- 1State Key Laboratory of Petroleum Resources and Prospecting, China University of Petroleum, Beijing, China
- 2College of Geosciences, China University of Petroleum, Beijing, China
Since the discovery of the Yuanba gas reservoir, there has been no unified conclusion of hydrocarbon accumulation. The Feixianguan Formation micrite and argillaceous limestone are controversial as the direct cap rock. This research analyzed the integrity of the limestone layer from delaminated fracturing to judge whether it can be as the caprock and then discuss the mechanism of accumulation. Delaminated fracturing and its controls on hydrocarbon accumulation were studied using seismic data, well logging data, rock mechanic measurements, and petroleum geological data. Mudstones from the Upper Permian Wujiaping Formation serve as the source rock. Changxing Formation dolomite from the Upper Permian is the reservoir rock. The caprock is marlites from the Lower Triassic Feixianguan Formation. The Changxing Formation had middle-high dip angle fractures formed by integrating pore fluid pressures and the tectonic stresses in the Late Triassic Indosinian Movements. The Feixianguan Formation had horizontal stylolites and fewer fractures, which kept the Feixianguan Formation marlites good caprocks. The adjacent Feixianguan and Changxing formations have their fracture system known as delaminated fractures. As a result, the Upper Permian Changxing Formation dolomite has good reservoir properties, while the Lower Triassic Feixianguan Formation limestone or marlites have good capping performance. These delaminated fractures are formed because of different lithologies and thicknesses in the carbonate rock series. It is the key factor in controlling the formation of Yuanba Gas Field with weak deformation. Delaminated fracturing suggests a significant exploration potential in carbonate strata with weak deformation, particularly in ultra-deep areas.
Introduction
Carbonate rocks are common in surface outcrops and underground rock formations and are good targets for petroleum exploration (Ehrenberg and Nadeau, 2005; Jin et al., 2006; Zhao et al., 2014 and Zhao et al., 2015; Zhu et al., 2019). Carbonate rocks are primarily composed of limestone and dolomite with various thicknesses. Carbonate rocks formed primarily in the Sinian, Cambrian, Ordovician, Devonian, Carboniferous, and Permian periods in geological history, accounting for 20% of the area occupied by sedimentary rocks. The reserve and production of oil and gas in carbonate rocks account for half of the world’s total reserves and production. Dolomite has been a good reservoir of carbonate rocks, even in ultra-deep formations (Bildstein et al., 2001; Ehrenberg and Nadeau, 2005; Bai, 2006; Davies and Smith, 2006; Jin et al., 2006; Zhao et al., 2014; Zhao et al., 2015).
The existence of caprocks and the occurrence of fractures or joints will determine the oil- and gas-bearing properties of the carbonate rock areas. The common caprocks are mudstone, shale, evaporites (salt and gypsum), and tight limestone. The tight limestone encased a small petroleum reserve. According to the statistical data from the world’s 334 largest oil and gas fields, mudstone caprock accounts for 65% of the reserve, evaporites account for 33% of the reserve, and tight limestone caprock accounts for 2% of the reserve. In China, the caprocks of large-scale gas fields were composed of 60% mudstone, 18% gypsum salt rocks, 11% muddy shale, and 11% carbonate rocks (Hu et al., 2009).
The fractures are significant in carbonate rocks. They increase the porosity and permeability of carbonate rocks, thereby improving their reservoir properties (Li et al., 2018; Wu et al., 2020; Yang et al., 2020). The fracture density of the different layers varies due to differences in lithology and thickness (Bai and Pollard, 2000; Zeng et al., 2007; Hooker et al., 2013). Conversely, some fractures are linked along the fracture plane or at the intersections of different strike fractures. Some fractures are separated from one another and do not intersect. Tectonic stresses determine the occurrence of fractures, and pore fluid pressures play an essential role in the occurrence of fractures (Handin, 1958; Hubbert and Rubey, 1959; Tingay et al., 2009; Hao et al., 2015). Although fractures can disrupt the continuity of limestones, they can also serve as caprocks and are necessary for carbonate rock areas, particularly in deep or ultra-deep formations (Heap et al., 2018; Toussaint et al., 2018; Bruna et al., 2019). The deep area is 4,500–6,000 m, while the ultra-deep area is more than 6,000 m (Dyman et al., 2002; Zhang et al., 2015). Deep or ultra-deep petroleum traps have recently received increased attention (Arouri et al., 2010; Sun et al., 2013; Katz and Everett, 2016).
The Yuanba Gas Field is an ultra-deep gas field located northeast of the Sichuan Basin. Its source–reservoir–cap assemblage lies in a series of marine strata, including the Upper Permian mudstone source, the Upper Permian dolomite reservoir, and the Lower Triassic limestone cap. Since the discovery of this gas reservoir, there have been discussions on the accumulation mechanism (Guo et al., 2014; Guo et al., 2018; Shao, 2012; Fan, 2012; Fan, 2014), but there has been no unified conclusion of the caprock of the reservoir. The Lower Triassic Jialinjiang Formation gypsum salt rock is generally accepted as the regional caprock, but the Feixianguan Formation micrite and argillaceous limestone are controversial as the direct caprock (Wu, 2020). We analyzed the integrity of the limestone layer from fractures to judge whether it can be as the caprock and then discuss the mechanism of accumulation. Thus, the objectives of this study are based on field observation, seismic data interpretation, core fracture observation, paleo-stress measurement, and fluid pressure analysis. This research will be an important guideline for petroleum exploration in carbonate rocks of ultra-deep formations with weak deformation.
Geological Setting
The Yuanba Gas Field, an ultra-deep carbonate gas reservoir, is located in a weak deformation zone with no faults cutting through the Sinian to Cretaceous strata (Figure 1). It has a general NWW-SSE trend, with the Jiulongshan anticline, Chixi sag, and Tongnanba anticline in the north and the Cangxi-bazhong gentle dip slope in the south (Figure 1).
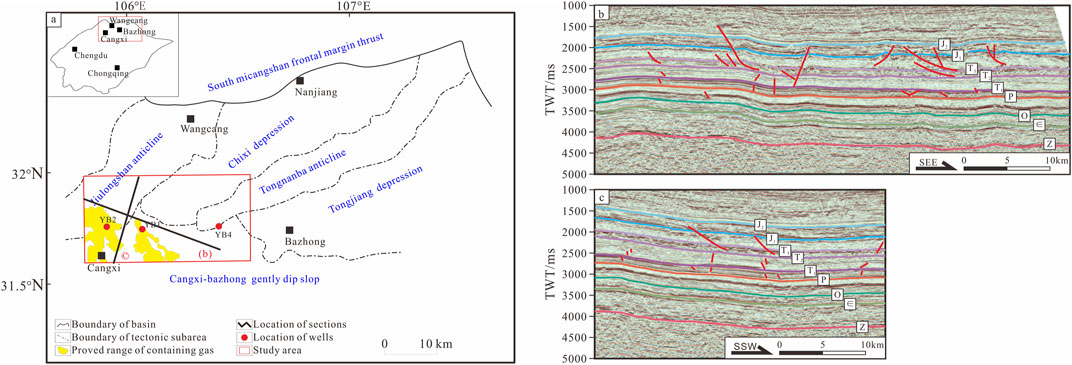
FIGURE 1. Tectonic location and 2D seismic profiles of the Yuanba Gas Field. (A) Location of the study area and seismic profiles (modified after Guo et al., 2018); (B) NWW-SEE seismic profile; (C) NNE-SSW seismic profile.
The strata associated with the Yuanba Gas Field include Permian, Triassic, Jurassic, and Cretaceous (Figure 2). The Permian was composed of marine sediments, shales, limestones, and dolomites. The lower-middle Triassic was composed of marine sediments like shales and limestones and salt-bearing layers like gyprocks, polyhalite rocks, and salt rocks (Zhu et al., 1986). The Permian and Triassic strata belong to continental margin sedimentation. The overlying strata were continental foreland basin sediments, including the Upper Triassic, Jurassic, and Cretaceous with lithologies of sandstones and shales. The Wujiaping Formation is shallow-water shelf facies carbonate rocks with a gentle slope. The lithology is mainly marl, carbonaceous limestone, bioclastic marl, and dark black argillaceous limestone. Based on the different depositional environments, the Changxing Formation can be divided into carbonate platform-type deposits, platform-margin reef-shoal-type deposits, platform-margin slope-type deposits, and shelf-type deposits (Guo et al., 2014). Among them, the platform-margin reef-shoal deposits have the largest thickness, about 200–300 m. They are mainly biogenic limestone, bioclastic limestone, bioclastic dolomite, and sandy dolomite. A set of oolitic limestone or oolitic dolomite develops in the lower part of the Feixianguan Formation, and evaporative platform mudstone or anhydrite develops in the upper part.
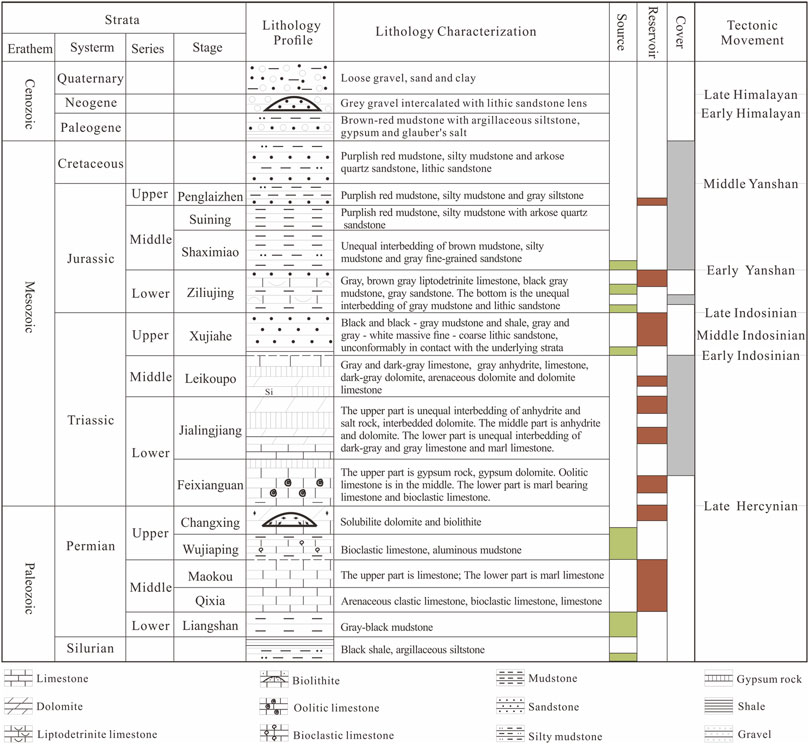
FIGURE 2. Comprehensive column of major source–reservoir–cap assemblages in the Yuanba Gas Field (modified after Guo et al., 2014).
The directions of tectonic stress fields changed several times after forming primary reservoir rocks in the Changxing Formation (Qin et al., 2017; Wang et al., 2019). They referred to several compressions, including the NW-SE compression of the Late Triassic Indosinian movements, the SN compression of the Yanshanian movements, the NW-SE or EW compression of the early and middle Himalayan movements, and the NE compression of the late Himalayan movements (Qin et al., 2017). The present maximum horizontal principal stress is in the NW-SE direction or at 110–120° based on imaging logging data, induced fracture data, and borehole caving data (Guo et al., 2010; Zhang, 2010; Zhao et al., 2017).
The Yuanba Gas Field was discovered in 2007. It was in an area with a gentle dip and weak deformation. The Upper Permian lover part mudstone served as the source rock, the Upper Permian dolomite served as the reservoir, and the Lower Triassic limestone served as the caprock. The paleo-pore fluid pressures, fractures, high temperatures, and confining forces all played important roles in forming the Yuanba Gas Field.
Reservoir Characteristics
Reservoir Type
The structures do not control the distribution of the Yuanba Gas Field, but it is done by organic reef and bank facies at the platform margin (Figure 3). It is a structural and lithologic gas field with reservoirs in both the Changxing and Feixianguan formations. The central pay zone of the Yuanba Gas Field is the Changxing Formation gas reservoir, with a burial depth of 6,682.9 m in the middle of the gas reservoir, which is an ultra-deep, pore-type, and fracture-pore-type gas reservoir (Guo et al., 2014 and Guo et al., 2018; Yuan et al., 2019). The Feixianguan Formation was composed of low-yield gas reservoirs only found in the northwest of Yuanba and had a burial depth of 6,317 m in the middle of the typical gas reservoir (Guo et al., 2014). The mechanism of hydrocarbon accumulation has been debated since the discovery of the Yuanba Gas Field (Fan, 2012; Shao, 2012; Fan, 2014; Guo et al., 2014; Guo et al., 2018). However, the accumulation mechanism is still unknown, such as the gas-bearing difference between the Changxing and Feixianguan formations and the stratum of the caprock.
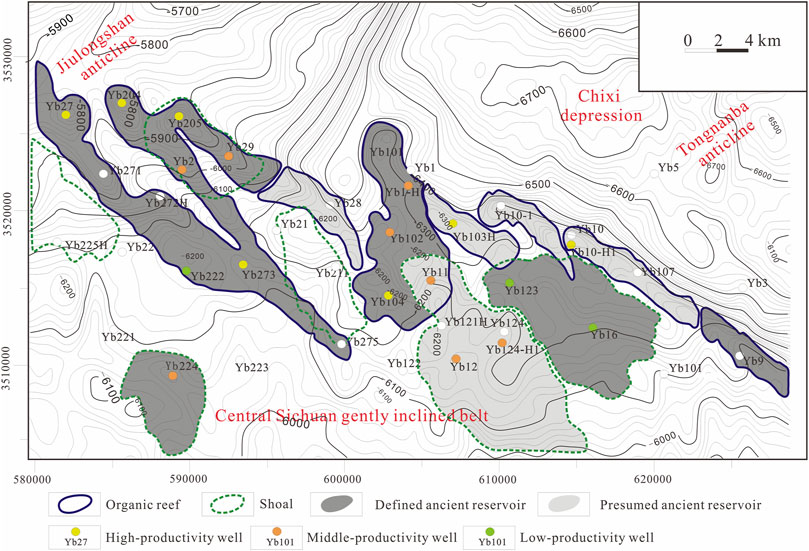
FIGURE 3. Distribution diagram of reservoirs in the Changxing Formation in the Yuanba Gas Field (modified after Guo, 2019).
Source–Reservoir–Caprock Assemblages
The Wujiaping Formation of the Upper Permian is the source rock of the Yuanba Gas Field, with bioclastic limestone and mudstone (Figure 4). The thickness of the source rock in the Yuanba area is 60,100 m. The organic matter in the source rock is primarily sapropel, with a significant part of terrestrial organic matter (Li, 2009).
The primary reservoir rock in the Yuanba Gas Field is the Upper Permian Changxing Formation, which has a burial depth of about 6,680 m, an ultra-deep pore-type fracture-pore-type gas reservoir (Guo et al., 2014; Guo et al., 2018; Yuan et al., 2019). Dolomite and biolimestone are the reservoir rocks (Figures 2, 4). The development and distribution of the reef flat influenced the distribution of the gas reservoir (Figure 3). The Changxing Formation has two reservoir types: porosity-type and fracture-porosity-type. The dominant lithology is crystalline dolomite, bioclastic dolomite, and clastic dolomite, with a trace of clastic limestone and bioclastic limestone (Figures 2, 4). The reservoir rock of the Changxing Formation is mainly found at the platform margins in reefs, retro-reef shoals, and reef flat complexes (Figure 3). The Changxing reservoir rock covers an area of 350,450 km2. The reservoir rock thicknesses range from 30 to 150 m, with an average of 70 m. The reservoir rock in the platform-margin reef flats is thick, whereas those in the retro-reef shoal and intra-platform flat are thin.
For the Yuanba Gas Field, the regional caprock is gypsum, polyhalite, salt rock, and gypsum dolomite of the Lower-Middle Triassic (Figures 2, 4). However, the main gas pay zone of the Yuanba Gas Field is the Changxing Formation of the Upper Permian, and the Feixianguan Formation directly above it only produces gas locally. The Changxing Formation has a local caprock, the marlites, in the Lower Triassic Feixianguan Formation base.
Formation Process of the Gas Reservoir
The Changxing gas reservoir was formed through a complex process, and it is a pyrolysis gas reservoir. The Wujiaping Formation reached its peak oil generation during the Late Triassic to the Early Jurassic, and the paleo-oil reservoir of the Changxing Formation was formed. The oil was generated from the Wujiaping Formation and injected into the Changxing Formation reservoir (Zhang, 2010; Guo et al., 2014; Li et al., 2016). The paleo-oil reservoir was pyrolyzed to form a paleo-gas field at the end of the Middle Jurassic (Zhang, 2010; Guo et al., 2014; Li et al., 2016). The paleo-gas reservoir was adjusted to its present status during the late Yanshanian movements and Cenozoic Himalayan movements.
Fracture Characteristics
It was found that tectonic deformation in the Yuanba Gas Field was weak, with low-magnitude anticlines and no large-scale faults (Figure 1). Different strata have been subjected to various tectonic movements, resulting in multiple fractures. In terms of attitudes, imaging logging data show that the fractures in the Changxing Formation are mostly steep (Figure 5 and Figure 6A) and strike NW-SE (Guo et al., 2010), with 8% at 0–30° dip angles, 35% at 30–60°, and 57% at 60–90°. The fractures in the Feixianguan Formation have multiple strikes and are mostly low-angle fractures and stylolites (Figures 5, 6B), with dip angles of 0–30° accounting for 19%, 3,060° accounting for 52%, and 60–90° accounting for 29%.
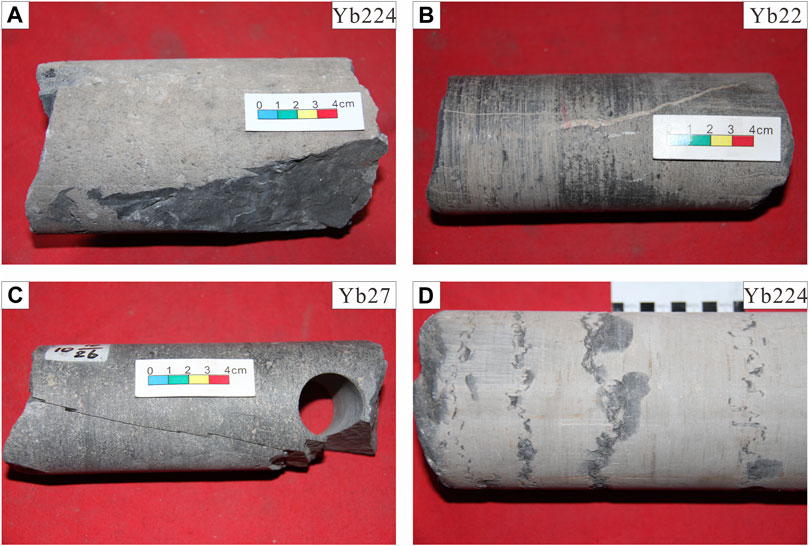
FIGURE 5. Fractures in the Yuanba area. (A) Steep fractures filled by pitch in the Changxing Formation; (B) steep dip fractures filled by calcite in the Changxing Formation; (C) Steep dip fractures without filling in the Changxing Formation; (D) horizontal stylolites in the Feixianguan Formation.
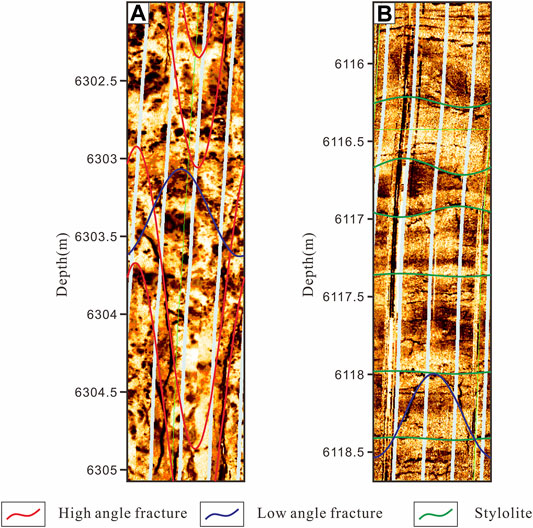
FIGURE 6. Imaging logging of Yb27. (A) High-angle fractures of the Changxing Formation; (B) Stylolites of the Feixianguan Formation.
In terms of fillings and filling degrees, we counted the fillings of the fractures on the core and identified the fillings. Fully filled fractures in the Changxing Formation account for 57%, semi-filled fractures account for 19%, and non-filled fractures account for 24% (Figure 7A). Based on the types and distribution of fillings, there are mainly five types of filling materials: dolomite, calcite, quartz, muddy, and asphalt. The fractures filled with dolomite, calcite, muddy, and asphalt account for 2.7, 15, 8, and 73%, respectively; others were filled with pyrite (Figure 7B). The fractures in the Feixianguan Formation have a filling degree of about 54%, followed by non-filled and semi-filled fractures, accounting for about 23% (Figure 7A). The fillings are mostly calcite materials (Figure 7B), accounting for about 65%, followed by mud accounting for about 16%, and the remaining part is asphalt.
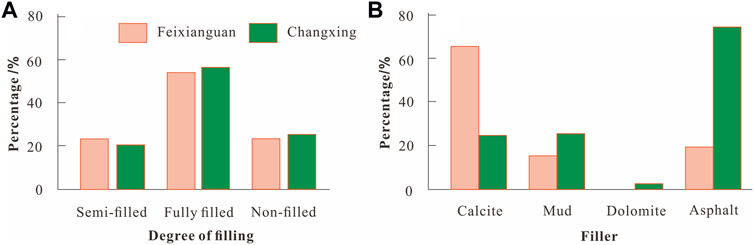
FIGURE 7. Fracture fillings of the Yuanba Gas Field. (A) Histogram of the filling degree; (B) histogram of the filling substance.
Discussion
Several factors influence the formation of the Yuanba Gas Field. The permeability and porosity of the dolomite reservoir were improved by delaminated fracturing. The delaminated fracturing was controlled by the mechanic layers, thicknesses, and pore fluid pressures. High confining pressures in the deep crust gave the marlite high plasticity, ensuring that it was caprock.
Delaminated Fracturing
Delaminated fracturing refers to various fracture densities and patterns in different layers. It is determined by the magnitude of tectonic stresses, the mechanical properties, and the thicknesses of the rocks. The fractures are required to improve the reservoir properties, but extensive deformation will result in fault formation, which will destroy the gas reservoirs. Because of differences in the inner structures, mineral composition, and grain size, the rocks in the Earth’s crust have varying mechanical properties. Different rocks have different fracture conditions, implying that the rock will fracture when the stress reaches the rock strength. In general, the compressive strengths (which also reflect shear and tensile strengths) of rocks increase in strength from dolomite to dense limestone, dense sandstone, anhydrite, mudstone, shale, and gypsum (Ferrill et al., 2017). In terms of strengths, given a rock section composed of shale (mudstone), dolomite, tight limestone, and marlite, the sequence of forming fractures should be shale (mudstone), marlstone, dense limestone, and dolomite. However, there are strains that different rocks can withstand before fractures can vary. As a result, some rocks with higher strengths will fracture earlier than rocks with lower strengths under identical tectonic movements (Figure 8; Ferrill et al., 2017).
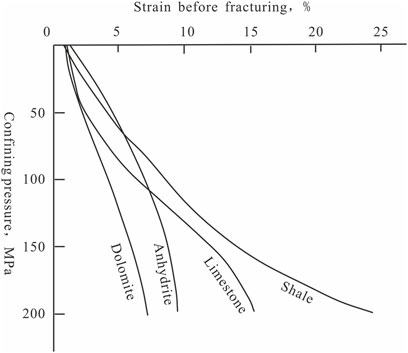
FIGURE 8. Strain curves of rocks before forming fractures under different confining pressures (modified after Ferrill et al., 2017).
For rocks with identical mechanical properties, the density and attribute of fractures formed in one-time deformation will vary due to various thicknesses. Researchers show that joint space in layered sedimentary rocks is proportional to the thickness of the fractured layer (Bai and Pollard, 2000). The delaminated fractures often are observed in outcrops around the Sichuan basin (Figure 9). The inter-bed cohesion will affect fracture development (Hooker et al., 2013; Surpless and Wigginton, 2020).
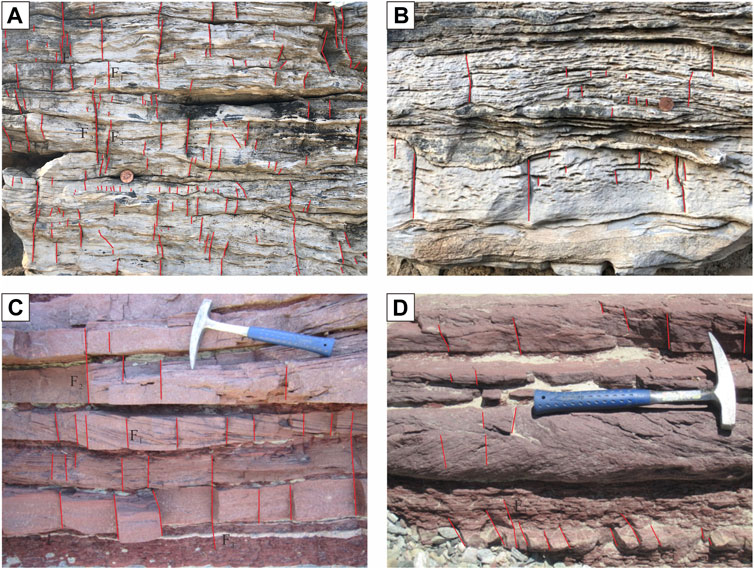
FIGURE 9. Outcrops with delaminated fractures. (A) Carbonate sequence with similar lithology and different thicknesses; (B) carbonate sequence with different lithology; (C) sandstone sequence with similar lithology and different thicknesses; (D) sand and shale sequence. F1 - intrastratal fracture, F2 - interlaminar fracture, and F3 - interlaminar extending fracture.
Medium deformation occurred in the Yuanba Gas Field, and the Feixianguan Formation and Changxing Formation are with fractures but without faults (Figures 1, 3, 10). Fractures increase the porosity and particularly the permeability of the dolomite reservoirs in the Changxing formation (Guo, 2011; Fan, 2012). Because the Upper Permian and Lower Triassic contacted conformally, the Changxing and Feixianguan formations experienced the same tectonic action processes (Figure 10). The Early Indosinian movements in the late Middle Triassic were responsible for forming the NW-SE fractures in the Changxing Formation (Figure 11). The density, dip, strike, and interstitial materials of fractures in the Feixianguan Formation differed from those in the Changxing Formation, indicating delaminated fracturing.
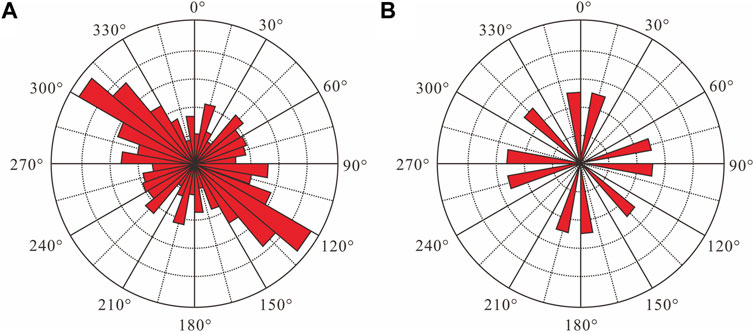
FIGURE 11. Strikes of the fractures in the Yuanba Gas Field. (A) Changxing Formation; (B) Feixianguan Formation.
Pore Fluid Pressure Helping the Fracture Formation
The Mohr envelope curves for dolomite and limestone were obtained by measuring the strength of rock samples collected from well cores in the Yuanba Gas Field and field crops (Table 1 and Figure 12).
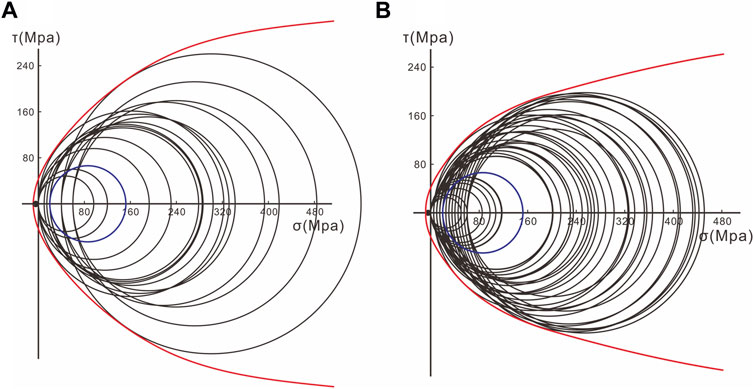
FIGURE 12. Mohr envelope curves in the Yuanba Gas Field. (A) Dolomite of the Changxing Formation; (B) limestone of the Feixianguan Formation.
The Mohr envelope curves of dolomite are as follows:
The Mohr envelope curves of limestone are as follows:
The burial depth of the Changxing and Feixianguan formations in the Late Triassic was 2,200 and 2,000 m, respectively (Figure 13). The density of the overburdened rocks was 2.63 g/cm3. The Poisson’s ratio of rocks was 0.35–0.448. The Poisson stress in the horizontal direction was 19.8 and 18 MPa for the Changxing and Feixianguan formations, respectively. The maximum principal stress measured by the acoustic emission in Indosinian movements was 152.1 MPa (Zhang, 2010). Due to zero porosity in the tight limestone, the paleo-pore fluid pressure was 28.6 MPa for dolomite in the Changxing Formation and 0 for limestone in the Feixianguan Formation (Zhang, 2010). Considering all the above, we got the maximum and minimum principal stresses of 123.5 and −8.8 MPa in Changxing Formation dolomite, respectively. The maximum and minimum principal stresses were 152.1 and 18 MPa in Feixianguan Formation limestone, respectively. Mohr stress circles were constructed for the dolomite and limestone according to the maximum and minimum principal stresses. The figure showed that fractures occurred in the dolomite of Changxing Formation but not in the limestone of the Feixianguan Formation (Figure 14).
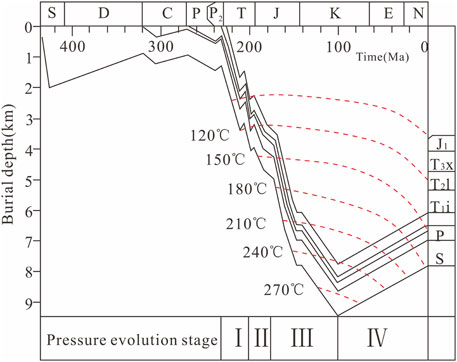
FIGURE 13. Burial history of well YB102 (modified after Zhang,2010)
Marlite Being an Efficient Cap Rock
Although there is a small amount of gas in the Feixianguan Formation, it is mainly caprock. The fractures may cut the marlite at normal temperature and pressure. Due to high confining pressure and temperature in the deep crust, ductility or plasticity increases, leading to good caprocks like shale. (Figure 8; Donath, 1970; Ferrill and Morris, 2003 and Ferrill and Morris, 2008; Ferrill et al., 2012 and Ferrill et al., 2017). The strength of the marlite decreases like salt (Weijermars et al., 1993; Jackson and Vendeville, 1994).
The deformation behavior of the marlite in limestone samples from well cores was similar to that of the Solnhofen limestone, according to the strength measurements of the marlite of the limestone samples (Figure 15 and Table 2). The limestone will undergo semi-brittle deformation, with a confining pressure of 53 MPa. The limestone will enter the plastic deformation stage when the confining pressure reaches 140 MPa.
When gas production peaked at the end of the Middle Jurassic, the burial depth of the Yuanba Gas Field was 5 km. The Poisson horizontal stress was 70.80 MPa, and the vertical stress was 131.5 MPa. Considering that the Sichuan basin was a foreland basin during the middle Jurassic period, the horizontal stress would have been greater than 70.80 MPa. The acoustic emission measurement on the J1z sandstone in well YB102 at a 3,921 m depth revealed two horizontal stresses of 101.1 and 96.1 MPa, with a vertical stress of 100.4 MPa. These stresses were referred to as Yanshanian movement stress strength. The marlite has progressed to the semi-brittle stage. Consequently, the marlite of the Feixianguan Formation was continuously buried before being uplifted by the late Yanshanian movements. Now, it has a 6,000 m burial depth and is denudated with thicknesses of 1,000–3,400 m (Qiu et al., 2008). The present maximum principal stress is in the NW-SE direction, according to borehole caving and fracturing data. The vertical stress in well YB1 is 186.0 MPa, and the horizontal stresses are 243.8 and 154.8 MPa at a depth of 7,081–7,150 m. The vertical stress in well YB2 is 173.0 MPa, and the horizontal stresses are 235.9 and 140.8 MPa at a depth of 6,677–6,700 m. As a result, the confining pressures in geological compression settings were greater than 53 MPa or 96.1 MPa. Marlite has been in a high plastic state to be a good caprock, similar to shale.
Furthermore, the well cores revealed stylolites formed in the Feixianguan Formation, with most of them filled with mud (Figure 5D). They were sealing materials for oil and gas (Heap et al., 2018; Toussaint et al., 2018; Bruna et al., 2019). Many factors, including delaminated fracturing, plastic deformation due to high confining pressures, and mud-filled stylolites, determined the Feixianguan Formation marlite to be a good cap (Figure 16). Since the late Jurassic, it has prevented the pyrolysis gas from leaking out of the Changxing Formation dolomite reservoir.
Gas Field Formation Mechanism
The major fractures in the Changxing Formation were formed during the initial formation of oil reservoirs in the Yuanba Gas Field from the Late Triassic to the Early Jurassic by integrating pore fluid pressure with the Indosinian movements in the Late Triassic. The fractures increased the porosity and permeability of the dolomite from the Changxing Formation. Due to the medium extent of deformation, no faults cutting through the stratum section from Permian to Jurassic systems were formed. The oil migrated through the fracture network from the underlying source rock, the Wujiaping formation, to charge the overlying Changxing Formation dolomite reservoirs (Figure 17). Later, in the Late Jurassic, the oil was pyrolyzed, resulting in the formation of a paleo-gas field. The subsequent tectonic movements had a minor impact on the paleo-gas field, and finally, the Yuanba Gas Field was formed.
A few vertical fractures were found in the overlying Feixianguan Formation marlite, and they were not connected to the fractures in the Changxing Formation dolomite. Because of the plasticity caused by the deep burial, high confining pressure, and mud-filled stylolites, the marlite served as a good caprock to keep the gas in the underlying Changxing Formation.
Conclusion
Delaminated fracturing is critical in controlling the ultra-deep Yuanba Gas Field formation. The interlayer fracturing was determined by the various rock mechanics, deformation layer thicknesses, the pore fluid pressures, and the magnitudes of the tectonic stress strengths. A medium deformation extensity is favorable for gas field formation because it ensures fractures and prevents the formation of a fault.
The Feixianguan Formation, which directly overlies the Changxing reservoir, was marlite. The Indosinian movement was not fractured due to the low pore fluid pressure. Due to high confining pressures, the marlite acquired high plasticity during the burial and uplifting processes, preserving the continuity of marlite. Dense horizontal stylolites formed in the marlite and were filled with mud to form sealing. These factors ensure that the Feixianguan Formation marlite will be caprock.
The Yuanba Gas Field is a reservoir filled with pyrolysis gas. The reservoir rock is dolomite of the Upper Permian Changxing Formation, the caprock is marlite of the Lower Triassic Feixianguan Formation, and the source rock is mudstone of the Upper Permian Wujiaping Formation. The paleo-oil reservoir in the Changxing Formation was formed from the Late Triassic to the Early Jurassic. The oil was stored in the pores and fractures of the dolomite. The fractures were formed by the interaction of the pore fluid pressure and the tectonic stresses of the Indosinian movements. The oil was pyrolysis gas from the Late Jurassic and was stored in the original pores and fractures.
Data Availability Statement
The original contributions presented in the study are included in the article/supplementary material, further inquiries can be directed to the corresponding author.
Author Contributions
XW: writing articles, processing experimental data, field observation, and collecting samples; SC: guidance; GF: processing and interpreting seismic data; ZX: drawing figures; HY: field observation and collecting samples; SX: testing samples; HZ: testing samples.
Funding
This work was financially supported by the Strategic Priority Research Program of the Chinese Academy of Sciences (Grant No. XDA14010306) and the National Natural Sciences Foundation of China (Grant No. 42172138).
Conflict of Interest
The authors declare that the research was conducted in the absence of any commercial or financial relationships that could be construed as a potential conflict of interest.
Publisher’s Note
All claims expressed in this article are solely those of the authors and do not necessarily represent those of their affiliated organizations or those of the publisher, the editors, and the reviewers. Any product that may be evaluated in this article or claim that may be made by its manufacturer is not guaranteed or endorsed by the publisher.
Acknowledgments
The authors would like to thank all the reviewers who participated in the review as well as MJEditor (https://www.mjeditor.com) for providing English editing services during the preparation of this manuscript.
Supplementary Material
The Supplementary Material for this article can be found online at: https://www.frontiersin.org/articles/10.3389/feart.2022.884935/full#supplementary-material
Supplementary Figure S1 | Mohr envelope curves and Mohr stress circles.
Supplementary Figure S2 | Strengths of the limestone in the Yuanba Gas Field under various confining pressures. The solid and dashed lines in b are the strengths of Solnhofen limestone (Rutter, 1986). The small squares are the measurement data. The average density of the overburden rocks is 2.63 g/cm3, and Poisson’ ratio is 0.35.
Supplementary Figure S3 | Reservoir formation mechanism of the Yuanba Gas Field.
Supplementary Figure S4 | Gas migration and charge via intersections of delaminated fractures. (A) Fractures in the upper layer; (B) fractures in the lower layer; (C) gas migrating from the lower layer to the upper layer via the intersections (small circles).
References
Arouri, K. R., Van Laer, P. J., Prudden, M. H., Jenden, P. D., Carrigan, W. J., and Al-Hajji, A. A. (2010). Controls on Hydrocarbon Properties in a Paleozoic Petroleum System in Saudi Arabia: Exploration and Development Implications. Bulletin 94 (2), 163–188. doi:10.1306/07060908133
Bai, G. P. (2006). Distribution Patterns of Giant Carbonate fields in the World. J. Palaeogeogr. (Chinese Edition) 08 (02), 241–250. doi:10.3969/j.issn.1671-1505.2006.02.010
Bai, T., and Pollard, D. D. (2000). Closely Spaced Fractures in Layered Rocks: Initiation Mechanism and Propagation Kinematics. J. Struct. Geology. 22 (10), 1409–1425. doi:10.1016/S0191-8141(00)00062-6
Bildstein, O., Worden, R. H., and Brosse, E. (2001). Assessment of Anhydrite Dissolution as the Rate-Limiting Step during Thermochemical Sulfate Reduction. Chem. Geology. 176 (1-4), 173–189. doi:10.1016/S0009-2541(00)00398-3
Bruna, P.-O., Lavenu, A. P. C., Matonti, C., and Bertotti, G. (2019). Are Stylolites Fluid-Flow Efficient Features? J. Struct. Geology. 125, 270–277. doi:10.1016/j.jsg.2018.05.018
Davies, G. R., and Smith, L. B. (2006). Structurally Controlled Hydrothermal Dolomite Reservoir Facies: An Overview. Bulletin 90 (11), 1641–1690. doi:10.1306/05220605164
Dyman, T. S., Crovelli, R. A., Bartberger, C. E., and Takahashi, K. I. (2002). Worldwide Estimates of Deep Natural Gas Resources Based on the U.S. Geological Survey World Petroleum Assessment 2000. Nat. Resour. Res. 11 (3), 207–218. doi:10.1023/a:1019860722244
Ehrenberg, S. N., and Nadeau, P. H. (2005). Sandstone vs. Carbonate Petroleum Reservoirs: A Global Perspective on Porosity-Depth and Porosity-Permeability Relationships. Bulletin 89, 435–445. doi:10.1306/11230404071
Fan, X. J. (2012). Causes of Differences of Natural Gas Pooling between the Changxing Formation and the Feixianguan Formation in Yuanba, Sichuan Basin. Nat. Gas Industry 32 (06), 15–20+104. doi:10.3787/j.issn.1000-0976.2012.06.003
Fan, X. J. (2014). Genetic Analysis of High-Yield wells of Ultra-deep Reef- Shoal Lithologic Gas Reservoirs:A Case Study of Reef- Shoal Facies Reservoirs in Changxing Formation, Yuanba Area, Northeastern Sichuan Basin. Pet. Geology. Exp. 36 (01), 70–76+82. doi:10.11781/sysydz201401070
Ferrill, D. A., and Morris, A. P. (2003). Dilational normal Faults. J. Struct. Geology. 25 (2), 183–196. doi:10.1016/s0191-8141(02)00029-9
Ferrill, D. A., and Morris, A. P. (2008). Fault Zone Deformation Controlled by Carbonate Mechanical Stratigraphy, Balcones Fault System, Texas. Bulletin 92 (3), 359–380. doi:10.1306/10290707066
Ferrill, D. A., Morris, A. P., and McGinnis, R. N. (2012). Extensional Fault-Propagation Folding in Mechanically Layered Rocks: the Case against the Frictional Drag Mechanism. Tectonophysics 576-577, 78–85. doi:10.1016/j.tecto.2012.05.023
Ferrill, D. A., Morris, A. P., McGinnis, R. N., Smart, K. J., Wigginton, S. S., and Hill, N. J. (2017). Mechanical Stratigraphy and normal Faulting. J. Struct. Geology. 94, 275–302. doi:10.1016/j.jsg.2016.11.010
Guo, T. L. (2011). Reservoir Characteristics and its Controlling Factors of the Changxing Formation Reservoir in the Yuanba Gas Field, Sichuan basin, China. Acta Petrologica Sinica 27 (8), 238–2391. doi:10.1134/S1075701511030068
Guo, T. L. (2019). Gas Accumulation Conditions and Key Exploration & Development Technologies in Yuanba Gas Field. Acta Petrologica Sinica 40 (6), 748–760. doi:10.7623/syxb201906011
Guo, T. L., Zhang, Y. C., and Zou, H. Y. (2010). Present Stress Field and Fracture Characteristics of marine Carbonate Reservoir in Northeast Sichuan. Fault-Block Oil & Gas Field 17 (06), 718–721.
Guo, X. S., Guo, T. L., Huang, R. C., and Duan, J. B. (2014). Cases of Discovery and Exploration of marine fields in China (Part 16): Yuanba Gas Field in Sichuan Basin. Mar. Origin Pet. Geology. 19 (04), 57–64. doi:10.3969/j.issn.1672-9854.2014.04.009
Guo, X. S., Hu, D. F., Li, Y. P., Duan, J. B., Ji, C. H., and Duan, H. (2018). Discovery and Theoretical and Technical Innovations of Yuanba Gas Field in Sichuan Basin, SW China. Pet. Exploration Dev. 45 (01), 14–26. doi:10.1016/S1876-3804(18)30002-8
Handin, J. W. (1958). Effects of Pore Pressure on the Experimental Deformation of Some Sedimentary Rocks. GSA Bull. 69, 1576–1577. doi:10.1306/BC743A87-16BE-11D7-8645000102C1865D
Hao, F., Liu, J. Z., Zou, H. Y., and Li, P. P. (2015). Mechanisms of Natural Gas Accumulation and Leakage in the Overpressured Sequences in the Yinggehai and Qiongdongnan Basins, Offshore South China Sea. Earth Sci. Front. 22 (1), 169–180. doi:10.13745/j.esf.2015.01.014
Heap, M., Reuschlé, T., Baud, P., Renard, F., and Iezzi, G. (2018). The Permeability of Stylolite-Bearing limestone. J. Struct. Geology. 116, 81–93. doi:10.1016/j.jsg.2018.08.007
Hooker, J., Laubach, S., and Marrett, R. (2013). Fracture-aperture Size-Frequency, Spatial Distribution, and Growth Processes in Strata-Bounded and Non-strata-bounded Fractures, Cambrian Mesón Group, NW Argentina. J. Struct. Geology. 54, 45–71. doi:10.1016/j.jsg.2013.06.011
Hu, G. Y., Wang, X. B., Wang, Y. F., Tao, X. W., Ni, Y. Y., and Yang, C. (2009). Cap Rock Characteristics of Medium and Large Gas fields in China. Nat. Gas Geosci. 20 (2), 162–166.
Jackson, M. P. A., and Vendeville, B. C. (1994). Regional Extension as a Geologic Trigger for Diapirism. Geol. Soc. America Bull. 106 (01), 57–73. doi:10.1130/0016-7606(1994)106<0057:reaagt>2.3.co;2
Jin, Z. J., Zhu, D. Y., Hu, W. X., Zhang, X. F., Wang, Y., and Yan, X. B. (2006). Geological and Geochemical Signatures of Hydrothermal Activity and Their Influence on Carbonate Reservoir Beds in the Tarim Basin. Acta Geologica Sinica 80 (2), 245–253. doi:10.1016/S1001-8042(06)60011-0
Katz, B. J., and Everett, M. A. (2016). An Overview of Pre-devonian Petroleum Systems - Unique Characteristics and Elevated Risks. Mar. Pet. Geology. 73, 492–516. doi:10.1016/j.marpetgeo.2016.03.019
King Hubbert, M., and Rubey, W. W. (1959). Role of Fluid Pressure in Mechanics of Overthrust Faulting. Geol. Soc. America Bull. 70, 115–166. doi:10.1130/0016-7606(1959)70[115:rofpim]2.0.co;2
Li, P. P. (2009). Conduit System and Petroleum Accumulation Mechanism of marine Carbonate Strata in Northeast of Sichuan Basin. Beijing: China University of Petroleum-Beijing.
Li, P. P., Guo, X. S., Hao, F., Zou, H. Y., Yu, X. Y., and Wang, G. W. (2016). Paleo-oil-reservoirs Reconstruction and Oil Correlation of Changxing Formation in the Yuanba Gas Field, Sichuan Basin. Earth Sci. 41 (03), 452–462. doi:10.3799/dqkx.2016.037
Li, Y., Kang, Z. J., Xue, Z. J., and Zheng, S. Q. (2018). Theories and Practices of Carbonate Reservoirs Development in China. Pet. Exploration Dev. 45 (04), 669–678. doi:10.1016/s1876-3804(18)30074-0
Qin, Q. R., Zhu, M. Y., Fan, C. H., Xing, J. X., Lei, P., and Li, H. (2017). Structural Features Analysis and Genetic Mechanism of Xujiahe Formation in the center of Yuanba Area, Sichuan basin. Reservoir Eval. Dev. 7 (02), 1–6. doi:10.13809/j.cnki.cn32-1825/te.2017.02.001
Qiu, N. S., Qin, Z. J., McInnes, B. I., Wang, J., Teng, G. E., and Zheng, L. J. (2008). Tectonothermal Evolution of the Northeastern Sichuan Basin: Constraints from Apatite and Zircon (U-Th)/He Ages and Vitrinite Reflectance Data. Geol. J. China Universities (02), 223–230. doi:10.16108/j.issn1006-7493.2008.02.013
Rutter, E. H. (1986). On The Nomenclature of Mode of Failure Transitions in Rocks. Tectonophysics 122, 381–387. doi:10.1016/0040-1951(86)90153-8
Shao, H. W. (2012). Exploration of Reservoir Formation Model of Changxing Formation in Yuanba Area, Northeast Sichuan. China Pet. Chem. Stand. Qual. 32 (08), 196.
Sun, L., Zou, C., Zhu, R., Zhang, Y., Zhang, S., Zhang, B., et al. (2013). Formation, Distribution and Potential of Deep Hydrocarbon Resources in China. Pet. Exploration Dev. 40 (6), 687–695. doi:10.1016/S1876-3804(13)60093-2
Surpless, B., and Wigginton, S. S. (2020). The Impact of Inter-bed Cohesion on Fold-Related Fracture Development, Stillwell Anticline, West Texas (USA). J. Struct. Geology. 134, 103974. doi:10.1016/j.jsg.2019.103974
Tingay, M. R. P., Hillis, R. R., Morley, C. K., King, R. C., Swarbrick, R. E., and Damit, A. R. (2009). Present-day Stress and Neotectonics of Brunei: Implications for Petroleum Exploration and Production. Bulletin 93 (1), 75–100. doi:10.1306/08080808031
Toussaint, R., Aharonov, E., Koehn, D., Gratier, J.-P., Ebner, M., Baud, P., et al. (2018). Stylolites: A Review. J. Struct. Geology. 114, 163–195. doi:10.1016/j.jsg.2018.05.003
Wu, M. N. (2020). Fracture Growth Experiment and Physical Properties for Gas Reservoir Caprock in Triassic Formation, Northeast Sichuan. Beijing): China University of Petroleum.
Wang, Y. F., Zhao, X. Y., and Liu, C. C. (2019). Development Characteristics and Main Controlling Factors of Natural Fractures in Reef-Flat Facies Reservoirs of Changxing Formation in Yuanba Area, Northeastern Sichuan Basin. Nat. Gas Geosci. 30 (07), 973–981. doi:10.11764/j.issn.1672-1926.2019.04.004
Weijermars, R., Jackson, M. P. A., and Vendeville, B. C. (1993). Rheological and Tectonic Modeling of Salt Provinces. Tectonophysics 217 (1-2), 143–174. doi:10.1016/0040-1951(93)90208-2
Wu, G., Zhao, K., Qu, H., Scarselli, N., Zhang, Y., Han, J., et al. (2020). Permeability Distribution and Scaling in Multi-Stages Carbonate Damage Zones: Insight from Strike-Slip Fault Zones in the Tarim Basin, NW China. Mar. Pet. Geology. 114, 104208. doi:10.1016/j.marpetgeo.2019.104208
Yang, H. J., Chen, Y. Q., Tian, J., Du, J. H., Zhu, Y. F., Li, H. H., et al. (2020). Great Discovery and its Significance of Ultra-deep Oil and Gas Exploration in Well Luntan-1 of the Tarim Basin. China Pet. Exploration 25 (02), 62–72. doi:10.3969/j.issn.1672-7703.2020.02.007
Yuan, Y. S., Hu, W. X., Chen, S. P., and Wang, G. J. (2019). The Main Controlling Factors and Evaluation Ideas of Ultra-deep Oil and Gas Preservation. Mar. Origin Pet. Geology. 24 (04), 47–56. doi:10.3969/j.issn.1672-9854.2019.04.005
Zeng, L. B., Li, Y. G., Zhang, G. B., and Chen, G. M. (2007). Controlling Factor S for Fracture Distribution in the Low- Permeability sandstone Reservoir of the Second Member of the Upper Triassic Xujiahe Formation in the South of Wester N Sichuan. Geology. China 34, 622–627. doi:10.3969/j.issn.1000-3657.2007.04.010
Zhang, G. Y., Ma, F., Liang, Y. B., Zhao, Z., Qin, Y. Q., Liu, X. B., et al. (2015). Domain and Theory-Technology Progress of Global Deep Oil and Gas Exploration. Acta Petrolei Sinica 36 (9), 1156–1166. doi:10.7623/syxb201509015
Zhang, Y. C. (2010). Evolution of Stress Field and Migration, Accumulation and Preservation of Oil and Gas of Permian and Triassic Formation in Northeast of Sichuan Basin. Beijing: China University of Petroleum-Beijing.
Zhao, W. Z., Shen, A. J., Zheng, J. F., Qiao, Z. F., Wang, X. F., and Lu, J. M. (2014). The Porosity Origin of Dolostone Reservoirs in the Tarim, Sichuan and Ordos Basins and its Implication to Reservoir Prediction. Sci. China: Earth Sci. 44, 1925–1939. doi:10.1007/s11430-014-4920-6
Zhao, W. Z., Shen, A. J., Hu, A. P., Zhou, J. G., and Ni, X. F. (2015). A Discussion on the Geological Background of marine Carbonate Reservoirs Development in Tarim, Sichuan and Ordos Basin, China. Acta Petrologica Sinica 31 (11), 3495–3508.
Zhao, X. Y., Hu, X. Y., Zeng, L. B., Xiao, K. H., Li, H. T., You, Y. C., et al. (2017). Evaluation on the Effectiveness of Natural Fractures in Reef-Flat Facies Reservoirs of Changxing Fm in Yuanba Area, Sichuan Basin. Nat. Gas Industry 37 (02), 52–61. doi:10.3787/j.issn.1000-0976.2017.02.007
Zhu, H. F., Liu, C. Z., and Lin, F. L. (1986). Genetic and Sedimentary Facies Characteristics of the Middle and Lower Triassic Salt-Bearing Rocks in Sichuan Basin. J. Mineralogy Pet. 03, 76–86+195. 196. doi:10.19719/j.cnki.1001-6872.1986.03.011
Keywords: delaminated fracturing, carbonate rock, medium-strength deformation, hydrocarbon accumulation, Yuanba ultra-deep gas field
Citation: Wang X, Chen S, Feng G, Xiao Z, Yuan H, Xu S and Zhao H (2022) Delaminated Fracturing and its Controls on Hydrocarbon Accumulation in Carbonate Reservoirs of Weak Deformation Regions: A Case Study of the Yuanba Ultra-Deep Gas Field in Sichuan Basin, China. Front. Earth Sci. 10:884935. doi: 10.3389/feart.2022.884935
Received: 27 February 2022; Accepted: 30 March 2022;
Published: 29 April 2022.
Edited by:
Chen Zhang, Chengdu University of Technology, ChinaReviewed by:
Jinyu Zhang, University of Texas at Austin, United StatesZhiping Wu, China University of Petroleum, China
Copyright © 2022 Wang, Chen, Feng, Xiao, Yuan, Xu and Zhao. This is an open-access article distributed under the terms of the Creative Commons Attribution License (CC BY). The use, distribution or reproduction in other forums is permitted, provided the original author(s) and the copyright owner(s) are credited and that the original publication in this journal is cited, in accordance with accepted academic practice. No use, distribution or reproduction is permitted which does not comply with these terms.
*Correspondence: Shuping Chen, csp21c@163.com