- 1Department of Earth and Atmospheric Sciences, University of Alberta, Edmonton, AB, Canada
- 2Grupo de Investigación en Ciencias de la Tierra y Energía, Bucaramanga, Colombia
- 3Vicepresidencia de Exploración, Bogotá, Colombia
- 4Centro de Innovación y Tecnología, Instituto Colombiano del Petróleo, Piedecuesta, Colombia
- 5Department of Structural Geology and Geodynamics, Georg-August-Universität Göttingen, Göttingen, Germany
The present-day Magdalena Valley, Eastern Cordillera, and Llanos Basin were part of a regional multiphase basin that started as an extensional basin in the Jurassic. Then, it was transformed into a retroarc foreland basin in the Late Cretaceous and subsequently separated into a hinterland and a foreland basin during the late Eocene. We incorporated new data from the Llanos Basin and Eastern Cordillera and chronostratigraphically correlated it with the adjacent basins. Their correlation contributed to establishing the stratigraphic boundaries formed during the first-order changes that marked the beginning and the end of the lifespan of the retroarc foreland basin. In the initial stage, the lateral extent along the dip of the significant depocenter that extended from the Magdalena Valley to the westernmost Llanos Basin was approximately 300–470 km. At the same time, the western flank of the Eastern Cordillera and most of the Llanos Basin were uplifting. The contact between the retrograding marine facies of the Upper Lidita, Buscavidas, Umir, Guadalupe, and Palmichal units marks a late Campanian maximum regressive surface formed during the initial exhumation of the orogen, the Central Cordillera. Therefore, it represents a first-order stratigraphic surface. This surface does not extend across the whole basin. In the uplifting areas, subaerial unconformities truncate the middle Campanian rocks. Their combination represents the lower first-order boundary of the sequence, and their diachroneity is the product of the northward migration of the orogen. The Teruel, Hoyon, Bogota, Cacho, Socha, Barco, and Cuervos formations are truncated by a regional subaerial unconformity. It was formed during orogenic unloading that marks the termination of the foreland basin in the Eocene. As it marks another first-order change, it is the upper boundary of the first-order sequence.
Introduction
Regionally, Colombia is in an active tectonic margin due to the interaction of the South American, Caribbean, and Nazca plates (Colleta et al., 1990; Dengo and Covey, 1993; Cooper et al., 1995a). This interplay caused the uplift of the three ranges (Western, Eastern, and Central) and also the separation of a Late Cretaceous–Paleogene regional basin into multiple basins (Gómez et al., 2003; Gómez et al., 2005a; Horton et al., 2010a; Parra et al., 2010; Saylor et al., 2012). Those are two of the main sedimentary basins in Colombia as they extend for hundreds of kilometers from south to north (Figure 1). The regional basin started as an extensional basin during the Jurassic; it was under a continental rift during the earliest Cretaceous, became a retroarc basin by the Late Cretaceous, and was separated by the uplift of the Eastern Cordillera during the Paleogene (Pindell and Erikson, 1994; Sarmiento, 2001; Horton et al., 2010b; Nie et al., 2010; Cediel et al., 2011; Bayona et al., 2013, 2020; Zapata et al., 2019). As a result, there are 140 My of sediment that preserves a complex structural evolution.
Colleta et al. (1990), Cooper et al. (1995a), Dengo and Covey (1993), and Villamill (1999) promoted regional geological models demonstrating how the tectonic plates interacted throughout time and how they produced the exhumation of the Colombian Cordilleras. Cortés (2006), Horton et al. (2010a), Mora et al. (2006), Mora et al. (2008), Mora et al. (2010a), Parra et al. (2010), and Sarmiento (2001) calibrated the timing of uplift of the Eastern Cordillera and presented some analysis of the sedimentology and basin-fill history during the Cretaceous and part of the Cenozoic. More recently, these works have been summarized and complemented in a series of paleogeographic maps and geological models presented by Bayona (2018), Bayona et al. (2020), Cardona et al. (2018), Cediel et al. (2011), Martínez and Roncancio (2011), Montes et al. (2019), Reyes-Harker et al. (2015), and Sarmiento (2011).
Regarding the Late Cretaceous to Paleogene geological model, Gómez et al. (2005b) and Parra et al. (2010) stated that the foredeep of the regional foreland basin extended from the Magdalena Valley in the west to the Llanos Foothills in the east, with most of the Llanos Basin being part of the forebulge. However, Bayona et al. (2003), Caballero (2010), and Terraza-Melo et al. (2002) indicated a gap in the sedimentary record, at least between the late Cretaceous to early-middle Paleocene. The data demonstrate the exhumation and uplifting of the western flank of the Eastern Cordillera from the latest Cretaceous to Paleogene and raise the question of how it was produced? Is that related to the forebulge located at the western flank of the Eastern Cordillera instead of the western Llanos Basin?
This study presents chronostratigraphic correlations using previous data from 14 locations across the Middle-Upper Magdalena Valley, Eastern Cordillera, and western Llanos Basin, including four new stratigraphic sections from the Llanos Foothills and Basin (Figure 1 and Table 1). The age of the strata in the new sections was established following the U-Pb ages of detrital zircons from Carvajal-Torres et al. (2019) and paleontological reports from the area. The correlations presented in Figure 1 were built following the methodology from Qayyum et al. (2017) and Wheeler (1959), along with results and analysis exposed by Carvajal-Torres et al. (2021). The interpretation of sedimentary environments was simplified based on the principles presented by Boggs Jr (2006), Nichols (2009), and Perry and Taylor (2007). We correlated the depositional environments about their position from the shoreline, regardless of the type of sedimentary shore (Figure 2B).
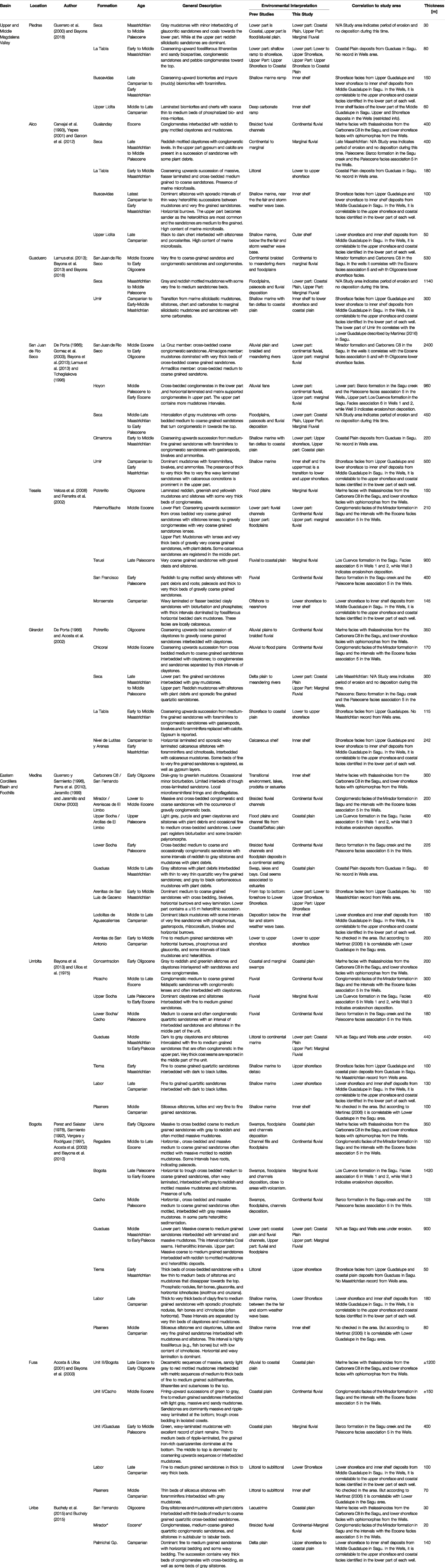
TABLE 1. Lithostratigraphic and depositional environments summary for each location shown in Figure 1.
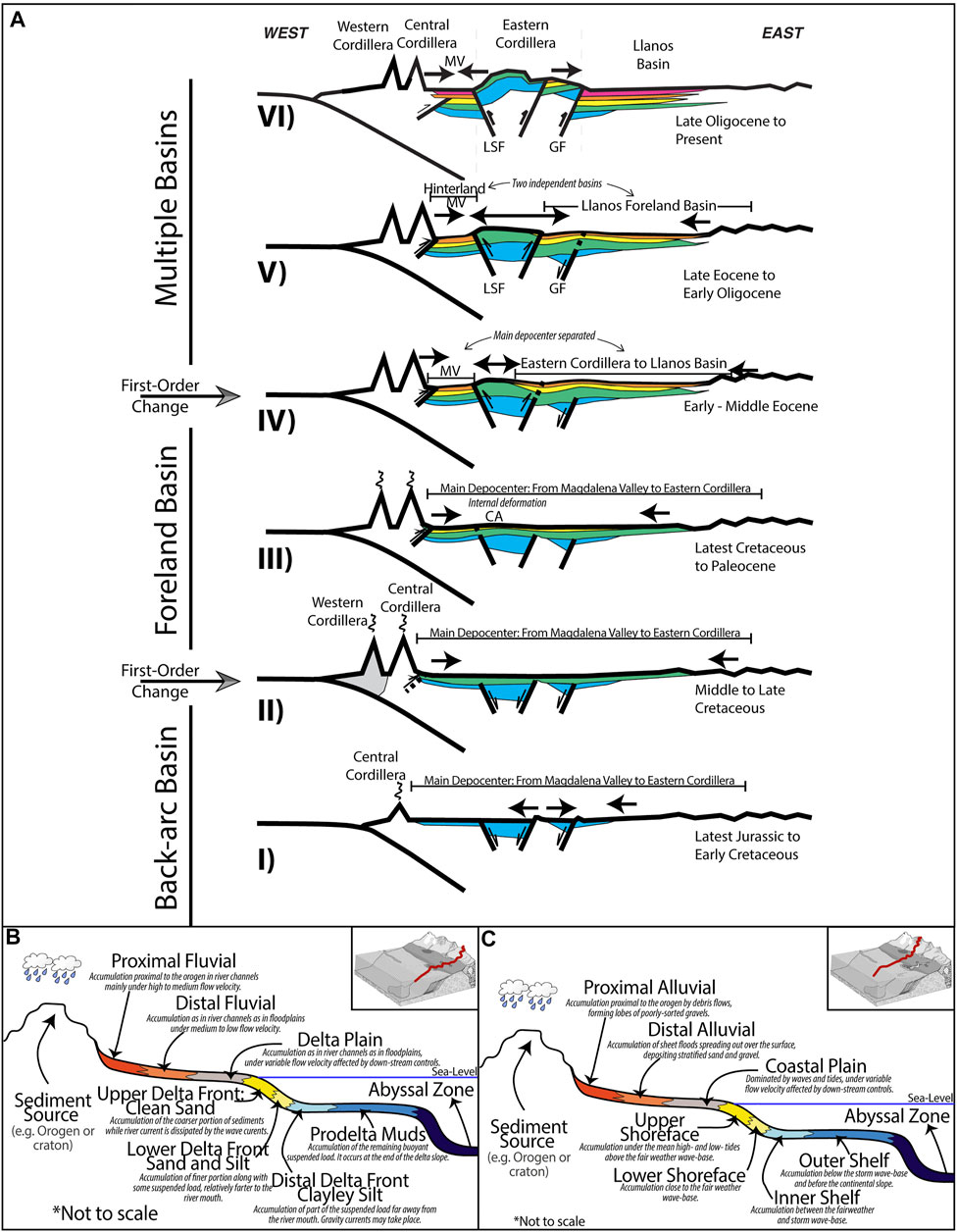
FIGURE 2. (A) Conceptual model of the tectonic evolution of Central Colombia modified from Horton B. K. et al. (2010). (B) and (C) Conceptual sedimentological model after Boggs Jr (2006), Catuneanu (2006), and Nichols (1999).
The delimitation of the forebulge was supported using paleogeographic palinspatically restored maps according to Bayona (2018), Cardona et al. (2018), and Mann et al. (2006). Its definition is relevant to constraining the geological model of the regional foreland basin. Likewise, understanding the forebulge unconformity is key to understanding how the tectonism controlled the accommodation and sedimentation throughout time. Those concepts can be applied to other analogous basins. Our results recognize the changes in the accommodation and sedimentation due to the first-order changes. Following Catuneanu (2019a, 2020) and Catuneanu and Zecchin (2017), we identified different stratigraphic surfaces. Two of them formed during the latest Campanian to Maastrichtian–Paleocene were classified as subaerial unconformities. They are located in the western flank of the Eastern Cordillera and the Western Llanos Basin, respectively.
These unconformities were evaluated as potential forebulge unconformities. The implications of its location should be reflected in the preserved sedimentary thickness. In the first scenario, where the forebulge is located along the western flank of the Eastern Cordillera, most of the Eastern Cordillera and westernmost Llanos Basin would comprise the backbulge. Therefore, the sedimentary thickness of the late Campanian to Paleogene succession should be lower than the sedimentary thickness preserved in the foredeep, the Upper and Middle Magdalena Valley Basin. Otherwise, the thickest sedimentary record should be conserved across the Upper and Middle Magdalena Valley and Eastern Cordillera basins.
Once the forebulge unconformity was located, its connection to the depocenter was established. Its association to a maximum regressive surface comprises the first-order compound surface formed when the retroarc foreland basin started. Likewise, a regional subaerial unconformity truncating the Paleocene–Eocene strata was determined as the upper first-order boundary of the sequence as it formed during the final stage of the regional foreland basin.
Regional Geological Framework
The study area is located in the central part of Colombia (Figure 1). The present-day configuration is a hinterland basin (Upper-Magdalena Valley) in the west, and a foreland basin in the east (Llanos Basin), separated by the Eastern Cordillera. Two fault systems delimit the latter, the Salina Fault system in the west and the Guaicaramo Fault system in the east (Figure 1). According to Colleta et al. (1990), Cooper et al. (1995b), and Horton et al. (2010b), the study area was part of a regional basin from the Jurassic until the Paleogene, when the exhumation of the Eastern Cordillera separated it.
Tectonic Setting
During the Late Cretaceous, a first-order change occurred when the extensional basin was transformed into a retroarc foreland basin when the Central Cordillera started is uplifting due to the subduction of the Nasca Plate under the South American plate (Cediel et al., 2011; Spikings et al., 2015; Mora et al., 2020) (Figure 2A). At that time, most of the basin was under marine deposition. By the early Maastrichtian, the orogenic advance resulted in the Central Cordillera acting as a sedimentary source for the Upper and Middle Magdalena Valley (Moreno et al., 2011; Villagómez and Spikings, 2013; Valencia-Gómez et al., 2020) (Figure 2A).
Paleogeographic maps from Bayona (2018), Bayona et al. (2013), Cardona et al. (2018), Reyes-Harker et al. (2015), and Sarmiento (2001) indicate regional progradation of the shoreline from the latest Campanian to Maastrichtian–Paleocene. Indeed, the Paleogene succession suggests fluvial deposition with no marine influence. However, a marine transgression occurred across the eastern flank of the Eastern Cordillera and the Llanos Basin during the Eocene–Oligocene times (e.g., Caballero et al., 2017). At this moment, the eastward migration of the deformation enhanced the exhumation of the Eastern Cordillera (Parra et al., 2009a; Silva et al., 2013). Thus, it separated the regional foreland basin into the Hinterland Magdalena Valley in the west and Foreland Llanos in the east, respectively (Figure 2A).
Stratigraphic Framework
The studied interval extends from the late Campanian to the early Oligocene, comprising the strata deposited during the two first-order changes, the start and end point of the regional retroarc foreland basin (e.g., Gómez et al., 2005a; Villagómez and Spikings, 2013; Mora et al., 2020). In the Upper and Middle Magdalena Valley, succession starts with the Campanian to Maastrichtian Monserrate, Upper Lidita, Nivel the Lutitas y Arenas, and Umir formations. Although they were deposited under shallow marine conditions, they preserve a prograding trend from shelf to shoreface conditions (Guerrero et al., 2000; Ferreira et al., 2002; Garzon et al., 2012). In the Eastern Cordillera and westernmost Llanos Basin, the equivalent units are the Palmichal Gp, Middle Guadalupe Gp (Paleners-Labor), Arenitas de San Antonio, and Lodolitas de Aguas Calientes (Pérez and Salazar, 1978; Guerrero and Sarmiento, 1996; Vergara et al., 1997; Bayona et al., 2003). A similar prograding trend is preserved in these units.
Progradation continued from Maastrichtian to Paleocene. In the Upper and Middle Magdalena Valley, La Tabla, Cimarrona, and Seca formations preserve a change from shallow marine conditions to coastal plain conditions (De Porta, 1965; Lamus et al., 2013; Tchegliakova, 1996). The Upper Guadalupe Gp (Tierna) and Guaduas formations indicate an equal progradation in the Eastern Cordillera and westernmost Llanos Basin (Sarmiento, 1992; Vergara et al., 1997; Caicedo et al., 2002). Nevertheless, subaerial exposure and erosion occurred from the latest Campanian to the earliest Paleocene along the western flank of the Eastern Cordillera and westernmost Llanos Basin (Bayona et al., 2003; Buchely et al., 2015).
The Paleocene–Eocene rock succession is dominated by fluvial deposition across the study area. However, its upper boundary is reported to be unconformable across most of the study area. The lacuna associated with this contact is variable and only inappreciable nearby Bogota. In the Upper and Middle Magdalena Valley, the fluvial deposition is represented by the Palermo/Bache, Seca, and Hoyon formations (Carvajal et al., 1993; Gómez et al., 2003; Lamus et al., 2013). On the contrary, the Bogota, Upper Socha, Los Cuervos, and Barco formations recorded the fluvial deposition along the Eastern Cordillera and westernmost Llanos Basin (Ulloa and Rodríguez, 1975; Guerrero and Sarmiento, 1996; Bayona et al., 2013).
During the Eocene–Oligocene, a change in the depositional conditions took place in the Eastern Cordillera and Llanos Basin. This is evidenced by the middle Eocene fluvial Mirador and Picacho units being overlaid by the Oligocene coastal to marine San Fernando, Concentracion, and Carbonera Fms (Jaramillo and Dilcher, 2000; Ochoa et al., 2012; Buchely et al., 2015; Caballero et al., 2017). Although fluvial conditions prevailed along the Upper and Middle Magdalena Valley, as depicted by Tesalia, San Juan del Rio Seco, Gualanday, and Chicoral units (De Porta, 1966; Ferreira et al., 2002; Veloza et al., 2008).
Data and Methods
We reviewed previous studies from 14 locations across the Upper and Middle Magdalena Valley and Eastern Cordillera. We incorporated new data from the eastern flank of the Eastern Cordillera and the western Llanos Basin (Figure 1). The Sagu and the Wells 1–3 were selected because they were in the marginal part of the late Campanian to Paleogene retroarc foreland basin. Therefore, their correlation with the other sections across the study area will support understanding the basin architecture throughout time. Likewise, the periods of sedimentation and subaerial exposure preserved in their rock successions are crucial for understanding the role of regional tectonism over sedimentation and accommodation rates.
New Data: Lithology and Stratigraphy
Field data from the Sagu creek in the southeastern flank of the Eastern Cordillera was incorporated with well data (cores, logs, and drilling reports) from three wells in the Llanos Basin (Figure 1). They are distributed with an eastward trend from the Sagu to well 3 (Figure 1). The well data was provided by the Geological Survey of Colombia (SGC). The presence of Maastrichtian rocks in Sagu Creek was established due to the Paleocystodinium and Proteacidites dehaani biozones reported nearby by ICP-Ecopetrol (2014a), Martínez (2016), and Vergara and Rodriguez (1997). At the same time, the Campanian age was determined following the Dinogymnium-Cerodinium biozones from Caballero (2014) and ECOPETROL (2012b). Also, the Paleocene and Eocene were defined using the detrital zircon ages and multidimensional analysis from Carvajal-Torres et al. (2019), and the biozones defined by Jaramillo et al. (2011). The stratigraphic columns are presented in Figures 3, 4.
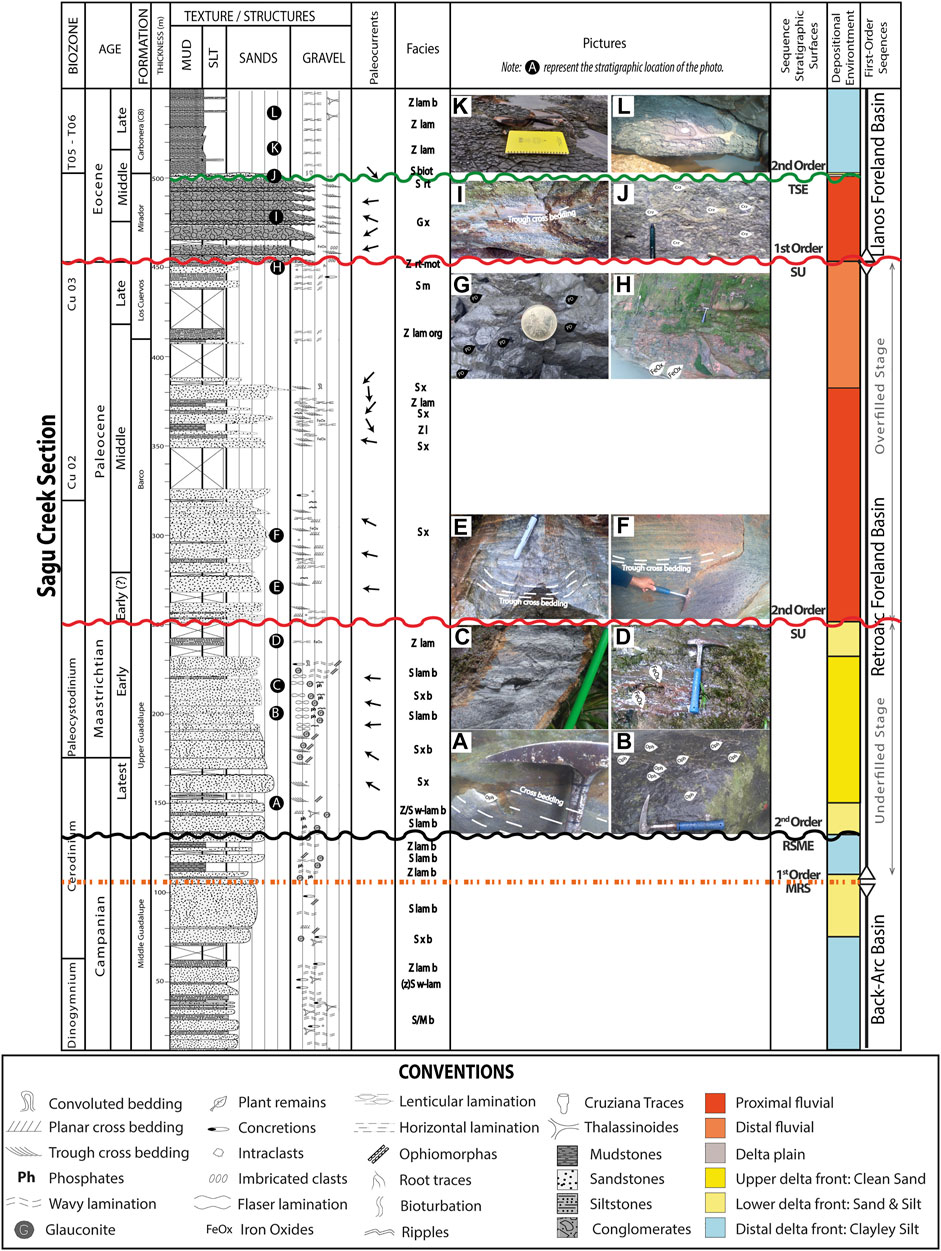
FIGURE 3. Stratigraphic column from the Sagu creek. See lithofacies and facies associations description in Table 2. Location in Figure 1.
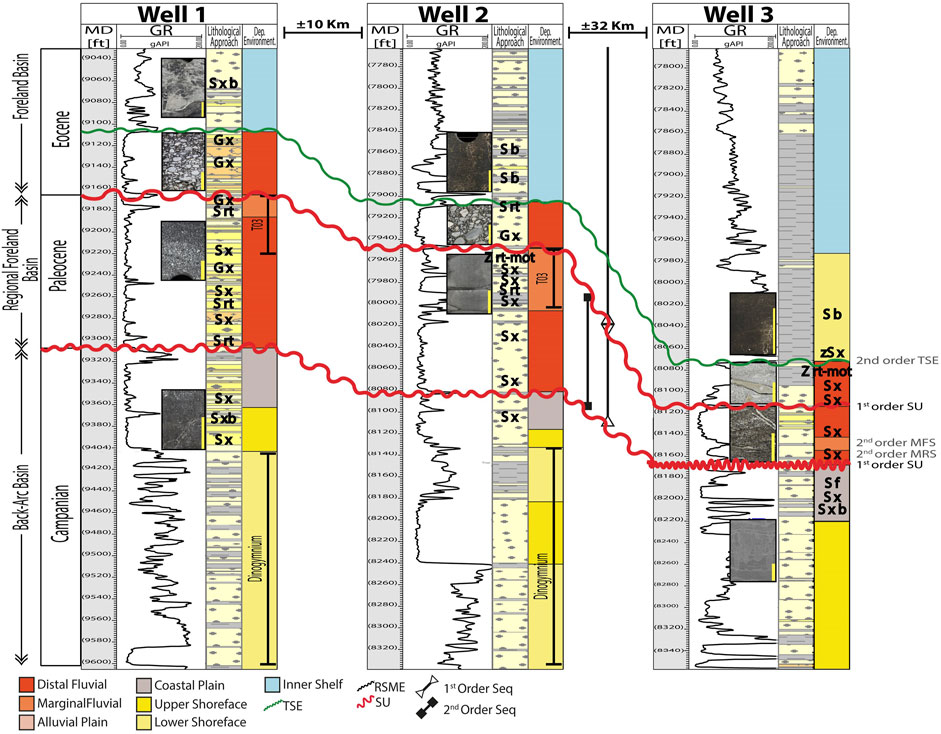
FIGURE 4. Stratigraphic section from wells 1–3 with its corresponding correlation. See lithofacies and facies associations in Table 2. Location in Figure 1.
Based on the lithology and sedimentary structures, 15 lithofacies and six facies associations were established (Tables 2). Their naming and classification scheme follows Farrell et al. (2012), and their description is presented in Tables 2. In general, the rock succession for these four locations is dominated by siliciclastic sediments with a coarsening-upwards general trend from the late Campanian to Eocene and an abrupt change to fine-grained rocks from the Eocene to the Oligocene (Figures 3, 4). The formations and units’ names were established according to previous studies nearby (e.g., Vergara and Rodriguez, 1997; De La Parra, 2009). We identified the Middle and Upper Guadalupe Gps, Barco, Los Cuervos, Mirador, and lower member (C8) of the Carbonera Fms (Figures 3, 4).
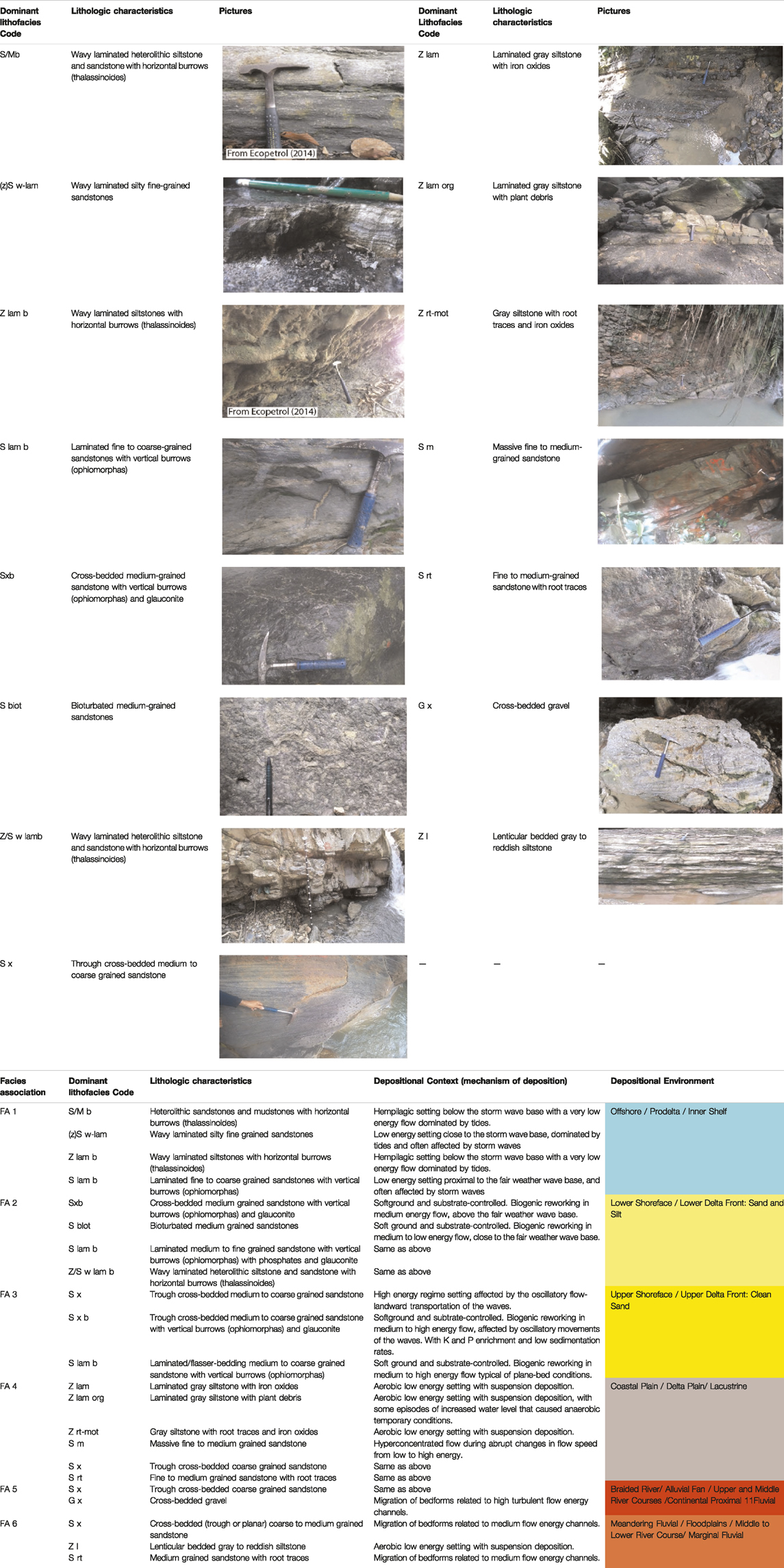
TABLE 2. A) Lithofacies identified in the stratigraphic sections from Sagu and wells, B) Facies associations and depositional environment interpretations.
Our lithostratigraphic analysis indicates that the Middle Guadalupe Gp consists of cross-bedded very fine to medium-grained sandstones with ophiomorpha intercalated with gray-to-black siltstones and mudstones with some thalassinoides. However, in the wells, the same unit is dominated by cross-bedded medium-grained sandstones with some ophiomorpha (Figure 4). This unit is unconformably underlying the Upper Guadalupe Gp, dominated by cross-bedded medium-grained sandstones with some ophiomorpha, phosphates, and glauconite (Figures 3A–C). However, the upper part of this unit preserves gray siltstones with iron oxides (Figure 3D). The Upper Guadalupe Gp unit is absent along the wells as the Paleocene rock units unconformably underlie the Middle Guadalupe.
Likewise, in the Sagu, the Paleocene Barco unit, dominantly composed of cross-bedded coarse-to-medium-grained sandstones, is unconformably on top of the early Maastrichtian Upper Guadalupe Gp. The Barco unit is also identified in each of the wells with a similar lithology but mostly pebbly (Figure 4). At the top of this unit, intercalation with laminated siltstones and silty mudstones is recognized in the Sagu. The following unit is Los Cuervos, composed of gray siltstones with some organic matter that changes to gray sandy siltstones with iron oxides and some roots toward the top in the Sagu (Figures 3G,H).
The portion of Los Cuervos identified in wells 1 and 2 comprises cross-bedded coarse–medium-grained sandstones intercalated with gray-white mottled sandy siltstones (Figure 4). The paleosols on top characterize this rock unit absent in well 3. The succession continues with the Mirador unit, uniformly composed of cross-bedded gravel with iron oxides along each section (Figures 3I, 4). An abrupt change marks its unconformable contact with the overlying Carbonera C8, as depicted by the fine-grained sandstones with ophiomorpha in Figures 3J, 4. Then, the succession changes into black to gray mudstones with thalassinoides (Figures 3K,L, and 4).
To estimate the location of the sediment source of the southeastern part of the Eastern Cordillera and the westernmost Llanos Basin, we measured paleocurrents in the Sagu. Following the methodology presented by DeCelles et al. (1983), we took nearly 2,000 paleocurrent measurements where the trough cross-bedding was exposed. Then, these values were corrected and processed using the Stereonet software. Our results indicate a dominant flow direction from west to east for the Upper Guadalupe, Barco, and Mirador units (Figure 3). However, the north-to-south trend is identified at the top of the Barco and Mirador units (Figure 3).
New Data: Facies Association and Depositional Environments
We identified six facies associations following the lithofacies associations throughout the rock successions; see FA1–FA6 in Table 2. Their depositional context and deposition mechanism were interpreted based on the conceptual depositional model presented in Figure 5. Our model indicates the relation of the interpreted depositional environment regarding the type of shoreline (Figure 4). For example, the upper shoreface and upper delta front in Figures 5A,B were deposited on different shorelines, but their distance from the shoreline remains similar across the same beachline. This model was simplified following the principles of Boggs Jr (2006), Catuneanu (2017), and Nichols (2009). Its purpose is to facilitate the correlation of the new data regionally, contributing to an easier but effective analysis of how the sedimentation and depositional settings changed over time across the entire study area. Although there were different sediment sources during the deposition of those rocks, the depositional environments can be correlated as they deposited along the same shoreline.
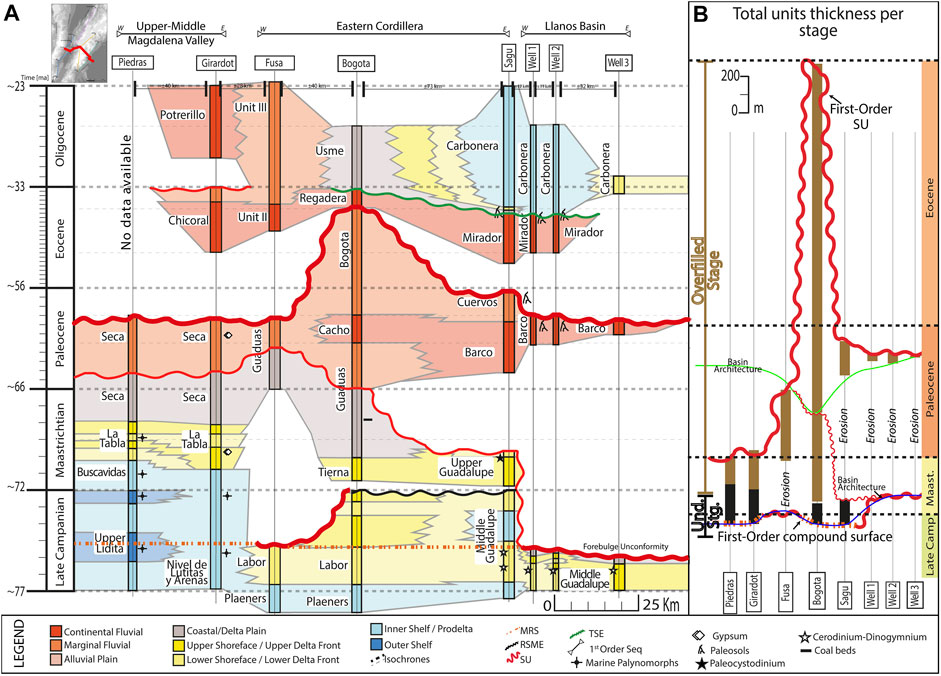
FIGURE 5. (A) Chronostratigraphic correlation from the Upper and Middle Magdalena Valley to the Llanos Basin. (B) Total thickness of strata. Location in Figure 1. Abbreviations: MFS: Maximum Flooding Surface, MRS: Maximum Regressive Surface, SU: Subaerial Unconformity, RSME: Regressive Surface of Marine Erosion, TSE: Transgressive Surface of Erosion. The thickness of the unconformities represents its hierarchy and magnitude.
The facies association suggests that the Middle Guadalupe strata in the Sagu deposited in a low-energy regime under shallow marine conditions. The dominant sedimentary structures and ichnofossils recognized in FA1 and FA2 suggest a deltaic shoreline. Therefore, our interpretation is that the Middle Guadalupe unit deposition is associated with prodelta and lower delta front areas, where finer sediments dominate (Figure 3 and Table 2). The same unit preserves coarser sediments in the wells, indicating a higher energy regime and shallower conditions. The facies associations FA2, FA3, and FA4 are identified within the rock units from the wells. In the lower parts of well 2 and well 3 rock successions, we determined FA2, which indicates lower delta front conditions. The following intervals in well 1 and well 2, plus most strata from well 3, preserve the facies association FA3, suggesting upper delta front conditions with a high-energy regime and more influence of the daily waves and tides (Figure 4 and Table 2). The uppermost part of the Upper Guadalupe records the FA4, indicative of delta plain depositional conditions with hyper-concentrated flows and episodes of increased water level in high-energy aerobic conditions (Figure 4 and Table 2).
In the Sagu, the Upper Guadalupe unit is characterized by FA2 and FA3 (Figure 3 and Table 2). The FA2 is present in the lowermost and uppermost parts of the unit (Figure 3). As mentioned before, this rock unit is absent in the wells. The overlaying Barco Fm is dominated by the FA5 among all sections, from Sagu to the wells. That suggests a high turbulent flow energy regime associated with river channels (Figures 3, 4 and Table 2). The predominance of FA6 distinguishes the following Los Cuervos Fm, indicating migration of channels in a medium-to-high-energy flow regime with periodic flooding and suspension deposition in stream channel banks (Figure 4 and Table 2). The FA5 is also a characteristic of the overlaying Mirador unit (Figure 3). Finally, the Carbonera C8 rock unit preserves the FA2 in the lowermost part but is dominated by FA1 (Figures 3, 4).
Sequence Stratigraphy and Regional Correlation
Following Carvajal Torres et al. (2021), Catuneanu (2017, 2019a), Catuneanu et al. (2011), and MacEachern et al. (2012), we identified some stratigraphic surfaces in the strata from the Sagu and the wells. The first surfaces identified were those related to the following unconformable contact cases: 1) FA2 (lower delta front) overlaying FA1 (prodelta), 2) FA2 (lower delta front) overlaying FA5 (continental proximal fluvial), 3) FA5 (continental proximal fluvial) overlaying FA 2 (lower delta front), 4) FA5 (continental proximal fluvial) overlaying FA4 (delta plain), 5) FA5 (continental proximal fluvial) overlaying FA 6 (marginal fluvial).
Accordingly, either fluvial or marine facies overlies the unconformable contacts. In the case of fluvial facies on top of the unconformable contact, this was defined as the subaerial unconformity (SU) by Van Wagoner (1988) regardless of the underlain facies, for instance, the Cretaceous–Paleocene (Barco-Upper/Middle Guadalupe) and Paleocene–Eocene (Mirador-Los Cuervos) contacts in Figures 3, 4. Meanwhile, in subaqueous conditions, we defined the transgressive surface of erosion (TSE) from Catuneanu (2017) when the marine facies overlies shallower facies; see Carbonera C8 and Mirador contact in Figures 3, 4. Otherwise, it is determined as the regressive surface of marine erosion (RSME) as the one preserved in the Upper-Middle Guadalupe in the Sagu (Figure 3).
We also defined stratigraphic surfaces following the contacts between prograding and retrograding successions. If the contact separates a prograding succession below and a retrograding succession above, it is defined as the maximum regressive surface (MRS) by Posamentier and Vail (1988). On the contrary, if the retrograding sequence underlies the prograding interval, we determined it as the maximum flooding surface (MFS) by Mitchum (1977). We defined a late Campanian MRS and MFS within the Middle Guadalupe unit in the Sagu. They are absent in the wells.
Approximately 27 studies and reports were analyzed in the locations presented in Figure 1. Table 1 summarizes the information compiled from all these studies, showing the age, name, thickness, general lithological description, and reported environmental setting of all the rock units per section. We adjusted the author’s environmental interpretation to the conceptual depositional model presented in Figure 2B.
Subsequently, chronostratigraphic correlations along the adjacent basins were assembled (Figures 5–10). These correlations are intended to cover the main depocenter of the late Campanian to Paleogene retroarc foreland basin. Two correlations depict the chronostratigraphic relationship from the Upper-Middle Magdalena in the west to the Llanos Basin in the west (Figures 5, 7). They expose how the missing gap in the western flank of the Eastern Cordillera correlates with the active depocenters at that time. The other three correlations provide insight into the chronostratigraphic correlations through the main depocenters and both flanks of the Eastern Cordillera (Figures 7–10). We attempted to reconstruct the missing record in the Cobardes Anticline following the vitrinite reflectance, thermochronological data, and nearby stratigraphic reports from Ayala-calvo et al. (2009), Caballero (2010), Caballero et al. (2013a), Porta (1958), and Silva et al. (2013) (Figure 8).
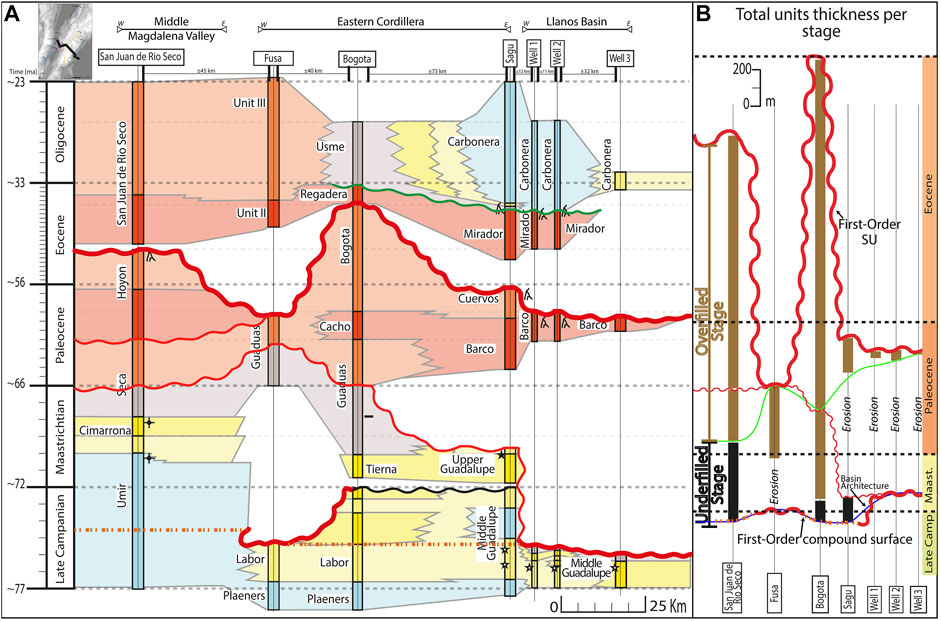
FIGURE 6. (A) Chronostratigraphic correlation from the Upper and Middle Magdalena Valley to the Llanos Basin. (B) Total thickness of strata. Location in Figure 1, conventions in Figure 5. The thickness of the unconformities represents its hierarchy and magnitude.
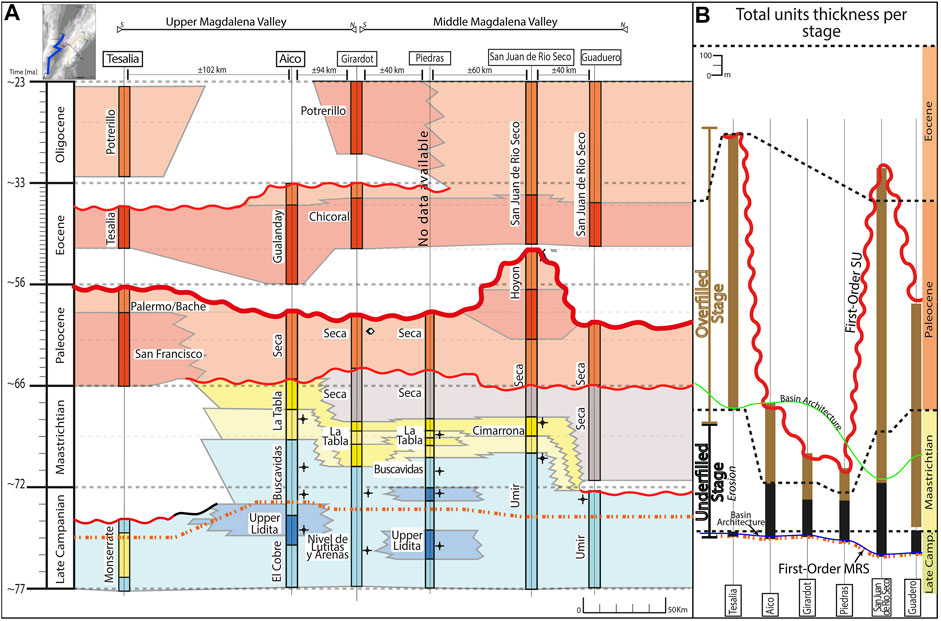
FIGURE 7. (A) Chronostratigraphic correlation from the Upper to Middle Magdalena Valley. (B) Total thickness of strata. Location in Figure 1, conventions in Figure 6. The thickness of the unconformities represents its hierarchy and magnitude.
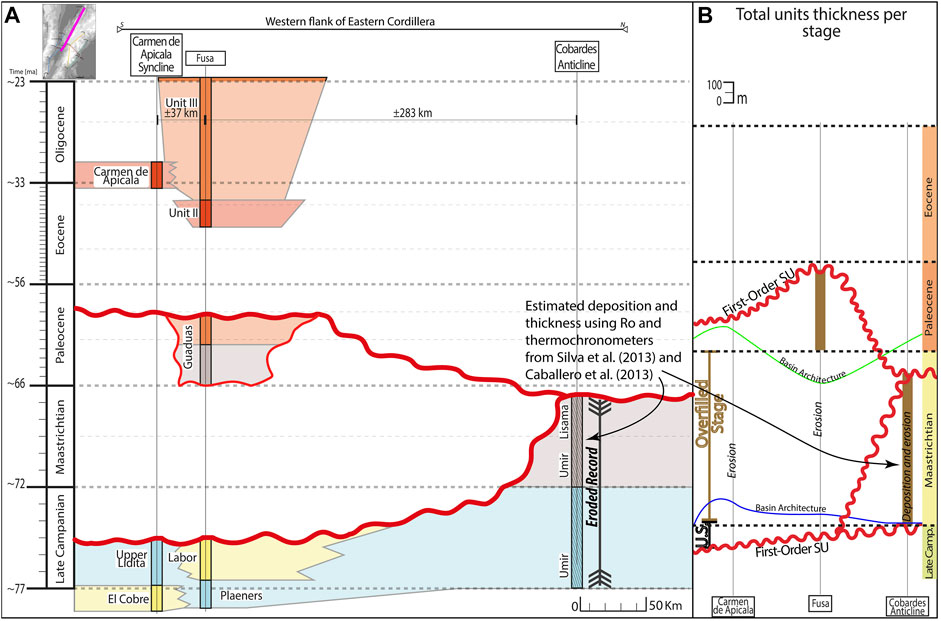
FIGURE 8. (A) Chronostratigraphic correlation from Carmen de Apicala to los Cobardes Anticline (along the western flank of the Eastern Cordillera). Location in Figure 1, conventions in Figure 5. (B) Total thickness of strata. The thickness of the unconformities represents its hierarchy and magnitude.
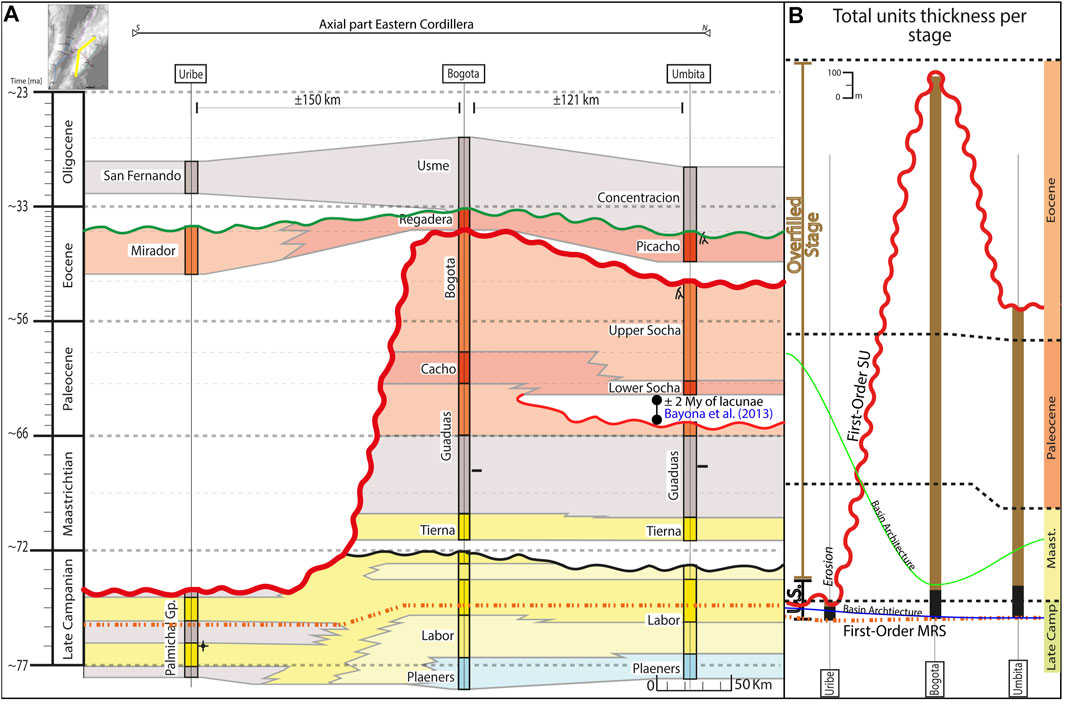
FIGURE 9. (A) Chronostratigraphic correlation from Uribe to Umbita (along the axial part of the Eastern Cordillera). Location in Figure 1, conventions in Figure 5. (A) Total thickness of strata. The thickness of the unconformities represents its hierarchy and magnitude.
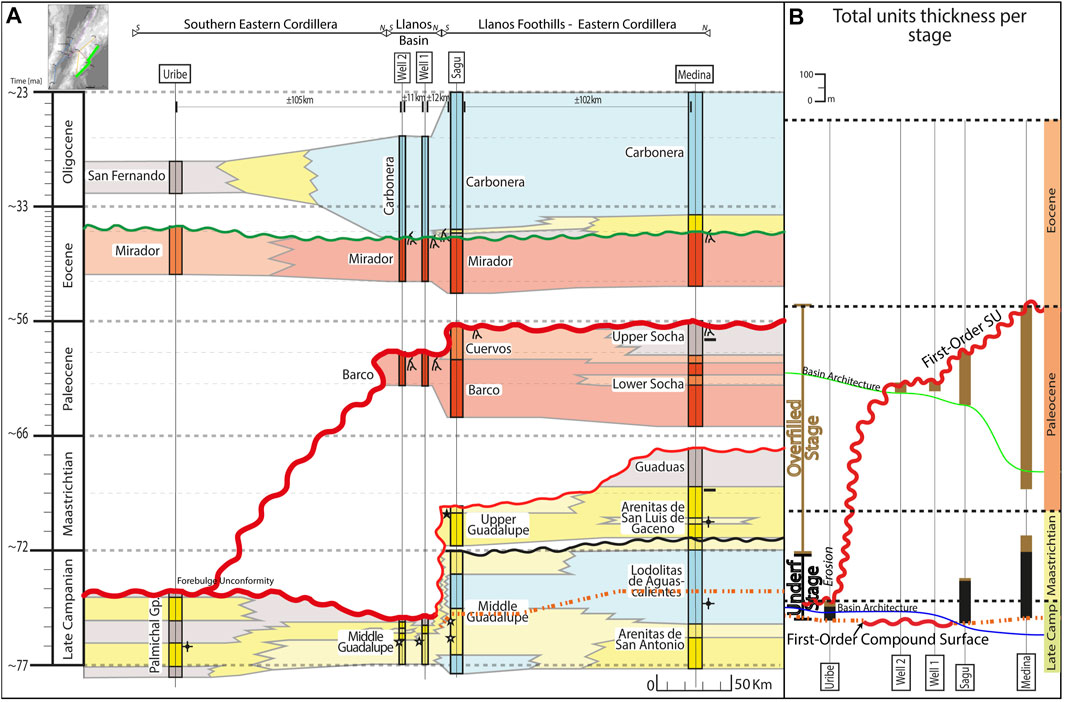
FIGURE 10. (A) Chronostratigraphic correlation along the western Llanos Basin. Location in Figure 1, conventions in Figure 5. (B) Total thickness of strata. The thickness of the unconformities represents its hierarchy and magnitude.
Regarding the stratigraphic surfaces determined in the Sagu and wells, we identified a strong correlation between the subaerial unconformities across the basins. The Cretaceous–Paleocene and Paleocene–Eocene contacts are unconformable and overlaid by fluvial facies. In the Upper and Middle Magdalena Valley, 1) top of the La Tabla and the lower part of the Seca unit; 2) top of Palermo/Bache, Hoyon, and upper Seca units; and 3) top of Tesalia, Chicoral, and Gualanday units (Figure 7A) are evidenced. The latter is restricted to this depocenter, as in Figures 5A, 7A.
A Maastrichtian—Paleocene unconformity is reported along the Eastern Cordillera. Across the axial part and the eastern flank, it is preserved on top of the Bogota and Upper Socha units from the sections in Bogota, Medina, and Umbita (Figures 9A, 10A). In Uribe, this SU truncates the late Campanian Palmichal Gp (Figure 9A). Similarly, the late Campanian Upper Lidita and Labor units from the Carmen de Apicala Syncline and Fusa locations are truncated by another SU that indicates subaerial exposure until the latest Maastrichtian–early Paleocene (Figure 9A).
In contrast, the presence of the subaqueous unconformities (RSME and TSE) is limited to the ECB and western LLB. The TSE is evident on top of the Mirador and Regadera units in the axial part and eastern flank of the Eastern Cordillera (Figures 9A, 10A). This TSE is absent from the western flank of the Eastern Cordillera to the Upper and Middle Magdalena Valley, where only fluvial facies have controlled the deposition since the Paleocene (Figures 5A, 6A). The RSME identified in the Sagu is more difficult to correlate. According to Catuneanu (2017) and MacEachern et al. (2012), the RSME separates inner shelf, prodelta, or offshore sediments below from the lower shoreface deposits above. In Bogota, Umbita, and Medina, a discordant contact is placed in the contact between Middle and Upper Guadalupe units (e.g., Pérez & Salazar, 1978; Guerrero and Sarmiento, 1996; Bayona, 2018). This contact is defined as a non-depositional + erosion period in Bogota and Umbita by Bayona et al. (2013). We correlated this surface as an RSME across the Eastern Cordillera (Figures 9A, 10A).
Likewise, we tracked contacts between prograding and retrograding intervals. In Bogota and Umbita, an interval of upper shoreface was identified within the late Campanian lower shoreface Labor formation, suggesting the presence of another late Campanian MRS. In Medina, Guerrero and Sarmiento (1996) reported a change within the prodelta facies of the Lodolitas de Aguascalientes. In this unit’s uppermost part, the succession of marine black mudstones is interrupted by an heterolithic interval with bivalves and U-shaped ichnofossils. That is accompanied by an abrupt reduction of the marine microfossils and a sudden increase in pollen. Based on this evidence, we delimited the late Campanian MRS in this rock interval (Figure 10A).
The characteristics are similar across the Upper and Middle Magdalena Valley. From Aico to Guaduero, the deposits were deposited under inner shelf conditions. Therefore, there is no similar trend to the one from the ECB and LLB. Nevertheless, late Campanian units preserve a peak of terrestrial microfossils. This peak can be found within the upper part of Monserrate, Upper Lidita, and Umir units from Tesalia, Aico, Piedras, and San Juan de Rio Seco. For instance, Garzon et al. (2012), Guerrero et al. (2000), and Yepes (2001a) reported an increase in pollen and spores for the late Campanian deposits from Aico and Piedras. We interpret this peak as correlatable with the MRS defined in Sagu (Figures 5A, 6A, 7A). This MRS is absent in the sections from Fusa, Carmen de Apicala, and the wells, as a SU truncates the middle to late Campanian strata (Figures 5A, 8A).
First-Order Retroarc Foreland Sequence Fill
Several studies have identified stratigraphic surfaces such as unconformities (e.g., Gómez et al., 2005b; Ramon and Rosero, 2006) and maximum flooding surface (MFS) (e.g., Caballero and Naranjo, 2015, 2017; Caballero et al., 2020). However, their relationship and meaning within the regional tectonic framework are not considered. Instead, the changes in the eustatic sea level are contemplated by some authors (e.g., Garzon et al., 2012) as the primary factor driving transgressions and regressions. Detailed studies focusing on the timing of the structures (e.g., Mora et al., 2010b, 2013b; Silva et al., 2013) demonstrated that active tectonism took place from late Campanian to Paleogene. Initially, the deformation started by folding, resulting in the exhumation of some areas prone to subaerial erosion. Since the Miocene, the contractional stress caused the formation of numerous reverse faults and the inversion of previous normal faults (e.g., Parra et al., 2012; Mora et al., 2013a; Jimenez et al., 2013; Tesón et al., 2013).
The changes in the tectonic setting caused the first-order changes in the study area and defined the lifespan of the regional foreland basin. We analyzed the relationship of our interpreted stratigraphic surfaces with the major and minor tectonic events from the late Campanian to the earliest Oligocene, which is the estimated lifespan of the retroarc foreland basin (e.g., Gómez et al., 2005b; Parra et al., 2009b; Mora et al., 2010a; Horton et al., 2010b; Villagómez, 2010; Moreno et al., 2011; Villagómez and Spikings, 2013). Thus, we determined the hierarchy of each one of those surfaces. Moreover, the basin architecture was estimated based on the sedimentary thickness of the units, which is exposed in Figures 5B, 6B, 7B, 8B, 9B, 10B.
According to Catuneanu (2019b), underfilled accommodation occurs below sea level in a sedimentary basin connected to the global ocean, whereas overfilled accommodation is controlled by fluvial deposition only. The retroarc foreland basin was connected to the global sea level (e.g., Mann et al., 2006). Therefore, we delimited these stages for the basin (Figures 5–9). To analyze how the sedimentation and accommodation were impacted by the tectonic activity and the orogenic advance, we combined the chronostratigraphic correlations with an update to the palinspatically restoration from Bayona (2018) with the new data from Sagu and wells and reviewed numerous paleogeographic restorations from previous authors (e.g., Sarmiento, 2001; Cediel et al., 2011; Reyes-Harker et al., 2015; Caballero et al., 2020). Figures 11, 12 highlighted the basin architecture and the tectonic framework during the first-order changes and the facies distribution during the different stages of the basin evolution.
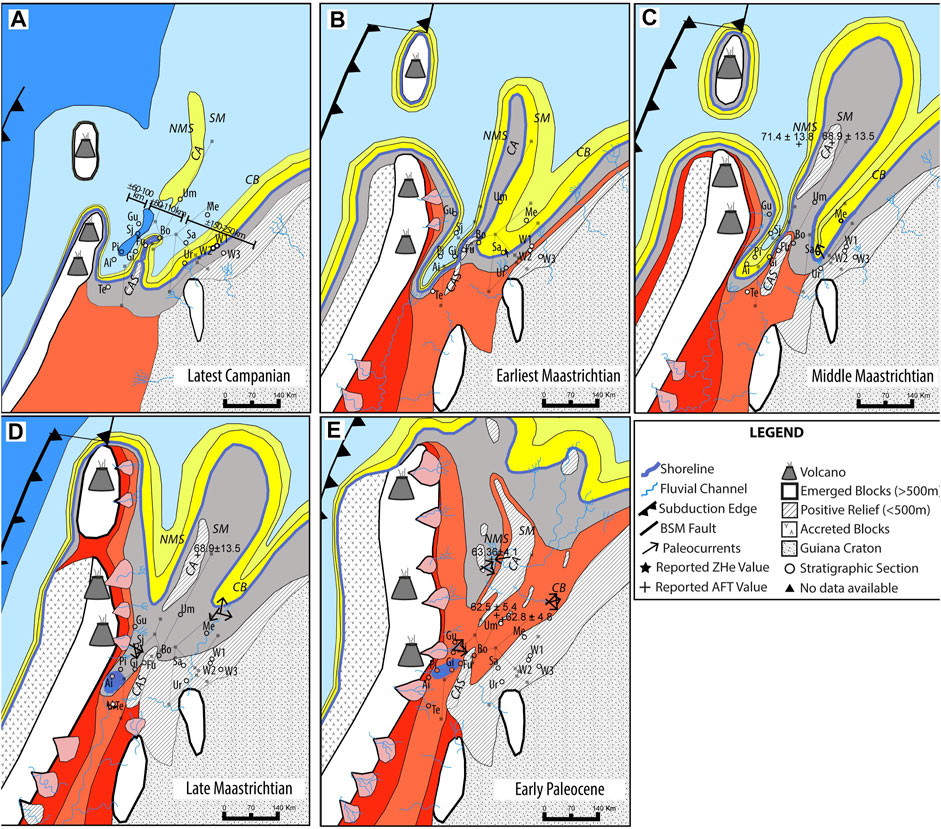
FIGURE 11. Palinspatically restored paleogeographic maps for (A) Latest Campanian, (B) Earliest Maastrichtian, (C) Middle Maastrichtian, (D) Late Maastrichtian, (E) Early Paleocene. Abbreviations: Te, Tesalia; Ai, Aico; Pi, Piedras; Gi, Girardot; Fu, Fusa; Bo, Bogota; Sj, San Juan de Rio Seco, Gu, Guadero; Sa, Sagu; Me, Medina; Um, Umbita; Ur: Uribe; W1/2/3, wells 1–3; CAS, Carmen de Apicala Syncline, NMS, Nuevo Mundo Syncline Area; CA, Cobardes Anticline Area; SM, Santander Massif Area; CB, Cocuy Area. The original positions are represented by the gray squares.
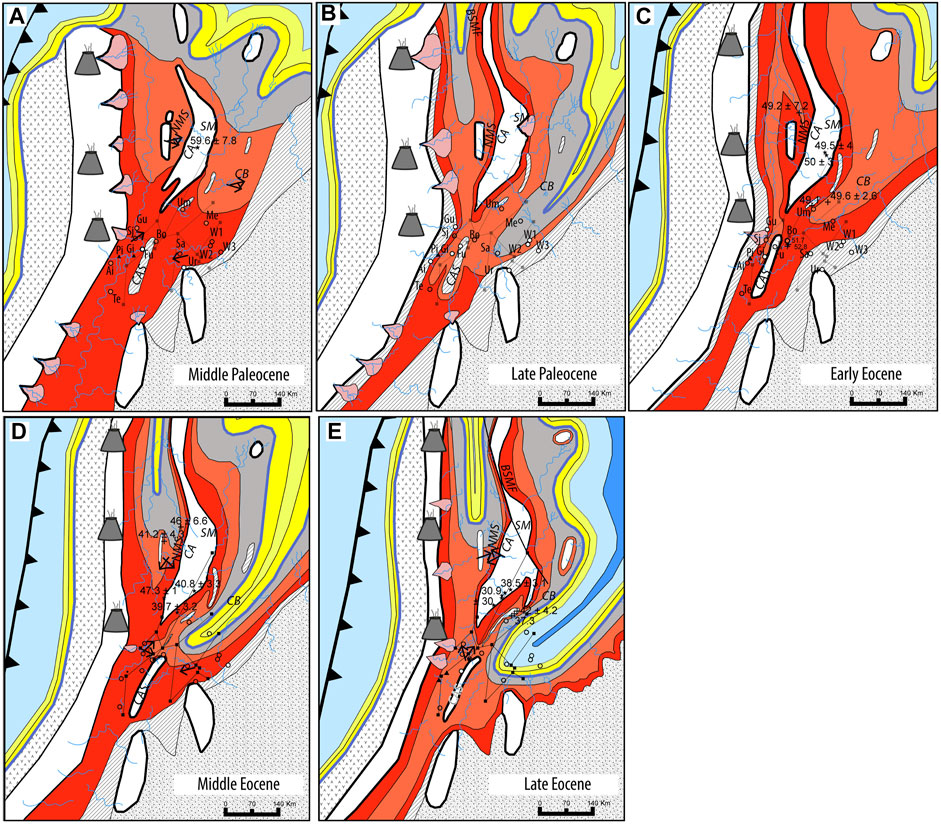
FIGURE 12. Palinspatically restored paleogeographic maps for (A) Middle Paleocene, (B) Late Paleocene, (C) Early Eocene, (D) Middle Eocene, and (E) Late Eocene. See abbreviations and conventions in Figure 11. The original positions are represented by the gray squares.
Late Campanian
The uplift of the paleo-Central Cordillera and the accretion of the oceanic terranes in the study area took place in the late Campanian, causing the end of the extensional basin (Villagómez, 2010; Villagómez and Spikings, 2013; Mora et al., 2020). The contact of a prodraging interval below and a retrograding succession above within the late Campanian strata indicates a shoreline regression across the whole study area (Figures 5–10). The lithological evidence is preserved within the transition from the Upper Lidita to Buscavidas and within the lowermost part of the Umir formation along the Upper and Middle Magdalena Valley (Figure 7). In Aico, Garzon et al. (2012) and Yepes (2001a) report an increase in continental palynomorphs (pollen and spores), whereas the marine palynomorphs content decreased.
Likewise, the Middle Guadalupe unit preserves a contact of upper shoreface facies below and lower shoreface facies succession above across the Easter Cordillera (Figures 9A, 10A). In the western Llanos Basin, the middle Guadalupe (Lodolitas de Aguascalientes in Medina) exposes lower shoreface facies within inner shelf facies (Figures 3, 5, 9). Indeed, the late Campanian rocks from the Medina section indicate a reduction in marine palynomorphs and increased continental and terrestrial microfossils (Guerrero and Sarmiento, 1996). In the Sagu creek, there is a 20–30 m thick lower delta front interval underlying a ±20 m prodelta succession from the late Campanian Middle Guadalupe (Figure 3).
However, there are no strata from the late Campanian in most Llanos Basin (Figures 4, 10). The stratigraphic section from the wells a SU on top of the upper delta front to delta plain middle-late Campanian rocks (Figures 4, 5A, 9A). According to the biostratigraphy from ECOPETROL (2012a) and ICP - Ecopetrol (2014b), the missing record corresponds to the late Campanian to early-middle Paleocene. Similarly, an unconformity is recognized in Tesalia, Fusa, and the Carmen de Apicala sections (Figures 5, 6). The palinspastic restoration put the location of the wells and Tesalia at the distal part of the basin (Figure 11A). On the contrary, the present-day sections of the Fusa and Carmen de Apicala were located within an uplifting block within the basin (Figure 11A). This uplifting block’s estimated lateral extension from west to east is ±80–110 km (Figure 11A).
At the end of the Campanian, the uplifting block was surrounded by two depocenters: the Upper and Middle Magdalena Valley to the west and the area from the Eastern Cordillera (at least from the axial part) to the LLB in the east. They extended ±60–100 km and ±150–250 km, respectively (Figure 11A). Figures 5B, 6B illustrate estimated basin architecture from west to east during the late Campanian to Maastrichtian (the underfilled stage). The data indicate that the emerging block corresponded to the western part of the Eastern Cordillera (Figures 5B, 6, 6B, 9A, 11A).
Early Maastrichtian
The eastward orogenic advance continued at this time. Provenance analysis (e.g., Nie et al., 2010; Lamus et al., 2013) demonstrates that the uplifting Central Cordillera started to be a sediment source by this time (Villagómez and Spikings, 2013; Valencia-Gómez et al., 2020). In addition, the basin records a change from the carbonatic to siliciclastic deposition that Bayona (2018) reported as xenoconformity. In Aico and Tesalia, the southernmost areas, this change occurred in the uppermost Campanian (e.g., Garzon et al., 2012). Although the shoreline moved northward, it still influenced the study area. The shoreline migrated northward and produced subaerial exposure and subsequent erosion in some areas (Figure 11B). Wells 1, 2, and 3 continued under subaerial exposure (Figure 11B).
Figure 12B illustrates the ongoing exhumation of the western Eastern Cordillera. The facies surrounding this uplifting block becomes shallower toward it (Figure 11B). The early Maastrichtian facies succession tends to be prograding across the study area. Figure 11B indicates how the shoreface and coastal deposition expanded along the Eastern Cordillera and western Llanos Basin during early Maastrichtian. At the same time, deposition under inner shelf conditions ceased across the Upper and Middle Magdalena Valley. However, the end of the early Maastrichtian is characterized by fining-upward successions. Guerrero et al. (2000), Tchegliakova (1995), and Yepes (2001b) reported an increase in marine palynomorphs within the Buscavidas and Umir across the Upper and Middle Magdalena Valley. Similarly, finer sediments are identified on the preserved early Maastrichtian rock units of the Sagu, Bogota, and Medina (e.g., Pérez and Salazar, 1978; Guerrero and Sarmiento, 1996).
The thickness of the sedimentary record of the Early Maastrichtian in the Upper/Middle Magdalena Valle is ±110–150 m, whereas the Eastern Cordillera and Llanos Basin records ±80–90 m. Although the thickness change from west to east is minor, data support an eastward pinch-out general trend (Figures 5, 6). From south to north, the thickness varies as well in each depocenter. However, data suggest a northward thickening trend (Figures 7B, 9B, 10B).
Middle Maastrichtian
During this time, the western flank of the Eastern Cordillera started its exhumation, as suggested by the reported AFT values from Caballero et al. (2013b) and Silva et al. (2013) along the Cobardes Anticline (Figure 11C). Also, the general sedimentary thickness increased compared to the early Maastrcithtian rock succession. In the Upper and Middle Magdalena Valley, the thickness rose to ±200 m, whereas it increased up to ±110 m along the Eastern Cordillera Basin. These facts evidence an advanced stage of the compressional retroarc foreland basin, where the tectonism triggered accommodation space across the depocenters.
Nevertheless, the shoreline was migrating northward, triggering a change from shoreface to coastal deposition across most of the Eastern Cordillera and subaerial exposure of the Sagu area (Figure 11C). Although a general regression occurred, another increase in marine microfossils (foraminifera and dinoflagellates) was reported across the Upper and Middle Magdalena Valley (e.g., Tchegliakova, 1996; Garzon et al., 2012), along with numerous exploitable coal beds from the Guaduas Fm in the Eastern Cordillera (Sarmiento, 1992; Bayona et al., 2013). We interpret this as an episode where the accommodation outpaced the sedimentation rate, enhancing the coastal plains’ flooding and reducing continental palynomorphs in the study area.
Late Maastrichtian
This period represents the starting point of the overfilled stage of the foreland basin. Although the sediment thickness (±220 m in the Upper and Middle Magdalena Valley and ±120 m in the Eastern Cordillera) suggests an increase in accommodation, most of the sedimentation occurred under fluvial conditions. The sediment derived from the Central Cordillera (e.g., San Juan del Rio Seco and Guadero in Bayona et al., 2013) enhanced the sediment supply along the basin and favored the northward migration of the shoreline. Coastal plain and marginal facies expanded along the study area (Figure 11D).
The reported presence of gypsum layers in the Aico area demonstrates that the regression of the shoreline produced brackish water bodies (lacustrine environments) in the southern part of the Upper Magdalena Valley. In the Eastern Cordillera, sedimentation only occurred near Bogota and Umbita as the other areas were under subaerial exposure (Figure 11D). The exhuming western ECB block grew and extended northward. Thermochronometers from Silva et al. (2013) revealed exhumation in the Santander Massif. However, neither sediment source controlled the fluvial channels, as indicated by the paleocurrents from Gómez et al. (2005a) and the kinematic restorations from Sanchez et al. (2012).
Early Paleocene
The tectonic activity characterized this period (Bayona et al., 2013; Mora et al., 2020). The eastward orogenic advance continued leading to 1) a more prominent topographic relief in the Cobardes Anticline (western Easter Cordillera) and 2) the establishment of a fluvial network across the study area (Figure 11E). According to paleocurrents measured by previous authors, the Cobardes Anticlne started to exert control in the fluvial net (e.g., Caballero, 2010). This net transported sediments from local sources, as indicated by an increased portion of unstable minerals in the early Paleocene rock units from San Juan del Rio Seco (Bayona et al., 2013).
The unconformable contact between the early Paleocene fluvial facies and the underlying coastal/deltaic plain to marginal facies of the late Maastrichtian is unconformable and diachronic. As mentioned before, this is defined as an SU. In the Sagu and the wells, the Barco Fm is interpreted as early-middle Paleocene following Carvajal-Torres et al. (2019), indicating that subaerial exposure occurred at least during the earliest Paleocene (Figures 3, 4, 9A, 11E). There is no lacunae related to this SU in the Middle/Upper Magdalena Valley and Eastern Cordillera, except for Tesalia, Uribe, and Carmen de Apicala.
Following the east to west chronostratigraphic correlations from Figures 5A, 6A, we identified this SU truncating older units eastward. In the eastern flank of the Llanos Basin, the SU is truncating the lower Maastrichtian Upper Guadalupe unit. In contrast, it truncates late Maastrichtian or earliest Paleocene units across the Middle/Upper Magdalena Valley and Eastern Cordillera (Figures 5A, 6A). Additionally, the south-to-north individual correlation along the Upper and Middle Magdalena Valley and Eastern Cordillera revealed that this SU truncates older units southward (Figures 7A, 10A). Therefore, this SU becomes younger toward the north and west.
Middle Paleocene
Intracontinental blocks exhumed in the MMVB (Figure 12A). For instance, the exhuming blocks across the Middle Magdalena Valley were documented by Caballero et al. (2013a) and Parra et al. (2012). These acted as a local sediment source (Moreno et al., 2011), as shown by the paleocurrent directions in Figure 12A. The tectonism also created new accommodation, evident due to the renewed sedimentation, excluding the Uribe section. High amalgamated channels dominated facies deposited across the study area despite the new accommodation. In the Upper and Middle Magdalena Valley, the San Francisco and lower Hoyon units preserve lithologies associated with high-energy regime stream channel deposition (e.g., Veloza et al., 2008; Lamus et al., 2013), as shown in Table 1.
In the Eastern Cordillera, these fluvial conditions are recorded within the Cacho, Lower Socha, and Barco units. Their lithologies and sedimentary structures indicate the sedimentation was related to higher energy fluvial channels (e.g., Ulloa and Rodríguez, 1975; Bayona et al., 2013). Although the sedimentation was uniformly distributed across the Eastern Cordillera, there is a gap of ±2 My in Umbita (Bayona et al., 2013) (Figure 9A). Compared to the previous period, the sedimentary record is approximately twice thicker in the study area (Figures 5–10). Most of the sedimentation took place in San Juan del Rio Seco and Bogota (Bayona et al., 2013; Lamus et al., 2013).
Paleocurrents across the study area demonstrate that the Central Cordillera, the western flank of the Eastern Cordillera, the Guiana Craton, and the intracontinental highs supplied sediments to the basin. Their continuous surface uplift enhanced the energy and transport capacity of the fluvial channels and triggered an increase in the sediment supply. As a result, aggradation of the facies took place in the study area. The facies distribution exposed in Figures 5, 6, 12A is analogous to the overfilled stage of a foreland basin presented by DeCelles and Giles (1997), DeCelles, (2012), and Nemčok et al. (2009), so it indicates the regional foreland basin was on its final stage.
Late Paleocene
Multiple authors (e.g., Cortés et al., 2006; Tesón et al., 2013; Cardona et al., 2018) affirm that the late Paleocene was a period with significant tectonic activity. Their data show an increase in the magmatic activity in the Central Cordillera, maximum shortening and exhumation of the western ECB, and an eastward expansion of the ongoing deformation. Although the sedimentation was not evenly distributed across the basin, the late Paleocene rock succession is the thickest, reflecting the new accommodation created across the depocenters (Table 1 and Figures 5B–10B). Bayona (2018) and Bayona et al. (2013) determined that rock units from this period register the highest sedimentation rate since the latest Campanian. The Upper and Middle Magdalena Valley and Eastern Cordillera areas preserve a significant change in the facies.
In the Upper and Middle Magdalena Valley, the facies exhibit a shift from high-amalgamated fluvial deposits to low-amalgamated fluvial deposits; the contact between those facies is transitional and conformable 7. Moreover, despite that fluvial conditions controlled the deposition, Ayala-calvo et al. (2009) and Caballero (2010) identified coastal plain facies in the north part of Colombia and marginal lacustrine facies in the Nuevo Mundo Syncline, respectively, documenting the influence of the shoreline located to the north of the study area (Figure 12B). On the contrary, brackish palynomorphs in the Medina section (Guerrero and Sarmiento, 1996) and carbonaceous material in the Sagu Creek unveil the existence of a coastal to marginal facies in the western LLB (Figures 5, 12B).
The high sedimentary thickness suggests an increase in accommodation space. Likewise, the change from high-amalgamated to low-amalgamated channels indicates another period where the accommodation outpaced the sediment supply. However, it was insufficient to allow a regional marine incursion. The marginal lacustrine facies in the Nuevo Mundo Syncline and the brackish palynomorphs in Medina evidence partial flooding with brackish marine waters. Due to the reported tectonic activity and the lack of influence of global eustasy, it is understood that these relatively synchronous changes were triggered by tectonism.
Early Eocene
Sedimentation ceased, and subsequent subaerial exposure occurred in various areas across the basin (Figure 12C). In the Sagu and the wells, Los Cuervos Fm is truncated by an unconformable surface on top (Figures 3, 4). Also, roots associated with the formation of paleosols were identified in each location (Figures 3, 4). Due to this unconformable contact overlaid by fluvial facies, it was classified as a SU. Following the detrital zircon ages from Carvajal-Torres et al. (2019) and ages reported from nearby (e.g., Caballero et al., 2020), the lacuna of this SU is estimated to be from the latest Paleocene to early-middle Eocene in the Sagu. This time gap increases eastward in the area of the wells, where it depicts a time gap from the middle Paleocene to the middle Eocene.
Similarly, paleosols are reported in the Upper and Middle Magdalena Valley and parts of the Eastern Cordillera. Hoyon unit preserves roots and paleosols on top (e.g., De Porta, 1966) (Figure 7A). The same characteristics are recorded in the Upper Socha rock unit in Umbita and Medina (e.g., Jaramillo, 1999) (Figures 8A, 10A). No deposition took place in most of the basin. In the Upper and Middle Magdalena Valley, the Girardot, Tesalia, Aico, and Guaduero areas remained under subaerial exposure, such as Uribe, the Cobardes Anticline, and Carmen de Apicala Syncline in the Eastern Cordillera.
However, deposition continued in the Bogota area. Although the sedimentation rate was the lowest registered since the Maastrichtian, around 500–1,000 m of sediment was deposited in Bogota Fm (Bayona et al., 2010). As the Bogota Fm is also truncated by another SU (Bayona et al., 2013), the SU surface recognized in the Sagu and the wells is correlatable across the study area.
Middle Eocene
Tectonic quiescence dominated during the middle Eocene (Gómez et al., 2005b; Parra et al., 2009b; Mora et al., 2013b; Reyes-Harker et al., 2015). However, areas such as the Nuevo Mundo Syncline were tectonically active (Caballero et al., 2010). Thermochronological data from Caballero et al. (2013a) and Silva et al. (2013) unveil that the western part of the ECB was uplifting. The sediments of the adjacent areas contain unstable minerals such as epidote, pyroxene, amphiboles, and others (titanite) (Bayona et al., 2013). In addition, young peaks in detrital zircon ages and immature continental fluvial deposits suggest a renewed fluvial net with closer sedimentary sources.
The sedimentation renewed at a very low rate. Figure 12D illustrates how the high amalgamated channel fluvial dominated deposition across the study area. However, the Medina area’s shoreface deposits identified by Guerrero and Sarmiento (1996) and Jaramillo and Dilcher (2000) indicate a new incursion of the sea level at the end of this period (Figure 12D). Likewise, the fluvial facies from the Mirador formation in the Sagu cand the wells are overlain by a 1 m thick bed of lower shoreface deposits (Figures 3J, 4). This proximity to the shoreline is not recorded within the sedimentary record from the Upper and Middle Magdalena Valley, as indicated by the fluvial deposits from Tesalia, Gualanday, Chicoral, and San Juan del Rio Seco units (Figures 7A, 12D). Due to the continued uplifting of the western flank of the Eastern Cordillera, this worked as an orographic barrier that impeded the marine incursion westward and further correlation of the facies.
Late Eocene to Earliest Oligocene
The Bucaramanga-Santa Marta strike-slip fault was active due to the compressional regime (Cardona et al., 2018). This compressional regime also caused the eastward migration of the deformation in the ECB and subsequent erosion of the Concentracion Fm (Ochoa et al., 2012). Therefore independent depocenters were created (Villamil, 1999), the Magdalena Valley in the west and the Llanos Basin in the east. Each one of those depocenters continued receiving sediments from the Eastern Cordillera. Thermochronological data and provenance analysis from Bayona et al. (2013), Caballero et al. (2013b), Horton et al. (2010a), Mora et al. (2010a), Moreno et al. (2011), Nie et al. (2010), Sánchez et al. (2012), and Silva et al. (2013) indicate that the Paleogene and Cretaceous rocks were exhumed and prone to erosion during the early Oligocene.
The Central Cordillera and Eastern Cordillera bounded from the west to the east of the hinterland basin in the Magdalena Valley. Low-amalgamated channels fluvial facies dominated in the south (Figure 8B). In the Nuevo Mundo Syncline, the deposition took place in a coastal to proximal fluvial facies (Caballero, 2010). However, the Llanos Basin, bounded in the west by the Eastern Cordillera, was under marine deposition as indicated by the facies from Sagu, wells, and Medina (Figures 3K, 3L, 4, 12E). The coastal facies in the Uribe section suggest the shoreline was located nearby (Figure 12E). Likewise, evidence of a marine incursion is preserved 600 across the western part of the Eastern Cordillera (e.g., Santos et al., 2008).
Discussion
According to Allen and Allen (2005), Catuneanu (2019b), and Nemčok et al. (2013), once the retroarc foreland initiates, the foredeep area subsides and hosts most of the sedimentation. In some scenarios, deep marine retrograding facies deposit across the foredeep, whereas shallow marine deposition occurs in the backbulge. However, the forebulge area uplifts and is subject to either subaerial or subaqueous conditions forming the forebulge unconformity. There are cases in which a condensed section is preserved on top of the forebulge, yet it requires dynamic subsidence, such as in the Western Interior Basin (Catuneanu, 2004, 2019a; Miall et al., 2008). This unconformity underlies the first-order foreland sequence, and its north-south diachroneity results from the diachronic exhumation of the forebulge (Catuneanu 2004).
In the central area of Colombia, once the uplifting of the Central Cordillera started, two areas were exposed. First, the western flank of the Eastern Cordillera was diachronically exposed from south to north. In the south, multiple authors (e.g., Caicedo et al., 2000, 2002; Ramon and Rosero, 2006) recognized an unconformity in the Carmen de Apicala synclines (Figures 8A, 11, 12), indicating exhumation and erosion from the Campanian to the Paleogene (Figure 12). Bayona et al. (2003) identified a similar unconformity in the Fusa section. The time gap associated implies that the exhumation and erosion occurred from the latest Campanian until the latest Maastrichtian–the earliest Paleocene. Then, the sedimentation was resumed with marginal fluvial conditions (Figures 11, 12). The Cobardes Anticline started to uplift north to the study area during the Middle Maastrichtian (Figure 11C). The sedimentation has not resumed since, and the Jurassic rocks are exposed in the structure’s core (Julivert, 1970). The facies overlaying this unconformable contact along the western flank of the Eastern Cordillera indicates it represents a SU with a younger northward pattern from south to north.
Also, most of the Llanos Basin area was uplifting, and it was under subaerial exposure from the late Campanian to the middle Paleocene (Figures 4, 11, 12). Once the compressional regime triggered the formation and uplift of the Central Cordillera in the late Campanian, the previous extensional basin experienced a reduction of accommodation. Subsequently, a northward regression of the shoreline took place. The compression also resulted in a lithospheric flexure that exhumed most of the Llanos Basin. Similar to the unconformity across the western flank of the Eastern Cordillera, it is underlined by the Barco formation’s fluvial facies and is classified as a SU (Figures 3, 8). However, the thickness of the preserved Paleocene sedimentary record is almost half of the one preserved in Fusa.
We evaluated scenarios to define which of the subaerial unconformities is the forebulge unconformity. In the first scenario, the presented estimated lateral extension supports the interpretation of a foredeep across the Upper and Middle Magdalena Valley, a forebulge through the Eastern Cordillera’s western flank, and a backbulge along the Eastern Cordillera and westernmost Llanos Basin. Figure 11A indicates that the presumed foredeep extended for ±60–100 km, whereas the backbulge and forebulge extended for ±80–100 km and ±150–250 km, respectively. Those distances may fit the regular extension for a foreland basin defined by DeCelles and Giles (1997). Nevertheless, the thickness of the late Campanian to Paleogene sedimentary record does not support this idea. Suppose the western flank of the Eastern Cordillera acted as the backbulge during the late Campanian to Paleogene. In that case, its sedimentary record should be just a tiny portion of the preserved thickness across the foredeep (DeCelles and Giles, 1997). Instead, the thickness measured in San Juan del Rio Seco in the Upper and Middle Magdalena Valley and Bogota in the Eastern Cordillera is similar (Figure 6B). The data suggest that the Eastern Cordillera and Upper/Magdalena Valley hosted equivalent sediment from the late Campanian to Paleogene. Therefore, the forebulge was not located along the western flank of the ECB.
In contrast, Gómez et al. (2005b), Gómez et al. (2003), and Parra et al. (2010) suggested that the forebulge of this late Campanian to Paleogene retroarc foreland basin was located across the Llanos Basin. The Middle/Upper Magdalena Valley, Eastern Cordillera, and Llanos Basin comprised the foredeep in this scenario. It extended for ±290–450 km by the latest Campanian, approximately. The thickness of the sedimentary record indicates a pattern of becoming thinner eastward (Figures 5B, 6B). Accordingly, the exhumation and subsequent erosion in Uribe, Sagu, and the wells’ area is associated with the forebulge deformation. The SU that truncates the late Campanian to Middle Maastrichtian strata is defined as the forebulge unconformity.
The diachroneity of the forebulge unconformity is related to the migration of the orogen. Villagómez (2010) stated that the uplifting of the orogen started in the south during the late Campanian and expanded northward. That is reflected in the sedimentary record. Figure 10A demonstrates how the interpreted forebulge unconformity truncates relatively younger strata northward. The unconformity truncates relatively older rock westward from west to east (Figures 5A,B). We interpret this as associated with the orogen’s eastward advance, suggesting the forebulge was not static.
During the late Campanian, the uplifting and exhumation of the forebulge resulted in the erosion of the Middle Guadalupe along the western Llanos Basin. Then, the forebulge expanded westward by the middle-late Maastrichtian, exhuming the rocks from the Sagu area (Figures 11, 12). This westward expansion reached the area of Medina during the early Paleocene (Figure 11E). Nonetheless, the data suggest that the forebulge migrated eastward by the middle-late Paleocene, promoting the deposition of Barco and Cuervos across the western part of the Llanos Basin (Figure 12). That interpretation aligns with the foredeep migration proposed by Bayona et al. (2007).
Catuneanu (2019b) stated that the forebulge unconformity is a first-order stratigraphic surface. It bounds the retroarc foreland sequence at the bottom. However, it is restricted to the forebulge area. Therefore, we analyzed the stratigraphic record across the late Campanian depocenters to determine the stratigraphic response of the depocenters during the start point of the retroarc foreland basin.
In the Sagu, the late Campanian deposits in the study area expose a progradation pattern and a subsequent retrogradation pattern. The upper part of the Middle Guadalupe unit preserves a contact between prograding facies below and retrograding facies above (Figure 3). That contact is absent in the wells because that area was prone to subaerial exposure and erosion. We classified that contact as an MRS. Across the Upper and Middle Magdalena Valley, the Upper Lidita, Buscavidas, and lowermost Umir units preserve a change in sedimentary structures and the distribution of microfossils. Garzon et al. (2012), Guerrero et al. (2000), and Yepes (2001a, 2001b) reported a decrease in marine palynomorphs while the continental microfossils (pores and spores) increased. This marks a coetaneous contact between the underlain prograding pattern and the above retrograding succession, which correlates with the MRS in the Sagu section (Figures 5, 6).
A similar change is recorded within the late Campanian strata across the Easter Cordillera. In the Medina area, the Lodolitas de Aguascalientes registers an increase in pollen content that coincides with an increase in sandy portion and shallow marine fossils and ichnofossils (Guerrero and Sarmiento, 1996). In Bogota and Umbita, at the top of the Middle Guadalupe unit, there is a contact of prograding below and retrograding facies above (e.g., Pérez and Salazar, 1978). We defined another MRS within those intervals. This MRS is absent in the western flank of the Eastern Cordillera due to that area was exhumed and prone to erosion during the late Campanian–Maastrichtian times. However, we define that as another SU.
The south-to-north extension of the late Campanian MRS throughout the depocenters is illustrated in Figures 6–8. The low-resolution isochrones impede the constraining of the exact age of this MRS. Nonetheless, the data indicate this surface is relatively younger in Guaduero than in Tesalia (Figure 6) and relatively younger in Medina than in Sagu (Figure 7). The surface is connected with the first SU formed in the western Eastern Cordillera and the Llanos Basin from west to east (Figures 5, 6). Hence, this suggests they were formed simultaneously, which is hierarchically similar. They are the first-order lower boundary of the retroarc foreland basin and along the overlying deposits belong to the first-order foreland basin sequence.
The upper boundary was formed during tectonic quiescence in the early-middle Eocene. According to Parra et al. (2009b), this tectonic quiescence is associated with orogenic unloading and the subsequent end of the retroarc foreland basin. At this time, the western flank of the Easter Cordillera separated the two depocenters, the Middle/Upper Magdalena Valle in the west, and the Eastern Cordiller–Llanos Basin in the east (Figures 2, 13). For that reason, we defined the SU truncating the Paleocene–Eocene strata across the study area as the upper first-order boundary of the retroarc foreland basin. The overlain units were deposited in a new tectonic setting where the Upper and Middle Magdalena Valley and Llanos Basin acted as independent depocenters.
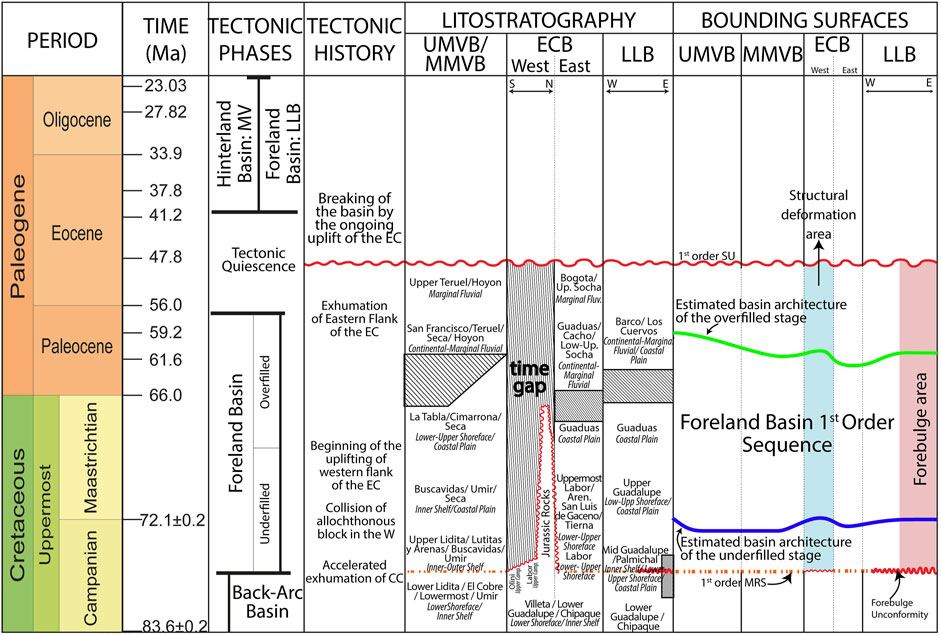
FIGURE 13. Sequence stratigraphic framework of the UMVB, MMVB, ECB, and LLB for the Middle Campanian to Middle Eocene. See the diachroneity of the unconformity along the western part of the ECB. Additionally, see how the forebulge unconformity connects toward the depocenter throughout a first-order MRS, forming the composite first-order lower boundary of the foreland sequence. The upper boundary formed during the Early Eocene due to a period of tectonic quiescence.
Our analysis suggests that the diachroneity of the first-order upper boundary is related to the tectonic stages of orogenic loading and unloading proposed by Parra et al. (2009a). In the Upper and Middle Magdalena Valley, this SU tends to truncate older units northward (Figure 7A). In the Eastern Cordillera, the time gap and age of the rocks truncated by this SU decreases toward Bogota (Figure 9A). At the same time, this SU truncates older units from west to east (Figures 5A, 6A). We associate the west-east diachroneity with the ongoing eastward advance of the tectonic deformation. In contrast, the north-south diachroneity may involve interacting with the Caribbean tectonic plate to the north, which flipped its subduction since the Paleocene times (Montes et al., 2019).
Regarding the continuous sedimentation identified near the Bogota area, the resolution of the data limits the entire understanding of this fact. We associate it with the eastward migration of the depocenters. Bayona (2018) proposed that dynamic subsidence may have occurred in that area. Notwithstanding, Catuneanu (2019a) clarified that dynamic subsidence results in positive accommodation along the forebulge, differing from the new data incorporated from Sagu and wells 1–3. Analyzing the outcrops across the western flank of the Eastern Cordillera and their correlation with the presented data is suggested to constrain the basin architecture and evolution.
Additionally, thicknesses should be decompacted to estimate and establish their temporal variation. Biostratigraphy, thermochronology, and detrital zircon are essential information to improve the understanding of the evolution of the forebulge. The stratigraphic surfaces presented are a powerful element to control the facies correlation. We recommend carrying out a detailed calibration of the palinspastic restorations to obtain a more precise measure of the retroarc foreland basin extent and architecture.
Conclusion
Understanding the lifespan of the retroarc foreland basin is fundamental not only to comprehending its evolution but also to a better understanding of the timing of significant events and their implication on the sedimentary record. Our results indicate that the western flank of the Eastern Cordillera and the western Llanos Basin uplifted and were under subaerial erosion once the retroarc foreland basin started during the late Campanian. Following the sedimentary thickness across the Upper and Middle Magdalena Valley and the Eastern Cordillera, the estimated basin architecture suggest that the uplifting of the western Llanos Basin was related to the forebulge deformation that resulted in the erosion of the Middle Guadalupe units in the area of the wells (Figure 13). The uplifting of the western part of the Eastern Cordillera is understood to be associated with the ongoing compressional deformation. An SU is an unconformity truncating the late Campanian deposits from the Carmen de Apicala and Fusa area, following Catuneanu (2017) and Van Wagoner (1988).
At the same time, the late Campanian Middle Guadalupe from the Sagu preserve a contact separating prograding facies below from retrograding facies above (Figures 3, 13). A similar contact was recognized within the Upper Lidita, Lutitas y Arenas, Buscavidas, and Upper Guadalupe units from the Middle/Upper Magdalena Valley and Eastern Cordillera. That contact is defined as a maximum regressive surface (MRS) according to Catuneanu (2017) and William et al., 1996. The combination of this MRS, the SU from the western flank of the Eastern Cordillera, and the forebulge unconformity represents the lower first-order boundary of the retroarc foreland stratigraphic sequence (Figure 13).
The correlation of the forebulge unconformity to the Sagu, Uribe, and Medina sections indicates that this surface truncates relatively younger units northward and eastward (Figures 5A, 10A). Likewise, the missing record from the Upper Guadalupe to Barco in Sagu and Guaduas to Lower Socha in Medina evidence that the forebulge expanded westward from the latest Campanian to the earliest Paleocene. By the Middle Paleocene, sedimentation resumed in those areas because the forebulge migrated eastward, resulting in the deposition of the Barco and Los Cuervos rock units. The north-to-south diachroneity of the forebulge unconformity is associated with the orogen’s northward migration. Therefore, the Uribe section did not host sediments since the late Campanian. The orogen moved northward, and no accommodation was created in that area.
In the Paleocene, most of the deposition across the depocenter was fluvial. Although the sedimentary thickness reveals that the accommodation space created was higher than in late Campanian to Maastrichtian times, sediment supply and sedimentation outpaced the accommodation rate. That enhanced the northward migration of the shoreline. Nonetheless, the Sagu and Medina strata unveil that brackish ocean water flooded the area by the late Paleocene (Figure 12B). Our interpretation is that the last tectonic pulse that triggered the accommodation rates to outpace the sedimentation took place. That also promoted the area’s flooding to the north of the Nuevo Mundo Syncline (Figure 12B).
During the latest Paleocene and early Eocene, tectonic quiescence ceased accommodation across the central part of Colombia. The SU on top of Los Cuervos Fm in the Sagu and the wells is correlatable across the Eastern Cordillera and the Upper and Middle Magdalena Valley. At this time, the western flank of the Eastern Cordillera started to separate the depocenter (Figures 2, 13). Therefore, this SU represents the end of the retroarc foreland basin, and we determine it as the upper first-order boundary (Figure 13). The Eocene Mirador unit from the Sagu and the wells along with the Regadera, Picacho, Tesalia, Gualanday, Chicoral, and San Juan del Rio Seco was deposited during a transition period.
Our analysis also identified patterns related to the shoreline trajectories during the retroarc foreland basin. We recognized reported changes in the population of marine palynomorphs (e.g., Piedras and Aico), variations in organic matter content and sediment structures (e.g., San Juan del Rio Seco and Girardot), and the presence of coetaneous thick exploitable coal seems (e.g., Bogota). Some of those patterns are contemporaneous and can be related to events where the accommodation outpaced the sedimentation rate, resulting in marine transgressions. The first-order sequence boundaries we present in this study intend to constrain that framework and facilitate the correlation of similar events in the central part of Colombia.
Data Availability Statement
The datasets presented in this study can be found in online repositories. The names of the repository/repositories and accession number(s) can be found below: https://era.library.ualberta.ca/items/a73d1859-2977-4e6b-a9e7-f65175dc92d9.
Author Contributions
Project design and definition of the study area and objectives: JC-T, OC, and AM. Data collection: JC-T and VC. Data interpretation and analysis of the results: JC-T, AM, OC, MR, and VC. Draft manuscript preparation: JC-T and OC. All authors reviewed the results and approved the final version of the manuscript.
Conflict of Interest
The authors declare that the research was conducted in the absence of any commercial or financial relationships that could be construed as a potential conflict of interest.
Publisher’s Note
All claims expressed in this article are solely those of the authors and do not necessarily represent those of their affiliated organizations, or those of the publisher, the editors and the reviewers. Any product that may be evaluated in this article, or claim that may be made by its manufacturer, is not guaranteed or endorsed by the publisher.
Acknowledgments
We thank the ICP-Ecopetrol of Colombia for supporting the fieldwork necessary to complete this work and the Colombian Geological Survey (SGC) for the data provided for the project’s development. Technical discussions and support with the understanding of the geological framework from Ph.D. Luis Quiroz, Gabriel Mendoza, PhD. German Bayona, Ph.D. Brian Horton and Ph.D. Mauricio Parra are gratefully appreciated. JC-T thanks the University of Alberta for the support throughout his academic life.
Supplementary Material
The Supplementary Material for this article can be found online at: https://www.frontiersin.org/articles/10.3389/feart.2022.876140/full#supplementary-material
References
Allen, P. A., and Allen, J. R. (2005). Basin Analysis - Principles and Application. Wiley. Hoboken, New Jersey, United States, Geology.
Ayala-calvo, R. C., Bayona, G., Ojeda-marulanda, C., Cardona, A., Valencia, V., Padrón, C. E., et al. (2009). Estratigrafia y procedencia de las unidades comprendidas entre el Campaniano y el Paleogeno en la subcuenca de Cesar: aportes a la evolución tectónica del área Stratigraphy and provenance of Campanian-Paleogene units in Cesar sub-basin: contributions to. Geologia Colombiana, 3–33
Bayona, G., Baquero, M., Ramírez, C., Tabares, M., Salazar, A. M., Nova, G., et al. (2020). Unravelling the Widening of the Earliest Andean Northern Orogen: Maastrichtian to Early Eocene Intra-basinal Deformation in the Northern Eastern Cordillera of Colombia. Basin Res. 33 (January), 809–845. doi:10.1111/bre.12496
Bayona, G., Cardona, A., Jaramillo, C., Mora, A., Montes, C., Caballero, V., et al. (2013). Onset of Fault Reactivation in the Eastern Cordillera of Colombia and Proximal Llanos Basin; Response to Caribbean-South American Convergence in Early Palaeogene Time. Geol. Soc. Lond. Spec. Publ. 377 (1), 285–314. doi:10.1144/SP377.5
Bayona, G., Cortés, M., Jaramillo, C., and Llinas, R. (2003). The Tertiary Fusagasugá succession; a record of the complex latest Cretaceous-Pre-Miocene deformation in an area between the Magdalena Valley and Sabana de Bogotá. Asociación Colombiana de Geólogos y Geofísicos del Petróleo. Memorias del VIII Simp. Boliv. Cuencas Subandinas. 33, 73. doi:10.3997/2214-4609-pdb.33.paper73
Bayona, G. (2018). El inicio de la emergencia en los Andes del norte: una perspectiva a partir del registro tectónico-sedimentológico del Coniaciano al Paleoceno. Rev. Acad. Colomb. Cienc. Ex. Fis. Nat. 42 (165), 364. doi:10.18257/raccefyn.632
Bayona, G., Jaramillo, C., Rueda, M., Reyes-harker, A., and Torres, V. (2007). Paleocene-Middle Miocene Flexural-Margin Migration of the Nonmarine Llanos Foreland Basin of Colombia. Cienc. Tecnol. Futuro 3 (3), 141
Bayona, G., Montenegro, O., Cardona, A., Jaramillo, C., Lamus, F., Morón, S., et al. (2010). Estratigrafía, procedencia, subsidencia y exhumación de las unidades Paleógenas en el Sinclinal de Usme, sur de la zona axial de la Cordillera Oriental. Geol. Colomb. 35, 5
Boggs, S. (2006). Principles Of Sedimentology And Stratigraphy. 4th ed. LondonPearson Prentice Hall: Merrill Publishing Company.
Buchely, F., Gomez, L., Buitrago, J., Cristancho, A., Moreno, M., Hincapie, G., et al. (2015). Geología de la plancha 305 - San Juan de Arama. Serv. Geol. Colomb. SGC, Mem. Explic., 0–120
Caballero, V. (2014). Correlaciones Cuenca de Llanos Sur - Informe privado ICP-Ecopetrol, Ecopetrol Internal Report: Unpublished.
Caballero, V. (2010). Evolución Tectonico-Sedimentaria del Sinclinal de Nuevo Mundo, Cuenca Sedimentaria Valle Medio del Magdalena Colombia, Durante el Oligoceno-Mioceno. Universidad Industrial de Santander. doi:10.1007/s13398-014-0173-7.2
Caballero, V., Mora, A., Quintero, I., Blanco, V., Parra, M., Rojas, L. E., et al. (2013a). Tectonic Controls on Sedimentation in an Intermontane Hinterland Basin Adjacent to Inversion Structures: the Nuevo Mundo Syncline, Middle Magdalena Valley, Colombia. Geol. Soc. Lond. Spec. Publ. 377 (1), 315–342. doi:10.1144/SP377.12
Caballero, V., Naranjo, J., De La Parra, F., Mora, A., and Reyes-harker, A. (2015). “Estratigrafía de secuencias de los principales reservorios de la Cuenca Llanos Orientales de Colombia – sector sur,” in Xv Congreso Colombiano De Geología. Sociedad Colombiana de Geologia.
Caballero, V., Naranjo, J., Gomez, P. D., Rodriguez, G., Sandoval, J. R., and De La Parra, F. (2017). “Registro de la transgresión marina del Eoceno medio y superior en la cuenca Llanos Sur: implicaciones en exploración de hidrocarburos,” in Xvi Congreso Colombiano De Geología. Santa Marta. Agosto 30 - Septiembre 1.
Caballero, V., Parra, M., and Andres, M. (2010). Levantamiento de la Cordillera Oriental De Colombia durante el Eoceno Tardío – Oligoceno Temprano: Proveniencia sedimentaria en el Sinclinal De Nuevo Mundo, Cuenca Valle Medio Del Magdalena. Bol. Geol. 32 (1), 45
Caballero, V., Parra, M., Mora, A., López, C., Rojas, L. E., and Quintero, I. (2013b). Factors Controlling Selective Abandonment and Reactivation in Thick-Skin Orogens: a Case Study in the Magdalena Valley, Colombia. Geol. Soc. Lond. Spec. Publ. 377 (1), 343–367. doi:10.1144/SP377.4
Caballero, V., Rodríguez, G., Naranjo, J. F., Mora, A., and De la Parra, F. (2020). From Facies Analysis, Stratigraphic Surfaces, and Depositional Sequences to Stratigraphic Traps in the Eocene – Oligocene Record of the Southern Llanos Basin and Northern Magdalena Basin. Geol. Colombia 3, 48. doi:10.32685/pub.esp.37.2019.10
Caicedo, J. C., Lara, H., and Acosta, J. (2002). Memoria Plancha 265 - Iconozco. Instituto de Invesigación e Ingormación Geocientífica: Minero-Ambiental y Nuclear, INGEOMINAS, Memoria Explicativa, Escala, 1–84.
Caicedo, J. C., Terraza Melo, R., and Acosta, J. E. (2000). Mapa Geología de la Plancha 264 Espinal. Ingeominas 1.
Cardona, A., León, S., Jaramillo, J. S., Montes, C., Valencia, V., Vanegas, J., et al. (2018). The Paleogene Arcs of the Northern Andes of Colombia and Panama: Insights on Plate Kinematic Implications from New and Existing Geochemical, Geochronological and Isotopic Data Tectonophysics the Paleogene Arcs of the Northern Andes of Colombia and Panam. Tectonophysics 749 (November), 88–103. doi:10.1016/j.tecto.2018.10.032
Carvajal, C., Fuquen, J., and Acosta, J. (1993). Geologia de la Plancha 282 - Chaparral. Inst. Investig. Geociencias, Minería Quím. INGEOMINAS
Carvajal Torres, J., Catuneanu, O., Catuneanu, O., and Mora, A. (2021). The Role of Tectonism in the Development of Stratigraphic Surfaces in the Colombian Llanos Foreland Basin. University of Alberta. Canada, doi:10.1130/abs/2018am-322116
Carvajal-Torres, J., Catuneanu, O., Andres, M., Caballero, V., and Reyes, M. (2019). “Sequence Stratigraphic Surfaces and Their Relationship with Oil Reservoirs: The Latest Cretaceous—Paleogene Succession of the Llanos Foreland Basin, Colombia,” in International Conference and Exhibition - AAPG (Buenos Aires: American Association of Petroleum Geologists).
Catuneanu, O. (2019a). First-order Foreland Cycles: Interplay of Flexural Tectonics, Dynamic Loading, and Sedimentation. J. Geodyn. 129, 290–298. doi:10.1016/j.jog.2018.03.001
Catuneanu, O., Galloway, W. E., Kendall, C. G. S. C., Miall, A. D., Posamentier, H. W., Strasser, A., et al. (2011). Sequence Stratigraphy: Methodology and Nomenclature. Newsletters Stratigr. 44 (3), 173–245. doi:10.1127/0078-0421/2011/0011
Catuneanu, O. (2019b). Model-independent Sequence Stratigraphy. Earth-Science Rev. 188 (October 2018), 312–388. doi:10.1016/j.earscirev.2018.09.017
Catuneanu, O. (2004). Retroarc Foreland Systems-Eevolution through Time. J. Afr. Earth Sci. 38 (3), 225–242. doi:10.1016/j.jafrearsci.2004.01.004
Catuneanu, O. (2020). Sequence Stratigraphy in the Context of the 'modeling Revolution'. Mar. Petroleum Geol. 116, 104309. doi:10.1016/j.marpetgeo.2020.104309
Catuneanu, O. (2017). Sequence Stratigraphy: Guidelines for a Standard Methodology. Stratigr. Timescales 2 (September), 1–57. doi:10.1016/bs.sats.2017.07.003
Catuneanu, O., and Zecchin, M. (2017). “Comment on Non-unique Stratal Geometries: Implications for Sequence Stratigraphic Interpretations,” in Basin Research. Editors P. M. Burgess, and G. D. Prince, 27, 351–365. doi:10.1111/bre.12192
Cediel, F., Leal-Mejia, H., Shaw, R. P., Melgarejo, J. C., and Restrepo-Pace, P. A. (2011). Regional Geology of Colombia. Petroleum Geol. Colombia 1, 47–81.
Colleta, B., Hébrard, F., Letouzey, J., Werner, P., and Rudkiewikz, J. L. (1990). “Tectonic Style and Crustal Structure of the Eastern Cordillera (Colombia), from a Balanced Cross Section,” in Petroleum and Tectonics in Mobile Belts. Editor J. Letouzey (Paris: Technip), 81
Cooper, M. A., Addison, F. T., Alvarez, R., Coral, M., Graham, R. H., Hayward, A. B., et al. (1995b). Basin Development and Tectonic History of the Llanos Basin, Eastern Cordillera, and Middle Magdalena Valley. colombia 10 (10), 1421–1443. doi:10.1306/522B4DF7-1727-11D7-8645000102C1865D
Cooper, M. A., Addison, F. T., Alvarez, R., Coral, M., Graham, R. H., Hayward, A. B., et al. (1995a). Basin Development and Tectonic History of the Llanos Basin, Eastern Cordillera and Middle Magdalena Valley, Colombia. AAPG Bull. Bol. Serv. Geol. Nac. 79 (1319), 1421–1443. doi:10.1306/7834d9f4-1721-11d7-8645000102c1865d
Cortés, M., Colletta, B., and Angelier, J. (2006). Structure and Tectonics of the Central Segment of the Eastern Cordillera of Colombia. J. S. Am. Earth Sci. 21 (4), 437–465. doi:10.1016/j.jsames.2006.07.004
De La Parra, F. (2009). Palynological Changes across the Cretaceous-Tertiary Boundary in Colombia. South America: University of Florida). Master’s Thesis.
De Porta, J. (1965). Geología del extremo S del Valle Medio del Magdalena entre Honda y Guataquí (Colombia). Bol. Geol. (Bucaramanga)
De Porta, J. (1966). La Estratigrafía del Cretácico Superior y Terciaro en el Extremo S del Valle Medio del Magdalena. Bol. Geol. (Bucaramanga) 19 22
DeCelles, P. G. (2012). “Foreland basin systems revisited: Variations in response to tectonic settings,” in Tectonics of Sedimentary Basins: Recent Advances. Editors C. Busby, and A. Azor Perez (1st Ed.) (Blackwell Publishing Ltd.). doi:10.1002/9781444347166.ch20
DeCelles, P. G., Richard, P. L., and Schwartz, R. K. (1983). Two New Methods of Paleocurrent Determination from Trough Cross-Stratification. SEPM J. Sediment. Res. 53 (2), 629–642. doi:10.1306/212f824c-2b24-11d7-8648000102c1865d
Dengo, C. A., and Covey, M. C. (1993). Structure of the Eastern Cordillera of Colombia: Implications for Trap Styles and Regional Tectonics. Am. Assoc. Petroleum Geol. Bull. doi:10.1306/BDFF8E7A-1718-11D7-8645000102C1865D
ECOPETROL (2012a). Reporte Bioestratigráfico Muestras Pozo Akacias Estratigráfico 2, UST-UST-F-018, Versión 1. Serv. Lab. plantas piloto Bioestratigr. Internal report: Unpublished.
Farrell, K. M., Harris, W. B., Mallinson, D. J., Culver, S. J., Riggs, S. R., Pierson, J., et al. (2012). Standardizing Texture and Facies Codes for A Process-Based Classification of Clastic Sediment and Rock. J. Sediment. Res. 82 (6), 364–378. doi:10.2110/jsr.2012.30
Ferreira, P., Nuñez, A., and Rodriguez, M. A. (2002). Levantamiento Geológico de la plancha 323 - Neiva. Serv. Geol. Colomb. SGC, Mem. Explic, 26–62. Escala 1:100.000.
Garzon, S., Warny, S., and Bart, P. J. (2012). A Palynological and Sequence-Stratigraphic Study of Santonian-Maastrichtian Strata from the Upper Magdalena Valley Basin in Central Colombia. Palynology 36 (1), 112–133. doi:10.1080/01916122.2012.675147
Gómez, E., Jordan, T. E., Allmendinger, R. W., and Cardozo, N. (2005a). Development of the Colombian Foreland-Basin System as a Consequence of Diachronous Exhumation of the Northern Andes. Bull. Geol. Soc. Am. 117 (9–10), 1272–1292. doi:10.1130/B25456.1
Gómez, E., Jordan, T. E., Allmendinger, R. W., Hegarty, K., Kelley, S., and Heizler, M. (2003). Controls on Architecture of the Late Cretaceous to Cenozoic Southern Middle Magdalena Valley Basin, Colombia. GSA Bull. 115 (2), 131–147. doi:10.1130/0016-7606(2003)115<0131
Gómez, E., Jordan, T. E., Allmendinger, R. W., Hegarty, K., and Kelley, S. (2005b). Syntectonic Cenozoic Sedimentation in the Northern Middle Magdalena Valley Basin of Colombia and Implications for Exhumation of the Northern Andes. Bull. Geol. Soc. Am. 117 (5–6), 547–569. doi:10.1130/B25454.1
Guerrero, J., and Sarmiento, G. (1996). Estratigrafía física, palinológica, sedimentológica y secuencial del Cretácico Superior y Paleoceno del Piedemonte Llanero. Implicaciones en exploración petrolera. Geol. Colomb. 5 (20), 3
Guerrero, J., Sarmiento, G., and Navarrete, R. (2000). The Stratigraphy of the W Side of the Cretaceous Colombian Basin in the Upper Magdalena Valley. Reevaluation of Selected Areas and Type Localities Including Aipe, Guaduas, Ortega, and Piedras. Geol. Colomb. 25, 45
Horton, B. K., Saylor, J. E., Nie, J., Mora, A., Parra, M., Reyes-Harker, A., et al. (2010b). Linking Sedimentation in the Northern Andes to Basement Configuration, Mesozoic Extension, and Cenozoic Shortening: Evidence from Detrital Zircon U-Pb Ages, Eastern Cordillera, Colombia. Bull. Geol. Soc. Am. 122 (9–10), 1423–1442. doi:10.1130/B30118.1
Horton, B., Parra, M., Saylor, J., Nie, J., Mora, A., Torres, V., et al. (2010a). Resolving Uplift of the Northern Andes Using Detrital Zircon Age Signatures. Gsat 20 (7), 4–10. doi:10.1130/GSATG76A.1
ICP-Ecopetrol (2014a). Registro de Descripción Sedimentológica y Estratigráfica - Pozo Chichimene SW 3. Ecopetrol Internal Report: Unpublished.
ICP-Ecopetrol (2014b). Reporte Biostratigráfico Piedemonte y Cuenca de Llanos. Bucaramanga. Ecopetrol Internal Report: Unpublished.
Jaramillo, C. A., and Dilcher, D. L. (2000). Microfloral Diversity Patterns of the Late Paleocene – Eocene Interval in Colombia. North. S. Am. (9), 815–818. doi:10.1130/0091-7613(2000)028<0815:mdpotl>2.3.co;2
Jaramillo, C. A., Rueda, M., and Torres, V. (2011). A Palynological Zonation for the Cenozoic of the Llanos and Llanos Foothills of Colombia. Palynology 35 (1), 46–84. doi:10.1080/01916122.2010.515069
Jaramillo, C. (1999). Middle Paleogene Palynology of Colombia, South America: Biostratigraphic, Sequence Stratigraphic, and Diversity Implications. Universty of Florida.
Jimenez, L., Mora, A., Casallas, W., Silva, A., Tesón, E., Tamara, J., et al. (2013). Segmentation and Growth of Foothill Thrust-Belts Adjacent to Inverted Grabens: the Case of the Colombian Llanos Foothills. Geol. Soc. Lond. Spec. Publ. 377 (1), 189–220. doi:10.1144/SP377.11
Julivert, M. (1970). Cover and Basement Tectonics in the Cordillera Oriental of Colombia, South America, and a Comparison with Some Other Folded Chains. Geol. Soc. Am. Bull. 81 (8), 2181–2202. doi:10.1130/0016-7606(1970)81[3623:cabtit]2.0.co;2
Lamus, F., Bayona, G., Cardona, A., and Mora, A. (2013). Procedencia de las unidades cenozoicas del sinclinal de guaduas: Implicación en la evolución tectónica del sur del valle medio del magdalena y orógenos adyacentes. Bol. Geol. 35 (1), 17
MacEachern, J. A., Dashtgard, S. E., Knaust, D., Catuneanu, O., Bann, K. L., and Pemberton, S. G. (2012). Sequence Stratigraphy. Dev. Sedimentology 64, 157–194. doi:10.1016/B978-0-444-53813-0.00006-X
Mann, P., Escalona, A., and Verónica, M. (2006). Regional Geologic and Tectonic Setting of the Maracaibo Supergiant Basin, Western Venezuela. Bulletin 90 (4), 445–477. doi:10.1306/10110505031
Martínez, A. (2016). “Reconstrucciónn Paleogeográfica Y Análisis De Facies Con Potencial Para Acumular Hidrocarburos En La Formación Guadalupe, Flanco Oriental De La Cordillera Oriental Entre Villavicencio Y Guamal(Meta),” (Universidad Industrial de Santander). (Master’s Thesis.
Miall, A. D., Catuneanu, O., Vakarelov, B. K., and Post, R. (2008).The Western Interior Basin, Sediment. Basins World, 5, 329–362. doi:10.1016/S1874-5997(08)00009-9
Mitchum, R. M. (1977). Seismic Straigraphy and Global Chandes of Sea Level, Part 11: Glossary of Terms Used in Seismic Stratpgraphy. Seismic Stratigr. - Appl. Hydrocarbon Explor., 205
Montes, C., Rodriguez-Corcho, A. F., Bayona, G., Hoyos, N., Zapata, S., and Cardona, A. (2019). Continental Margin Response to Multiple Arc-Continent Collisions: The Northern Andes-Caribbean Margin Earth-Science Reviews Continental Margin Response to Multiple Arc-Continent Collisions: The Northern Andes-Caribbean Margin. Earth-Science Rev. 198 (September), 102903. doi:10.1016/j.earscirev.2019.102903
Mora, A., Blanco, V., Naranjo, J., Sanchez, N., Ketcham, R. A., Rubiano, J., et al. (2013a). On the Lag Time between Internal Strain and Basement Involved Thrust Induced Exhumation: The Case of the Colombian Eastern Cordillera. J. Struct. Geol. 52 (1), 96–118. doi:10.1016/j.jsg.2013.04.001
Mora, A., Horton, B. K., Mesa, A., Rubiano, J., Ketcham, R. A., Parra, M., et al. (2010a). Migration of Cenozoic Deformation in the Eastern Cordillera of colombia Interpreted from Fission Track Results and Structural Relationships: Implications for Petroleum Systems. Bulletin 94 (10), 1543–1580. doi:10.1306/01051009111
Mora, A., Parra, M., Strecker, M. R., Kammer, A., Dimaté, C., and Rodríguez, F. (2006). Cenozoic Contractional Reactivation of Mesozoic Extensional Structures in the Eastern Cordillera of Colombia. Tectonics 25 (2), a–n. doi:10.1029/2005TC001854
Mora, A., Parra, M., Strecker, M. R., Sobel, E. R., Hooghiemstra, H., Torres, V., et al. (2008). Climatic Forcing of Asymmetric Orogenic Evolution in the Eastern Cordillera of Colombia. Bull. Geol. Soc. Am. 120 (7–8), 930–949. doi:10.1130/B26186.1
Mora, A., Parra, M., Strecker, M. R., Sobel, E. R., Zeilinger, G., Jaramillo, C., et al. (2010b). The Eastern Foothills of the Eastern Cordillera of colombia: An Example of Multiple Factors Controlling Structural Styles and Active Tectonics. Bull. Geol. Soc. Am. 122 (11–12), 1846–1864. doi:10.1130/B30033.1
Mora, A., Reyes-Harker, A., Rodriguez, G., Tesón, E., Ramirez-Arias, J. C., Parra, M., et al. (2013b). Inversion Tectonics under Increasing Rates of Shortening and Sedimentation: Cenozoic Example from the Eastern Cordillera of Colombia. Geol. Soc. Lond. Spec. Publ. 377 (1), 411–442. doi:10.1144/SP377.6
Mora, A., Villagómez, D., Parra, M., Caballero, V., Spikings, R. A., and Horton, B. K. (2020). Late Cretaceous to Cenozoic Uplift of the Northern Andes: Paleogeographic Implications. Geol. Colombia 3 (4), 89–121. doi:10.1007/978-1-4939-6911-1
Moreno, C. J., Horton, B. K., Caballero, V., Mora, A., Parra, M., and Sierra, J. (2011). Depositional and Provenance Record of the Paleogene Transition from Foreland to Hinterland Basin Evolution during Andean Orogenesis, Northern Middle Magdalena Valley Basin, Colombia. J. S. Am. Earth Sci. 32 (3), 246–263. doi:10.1016/j.jsames.2011.03.018
Muller, J., de di Giacomo, E., Erve Van, A. W., and Maraven, S. A. (1987). A Palynological Zonation for the Cretaceous, Tertiary and Quaternary of Northern South America. Am. Assoc. Stratigr. Palynologists, Contribution Ser. 19, 7
Nemčok, M., Glonti, B., Yukler, A., and Marton, B. (2013). Development History of the Foreland Plate Trapped between Two Converging Orogens; Kura Valley, Georgia, Case Study. Geol. Soc. Lond. Spec. Publ. 377 (1), 159–188. doi:10.1144/SP377.9
Nemčok, M., Schamel, S., and Gayer, R. (2009). Thrustbelts: Structural Architecture, Thermal Regimes and Petroleum Systems. Cambridge: Cambridge University Press.
Nichols, G. (2009). Sedimentology and Stratigraphy. 2nd ed. Oxford, United Kingdom: Wiley-Blackwell.
Nie, J., Horton, B. K., Mora, A., Saylor, J. E., Housh, T. B., Rubiano, J., et al. (2010). Tracking Exhumation of Andean Ranges Bounding the Middle Magdalena Valley Basin, Colombia. Geology 38 (5), 451–454. doi:10.1130/G30775.1
Ochoa, D., Hoorn, C., Jaramillo, C., Bayona, G., Parra, M., and De la Parra, F. (2012). The Final Phase of Tropical Lowland Conditions in the Axial Zone of the Eastern Cordillera of Colombia: Evidence from Three Palynological Records. J. S. Am. Earth Sci. 39, 157–169. doi:10.1016/j.jsames.2012.04.010
Parra, M., Mora, A., Jaramillo, C., Strecker, M. R., Sobel, E. R., Quiroz, L., et al. (2009b). Orogenic Wedge Advance in the Northern Andes: Evidence from the Oligocene-Miocene Sedimentary Record of the Medina Basin, Eastern Cordillera, Colombia. Geol. Soc. Am. Bull. 121 (5–6), 780–800. doi:10.1130/B26257.1
Parra, M., Mora, A., Jaramillo, C., Torres, V., Zeilinger, G., and Strecker, M. R. (2010). Tectonic Controls on Cenozoic Foreland Basin Development in the North-Eastern Andes, Colombia. Basin Res. 22 (6), 874–903. doi:10.1111/j.1365-2117.2009.00459.x
Parra, M., Mora, A., Lopez, C., Ernesto Rojas, L., and Horton, B. K. (2012). Detecting Earliest Shortening and Deformation Advance in Thrust Belt Hinterlands: Example from the Colombian Andes. Geology 40 (2), 175–178. doi:10.1130/G32519.1
Parra, M., Mora, A., Sobel, E. R., Strecker, M. R., and González, R. (2009a). Episodic Orogenic Front Migration in the Northern Andes: Constraints from Low-Temperature Thermochronology in the Eastern Cordillera, Colombia. Tectonics 28 (4), a–n. doi:10.1029/2008TC002423
Perry, C., and Taylor, K. (2007). “Environmental Sedimentology,” in Journal of Soils and Sediments. Editors C. Perry, and K. Taylor (Blackwell Publishing Ltd), 7. doi:10.1065/jss2007.11.263
Pindell, J., and Erikson, J. P. (1994). The Mesozoic Passive Margin of Northern South America, Editor J. A. Salfity (Cretaceous Tectonics of the Andes). doi:10.1007/978-3-322-85472-8
Posamentier, H. W., Vail, P., Wilgus, Cheryl K., and Hastings, Bruce S. (1988). “Eustatic Controls on Clastic Deposition II—Sequence and Systems Tract Models,” in Sea-Level Changes: An Integrated Approach. Editors H. Posamentier, W. John Van, and C. A. Ross (Christopher G. St. C. Kendall).
Qayyum, F., Betzler, C., and Catuneanu, O. (2017). The Wheeler Diagram, Flattening Theory, and Time. Mar. Petroleum Geol. 86 (January 2018), 1417–1430. doi:10.1016/j.marpetgeo.2017.07.034
Ramon, J. C., and Rosero, A. (2006). Multiphase Structural Evolution of the Western Margin of the Girardot Subbasin, Upper Magdalena Valley, Colombia. J. S. Am. Earth Sci. 21 (4), 493–509. doi:10.1016/j.jsames.2006.07.012
Reyes-Harker, A., Ruiz-Valdivieso, C. F., Mora, A., Ramírez-Arias, J. C., Rodriguez, G., De La Parra, F., et al. (2015). Cenozoic Paleogeography of the Andean Foreland and Retroarc Hinterland of Colombia. Bulletin 99, 1407–1453. doi:10.1306/06181411110
Sánchez, J., Horton, B. K., Tesón, E., Mora, A., Ketcham, R. A., and Stockli, D. F. (2012). Kinematic Evolution of Andean Fold-Thrust Structures along the Boundary between the Eastern Cordillera and Middle Magdalena Valley Basin, Colombia. Tectonics 31 (3), a–n. doi:10.1029/2011TC003089
Santos, C., Jaramillo, C., Bayona, G., Rueda, M., and Torres, V. (2008). Late Eocene Marine Incursion in North-Western South America. Palaeogeogr. Palaeoclimatol. Palaeoecol. 264, 140–146. doi:10.1016/j.palaeo.2008.04.010
Sarmiento, G. (1992). Estratigrafía y Medios de Depósito de la Formación Guaduas. Bol. Geol. - Inst. Investig. Geociencias, Minería Quím. INGEOMINAS 32 (1–3), 3–44. doi:10.32685/0120-1425/bolgeol32.1-3.1992.193
Sarmiento, L. F. (2011b). Petroleum Geology of Colombia: Geology and Hydrocarbon Potential Middle Magdalena Basin, 11, 191.
Sarmiento, L. (2001). Mesozoic Rifting and Cenozoic Basin Inversion History of the Eastern Cordillera, Colombian Andes: Inferences from Tectonic Models. Vrije Universiteit Amsterdam.
Saylor, J. E., Horton, B. K., Stockli, D. F., Mora, A., and Corredor, J. (2012). Structural and Thermochronological Evidence for Paleogene Basement-Involved Shortening in the Axial Eastern Cordillera, Colombia. J. S. Am. Earth Sci. 39, 202–215. doi:10.1016/j.jsames.2012.04.009
Silva, A., Mora, A., Caballero, V., Rodriguez, G., Ruiz, C., Moreno, N., et al. (2013). Basin Compartmentalization and Drainage Evolution during Rift Inversion : Evidence from the Eastern Cordillera of Colombia. Geol. Soc. Lond. Spec. Publ. Oct, 369–409. doi:10.1144/SP377.15
Spikings, R., Cochrane, R., Villagomez, D., Van der Lelij, R., Vallejo, C., Winkler, W., et al. (2015). The Geological History of Northwestern South America: from Pangaea to the Early Collision of the Caribbean Large Igneous Province (290-75Ma). Gondwana Res. 27 (1), 95–139. doi:10.1016/j.gr.2014.06.004
Tchegliakova, N. (1995). Los Foraminiferos de la Formacion Umir (Seccion Quebrada La Julia): Registro del Cretacico Superior cuspidal (Maastrichtiano) en el Valle Medio del Magdalena. Colombia: Geologia Colombiana.
Tchegliakova, N. (1996). Registro de las Biozonas de Foraminlferos Planct6nicos Gansserina gansseri y Abathomphalus mayaroensis (Maastrchtiano Medio y Superior) en el Extremo Meridional del Valle Medio del Magdalena. Colombia, Sur America.
Terraza Melo, R., Caicedo, J. C., Jiménez, D. M., and Morales, C. J. (2002). Geología de la Plancha 264 Espinal, Escala 1:100.000, Memoria Explicativa. Ingeominas 128, 32–77.
Tesón, E., Mora, A., Silva, A., Namson, J., Teixell, A., Castellanos, J., et al. (2013). Relationship of Mesozoic Graben Development, Stress, Shortening Magnitude, and Structural Style in the Eastern Cordillera of the Colombian Andes. Geol. Soc. Lond. Spec. Publ. 377 (1), 257–283. doi:10.1144/SP377.10
Ulloa, C., and Rodríguez, E. (1975). Mapa geológico de colombia cuadrángulo k-12 guateque planchas 210 guateque - 229 gachalá. Mem. Explic. SGC.
Valencia-Gómez, J. C., Cardona, A., Bayona, G., Valencia, V., and Zapata, S. (2020). Análisis de procedencia del registro sin-orogénico Maastrichtiano de la Formación Cimarrona, flanco Occidental de la Cordillera Oriental colombiana. Bol. Geol. 42 (3), 171–204. doi:10.18273/revbol.v42n3-2020008
Van Wagoner, J. C. (1988). An Overview of the Fundamentals of Seqence Stratigraphy and Key Definitions. SEPM Special Publication.
Veloza, G. E., Mora, A., Freitas, M. De, and Mantilla, M. (2008). Dislocación De Facies En El Tope De La Secuencia Cretácica De La Subcuenca De Neiva , Valle Superior Del Magdalena Y Sus Implicaciones En El Modelo Estratigráfico Secuencial Colombiano. Bol. Geol. 30 (1).
Vergara, L., Rodríguez, G., Martínez, I., Rodriguez, G., and Martinez, I. (1997). Agglutinated Foraminifera and Sequence Stratigraphy from the Chipaque Formation (Upper Cretaceous) of El Crucero Section, Colombia, South America. Micropaleontology 43 (2), 185–201. doi:10.2307/1485781
Vergara, S., L. E., and Rodriguez, C., G. (1997). The Upper Cretaceous and Lower Paleocene of the Eastern Bogota Plateau and Llanos Thrustbelt, Colombia: Alternative Appraisal to the Nomenclature and Sequence Stratigraphy. Geol. Colomb. (22), 51
Villagómez, D., and Spikings, R. (2013). Thermochronology and Tectonics of the Central and Western Cordilleras of Colombia: Early Cretaceous-Tertiary Evolution of the Northern Andes. Lithos 160-161, 228–249. doi:10.1016/j.lithos.2012.12.008
Villagómez, D. (2010). “Thermochronology, geochronology and geochemistry of the Western and Central cordilleras and Sierra Nevada de Santa Marta, Colombia: The tectonic evolution of NW South America,” (Geneva, Switzerland: Université De Genève). THÈSE.
Villamil, T. (1999). Campanian-Miocene Tectonostratigraphy, Depocenter Evolution and Basin Development of Colombia and Western Venezuela. Palaeogeogr. Palaeoclimatol. Palaeoecol. 153 (1–4), 239–275. doi:10.1016/S0031-0182(99)00075-9
Wheeler, H. E. (1959). Stratigraphic Units in Space and Time. Am. J. Sci. 257, 692–706. doi:10.2475/ajs.257.10.692
William, H.-H., Ole, J., Mart, W., and Martinsen, O. J. (1996). Shoreline Trajectories and Sequences: Description of Variable Depositional-Dip Scenarios. SEPM J. Sediment. Res. 66. doi:10.1306/d42683dd-2b26-11d7-8648000102c1865d
Yepes, O. (2001a). Dinoflagellates from the Upper Campanian - Maastrichtian of Colombia and Western Venezuela: Biostratigraphic and Sequence Stratigraphic Implications. College Staton, TX: Texas A&M University.
Yepes, O. (2001b). Maastrichtian-Danian Dinoflagellate Cyst Biostratigraphy And Biogeography From Two Equatorial Sections In Colombia And Venezuela. Palynology 25, 217–249. doi:10.2113/0250217
Keywords: stratigraphy-historical geology, paleoecology, sequence stratigraphic, first-order surface, subaerial unconformity, transgressive surface of erosion, foreland, forebulge unconformity
Citation: Carvajal-Torres J, Catuneanu O, Mora A, Caballero V and Reyes M (2022) First-Order Stratigraphic Boundaries of the Late Cretaceous–Paleogene Retroarc Foreland Basin in Colombia. Front. Earth Sci. 10:876140. doi: 10.3389/feart.2022.876140
Received: 15 February 2022; Accepted: 09 June 2022;
Published: 12 September 2022.
Edited by:
Julie Fosdick, University of Connecticut, United StatesReviewed by:
Elizabeth Balgord, Weber State University, United StatesCecilia Eugenia Del Papa, CONICET Rosario, Argentina
Amanda Calle, University of Texas at Austin, United States
Copyright © 2022 Carvajal-Torres, Catuneanu, Mora, Caballero and Reyes. This is an open-access article distributed under the terms of the Creative Commons Attribution License (CC BY). The use, distribution or reproduction in other forums is permitted, provided the original author(s) and the copyright owner(s) are credited and that the original publication in this journal is cited, in accordance with accepted academic practice. No use, distribution or reproduction is permitted which does not comply with these terms.
*Correspondence: Juan Carvajal-Torres, amNhcnZhamFAdWFsYmVydGEuY2E=