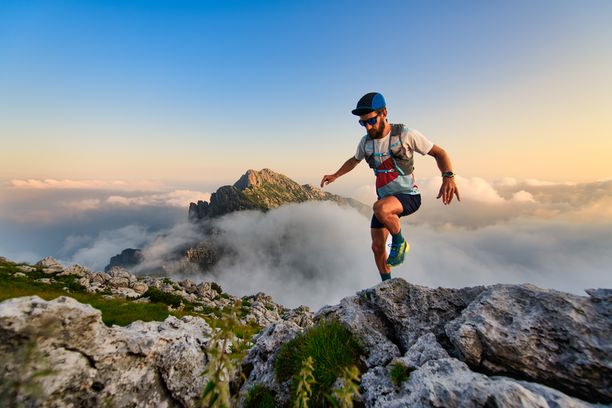
94% of researchers rate our articles as excellent or good
Learn more about the work of our research integrity team to safeguard the quality of each article we publish.
Find out more
ORIGINAL RESEARCH article
Front. Earth Sci., 19 July 2022
Sec. Geochemistry
Volume 10 - 2022 | https://doi.org/10.3389/feart.2022.891252
This article is part of the Research TopicIsotopic Geochemistry of Natural GasView all 14 articles
The Middle Triassic Leikoupo Formation is largely extended through the Sichuan Basin, SW China. In this formation, several commercially exploited gas reservoirs have been discovered in western and central parts of the basin. Due to the complicated geochemical signatures of the natural gases in these reservoirs, there are contrasted interpretations about their sources, which hamper the evaluation and exploration for new gas resources in the area. To obtain complete understanding of the natural gas sources, the Leikoupo Formation gas reservoirs discovered so far in the Zhongba, Yuanba, Longgang, and Moxi gas fields were selected as the research object of this study. The genetic types and sources of the natural gases in the Leikoupo Formation are discussed based on gas geochemistry combined with their geological background. The natural gas in the top members of the Leikoupo Formation (T2l4 or T2l3) is partially originated from a humic kerogen contained in the source rocks from the overlying Upper Triassic Xujiahe Formation and from sapropel kerogen from the source rocks of the Leikoupo Formation itself. The natural gas of T2l1 member in the lower part of the Leikoupo Formation is mainly sapropel-type probably from the source rock of the Permian Wujiaping Formation, where the Permian Longtan Formation undergoes a phase change into the Wujiaping Formation The reversed δ13C1 and δ13C2 trend in the Leikoupo Formation of the Yuanba gas field is due to the more sapropelic source rocks and higher degree of maturity.
The Middle Triassic Leikoupo Formation is the latest set of marine strata developed in the Sichuan Basin, China, which is not the main strata for oil and gas exploration, and the exploration and research conducted for the Leikoupo Formation is relatively low. Back in the 1970s, small-scale gas pools were discovered in this set of strata, for example, the third member (T2l3) of the Leikoupo Formation in the Zhongba gas field in NW Sichuan (Qin et al., 2007), and the first member (T2l1) of the Leikoupo Formation in the Moxi gas field in central Sichuan. With the increasing natural gas exploration, commercial gas reservoirs have been found in the fourth section (T2l4) of the Leikoupo Formation in the Longgang and Yuanba gas fields successively in this century, showing that the Leikoupo Formation has good potential for gas exploration. Since the Leikoupo Formation is dominated by evaporative platform facies as a whole, and evaporites mainly composed of gypsum and dolomite are deposited, it is doubtful whether the Leikoupo Formation formed in such an environment can develop effective source rocks. In addition, the geochemical characteristics of natural gas in the Leikoupo Formation are complex, and the types of natural gas origin are diverse. It is difficult to identify the gas sources, and there are different opinions on the source of natural gas. For example, in the Leikoupo Formation in the Zhongba gas field in western Sichuan, it was believed that all natural gas came from the underlying Permian hydrocarbon source rocks (Dai, 1980). It was also believed that the gas came from biogenic limestone and mudstone of the Leikoupo Formation (Li, 1993; Qin et al., 2007). Some studies suggested that the gas came from Permian sapropel source rock, mixed with a small amount of gas from coal measures (Liao et al., 2013), and some even thought that the gas mainly came from overlying coal measures (Wang, et al., 1989; Zheng, et al., 1990). In the central Sichuan Basin, recoverable gas reservoirs have been found in both the T2l4 and T2l1 members of the Leikoupo Formation, but the gas geochemical characteristics differ significantly between the reservoirs, and the gas source is highly controversial (Huang, 2014; Liu, et al., 2014; Zhou, et al., 2015; Liu, et al., 2019). Even within the T2l4 member, the gas’ geochemical signatures are highly variable; the gas indicated to be not only humic-type gas but also sapropel-type, and it is difficult to explain the source of gas.
In this study, the gas reservoirs of the Leikoupo Formation in the Zhongba, Moxi, Longgang, and Yuanba gas fields that have been discovered so far are systematically analyzed using natural gas geochemical methods. The source of natural gas has been elucidated based on the comparative study of Triassic and Permian natural gas types and their source rocks, which in turn provides a basis for natural gas exploration in the Leikoupo Formation.
The Indo-Chinese early episodic movement at the end of the Middle Triassic made the central Sichuan area rise to land, the seawater withdrew, and the large inland lake basin began to appear, which was an important turning period from marine sedimentation to lacustrine sedimentation in the Sichuan Basin as it ended the deposition of the carbonate platform in Sichuan. The uplift of the Sichuan Basin suffered from denudation, forming the erosion surface at the top of the Middle Triassic Leikoupo Formation. Since the Late Triassic, it has received continental deposits and developed multiple sets of coal-measure source rocks and multiple sets of interbedded sedimentary assemblages of sandstones.
There are many oil- and gas-bearing layers in the Sichuan Basin. This study focuses on the gas reservoir in the Leikoupo Formation. The related layers span the Triassic and the upper Permian. The strata from top to bottom are the Upper Triassic Xujiahe Formation (T3x), Middle Triassic Leikoupo Formation (T21), Lower Triassic Jialingjiang Formation (T1J) and Feixianguan Formation (T1f), and Upper Permian Changxing Formation (P3ch) and Longtan Formation (P31) (Figure 1).
FIGURE 1. Distribution of main gas fields and the Permian–Triassic petroleum system in the central and western Sichuan Basin.
The Xujiahe Formation is a fluvial–swamp–lacustrine deposit developed in a humid environment (Luo, 1983, 2011; Li, 2011). Several sets of coal-measure source rocks and sandstone reservoirs are developed. The coal-measure source rocks and sandstones were stacked on top of each other. The Xujiahe Formation is subdivided into T3x1 to T3x6 members from bottom to top, in which T3x1, T3x3, and T3x5 are mainly coal-measure sources, and the organic matter is mainly kerogen-Ⅲ type, which is a gas dominated source rock. The T3x2, T3x4, and T3x6 members are dominated by sandstone (Wang, et al., 1997; Yang, et al., 2005; Li et al., 2010) and are good reservoirs for gas storage.
The top of the Leikoupo Formation (T2l) suffered from dissolution and contact with the overlying Xujiahe Formation in parallel unconformity. The T2l is mainly composed of grayish-white medium-thick microcrystalline dolomite, argillaceous dolomite, and gray dolomite, with light gray gypsum and thin gray-black shale, which is a high-quality caprock in the whole area, in which the gray-black shale can be used as a hydrocarbon source rock. It is subdivided into T2l1 to T2l4 members from bottom to top, among which the natural gas reservoirs have been discovered in T2l1, T2l3, and T2l4 members.
The Jialingjiang Formation (T1j) is composed of gray micrite limestone and dolomite interbedded with a gypsum layer, dolomitic gypsum, and argillaceous dolomite, which is not only a high-quality caprock in the whole area but also a gas reservoir in the Jialingjiang Formation. The top of the Feixianguan Formation (T1f) consists of mudstone, dolomitic mudstone, gypsum, dolomicrite, micrite, and marlstone. The middle and lower part of the Feixianguan Formation is karst oolitic dolomite and limestone, which has good porosity and permeability and a regional high-quality reservoir rock (Wen, et al., 2012; Zhang, et al., 2013; Zhu, et al., 2013).
The Upper Permian (P3) includes the Changxing Formation (P3ch) and the Longtan Formation (P31) from top to bottom. The Changxing Formation is mainly composed of bioclastic micrite limestone, reef limestone, and dolomite, which is also an important reservoir in central Sichuan (Peng, et al., 2011). The Longtan Formation is mainly composed of marine–continental transitional coal measures and marine biological limestone, which is an important regional hydrocarbon source rock series and the main source rock of Changxing and Feixianguan gas reservoirs such as Longgang and Yuanba gas fields. It should be pointed out that the coal-measure source rocks of the Longtan Formation have undergone phase transformation in some areas and become marine source rocks of the Wujiaping Formation (P3w), and the hydrocarbon-generating material changed from humic to sapropel-type.
The Leikoupo Formation gas reservoirs include T2l1, T2l3, and T2l4 members. A weathering crust and a karst reservoir are developed at the top of the Leikoupo Formation, and their distribution are controlled by lithology and paleogeomorphology of exposed strata at the top (Bian, et al., 2019). The T2l3 gas reservoir of the Leikoupo Formation in the Zhongba gas field is distributed in the dolomite layer, which is an anticline reservoir type. The top is in an unconformable contact with the overlying Xujiahe Formation, missing the T2l4 member (Zeng, et al., 2007). The top surface of the Leikoupo Formation in the Yuanba gas field was affected by denudation and karstification, and a dolomite karst reservoir was formed in the T2l4 member. The reservoir is controlled by paleogeomorphology and has strong lateral heterogeneity (Fan, 2014), there is no unified gas–water interface, and natural gas tends to be distributed in structural highs (Liu, et al., 2019); therefore, the reservoir is a karst-controlled structural and lithologic gas reservoir. The reservoir of the T2l4 in the Longgang gas field is related to Indosinian denudation and karstification (Yang, et al., 2014), and the natural gas between different wells in the lateral direction is quite different, with obvious heterogeneity, which is a structural and lithologic gas reservoir. The T2l1 gas reservoir is currently mainly discovered in the Moxi gas field. This reservoir has a large trap area and is an anticline porous carbonate gas reservoir with a high degree of fullness. It has a unified gas–water interface and is a monolithic gas reservoir.
The reason why it is difficult to judge the source of natural gas in the Leikoupo Formation is that there are several sets of different types of source rocks developed in the Leikoupo Formation and it’s overly and underlying strata, which may provide a gas source for the Leikoupo gas reservoirs under appropriate geological conditions. This increases the difficulty for identification of Leikoupo gas sources.
The natural gas generated by coal-measure source rocks of the Xujiahe Formation has formed many large- and medium-sized gas fields in the Sichuan Basin. The source rocks directly cover the unconformities of the Leikoupo Formation and might partly provide gas for the karst reservoirs of the Leikoupo Formation.
The Jialingjiang, Feixianguan, and Changxing formations under the Leikoupo Formation did not develop source rocks. Under the Changxing Formation, the marine–terrestrial transitional coal-measure source rocks developed in the Formation had acted as a source rock for many large gas reservoirs in Changxing and Feixianguan formations of Longgang, Yuanba, and Puguang gas fields (Hao, et al., 2008; Wu et al., 2015; Qin, et al., 2016a; Deng, et al., 2018). If conditions permitted, it might also be possible to indirectly supply gas sources to the Leikoupo gas reservoir through the Changxing, Feixianguan, and Jialingjiang formations.
The Leikoupo Formation was formed in a salinized evaporation environment. Many researchers questioned that such an environment could form high-quality source rocks. Theoretically, such a saline environment has weak hydrodynamics, limited seawater circulation, high salinity, and alternating deposition of gypsum salt and carbonate rocks, which is conducive to the preservation of organic matter, and some of the strata can develop high-quality source rocks. Early studies believed that the Leikoupo Formation in the western Sichuan Basin was an algae-rich carbonate rock deposited in deep-water lagoon facies on a confined-evaporative platform and had favorable conditions for forming the main source rock of large- and medium-sized gas fields (Xu et al., 2013). The gypsum-bearing carbonate samples of the Leikoupo Formation in the western Sichuan Basin have relatively high TOC, and the gypsum-dolomite flat and gypsum-bearing lagoon facies with more evaporative platform facies are deposited, which are favorable sedimentary facies belts and lithologic assemblages for the development of high-quality source rocks (Yang, 2016; Wang, et al., 2018b). Other studies have concluded that the TOC of organic-rich shale in the Leikoupo Formation in the Sichuan Basin is 0.49%–1.08%, with an average of 0.77%, and the Ro values are 2.36%–2.40% (Sun, et al., 2021). According to the evaluation standard of a highly mature source rock (Dai, et al., 2008), it is evaluated as a high-quality source rock. The source rock was formed in a dry heat, salt water, and anoxic environment. Drilling revealed that the actual drilling thickness of the Leikoupo Formation in the central and northern Sichuan area is 960 m, of which the T2l3 member is the most likely the source rock with a thickness of 530 m, and the lithology is argillaceous limestone, micrite limestone, organic-rich shale rock, salt rock, and gypsum. The T2l1, T2l2, and T2l4 members are dominated by dolomite and gypsum rock, and no source rocks have been found (Sun, et al., 2021).
In fact, it is not uncommon to form source rocks in evaporative environments. In both the Bohai Bay Basin and the tertiary of the Qaidam Basin, the symbiotic phenomenon of evaporite and the source rock had been found (Jin, et al., 2006). In the Junggar Basin (Yu, et al., 2018), Polish Basin (Krzywiec, et al., 2017), Mexico Basin (Xie, et al., 2019), and West Texas–New Mexico (Hussain, et al., 1991), source rocks had been found in an evaporative environment.
Natural gas samples were taken from the Xujiahe, Leikoupo, Jialingjiang, and Changxing formations in the central Sichuan Basin. To eliminate the interferences of external factors and ensure representativeness of the natural gas in these reservoirs, all samples were collected from wells with long-term normal production without application of de-foaming or any other chemical agents recently.
Gas samples from the reservoirs were taken at the wellheads by using double valve steel cylinders. To take such samples, the pressure gauge was dismantled before connecting the steel cylinder with the sampling tubing. Prior to taking samples, wellhead natural gas was used to flush the steel cylinder thoroughly for about 3 min. The sampling steel cylinder was then filled with natural gas equilibrated to the wellhead pressure.
Natural gas compositions were determined using an Agilent 6890N gas chromatograph (GC) with He and N2 as the carrier gases. Double thermal conductivity detectors (TCD) and a 30 m × 0.25 mm × 0.25 μm quartz capillary column were used. The inlet temperature was 150 °C, and the TCD temperature was 200 °C. The initial oven temperature was maintained at 40 °C for 7.5 min isothermally, then rose from 40 °C to 90 °C at 15 °C/min, and finally rose from 90 °C to 180 °C at 6 °C/min.
An on-line analysis was conducted for the measurement of carbon isotopic compositions with a MAT 253 gas isotopic mass spectrometer. Natural gas samples were separated to methane, ethane, propane, butane, and CO2 using the chromatography column of an SRI 8610C gas chromatograph, which were then transferred into a combustion furnace by the carrier gas (He) and oxidized into CO2 by CuO at 850°C. All of the converted species were transferred by the carrier gas (He) into MS to measure the isotopic compositions. A dual inlet analysis was performed with the international measurement standard of NBS-19 CO2 (δ13CVPDB=1.95 ± 0.04‰, International Atomic Energy Agency, 1995), and the stable carbon isotopic values were reported in the δ notation in per mil (‰) relative to the Peedee belemnite standard (VPDB). Reproducibility and accuracy were estimated to be ± 0.2‰ with respect to the VPDB standard.
The hydrocarbon gas contents of the 38 samples in this study range from 60.19% to 99.71%, with an average of 92.77%. Samples also contain a small amount of N2, CO2, and H2S (Table 1). A small number of samples have N2 content exceeding 10%; other samples have N2 content of 0–3.8%, with an average of 1.01%. Most of the samples have low CO2 content, less than 5%. A few samples have higher CO2 content. The marine carbonate rocks in the reservoirs are accompanied by gypsum salt. This results in the gas reservoirs generally containing H2S. Most of the natural gas containing H2S in the world is distributed in such type of strata, and it is considered to be formed by TSR action (Krouse, et al., 1988; Worden, et al., 1995; Machel, 2001; Cross et al., 2004). The content of heavy hydrocarbon gas such as ethane in the hydrocarbon gas is very low, and the dry coefficient (C1/C1+) of natural gas is very high, ranging from 0.940 to 0.998, with an average of 0.983. Taking more than 0.95 as the criterion for dry gas, all the natural gas in the Leikoupo Formation is dry gas. Among them, the T2l1 gas reservoir in the Moxi gas field has the highest dry coefficient, with an average of 0.998, and the T2l3 gas reservoir in the Zhongba gas field is the lowest, with an average of 0.97. The T2l4 gas reservoirs in the Longgang and Yuanba gas fields are 0.971 and 0.991, respectively. Although both belong to T2l4 gas reservoir, the dry coefficient of the Yuanba gas field is slightly larger than that of the Longgang gas field, reflecting the difference in the maturity of the source rocks.
TABLE 1. Composition and isotopic data of Permian and Triassic natural gas in the central Sichuan Basin.
The natural gas in the upper and lower strata of the Leikoupo Formation is mainly hydrocarbon gas, the content of non-hydrocarbon gas is relatively low, and there are obvious differences in natural gas components between different strata.
The most important feature of natural gas in the Xujiahe Formation is that it does not contain H2S; the content of N2 is 0−16.5%, with an average of 1.36%, and the content of CO2 is 0−8.13%, with an average of 0.78%. The content of ethane and other heavy hydrocarbon gas in alkane gas varies greatly for different gas reservoirs. The content of heavy hydrocarbon in the Zhongba gas field and the Longgang gas field is similar. The content in the Zhongba gas field and the Longgang gas field is between 2.22% and 11.37%, respectively, with an average of 7.32%, and the dry coefficient is between 0.89% and 0.98%, with an average of 0.92. There are both dry gas and wet gas in natural gas, indicating that the characteristics of gas source rocks are more complex. The content of heavy hydrocarbon gas in the Yuanba gas field is not only relatively low but also relatively concentrated, 0.71%–2.82%, with an average of 1.40%, and the dry coefficient is 0.97–0.99, with an average of 0.99. This shows that the source rock characteristics of natural gas in the Yuanba gas field are located under and adjacent to the T2l1 gas reservoir. The composition characteristics of natural gas are highly consistent with that of the T2l1 gas reservoir, and the dry coefficient of natural gas is close to 1.0%. The average content of N2 is 1.1% and that of CO2 is 0.2% (Table 1).
The gas reservoirs of Feixuan and Changxing formations under the Jialingjiang Formation have the same source of natural gas (Qin, et al., 2016a), and the geochemical characteristics of natural gas are completely the same. The natural gas dry coefficient is close to 1; contains higher H2S, nitrogen, and carbon dioxide content of most samples less than 5%; and also there are some samples have high content (Table 1).
The carbon isotopes of natural gas in the Leikoupo Formation are generally less negative, and the overall characteristics are that the δ13C1 value is relative concentrated, ranging from −38.4‰ to −31.7‰, with an average of −35.4%, but the δ13C2 values vary widely, ranging from −36.7‰ to −25.1‰, with an average of −31.4‰. As the propane content is very low, only part of the samples were detected, with the δ13C3 values ranging from −33.4‰ to −22.1‰, with an average of −26.9‰.
The carbon isotopes of different gas reservoirs are obviously different. The carbon isotope values of the T2l1 gas reservoir in the Moxi gas field are the most concentrated, with δ13C1 ranging from −35.8‰ to −32.8‰, with an average of −34.4‰, and δ13C2 ranging from −33.8‰ to −29.7‰, with an average of −32.3‰. Although the δ13C1 values are concentrated in the T2l3 gas reservoir in the Zhongba gas field and the T2l4 gas reservoir in Longgang and Yuanba gas fields, the range of the δ13C2 value is widely distributed, ranging from −36.7‰ to −25.1‰. The carbon isotope characteristics of natural gas in the Jialingjiang Formation are consistent with those of the T2l1 reservoir, with δ13C1 ranging from −34.8‰ to −32.4‰, with an average of −34.1‰, and δ13C2 ranging from −34.6‰ to −32.9‰, with an average of −33.7‰.
The δ13C1 of Changxing and Feixianguan formations in Yuanba and Longgang fields are the least negative in this area, and the variation range is relatively narrow, indicating the relatively consistent gas source rocks. The δ13C1 ranges from −31‰ to −27.8‰, with an average of −29.2‰, and δ13C2 ranges from −29.7‰ to −21.7‰, with an average of −25.8‰ (Table 1).
The δ13C1 of the Xujiahe Formation gas reservoir ranges from −42.2‰ to −30.0‰, with an average of −34.7‰, and the δ13C2 ranges from −35.4‰ to −20.8‰, with an average of −26.3‰. The carbon isotopes of natural gas vary widely, especially the ethane carbon isotopes that reflect the genetic types of natural gas. The natural gas of the Xujiahe Formation mainly comes from its own coal-measure source rocks, and its carbon isotopes vary greatly, which reflects that the source rock type of natural gas may not be single.
In addition, the natural gas in Leikoupo and Xujiahe gas reservoirs in the Yuanba gas field show the inversion of δ13C1 and δ13C2, that is, δ13C1 > δ13C2 (Table 1; Figure 2).
FIGURE 2. Comparison of carbon isotope characteristics of Permian–Triassic natural gas in the Sichuan Basin.
According to the carbon isotope distribution chart, it can be seen that among the natural gas in the Leikoupo Formation, the Longgang gas field is the most negative, followed by the Zhongba gas field. Yuanba and Moxi gas fields are relatively less negative (Figure 2). The source maturity of natural gas in the Leikoupo Formation of Longgang, Zhongba, Yuanba, and Moxi gas fields increases sequentially. The gas samples of T1j and T2l1 gas reservoirs in the Moxi gas field completely overlap in Whiticar’s chart. The natural gas of Changxing and Feixiangguan formations in Yuanba and Longgang gas fields also fall in the same area, and the maturity of their source rocks are much higher than that of the Leikoupo gas reservoir (Figure 3). In order to judge the genetic type of natural gas more precisely, this study adopts the identification standard of genetic type of natural gas proposed by Dai, that is, the value of δ13C2 more negative than −28.8‰ indicate the sapropel-type gas (Dai, 1993).
FIGURE 3. Identification of natural gas from the Leikoupo Formation in the Sichuan Basin using Whiticar’ identification Chart [(modified after Whiticar (1999)].
It can be seen from Table 1 that the δ13C2 values of natural gas in the T2l1 reservoir of the Moxi gas field are more negative than −28.8‰, the genetic type is relatively simple, and all samples are sapropel gas. Among the three natural gas samples of the T2l3 member in the Zhongba gas field, two samples are sapropel gas and one is humic. The genesis of natural gas in the T2l4 reservoirs of Longgang and Yuanba gas fields is complicated; some samples are humic gas and some are sapropel-type, and the heterogeneity of the gas reservoir is very obvious. Half of the natural gas samples of the T2l4 gas reservoir in the Longgang gas field are humic-type, while only a few samples of the T2l4 gas reservoir in the Yuanba gas field are humic-type, and the natural gas is mainly sapropel-type (Figure 4). The carbon isotope of alkane gas series can better reflect the overall appearance of natural gas. Half of the samples of the T2l4 gas reservoir in the Longgang gas field are humic-type and the other half are sapropel-type, while the gas samples of the T2l4 gas reservoir in the Yuanba gas field are mainly sapropel-type, and only a few are humic gas (Figure 5).
FIGURE 5. Comparison of δ13C of natural gas between the T2l4 reservoir and the P3ch-T1f reservoirs of the Sichuan Basin.
The paleokarst on the top of the Leikoupo Formation developed and formed a good karst reservoir, which showed an unconformable contact with the coal-measure source rocks of the overlying Xujiahe Formation (Song, et al., 2012; Wang, et al., 2018a). Some corrosion ditches are formed in the Leikoupo Formation, and the corrosion ditches will be filled by the Xujiahe Formation. Theoretically, the natural gas generated by the source rocks of the Xujiahe Formation has the opportunity to migrate to the top reservoir of the Leikoupo Formation; therefore, the samples showing humic-type in T2l4 reservoir should come from the source rocks of the Xujiahe Formation.
It is researched that there is a close relationship between the δ13C2 values and the C2H6 content in the T2l4 reservoir of Longgang and Yuanba gas fields. The higher the ethane content, the less negative of the carbon isotope, showing the characteristics of humic gas. Taking the Longgang gas field as an example, the samples with ethane content more than 2% in the T2l4 reservoir are humic gas, and on the contrary, those with the ethane content less than 2% are sapropel gas. A similar situation has occurred in the Yuanba gas field. Because the maturity of the source rocks is higher than that of the Longgang gas field, the ethane content is generally lower than that of the Longgang, but the δ13C2 also tends to become less negative with the increase of the ethane content. The ethane content of several humic-type gas samples in T2l4 is more than 1%. The ethane content of sapropel gas samples is less than 1% (Figure 4). The δ13C2 value can best reflect the type of source rock, and the content of ethane can often reflect the content of heavy hydrocarbon gas, which indirectly reflects the dry coefficient (or humidity coefficient) of natural gas; the higher value of the ethane content, the lower the dry coefficient (high humidity coefficient). The humidity coefficient is related to the maturity of source rocks. For the same type of source rocks, the smaller the humidity coefficient, the higher the maturity of source rocks (Dai, et al., 2016b). Under the same degree of evolution, different types of source rocks have different dry coefficients, the sapropel-type is lower than humic-type. The ethane content of sapropel gas is less than that of sapropel gas in T2l4, indicating that the maturity of sapropel gas of hydrocarbon source rocks is higher than that of humic gas.
According to the carbon isotope of the alkane gas series of the T2l4, there are obvious differences in carbon isotopes of heavy hydrocarbons such as ethane between sapropel and humic gas; however, there is no obvious difference in the δ13C1 values (Figure 5). Based on this, it is concluded that the sapropel gas in T2l4 can only come from the source rocks of the Leikoupo Formation or other underlying strata, mainly sapropel organic matter. Due to the fact that the δ13C1 value generated by sapropelic source rocks is more negative than that generated by humic source rocks under the same maturity, the δ13C2 value is highly related to the type of organic matter and does not change obviously with the increase of the maturity of source rocks, and the genetic type of natural gas is therefore often identified according to the ethane carbon isotope.
As the Xujiahe Formation is the first terrestrial strata deposited after the end of marine sedimentation in the Sichuan Basin, in addition to humic source rocks, the T3x1 member may also develop marine sapropel source rocks at some local area. It is unlikely that the sapropel gas in the T2l4 reservoir come from the sapropel source rocks that may undergo phase transformation corresponding to the T3x1 member in contact with it. If the sapropel gas in the T2l4 comes from the sapropel source rock in the T3x1 member, the maturity of this source rock should be similar to other humic hydrocarbon source rocks in the T3x1. As mentioned before, the δ13C1 from rocks with the same maturity should be lighter than those from humic source rocks. In fact, the δ13C1 of sapropel and humic gas in T2l4 are similar, but the δ13C2 is quite different (Figure 5). The ethane content of sapropel gas in the T2l4 reservoir is less than that of humic gas (Figure 4). Therefore, it is judged that the sapropel gas in T2l4 comes from source rocks with higher maturity than the source rocks in the T3x1 member.
It is not possible that the sapropel gas in T2l4 came from the source rocks of the Longtan Formation below. This is because the hydrocarbon source rocks of the Longtan Formation and the T2l4 reservoir are separated by multiple sets of gypsum strata from the Jialingjiang Formation and T2l1 to T2l3 members, and there are many sealing layers, making it difficult for natural gas to migrate to the T2l4 reservoir. In addition, the natural gas from the Changxing and Feixianguan formations in Longgang and Yuanba gas fields all come from coal-measure source rock of the Longtan Formation; the carbon isotopes of methane and ethane are much less negative, and their characteristics are very different from those in T2l4 reservoirs, uncorrelated with the gas in T2l4 reservoir (Figures 3, 5). Collectively, it is judged that the sapropel gas in T2l4 should come from the source rocks developed in the T2l3, and the humic gas comes from the Xujiahe coal-measure source rock.
The forming condition of T2l3 gas reservoir is similar to that of the T2l4, in which the humic gas comes from the overlying Xujiahe Formation source rocks and the sapropel gas comes from the T2l3 source rocks. In this study, the T2l3 gas reservoir occurs in the Zhongba gas field, where the T2l4 member is depleted and the T2l3 section is in unconformity contact with the overlying Xujiahe Formation. The T3x2 gas reservoir develops above the T3x2 member in the gas field. The δ13C1 of the gases in T2l3 and T3x2 reservoirs are similar, with the former averaging −37‰ and the latter −36‰, but δ13C2 are more different, with the Leikoupo Formation being more negative, averaging −29.7‰, and the T3x2 averaging −24.7‰, both of which are from different hydrocarbon source rocks. The T2l3 itself has source rock, and the sapropel-type gas should come from the T2x3 member source rock.
In this study, all gas data of T2l1 come from the Moxi gas field, where the T2l1 gas reservoir is adjacent to the underlying Jialingjiang gas reservoir, and its geochemical characteristics are highly consistent with the natural gas in the Jialingjiang Formation based on the analysis of the samples in this study (Figures 2, 3, 6). The gas in both reservoirs should be from the same source, but it is difficult to determine which set of hydrocarbon source rocks the gas comes from. First, it is impossible for the gas to come from the coal-measure source rocks of the Xujiahe Formation because the gas characteristics of the T2l1 do not match the humic gas and there are effective interlayers between the Xujiahe Formation and T2l1, T2l2, T2l3, and T2l4 members, and it is difficult for the gas of the Xujiahe Formation to traverse downward through the multilayered gypsum rocks to reach the T2l1 reservoir.
FIGURE 6. Comparison of δ13C1 and δ13C2 in natural gas between the Leikoupo Formation and underlying gas reservoirs.
Comparing the isotopic data of typical sapropel gas samples from the T2l4 section of the Longgang and Yuanba gas fields with the T2l1 gas reservoir, it is found that the T2l1 gas is closer to the sapropel gas of the T2l4 reservoir in Yuanba. The difference is that most of the sapropel gas samples from the T2l4 reservoir in Yuanba show carbon isotope inversion, while the carbon isotopes of gas from the T2l1 reservoir in Moxi do not. The δ13C2 (average −32.3‰) is significantly less negative than that of sapropel gas (average 35.3‰) in the T2l4 gas reservoir in Yuanba (Figure 7), and the source rock may be relatively humic. Although there are some similarities between the carbon isotopes of T2l1 gas and T2l4, we cannot suggest that T2l1 gas may also come from T2l3 hydrocarbon source rocks because the direct caprock of the T2l1 gas reservoir is the gypsum layer and gypsum dolomite above the gas reservoir. There are three layers of anhydrite layers with a total thickness of about 5 m on the gas reservoir, and 20 layers of anhydrite and gypsum dolomite are sandwiched up together with the T2l1 member, with a thickness of 160 m, which together constitute a good direct cover for the T2l1 gas reservoir (Dai, et al., 1996). Therefore, it is difficult for natural gas in the T2l3 member to migrate down to the T2l1 reservoir across such a thick high-quality caprock.
FIGURE 7. Comparison of δ13C1 and δ13C2 of natural gas in the T2l1 reservoir with T2l4, P3ch and T1f reservoirs.
Earlier studies suggested that the natural gas in the T2l1 gas reservoir of the Moxi gas field was mainly from the coal-measure source rock of the Longtan Formation based on the less negative of δ13C2 value in some drilled wells (Wang, et al., 1998). However, the average δ13C2 value of T2l1 gas is −32.3‰, which is typical of sapropel gas (Figure 6). Its methane and ethane carbon isotopes are very different from the natural gas from the Longtan Formation coal-measure source rocks in the Changxing and Feixianguan formation gas reservoirs of the Longgang and Yuanba gas fields, and the carbon isotopes of the Changxing and Feixianguan natural gas are much less negative (Figure 7). Therefore, the natural gas in T2l1 gas reservoir does not come from the coal-measure rocks of the Longtan Formation. However, the coal-measure hydrocarbon source rocks of the Longtan Formation would have undergone a phase change in some areas of the Sichuan Basin and become marine hydrocarbon source rocks of the Wujiaping Formation (the Upper Permian), with a shift in organic matter type from humic to sapropelic. Compared with the coal measures of the Longtan Formation, the carbon isotope of the natural gas generated by the source rocks of the Wujiaping Formation would be relatively more negative, which can completely match the natural gas of the T2l1 member. Therefore, it is believed that the natural gas from the T2l1 member of the Moxi gas field comes from source rocks of the Wujiaping Formation.
The phenomenon of carbon isotope inversion in alkane gas has been reported for a long time (Stahl et al., 1975; Fuex, 1977; Burruss et al., 2010) and later studied by Tilley et al. (2011), Zumberge et al. (2012), and many others. This phenomenon is often found in major oil and gas basins in China, especially shale gas, where carbon isotopes are mostly in reverse order (Dai et al., 2016a). There are various explanations for the isotope inversion, but none is convincing. Most of the carbon isotope inversions in this study occurred in the T2l4 member of the Yuanba gas field, nine of the 14 samples have carbon isotope inversions of methane and ethane, that is, δ13C2<δ13C1. The inverted samples were nearly 65% (Table 1; Figure 7). Some samples from the Xujiahe Formation in the Yuanba gas field have carbon isotope inversions of methane and ethane, and the samples are all distributed in the T3x2 member, such as wells YL 9 and YL 10 (Table 1). No carbon isotope inversions were found in either T2l4 or Xujiahe Formation gas reservoirs in the Longgang gas field, and no carbon isotope inversions occurred in any of the other gas reservoirs.
First, we think that the carbon isotope inversion presented in this study can rule out the reason caused by mixing different natural gases. The natural gas generated from the Xujiahe Formation can migrate downward to the T2l4 gas reservoir, which is common in the Longgang gas field. Half of the samples from the T2l4 gas reservoir in the Longgang gas field are humic gas, while only a few samples from T2l4 gas reservoir in the Yuanba gas field are humic gas (Figure 8). This indicates that the scale of humic gas of the Xujiahe Formation mixed into the T2l4 gas reservoir in Longgang is larger than that in Yuanba. However, the natural gas of the Longgang T2l4 member does not appear reversed, while most samples of the Yuanba T2l4 member with little humic gas appear carbon isotope inversion. In addition, no sapropel gas samples were found in the Xujiahe Formation gas reservoir in the Longgang gas field, indicating that the natural gas in T2l4 member did not migrate upward obviously. However, more than half of the samples from the Xujiahe Formation gas reservoir in the Yuanba gas field are sapropel gas, indicating that the natural gas from the underlying T2l4 gas reservoir has massively upwardly migrated into the Xujiahe Formation gas reservoir (Figure 9). Only a few individual samples showed carbon isotope inversions in the case of large amounts of sapropel gas mixed into the Xujiahe Formation in the Yuanba gas field, and the inverted samples were all found in the T3x2 member, which is close to the T2l4 member, and showed typical sapropel gas, which had already inverted before leaving the T2l4 member and entering the Xujiahe Formation.
From the carbon isotope of sapropel gas in the T2l4 member of Longgang and Yuanba gas fields, the δ13C2 in T2l4 member of Yuanba is 2‰ more negative than that of Longgang, while the methane carbon isotope is 2‰ less negative than that of Longgang. This indicates that the hydrocarbon source rock type in the T2l3 member of the Yuanba gas field is of a higher quality than that of Longgang gas field. The hydrocarbon source rock maturity is higher than Longgang. Therefore, it can be concluded that the carbon isotope inversion of natural gas in the Yuanba T2l4 member occurred because of its good hydrocarbon source rock type and higher maturity. Because the hydrocarbon source rock type is good and very favorable for oil generation, the ethane carbon isotope will be relatively more negative and will change less with increasing evolution, while methane carbon isotopes become less negative rapidly and become less negative than ethane as source rock maturity reaches a certain level. It is difficult for humic gas to have the carbon isotope inversion of methane and ethane. Because the δ13C2 in humic gas is inherently much less negative, the carbon isotope of methane generated during the evolution of source rocks is difficult to be less negative than ethane. This also explains why the methane and ethane carbon isotopes in the Changxing and Feixianguan formation gas reservoirs did not reverse.
The natural gas of the Leikoupo Formation in the Sichuan Basin has complex genetic types and various gas sources. The natural gas in the T2l1 gas reservoir of the Moxi gas field is all sapropel-type, which comes from the sapropel source rock of the Upper Permian Wujiaping Formation. The natural gas in the T2l3 gas reservoir of the Zhongba gas field is mainly sapropel gas, which comes from hydrocarbon source rocks of T2l3 itself. Half of the natural gas in T2l4 gas reservoir of the Longgang gas field is humic gas from the humic source rocks of the Xujiahe Formation, and the other half is sapropel gas from the T2l3 source rocks. The gas in T2l4 gas reservoir of the Yuanba gas field is mainly sapropel gas from the T2l3 hydrocarbon source rocks, and a very small portion is humic gas from the Xujiahe Formation source rocks. The natural gas with inversed carbon isotopes of methane and ethane was formed from favorable quality source rocks at a higher evolution stage.
The original contributions presented in the study are included in the article/Supplementary Material; further inquiries can be directed to the corresponding author.
SQ put forward the opinion of article and wrote the manuscript. BZ compiled the diagrams. CH collected data. JL collected sample, performed analysis, and helped with manuscript translation. JW helped with sample analysis and manuscript translation. GT helped with sample analysis and manuscript translation. ZZ helped some data interpretation and manuscript revision.
Authors SQ, BZ, and CH were employed by the company Southwest Oil and Gas Company, PetroChina.
The remaining authors declare that the research was conducted in the absence of any commercial or financial relationships that could be construed as a potential conflict of interest.
All claims expressed in this article are solely those of the authors and do not necessarily represent those of their affiliated organizations, or those of the publisher, the editors, and the reviewers. Any product that may be evaluated in this article, or claim that may be made by its manufacturer, is not guaranteed or endorsed by the publisher.
This study was jointly sponsored by the National Natural Science Foundation of China (Grant Nos. 41872162, 42141022). We thank Jamie Beagle for language correction. We are grateful to the editor for handling this manuscript and reviewers for helpful comments and suggestions that have improved the manuscript.
Bian, C., Wang, Z., Jiang, Q., Chi, Y., and Xu, Z. (2019). Characteristics and Distribution of Karst Reservoirs in the Leikoupo Formation, Western Sichuan Basin. China Pet. Explor. 24 (1), 82–94. doi:10.3969/j.issn.1672-7703.2019.01.009
Burruss, R. C., and Laughrey, C. D. (2010). Carbon and Hydrogen Isotopic Reversals in Deep Basin Gas: Evidence for Limits to the Stability of Hydrocarbons. Org. Geochem. 41, 1285–1296. doi:10.1016/j.orggeochem.2010.09.008
Cross, M. M., Manning, D. A. C., Bottrell, S. H., and Worden, R. H. (2004). Thermochemical Sulphate Reduction (TSR): Experimental Determination of Reaction Kinetics and Implications of the Observed Reaction Rates for Petroleum Reservoirs. Org. Geochem. 35 (4), 393–404. doi:10.1016/j.orggeochem.2004.01.005
Dai, H., Huang, D., Liu, X., Yang, Y., He, X., Peng, H., et al. (2008). Characteristics and Evaluation of Marine Source Rock in Southwestern Shunan. Nat. Gas. Geosci. 19 (4), 503–508. doi:10.11764/j.issn.1672-1926.2008.04.503
Dai, J. (1993). Characteristics of Carbon and Hydrogen Isotopes of Natural Gases and Their Discriminations. Nat. Gas. Geosci. 2 (3), 1–40. doi:10.11764/j.issn.1672-1926.1993.02.1
Dai, J., Chen, J., Zhong, N., and Qin, S. (2003). The Gas Fields and Their Origins in China. Beijing: Science Press, 28–33.
Dai, J., Ni, Y., Huang, S., Gong, D., Liu, D., Feng, Z., et al. (2016a). Secondary Origin of Negative Carbon Isotopic Series in Natural Gas. J. Nat. Gas Geoscience 1 (1), 1–7. doi:10.1016/j.jnggs.2016.02.002
Dai, J., Ni, Y., Zhang, W., Huang, S., Gong, D., Liu, D., et al. (2016b). Relationships between Wetness and Maturity of Coal-Derived Gas in China. Petroleum Explor. Dev. 43 (5), 675–678. doi:10.1016/s1876-3804(16)30088-x
Dai, J., Pei, X., and Qi, H. (1996). Natural Gas Geology in China Vol. 1. Beijing: The Petroleum Industry Press, 14–15.
Dai, J. (1980). Preliminary Research on Natural Gas in Coal Series in China. Acta Pet. Sin. 1 (4), 27–37. doi:10.7623/syxb198004003
Deng, Y., Hu, G., and Zhao, C. (2018). Geochemical Characteristics and Origin of Natural Gas in Changxing-Feixianguan Formations from Longgang Gas Field in the Sichuan Basin, China. Nat. Gas. Geosci. 29 (6), 892–907. doi:10.11764/j.issn.1672-1926.2018.03.016
Fan, Z. (2014). Distribution of Ancient Ditches in Leikoupo Formation and its Control over Gas Accumulations in Yuanba Gas Field. Petroleum Geol. Exp. 36 (5), 562–566. doi:10.11781/sysydz201405562
Fuex, A. N. (1977). The Use of Stable Carbon Isotopes in Hydrocarbon Exploration. J. Geochem. Explor. 7, 155–188. doi:10.1016/0375-6742(77)90080-2
Guo, X., and Guo, T. (2012). Theory and Practice of Exploration of Large Gas Fields at the Margin of the Puguang and Yuanba Carbonate Platforms. Beijing: Science Press, 413.
Hao, F., Guo, T., Zhu, Y., Cai, X., Zou, H., and Li, P. (2008). Evidence for Multiple Stages of Oil Cracking and Thermochemical Sulfate Reduction in the Puguang Gas Field, Sichuan Basin, China. Bulletin 92 (5), 611–637. doi:10.1306/01210807090
Hu, G., Yu, C., Gong, D., Tian, X., and Wu, W. (2014a). The Origin of Natural Gas and Influence on Hydrogen Isotope of Methane by TSR in the Upper Permian Changxing and the Lower Triassic Feixianguan Formations in Northern Sichuan Basin, SW China. Energy Explor. Exploitation 32 (1), 139–158. doi:10.1260/0144-5987.32.1.139
Hu, W., Zhu, Y., Li, Y., Zou, H., and Guo, T. (2014b). Geochemical Characteristics and Origin of Natural Gases from Terrestrial Strata in Yuanba Area of the Northeastern Sichuan Basin. J. Zhejiang Univ. Sci. Ed. 41 (4), 468–476. doi:10.3785/j.issn.1008-9497.2014.04.020
Huang, R. (2014). Source and Accumulation of Natural Gas in Leikoupo Formation, Yuanba Area, Eastern-Northern Sichuan Basin. Geoscience 28 (2), 412–418. doi:10.3969/j.issn.1000-8527.2014.02.020
Hussain, M., and Warren, J. K. (1991). Source Rock Potential of Shallow-Water Evaporites: An Investigation in Holocenepleistocene Salt Flat Sabkah (Playa), West Texas-New Mexico. Carbonates Evaporites 6 (2), 217–224. doi:10.1007/BF03174424
Jin, Q., and Zhu, G. (2006). Progress in Research of Deposition of Oil Source Rocks in Saline Lake and Their Hydrocarbon Generation. Geol. J. China Univ. 12 (4), 483. doi:10.3969/j.issn.1006-7493.2006.04.009
Krouse, H. R., Viau, C. A., Eliuk, L. S., Ueda, A., and Halas, S. (1988). Chemical and Isotopic Evidence of Thermochemical Sulphate Reduction by Light Hydrocarbon Gases in Deep Carbonate Reservoirs. Nature 333, 415–419. doi:10.1038/333415a0
Krzywiec, P., Peryt, T. M., Kiersnowski, H., Pomianowski, P., Czapowski, G., and Kwolek, K. (2017). Permo-Triassic Evaporites of the Polish Basin and Their Bearing on the Tectonic Evolution and Hydrocarbon System, an Overview. Permo-Triassic salt Prov. Eur. North Afr. Atl. Margins 2017, 243–261. doi:10.1016/B978-0-12-809417-4.00012-4
Li, W. (2011). Formation of a Saline Enviroment and Evolution of a Sedimentary System in the Late Triassic of the Sichuan Basin. Nat. Gas. Ind. 31 (9), 31–38. doi:10.3787/j.issn.1000-0976.2011.09.006
Li, W., Zou, C., Yang, J., Wang, K., Yang, J., Wu, Y., et al. (2010). Types and Controlling Factors of Accumulation and High Productivity in the Upper Triassic Xujiahe Formation Gas Reservoirs, Sichuan Basin. Acta Sedimentol. Sin. 28 (5), 1037–1045. doi:10.14027/j.cnki.cjxb.2010.05.018
Li, Z. (1993). Surveying Gas Prospects of Leikoupo Formation in West Sichuan. Nat. Gas. Ind. 13 (2), 28–33. doi:10.11821/yj1983040003
Liao, F., Wu, X., Huang, S., and Yu, C. (2013). Geochemical Characeristics and Gas Source Correlation of Leikoupo Formation in Zhongba Field, Northwest Sichuan Basin. Nat. Gas. Geosci. 24 (1), 108–115. doi:10.11764/j.issn.1672-1926.2013.01.108
Liu, J., Liu, G., Wang, L., and Wu, X. (2014). Geochemical Characteritics and Origin of Permain and Triassic Natural Gas in Yuanba-Tongnanba Area, Northeastern Sichuan Basin. Acta Pet. Sin. 35 (3), 417–428. doi:10.7623/syxb201403002
Liu, R., Guo, T., and Shao, M. (2011). Source and Genetic Types of Gas in the Middle-Shallow Strata of the Yuanba Area, Northeast Sichuan Basin. Nat. Gas. Ind. 31 (6), 34–38. doi:10.3787/j.issn.1000-0976.2011.06.005
Liu, S., Sun, W., Song, J., Yong, Z., Wang, H., and Zhao, C. (2019). The Key Geological Promblems of Natural Gas Exploration in the Middle Triassic Formation in Sichuan Basin. Nat. Gas. Geosci. 30 (2), 151–167. doi:10.11764/j.issn.1672-1926.2018.12.011
Luo, Q. (1983). Discovery of Water-Transgression Cause Filling Sand-Bodies in Ancient Sediments-An Approach to the Genesis of Certain Upper Triassic Sand-Bodies in the Middle-Western Part of the Sichuan Basin and Discussion on Water-Transgression Delta. Acta Sedimentol. Sin. 1 (3), 59–67. CNKI:SUN:CJXB.0.1983-03-004.
Luo, Q. (2011). Understanding of the Upper Triassic Sedimentary Facies in the Sichuan Basin. Nat. Gas. Ind. 31 (9), 12–15. doi:10.3787/j.issn.1000-0976.2011.09.003
Machel, H. G. (2001). Bacterial and Thermochemical Sulfate Reduction in Diagenetic Settings-Old and New Insights. Sediment. Geol. 140 (1-2), l43–175. doi:10.1016/S0037-0738(00)00176-7
Peng, C., Liu, K., Zhang, Y., and Zhu, P. (2011). Seismic Sedim Entology of Organic Reef from the Changxing Form Ation of Central Sichuan. Nat. Gas. Geosci. 22 (3), 460–464. doi:10.11764/j.issn.1672-1926.2011.03.460
Qin, H., Pan, L., Yin, F., and Shen, J. (2016b). Discution on Source of Natural Gas and Causation of Reversed Orders of δ13C in Alkane Gas from Leikoupo Formation in Yuanba, Sichuan Basin, China. J. Chengdu Univ. Technol. Sci. Technol. Ed. 43 (5), 591–600. doi:10.3969/j.issn.1671-9727.2016.05.09
Qin, S., Tao, S., Tu, T., Wei, X., and Song, M. (2007). Characeristics of Natural Gas Geochemistry and Accumulation in Western Sichuan Depression. Petroleum Explor. Dev. 34 (1), 34–38. doi:10.3321/j.issn:1000-0747.2007.01.007
Qin, S., Yang, Y., Lü, F., Zhou, H., and Li, Y. (2016a). The Gas Origin in Changxing-Feixianguan Gas Pools of Longgang Gasfield in Sichuan Basin. Nat. Gas. Geosci. 27 (1), 40–48. doi:10.11764/j.issn.1672-1926.2016.01.0041
Song, W., Liu, L., Gan, X., Qin, Q., Su, P., and Fan, C. (2012). Weathering Crust Karstification in Leikoupo Formation in Central Sichuan Area. Nat. Gas. Geosci. 23 (6), 1019–1024. CNKI:SUN:TDKX.0.2012-06-007.
Stahl, W. J., and Carey, B. D. (1975). Source-rock Identification by Isotope Analyses of Natural Gases from Fields in the Val Verde and Delaware Basins, West Texas. Chem. Geol. 16, 257–267. doi:10.1016/0009-2541(75)90065-0
Sun, H., Luo, B., Wen, L., Wang, J., Zhou, G., Wen, H., et al. (2021). The First Discovery of Organic-Rich Shale in Leikoupo Formation and New Areas of Subsalt Exploration, Sichuan Basin. Nat. Gas. Geosci. 32 (2), 233–247. doi:10.11764/j.issn.1672-1926.2020.11.011
Tilley, B., McLellan, S., Hiebert, S., Quartero, B., Veilleux, B., and Muehlenbachs, K. (2011). Gas Isotope Reversals in Fractured Gas Reservoirs of the Western Canadian Foothills: Mature Shale Gases in Disguise. Bulletin 95 (8), 1399–1422. doi:10.1306/01031110103
Wang, S., Dai, H., Wang, T., and Lin, F. (1998). Gas Source and Migration of High-Mature Natural Gas in Moxi Gas Field. Petroeum Explor. 3 (2), 5–8. CNKI:SUN:KTSY.0.1998-02-002.
Wang, S., Luo, Q., and Wu, D. (1997). Organic Petrology of Source Rocks from the Upper Triassic Coal Measures in the Central and Western Sichuan Basin. J. Mineral Pet. 17 (1), 63–70.
Wang, T., Zhen, Y., Li, S., Zeng, Q., and He, J. (1989). From Geochemical Characteristics of Oil and Gas to Discuss the Gas Source of Lei-3 Reservoir, Zhongba Gas Field, in Northwest Sichuan. Nat. Gas. Ind. 9 (5), 20–26. CNKI:SUN:TRQG.0.1989-05-004.
Wang, W., Xu, G., Dan, Y., Song, X., Wang, Q., Feng, X., et al. (2018a). Unconformity Characeristics of the Top of Leikoupo Formation and Their Effect on Reservoirs in the Western Sichuan Basin. Carsologica Sin. 37 (4), 592–601. doi:10.11932/karst20180413
Wang, Y., Chen, Y., Hu, Y., Zeng, H., and Wu, X. (2018b). Discussion on Hydrocarbon Generation Ability of Evaporation Environment: A Case Study of Leikoupo Formation in West Sichuan Depression. Fault-Block oil Gas Fied 25 (4), 426–430. doi:10.6056/dkyqt201804004
Wen, L., Zhang, Q., Yang, Y., Liu, H., Che, Q., Liu, W., et al. (2012). Factors Controlling Reef-Bank Reservoirs in the Changxing-Feixianguan Formations in the Sichuan Basin and Their Play Fairways. Nat. Gas. Ind. 32 (1), 39–44. doi:10.3787/j.issn.1000-0976.2012.01.007
Whiticar, M. J. (1999). Carbon and Hydrogen Isotope Systematics of Bacterial Formation and Oxidation of Methane. Chem. Geol. 161 (1/2/3), 291–314. doi:10.1016/S0009-2541(99)00092-3
Worden, R. H., Smalley, P. C., and Oxtoby, N. H. (1995). Gas Souring by Thermochemical Sulfate Reduction at 140øC. Bulletin 79, 854–863. doi:10.1306/8d2b1bce-171e-11d7-8645000102c1865d
Wu, X., Liu, G., Liu, Q., Liu, J., and Yuan, X. (2015). Geochemical Characteristics and Genetic Types of Natural Gas in the Changxing-Feixianguan Formations from Yuanba Gasfield in the Sichuan Basin. Nat. Gas. Geosci. 26 (11), 460–464. doi:10.11764/j.issn.1672-1926.2015.11.2155
Xie, F., Wu, Q., Wang, L., Shi, Z., Zhang, C., Liu, B., et al. (2019). Passive Continental Margin Basins and the Controls on the Formation of Evaporites: A Case Study of the Gulf of Mexico Basin. Carbonates Evaporites 34 (2), 405–418. doi:10.1007/s13146-017-0404-z
Xu, G., Song, X., Feng, X., Long, K., Wang, Q., Shi, G., et al. (2013). Gas Potential of the Middle Triassic Leikoupo Fm in the Western Sichuan Basin. Nat. Gas. Ind. 33 (8), 8–14. doi:10.3787/j.issn.1000-0976.2013.08.002
Yang, G., Shi, X., Huang, D., Wang, H., and Ding, W. (2014). Characteristics and Major Controls of Weathering Crust Reservors in T2l43 in the Longgang Gas Field, Sichuan Basin. Nat. Gas. Ind. 34 (9), 17–24. doi:10.3787/j.issn.1000-0976.2014.09.003
Yang, K. (2016). Hydrocarbon Potential of Source Rocks in the Middle Triassic Leikoupo Formation in the Western Sichuan Depression. Petroleum Geol. Exp. 38 (3), 366–374. doi:10.11781/sysydz201603366
Yang, X., Zou, C., Tao, S., Wang, Z., Li, J., and Wang, S. (2005). Characteristics of Upper Triassic-Jurassic Oil and Gas System in Sichuan Basin and Oli and Gas Abundance Law. China Pet. Explor. 10 (2), 15–22. doi:10.3969/j.issn.1672-7703.2005.02.003
Yin, F., Liu, R., Wang, W., Zhang, Y., and Pan, L. (2013). Geochemical Characters of the Tight Sandstone Gas from Xujiahe Formation in Yuanba Gas Field and its Gas Source. Nat. Gas. Geosci. 24 (3), 621–627. doi:10.11764/j.issn.1672-1926.2013.03.621
Yu, K., Cao, Y., Qiu, L., and Sun, P. (2018). The Hydrocarbon Generation Potential and Migration in an Alkaline Evaporite Basin: The Early Permian Fengcheng Formation in the Junggar Basin, Northwestern China. Mar. Petroleum Geol. 98, 12–32. doi:10.1016/j.marpetgeo.2018.08.010
Zeng, D., Wang, X., Zhang, F., Song, Z., Zhang, R., Zhu, Y., et al. (2007). Study on Reservoir of the Leikoupo Formation of Middle Triassic in Northwestern Sichuan Basin. J. Palaeogeogr. 9 (3), 253–266. doi:10.3969/j.issn.1671-1505.2007.03.003
Zhang, J., Zhou, J., Pan, L., Wang, X., Wang, F., Hao, Y., et al. (2013). The Main Origins of High Quality Reservoir in Feixianguan Formation in Northeast Sichuan Basin: Atmospheric Water Eluviation and Seepage-Reflux Dolomitization. Nat. Gas. Geosci. 24 (1), 9–18.
Zheng, Y., Lin, F., Wang, T., and Yan, W. (1990). Geologic and Geochemical Conditions of Formation of Zhongba Condensate Gas Fields in Northwest Sichuan. J. Southwest Petroleum Inst. 12 (4), 18–30. doi:10.3863/j.issn.1000-2634.1990.04.003
Zhou, S., Wang, X., Zeng, D., He, B., and Zhou, X. (2015). Geochemistry and Accumulation Analysis of Gas Reservoir of Leikoupo 43 Sub-member of Middle Triassic in Longgang Area, Central Sichuan Basin. Xinjiang Pet. Geol. 36 (4), 415–422. doi:10.7657/XJPG20150407
Zhu, H., and Zhong, D. (2013). Characteristics and Formation Mechanism of the Triassic Feixianguan Formation Reservoir in Longgang Gas Field, Sichuan Basin. J. Palaeogeogr. 15 (2), 275–282. doi:10.7605/gdxh.2013.02.023
Keywords: Sichuan Basin, Leikoupo Formation, natural gases, geochemistry, genetic type, gas source
Citation: Qin S, Zhang B, Huang C, Li J, Wang J, Tao G and Zhou Z (2022) Genetic Type and Source Analysis of Natural Gas in the Leikoupo Formation of the Sichuan Basin in China. Front. Earth Sci. 10:891252. doi: 10.3389/feart.2022.891252
Received: 07 March 2022; Accepted: 22 June 2022;
Published: 19 July 2022.
Edited by:
Maowen Li, SINOPEC Petroleum Exploration and Production Research Institute, ChinaReviewed by:
Shuai Yin, Xi’an Shiyou University, ChinaCopyright © 2022 Qin, Zhang, Huang, Li, Wang, Tao and Zhou. This is an open-access article distributed under the terms of the Creative Commons Attribution License (CC BY). The use, distribution or reproduction in other forums is permitted, provided the original author(s) and the copyright owner(s) are credited and that the original publication in this journal is cited, in accordance with accepted academic practice. No use, distribution or reproduction is permitted which does not comply with these terms.
*Correspondence: Shengfei Qin, cXNmQHBldHJvY2hpbmEuY29tLmNu
Disclaimer: All claims expressed in this article are solely those of the authors and do not necessarily represent those of their affiliated organizations, or those of the publisher, the editors and the reviewers. Any product that may be evaluated in this article or claim that may be made by its manufacturer is not guaranteed or endorsed by the publisher.
Research integrity at Frontiers
Learn more about the work of our research integrity team to safeguard the quality of each article we publish.