- 1PetroChina Research Institute of Petroleum Exploration and Development, Beijing, China
- 2PetroChina Tarim Oilfield Company, Xinjiang, China
The Bozi 3 gas reservoir in the Tarim Basin is a typical ultra-deep fractured tight sandstone gas reservoir, in which the main formations are the Cretaceous Bashijiqike Formation and the Baxigai Formation. The reservoir has the characteristics of deep burial, tight reservoir matrix, well-developed fractures, large differences in well productivity, and regional pressure distribution. These characteristics bring development challenges in well deployment, production allocation, gas production rate optimization, and production improvement. The main reservoir of Bozi 3 is deposited in the braided river delta, fan delta, and shallow lake environment with adequate provenance and a strong hydrodynamic environment, which provides favorable depositional conditions for the formation of thick sandbodies. Although the buried depth of the Bozi 3 gas reservoir is over 6,000 m, it has undergone an evolutionary process of long-term shallow burial in the early stage and rapid deep burial in the late stage, which protects the primary pore, and the finally formed reservoir has the characteristics of an extra-low pore and ultra-low permeability pore structure. Late tectonic movements caused the Bozi 3 gas reservoir to experience strong uplift under a strong nappe-thrust environment, forming a large number of high-angle fractures around the faults. Although the fracture distribution is inhomogeneous, tensile fractures at the top anticline have a low-filling degree and good effectiveness, which effectively improve Bozi 3 reservoir properties. Through analysis of the reservoir characteristics and influencing factors of the Bozi 3 gas reservoir, it is concluded that the difference in reservoir characteristics is the main reason for the large difference in production of adjacent wells and zonal distribution of formation pressure at the early development. This study can also provide a reliable geological basis for well deployment, production allocation rationalization, and gas production rate optimization in the study area.
Introduction
A fractured tight sandstone gas reservoir is one of the important reservoir types, with the general reservoir characteristics of tight reservoir matrix and well-developed fractures (Baytok and Pranter, 2012; Baytok and Pranter, 2013; Zeng et al., 2013; Gong et al., 2019). By summarizing the connotation and geophysical response characteristics of “fault-fracture body,” Liu et al. (2021) proposed a prediction technique of “fault-fracture body” based on the chaotic property and the maximum likelihood property, which achieved good results in predicting fractures of the Xinchang tight sandstone reservoir. Wang et al. (2018) conducted a comprehensive study of the fractured tight sandstone reservoir of the Bashijiqike Formation in Keshen 8 gas reservoir by thin section observation, scanning electron microscopy, laser confocal, and electron probe. Through analyzing the lithological characteristics, pore structure, and fracture distribution pattern, they established a porosity–permeability model and a fracture model in order to identify the high production zone. By statistically analyzing the experimental data of the Jurassic Dibei tight sandstone gas reservoir in the eastern Kuqa foreland basin, Lu et al. (2015) concluded that fractures play a key role in improving the matrix properties of tight reservoirs, and the formation time and development location of fractures control the reservoir accumulation enrichment and high production. Sakhaee-Pour and Bryant (2014) studied laboratory data by establishing a pore structure prediction model (multitype pore space model) for a tight sandstone gas reservoir, and they concluded that artificial fractures, natural fractures, and pore structure are the main factors affecting productivity. Through studying the formation mechanism and preservation mechanism of natural fractures, Olson et al. (2009) believed that it is possible to sustain opening-mode fracture growth with sublithostatic pore pressure without associated or preemptive shear failure. Through studying the genesis and effectiveness of Gulong shale oil, Gong et al. (2021) concluded that the good effectiveness of the structural fracture is one of the main controlling factors for the high production of the Qing-1 Member shale oil reservoir. Bozi 3 gas reservoir in the Tarim Basin is a fractured tight sandstone gas reservoir, which exhibits the development dynamic characteristics such as large differences in productivity between adjacent wells and zonal distribution of formation pressure at the early development. From the reservoir characteristics and fracture development pattern, this article discusses the influence of sedimentation, diagenesis, tectonics, and other factors on the reservoir characteristics. It can also provide a reliable geological basis for well deployment, production allocation rationalization, and gas production rate optimization in the study area.
Geological Setting
Bozi 3 gas reservoir was formed in the Late Himalayan period and is located in the Kelasu structural belt in the Northern Kuqa depression, Tarim Basin (Feng and Zhang, 2013). Under the strong compressive stress of the Tianshan Mountains, the Kelasu structural belt developed a series of fault systems and fault–fold-related rows of structural traps (Wei et al., 2020). The tectonic pattern is mainly the thrust imbricate structure in which fault blocks, fault anticlines, and burst structures are superimposed on each other (Neng et al., 2013; Zeng et al., 2010). The tectonic belt is generally distributed in the NEE and EW directions, with a length of about 20 km in the north–south direction and about 160 km in the east–west direction (Gao et al., 2018; Ma et al., 2013). The Kelasu structural belt is divided into Keshen section, Dabei section, Bozi section, and Awat section from east to west, of which the Bozi 3 gas reservoir is located in the third row of structures within the Bozi section (Figure 1) (Jiang et al., 2021).
In 2017, Well Bozi 3 produced 335,000 cubic meters of gas per day (6 mm nozzle) after acid fracturing stimulation, with an oil pressure of 81.8 MPa and an absolute open flow potential of 975,000 cubic meters per day. The oil test of Well Bozi 3 marked the successful discovery of the Bozi 3 condensate gas reservoir. In October 2019, Bozi 3 gas reservoir stepped into the initial development stage, with both the exploration evaluation wells and development wells successfully obtaining industrial gas flow. There are six production wells in the whole gas reservoir, four of which are currently open. Cumulative gas production is 2.44 billion cubic meters per day with the cumulative oil production of 379,000 tons, and the geological reserve recovery is 10.6%.
Data and Methods
The data used in this article include core data, experimental data, and logging data, which were provided by PetroChina Tarim Oil Field Company. As the most important and reliable data of subsurface reservoirs, cores are widely used in exploration, development, and production of any hydrocarbon field (Tavakoli, 2018; Mirzaei-Paiaman et al., 2020). Cores can be observed directly to obtain the lithology, sedimentary structure, and fracture parameters. There are three coring wells in Bozi 3 gas reservoir, with nine tubes of 48.44 m.
The cores are sent to the laboratory to perform further analysis after coring, which includes macroscopic study and microscopic study. The microscopic study is one of the fundamental geological studies to obtain rock information and petrophysical parameters (Al-Dhafeeri and Nasr-El-Din, 2007; Saxena et al., 2021a; Saxena et al., 2021b). In this study, the microscopic study includes 218 samples for helium porosity and permeability analysis, 10 samples for overburden pressure and NMR analysis, and 171 samples for thin-section and X-ray diffraction analysis. The experimental results show rock type, porosity, permeability, pore type, pore structure, filling minerals, and so on.
Well logging is the most important formation evaluation method, which is used to measure the formation properties with electrically powered instruments (Kobr et al., 2005; Ellis and Singer, 2007). There are six wells logged by ECLIPS-5700 and MAXIS-500, which include gamma-ray log, spontaneous potential log, resistivity log, neutron log, density log, array acoustic log, formation lithology log, and imaging log. Log interpretation results can obtain the formation properties including lithology, porosity, permeability, and oil saturation. An imaging log is used to identify borehole and formation data, including lithology, sedimentary structures, natural fractures, and faults. There are five imaging logging wells in Bozi 3 gas reservoir, and fracture parameters of azimuth, dip angle, aperture, and density are obtained after interpretation.
Results
Under the Cretaceous braided river delta and fan delta depositional environment, clastic particles from the Tianshan Mountains were deposited rapidly after the mountain pass. Vertically, multi-phase channel sandbodies are superimposed on each other, and multiple fan bodies are connected with each other on the plane, forming the main Bashijiqike Formation and Baxigai Formation with a thickness of 115–136 m. The lithology of Bozi 3 gas reservoir is mainly medium and fine sandstone, interbedded with thin-layer mudstone and silty mudstone, and mud-graveled sandstone deposited locally. Parallel bedding, cross bedding, and erosion surfaces can be observed on the core, which reflects a relatively strong hydrodynamic environment during the sedimentation (Figure 2). The reservoir is mainly feldspathic lithic sandstone and lithic feldspathic sandstone, and the grain sorting is medium-good. Roundness is sub-edge to sub-circular, and the particles are mainly in point-line contact. The main pores are primary intergranular pores, followed by intergranular dissolved pores and intragranular dissolved pores, and few microfractures and micropores can be seen under the microscope. Diagenetic cementation is strong with mainly calcite cementation. The core test results show that the porosity of the Bashijiqike Formation is 3.5–11% with an average of 6.1%, and the permeability is 0.055–1.272 × 10−3μm2 with an average of 0.237 × 10−3μm2. Bozi 3 belongs to a tight sandstone gas reservoir with an ultra-low porosity and ultra-low permeability.
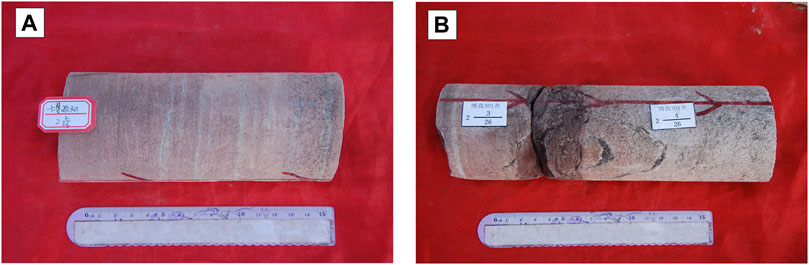
FIGURE 2. Sedimentary structures of Bozi 3 gas reservoir. (A) Well BZ301, Third Bashijiqike Formation, fine sandstone interbedded with silty mudstone, and parallel bedding and cross bedding structure can be seen; (B) Well BZ301, Third Bashijiqike Formation, medium to fine sandstone, and interbedded with mudstone and mud-graveled sandstone can be seen with erosion surface structure on the core.
Under several tectonic movements during the Yanshanian and Himalayan periods, Bozi 3 suffered strong tectonic deformation, and the fractures were relatively developed in the whole area. Core observation and imaging logging are the main measurements for fracture analysis (Laubach and Gale, 2006; Ortega et al., 2006; Hooker et al., 2013). The core observation results show that the fracture density is 2–7 fractures per meter. The fracture dip angle is mainly 45–85°, and the fracture length is 5–150 cm. The fracture aperture is 0.1–1 mm, and the fracture is mainly unfilled (Figure 3). The imaging logging results show that there are 197 fractures in Bozi 3 gas reservoir. The dip angle is in the range of 48.4–90°, and the fractures of 75–90° account for 60.8%, which are mainly high-angle or vertical structural fractures. The fracture strike was mainly in the NS direction, and there were 146 fractures developed in the NS direction, accounting for 74.1% in total. There are 51 fractures in the EW direction, accounting for 25.9% in total. On the plane, the fractures in Well BZ301 are the most developed, with 64 fractures developed and a density of 0.52 fractures per meter. The major principal stress is in the NNW direction, and the angle between the fracture strike and the major principal stress is small (15–40°), and the effectiveness of the fracture is good. Vertically, fractures are most developed in the Third Bashijiqike Formation with the density of 0.22–1.13 fractures per meter, followed by the Second Baxigai Formation with a density of 0.12–0.5 fractures per meter. Fractures are less developed in the Second Baxigai Formation, with a density of 0.12–0.27 fractures per meter (Figure 4).
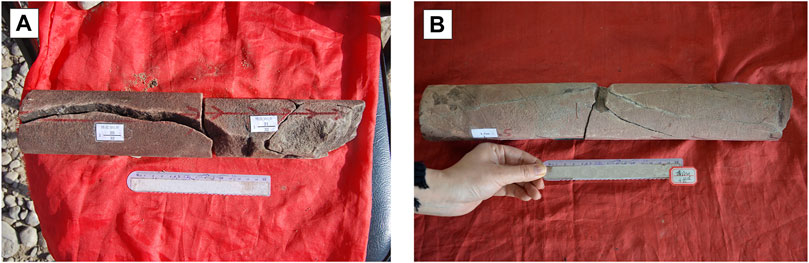
FIGURE 3. Core fractures of Bozi 3 gas reservoir. (A) Well BZ301, high-angle fractures, unfilled; (B) Well BZ301, high-angle fractures, half-filled.
Discussion
Influencing Analysis of Sedimentation on the Reservoir Characteristics
The main formations of Bozi 3 gas reservoir are the Cretaceous Bashijiqike Formation and Baxigai Formation. The hot and dry climate during the Cretaceous Baxigai sedimentation intensified the physical weathering in the parent rock of the basin margin, which produced a large amount of continental margin debris and provided sufficient provenance to the basin (Liu et al., 2019). During this period, the tectonic movement was in a relatively calm period, the terrain was relatively gentle, and the hydrodynamics was relatively weak, which was a lacustrine deltaic or shore-shallow lake sedimentary system, making the provenance of debris particles fine (Jia, 2009). During the sedimentation period of the Third Bashijiqike Formation, the climate was hot and dry, and the tectonic movement was relatively strong. The detrital materials from the Tianshan Mountains were rapidly deposited at the entrance to the lake, and the debris particles were coarse, forming a fan delta sedimentary system (Gu et al., 2001; Lai et al., 2018). Vertically, multi-phase channel sandbodies are superimposed on each other, and multiple fan bodies are connected with each other on the plane (Figure 5) (Zhang et al., 2008; Pan et al., 2013). The Tianshan Mountains have sufficient provenance, strong hydrodynamic environment, and overall gentle lake sedimentation, which provide favorable depositional conditions for the formation of thick Bozi 3 sandbodies. By statistically analyzing the properties of the sandbodies under different sedimentary microfacies, sandbodies of the fan delta front have good reservoir properties. Subaqueous distributary channel reservoir in the fan delta front has the best reservoir properties, with an average matrix porosity of 6.4% and an average matrix permeability of 0.31 × 10−3 μm2, which is the most developed favorable sedimentary microfacies sandbody.
Bozi 3 gas reservoir has stable sedimentation, while the sandbody distribution is inhomogeneous. Reservoir thickness is 114.5–136.3 m, with an average of 125.3 m. Affected by the uplift of the Wensu paleo-uplift in the southwest, the top Bashijiqike Formation suffered strong erosion, and only part of the Third Bashijiqike Formation remains. The reservoir thickness of the Third Bashijiqike Formation is 19.5–24.8 m with an average of 22.2 m, and the sand ratio is 79.1–91.6% with an average of 88.4%. The Baxigai Formation is further divided into First Baxigai Formation and Second Baxigai Formation. The reservoir thickness of the First Baxigai Formation is 52–65 m with an average of 57.3m, and the sand ratio is 19.8–28.2% with an average of 26.2%. The reservoir thickness of the Second Baxigai Formation is 37–51 m with an average of 43.6m, and the sand ratio is 56.2–68.8% with an average of 64.8%. The lithology of Bozi 3 reservoir is mainly medium and fine sandstone, and mudstone interlayers are distributed locally. The sedimentary facies of the Third Bashijiqike Formation are the fan delta front, and the lithology is mainly medium to fine sandstone with the highest sandstone content. The sedimentary facies of the First Baxigai Formation are the braided river delta front, and the lithology is mainly mudstone with mixed siltstone and fine sandstone (Figure 6).
Influencing Analysis of Diagenesis on the Reservoir Characteristics
Reservoir properties are mainly affected by lithology, pore structure, cementation type, and other diagenesis (Laubach and Ward, 2006; Fall et al., 2016; Weisenberger et al., 2019). Early long-term shallow burial and late rapid deep burial are the main mechanisms for the effective preservation of the reservoir pore space, which provides favorable conditions for the better reservoir properties of Bozi 3 gas reservoir (Yang et al., 2021). The whole area experienced long-term shallow burial in the early burial period (before 5 Ma), and the clastic particles have high maturity, which makes the rock have certain compaction resistance and protects the primary pore structure. The whole area experienced rapid deep burial in the late burial period (since 5 Ma), which delayed the compaction of the overlying strata and made the primary pore well preserved even at 6,000 m. The strong compression of late tectonics reduced porosity, but the compression fractures provided the dissolution source, especially the fractures formed during the Late Himalayan tectonics were unfilled, and the connected pores formed an effective oil and gas migration channel, which is one of the key factors for the high production of Bozi 3 gas reservoir.
The rock type of Bozi 3 gas reservoir is mainly medium to fine feldspar lithic sandstone, with medium to good sorting of clastic particles. Roundness is sub-edge to sub-circular, and the particles are mainly in point-line contact. The main pores are primary intergranular pores, accounting for 72% of the total surface porosity, followed by intergranular dissolved pores and intragranular dissolved pores, accounting for 21% of the total surface porosity. Few microfractures and micropores can be seen under the microscope, and the total surface porosity is less than 7% (Figure 7). The diagenetic cementation is serious and mainly calcite cementation, with 15% cement content. Experimental displacement pressure is 1.5–5Mpa, and the average pore roar radius is 0.03–0.07 μm. Low displacement pressure and small pore throat radius show the pore structure characteristics of ultra-low porosity and ultra-low permeability.
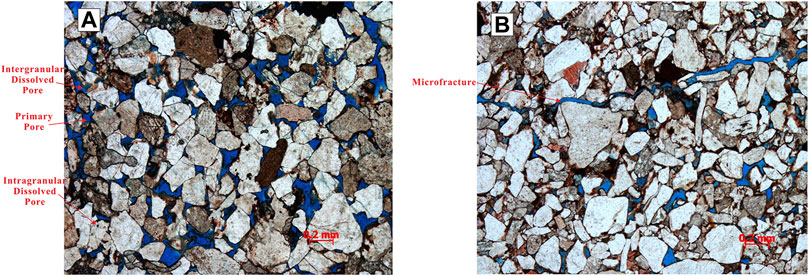
FIGURE 7. Petrological characteristics of Bozi 3 gas reservoir observed under the microscope. (A) Well BZ301, 5,932.85 m, Third Bashijiqike Formation; (B) BZ301, 5,838.82 m, Third Bashijiqike Formation.
From the analysis of core tests, the porosity of the Bashijiqike Formation is 3.5–11% with an average of 6.1%, while the permeability is 0.055–1.272 × 10−3μm2 with an average of 0.237 × 10−3μm2. Logging interpretation porosity of the Third Bashijiqike Formation is 3.5–11% with an average of 6.8%, while the permeability is 0.055–1.97 × 10−3μm2 with an average of 0.275 × 10−3μm2. Logging interpretation porosity of the First Baxigai Formation is 3.5–7.8% with an average of 5.3%, while the permeability is 0.055–0.411 × 10−3μm2 with an average of 0.142 × 10−3μm2. Logging interpretation porosity of the Second Baxigai Formation is 3.5–9% with an average of 5.9%, while the permeability is 0.055–0.472 × 10−3μm2 with an average of 0.170 × 10−3μm2. The comprehensive analysis shows that the reservoir properties of the Third Bashijiqike Formation are the best, followed by the Second Baxigai Formation, and the reservoir properties of the First Baxigai Formation are poor (Figure 8).
Influencing Analysis of Tectonics on Reservoir Characteristics
Affected by the Tianshan tectonic activity, Bozi 3 gas reservoir mainly experienced four tectonic movements: Late Yanshanian, Early Himalayan, Middle Himalayan, and Late Himalayan. Regional tectonic stress gradually changed from tensile stress to compressive stress (Feng et al., 2020). During the late tectonic movement, Bozi 3 gas reservoir experienced strong uplift through the nappe-thrust tectonic. A large number of high-angle fractures were formed near the fault, and the local tensile stress field at the top of the anticline developed a large number of tensile fractures (Lahiri, 2021; Silva et al., 2021; Zheng et al., 2016; Zeng et al., 2004) (Figure 9). These fractures have low-filling degrees and good effectiveness, which provide channels for petroleum migration and effectively improve the reservoir properties of the low permeability (Olson, 2007; Olson et al., 2007; Hooker et al., 2009; Laubach et al., 2009; Olson et al., 2009). Bozi 3 gas reservoir has developed high-angle structural fractures with good effectiveness, providing favorable petroleum migration conditions for improving the reservoir properties.
The vertical stress difference makes Bozi 3 gas reservoir show vertical stratification. Under the compressive stress of the Tianshan Mountains and the lower formation uplifting, the top of the anticline is under tensile stress, and the bottom of the anticline is under compressive stress. In the middle, there developed an undeformed neutral surface (Cornu et al., 2003). Correspondingly, from bottom to top, the reservoir develops a compressive section, transition section, and tensional section (Figure 10). The compressive section is mainly under relatively high compressive stress, with the stress curve locally elevating. The reservoir in this section is relatively tight with the developed shear network fractures, which have the characteristics of small aperture, short length, large density, and weak dissolution. Neutral surface has a transitional stress from compressive stress to tensile stress, with decreasing stress in the stress curve. There develop the late tension fractures and early shear network fractures in this section, with the increasing porosity from bottom to top. The tensional section is mainly controlled by the tensile stress. The reservoir properties are relatively better with tension fractures, which have the characteristics of large aperture, long length, low density, and strong dissolution. Different reservoir properties with different fracture patterns form the strong inhomogeneous reservoir characteristics in Bozi 3 gas reservoir, which is the main reason for the large difference in the production of adjacent wells and zonal distribution of formation pressure at the early development.
Conclusion
Because of good reservoir conditions formed under the sedimentary environment, diagenesis, and tectonics, Bozi 3 gas reservoir still has industrial gas production capacity with a burial depth of more than 6,000 m. Difference in reservoir characteristics from various patterns of fractures and matrix is the main reason for the large difference in production of adjacent wells and zonal distribution of formation pressure at the early development.
The sedimentary environment of the braided river delta and fan delta with the strong hydrodynamic and sufficient provenance provides the sandbody basis for the effective formation with large area distribution. The thickness of Bozi 3 gas reservoir is 125.3 m, and the sand ratio is 59.8%, mainly medium-fine sandstone.
The accumulation mechanism of long-term shallow burial in the early stage and rapid deep burial in the late stage effectively protects the primary pore structure, and the later pressure dissolution improves the reservoir permeability. The porosity of Bozi 3 gas reservoir is 6.1%, and the permeability is 0.196 × 10−3 μm2, belonging to the ultra-low porosity and ultra-low permeability reservoir.
Tectonic movements result in the fracture development in the whole area, especially a large number of tensile fractures formed at the top of the anticline during the Late Himalayan period. These fractures have the characteristics of large aperture, long length, low density, and strong dissolution. Fractures are the main channels for petroleum migration, and they effectively improve the reservoir seepage capability.
Data Availability Statement
The raw data supporting the conclusion of this article will be made available by the authors, without undue reservation.
Author Contributions
All the authors listed have made a substantial, direct, and intellectual contribution to the work and approved it for publication.
Conflict of Interest
All authors were employed by PetroChina.
Publisher’s Note
All claims expressed in this article are solely those of the authors and do not necessarily represent those of their affiliated organizations, or those of the publisher, the editors, and the reviewers. Any product that may be evaluated in this article, or claim that may be made by its manufacturer, is not guaranteed or endorsed by the publisher.
References
Al-Dhafeeri, A. M., and Nasr-El-Din, H. A. (2007). Characteristics of High-Permeability Zones Using Core Analysis, and Production Logging Data. J. Pet. Sci. Eng. 55, 18–36. doi:10.1016/j.petrol.2006.04.019
Baytok, S., and Pranter, M. J. (2013). Fault and Fracture Distribution within a Tight-Gas Sandstone Reservoir: Mesaverde Group, Mamm Creek Field, Piceance Basin, Colorado, USA. Pet. Geosci. 19, 203–222. doi:10.1144/petgeo2011-093
Baytok, S., and Pranter, M. (2012). “Seismic Attribute Analysis of Faults and Fractures within a Tight-Gas sandstone Reservoir: Williams Fork Formation, Mamm Creek Field, Piceance Basin, Colorado,” in International Geophysical Conference and Oil & Gas Exhibition. doi:10.1190/IST092012-001.24
Cornu, T., Schneider, F., and Gratier, J.-P. (2003). 3D Discrete Kinematic Modelling Applied to Extensional and Compressional Tectonics. Geol. Soc. Lond. Spec. Publications 212, 285–294. doi:10.1144/gsl.sp.2003.212.01.19
Fall, A., Ukar, E., and Laubach, S. E. (2016). Origin and Timing of Dauphiné Twins in Quartz Cement in Fractured Sandstones from Diagenetic Environments: Insight from Fluid Inclusions. Tectonophysics 687, 195–209. doi:10.1016/j.tecto.2016.08.014
Feng, J., Sun, J., Zhang, Y., Dai, J., Wei, H., Quan, L., et al. (2020). Control of Fault-Related Folds on Fracture Development in Kuqa Depression, Tarim Basin. Oil Gas Geology. 41, 543–557. doi:10.11743/ogg20200311
Feng, S., and Zhang, Z. (2013). Accumulation Process and Characteristics of Overpressured Large Gas Field in Keshen Belt of Kelasu Tectonic Zone. J. Hefei Univ. Techn. (Natural Science) 36, 1242–1248. doi:10.3969/j.issn.1003-5060.2013.10.019
Gao, W., Li, X., Zhang, G., Wei, Q., Zhang, J., Qi, S., et al. (2018). The Relationship Research between Densification of Reservoir and Accumulation of the Deep Tight Sandstone Gas Reservoirs of the Kelasu Tectonic Zone in Kuqa Depression, Tarim Basin. Nat. Gas Geosci. 29, 226–235. doi:10.11764/j.issn.1672-1926.2017.12.007
Gong, L., Fu, X., Wang, Z., Gao, S., Jabbari, H., Yue, W., et al. (2019). A New Approach for Characterization and Prediction of Natural Fracture Occurrence in Tight Oil Sandstones with Intense Anisotropy. Bulletin 103, 1383–1400. doi:10.1306/12131818054
Gong, L., Wang, J., Gao, S., Fu, X., Liu, B., Miao, F., et al. (2021). Characterization, Controlling Factors and Evolution of Fracture Effectiveness in Shale Oil Reservoirs. J. Pet. Sci. Eng. 203, 108655. doi:10.1016/j.petrol.2021.108655
Gu, J., Fang, H., and Jia, J. (2001). Diagenesis and Reservoir Characteristics of Cretaceous Braided Delta Sandbody in Kuqa Depression, Tarim Basin. Acta Sedimentologica Sinica 19, 517–523. doi:10.3969/j.issn.1000-0550.2001.04.007
Hooker, J. N., Gale, J. F. W., Gomez, L. A., Laubach, S. E., Marrett, R., and Reed, R. M. (2009). Aperture-size Scaling Variations in a Low-Strain Opening-Mode Fracture Set, Cozzette Sandstone, Colorado. J. Struct. Geology. 31, 707–718. doi:10.1016/j.jsg.2009.04.001
Hooker, J. N., Laubach, S. E., and Marrett, R. (2013). Fracture-aperture Size-Frequency, Spatial Distribution, and Growth Processes in Strata-Bounded and Non-strata-bounded Fractures, Cambrian Mesón Group, NW Argentina. J. Struct. Geology. 54, 54–71. doi:10.1016/j.jsg.2013.06.011
Jia, J. (2009). Sedimentary Characteristics and Palaeogeography of the Early Cretaceous in Tarim Basin. J. Palaeogeogr. 11, 167–176. doi:10.7605/gdlxb.2009.02.004
Jiang, T., Zhang, H., Xu, K., Yin, G., Wang, H., Wang, Z., et al. (2021). Technology and Practice of Quantitative Optimization of Borehole Trajectory in Ultra-deep Fractured Reservoir: A Case Study of Bozi A Gas Reservoir in Kelasu Structural Belt, Tarim Basin. China Pet. Exploration 26, 149–161. doi:10.3969/j.issn.1672-7703.2021.04.012
Kobr, M., Mareš, S., and Paillet, F. (2005). “Geophysical Well Logging,” in Hydrogeophysics (Dordrecht: Springer), 291–331.
Lahiri, S. (2021). Estimating Effective Permeability Using Connectivity and branch Length Distribution of Fracture Network. J. Struct. Geology. 146, 104314. doi:10.1016/j.jsg.2021.104314
Lai, J., Han, N., Jia, Y., Ji, Y., Wanf, G., Pang, X., et al. (2018). Detailed Description of the Sedimentary Reservoir of a Braided Delta Based on Well Logs. Geology. China 45, 304–318. doi:10.12029/gc20180207
Laubach, S. E., and Gale, J. F. W. (2006). Obtaining Fracture Information for Low-Permeability (Tight) Gas Sandstones from Sidewall Cores. J. Pet. Geol 29, 147–158. doi:10.1111/j.1747-5457.2006.00147.x
Laubach, S. E., Olson, J. E., and Gross, M. R. (2009). Mechanical and Fracture Stratigraphy. Bulletin 93, 1413–1426. doi:10.1306/07270909094
Laubach, S. E., and Ward, M. E. (2006). Diagenesis in Porosity Evolution of Opening-Mode Fractures, Middle Triassic to Lower Jurassic La Boca Formation, NE Mexico. Tectonophysics 419, 75–97. doi:10.1016/j.tecto.2006.03.020
Liu, Y., Xia, H., Sun, Q., Lin, C., Zhao, H., Li, H., et al. (2019). Sequence Stratigraphy and Depositional Evolution of the Bashijiqike Formation in the Western Tabei Uplift, Tarim Basin. Nat. Gas Geosci. 30, 62–73. doi:10.11764/j.issn.1672-1926.2018.10.003
Liu, Z., Liu, Z., Guo, Y., Ji, Y., Li, W., Lin, T., et al. (2021). Concept and Geological Model of Fault-Fracture Reservoir and Their Application in Seismic Fracture Prediction: A Case Study on the Xu 2 Member Tight Sandstone Gas Pool in Xinchang Area,Western Sichuan Depression in Sichuan Basin. Oil Gas Geology. 42, 973–980. doi:10.11743/ogg20210417
Lu, H., Lu, X., Fan, J., Wang, X., Fu, X., Wei, H., et al. (2015). The Controlling Effects of Fractures on Gas Accumulation and Production in Tight Sandstone: A Case of Jurassic Dibei Gas Reservoir in the East Kuqa Foreland Basin. Nat. Gas Geosci. 26, 1047–1056. doi:10.11764/j.issn.1672-1926.2015.06.1047
Ma, Y., Zhuo, Q., Yang, X., Fang, S., Tang, Y., Guo, X., et al. (2013). Petroleum Dynamic Accumulation Process and its Implications in Kelasu Structural Belt, Kuqa Depression, Tarim Basin. Pet. Geology. Exp. 35, 249–254+268. doi:10.11781/sysydz201303249
Mirzaei-Paiaman, A., Asadolahpour, S. R., Saboorian-Jooybari, H., Chen, Z., and Ostadhassan, M. (2020). A New Framework for Selection of Representative Samples for Special Core Analysis. Pet. Res. 5, 210–226. doi:10.1016/j.ptlrs.2020.06.003
Neng, Y., Xie, H., Sun, T., Lei, G., and Xu, L. (2013). Structural Characteristics of Keshen Segmentation in Kelasu Structural Belt and its Petroleum Geological Significance. China Pet. Exploration 18, 1–6. doi:10.3969/j.issn.1672-7703.2013.02.001
Olson, J. E. (2007). Fracture Aperture, Length and Pattern Geometry Development under Biaxial Loading: a Numerical Study with Applications to Natural, Cross-Jointed Systems. Geol. Soc. Lond. Spec. Publications 289, 123–142. doi:10.1144/sp289.8
Olson, J. E., Laubach, S. E., Lander, R. H., Lonergan, L., Jolly, R. J. H., Rawnsley, K., et al. (2007). Combining Diagenesis and Mechanics to Quantify Fracture Aperture Distributions and Fracture Pattern Permeability. Geol. Soc. Lond. Spec. Publications 270, 101–116. doi:10.1144/GSL.SP.2007.270.01.08
Olson, J. E., Laubach, S. E., and Lander, R. H. (2009). Natural Fracture Characterization in Tight Gas Sandstones: Integrating Mechanics and Diagenesis. Bulletin 93, 1535–1549. doi:10.1306/08110909100
Ortega, O. J., Marrett, R. A., and Laubach, S. E. (2006). A Scale-independent Approach to Fracture Intensity and Average Spacing Measurement. Bulletin 90, 193–208. doi:10.1306/08250505059
Pan, R., Zhu, X., Liu, F., Li, Y., Ma, Y., Di, H., et al. (2013). Sedimentary Characteristics of Braided Delta and Relationship to Reservoirs in the Cretaceous of Kelasu Tectonic Zone in Kuqa Depression, Xinjiang. J. Palaeogeogr. 15, 707–716. doi:10.7605/gdlxb.2013.05.058
Sakhaee-Pour, A., and Bryant, S. L. (2014). Effect of Pore Structure on the Producibility of Tight-Gas Sandstones. Bulletin 98, 663–694. doi:10.1306/08011312078
Saxena, N., Alpak, F. O., Hows, A., Freeman, J., Hofmann, R., and Appel, M. (2021a). Estimating Fluid Saturations from Capillary Pressure and Relative Permeability Simulations Using Digital Rock. Transp Porous Med. 136, 863–878. doi:10.1007/s11242-021-01543-z
Saxena, N., Dietderich, J., Alpak, F. O., Hows, A., Appel, M., Freeman, J., et al. (2021b). Estimating Electrical Cementation and Saturation Exponents Using Digital Rock Physics. J. Pet. Sci. Eng. 198, 108198. doi:10.1016/j.petrol.2020.108198
Silva, J. P., Gomes, I. F., Santos, R. F. V. C., Miranda, T. S. d., Guedes, R. P., Barbosa, J. A., et al. (2021). Topological Analysis of Fracture Networks Integrated with Flow Simulation Models for Equivalent Fracture Permeability Estimation. J. Struct. Geology. 147, 104338. doi:10.1016/j.jsg.2021.104338
Tavakoli, V. (2018). Geological Core Analysis: Application to Reservoir Characterization. Cham: Springer.
Wang, K., Zhang, R., Fang, X., Wang, J., and Zhang, T. (2018). Characteristics and Property Modeling of Ultra-deep Fractured-Porous Tight Sandstone Reservoir: A Case Study on the Keshen 8 Gas Reservoir in Kuqa Depression. China Pet. Exploration 23, 87–96. doi:10.3969/j.issn.1672-7703.2018.06.010
Wei, G., Wang, J., Zeng, L., Tang, Y., Wang, K., Liu, T., et al. (2020). Structural Reworking Effects and New Exploration Discoveries of Subsalt Ultra-deep Reservoirs in the Kelasu Tectonic Zone. Nat. Gas Industry 40, 20–30. doi:10.3787/j.issn.1000-0976.2020.01.003
Weisenberger, T. B., Eichhubl, P., Laubach, S. E., and Fall, A. (2019). Degradation of Fracture Porosity in Sandstone by Carbonate Cement, Piceance Basin, Colorado, USA. Pet. Geosci. 25, 354–370. doi:10.1144/petgeo2018-162
Yang, X., Tian, J., Wang, Q., Li, Y., Yang, H., Li, Y., et al. (2021). Geological Understanding and Favorable Exploration Fields of Ultra-deep Formations in Tarim Basin. China Pet. Exploration 26, 17–28. doi:10.3969/j.issn.1672-7703.2021.04.002
Zeng, L., Su, H., Tang, X., Peng, Y., and Gong, L. (2013). Fractured Tight Sandstone Oil and Gas Reservoirs: A New Play Type in the Dongpu Depression, Bohai Bay Basin, China. Bulletin 97, 363–377. doi:10.1306/09121212057
Zeng, L., Tan, C., and Zhang, M. (2004). Mesozoic-Cenozoic Tectonic Stress Field and its Hydrocarbon Migration and Accumulation Effect in Kuqa Depression, Tarim Basin. Sci. China Ser. D: Earth Sci. 34, 98–106. doi:10.3969/j.issn.1674-7240.2004.z1.011
Zeng, L., Wang, H., Gong, L., and Liu, B. (2010). Impacts of the Tectonic Stress Field on Natural Gas Migration and Accumulation: A Case Study of the Kuqa Depression in the Tarim Basin, China. Mar. Pet. Geology. 27, 1616–1627. doi:10.1016/j.marpetgeo.2010.04.010
Zhang, R., Zhang, H., Shou, J., Shen, Y., and Li, C. (2008). Geological Analysis on Reservoir Mechanism of the Lower Cretaceous Bashijiqike Formation in Dabei Area of the Kuqa Depression. Chin. J. Geology 43, 507–517. doi:10.3321/j.issn:0563-5020.2008.03.006
Keywords: tight sandstone gas reservoir, fracture, deep formation, reservoir characteristics, Bozi 3 gas reservoir in the Tarim basin
Citation: Liu Z, Zhang Y, Zhang Y, Zhao L, Peng P, Liu H, Chang B, Huang W and Zhou P (2022) Influencing Factor Analysis on the Fractured Tight Sandstone Gas Reservoir Characteristics: A Case Study of Bozi 3 Gas Reservoir in the Tarim Basin. Front. Earth Sci. 10:881934. doi: 10.3389/feart.2022.881934
Received: 23 February 2022; Accepted: 21 March 2022;
Published: 14 April 2022.
Edited by:
Lei Gong, Northeast Petroleum University, ChinaReviewed by:
Jianwei Feng, China University of Petroleum, ChinaZhenwei Guo, Central South University, China
Copyright © 2022 Liu, Zhang, Zhang, Zhao, Peng, Liu, Chang, Huang and Zhou. This is an open-access article distributed under the terms of the Creative Commons Attribution License (CC BY). The use, distribution or reproduction in other forums is permitted, provided the original author(s) and the copyright owner(s) are credited and that the original publication in this journal is cited, in accordance with accepted academic practice. No use, distribution or reproduction is permitted which does not comply with these terms.
*Correspondence: Zhaolong Liu, bGl1emhhb2w2OUBwZXRyb2NoaW5hLmNvbS5jbg==