 393–404. doi:10.1144/jgs2016-063
Santos, M. G. M., and Owen, G. (2016). Heterolithic Meandering-Channel Deposits from the Neoproterozoic of NW Scotland: Implications for Palaeogeographic Reconstructions of Precambrian Sedimentary Environments. Precambrian Res. 272, 226–243. doi:10.1016/J.PRECAMRES.2015.11.003
Sarkar, S., Samanta, P., Mukhopadhyay, S., and Bose, P. K. (2012). Stratigraphic Architecture of the Sonia Fluvial Interval, India in its Precambrian Context. Precambrian Res. 214-215, 210–226. doi:10.1016/j.precamres.2012.01.001
Sharma, R. (2021). Depositional Modelling, Stratigraphic Reappraisal and Geochemical Nuances during Late Neoproterozoic-Cambrian Transition from the Girbakhar Formation and Bilara Group of Rocks, Marwar Supergroup. Rajasthan, IndiaIndia: Unpublished Ph.D thesis, University of Delhi.
Singh, A. P., and Mishra, D. C. (2002). Tectonosedimentary Evolution of Cuddapah Basin and Eastern Ghats Mobile Belt (India) as Proterozoic Collision: Gravity, Seismic and Geodynamic Constraints. J. Geodyn. 33, 249–267. doi:10.1016/S0264-3707(01)00066-7
Sønderholm, M., and Tirsgaard, H. (1993). Lithostratigraphic Framework of the Upper Proterozoic Eleonore Bay Supergroup of East and North-East Greenland. Bull. Grønl. Geol. Unders. 167, 1–38.
Tirsgaard, H., and Øxnevad, I. E. i. (1998). Preservation of Pre-vegetational Mixed Fluvio-Aeolian Deposits in a Humid Climatic Setting: an Example from the Middle Proterozoic Eriksfjord Formation, Southwest Greenland. Sediment. Geol. 120, 295–317. doi:10.1016/S0037-0738(98)00037-2
Todd, S. P. (1989). Stream-driven, High-Density Gravelly Traction Carpets: Possible Deposits in the Trabeg Conglomerate Formation, SW Ireland and Some Theoretical Considerations of Their Origin. Sedimentology 36, 513–530. doi:10.1111/j.1365-3091.1989.tb02083.x
Vallance, J. W. (2000). “Lahars,” in Encyclopedia of Volcanoes. Editors H. Sigurdsson, B. F. Houghton, R. S. Mcnutt, H. Rymer, and J. Stix (SanDiego: Academic Press), 601–615.
Van der Neut, M., and Eriksson, P. G. (1999). Palaeohydrological Parameters of a Proterozoic Braided Fluvial System (Wilgerivier Formation, Waterberg Group, South Africa) Compared with a Phanerozoic Example. Pretoria, South Africa: Fluv. Sedimentol. VI, 381.
Vandenberghe, J. (2003). Climate Forcing of Fluvial System Development: an Evolution of Ideas. Quat. Sci. Rev. 22, 2053–2060. doi:10.1016/S0277-3791(03)00213-0
Verma, A., and Shukla, U. K. (2015). Deposition of the Upper Rewa Sandstone Formation of Proterozoic Rewa Group of the Vindhyan Basin, M.P., India: A Reappraisal. J. Geol. Soc. India 86, 421–437. doi:10.1007/s12594-015-0330-4
Verma, A., and Shukla, U. K. (2020). Heterolithic Lower Rewa Sandstone of the Neoproterozoic Rewa Group, Vindhyan Basin, U. P., India: An Example of Tidal Point Bar. Precambrian Res. 350, 105932. doi:10.1016/j.precamres.2020.105932
Keywords: Precambrian river system, scree cones, alluvial fan, braid plains, paleohydraulics, Indian Peninsula
Citation: Chakraborty PP, Bailwal R, Paul PP and Sharma A (2022) Alluvial Sedimentary Records in Indian Precambrian Basins: Implications Toward Unique Precambrian Sedimentary Environment?. Front. Earth Sci. 10:892717. doi: 10.3389/feart.2022.892717
Received: 09 March 2022; Accepted: 27 May 2022;
Published: 11 July 2022.
Edited by:
Mauricio G.M. Santos, Federal University of ABC, BrazilReviewed by:
Pradip Samanta, University of North Bengal, IndiaNarayana A. C, University of Hyderabad, India
Copyright © 2022 Chakraborty, Bailwal, Paul and Sharma. This is an open-access article distributed under the terms of the Creative Commons Attribution License (CC BY). The use, distribution or reproduction in other forums is permitted, provided the original author(s) and the copyright owner(s) are credited and that the original publication in this journal is cited, in accordance with accepted academic practice. No use, distribution or reproduction is permitted which does not comply with these terms.
*Correspondence: Partha Pratim Chakraborty, cGFydGhhZ2VvbG9neUBnbWFpbC5jb20=