- 1Facaulty of Engineering, China University of Geosciences, Wuhan, China
- 2Central Southern China Electric Power Design Institute Co., Ltd. of China Power Engineering Consulting Group, Wuhan, China
Complex geological environment and climatic conditions lead to frequent geological disasters in the Three Gorges Reservoir area. In particular, due to the impact of reservoir impoundment, a large number of landslides have occurred, resulting in huge economic losses and casualties. In this study, the bedding rock landslide is taken as the research object. First, the DTA-138 geotechnical ring shear apparatus system is used for the ring shear test. Second, a two-dimensional numerical model of Shanshucao landslide was established, and simulation was carried out. The main conclusions are as follows: 1) The shear stress–shear displacement curves of sliding zone soil under different shear rates show typical strain softening characteristics. 2) The shear rate–internal friction angle curve showed a good logarithmic relationship. 3) The fundamental reason for the rapid sliding into the river after the instability of the main sliding zone of the Shanshucao landslide is the negative rate effect of the argillized interlayer sliding zone soil in the main sliding zone.
Introduction
Bedding slope in the Three Gorges Reservoir area is numerously developed. According to the literature, the length of reservoir bank with bedding slope in the Three Gorges Reservoir area is 981.83 km (Yin, 2002), and about 62% of bedding slopes in the reservoir are rock slope. As the influence of concentrated rainfall and water level fluctuation, bedding rock slope is more prone to deformation and failure than other types of slope (Zou, 2014). In addition, landslide of this type often slide fast and occurred suddenly, which is often difficult to predict and easily causes huge damage, such as the Jibazi landslide of Yunyang in July 1982, Qianjiangping landslide in 2007 (Wang et al., 2008), and Jiweishan landslide of Wulong in 2009 (Liu, 2010).
Geological disasters frequently occur in the Three Gorges Reservoir area (Cai, 2019; Fu et al., 2017; Fu et al., 2020; Zhou et al., 2022). Zigui County has high frequency of geological disasters, heavy disaster, and high density, and it is one of the high-incidence areas of geological disasters in China (Li et al., 2019). In addition to the Qianjiangping landslide mentioned above, the Shanshucao landslide is the most serious bedding rock landslide in this area which occurred in recent years. Xu et al. (2015) gave a report on the landslide, which occurred on 2 September 2014, in the town of Shazhenxi, on the left bank of the Luogudong River, a secondary tributary of the Yangtze River. As reported, it was affected by continuous rainfall for several days and due to the rise of reservoir water, an obvious deformation appeared on the slope on that day, and then, its instability accelerated the decline. Landslide rushed into the river straightly like a warship. Meanwhile, it caused a large-scale decline in the soil traction area, the Daling hydropower station and staff dormitory building on the slope were completely damaged. According to the memories of witnesses at the scene, the landslide experienced a sliding process of acceleration, blocked deceleration and stop, and the whole process lasted about 3–10 min. Due to the obstruction of the right slope body during the sliding process, the sliding direction was deflected and the obstruction of the riverbed at the front edge reduced the sliding speed substantially. Finally, the landslide was calmly drawn into the river without causing surges. The maximum slip distance of landslide is about 160 m, according to the estimation of the shortest time for 3 min, with an average sliding velocity of 53 m/min. According to the classification methods (Hutchinson and Tika, 1999), the landslide was a medium-high-speed landslide.
The Shanshucao landslide is not a high-speed and long-distance landslide, but its destructive power can’t be underestimated. Obviously, it is very important to study the sliding mechanism of this type of landslide. The essence of studying landslide sliding mechanism from the perspective of mechanical test is to reveal landslide materials, especially the change rule of shearing strength of the sliding zone soil. According to the rock mechanics theory, the shearing strength of the sliding zone soil is a dynamic parameter with time-efficient. If the shearing strength is considered as a static parameter to be introduced into the dynamic landslide deformation analysis, the stability condition of landslide can’t be accurately reflected. When a landslide occurs, the shearing strength provided by the slip soil is close to the residual strength. With the change of shear rate of the sliding zone, the residual strength will change, which directly affects the deformation behavior of the landslide.
Ring shear test is mostly used in the study of residual strength of sliding zone soil, such as the Vaiont landslide (Liu, 2002), Qianjiangping landslide studied by Wang et al. (2007), and Yigong landslide studied by Hu et al. (2009). The ring shear apparatus can simulate the shear conditions during long-distance sliding of landslide soil mass, and can complete maximum deformation under vertical pressure. It is suitable for measuring residual strength under large deformation shear, especially for research of long-distance landslide and debris flow. Wang et al. (2012) carried out ring shear tests on the sliding zone soil of a landslide and proposed that the residual strength is significantly affected by shear rate. It can be seen that the existing research focuses on the rate effect and its generating mechanism of residual strength of soil mass, but lacks the research on the change rules of rate effect of residual strength of sliding zone soil under the main control factor. Meanwhile, the study of relationship between rate effect and deformation behavior of landslide is less. In this article, the author takes the Shanshucao landslide as an example to conduct consolidation drained tests on the remodeling sample of slip soil, and studying the influence of residual strength change of Shanshucao sliding zone soil of on deformation behavior of landslide and taking tests by using ring shear apparatus with different rates as variables.
Overview of the Shanshucao Landslide
Description of the Shanshucao Landslide
The Shanshucao landslide is located in Shazhenxi town in the west of Zigui County. According to the published literature (Zhou et al., 2020), the average elevation in this area is greater than 1,000 m and the terrain elevation difference is 300∼1,000 m. It is located in the ExiFold tectonic zone. There are many roads in the region and the traffic is unobstructed. G348 national highway passes through the territory, there are also waterways connected with other cities. The landslide is about 90 km from Zigui county highway and 42 km from the Three Gorges Dam. According to the attitude of sediments, the mountains in this region can be considered to be in the formation of asymmetric slopes.
As mentioned previously, the Shanshucao landslide occurred on 2 September 2014, along the left bank of the Luogudong River, the second tributary of the Yangtze River (Kang et al., 2020). The ridge elevation is 440 m and the riverbed elevation is 140∼145 m. The difference between the two is about 300 m. The average slope of the Shanshucao landslide is 18°∼25°. The landslide below 175 m is affected by the fluctuation of the reservoir water level. The landslide eventually formed an area of 3.8 × 104 m2 (Figure 1) and caused the plant and units of the Daling Hydropower Station, the 5-storey staff dormitory and the 200 m route along the G348 national highway to slide into the Three Gorges Reservoir. Moreover, the landslide also damaged two rural highways total 450 m (Tian et al., 2018).
Geological Condition of the Shanshucao Landslide
The west boundary of the Shanshucao landslide is a rock wall which has strike about 35°, the dip is 85° and the size is 24 × 20 m2. The southern boundary is about 20 m high, about 107° in strike, about 86° in dip, and about 90° at the elevation of 235 m in the middle. The northern boundary is a 20 m cliff on the left. The eastern boundary is the steep wall formed by the cutting of Luogudong River in the lower part of the slope, which is about 15 m high and close to upright. The modified interlayer constitutes the bottom sliding surface of the landslide and is about 15 cm thick (Figure 2).
The landslide had an irregular rectangular shape, with a length of 350 m and a maximum width of 140 m. The highest elevation of the main scarp is about 283 m. Based on the sliding distance and substance of sliding mass, the Shanshucao landslide was divided into three subzones (Figure 3): the main sliding area (I) and two separated sliding areas (II and III).
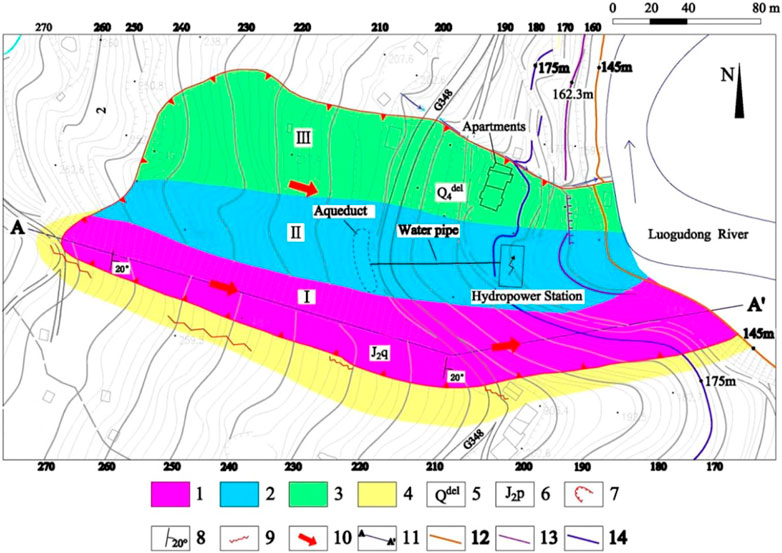
FIGURE 3. Engineering geological plane and zoning map of landslide. 1, The main rock sliding zone (I); 2, tractive zone (II); 3, tractive zone (III); 4, unloading area; 5, landslide formation; 6, Middle Jurassic Qianfoya formation; 7, boundary of the landslide; 8, attitude of strata; 9, fractures; 10, sliding direction; 11, longitudinal section trace; 12, the lowest water level; 13, water level on the day of the event; and 14, the highest water level.
The main sliding area and unloading zone were in the main rock sliding area, the maximum length and width of rock sliding mass were 350 and 40 Shear ratem, respectively, for an area of 1.4ha. The sliding plane was exposed at the main scarp, with a length of 160 m, and the toe slid into the Luogudong River. Due to the long displacement of the main rock sliding mass (I), an unloading zone area about 1700 m2 was induced along the new-born overhanging rock cliff on the right side. On the left side of the main rock sliding mass (I), two soil-slipped zones (II and III) were developed, with a length of 260 m and a width of 90 m. The bulk volume of Shanshucao landslide is 55.1 × 104 m3.
The longitudinal section of the main sliding block of Shanshucao landslide is shown in Figure 4. The bedrock is feldspathic quartz sandstone, silty mudstone, and sandy shale of the Middle Jurassic Qianfoya formation (J2q), with the dip direction of 120° and dip angle of 20°.
The sliding zone soil develops between feldspar quartz sandstone and sandy shale at a certain depth. It was an argillized interlayer formed by the softening and argillization of thin mudstone under the long-term action of groundwater, and the color is gray-green. The thickness was thin and extremely uneven, generally 5∼15 cm.
The particle size analysis and liquid-plastic limit combined method are carried out according to the standard for geotechnical test methods. The results are shown in the Figure 5 and the Table 1. The results show that the proportion of particles larger than 0.075 mm is about 23%, which is higher than other landslides, such as the Qianjiangping landslide.
The content of silt and clay was relatively high, and the natural sliding zone soil was in a plastic state, which is closely related to the weak permeability and relative water resistance of the sliding zone soil.
Geological Analysis
Due to the effect of regional tectonic stress, two groups of joints L1 (15°∠86°) and L2 (130°∠85°) were measured on the south side of Shanshucao, and both of them were tensile fractures. By drawing the stereographic projection of the slope structure (Figure 6). It clearly shows the slope structure which is highly instable, composed of layer, fracture surface, and weak layer. As shown in the figure, in the two groups of tension fractures, L1 forms the right boundary, L2 forms the back boundary, and both structural planes are separated structural planes (Zhang et al., 2021). Fractures and weak layers cut the landslide into cube blocks to promote rock weathering.
Because of the above reasons, there are many factors of landslide. According to the findings, there was continuous rainfall in the Shanshucao area before the landslide. The rainfall lasted for a long time and it was a heavy rainfall, which had a huge adverse impact on the slope. Secondly, the reservoir water level of the Three Gorges Reservoir rose rapidly before the landslide, which also reduced the stability of the slope. Besides, human beings have built a lot of projects in the landslide area, which have changed the stress direction of the landslide, and it made the landslide more instable.
Ring Shear Test and Back Analysis of Parameters
Ring shear apparatus is a geotechnical test equipment to study the mechanical properties of soil under large shear displacement (Sun et al., 2009). The ring shear test can effectively study the residual strength of the excavated body on the shear plane (Hong et al., 2009). Through the shear test of sliding soil, the variation law of residual strength of sliding soil with different shear rates was obtained.
Testing Equipment
At present, the ring shear apparatus is mainly Bishop type and Bromhead type. The test principles of the two instruments are the same, but the test shear boxes are different (Figures 7A,B). This test adopts the DTA-138 georing-shear instrument system developed by Japan Cheng Research Institute (Figure 7C), which is mainly composed of vertical pressure system, rotation system and data acquisition instrument. The structure and working principle of the shear box of the ring shear apparatus are similar to those of the Bishop ring shear apparatus. It is a shear box with upper and lower separations. The outer sleeve is equipped with a guide tube attached to the rubber ring, which cannot only prevent the sample from extrusion, but also reduce the friction force. The instrument is mainly used to study the relationship between shear stress and shear displacement of soil after peak strength. The characteristic is that the adjustable range of shear rate is large (0.00055–109 mm/min), and the drainage conditions in the shear process can be effectively controlled.
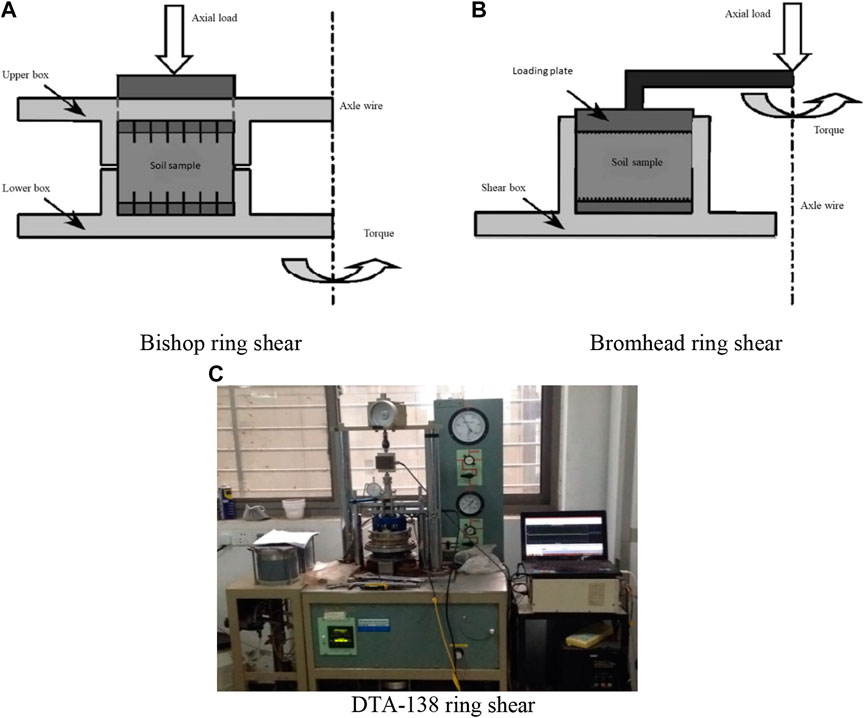
FIGURE 7. Testing equipment. (A) Bishop ring shear. (B) Bromhead ring shear. (C) DTA-138 ring shear.
Experimental Principles
Since the width of the specimen is narrower than the radius of the soil ring, it is generally assumed that the shear stress is uniformly distributed along the shear surface. During the ring shear test, the shear deformation of the interface changes with the radius. Therefore, the average shear stress and average shear displacement are used, and the calculation formula is
In this formula, M is torque, recorded by DTA-138 ring shear system, unit KN;
In the formula, P is the vertical load acting on the sample, and the unit is KN. DTA-138 ring shear apparatus records torque, shear time, friction, and other related parameters through sensors, and then calculates the stress and displacement data through the above formula.
Sample Preparation
The preparation process of remolded samples is as follows: first, the fresh sliding soil is dried in an oven (the oven temperature is 105°C for more than 24 h), and the dried soil sample is hammered and sieved (the sieve aperture is 2 mm); second, addition of distilled water to configure natural moisture content (16.5%) soil samples and wrapping them with preservative film for more than 12 h; the annular sample was prepared by pressure sample method, and the saturated sample was obtained by vacuum saturation method (extracting 2 h and soaking in distilled water for more than 10 h). The production process is shown in Figure 8.
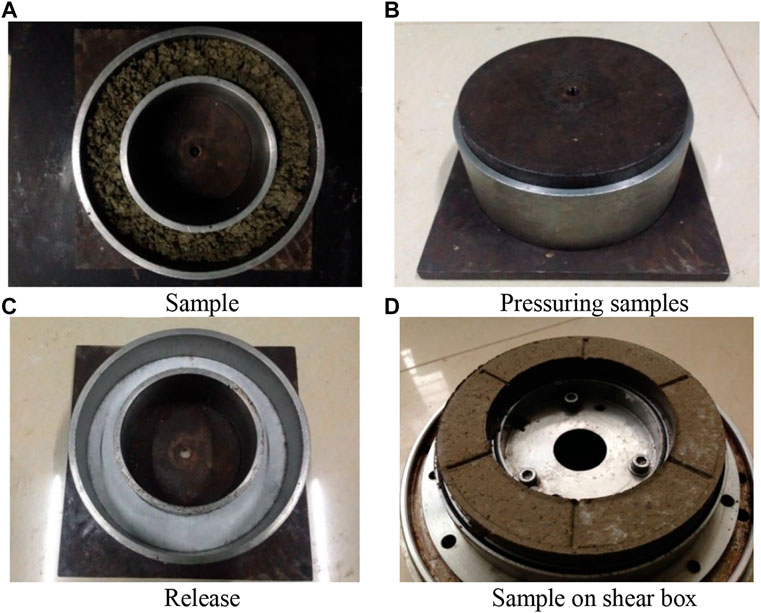
FIGURE 8. Preparation process of remodelling ring shears. (A) Sample. (B) Pressuring samples. (C) Release. (D) Sample on the shear box.
Test Scheme
At present, the main ring shear test methods are single-stage shear, multi-stage shear, and pre-shear. The single-stage shear was used in this experiment. Although it required more soil samples and took a long time, the residual strength obtained was relatively more accurate (Wang et al., 2012).
The existing research shows that the residual strength of soil has nothing to do with the stress history and initial structure (Liu et al., 2004). The residual strength could be obtained by laboratory test of remolded soil samples without using undisturbed soil samples.
The shear process adopts consolidated drainage shear, that is, the sample is consolidated under different normal stress for more than 24 h before shear, and the drainage valve is opened during the shear process. The reason for adopting drainage shear is mainly considering the occurrence environment of sliding zone soil. The upper part is weathered shale mudstone, and the lower part is relatively water-resisting sandstone. During the shear process, groundwater is concentrated at the bottom of the sliding body.
Four shear rates of 0.1,1,10,40 mm/min were selected for ring shear test. The numerical selection of shear rate is based on the classification of landslide movement speed by IUGS landslide working group, which are slow speed (0.003 ∼ 0.3 mm/min), medium speed (0.3 ∼ 30 mm/min), fast speed (30 ∼ 3000 mm/min), and high speed (3,000 ∼ mm/min). Several scholars (Skempton, 1985; Chen, 2012; Bhat and Yatabe, 2015) found that the slow shear rate (less than 0.01 mm/min) in the conventional test had little effect on the residual strength of soil, which could be ignored. In addition, the shear rate exceeds a certain value (100 mm/min) It is easy to cause serious soil compaction in the test, and the test results are discrete (Hu, 2012).
The average sliding velocity of the Shanshucao landslide is about mm/min, which is a rapid landslide.
Test Results and Back Analysis of Parameters
The failure surface morphology of shear argillized interlayer sliding soil formed at different rates is shown in Figure 9. When the shear rate is low, the scratches are scattered and not obvious, and the surface is rough. When the shear rate is high, the failure surface of the sample is smooth as mirror, and the gray-black particle plane formed by friction is visible.
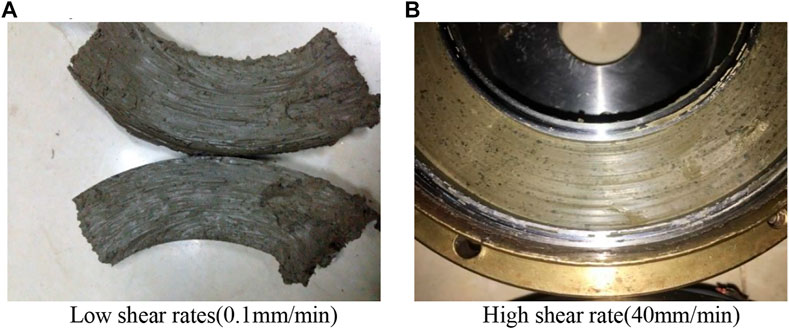
FIGURE 9. Failure surfaces of clayey interlayer sliding soil samples with different shear rates(400 kPa). (A) Low shear rates(0.1 mm/min). (B) High shear rate (40 mm/min).
The results of shear stress-shear displacement curves (400 kPa) of sliding zone soil under four different shear rates are shown in Figure 10.
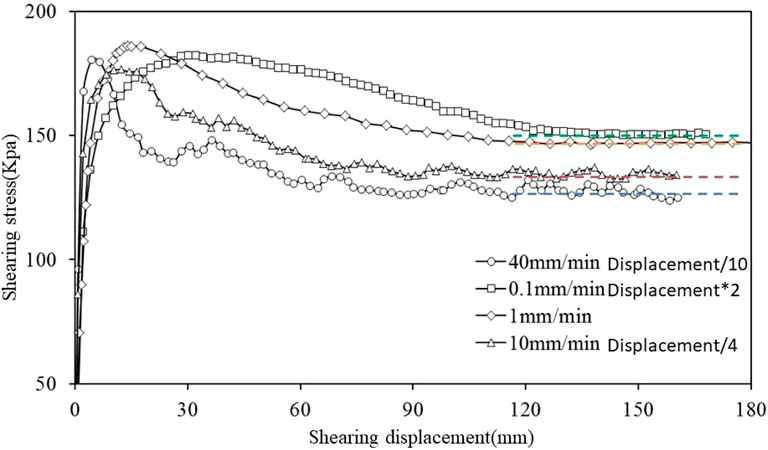
FIGURE 10. The shear stress–shear displacement curves of argillized interlayer sliding soil under different shear rate.
The curve shows typical strain softening characteristics. When the shear rate was low (0.1 mm/min, 1 mm/min), the curve changed gently and showed a slowly decreasing trend. When the shear rate was high (10 mm/min, 40 mm/min), the curve fluctuated greatly, and the residual state needed more deformation. It shows that the soil particle adjustment is more complete when the shear rate is large, and the pore pressure excited by high speed is larger, and the dissipation time is longer. The excitation-dissipation process will also strengthen the fluctuation of the curve. Compared with the shear stress-shear displacement curve of specimens sheared at 0.1 ∼ 40 mm/min, the deformation required to reach the residual state increases significantly, but faster. When the rate increases from 0.1 mm/min to 40 mm/min, the peak stress of the curve is similar, but the residual stress decreases significantly and the peak residual stress increases gradually.
The residual strength of soils with different shear rates is shown in Table 2. Through comparison, it is found that the greater the normal stress, the greater the residual strength. The reason is that the larger normal stress helps to enhance the friction and occlusion between particles, which makes the residual strength relatively high (Wang et al., 2017). At the same time, the residual strength increases with the increase of shear rate.
According to the ring shear test results, the residual cohesion and residual internal friction angle corresponding to residual cohesion and residual internal friction angle can be obtained by fitting the residual strength envelope curves under different rates. With the increase of shear displacement, the interaction force between soil particles becomes smaller and the cementation becomes worse, so the cohesive force Cr is generally a small value. The variation of residual strength of sliding zone soil is essentially the analysis of the variation of residual friction angle φr (Tiwari and Marui, 2004; Wu et al., 2011).
The values of cr and φr fitted by the test results are shown in the Figure 11. It can be seen that the two residual strength indexes change simultaneously under different shear rates. In order to obtain the one-to-one correspondence between shear rate and shear strength, the calculation formula of φe can be derived from the concept of equivalent internal friction angle (φe) in rock mass according to the principle of equal shear strength.
According to Eq. 5, the equivalent internal friction angles of different shear rates are calculated, and the relationship curve between shear rate and internal friction angle is obtained. It can be seen that the curve has a good logarithmic relationship, which is basically consistent with the research results of SUZUKI (Suzuki et al., 2007).
The residual strength index of cohesive interlayer sliding zone soil under different shear rates is shown in Table 3. The higher the shear rate is, the lower the residual cohesion is, lower the residual internal friction angle is, and lower the comprehensive internal friction angle . Using the relationship between the shear rate and the comprehensive internal friction angle in Figure 12, it can be seen that there is a decreasing non-linear relationship between the two parameters.

TABLE 3. Residual strength index of clayey interlayer sliding zone soil under different shear rates.
The back analysis calculation is an indirect method to determine the residual strength parameters of sliding zone soil according to the existing deformation investigation and stability judgment, which is an important supplement to the laboratory test parameters. The combination relationship of c and φ values in three different stable states under natural and heavy rainfall conditions is calculated (Figure 13). If c value is 0, the φ values in the critical sliding limit equilibrium state under natural and heavy rainfall conditions are 18.7° and 21.4°, respectively. The comprehensive residual friction angle obtained from the ring shear test method is not much different from the results calculated by the inverse analysis.
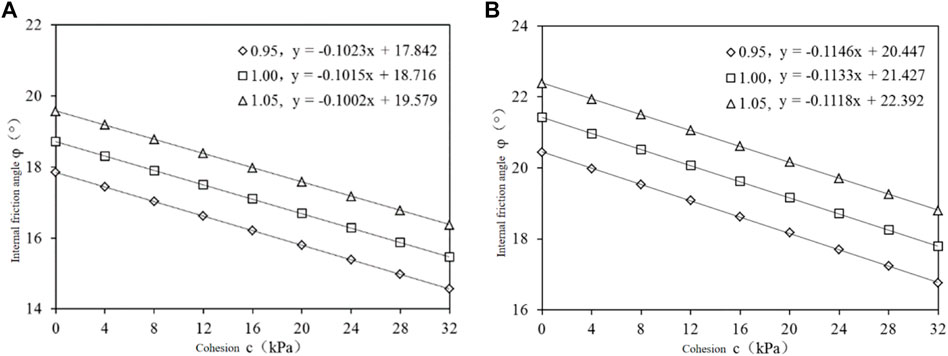
FIGURE 13. Calculation results of inverse analysis. (A) Natural groundwater-free state. (B) Groundwater in heavy rainfall.
Discussion
According to the experimental results, internal friction angle φ E is a function of shear rate V, expressed as follows:
In other words, the residual strength of sliding zone soil is a dynamic parameter of shear rate. There are three effects of shear rate on residual strength of sliding zone soil (Lemos, 2003), namely positive rate effect, no rate effect, and negative rate effect. The rate effect of residual strength of sliding zone soil depends on its own properties. For example, sliding zone soil with low clay content is easy to show negative rate effect, while sliding zone soil with high clay content is more likely to show positive rate effect (Miao, 2012).
The above test results show that the residual strength of sliding zone soil of Shanshucao landslide is a typical negative rate effect, which is consistent with its low clay content and high coarse particle content, that is, the residual strength decreases non-linearly with the increase of shear rate. In other words, once the landslide starts, it is difficult to control and easy to develop into a fast or high-speed landslide, which is consistent with the case of the deformation and decline of Shanshucao landslide. Representative landslides with the same characteristics include the Vaiont landslide (Liu, 2002), Qianjiangping landslide (Wang et al., 2007), and Yigong landslide (Hu et al., 2009).
In order to verify the correctness of the above analysis, a two-dimensional numerical model of Shanshucao landslide is established by FLAC3D to simulate the deformation after landslide instability under negative rate effect. The yield criterion of the model is Mohr-Coulomb criterion, and the sliding zone soil adopts low strength solid element. Set the boundary conditions of rainfall and reservoir water level change during 8.2 ∼ 9.2 (see Figure 14), and select the landslide instability on September 3 as the initial calculation point.
The specific simulation method is to use Fish language, extract the average rate of each unit of sliding zone soil, bring it into the fitting formula (Eq. 6) obtained in Figure 5, and update it every 50 steps, so as to realize the dynamic change of residual strength of sliding zone soil.
As the shear rate of the landslide is very small at the beginning, the result is even less than 0 kPa. Therefore, it is assumed that when the deformation rate of landslide is less than 0.1 mm/min, φe is a certain value (the rate is 0.1 mm/min). Only when it is bigger than this rate, it will be brought into the calculation of rate effect formula φe value.
Table 4 shows the physical and mechanical parameters of landslide rock and soil mass, which mainly refers to the indoor soil test result, and unifies the engineering geology analogy obtains.
Figure 15 shows the horizontal displacement rate and the cumulative horizontal displacement curve of monitoring point D at the sliding belt. It can be seen that the landslide continues to accelerate deformation, and the rate of first 3,000 steps increases faster and acceleration is bigger. After 8,000 steps, the rate reaches 52.1 mm/min, and produces 6.7 m cumulative horizontal displacement.
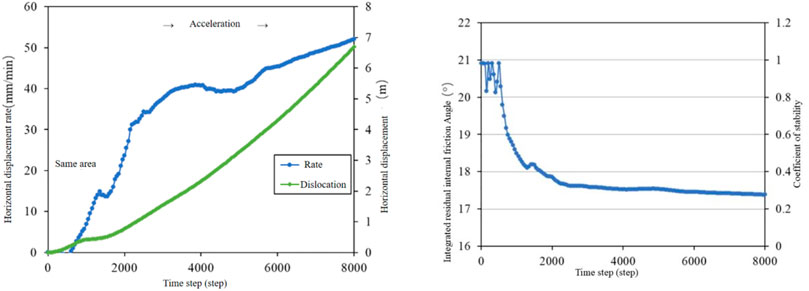
FIGURE 15. Horizontal displacement rate and horizontal cumulative displacement curve of monitoring point D.
The residual strength variation of sliding zone soil can be divided into two parts: one part is the complex fluctuation in the first 500 steps, and the other part is the logarithmic curve attenuation after 500 steps, but the variation law is not a simple linear change obtained in laboratory test. In the 8,000 time step calculated in this study, the comprehensive internal friction angle decreases by 3.5°, the landslide stability coefficient decreases by 0.2, the horizontal deformation rate increases to 52.1 mm/min, and the cumulative horizontal displacement of 6.7 m is generated. It can be seen that the negative rate effect of argillization interlayer sliding zone soil in the main sliding zone of Shanshucao landslide is the fundamental reason for the rapid sliding into the river after the instability of the main sliding zone and the maximum 160 m displacement.
6 Conclusion
In this article, taking the Shanshucao bedding rock landslide as the research object, the DTA-138 geotechnical ring shear system is used to carry out the ring shear test and FLAC3D is used to establish a two-dimensional numerical model of the Shanshucao landslide to simulate the deformation after landslide instability under negative rate effect. The conclusions are as follows:
(1) The shear stress–shear displacement curve of sliding zone soil under different shear rates shows typical strain softening characteristics. When the shear rate was low (0.1 mm/min, 1 mm/min), the curve changed gently and showed a slowly decreasing trend. When the shear rate was high (10 mm/min, 40 mm/min), the curve fluctuated greatly and the residual state needed more deformation.
(2) According to the results of the ring shear test, the envelope curve of residual strength under different rate conditions can be fitted, and the corresponding residual cohesive force and internal friction angle can be obtained. The curve of shear rate–internal friction angle shows a good logarithmic relationship.
(3) In order to verify the test results, an FLAC3D numerical model was used to simulate the deformation after landslide instability under the negative rate effect. The final results show that the negative rate effect of the clayed interlayer sliding zone soil in the main sliding zone is the fundamental reason for the rapid sliding into the river after the instability of the main sliding zone of the Shanshucao landslide, and the maximum displacement is 160 m.
Data Availability Statement
The original contributions presented in the study are included in the article/Supplementary Material, further inquiries can be directed to the corresponding author.
Author Contributions
W-nL contributed to the conception, methodology, formal analysis, investigation, data curation, original draft preparation, review and editing, and visualization. G-lX contributed to the conception, methodology, investigation, review and editing, supervision, and project administration. ZY curated the data and contributed to the visualization.
Funding
This study was sponsored by the Key R&D Plan of the Hubei Provincial Department of Science and Technology (No. 2021BCA219).
Conflict of Interest
Author YZ was employed by Central Southern China Electric Power Design Institute Co., Ltd. of China Power Engineering Consulting Group.
The remaining authors declare that the research was conducted in the absence of any commercial or financial relationships that could be construed as a potential conflict of interest.
Publisher’s Note
All claims expressed in this article are solely those of the authors and do not necessarily represent those of their affiliated organizations, or those of the publisher, the editors, and the reviewers. Any product that may be evaluated in this article, or claim that may be made by its manufacturer, is not guaranteed or endorsed by the publisher.
References
Bhat, D. R., and Yatabe, R. (2015). Effect of Shearing Rate on Residual Strength of Landslide Soils. Eng. Geology. Soc. Territory 2, 1211–1215. doi:10.1007/978-3-319-09057-3_212
Cai, C. (2019). Study on the Types and Mechanism of Geological Disasters in the Three Gorges Reservoir Area[J]. Disaster Sci. 34 (02), 37–42. doi:10.3969/j.issn.1000-811X.2019.02.008
Chen, T. (2012). Experimental Study on Residual Strength of Slip Soil Guangzhou: College of Technology. Jinan: Jinan University, 16–17.
Fu, X., Sheng, Q., Li, G., Zhang, Z., Zhou, Y., and Du, Y. (2020). Analysis of Landslide Stability under Seismic Action and Subsequent Rainfall: a Case Study on the Ganjiazhai Giant Landslide along the Zhaotong-Qiaojia Road during the 2014 Ludian Earthquake, Yunnan, China. Bull. Eng. Geol. Environ. 79, 5229–5248. doi:10.1007/s10064-020-01890-z
Fu, X., Sheng, Q., Zhang, Y., Chen, J., Zhang, S., and Zhang, Z. (2017). Computation of the Safety Factor for Slope Stability Using Discontinuous Deformation Analysis and the Vector Sum Method. Comput. Geotechnics 92, 68–76. doi:10.1016/j.compgeo.2017.07.026
Hong, Y., Sun, T., Maotian, L., Zheng, X., and Wang, F. (2009). Geotechnical Ring Shear Apparatus Development and Application Research Status [J]. Geotechnical Mech. 30 (03), 628–634. doi:10.16285/j.rsm.2009.03.043
Hu, M., Cheng, Q., and Wang, F. (2009). Experimental Study on Formation Of Yigong Long-distance High-speed Landslide [J]. Chinese J. Rock Mech. Eng. 28 (1), 138–143.
Hu, X. (2012). Residual Strength Variation of Debris Landslide Slip Soils under Different Shear Rates [D]. Wuhan, China: University of Geoscience, Faculty of Engineering, 39–60.
Hutchinson, J. N., and Tika, T. E. (1999). Ring Shear Tests on Soil from the Vaiont Landslide Slip Surface[J]. Geotechnique 49 (1), 59–74.
Kang, X., Xu, G., Yu, Z., Wang, S., and Wang, M. (2020). Experimental Investigation of the Interaction between Water and Shear-Zone Materials of a Bedding Landslide in the Three Gorges Reservoir Area, China. Bull. Eng. Geol. Environ. 79 (8), 4079–4092. doi:10.1007/s10064-020-01812-z
Lemos, L. J. L. (2003). “Shear Behaviour of Pre-existing Shear Zones under Fast Loading–Insights on the Landslide Motion[C],” in Proceedings of International Workshop on Occurrence and Mechanisms of Flow-like Landslides in Natural Slopes and Earthfills, Sorrento, Naples, 14–16.
Li, L., Pan, A., Liao, W., Ding, Y., Wang, S., and Tan, J. (2019). Research on the Disaster Model of Typical Geological Disasters in Zigui County [J]. Resource Environ. Eng. 33 (04), 520–524. doi:10.16536/j.cnki.issn.1671-1211.2019.04.015
Liu, C. (2010). Research on the Evolution Dynamics of the Consequent Bedding Rockslides Wulong, Chongqing, June 5, 2009[J]. J. Eng. Geology. 18 (3), 297–304.
Liu, X., Deng, J., and Li, G. (2004). Shear Strength Properties of Slip Soils of Landslides: an Overview [J]. Rock and Soil Mechanics 25 (11), 1849–1854. doi:10.16285/j.rsm.2004.11.038
Miao, H. (2012). Deformation-failure Mechanism and Prediction of the Landslides in Jurassic Red Beds in the Three Gorges Reservoir [D]. Wuhan: China University of Geoscience Faculty of Engineering, 50–51.
Skempton, A. W. (1985). Residual Strength of Clays in Landslides, Folded Strata and the Laboratory. Géotechnique 35 (1), 3–18. doi:10.1680/geot.1985.35.1.3
Sun, T., Hong, Y., Maotian, L., and Rong, C. (2009). Study on Shear Strength Characteristics of Overconsolidated clay by Ring Shear Apparatus [J]. Geotechnical Mech. 30 (07), 2000–2004. doi:10.16285/j.rsm.2009.07.039
Suzuki, M., Yamamoto, T., and Tanikawa, K. (2007). Variation in Residual Strength of clay with Shearing Speed [J]. Mem. Fac. Eng. Yamaguchi Univ. 52 (7), 45–49. doi:10.1038/sj.bdj.2013.990
Tian Pan, H. Z., Jiao, Z., Fu, X., Fan, Y., and Yang, J. (2018). Operation and Effectiveness Analysis of Geological Disaster Monitoring and Early Warning System in the Three Gorges Reservoir Area [J]. Geology. mineral Resour. South China 34 (04), 354–359. doi:10.3969/j.issn.1007-3701.2018.04.011
Tiwari, B., and Marui, H. (2004). Objective Oriented Multistage Ring Shear Test for Shear Strength of Landslide Soil. J. Geotech. Geoenviron. Eng. 130 (2), 217–222. doi:10.1061/(asce)1090-0241(2004)130:2(217)
Wang, F., Zhang, Y., and Wang, G. (2007). Deformation Characteristics of Shuping Landslide Caused by Reservoir Water Level Changes in the Three Gorges Reservoir Area [J]. Chinese Rock Mech. Eng. 26 (3), 509–517. doi:10.3321/j.issn:1000-6915.2007.03.010
Wang, F., Zhang, Y., Huo, Z., Peng, X., Wang, S., and Yamasaki, S. (2008). Mechanism for the Rapid Motion of the Qianjiangping Landslide during Reactivation by the First Impoundment of the Three Gorges Dam Reservoir, China. Landslides 5 (4), 379–386. doi:10.1007/s10346-008-0130-7
Wang, L. M., Yan, E., Song, K., Yang, G., and Wang, J. (2017). The Rate Effect of Landslide Residual Strength and its Influence on Landslide Deformation Behavior [J]. J. Cent. South Univ. ( Nat. Sci. Edition ) 48 (12), 3350–3358. doi:10.11817/j.issn.1672‐7207.2017.12.028
Wang, S., Xiang, W., Cui, D., Jin, Y., and Huang, X. (2012). Experimental Study on Residual Strength of Sliding-Zone Soil with Different Shear Ring [J]. Rock and Soil Mechanics 33 (10), 2967–2972. doi:10.16285/j.rsm.2012.10.006
Wu, D., Jian, W., and Xu, C. (2011). Research on Shear Strength of Residual Soils by Ring Shear Tests [J]. Rock and Soil Mechanics 32 (7), 2045–2050. doi:10.16285/j.rsm.2011.07.014
Xu, G., Li, W., Yu, Z., Ma, X., and Yu, Z. (2015). The 2 September 2014 Shanshucao Landslide, Three Gorges Reservoir, China. Landslides 12 (6), 1169–1178. doi:10.1007/s10346-015-0652-8
Yin, Y. (2002). Geohazards and their Prevention in Resettlement Areas of Emigrants From the Reservoir Region of the Three Gorges project [J]. Geological Bulletin of China 21 (12), 876–880.
Zhang, Q., Hu, H., Zhang, Y., and Zhao, X. (2021). Comparison of Traditional and Whole-Space Stereographic Projection in Block Stability Analysis [J]. Chinese J. Geotechnical Engineering, 1–10.
Zhou, J., Deng, M., Li, Z., Li, Z., Zhang, F., and Lin, Y. (2020). Analysis on the Formation and Apparent‐dip Lateral Sliding Mechanism of Shanshucao Landslide in the Three Gorges Reservoir Area [J]. J. Disaster Prevention and Mitigation Engineering 40 (6), 860–866 + 833. doi:10.13409/j.cnki.jdpme.2020.06.003
Zhou, Y., Sheng, Q., Li, N., and Fu, X. (2022). The Dynamic Mechanical Properties of a Hard Rock under True Triaxial Damage-Controlled Dynamic Cyclic Loading with Different Loading Rates: a Case Study [J]. Rock Mech. Rock Eng. doi:10.1007/s00603-021-02756-w
Keywords: Three Gorges Reservoir (TGR), landslide, ring shear test, numerical simulation, bedding rock
Citation: Li W-n, Xu G-l and Yu Z (2022) Analysis of Failure Behavior Based on the Ring Shear Test in a Bedding Rock Landslide of the Three Gorges Reservoir. Front. Earth Sci. 10:885152. doi: 10.3389/feart.2022.885152
Received: 27 February 2022; Accepted: 21 March 2022;
Published: 28 April 2022.
Edited by:
Xiaodong Fu, Institute of Rock and Soil Mechanics (CAS), ChinaReviewed by:
Lipeng Liu, China Institute of Water Resources and Hydropower Research, ChinaGan Li, Ningbo University, China
Copyright © 2022 Li, Xu and Yu. This is an open-access article distributed under the terms of the Creative Commons Attribution License (CC BY). The use, distribution or reproduction in other forums is permitted, provided the original author(s) and the copyright owner(s) are credited and that the original publication in this journal is cited, in accordance with accepted academic practice. No use, distribution or reproduction is permitted which does not comply with these terms.
*Correspondence: Guang-li Xu, eHUxOTYzQGN1Zy5lZHUuY24=, orcid.org/0002-3631-1180