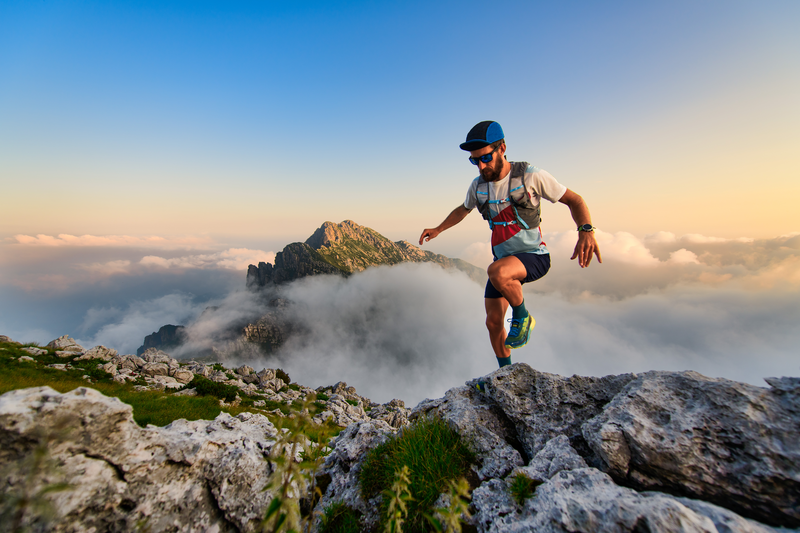
95% of researchers rate our articles as excellent or good
Learn more about the work of our research integrity team to safeguard the quality of each article we publish.
Find out more
ORIGINAL RESEARCH article
Front. Earth Sci. , 22 June 2022
Sec. Paleontology
Volume 10 - 2022 | https://doi.org/10.3389/feart.2022.884685
This article is part of the Research Topic Crustose Coralline Red Algae Frameworks and Rhodoliths: Past and Present View all 10 articles
Shallow-water rhodolith beds are rare in the Mediterranean Sea and generally poorly known. The Punta de la Mona rhodolith bed extends for 16,000 square meters in shallow and oligotrophic waters at the southern coast of Spain, off Almuñecar in the Alborán Sea. We present a detailed analysis of the structure (rhodolith cover and density, rhodolith size and shape, sediment granulometry) and morphospecies composition of the bed along a depth gradient. A stratified sampling was carried out at six depths (9, 12, 15, 18, 21, and 24 m), estimating rhodolith cover and abundance; rhodoliths were collected from one 30 by 30 cm quadrat for each transect, resulting in 18 samples and a total of 656 rhodoliths. The collected rhodoliths were measured and the coralline algal components identified morphoanatomically through a stereomicroscope and SEM. Sediment on the seafloor mainly consisted of pebbles and cobbles; the highest rhodolith cover occurred between 15 and 18 m, and the lowest at the shallowest and deepest transects (9 and 24 m). Mean Rhodolith size was similar throughout the depth range (23–35 mm) with a slight increase at 24 m, although the largest rhodoliths occurred at 21 m. In monospecific rhodoliths, size depended more on the forming species than on depth. We found 25 non-geniculate coralline morphospecies, nearly all rhodolith-forming morphospecies reported in the Mediterranean Sea in recent accounts. The highest morphospecies richness (18–19) and proportional abundance were found at intermediate depths (15–18 m), where rhodolith cover is also highest. Lithophyllum incrustans and Lithophyllum dentatum dominated at shallow depths (9–12 m), whereas Lithothamnion valens was the dominant species at intermediate and greater depths. Overall, the latter species was the most common in the rhodolith bed. The shallow-water rhodolith bed in Punta de la Mona is probably the most diverse in the Mediterranean Sea. This highlights the importance of the conservation of this habitat and, in general, emphasizes the role of the Alborán Sea as a diversity center of coralline algae. The Punta de la Mona example contradicts the common assumption in the geological literature that rhodolith beds are indicative of oligophotic environments with high nutrients levels.
Rhodolith beds are living or fossil concentrations of free-living coralline algae (Riosmena-Rodríguez, 2017). The largest rhodolith beds occur in Brazil, where they cover a substantial part of the continental shelf, with some extending for more than 20,000 km2 (Amado-Filho et al., 2012). They are also widespread around islands and capes, and on seamounts in Baja California, northern Atlantic, Mediterranean Sea, and Australia (Foster, 2001; Steller et al., 2003; Harvey et al., 2016; Basso et al., 2017; Hernández-Kantún et al., 2017; Kamenos et al., 2017). Fragkopoulou et al. (2021) estimate that rhodolith beds might cover up to 4.1 × 106 km2 on the seafloor, being one of the largest benthic communities dominated by macrophytes, together with seagrass beds, kelp forests and coralligènes. The main environmental factors that condition the development of rhodolith beds are light, temperature, nutrients, and currents or bioturbation phenomena that avoid the rhodoliths being buried by sediments (Jacquotte, 1962; Birkett et al., 1998; Marrack, 1999; Carvalho et al., 2020).
Rhodolith beds are considered critical ecosystems for marine biodiversity (Fredericq et al., 2019), which depends on their structural complexity (Bordehore et al., 2003; Teichert et al., 2014). In fact, rhodoliths are ecosystem engineers (Nelson, 2009), which generate structural complexity and hard substrates on detrital sea floors (Cabioch, 1969), fostering biodiversity by providing habitat to species of both hard and mobile bottoms (Keegan, 1974; Barberá et al., 2003), from microfauna (Steller et al., 2003; Foster et al., 2007) to macroalgae (Peña et al., 2014), and to species of commercial interest (Hall-Spencer et al., 2003; Kamenos et al., 2004; Steller and Cáceres-Martínez, 2009). Rhodolith beds are vulnerable to changes in the environment, especially those resulting from human activities, which result in reduced complexity and biodiversity (Peña and Bárbara, 2010). Moreover, rhodolith beds provide environmental services, such as carbonate sequestration and habitat complexity with unique benthic diversity and associated fish assemblages, thus justifying urgent actions to protect them (Amado-Filho et al., 2017). The knowledge of their distribution, structure and composition is, therefore, necessary to preserve the ecosystem services of rhodolith beds (Salomidi et al., 2012; Basso et al., 2016).
Reported rhodolith beds are scarce in the Alborán Sea, the southwestern sector of the Mediterranean (Robles, 2010). The largest one occurs on the Alborán island shelf, with several square kilometers extending from 20 to 100 m depth (Betzler et al., 2011; Gofas et al., 2014). Twenty-one species of coralline algae were recognized by molecular analysis in this bed, ten of which remain unnamed (Gofas et al., 2014). Rhodolith beds have also been recorded in the Tres Forcas cape, in Morocco (CAR/ASP-PNUE/PAM, 2013), Gibraltar Straits (García-Gómez et al., 2003), Ceuta (Ocaña et al., 2009) and the Almería shelf, in southeastern Spain (Ministerio de Agricultura, Alimentación y Medio Ambiente, 2012), although their structure and composition were not reported. The composition of coralline algal assemblages, including those forming rhodoliths, was described at the northeastern limit of the Alborán Sea, in the waters of the Cabo de Gata Natural Park in Almería (Braga et al., 2009; Bassi et al., 2020).
This work aims to describe a shallow-water rhodolith bed located off the Punta de la Mona, Granada, (southern Spain) in the northern shelf of the Alborán Sea. We assess the rhodolith cover, size, shape, morphospecies composition and diversity along a depth range from 9 to 24 m, and relate the observed patterns to environmental variables, such as temperature and illuminance. We stress the uniqueness and originality of this bed and the need for conservation of marine biodiversity reserves.
The study area is located at the southern coast of Spain, near Almuñecar in the province of Granada. The area encompasses a rhodolith bed of 16,000 m2 extending from 9 to 24 m depth in a small bay east of Punta de la Mona (36°41′13´´ N, 3°43′39´´ W). This is a cape running N-S for approximately 1,000 m and then bending to a E-ESE direction. As a result, the small bay in which the rhodoliths occur is protected from the westerlies (Figure 1).
According to the wave modeling SIMAR for the Punta de la Mona (site 2039080), during the period January 1958-July 2021, dominant wave directions were from E-ESE (41.8%) and W-WSW (30.3%). Waves had heights above 2 m only 4.5% of the time, and calms and waves <0.5 m occurred 55% of the time (Puertos del Estado, 2021a).
The levels of chlorophyll A from 2000 to 2020 obtained from the AQUA MODIS satellite indicate an oligotrophic environment, with mean values of 1–1.5 mg/cm3, peaking in spring (1.5–2 mg/cm3) and dropping in autumn-winter (>0.01 mg/cm3) (REDIAM, 2021).
Sea surface temperature (SST) in 2020–2021, estimated by the satellite Sentinel (site 2039080), ranged between 27.1°C in summer and 13.3°C in winter (Puertos del Estado, 2021b). Measuring every 30 min at 16 m depth, in 2010–2011 the average temperature was 17°C, ranging from 26.5°C in summer to 12.5°C in winter. The summer temperatures underwent strong fluctuations due to upwelling events related to storms from the west, whilst winter temperatures were more stable around 15°C (Figure 2). New temperature measurements were carried out for this study (see below).
FIGURE 2. Water temperature at 16 m in the Punta de la Mona between February 2010 and December 2011.
The extent of the rhodolith bed was delimited by mapping the seafloor by SCUBA diving. A stratified sampling design at six depths (9, 12, 15, 18, 21 and 24 m) was used to analyze distribution and density of rhodoliths. Three 20 m long line transects were placed at each depth. To estimate the living (coloured) rhodolith cover, photographs of ten 50x50 cm quadrats were taken in each transect, i.e., 30 photographs in each depth and 180 photos in total (Figure 3A). The pictures were processed with the software ImageJ (National Institutes of Health, United States, https://imagej.nih.gov) to obtain the area covered by living-rhodoliths, cobbles, pebbles, and sand in each quadrat. The proportion of dead rhodoliths was negligible. All rhodoliths in a randomly placed 30 × 30 cm quadrat were collected in each transect, i.e., 3 collections from each depth and 18 in total for measuring and identification of coralline algal composition. Collections were done between May and December 2020 (Figure 3B). Both unattached coralline growths and pebbles coated by corallines (independently of coating proportion) were collected and air dried for at least 2 days.
FIGURE 3. (A) Line transect and quadrat (50 × 50-cm) for living-rhodolith cover estimation. (B) Quadrat (30 × 30-cm) for rhodolith collection.
Six dataloggers (HOBO Pendant® MX, Temp/Light, www.onsensetcomp.com) were installed for data acquisition of temperature (°C) and illumination (lux) at each sampling depth, using the maximum daily illuminance by depth from measurements taken every 10 min (Otero-Ferrer et al., 2020). Two 50-ml samples of sediment smaller than pebbles were collected in each sampling depth to estimate granulometry. The samples were dried and then sieved in >1, 0.5, 0.250, and <0.125 mm fractions.
The long, intermediate and short axes of all collected rhodoliths (n= 656) were measured to estimate size, using the mean of the three axes (L + I + S)/3 (Bosellini and Ginsburg, 1971) and the sphericity
Identification of coralline algae forming the rhodoliths was performed with a stereomicroscope and ESEM (FEG-ESEM QuemScan650F). The morphospecies identification followed the available literature on Atlantic and Mediterranean coralline algae and the taxonomy proposed by Cormaci et al. (2017) and AlgaeBase (Guiry and Guiry, 2022). Once the component species in each rhodolith were identified, a visual estimation of their relative proportions on the rhodolith surface was performed. Vouchers of the rhodoliths will be deposited at the University of Granada Herbarium (GDA).
The diversity indexes based in abundance models allow us to compare the diversity of rhodoliths taking into consideration the relative abundance of species in an ecosystem (Magurran, 2004), that is, in our study the relative species-cover (i.e., relative proportions of individual species on the rhodolith surface) or species-frequency (i.e., relative abundance of species) that form the rhodoliths in different depths. The calculation of the Shannon-Wiener index (Magurran, 2004) was made through the formula
Statistical comparisons between groups were performed on R Studio 2021.09.1+372 (RStudio Team, 2020) running R 4.1.2 (R Core Team, 2021). Data manipulation was facilitated by the dplyr 2.1.1, tidyverse 1.3.1, and broom 0.7.10 packages. Plots and graphs were generated by the ggplot2 3.3.5 package and finalized in Inkscape 1.1.1 (http://inkscape.org). All other statistical tests were from the base package of R.
The non-parametric Kruskal–Wallis rank sum test was used to compare the differences among groups since the data did not fulfill the assumptions of ANOVA. Dunn’s test with Holm’s correction was used as the post-hoc test when a significant difference resulted from Kruskal–Wallis tests. Comparisons across the sampled depths were made for the benthic cover of 1) living-rhodoliths, 2) different grain sizes (cobbles, pebbles, sand), and the sizes of 3) all rhodoliths, and 4) species of the monospecific rhodoliths, excluding encrusting species (Lithophyllum incrustans) and those with <5 samples. 5) Size differences among species of selected monospecific rhodoliths were also compared.
Data on temperature and illumination were obtained from 21 February to 21 March 2021 at 15, 21 and 24 m depth, from 4 to 21 December 2021 at 12, 15, 18, 21 and 24 m, and from 15 to 31 January 2022 at 15, 18 and 21 m.
The average temperature at the end of the winter in 2021 ranged from 15.8°C at 15 m to 15.7°C at 24 m, whereas in December 2021 they were slightly lower ranging between 15.6°C at 12 m and 15.5°C at 24 m, with no significant differences among depths. In January 2022, the temperature was 15.1°C between the depths of 15–21 m (Supplementary Table S1).
Illuminance measured every 10 min showed that the daily maxima was highly variable from 21 February to 21 March 2021, with a maximum value of 10,875 lux at 15 m and a minimum of 486 lux at 24 m (Figure 4A). The average maximum illuminance at the end of the winter showed a marked gradient with depth (Figure 4D). In a similar way, in December 2021 the daily maximum illuminance ranged from 9,224 lux at 12 m and 170 lux at 24 m (Figure 4B). The decreasing gradient with depth showed a reversal at 18 m where the values were lower than at 21 m (Figure 4D). In January 2022, the maximum daily illuminance ranged between 10,416 and 827 lux at 15 m (Figure 4C), with a reduction in mean illuminance to 93.6% at 18 m and 61.4% at 21 m with respect to at 15 m (Supplementary Table S2). In the three periods, the reduction in maximum illuminance between 15 and 21 m was similar, with values of 62.5, 61.8 = (3543.7/5734.6); 53.5% in Supplementary Table S2 because the basis was the lux at 12 m, and 61.4% (Figure 4D; Supplementary Table S2).
FIGURE 4. Daily maximum illuminance from (A) 21 February to 21 March2021 and (B) 4 to 20 December 2021, (C) 15 to 31 January 2022 and (D) variation of mean maximum illuminances by depth.
Regarding grain size on the seafloor, pebbles were the commonest grains at all depths, followed by cobbles (Supplementary Table S3). Significant differences were found across depths for cobbles (p-value < 0.001), pebbles (p-value = 1.72e−12), and sand (p-value < 2.2e−16), but only sand cover exhibited a clear pattern with depth. The proportion of sand-sized grains increased from 0.5% at 9 m to 23.7% at 24 m. (Figure 5A). Within the sand-sized particles, the proportion of very coarse grains decreased with depth, whereas other fractions tended to increase, except very fine sand and mud which showed the highest percentage at 18 m (Figure 5B, Supplementary Table S3). A weak current was perceived at this depth while sampling.
FIGURE 5. (A) Proportion of rhodoliths and grain-size classes (% cover) at different depths. (B) Proportion of sand-sized fractions (<2 mm) at different depths.
The average cover of living-rhodolith (mean ± SD) ranged between 1.6% (±1.1) and 1.4% (±0.8) at 24 and 9 m, respectively, and 15.9% (±8.3) at 18 m (p-value < 2.2e−16). Highest rhodolith cover in sampling quadrats ranged between 3.7% at 24 m and 34.7% at 18 m. Rhodolith distribution was heterogeneous with dispersed nodules at the bed margins at 9 and 24 m and density increased towards intermediate depths from 12 to 21 m, where rhodoliths occurred in patches whose average cover ranged from 5% (±3.3) to 15.2% (±8.3) and maximum cover from 11.58 to 34.2% (Figure 6).
FIGURE 6. (A) Rhodolith cover (mean and SD) and (B) boxplots of rhodolith cover illustrating significant differences detected across depths.
Twenty-two morphospecies belonging to 6 genera (Lithophyllum, Spongites, Neogoniolithon, Lithothamnion, Mesophyllum, and Phymatolithon) were identified (Figure 7). Other thalli were only identified at the genus level, due to the lack of diagnostic characters. In three genera no individual species were recognised (Pneophyllum sp., Sporolithon sp., and Harveylithon sp.), for a total of 9 genera. Seven species dominated the assemblages, with occurrence proportions higher than 4%: Lithothamnion valens Foslie (23.1%), Lithophyllum incrustans Philippi (21.1%), Phymatolithon calcareum (Pallas) W.H. Adey et D.L. McKibbin ex Woelkerling et L.M. Irvine (4.8%), Lithophyllum dentatum (Kützing) Foslie (4.4%), Lithophyllum racemus (Lamarck) Foslie (4.3%), Lithothamnion minervae Basso (4.3%) and Neogoniolithon brassica-florida (Harvey) Setchell et L.R. Mason (4.1%). (Figure 8A; Table 1).
FIGURE 7. SEM images of selected examples of identified morphospecies: (A) Sporangial conceptacle of Lithophyllum hibernicum. Black lines mark the contour of funnel-like depression on top of conceptacle pore filled by overgrowing filaments; (B) Bisporangial conceptacle of Lithophyllum orbiculatum. Arrowheads point to bispores; (C) Tetrasporangial conceptacle of Lithophyllum nitorum. Arrowheads point to elongated cells at the base of conceptacle roof; (D) Tetrasporangial conceptacle of Phymatolithon calcareum; (E) Tetrasporangial conceptacle of Lithothamnion corallioides; (F) Tetrasporangial conceptacle of Lithothamnion valens.
FIGURE 8. (A) Relative abundance of the identified taxa at the sampling depths. (B) Shannon-Wiener index diversity measures (based on species frequency or cover) at the sampling depths.
Depth distribution of morphospecies was heterogeneous. The relative abundance of Lithophyllum incrustans decreased with depth from 58.5% of the algal assemblage at 9 m to 5.9% at 21 m. In contrast, the proportion of Lithothamnion valens increased with depth from being absent at 9 m to 38.3% at 21 m. Other species showed limited depth ranges: Lithophyllum orbiculatum (Foslie) Foslie only occurred at shallow depths (9–12 m), Lithophyllum dentatum was most abundant around 12 m, Lithothamnion minervae and Phymatolithon calcareum were more abundant at 15 m, and Mesophyllum macroblastum (Foslie) W.H. Adey, only occurred below 15 m, with the highest counts at 24 m. Other morphospecies, such as Lithophyllum pustulatum (J.V. Lamouroux) Foslie, Lithophyllum nitorum W.H. Adey et PJ Adey, and Lithothamnion corallioides (P.Crouan et H. Crouan) P. Crouan et H. Crouan had homogeneous relative abundance at all depths (Table 1).
Monospecific rhodoliths comprised 34% of the analyzed nodules, varying from 17.9% at 9 m to 39.8% at 21 m. Lithothamnion valens was the most common species building monospecific rhodoliths (44.4%), followed by Lithophyllum incrustans (15.2%), Phymatolithon calcareum (10.3%) and Lithothamnion minervae (8.1%). Two nodules of Lithophyllum pustulatum and Lithothamnion sonderi Hauck, encrusting bioclastic and lithic nuclei, respectively, were monospecific as well (Table 2).
Mean size of rhodoliths (mean ± SD) ranges from 23.7 mm (±9.5) at 18 m to 35.8 mm (±12.3) at 24 m, where the rhodoliths were significantly bigger than in the other depths (p-value = 2.31e−09). Maximum size of rhodoliths increased from 49 mm at 9 m to 101 mm at 21 m and decreased to 53 mm at 24 m (Figure 9). The number of large rhodoliths (>50 mm) also increased from 0 at 9 m to 9 at 21 m and decreased to 2 at 24 m (Table 3).
FIGURE 9. (A) Rhodolith sizes and (B) boxplots illustrating significant differences detected across depths.
Monospecific rhodoliths of the most abundant species (see below) were grouped in two size classes (p-value = 9.58e−09). Based on the size ranges, Lithophyllum dentatum (Figure 10A), Lithothamnion valens (Figure 10D) and Neogoniolithon brassica-florida form the larger rhodoliths with mean sizes of 34.7 mm (±8.3), 28.8 mm (±11.7), and 27.1 mm (±8.2), respectively, whereas rhodoliths of Lithophyllum racemus (Figure 10B), Lithothamnion minervae (Figure 10C) and Phymatolithon calcareum were smaller, with mean sizes of 21.4 mm (±4.7 mm), 18.1 mm (±4.3 mm) and 18.4 mm (±3.8 mm) (Figure 11A), respectively. No significant differences were observed in the mean sizes of monospecific rhodoliths at the different depths in which they occur (p-value > 0.05, Figure 11B).
FIGURE 10. Monospecific rhodoliths: (A) Lithophyllum dentatum; (B) Lithopyllum racemus; (C) Lithothamnion minervae; (D) Lithothamnion valens.
FIGURE 11. (A) Boxplots of sizes of monospecific rhodoliths illustrating significant differences detected across species and (B) their mean size at different depths.
The maximum sphericity projection (Ψ) ranged between 0.65 at 9 m and 0.74 at 21 m, with similar values from 12 to 24 m (Supplementary Table S4). Most rhodoliths were spheroidal (62.5%), with lesser proportions of discoidal (19.9%) and ellipsoidal (17.6%) nodules. Proportions of shape classes were similar at all depths from 12 to 21, with a dominance of spheroidal nodules. In contrast, at 9 m, discoidal (33.7%) and ellipsoidal (28.4%) were more common (Figure 12A).
FIGURE 12. (A) Triangular diagrams of rhodolith shape classes at each sampling depth (Sneed and Folk, 1958; Bosence, 1983; Graham and Midgley, 2000) and (B) relative proportions of rhodolith morphotypes at each sampling depth. Other includes coatings on lithoclasts and bioclasts, and compact rhodoliths of encrusting coralline algae.
Most collected rhodoliths were pralines (47.7%, Figure 12B; Supplementary Table S5). This morphotype is the most abundant below 9 m. The proportion of boxwork rhodoliths increased with depth, whereas coatings and compact rhodoliths built by encrusting corallines (as other in Figure 12B), which were the most abundant at 9 m, tended to be less common in deeper transects. Branches were rare from 15 to 21 m and absent in the rest of depths (Supplementary Table S5).
The diversity of rhodoliths (Shannon-Wiener index, H′), both based on species frequency and cover, was highest at medium depths (15 and 18 m). The maximum rhodolith diversity was 2.51 for species frequency and 2.16 for species cover at 15 m, and the minimum rhodolith diversity was 1.74 for species frequency and 1.33 for species cover at 9 m (Figure 8B).
The Punta de la Mona rhodolith bed occupies a small surface and a narrow depth range, showing that good conditions for rhodolith growth are limited to the eastern side of a small cape enclosing a small embayment. In this small area, bottom currents generated by storms from the east hit the submarine cliff and flow out of the embayment (Figure 1). The generally coarse grain size on the seafloor, dominated by cobbles and pebbles (Figure 5), indicates that currents sweep away fine sediment. Above the upper limit of the bed, the seafloor is covered by boulders and cobbles derived from rock fall from the cliff, and high turbulence seems to prevent rhodolith development, although boulders and cobbles are partially covered by coralline algae. Below 24 m, fine-grained sediments bury the rhodoliths hindering their significant development (Steller and Foster, 1995; Wilson et al., 2004; Villas-Bôas et al., 2014).
The highest average cover of living-rhodoliths occurs at 18 m, where the values are three times higher than those at 12 and 21 m (Figure 6). The optimum conditions for rhodolith growth might be due to a flatter seafloor between 15 and 20 m (Figure 1). Despite the weak current perceived, the highest proportion of very fine sand and mud occurs at 18 m. This can be due to the well-known baffling effect of dense concentrations of branching rhodoliths, which stabilize fine-grained sediment below them (Bassi et al., 2009; Millar and Gagnon, 2018; O’Connell et al., 2021).
The mean rhodolith size is relatively small (23.7 mm). The maximum size and the number of rhodoliths larger than 50 mm increases with depth (Figure 9), probably related to the turbulence gradient (Amado-Filho et al., 2007; Gagnon et al., 2012; Sañé et al., 2016). The size of monospecific rhodoliths is species-dependent. Two size classes can be distinguished for monospecific rhodoliths: Lithophyllum dentatum and Lithothamnion valens, build relatively large rhodoliths, whereas the rhodoliths of Lithothamnion minervae and Phymatolithon calcareum are significantly smaller (Figure 11). Rhodolith sizes within individual species do not show significant differences among depths, suggesting that genetic signatures control the sizes of monospecific rhodoliths rather than environmental conditions, at least at the small scale of the Punta de la Mona bed.
The high abundance of discoidal rhodoliths at 9 m (Figure 12A) reflects the flat, discoidal shape of lithoclastic nuclei, commonly coated by Lithophyllum incrustans at this depth. In contrast with their distribution in the Punta de la Mona, discoidal rhodoliths tend to be more common at deeper depths, because of reduced overturning due to lower energy (Bosellini and Ginsburg, 1971; Bosence, 1976; Peña and Bárbara, 2009). This is an additional example confirming that other factors, such as nucleus shape and building species, influence rhodolith shape, which is not exclusively controlled by hydrodynamic energy (Aguirre et al., 2017; Braga, 2017; O’Connell et al., 2021).
Coatings and compact rhodoliths made of encrusting corallines are the most abundant morphotype at 9 m (Figure 12B), reflecting the dominance of encrusting Lithophyllum morphospecies at this depth. The general decrease of this morphotype with increasing depth parallels the decrease of encrusting plants of this genus. In a similar way, the increase of the relative proportion of boxwork rhodoliths with depth parallels the increase of Mesophyllum. The dominance of mono or paucispecific pralines formed by different species and the scarcity of branches (Figure 12B) probably reflect a strong to intermediate hydrodynamic regime (Bracchi et al., 2022) suggested by the coarse grain size.
The morphospecies richness in the Punta de la Mona bed (25 morphospecies, 22 of them identified at the species level, in 9 genera) is the highest described in the Mediterranean so far in a single bed. Traditionally, the Mediterranean rhodolith beds have been considered richer than those of the eastern Atlantic at similar latitudes (Basso et al., 2017), although only 4–13 species have been reported from beds in the western Mediterranean (Ballesteros, 1989; Basso, 1998; Lanfranco et al., 1999; Mannino et al., 2002; Sciberras et al., 2009; Barberá et al., 2012; Basso et al., 2014; Nitsch et al., 2015; Sañé et al., 2016; Bracchi et al., 2019; Rendina et al., 2020b; Chimienti et al., 2020). The majority of rhodolith-forming species living in the Mediterranean (Basso et al., 2017; Rindi et al., 2019) have been recorded in the Punta de la Mona bed, except for Phymatolithon lusitanicum V. Peña (Peña et al., 2015), and Neogoniolithon hauckii (Rothpletz) RA Townsend & Huisman (Townsend and Huisman, 2018). Although we were not able to identify the recently described Lithophyllum pseudoracemus Caragnano, Rodondi and Rindi separately from Lithophyllum racemus, the former taxon was collected in the Punta de la Mona bed (Caragnano et al., 2020). This exemplifies that the cryptic molecular diversity is probably higher than the morphospecies richness assessed in our study (Gabrielson et al., 2018; De Jode et al., 2019; Pezzolesi et al., 2019; Caragnano et al., 2020; Kato et al., 2022).
Lithothamnion valens, the dominant species in the Punta de la Mona bed, is a Mediterranean endemic (Guiry and Guiry, 2022), which was considered a major structural component of a type of detrital bottom, called “facies de Lithothamnion valens,” since the early descriptions of Mediterranean benthic habitats (Pérès and Picard, 1964). Rhodolith beds dominated by this species have been reported off Murcia in Spain (Aguado-Giménez and Ruiz-Fernández, 2012) and several locations, such as the Balearic Islands (Barberá et al., 2012), Tyrrhenian Sea (Basso, 1995; Bracchi et al., 2019; Rendina et al., 2020b) and Adriatic Sea (Chimienti et al., 2020). The composition of Mediterranean rhodolith beds, however, is heterogeneous even in small areas (Chimienti et al., 2020). No clear pattern of species dominance can be observed at the regional scale, since Lithophyllum racemus (Sañé et al., 2016; Chimienti et al., 2020), Lithothamnion minervae (Rendina et al., 2020b), Spongites fruticulosus (Ballesteros, 1989; Joher et al., 2015), and Lithothamnion corallioides (Barberá et al., 2017) have been reported as dominant species in different beds. Nevertheless, despite the heterogeneity, these species, together with Lithothamnion valens, Phymatolithon calcareum and Neogoniolithon brassica-florida are the rhodolith-forming species characteristic of the Mediterranean. Within the Punta de la Mona bed, the identified morphospecies show patterns of depth distribution probably related to illuminance gradients. Lithophyllum dentatum is rare below 15 m and the abundance of Lithophyllum incrustans markedly decreases with depth. In contrast, Mesophyllum macroblastum does not occur at shallow depths (9–12 m) and has higher relative abundance at 24 m where the maximum illuminance is only 21% of that at 12 m.
Among the small and rare species identified in the multispecific rhodoliths, Lithophyllum orbiculatum (Foslie) Foslie, Lithothamnion crispatum Hauck, and Lithothamnion sonderi Hauck have not been reported in the Alborán Sea so far (Gallardo et al., 2016).
The Shannon-Wiener index (H′) values are higher than those obtained in deeper (40–60 m) Mediterranean enclaves (Rendina et al., 2017). In maërl communities of shallow European Atlantic beds, Peña and Bárbara (2010) obtained similar H′ indexes (1.98–2.96), but for all associated species, not just for coralline algae. Likewise, our results are similar to those obtained by Costa et al. (2019) in a tropical environment and at depths shallower than our study. The coralline algae diversity, as the proportional distribution of species in the ecosystem, is remarkable due to its high values, and the Punta de la Mona can be considered as a “nano-hotspot” (in the sense of Cañadas et al., 2014 for terrestrial regions) of rhodolith biodiversity in the biogeographical context of the Alborán Sea.
The Punta de la Mona rhodolith bed is unique due to the scarcity of this kind of formation in the Alborán Sea, and its shallow depth range, contrasting with the generally deeper depth distribution of rhodolith beds in the Mediterranean (Basso et al., 2017). In this sea, the majority of rhodolith beds occur at 30–75 m, although in oligotrophic clear waters may reach depths close to 150 m, such as in the Balearic Islands (Massutí et al., 2022). Beds shallower than 25 m are scarce, although they occur from 2 m depth in Tunisia (CAR/ASP-PNUE/PAM, 2011), at 9–10 m in Israel (Ramos-Esplá et al., 2012), and in the Apulian shelf in southern Italy (Chimienti et al., 2020). In addition, the Punta de la Mona bed develops in oligotrophic waters, challenging the simplistic assumption, widespread in the geological literature, that rhodolith beds are indicative of oligophotic, mesotrophic to eutrophic environments.
The Punta de la Mona rhodolith bed is protected as a special conservation area (SCA) with the Natura 2000 network. Despite this protection, this habitat is threatened by nautical and fishing activities, affecting the seafloor by the frequent anchoring of boats that use the area as a refuge (Dolinar et al., 2020), the tangling of large branching rhodoliths in fishing nets (Borg et al., 1998), abandoned fishing nets and lines (Rendina et al., 2020a), and the disruption of trophic networks due to intense sport fishing with uncertain consequences on coralline algal communities (O’Leary and McClanahan, 2010). Appropriate regulations for the SCA should be imposed to prevent all these impacts in the future. Restrictions to fishing activities in marine protected areas resulted in increasing rhodolith covers (Barberá et al., 2017). This improved the environmental services provided by the rhodolith beds, including higher biodiversity (Barberá et al., 2003) and larger fish populations (Ordines et al., 2015). Nevertheless, the main threat for the rhodolith bed is the Asian alga Rugulopteryx okamurae (E.Y. Dawson) I.K.Hwang, W.J.Lee & H.S.Kim (Altamirano-Jeschke et al., 2016) first detected in 2020, which is quickly expanding from the Gibraltar straits and nearly completely covering the rocky substrates between 5 and 30 m (García-Gómez et al., 2020), displacing the native algal communities and disturbing the associated invertebrate communities (Navarro-Barranco et al., 2019; Sempere-Valverde et al., 2021). In the first weeks of 2022, the occurrence of many rhodoliths settled by Rugulopterix okamurae makes the future of the rhodolith bed uncertain, and needs to be monitored in the following years. This exemplifies that it is necessary to be cautious and incorporate the knowledge of these communities into the spatial planning framework of Punta de la Mona and other marine protected areas.
The original contributions presented in the study are included in the article/Supplementary Material, further inquiries can be directed to the corresponding author.
JD: Research design, sampling, underwater pictures, taxonomic identification, writing the manuscript; DR: Taxonomic identification, statistics, editing the manuscript; LS-T: Sampling, underwater pictures; JP: Sampling, statistics, editing the manuscript; JB: Taxonomic identification, editing the manuscript.
This work was partly funded by the Junta de Andalucía Research Group RNM 190.
The authors declare that the research was conducted in the absence of any commercial or financial relationships that could be construed as a potential conflict of interest.
All claims expressed in this article are solely those of the authors and do not necessarily represent those of their affiliated organizations, or those of the publisher, the editors and the reviewers. Any product that may be evaluated in this article, or claim that may be made by its manufacturer, is not guaranteed or endorsed by the publisher.
DAR work was funded by EU Horizon 2020 research and innovation program Marie Sklodowska-Curie Grant Agreement No. 813360. We would like to thank Isabel Sánchez for facilitating the SEM imaging remotely. We are grateful to two reviewers whose comments helped to improve the article.
The Supplementary Material for this article can be found online at: https://www.frontiersin.org/articles/10.3389/feart.2022.884685/full#supplementary-material
Aguado-Giménez, F., and Ruiz-Fernández, J. M. (2012). Influence of an Experimental Fish Farm on the Spatio-Temporal Dynamic of a Mediterranean Maërl Algae Community. Mar. Environ. Res. 74, 47–55. doi:10.1016/j.marenvres.2011.12.003
Aguirre, J., Braga, J. C., and Bassi, D. (2017). “Rhodoliths and Rhodolith Beds in the Rock Record,” in Rhodolith/Maërl Beds: A Global Perspective Coastal Research Library. Editors R. Riosmena-Rodríguez, W. Nelson, and J. Aguirre (Cham: Springer International Publishing), 105–138. doi:10.1007/978-3-319-29315-8_5
Altamirano Jeschke, M., De la Rosa Álamos, J., and Martínez Medina, F. J. (2016). Arribazones de la Especie Exótica Rugulopteryx Okamurae (E.Y. Dawson) I.K. Hwang, W.J. Lee and H.S. Kim (Dictyotales, Ochrophyta) en el Estrecho de Gibraltar: Primera Cita Para el Atlántico y España. Algas 52, 20.
Amado-Filho, G., Maneveldt, G., Manso, R., Marins-Rosa, B., Pacheco, M., and Guimarães, S. (2007). Structure of Rhodolith Beds from 4 to 55 Meters Deep along the Southern Coast of Espírito Santo State, Brazil. Cienc. Mar. 33, 399–410. doi:10.7773/cm.v33i4.1148
Amado-Filho, G. M., Bahia, R. G., Pereira-Filho, G. H., and Longo, L. L. (2017). “South Atlantic Rhodolith Beds: Latitudinal Distribution, Species Composition, Structure and Ecosystem Functions, Threats and Conservation Status,” in Rhodolith/Maërl Beds: A Global Perspective Coastal Research Library. Editors R. Riosmena-Rodríguez, W. Nelson, and J. Aguirre (Cham: Springer International Publishing), 299–317. doi:10.1007/978-3-319-29315-8_12
Amado-Filho, G. M., Moura, R. L., Bastos, A. C., Salgado, L. T., Sumida, P. Y., Guth, A. Z., et al. (2012). Rhodolith Beds Are Major CaCO3 Bio-Factories in the Tropical South West Atlantic. PLOS ONE 7, e35171. doi:10.1371/journal.pone.0035171
Ballesteros, E. (1989). Composición y estructura de los fondos de maërl de Tossa de Mar (Gerona, España). Collect. Bot. 17, 161–182. doi:10.3989/collectbot.1989.v17.137
Barberá, C., Bordehore, C., Borg, J. A., Glemarec, M., Grall, J., Hall-Spencer, J. M., et al. (2003). Conservation and Management of Northeast Atlantic and Mediterranean Maerl Beds. Aquat. Conserv. Mar. Freshw. Ecosyst. 13, S65–S76. doi:10.1002/aqc.569
Barberá, C., Moranta, J., Ordines, F., Ramón, M., de Mesa, A., Díaz-Valdés, M., et al. (2012). Biodiversity and Habitat Mapping of Menorca Channel (Western Mediterranean): Implications for Conservation. Biodivers. Conserv. 21, 701–728. doi:10.1007/s10531-011-0210-1
Barberá, C., Mallol, S., Vergés, A., Cabanellas-Reboredo, M., Díaz, D., and Goñi, R. (2017). Maerl Beds inside and outside a 25-Year-Old No-take A-rea. Mar. Ecol. Prog. Ser. 572, 77–90. doi:10.3354/meps12110
Bassi, D., Nebelsick, J. H., Checconi, A., Hohenegger, J., and Iryu, Y. (2009). Present-day and Fossil Rhodolith Pavements Compared: Their Potential for Analysing Shallow-Water Carbonate Deposits. Sediment. Geol. 214, 74–84. doi:10.1016/j.sedgeo.2008.03.010
Bassi, D., Braga, J. C., Owada, M., Aguirre, J., Lipps, J. H., Takayanagi, H., et al. (2020). Boring Bivalve Traces in Modern Reef and Deeper-Water Macroid and Rhodolith Beds. Prog. Earth Planet. Sci. 7 (1), 1–17. doi:10.1186/s40645-020-00356-w
Basso, D., Babbini, L., Kaleb, S., Bracchi, V. A., and Falace, A. (2016). Monitoring Deep Mediterranean Rhodolith Beds. Aquat. Conserv. Mar. Freshw. Ecosyst. 26, 549–561. doi:10.1002/aqc.2586
Basso, D., Babbini, L., Ramos-Esplá, A. A., and Salomidi, M. (2017). “Mediterranean Rhodolith Beds,” in Rhodolith/Maërl Beds: A Global Perspective Coastal Research Library. Editors R. Riosmena-Rodríguez, W. Nelson, and J. Aguirre (Cham: Springer International Publishing), 281–298. doi:10.1007/978-3-319-29315-8_11
Basso, D. (1995). Living Calcareous Algae by a Paleontological Approach: the Genus Lithothamnion Heydrich Nom. Cons. From the Soft Bottoms of the Tyrrhenian Sea (Mediterranean). Riv. Ital. Paleontol. Stratigr. 101, 349–366. doi:10.13130/2039-4942/8592
Basso, D. (1998). Deep Rhodolith Distribution in the Pontian Islands, Italy: a Model for the Paleoecology of a Temperate Sea. Palaeogeogr. Palaeoclimatol. Palaeoecol. 137, 173–187. doi:10.1016/S0031-0182(97)00099-0
Basso, D. (2012). Carbonate Production by Calcareous Red Algae and Global Change. Geodiversitas 34, 13–33. doi:10.5252/g2012n1a2
Basso, D., Rodondi, G., and Caragnano, A. (2014). “Coralline Species Composition of Tyrrhenian Maerl Beds (Western Mediterranean),” in UNEP/MAP - RAC/SPA Proceedings of the 2nd Mediterranean Symposium on the Conservation of Coralligenous and Other Calcareous Bio-Concretions. Editors f. C. Bouafi, H. Langar, and A. Ouerghi (Portorož, Slovenia, 197–198.
Betzler, C., Braga, J. C., Jaramillo-Vogel, D., Römer, M., Hübscher, C., Schmiedl, G., et al. (2011). Late Pleistocene and Holocene Cool-Water Carbonates of the Western Mediterranean Sea. Sedimentology 58, 643–669. doi:10.1111/j.1365-3091.2010.01177.x
Birkett, D. A., Maggs, C. A., and Dring, M. J. (1998). An Overview of Dynamic and Sensitivity Characteristics for Conservation Management of Marine SACs (Vol. 5. Maerl, [UK Marine SACs Project]). Scott. Assoc. Mar. Sci. (SAMS) 5, 1–90.
Bordehore, C., Ramos-Esplá, A. A., and Riosmena-Rodríguez, R. (2003). Comparative Study of Two Maerl Beds with Different Otter Trawling History, Southeast Iberian Peninsula. Aquat. Conserv. Mar. Freshw. Ecosyst. 13, S43–S54. doi:10.1002/aqc.567
Borg, J. A., Lanfranco, E., Mifsud, J. R., Rizzo, M., and Schembri, P. J. (1998). in Does Fishing Have an Impact on Maltese Maerl Grounds? Heraklion, Crete, 18.
Bosellini, A., and Ginsburg, R. N. (1971). Form and Internal Structure of Recent Algal Nodules (Rhodolites) from Bermuda. J. Geol. 79, 669–682. doi:10.1086/627697
Bosence, D. W. J. (1983). “Description and Classification of Rhodoliths (Rhodoids, Rhodolites),” in Coated Grains. Editor T. M. Peryt (Berlin: Springer-Verlag), 217–224. doi:10.1007/978-3-642-68869-0_19
Bosence, D. W. J. (1976). Ecological Studies on Two Unattached Coralline Algae from Western Ireland. Paleontology 19, 365–395.
Bracchi, V. A., Angeletti, L., Marchese, F., Taviani, M., Cardone, F., Hajdas, I., et al. (2019). A Resilient Deep-Water Rhodolith Bed off the Egadi Archipelago (Mediterranean Sea) and its Actuopaleontological Significance. Alp. Mediterr. Quat. 32, 131–150. doi:10.26382/AMQ.2019.09
Bracchi, V. A., Caronni, S., Meroni, A. N., Burguett, E. G., Atzori, F., Cadoni, N., et al. (2022). Morphostructural Characterization of the Heterogeneous Rhodolith Bed at the Marine Protected Area “Capo Carbonara” (Italy) and Hydrodynamics. Diversity 14 (1), 51. doi:10.3390/d14010051
Braga, J. C., Aguirre, J., and Esteban, J. (2009). “Calcareous algae of Cabo de Gata-Níjar Nature Park. Field guide/Algas calcáreas del Parque Natural de Cabo de Gata. Guía de campo (Bilingual English/Spanish),” in ACUMEN y Consejería de Medio Ambiente (Junta de Andalucía=. Editors M. Villalobos, and A. B. Pérez-Muñoz (Madrid: Aguas de las Cuencas Mediterráneas).
Braga, J. C. (2017). “Neogene Rhodoliths in the Mediterranean Basins,” in Rhodolith/Maërl Beds: A Global Perspective Coastal Research Library. Editors R. Riosmena-Rodríguez, W. Nelson, and J. Aguirre (Cham: Springer International Publishing), 169–193. doi:10.1007/978-3-319-29315-8_7
Cabioch, J. (1969). Les fonds de Maerl de la Baie de Morlaix et leur peuplement végétal. Cah. Biol. Mar. 10, 139–161.
Cañadas, E. M., Fenu, G., Peñas, J., Lorite, J., Mattana, E., and Bacchetta, G. (2014). Hotspots within Hotspots: Endemic Plant Richness, Environmental Drivers, and Implications for Conservation. Biol. Conserv. 170, 282–291. doi:10.1016/j.biocon.2013.12.007
Caragnano, A., Rodondi, G., Basso, D., Peña, V., le Gall, L., and Rindi, F. (2020). Circumscription of Lithophyllum Racemus (Corallinales, Rhodophyta) from the Western Mediterranean Sea Reveals the Species Lithophyllum Pseudoracemus Sp. Nov. Phycologia 59, 584–597. doi:10.1080/00318884.2020.1829348
Carvalho, V. F., Assis, J., Serrão, E. A., Nunes, J. M., Anderson, A. B., Batista, M. B., et al. (2020). Environmental Drivers of Rhodolith Beds and Epiphytes Community along the South Western Atlantic Coast. Mar. Environ. Res. 154, 104827. doi:10.1016/j.marenvres.2019.104827
Chimienti, G., Rizzo, L., Kaleb, S., Falace, A., Fraschetti, S., Giosa, F. D., et al. (2020). Rhodolith Beds Heterogeneity along the Apulian Continental Shelf (Mediterranean Sea). J. Mar. Sci. Eng. 8, 813. doi:10.3390/jmse8100813
Cormaci, M., Furnari, G., and Alongi, G. (2017). Flora marina bentonica del Mediterraneo: Rhodophyta (Rhodymeniophycidae escluse). Boll. Accad. Gioenia Sci. Nat. 50, 1–391.
Costa, D. d. A., Da Silva, F. d. A., Silva, J. M. d. L., Pereira, A. R., Dolbeth, M., Christoffersen, M. L., et al. (2019). Is Tourism Affecting Polychaete Assemblages Associated with Rhodolith Beds in Northeastern Brazil? Rev. Biol. Trop. 67, S1–S15. doi:10.15517/rbt.v67is5.38922
CAR/ASP - PNUE/PAM (2013). in Communautés biologiques marines du Cap des Trois Fourches ( Mediterranée, Maroc): caracterisation, cartographie et orientations de gestion. Editors H. Par Bazairi, A. Limam, A. Benhoussa, C. Navarro-Barrancoa, A. R. González, M. Maestreet al. (Tunis: CAR/ASP-Projet MedMPAnet).
CAR/ASP - PNUE/PAM (2011). in Habitats marins et principales espèces des îles Kuriat (Tunisie) – Etude complémentaire: Formations naturelles d’intérêt pour la conservation. Editors H. Par Langar, C. Bouafif, A. Charfeddine, S. El Asmi, A. Limam, A. Ouerghiet al. (Tunis: CAR/ASP-Projet MedMPAnet).
De Jode, A., David, R., Haguenauer, A., Cahill, A. E., Erga, Z., Guillemain, D., et al. (2019). From Seascape Ecology to Population Genomics and Back. Spatial and Ecological Differentiation Among Cryptic Species of the Red Algae Lithophyllum Stictiforme/L. Cabiochiae, Main Bioconstructors of Coralligenous Habitats. Mol. Phylogenetics Evol. 137, 104–113. doi:10.1016/j.ympev.2019.04.005
Dolinar, D., Steller, D., Gabara, S., Beckley, B., Kim, J.-H., and Edwards, M. (2020). Impacts of Boat Mooring Disturbance on Productivity and Respiration in Rhodolith Beds from Catalina Island, USA. Cienc. Mar. 46, 253–267. doi:10.7773/cm.v46i4.3135
Foster, M., McConnico, L., Lundsten, L., Wadsworth, T., Kimball, T., Brooks, L., et al. (2007). Diversity and Natural History of a Lithothamnion Muelleri-Sargassum Horridum Community in the Gulf of California. Cienc. Mar. 33, 367–384. doi:10.7773/cm.v33i4.1174
Foster, M. S. (2001). Rhodoliths: Between Rocks and Soft Places. J. Phycol. 37, 659–667. doi:10.1046/j.1529-8817.2001.00195.x
Fragkopoulou, E., Serrão, E. A., Horta, P. A., Koerich, G., and Assis, J. (2021). Bottom Trawling Threatens Future Climate Refugia of Rhodoliths Globally. Front. Mar. Sci. 7, 594537(Accessed January 21, 2022). doi:10.3389/fmars.2020.594537
Fredericq, S., Krayesky-Self, S., Sauvage, T., Richards, J., Kittle, R., Arakaki, N., et al. (2019). The Critical Importance of Rhodoliths in the Life Cycle Completion of Both Macro- and Microalgae, and as Holobionts for the Establishment and Maintenance of Marine Biodiversity. Front. Mar. Sci. 5, 502. doi:10.3389/fmars.2018.00502
Gabrielson, P. W., Hughey, J. R., and Diaz‐Pulido, G. (2018). Genomics Reveals Abundant Speciation in the Coral Reef Building Alga Porolithon Onkodes (Corallinales, Rhodophyta). J. Phycol. 54, 429–434. doi:10.1111/jpy.12761
Gagnon, P., Matheson, K., and Stapleton, M. (2012). Variation in Rhodolith Morphology and Biogenic Potential of Newly Discovered Rhodolith Beds in Newfoundland and Labrador (Canada). Bot. Mar. 55, 85–99. doi:10.1515/bot-2011-0064
Gallardo, T., Bárbara, I., Alfonso-Carrillo, J., Bermejo, R., Altamirano, M., Gómez-Garreta, A., et al. (2016). Nueva lista crítica de las algas bentónicas marinas de España. Algas 51, 7–52.
García-Gómez, J. C., Corzo, J. R., López-Fé de la Cuadra, C. M., Sánchez Moyano, J. E., Corzo, M., Rey, J., et al. (2003). Metodología cartográfica submarina orientada a la gestión y conservación del medio litoral: mapa de las comunidades bentónicas del frente litoral norte del estrecho de Gibraltar. Bol. Inst. Esp. Oceanogr. 19, 149–163.
García-Gómez, J. C., Sempere-Valverde, J., González, A. R., Martínez-Chacón, M., Olaya-Ponzone, L., Sánchez-Moyano, E., et al. (2020). From Exotic to Invasive in Record Time: The Extreme Impact of Rugulopteryx Okamurae (Dictyotales, Ochrophyta) in the Strait of Gibraltar. Sci. Total Environ. 704, 135408. doi:10.1016/j.scitotenv.2019.135408
Gofas, S., Goutayer, J., Luque, Á. A., Salas, C., and Templado, J. (2014). Espacio Marino de Alborán. Proyecto LIFE+ INDEMARES Fundación Biodiversidad del Ministerio de Agricultura, Alimentación y Medio Ambiente.
Graham, D. J., and Midgley, N. G. (2000). Graphical Representation of Particle Shape Using Triangular Diagrams: an Excel Spreadsheet Method. Earth Surf. Process. Landforms 25, 1473–1477. doi:10.1002/1096-9837(200012)25:13<1473::aid-esp158>3.0.co;2-c
Guiry, M. D., and Guiry, G. M. (2022). AlgaeBase. World-wide Electronic Publication, National University of Ireland, Galway. Available at: https://www.algaebase.org (Accessed January 20, 2022).
Hall-Spencer, J. M., Grall, J., Moore, P. G., and Atkinson, R. J. A. (2003). Bivalve Fishing and Maerl-Bed Conservation in France and the UK?retrospect and Prospect. Aquat. Conserv. Mar. Freshw. Ecosyst. 13, S33–S41. doi:10.1002/aqc.566
Harvey, A. S., Harvey, R. M., Merton, E., Harvey, A. S., Harvey, R. M., and Merton, E. (2017). The Distribution, Significance and Vulnerability of Australian Rhodolith Beds: a Review. Mar. Freshw. Res. 68, 411–428. doi:10.1071/MF15434
Hernández-Kantún, J. J., Hall-Spencer, J. M., Grall, J., Adey, W., Rindi, F., Maggs, C. A., et al. (2017). “North Atlantic Rhodolith Beds,” in Rhodolith/Maërl Beds: A Global Perspective Coastal Research Library. Editors R. Riosmena-Rodríguez, W. Nelson, and J. Aguirre (Cham: Springer International Publishing), 265–279. doi:10.1007/978-3-319-29315-8_10
Jacquotte, R. (1962). Étude des fonds de maërl de Méditerranée. Rec. Trav. St. Mar. End. 26, 141–235.
Joher, S., Ballesteros, E., and Rodrigues-Prieto, C. (2015). Contribution to the Study of Deep Coastal Detritic Bottoms: the Algal Communities of the Continental Shelf off the Balearic Islands, Western Mediterranean. Medit. Mar. Sci. 16, 573–590. doi:10.12681/mms.1249
Kamenos, N. A., Burdett, H. L., and Darrenougue, N. (2017). “Coralline Algae as Recorders of Past Climatic and Environmental Conditions,” in Rhodolith/Maërl Beds: A Global Perspective Coastal Research Library. Editors R. Riosmena-Rodríguez, W. Nelson, and J. Aguirre (Cham: Springer International Publishing), 27–53. doi:10.1007/978-3-319-29315-8_2
Kamenos, N., Moore, P., and Hall-Spencer, J. (2004). Nursery-area Function of Maerl Grounds for Juvenile Queen Scallops Aequipecten Opercularis and Other Invertebrates. Mar. Ecol. Prog. Ser. 274, 183–189. doi:10.3354/meps274183
Kato, A., Basso, D., Caragnano, A., Rodondi, G., Le Gall, L., Peña, V., et al. (2022). Morphological and Molecular Assessment of Lithophyllum Okamurae with the Description of L. Neo-Okamurae Sp. Nov. (Corallinales, Rhodophyta). Phycologia 61, 117–131. doi:10.1080/00318884.2021.2005330
Keegan, B. F. (1974). The Macrofauna of Maërl Substrates on the West Coast of Ireland. Cah. Biol. Mar. 15, 513–530. doi:10.1016/s0033-3182(74)71273-7
Lanfranco, E., Rizzo, M., Hall-Spencer, J., Borg, J. A., and Schembri, P. (1999). Maerl-forming Coralline Algae and Associated Phytobenthos from the Maltese Islands. Cent. Mediterr. Nat. 3, 1–6.
Mannino, A. M., Castriota, L., Beltrano, A. M., and Sunseri, G. (2002). The Epiflora of a Rhodolith Bed from the Island of Ustica (Southern Tyrrhenian Sea). Flora Mediterr. 12, 11–28.
Marrack, E. C. (1999). The Relationship between Water Motion and Living Rhodolith Beds in the Southwestern Gulf of California, Mexico. Palaios 14, 159–171. doi:10.2307/3515371
Massutí, E., Sánchez-Guillamón, O., Farriols, M. T., Palomino, D., Frank, A., Bárcenas, P., et al. (2022). Improving Scientific Knowledge of Mallorca Channel Seamounts (Western Mediterranean) within the Framework of Natura 2000 Network. Diversity 14, 4. doi:10.3390/d14010004
Millar, K., and Gagnon, P. (2018). Mechanisms of Stability of Rhodolith Beds: Sedimentological Aspects. Mar. Ecol. Prog. Ser. 594, 65–83. doi:10.3354/meps12501
Ministerio de Agricultura, Alimentación y Medio Ambiente (2012). Estrategia marina demarcación marina del Estrecho y Alborán. Parte IV: Descriptores del buen estado ambiental. Descriptor 1: Biodiversidad, evaluación inicial y buen estado ambiental. Madrid.
Navarro-Barranco, C., Muñoz-Gómez, B., Saiz, D., Ros, M., Guerra-García, J. M., Altamirano, M., et al. (2019). Can Invasive Habitat-Forming Species Play the Same Role as Native Ones? the Case of the Exotic Marine Macroalga Rugulopteryx Okamurae in the Strait of Gibraltar. Biol. Invasions 21, 3319–3334. doi:10.1007/s10530-019-02049-y
Nelson, W. A. (2009). Calcified Macroalgae - Critical to Coastal Ecosystems and Vulnerable to Change: a Review. Mar. Freshw. Res. 60, 787–801. doi:10.1071/MF08335
Nitsch, F., Nebelsick, J. H., and Bassi, D. (2015). Constructional and Destructional Patterns-Void Classification of Rhodoliths from Giglio Island, Italy. Palaios 30, 680–691. doi:10.2110/palo.2015.007
O'Connell, L. G., James, N. P., Harvey, A. S., Luick, J., Bone, Y., and Shepherd, S. A. (2021). Reevaluation of the Inferred Relationship between Living Rhodolith Morphologies, Their Movement, and Water Energy: Implications for Interpreting Paleoceanographic Conditions. Palaios 35, 543–556. doi:10.2110/palo.2019.101
Ocaña, O., Ramos, A., and Templado, J. (2009). Los paisajes sumergidos de la región de Ceuta y su biodiversidad. Ceuta: Fundación Museo del Mar de Ceuta.
O’Leary, J. K., and McClanahan, T. R. (2010). Trophic Cascades Result in Large-Scale Coralline Algae Loss through Differential Grazer Effects. Ecology 91, 3584–3597. doi:10.1890/09-2059.1
Ordines, F., Bauzá, M., Sbert, M., Roca, P., Gianotti, M., and Massutí, E. (2015). Red Algal Beds Increase the Condition of Nekto-Benthic Fish. J. Sea Res. 95, 115–123. doi:10.1016/j.seares.2014.08.002
Otero-Ferrer, F., Cosme, M., Tuya, F., Espino, F., and Haroun, R. (2020). Effect of Depth and Seasonality on the Functioning of Rhodolith Seabeds. Estuar. Coast. Shelf Sci. 235, 106579. doi:10.1016/j.ecss.2019.106579
Peña, V., and Bárbara, I. (2009). Distribution of the Galician Maerl Beds and Their Shape Classes (Atlantic Iberian Peninsula): Proposal of Areas in Future Conservation Actions. Cah. Biol. Mar. 50, 353–368. doi:10.1080/09670261003586938
Peña, V., and Bárbara, I. (2010). Seasonal Patterns in the Maërl Community of Shallow European Atlantic Beds and Their Use as a Baseline for Monitoring Studies. Eur. J. Phycol. 45, 327–342. doi:10.1080/09670261003586938
Peña, V., Bárbara, I., Grall, J., Maggs, C. A., and Hall-Spencer, J. M. (2014). The Diversity of Seaweeds on Maerl in the NE Atlantic. Mar. Biodiv 44, 533–551. doi:10.1007/s12526-014-0214-7
Peña, V., Pardo, C., López, L., Carro, B., Hernández-Kantún, J., Adey, W. H., et al. (2015). Phymatolithon Lusitanicumsp. Nov. (Hapalidiales, Rhodophyta): The Third Most Abundant Maerl-Forming Species in the Atlantic Iberian Peninsula. Cryptogam. Algol. 36, 429–459. doi:10.7872/crya/v36.iss4.2015.429
Pérès, J. M., and Picard, J. (1964). Nouveau manuel de bionomie benthique de la Mer Méditerranée. Rec. Trav. St. Mar. End. 31, 5–137.
Pezzolesi, L., Peña, V., Le Gall, L., Gabrielson, P. W., Kaleb, S., Hughey, J. R., et al. (2019). MediterraneanLithophyllum stictiforme(Corallinales, Rhodophyta) Is a Genetically Diverse Species Complex: Implications for Species Circumscription, Biogeography and Conservation of Coralligenous Habitats. J. Phycol. 55, 473–492. doi:10.1111/jpy.12837
Puertos del Estado (2021a). Clima medio de oleaje. Nodo SIMAR 2039080. Banco de datos oceánicos de Puertos del Estado. Visor Portus. Madrid: Ministerio de Transporte, Movilidad y Agenda Urbana. Available at: https://portus.puertos.es.
Puertos del Estado (2021b). Temperatura Agua. Punto Satélite Sentinel 2039080. Visor Portus. Madrid: Ministerio de Transporte, Movilidad y Agenda Urbana. Available at: https://portus.puertos.es.
R Core Team (2021). R: A Language and Environment for Statistical Computing. Vienna, Austria: R Foundation for Statistical Computing. Available at: https://www.R-project.org/.
Ramos-Esplá, A. A., Riosmena-Rodríguez, R., and Galil, B. (2012). “Contribution to the Knowledge of Maerl Beds in the Levantine Basin,” in Eastern Mediterranean (Granada, Spain: IV International Rhodolith Workshop), 63.
REDIAM (2021). WMS Serie de imágenes de satélite AQUA MODIS. Clorofila-a medias estacionales filtradas de la serie histórica: Años 2000-2020, 1100 m. Océano Atlántico y Mar de Alborán. Red de Información Ambiental de Andalucía. Junta de Andalucía. Available at: https://www.juntadeandalucia.es/medioambiente/mapwms/REDIAM_aquamodis_cl_a_estacional_filtrada_2000_2020?
Rendina, F., Donnarumma, L., Appolloni, L., Bruno, R., Ferrigno, F., Di Stefano, F., et al. (2017). First Description of a Rhodolith Bed off the Island of Capri and its Associated Benthic Fauna. Biol. Mar. Mediterr. 24, 126–127.
Rendina, F., Ferrigno, F., Appolloni, L., Donnarumma, L., Sandulli, R., and Fulvio, G. (2020a). Anthropic Pressure Due to Lost Fishing Gears and Marine Litter on Different Rhodolith Beds off the Campania Coast (Tyrrhenian Sea, Italy). Ecol. Quest. 31, 1–51. doi:10.12775/EQ.2020.027
Rendina, F., Kaleb, S., Caragnano, A., Ferrigno, F., Appolloni, L., Donnarumma, L., et al. (2020b). Distribution and Characterization of Deep Rhodolith Beds off the Campania Coast (SW Italy, Mediterranean Sea). Plants 9, 985. doi:10.3390/plants9080985
Rindi, F., Braga, J. C., Martin, S., Peña, V., Le Gall, L., Caragnano, A., et al. (2019). Coralline Algae in a Changing Mediterranean Sea: How Can We Predict Their Future, if We Do Not Know Their Present? Front. Mar. Sci. 6, 723. doi:10.3389/fmars.2019.00723
Riosmena-Rodríguez, R. (2017). “Natural History of Rhodolith/Maërl Beds: Their Role in Near-Shore Biodiversity and Management,” in Rhodolith/Maërl Beds: A Global Perspective Coastal Research Library. Editors R. Riosmena-Rodríguez, W. Nelson, and J. Aguirre (Cham: Springer International Publishing), 3–26. doi:10.1007/978-3-319-29315-8_1
Robles, R. (2010). Conservación y desarrollo sostenible del mar de Alborán = Conservation et développement durable de la mer d’Alboran. Málaga, Spain: IUCN. Available at: https://portals.iucn.org/library/node/9542 (Accessed January 26, 2022).
RStudio Team (2020). RStudio: Integrated Development for R. Boston, MA: RStudio, PBC. Available at: http://www.rstudio.com.
Salomidi, M., Katsanevakis, S., Borja, A., Braeckman, U., Damalas, D., Galparsoro, I., et al. (2012). Assessment of Goods and Services, Vulnerability, and Conservation Status of European Seabed Biotopes: a Stepping Stone towards Ecosystem-Based Marine Spatial Management. Medit. Mar. Sci. 13, 49–88. doi:10.12681/mms.23
Sañé, E., Chiocci, F. L., Basso, D., and Martorelli, E. (2016). Environmental Factors Controlling the Distribution of Rhodoliths: An Integrated Study Based on Seafloor Sampling, ROV and Side Scan Sonar Data, Offshore the W-Pontine Archipelago. Cont. Shelf Res. 129, 10–22. doi:10.1016/j.csr.2016.09.003
Sciberras, M., Rizzo, M., Mifsud, J. R., Camilleri, K., Borg, J. A., Lanfranco, E., et al. (2009). Habitat Structure and Biological Characteristics of a Maerl Bed off the Northeastern Coast of the Maltese Islands (Central Mediterranean). Mar. Biodiv. 39, 251–264. doi:10.1007/s12526-009-0017-4
Sempere-Valverde, J., Ostalé-Valriberas, E., Maestre, M., González Aranda, R., Bazairi, H., and Espinosa, F. (2021). Impacts of the Non-indigenous Seaweed Rugulopteryx Okamurae on a Mediterranean Coralligenous Community (Strait of Gibraltar): The Role of Long-Term Monitoring. Ecol. Indic. 121, 107135. doi:10.1016/j.ecolind.2020.107135
Sneed, E. D., and Folk, R. L. (1958). Pebbles in the Lower Colorado River, Texas a Study in Particle Morphogenesis. J. Geol. 66, 114–150. doi:10.1086/626490
Steller, D., and Cáceres-Martínez, C. (2009). Coralline Algal Rhodoliths Enhance Larval Settlement and Early Growth of the Pacific Calico Scallop Argopecten Ventricosus. Mar. Ecol. Prog. Ser. 396, 49–60. doi:10.3354/meps08261
Steller, D. L., and Foster, M. S. (1995). Environmental Factors Influencing Distribution and Morphology of Rhodoliths in Bahía Concepción, B.C.S., México. J. Exp. Mar. Biol. Ecol. 194, 201–212. doi:10.1016/0022-0981(95)00086-0
Steller, D. L., Riosmena-Rodríguez, R., Foster, M. S., and Roberts, C. A. (2003). Rhodolith Bed Diversity in the Gulf of California: the Importance of Rhodolith Structure and Consequences of Disturbance. Aquat. Conserv. Mar. Freshw. Ecosyst. 13, S5–S20. doi:10.1002/aqc.564
Steneck, R. S. (1986). THE ECOLOGY of CORALLINE ALGAL CRUSTS: Convergent Patterns and Adaptative Strategies. Annu. Rev. Ecol. Syst. 17, 273–303. doi:10.1146/annurev.es.17.110186.001421
Teichert, S., Woelkerling, W., Rüggeberg, A., Wisshak, M., Piepenburg, D., Meyerhöfer, M., et al. (2014). Arctic Rhodolith Beds and Their Environmental Controls (Spitsbergen, Norway). Facies 60, 15–37. doi:10.1007/s10347-013-0372-2
Townsend, R. A., and Huisman, J. M. (2018). “Coralline Algae,” in Algae of Australia. Marine Benthic Algae of North-Western Australia. 2. Red Algae. Editor J. M. Huisman (Canberra & Melbourne: ABRS & CSIRO Publishing), 143–146. 86–97, 105–137.
Villas-Bôas, A. B., Tâmega, F. T. D. S., Andrade, M., Coutinho, R., and Figueiredo, M. A. D. O. (2014). Experimental effects of sediment burial and light attenuation on two coralline algae of a deep water rhodolith bed in Rio de Janeiro, Brazil. Cryptogam. Algol. 35, 67–76. doi:10.7872/crya.v35.iss1.2014.67
Keywords: coralline red algae, depth-gradient patterns, rhodolith cover and size, rhodolith diversity, Alboran sea
Citation: Del Río J, Ramos DA, Sánchez-Tocino L, Peñas J and Braga JC (2022) The Punta de la Mona Rhodolith Bed: Shallow-Water Mediterranean Rhodoliths (Almuñecar, Granada, Southern Spain). Front. Earth Sci. 10:884685. doi: 10.3389/feart.2022.884685
Received: 14 March 2022; Accepted: 30 May 2022;
Published: 22 June 2022.
Edited by:
Daniela Basso, University of Milano-Bicocca, ItalyReviewed by:
Nadine Schubert, University of Algarve, PortugalCopyright © 2022 Del Río, Ramos, Sánchez-Tocino, Peñas and Braga. This is an open-access article distributed under the terms of the Creative Commons Attribution License (CC BY). The use, distribution or reproduction in other forums is permitted, provided the original author(s) and the copyright owner(s) are credited and that the original publication in this journal is cited, in accordance with accepted academic practice. No use, distribution or reproduction is permitted which does not comply with these terms.
*Correspondence: Juan Carlos Braga, amJyYWdhQHVnci5lcw==
Disclaimer: All claims expressed in this article are solely those of the authors and do not necessarily represent those of their affiliated organizations, or those of the publisher, the editors and the reviewers. Any product that may be evaluated in this article or claim that may be made by its manufacturer is not guaranteed or endorsed by the publisher.
Research integrity at Frontiers
Learn more about the work of our research integrity team to safeguard the quality of each article we publish.