- Research Institute of Exploration and Development, Tarim Oilfield Company, PetroChina, Korla, China
Fracture effectiveness evaluation has an important impact on the efficient exploration and development of ultra-deep fractured reservoirs. Based on the liquid production profile results collected during the development of the Keshen gas field and based on the traditional static evaluation of fracture density, opening and filling degree, the fracture effectiveness evaluation of ultra-deep reservoirs is carried out from the perspectives of fracture activity, fracture opening and fracture connectivity. The results show that the static characteristics, such as fracture density and fracture properties, are only some factors that determine the effectiveness of fractures. From the perspective of contributions to reservoir permeability, dynamic parameters such as fracture activity, fracture opening and fracture connectivity are the key factors in determining the effectiveness of fractures. Practice has proven that fractures with a high shear ratio, low stress environment and high connection with the fracture system around the well are the main seepage channels for oil and gas supply in the development process. The research results provide a scientific basis for well location deployment, optimization of reconstruction intervals and optimization of development schemes.
Introduction
In recent years, ultra-deep layers have gradually become the key field of oil and gas resource development and the hotspot of exploration and development (Jia et al., 2014; Li et al., 2020). According to the classification of oil and gas reservoir types in China, a burial depth of more than 4,500 m is generally defined as an ultra-deep layer, while an ultra-deep layer in western China generally refers to a burial depth of more than 6,000 m (He et al., 2019). The Keshen gas field in the Kuqa depression, Tarim Basin, is one of the typical ultra-deep oil and gas resource enrichment areas in China. Exploration breakthroughs have been achieved in Keshen 2, Keshen 5 and Keshen 24. The Cretaceous sandstone reservoirs of ks 2 and ks 5 are buried more than 6,000 m, and the reservoirs of Keshen 9 and Keshen 21 are even more than 8,000 m, belonging to the category of typical ultra-deep (Tian et al., 2020; Wang et al., 2021; Tian et al., 2021).
However, at this depth, the rock is in a very strong in situ stress environment (Xu et al., 2020), and the horizontal principal stress and vertical principal stress exceed 200 MPa. Under the action of strong compaction and compression, the diagenesis of reservoir rocks is strong, the porosity is low, approximately 2–7%, and the matrix permeability is as low as 0.01∼1mD. Strong compression causes extremely developed natural fractures in the formation, which greatly improves the reservoir permeability and provides the main migration channel for underground oil and gas (Tang et al., 2017; Shi C. et al., 2020; Zhang et al., 2020; Wang et al., 2021).
At present, scholars have carried out research on the mechanism of fracture genesis (Wang et al., 2018; Gong et al., 2019; Yang et al., 2021), multiscale fracture division and description (Liu C. et al., 2017; Liu et al., 2019; Espejel et al., 2020), and quantitative prediction of structural fracture parameters for ultra-deep fractures in the Kuqa depression (Liu J. et al., 2017; Li et al., 2020; Lan et al., 2021). They generally use parameters such as fracture density and fracture opening to characterize the effectiveness of fractures (Ju et al., 2020; Mao et al., 2020; Shi J. et al., 2020; Lv et al., 2021) and believe that the parts with dense fracture development and large openings in the high part of the anticline are conducive to the high production of gas wells (Yang et al., 2016; Zhou et al., 2018). However, the practice of the Tarim oilfield shows that the ultra-deep reservoir in the Kuqa depression has strong heterogeneity and great productivity differences between wells. The conventional physical properties, fracture density and other parameters cannot well reflect the reservoir quality and cannot be generally considered the main controlling factor of high production of oil and gas wells (Zhang et al., 2019; Wang J. et al., 2021). In the anticline structure, the traditional well location deployment mode of “along the long axis and occupying the high point” also faces certain limitations in the ultra-deep oil and gas exploration and development of the Kuqa depression. How to define effective fractures, find favorable reservoirs and realize efficient exploration and development has become an urgent task.
To solve this problem, the key is to clarify the contribution of different fractures to the reservoir seepage capacity and the control effect on productivity under the background of ultra-deep complex and strong in situ stress and reveal the main controlling factors of high production of oil and gas wells. Therefore, based on the production practice data and the research of traditional fracture density and filling degree, the author carries out geomechanical research, reveals the relationship between fractures and reservoir and productivity, and puts forward the fracture effectiveness evaluation method to support the efficient development of ultra-deep oil and gas.
Geological Setting
The Kuqa depression is located in the northern margin of the Tarim Basin, adjacent to the Tianshan fold belt in the north and the Tabei Uplift in the south. It is a Mesozoic Cenozoic superimposed foreland basin developed on the basis of Paleozoic passive continental margins, with a total area of approximately 2.85 ha × 104 km2. It includes seven secondary structural units: the Northern structural belt, Kelasu structural belt, Qiulitage structural belt, Baicheng sag, Yangxia sag, Wushi sag and southern slope belt (Figure 1). The Kuqa depression began to develop in the late Hercynian period and experienced many tectonic movements, such as the development period of the paleoforeland basin in the Late Permian Triassic, the extension depression period in the Jurassic Paleogene and the development period of intracontinental foreland thrust in the Neogene Quaternary (Li et al., 2017). Under the background of this large deformation scale and complex structural evolution, the Kuqa depression develops a large number of multistage superimposed structural fractures. Fractures of different degrees are developed in the Keshen gas reservoir, Bozi Dabei gas reservoir, Dina gas reservoir in the Qiulitage structural belt, mid-autumn gas field and Dibei tight gas reservoir in the northern structural belt. The structural style of Keshen gas field is a typical foreland thrust imbricate structure, which was formed in the Mesozoic Yanshan movement and the Cenozoic Himalayan movement. Several fault anticline natural gas reservoirs such as Keshen 2, Keshen 8, Keshen 9 and Keshen 13 are developed from north to south (Figure 2), with a total resource of more than 1.0 × 1012m ³ (Neng et al., 2013).
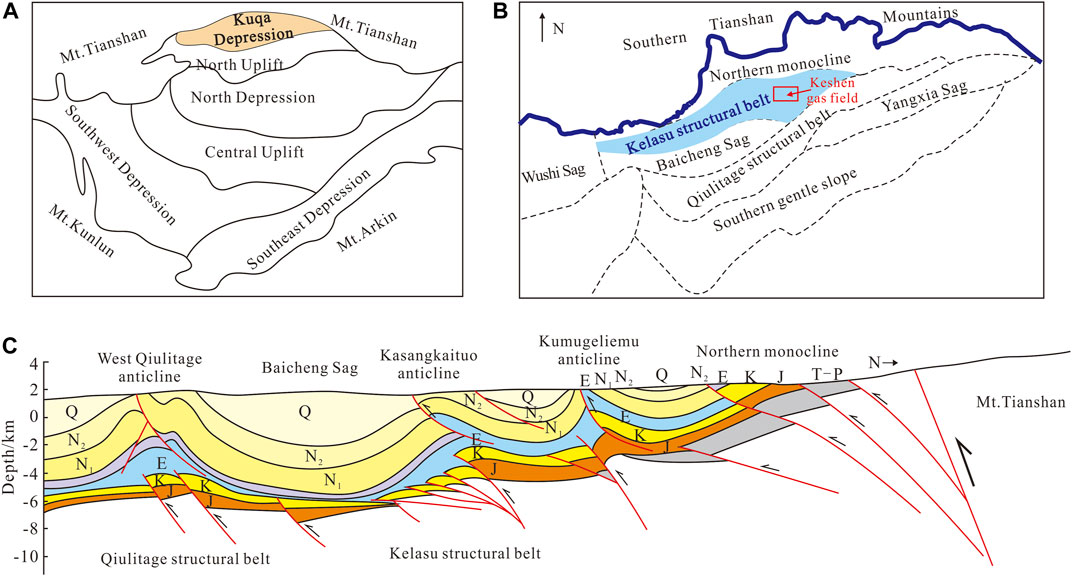
FIGURE 1. Division of structural units and geological profile of the Kuqa Depression. (A) Kuqa depression is located in the north of Tarim Basin; (B) Kuqa depression is divided into seven secondary structural units. Keshen gas field is located in the east of Kelasu structural belt; (C) Thrust imbricate structures are widely developed in Kelasu structural belt.
The strata in the study area are Quaternary, Neogene, Paleogene and Cretaceous from top to bottom. The Neogene is further divided into the Kuqa Formation (N2k), Kangcun Formation (N1k)and Jidick Formation (N1j); the Suweiyi Formation (E2-3s) and Kumgliem Group (E1-2km) developed in the Paleogene, and the Kumgliem Group developed with an extremely thick salt gypsum layer (the thickest is more than 5,800 m), which is a regional caprock. The Bashkiqike Formation (K1bs), Brazil reorganization (K1bx) and shushanhe Formation (K1s) developed in the Cretaceous. The main horizon of the Keshen gas reservoir in the Kelasu structural belt is the Bashijiqike Formation, which belongs to the sedimentary background of the depression lake basin (Zhou et al., 2016). It is dominated by massive sand and widely covers a very thick sand body. The sedimentary facies belt in the Kelasu structural belt is characterized by an east–west distribution and north–south zoning. According to the lithology and lithologic combination sequence, the strata of the Bashijiqike Formation can be further divided into three members, namely, the Ba 1 member, Ba 2 member and Ba 3 member. Among them, the Ba 3 member belongs to a fan delta sedimentary environment, the Ba 2 member and Ba 1 member belong to a braided river delta sedimentary environment, and the sand body types are mainly braided river delta underwater distributary channel sand and estuary bar sand (Pan et al., 2013; Zhang et al., 2015).
The rock intensity of the Bashijiqike Formation is mainly lithic arkose, in which the content of quartz particles is 45–65.0%, the content of feldspar particles is 20.0–35.0%, and the content of rock cuttings is 14.0–30.0% (Han et al., 2009; Pan et al., 2014). The miscellaneous base includes argillaceous and argillaceous iron, and the cement is mainly calcite, dolomite and anhydrite. The clay minerals are mainly the illite/montmorillonite mixed layer, illite and chlorite. The clastic particles are moderately well sorted and sub rounded. The particles are mainly in point and point line contact. The rock components generally show the characteristics of medium to high structural maturity. The reservoir space is dominated by residual primary intergranular pores and intergranular dissolved pores, followed by intragranular dissolved pores and micropores.
Fracture Characteristics
The Cretaceous Bashijiqike Formation in the Keshen gas field is strongly compressed by Himalayan tectonic movement, and the fractures are mainly of structural origin. Affected by lithology, stress neutral plane and other factors, the development characteristics of structural fractures are quite different. From the perspective of mechanical mechanisms, fractures can be divided into tensile fractures and shear fractures, both of which are developed to varying degrees. From the perspective of fracture occurrence, fractures can be divided into vertical fractures, high-angle fractures, low-angle fractures and horizontal fractures. Among them, vertical fractures and high-angle fractures are mainly developed, accounting for more than 70%, and a small number of low-angle fractures and horizontal fractures account for less than 30%. There are many angles to describe the fracture development characteristics. This study describes the fracture development characteristics of different scales from the perspective of the fracture observation scale.
Microfracture Development Characteristics
Microfractures are mainly observed and studied by casting thin sections, laser confocal microscopy and micron CT. Such fractures are mainly distributed between particles. Sometimes microfractures cutting through particles can be observed. The fracture width is generally 1–100 μm, and the main body is concentrated between 20 µm. The fracture length distribution is unequal, between 1 and 10 mm. The fracture filling degree is low, and the opening property is good. There are various fracture forms, which are mainly affected by the composition and distribution of clastic particles. Even the same microfracture shows great differences in different positions. Some sections show straight fractures, while others have a large bending degree. On the whole, a large number of microfractures are developed, which reflects the strong structural compression of the reservoir to a certain extent (Figure 3).
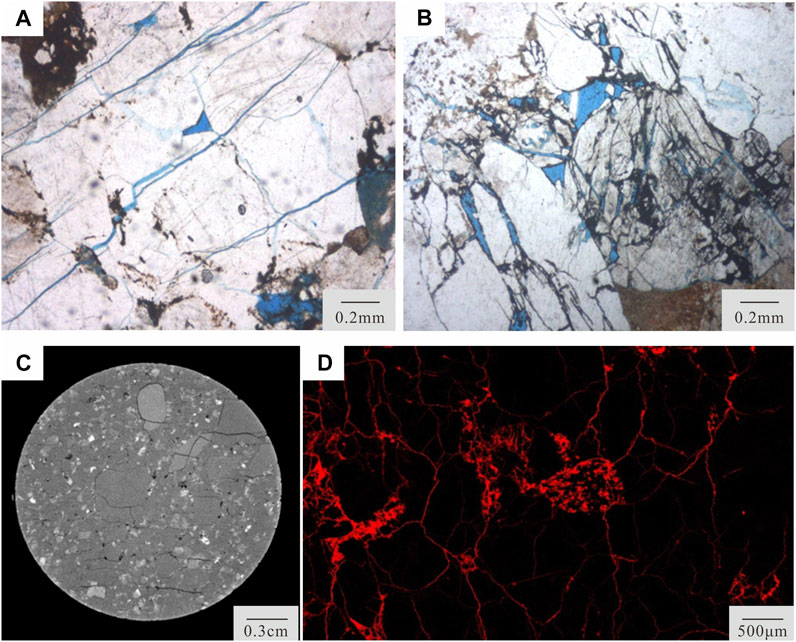
FIGURE 3. (A) Well ks2, 6,048.73 m, several microfractures are appeared in thin section, (B) Well ks2, 6,049.56 m, a series of microfractures in thin sections (C–D) Well ks2, laser confocal and micron CT of well ks2 in the Keshen gas field.
Core Fracture Development Characteristics
Core-scale fractures are mainly observed and studied by magnifying glass or the naked eye. Natural fractures often have the following characteristics on the core: the fracture surface is filled with minerals (Figure 4D), the fractures are developed inside the core (Figure 4B,C), the directivity is obvious, and the extension is long (Figures 4A,C,D); However, the artificial fracture forms an irregular shape such as ring on the core, which is mainly unloading fracture. It can be seen that the core fractures shown in Figure 3 are natural fractures. A large number of wells in the Keshen gas field have collected core data, and most of them are high angle oblique or vertical orthogonal tensile fractures or shear fractures, and some of them can see the development of network fractures. The dip angle of the fracture is between 60° and 90°, and a few low angle fractures are developed. The fracture opening is greater than 0.1 mm, generally between 0.1 and 0.5 mm, and even greater than 5 mm. The fracture length is more than 5 cm, generally between 5 and 50 cm. The degree of fracture filling varies greatly, which can be divided into full filling, partial filling and unfilled. Among them, tensile fractures with large openings and early formation generally have a high filling degree, and most of the filling materials are calcite. The openings are relatively small, the shear joints formed later are mostly semifilled or unfilled, and the filling materials are mostly calcite. According to the cutting relationship and filling degree of fractures, predecessors have performed much research on fracture stages.
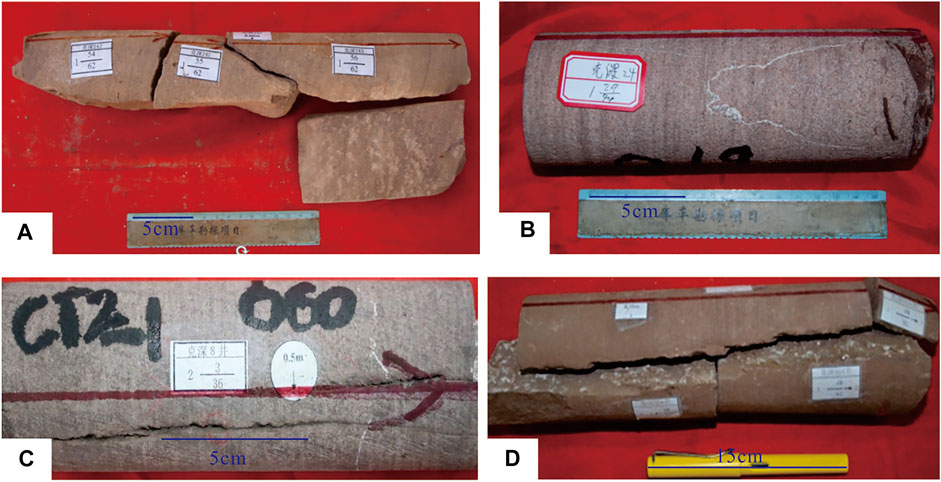
FIGURE 4. (A) Well ks242, K1bs, 6,451.5 m, Medium sandstone, it can be seen that high angle fracture and vertical fracture intersect obliquely; (B) Well ks24, K1bs, 6,179.2 m, a medium high angle fracture filled with calcite; (C) Well ks8, K1bs, 6,737.5 m, opened high angle fractures; (D) Well ks8, K1bs, 6874 m, half-filled vertical fracture.
Imaging Fracture Development Characteristics
Imaging scale fractures are mainly observed through acoustic and electrical imaging logging. Imaging logging can provide continuous borehole wall images of formations and help to study the distribution relationship of vertical fractures and the change in fracture occurrence. According to the imaging logging data, the fractures in the Keshen gas field are mostly high-angle oblique fractures, reticular fractures are developed in some strata, and low-angle fractures and horizontal fractures are not developed. The development characteristics of the fracture strike vary greatly at different positions of the structure. In the core area of the anticline structure, E-W tensile fractures along the axis of the anticline are relatively developed, and NW-SE or NE-SW shear fractures are mainly developed at a position far from the core area of the anticline. The fracture width is between 0.1 and 2 mm, and the fracture length is between 0.5 and 20 m. The fracture filling degree is similar to that observed in the core, with a great difference. It can be divided into fully filled fractures, partially filled fractures and unfilled fractures (Figure 5).
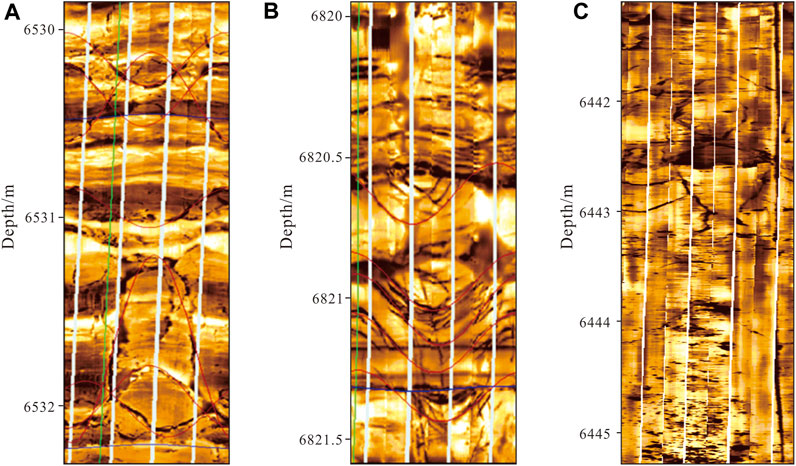
FIGURE 5. (A) Well ks204, 6,530 ∼ 6,532 m, network fractures; (B) Well ks803, 6,820 ∼6,821.5 m, a group of parallel fractures; (C) Well ks24, 6,442 ∼6,445 m, Several medium and low angle fractures.
Fractures of different scales have different contributions to reservoir permeability. Imaging fractures and core-scale fractures have a direct contribution to reservoir permeability. The influence of microfractures on reservoir permeability is still unclear. According to previous studies, microfractures may increase reservoir pore space.
Evaluation Method of Fracture Effectiveness in Ultra-Deep Reservoir
Fracture effectiveness is the degree to which the fracture is open under the condition of oil and gas reservoirs and can provide effective circulation space for underground fluid flow. The effectiveness evaluation of fractures is very important for fractured reservoirs. Due to strong compaction, the porosity of the ultra-deep reservoir (6,000–8,000 m) in the Keshen area is only 4.0–8.0%, and the permeability is only 0.001 × 10–3 μm2∼0.500 × 10–3 μm2, but the well test permeability in this area is indeed as high as 1 × 10−3µm2∼100 × 10–3 μm2. Natural fractures provide the main permeability channel of the reservoir. According to the statistics of block development data, the average contribution rate of natural fracture permeability to formation permeability is 99.08%.
Traditionally, qualitative or semiquantitative evaluation methods have been established for fracture effectiveness evaluation from the factors of fracture itself, such as fracture density, fracture opening and fracture type, as well as external factors, such as in situ stress and fracture filling degree. It is considered that the filling degree, density and opening of fractures have a great influence on the effectiveness of fractures. However, according to the long-term development data and industrial profile data, the real contribution interval of formation fluid is inconsistent with the understanding of fracture density, fracture opening and whether the fracture is filled. For example, in well ks208 in the kes 2 well block, a large number of natural fractures are developed in the target layer (more than 100), but the test production is only 10 × 104 m3/d. However, only a small number of natural fractures are developed in the adjacent well ks228, but the test production is more than 30 × 104 m3/d. Similarly, according to the test results of the single-well liquid profile, only a small number of natural fractures are developed in the main production interval of well ks24-5 in the keshen 24 block (Figure 6). A stratum with a large number of natural fractures is not the main gas supply interval or even the seepage channel of underground fluid. It can be seen that fracture effectiveness is related not only to fracture density, fracture opening and other parameters but also to other control factors.
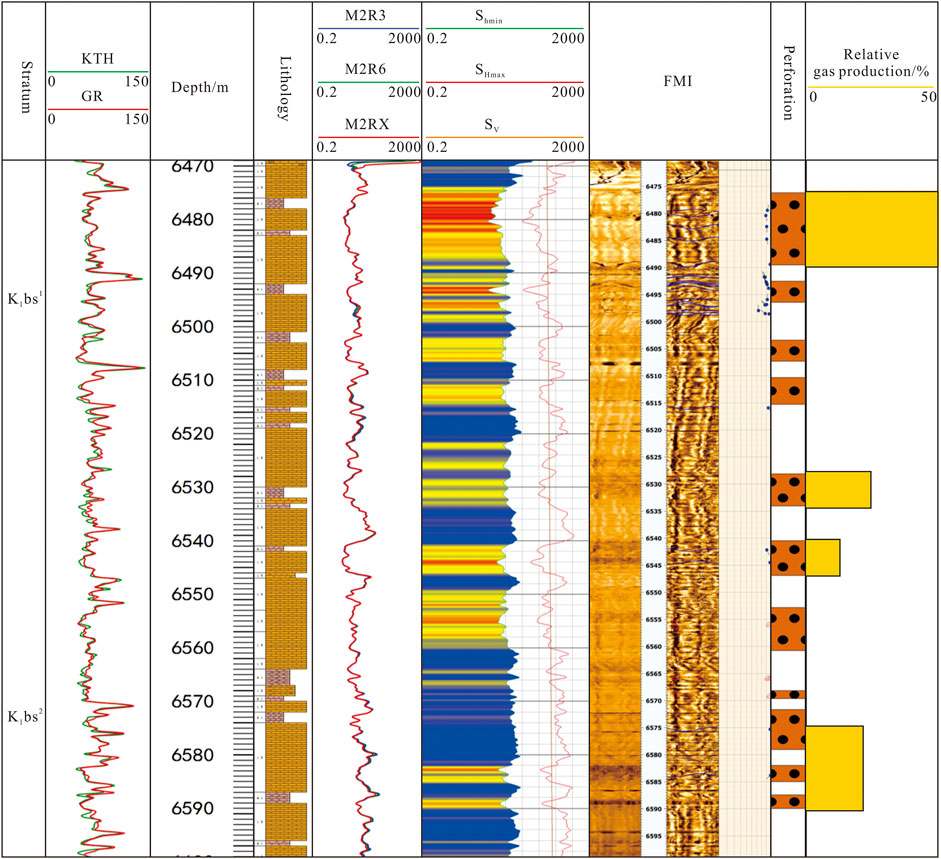
FIGURE 6. The liquid production profile of well ks24-5; The first trace in the figure is the stratum, the KTH in the second trace is the de uranium gamma, the fifth trace is the formation resistivity measurement results of different radial depths, m, and the sixth trace is the current in-situ stress, in which Shmin is the current minimum horizontal principal stress, MPa; Shmax is the current maximum horizontal principal stress, MPa; Sv is the vertical stress, MPa, the eighth channel is the perforation position, and the ninth channel is the contribution ratio of oil and gas production of each formation,%.
Therefore, based on the understanding of traditional fracture effectiveness evaluation and combined with the long-term development performance data of the ultra-deep gas field, the author innovates to carry out fracture effectiveness evaluation research from three aspects: fracture activity, fracture opening and fracture connectivity.
Fracture Activity
Fracture activity refers to the ability of natural fractures to shear slip under the action of in situ stress, which is usually used as the shear stress acting on the fracture surface τ and the effective normal stress on the fracture surface σ expressed as the ratio of n. The flow of oil, gas and other fluids in fractures is largely affected by the in situ stress state. Under the action of underground three-dimensional stress, the stress state of fractures with different occurrences is different. The stress acting on the natural fracture surface can be decomposed into the shear stress parallel to the fracture surface τ and the effective normal stress of the vertical fracture surface σn. The greater the angle between the natural fracture surface and the maximum horizontal principal stress is, the smaller the shear stress and the greater the effective normal stress; that is, the ratio of the τ/σn is low, the fracture tends to be stable. When the angle between the natural fracture surface and the maximum horizontal principal stress is smaller, the shear stress is larger, and the effective normal stress is smaller; that is, the ratio of the τ/σ is high, the fracture tends to shear slip. The fracture in the critical sliding state has better seepage ability, and the shear deformation activity ability of the fracture surface can be regarded as one of the symbols to judge the high-quality fracture.
Based on the productivity test data of fractured reservoirs, the stress of natural fractures is obviously related to productivity. Figure 7 shows the statistics of the fracture shear ratio and gas well production of more than 20 wells in the Keshen gas field. It can be found that the fracture shear ratio is positively related to gas well production. The research shows that the contribution of fracture activity to gas well productivity is even more important than its development degree (Zhang et al., 2019).
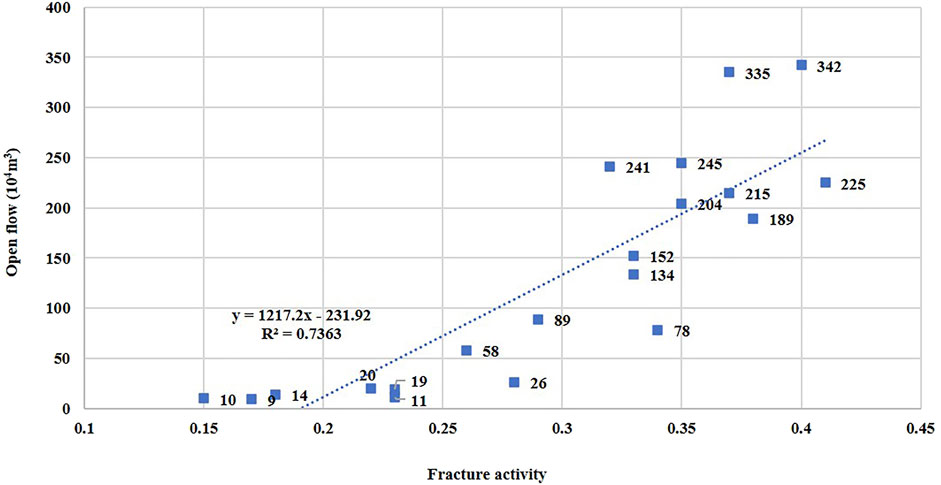
FIGURE 7. The relationship between fracture activity and open flow, the horizontal axis is fracture activity, and the vertical axis is open flow of gas well.
Fracture Opening
The natural productivity of ultra-deep fractured reservoirs is low and cannot meet the development needs. Reservoir reconstruction is often needed to meet the needs of efficient development. However, the minimum horizontal principal stress of the 6,000~8,000 m reservoir exceeds 140 MPa, and the reservoir fracture pressure is very high. At present, the engineering construction conditions have difficulty meeting the conditions for the formation of hydraulic fractures in the fractured reservoir matrix. The fracturing transformation of ultra-deep reservoirs is more likely to open natural fractures, connect wellbore fractures with the natural fracture network of the reservoir around the well, and form a dominant seepage channel to increase the productivity of oil and gas wells. Therefore, the development of fractures is conducive to reducing the difficulty of fracturing transformation. The opening pressure of natural fractures is very important for ultra-deep hydraulic fracturing and is an important index to measure the effectiveness of fractures.
When the fracture surface is closed, the normal stress of the section decreases due to the increase in pore pressure at the fracture surface, and the fracture surface may change from closed to open. The pressure when it changes to open is called the opening pressure. Theoretically, under the condition of complete elasticity, when the fluid pressure is greater than the principal stress on the joint surface, the joint surface can open and become a channel for dredging. In fact, due to the deformation characteristics of the rock stratum itself, even under the above conditions, the joint surface is not necessarily open, and the joint surface can be opened only by overcoming the deformation strength of the rock. According to the factors affecting the original stress state of natural fractures in the reservoir, the opening pressure of natural fractures can be calculated by the following methods (Zhu et al., 2016):
where
According to the Formula, the influencing factors of the natural fracture opening pressure mainly include the fracture burial depth, fracture dip angle and strike, formation fluid pressure and current in situ stress orientation and size. The deeper the fracture is buried, the greater the stress on the fracture, the smaller the fracture dip angle, the smaller the formation fluid pressure, the greater the included angle between the fracture strike and the azimuth of the maximum principal stress, and the greater the opening pressure of the fracture; in contrast, the smaller the fracture opening pressure is (Figure 8).
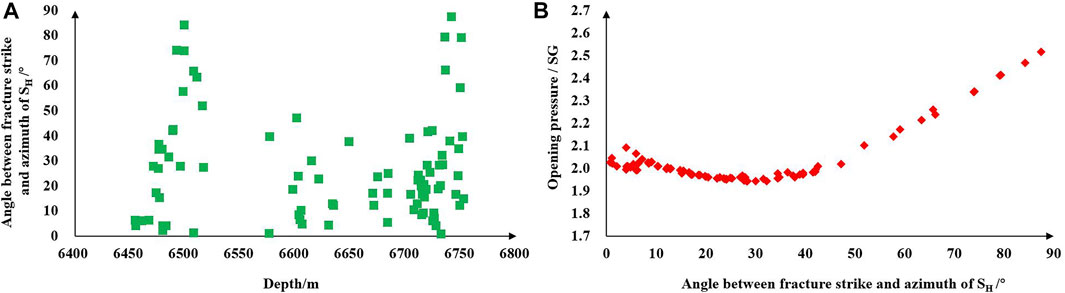
FIGURE 8. (A) is the statistical diagram of the included angle between the natural fracture strike and the current maximum principal stress azimuth of the stratum at different depths of well ks504. It can be seen from the figure that the included angle between the natural fracture strike and the current maximum principal stress azimuth of the well is widely distributed, with a large number of development at 0–90 °. (B) is the opening pressure distribution diagram of natural fractures with different included angles in well ks 504 calculated according to the above opening pressure calculation method. It can be seen from the figure that the variation range of fracture opening pressure is 1.94–2.52MPa/100 m, among which the opening pressure of natural fractures with an included angle of 28.8 ° is the lowest, which is 1.94MPa/100 m (the depth of fracture development formation is 6,733 m, and the opening pressure is 128 MPa), The maximum opening pressure of natural fractures with an included angle of 87.5 ° is 2.52MPa/100 m (the depth of fracture development formation is 6741m, and the opening pressure is 166.6 MPa).
The natural fractures in the ultra-deep reservoir of the Keshen gas field are buried deep (6,000–8,000 m), the original field stress is large (the minimum horizontal principal stress is greater than 140 MPa), and the fracture opening pressure is large. However, due to the high formation fluid pressure (greater than 100 MPa), the included angle between the natural fracture strike and the maximum principal stress azimuth is generally small, and the fracture activity is good, which reduces the fracture opening pressure to a certain extent. Therefore, in the process of optimizing the production well section and perforated interval, it is very important to select natural fractures with developed natural fractures and low opening pressure for ultra-deep reservoir reconstruction.
Figure 9 shows the in situ stress profile of well ks10X and the fracturing operation curve of the two layers. The fracture of the Shiji Formation is concentrated at the top of the Shiji Formation, and the fracture of the Qiji Formation is not obvious. In Brazil, a certain number of fractures are developed in the first section. From the fracturing curve of 7,263.00–7,320.00 m in the first section, it can be seen that with the increase in stage fluid volume, the oil pressure increases, and then there is an obvious decrease in oil pressure, indicating that the formation has been pressed open by high-pressure fluid. Then, with the increase in the stage fluid volume, the oil pressure keeps increasing, and there is an obvious decrease in the oil pressure. This cycle shows that the fracture is constantly formed and expanded; in contrast, the fracturing curve of 7,203.00–7,239.50 in the second section shows that with the increase in the stage fluid volume, the oil pressure gradually increases and does not decrease. It is still the same after several cycles, indicating that the formation has not been pressed open and has not been successfully fractured. This shows that under the current construction conditions, there are difficulties caused by ultra-deep fracturing, which is another major factor restricting high production.
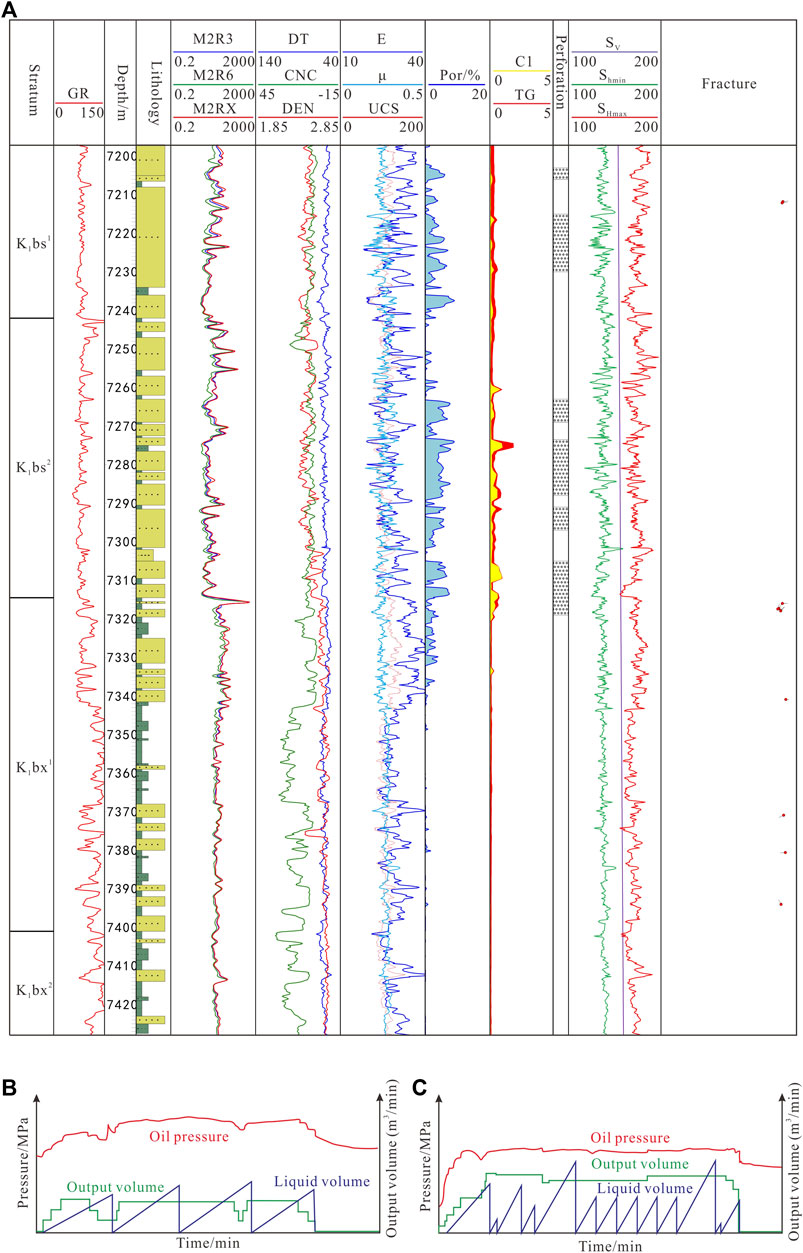
FIGURE 9. Geomechanical column and fracturing curve of Well ks10X. (A) is one-dimensional geomechanics and fracture development profile of well ks10x. Only two natural fractures are developed in 7200-7310 m section; Eight natural fractures are developed in the 7310-7400 m section. The overall stress of the shaft is high, and the minimum horizontal principal stress exceeds 140 MPa. From the perforation position, four natural fractures are developed at 7263-7320 m of the first fracturing section; However, natural fractures are not developed in the 7203-7239.5 m perforated interval of the second fracturing section. (B) is the fracturing construction curve of 7263-7320 m formation, (C) is the fracturing construction curve of 7203-7239.5 m formation.
Fracture Connectivity
Fracture connectivity refers to the connectivity and dredging ability of wellbore fractures and natural fracture networks around wells. The development of natural fractures in the Keshen ultra-deep reservoir has the characteristics of strong heterogeneity and strong anisotropy. High yield can be obtained only when the borehole meets the natural fracture network or establishes an effective channel between the wellbore fracture and the natural fracture network around the well through hydraulic fracturing. Therefore, developing the connectivity between wellbore fractures and peripheral fractures is also the key factor to measure the effectiveness of fractures.
There are two flow processes in gas well production, that is, gas flows from fracture to wellbore and from matrix to fracture. The degree of fracture development and activity determine the flow of gas from the fracture to the wellbore. Reservoir fracturing has enhanced the flow of fluid from the matrix to the fracture to a certain extent through engineering means. However, the scale and communication ability of wellbore periwellbore matrix fracture systems are prerequisites for determining the high and stable production of gas wells.
A geomechanical prediction method of near wellbore fractures with structural sedimentary constraints is established according to the characteristics of the reservoir over depth and overpressure, strong transverse stress and unavailable seismic data in the Keshen gas field (Figure 10). First, the fine sedimentary microfacies model is used to optimize the near wellbore rock mechanics parameter model, and the correlation between thickness and width is established by using the quantitative statistical microfacies geometric parameters. Combined with the distribution direction of sedimentary microfacies, a near wellbore sedimentary microfacies model is established. Second, the fracture parameters are improved according to the fracture development mode, and a more reasonable fracture density model formula is established. The principal stress difference/compressive strength in the block is compared with the fractured wells in different fault blocks, and the appropriate empirical values in different blocks are summarized. Finally, combined with the correlation between fracture opening and length, a reasonable near wellbore natural fracture model is established. Using the near wellbore fine sedimentary microfacies model to restrict the geomechanical modeling of fractures, the three-dimensional spatial prediction accuracy of near wellbore fractures is improved, and the connectivity evaluation method between wellbore fractures and periwellbore fractures is established.
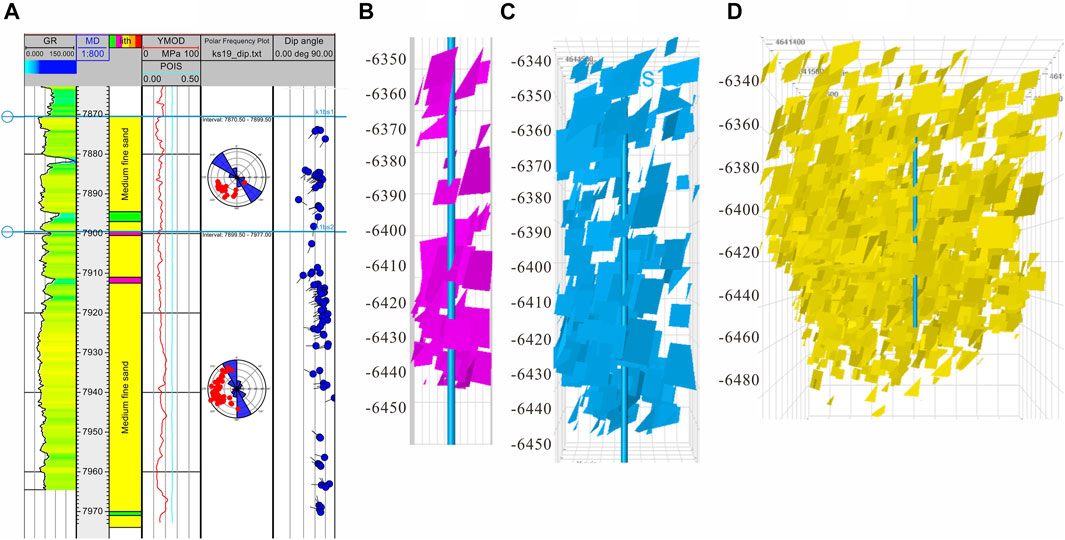
FIGURE 10. The diagram of well ks19 fracture connectivity prediction. (A) is the natural fracture development profile at the wellbore position of ks19, (B) is the prediction diagram of natural fracture development 50 m around the wellbore; (C) is the prediction diagram of natural fracture development 100 m around the wellbore; (D) It is the prediction diagram of natural fracture development 150 m around the wellbore.
Countermeasures and Practice
Drilling or constructing an effective fracture system is the key factor for gas wells in the Keshen gas field to achieve high production. The fracture effectiveness evaluation method obtained based on the research makes up for the traditional effectiveness evaluation method based on static parameters such as fracture density and fracture opening, and in well location deployment, well trajectory optimization, completion and reservoir testing section optimization, perforation interval optimization and even development scheme optimization are widely used. Five principles are comprehensively considered in the optimization of high-efficiency well points: ① relatively developed fractures; ② the area where the minimum principal stress is relatively low; ③ far away from the large fracture that may connect the edge and bottom water; ④ the area where the angle distribution between the fracture strike and the direction of horizontal maximum principal stress is relatively small; and ⑤ the wells are arranged in relatively high parts to enhance the water avoidance ability. Figure 11 is the prediction diagram of the natural fracture effectiveness of the ks 19–21 structure. Red indicates high natural fracture effectiveness, and blue indicates low natural fracture effectiveness. According to the distribution law of natural fracture effectiveness, the deployment of the ks 20 and ks 31 wells is optimized.
Conclusion
The fracture effectiveness evaluation method based on fracture activity, fracture opening pressure and fracture connectivity effectively complements the traditional methods based on static parameters such as fracture density and fracture opening, improves the accuracy of natural fracture effectiveness evaluation, and is applied in well location deployment and well trajectory optimization. It is widely used in the optimization of well completion reconstruction schemes and development schemes.
① The production performance data reveal that only the stratum with low stress, natural fracture development and small azimuth angle between the natural fracture and maximum principal stress is the main supply channel of underground oil and gas.
② The fracture effectiveness evaluation method based on dynamic parameters such as fracture activity, fracture opening pressure and fracture connectivity is more consistent with the production practice results.
③ The well location, well trajectory optimization and reconstruction scheme optimization established based on fracture effectiveness evaluation improve the proportion of high-efficiency wells.
Data Availability Statement
The raw data supporting the conclusion of this article will be made available by the authors, without undue reservation.
Author Contributions
ZW, conception and design of the study, first draft; KX, statistical analysis, manuscript revision; HZ, approved the submitted version; HW, organized the database. GY, organized the database. ZW, organized the database. WZ, organized the database.
Funding
This study is funded by the Major National Science and Technology Project (2016ZX05051), Major Science and Technology Project of PetroChina Company Limited (2018E-1803).
Conflict of Interest
Authors ZW, KX, HZ, HW, GY, ZW, and WZ were employed by PetroChina Tarim Oilfield Company.
Publisher’s Note
All claims expressed in this article are solely those of the authors and do not necessarily represent those of their affiliated organizations, or those of the publisher, the editors and the reviewers. Any product that may be evaluated in this article, or claim that may be made by its manufacturer, is not guaranteed or endorsed by the publisher.
References
Espejel, R. L., Alves, T. M., and Blenkinsop, T. G. (2020). Multi-scale Fracture Network Characterisation on Carbonate Platforms. J. Struct. Geol. 140, 104160. doi:10.1016/j.jsg.2020.104160
Gong, L., Su, X., Gao, S., Fu, X., Jabbari, H., Wang, X., et al. (2019). Characteristics and Formation Mechanism of Natural Fractures in the Tight Gas Sandstones of Jiulongshan Gas Field, China. J. Pet. Sci. Eng. 175, 1112–1121. doi:10.1016/j.petrol.2019.01.021
Han, D., Li, Z., and Han, Y. (2009). Sealing Feature of Burial Diagenesis Environment and its Controls on Differentiation of Cementation in Cretaceous sandstone Reservoir in Kelasu Structure Zone, Kuqa Depression. Acta Petrol. Sin. 25 (10), 2351–2362.
He, D., Ma, Y., and Liu, B. (2019). Main Progress and Scientific Problems of Deep Exploration in Petroliferous Basins in China. Geosci. Frontier 26 (01), 1–12. doi:10.13745/j.esf.sf.2019.1.20
Jia, C., Zhang, Y., and Zhao, X. (2014). Prospects of and Challenges to Natural Gas Industry Development in China. Nat. Gas Industry 34 (2), 1–11. doi:10.3787/j.issn.1000-0976.2014.02.001
Ju, W., Niu, X., and Feng, S. (2020). Evaluation of Current In-Situ Stress Field and Fracture Effectiveness of Shale Oil Reservoir -- a Case Study of Chang 7 Oil Formation in Yanchang, Ordos Basin. J. China Univ. Mining Technol. 49 (05), 931–940. doi:10.13247/j.cnki.jcumt.001138
Lan, S. R., Song, D. Z., Li, Z. L., and Liu, Y. (2021). Experimental Study on Acoustic Emission Characteristics of Fault Slip Process Based on Damage Factor. J. Mining Strata Control. Eng. 3 (3), 033024. doi:10.13532/j.jmsce.cn10-1638/td.20210510.002
Li, H., Lin, C., and Ren, L. (2020). Study on Structural Fracture Prediction Based on Coupling Constraints of Lithofacies and Fault Fracture Zone -- Taking the Middle Member of Sha3 in Daluhu Oilfield in Boxing Depression as an Example. J. China Univ. Mining Technol. 49 (02), 305–317.
Li, Y., Qi, J., and Shi, J. (2017). Characteristics and Genesis of Mesozoic basin in Kuqa Depression. Tarim Basin Geotectonics Metall. 41 (05), 829–842. doi:10.16539/j.ddgzyckx.2017.05.003
Li, Y., Xue, Z., Cheng, Z., Jiang, H., and Ruyue, W. (2020). Progress and Development Direction of Deep Oil and Gas Exploration and Development in China. China Pet. Explor. 25 (01), 45–57. doi:10.3969/j.issn.1672-7703.2020.01.005
Liu, C., Zhang, R., and Zhang, H. (2017). Origin and Reservoir Significance of Multi-Scale Fractures in Kuqa Foreland Thrust belt. Pet. Explor. Dev. 44 (03), 463–472. doi:10.11698/PED.2017.03.17
Liu, J., Ding, W., and Xiao, Z. (2019). Research Progress on Comprehensive Characterization and Prediction of Reservoir Fractures. Adv. Geophys. 34 (06), 2283–2300. doi:10.6038/pg2019CC0290
Liu, J., Ding, W., Wang, R., Yin, S., Yang, H., and Gu, Y. (2017). Simulation of Paleotectonic Stress fields and Quantitative Prediction of Multi-Period Fractures in Shale Reservoirs: A Case Study of the Niutitang Formation in the Lower Cambrian in the Cen'gong Block, South China. Mar. Pet. Geol. 84, 289–310. doi:10.1016/j.marpetgeo.2017.04.004
Lv, W., Zeng, L., and Chen, S. (2021). Multi Scale Natural Fracture Characterization Method for Tight Low Permeability sandstone Reservoir. Geol. Rev. 67 (02), 543–556. doi:10.16509/j.georeview.2021.02.020
Mao, Z., Zeng, L., and Liu, G. (2020). Fractures and Their Effectiveness of Jurassic Deep Tight sandstone Reservoir in the Southern Margin of Junggar Basin. Oil Gas 41 (06), 1212–1221. doi:10.11743/ogg20200609
Neng, Y., Xie, H., and Sun, T. (2013). Structural Characteristics of Kelasu Structural belt and its Petroleum Geological Significance. China Pet. Explor. 18 (2), 1–6. doi:10.3969/j.issn.1672-7703.2013.02.001
Pan, R., Zhu, X., and Liu, F. (2013). Sedimentary Characteristics of Braided delta and Relationship to Reservoirs in the Cretaceous of Kelasu Tchtonic Zone in Kuqa Depression. Xinjiang J. Palaeogeogr. 15 (5), 707–716. doi:10.7605/gdlxb.2013.05.058
Pan, R., Zhu, X-m., and Liu, F. (2014). Jian-fengCretaceous Diagenesis and its Control on Reservoir in Kelasu Structure Zone, Kuqa Depression. Acta Sedimentol. Sinica 32 (5), 973–980.
Shi, C., Wang, Z., and Zhu, W. (2020). Fracture Characteristics of Ultra Deep Reservoir and its Control on Reservoir in Dabei Area of Kelasu Structural belt, Kuqa Depression, Tarim Basin. Nat. Gas Geosci. 31 (12), 1687–1699. doi:10.11764/j.issn.1672-1926.2020.02.001
Shi, J., Zeng, L., and Tan, Q. (2020). Effectiveness of Natural Fractures in Coal Reservoirs in the South of Qinshui Basin and its Impact on Coalbed Methane Development. Oil Gas Geology. 41 (03), 617–626. doi:10.11743/ogg20200317
Tang, Y., Zhou, P., and Xu, Z. (2017). Influence of Stress Environment on Sub Salt Reservoir in Kelasu Structural belt. J. Geology. Colleges Univ. 23 (01), 95–103.
Tian, J., Yang, H., and Wu, C. (2020). The Discovery of Bozi 9 Well and the Exploration Potential of Ultra Deep Natural Gas in Tarim Basin. Nat. gas industry 40 (01), 11–19. doi:10.3787/j.issn.1000-0976.2020.01.002
Tian, J., Wang, Q., and Yang, H. (2021). History and Enlightenment of Oil and Gas Exploration in Tarim Basin. Xinjiang Pet. Geol. 42 (03), 272–282. doi:10.7657/X.1PC20210303
Wang, J., and Wang, X. L. (2021). Seepage Characteristic and Fracture Development of Protected Seam Caused by Mining Protecting Strata. J. Mining Strata Control. Eng. 3 (3), 033511. doi:10.13532/j.jmsce.cn10-1638/td.20201215.001
Wang, K., Yang, H., and Zhang, H. (2018). Structural Fracture Characteristics and Effectiveness of Ultra Deep Tight sandstone Reservoir. Oil Gas Geol. 04 (09), 719–728. doi:10.11743/ogg20180409
Wang, Ke., Zhang, R., and Wang, J. (2021). Distribution Characteristics and Genesis of Structural Fractures in Ultra Deep Tight sandstone Reservoir -- Taking Keshen Gas Field in Kuqa Foreland Thrust belt in Tarim Basin as an Example. Pet. Nat. gas 42 (02), 338–353. doi:10.11743/ogg20210207
Xu, K., Tian, J., and Yang, H. (2020). Prediction and Application of Current In-Situ Stress Field of Deep Tight sandstone Reservoir -- Taking Keshen 10 Gas Reservoir in Kelasu Structural belt of Tarim Basin as an Example. J. China Univ. Mining Technol. 49 (04), 708–720. doi:10.13247/j.cnki.jcumt.001134
Yang, X., Mao, Y., and Zhong, D. (2016). Control of Structural Compression on the Vertical Distribution Difference of sandstone Reservoirs -- Taking the Cretaceous Bashjiqike Formation in Kuqa Foreland Thrust belt as an Example. Nat. Gas Geosci. 27 (04), 591–599. doi:10.11762/j.issn.1672-1926.2016.02.0591
Yang, J. X., Luo, M. K., Zhang, X. W., Huang, N., and Hou, S. J. (2021). Mechanical Properties and Fatigue Damage Evolution of Granite under Cyclic Loading and Unloading Conditions. J. Mining Strata Control. Eng. 3 (3), 033016. doi:10.13532/j.jmsce.cn10-1638/td.20210510.001
Zhang, R., Wang, J., and Ma, Y. (2015). Deep Sedimentary Microfacies Paleogeomorphology and its Control on Natural Gas Enrichment in Kuqa Depression. Tarim Basin Nat. Gas Geosci. 26 (04), 667–678. doi:10.11762/j.issn.1672-1926.2015.02.0667
Zhang, H., Yin, G., and Haiying, W. (2019). Influence of Geomechanical Response of Natural Fractures on Gas Well Productivity in Kuqa Depression. Tarim Basin Nat. Gas Geosci. 30 (03), 379–388. doi:10.11762/j.issn.1672-1926.2018.10.020
Zhang, H., Ju, W., Yin, G., Liu, X., Wang, Z., and Liu, S. (2020). Natural Fracture Prediction in Keshen 2 Ultra-deep Tight Gas Reservoir Based on R/S Analysis, Kuqa Depression, Tarim Basin. Geosci. J. 25 (4), 525–536. doi:10.1007/s12303-020-0041-8
Zhou, P., Tang, Y., and Yin, H. (2016). Etc Development Characteristics of Reservoir Fracture Zone and its Relationship with Production of Keshen 2 Gas Reservoir in Kelasu Structural belt, Tarim Basin. Nat. Gas Geosci. 28 (01), 135–145. doi:10.11762/j.issn.1672-1926.2016.11.028
Zhou, P., Yin, H., and Zhou, L. (2018). Main Controlling Factors and Prediction Methods of Reservoirs in Fault Anticline Strain Neutral Extensional Section -- Taking Kelasu Thrust belt as an Example. Geotectonics Metall. 42 (01), 50–59. doi:10.16539/j.ddgzyckx.2018.01.004
Keywords: ultra-deep layer, fracture effectiveness, reservoir, capacity of production, Tarim Basin
Citation: Wang Z, Xu K, Zhang H, Wang H, Yin G, Wang Z and Zhao W (2022) Fracture Effectiveness Evaluation of Ultra-Deep Tight Sandstone Reservoirs: A Case Study of the Keshen Gas Field, Tarim Basin, West China. Front. Earth Sci. 10:883479. doi: 10.3389/feart.2022.883479
Received: 25 February 2022; Accepted: 15 March 2022;
Published: 12 April 2022.
Edited by:
Wei Ju, China University of Mining and Technology, ChinaReviewed by:
Jingshou Liu, China University of Geosciences Wuhan, ChinaWenlong Ding, China University of Geosciences, China
Copyright © 2022 Wang, Xu, Zhang, Wang, Yin, Wang and Zhao. This is an open-access article distributed under the terms of the Creative Commons Attribution License (CC BY). The use, distribution or reproduction in other forums is permitted, provided the original author(s) and the copyright owner(s) are credited and that the original publication in this journal is cited, in accordance with accepted academic practice. No use, distribution or reproduction is permitted which does not comply with these terms.
*Correspondence: Zhimin Wang, NzE3MjY1OTcxQHFxLmNvbQ==