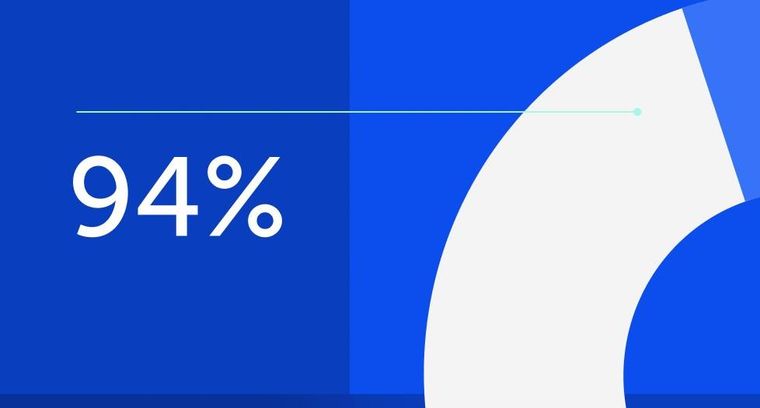
94% of researchers rate our articles as excellent or good
Learn more about the work of our research integrity team to safeguard the quality of each article we publish.
Find out more
ORIGINAL RESEARCH article
Front. Earth Sci., 11 April 2022
Sec. Geohazards and Georisks
Volume 10 - 2022 | https://doi.org/10.3389/feart.2022.875010
This article is part of the Research TopicApplications of Geotechnical Mechanics in Underground EngineeringView all 41 articles
Microbial carbonization based on carbon-capturing bacteria induced calcium carbonate precipitation has become a new construction material for soil improvement. This paper presents a new technique for dust control in sand using Streptomyces microflavus induced calcium carbonate precipitation. This kind of carbon-capturing bacteria can capture and transform CO2 into carbonate then precipitate calcium carbonate crystals through the microbial carbonization process, which then acts as cementitious materials to bind sand particles. In this way, loose sand particles can be bridged and increase their hardness, consequently forming a consolidated layer for dust control. The Shore hardness of the consolidated layer using Streptomyces microflavus could be improved to 25 HD with a calcium carbonate content of 5.5% after 7 times of carbonization treatment. The microstructure, Shore hardness, and obtained calcium carbonate content were also compared with sand samples treated by Paenibacillus mucilaginosus. These results reveal that the consolidated sand layer using Streptomyces microflavus is sufficient to against wind erosion or rainfall erosion and has better geomechanical performance than Paenibacillus mucilaginosus.
Fugitive dust is a common issue in desert areas that leads to sandstorms (Raymond et al., 2019). Planting is the most adopted method for dust control in the desert area (Grantz et al., 1998). However, the growth cycle of planting is too long to be effective for dust control. Apart from the planting, using Portland Cement (PC) is another conventional method for dust control. Considering the complex operation, unexpected energy consumption, environmental concerns, and considerable CO2 emission associated with PC production, investigation on alternative cement has been raised (Benhelal et al., 2013; Ruan and Unluer, 2017).
Microbial cement has been proposed in various applications such as erosion control, mitigation of liquefaction, and slope reinforcement (Yu et al., 2019; Yu and Rong, 2022). Unlike cement paste, the small size of microorganisms (2–5 μm) can easily penetrate the desert sand (0.1–0.25 mm) particles without injection, bridging the loose sand particles together and forming a consolidated layer with certain hardness (Hamdan and Kavazanjian, 2016; Zhan et al., 2016). More importantly, the production of microbial cement does not need either a high temperature or to generate substantial CO2 emission, thereby reducing energy consumption and environmental pollution (Whiffin, 2004; Zhang et al., 2022). The microbial cement also has many other advantages, such as simple components, easy operation, environmentally friendly, and strong aging resistance (DeJong et al., 2010; Harkes et al., 2010). This allows the microbial cement to be more feasible in dust control.
Studies have been carried out to use microbial cement for dust control in desert area, which is usually accomplished through a microbially induced calcite precipitation (MICP) process (Mujah et al., 2017; Lo et al., 2020; Shi et al., 2021a; Shi et al., 2021b; Sun et al., 2021). The precipitates act as a binding material to connect soil particles, consequently providing strength but keeping certain permeability (Cheng, 2012; Cheng and Cord-Ruwisch, 2014). This leads to microbial cement becoming an innovative method for dust control. Other than the microbial method, enzyme induced calcite precipitation (EICP) is another widely used method to produce calcium carbonate crystals that can bind sand particles as a dust suppressant (Hamdan and Kavazanjian, 2016; Sun et al., 2021). The urease enzyme from either microorganisms or plant seeds can decompose urea to form NH3 and CO2, further precipitating calcium carbonate in a calcium-rich environment. However, the big cost of urea and calcium source is a big obstacle to applying the urease-based calcite precipitation for field application. Furthermore, the NH3 emission is also a public concern on environmental pollution.
To minimize the cost and avoid NH3 emission, a method without the supply of substrate is promising. Thus, a more sustainable method using the microbial carbonization process via carbon-capturing bacteria was developed to overcome this problem. The carbon-capturing bacteria can adsorb and transform CO2 to generate carbonate ions under the enzymatic action of bacteria (Xu et al., 2021; Zhan et al., 2021). This is because the CO2 can be captured by the protein of carbonic anhydrase (CA), an endo-enzyme, in an aqueous solution, and forming HCO3− solution, which is 107 times more than that without the catalyzation of CA secreted by microorganisms (Xu et al., 2021). The HCO3− then combines with the soluble Ca2+ to generate calcium carbonate precipitation (Eqs. 1–3), as shown in Figure 1.
Recently, Xu et al. (2021) reported a method to consolidate dredger fill using Streptomyces microflavus, which was accomplished via the enzymatic action to capture and transform CO2, consequently forming calcium carbonate precipitations that strengthen the soil. The consolidated samples achieved the highest compressive strength up to 2.29 MPa. Although Zhan et al. (2016) have investigated the possibility to use Paenibacillus mucilaginosus to cement fugitive dust through the microbial carbonization process, the carbonization efficiency is low.
The feasibility of using Streptomyces microflavus for dust control is investigated in this technical note, which can accelerate the conversion of CO2 into HCO3− in an aqueous environment. The method for dust control and several parameters related to the consolidation performance using Streptomyces microflavus are discussed. These include the Shore hardness of the consolidated layer, the content of precipitates, and the microstructure of consolidated sand.
The carbon-capturing bacteria of Streptomyces microflavus (DSM 40070) adopted in this study was purchased from Leibniz Institute DSMZ-German Collection of Microorganisms and Cell Cultures GmbH. The growth culture of Streptomyces microflavus comprises 15 g/L of glucose, 10 g/L of yeast extract, and 5 g/L of peptone with an initial pH of 9 adjusted by 1 M of NaOH. The growth culture was put into a sterilization pot at 121°C for 25 min and then cooled to room temperature. Inoculation of the Streptomyces microflavus strain solution (4%) was carried out on a sterile operation platform, followed by shaking in an orbital shaker at 260 rpm for 72 h. The harvested bacteria were stored in a refrigerator at 4°C prior to use. The average concentration of CA was measured by the enzyme linked immunosorbent assay (ELISA) kit method (Xu et al., 2021) and determined as 0.0082 mg/L. The OD values of Streptomyces microflavus were 2.4 ± 0.1. The pH of the Streptomyces microflavus was about 5–6.
Petri dish (90 mm in diameter) was filled with 50 g of Ottawa sand with a mean grain size of D50 = 0.4 mm. The sand was evenly spread out to form a sand layer with a thickness of 0.5 cm. This sand layer had a void volume of 12 cm3. During treatment, a mixture of 15 ml of Streptomyces microflavus and 0.01 M of Ca(NO3)2 (volume ratio 1 : 1) was evenly sprayed on sand surface, followed by curing in the carbonation box with a 10% of carbon dioxide concentration. Treatment was repeated 1, 3, 5, and 7 times and each treatment was 24 h. After carbonization, all the sand samples were rinsed with tap water and oven-dried at 70°C for 48 h prior to hardness testing and calcium carbonate content measurement. A comparative study using Paenibacillus mucilaginosus (DSM 24461) was also conducted with the same cultivation and treatment process. All tests were conducted in duplicate.
A digital Shore hardness tester was used to determine the hardness of the consolidated sand layer. The calcium carbonate content was determined by the acid-washing and rinsing method (Cheng et al., 2013). For each test, hardness and calcium carbonate content were measured at least three times to obtain an average value.
The microstructure of all microbial carbonized sand samples was analyzed by Field Emission Scanning Electron Microscope (FESEM) (Zeiss EV050, United Kingdom). All samples were rinsed with tap water and oven-dried at 105°C for 24 h prior to the SEM analysis. Chemical composition of all samples was determined by powder X-ray diffraction analysis (5–80°) (XRD, Bruker D8-Advance, λ = 1.5406Å, Bruker Company, Karlsruhe, Germany).
The correlation between the hardness and the treatment times using different carbon-capturing bacteria species is presented in Figure 2. It can be seen that the hardness of all sand samples increased after the consolidation test using Streptomyces microflavus and Paenibacillus mucilaginosus. This was because the CO2 was adsorbed, transformed, and generated carbonate ions under the enzymatic action of both carbon-capturing bacteria. Consequently, the carbonate ions could react with soluble calcium and form calcium carbonate precipitation, bridging the loose sand particles together and forming a consolidated sand layer that has mechanical strength. A similar observation was made by Zhan et al. (2016).
More importantly, the hardness increased with the increase of treatment times, which was attributed to the higher calcium carbonate precipitation that was associated with the greater hardness as previous literature reported (DeJong et al., 2013; Mousavi et al., 2021). As shown in Figure 3, the average shore hardness of consolidated layer using Streptomyces microflavus and Ca(NO3)2 were 0.95, 10.70, 15.00, and 24.70 HD after 1, 3, 5, and 7 times of treatment, separately. In comparison, the samples treated by Paenibacillus mucilaginosus and Ca(NO3)2 had average shore hardness of 0.25, 4.42, 11.42, and 15.25 HD, respectively. The results suggested that the carbonization efficiency of Streptomyces microflavus improved 62% after 7 treatments compared to Paenibacillus mucilaginosus. This was probably due to the Paenibacillus mucilaginosus being characterized as a facultatively anaerobic bacterium (Liu and Lian, 2019). The 10% of carbon dioxide concentration inhibited the performance of CA from Paenibacillus mucilaginosus. Thus, the absorption rate of CO2 catalyzed by CA from Paenibacillus mucilaginosus was lower than Streptomyces microflavus in the carbonation box. The result suggests the consolidation layer strengthened by Streptomyces microflavus has prospective application like dust control that require certain mechanical properties. For example, the calcite-consolidation layer could cement fugitive dust and against wind-erosion with the hardness of 24.6 HD (Zhan et al., 2016).
FIGURE 3. Calcium carbonate content of consolidated sand samples prepared by spraying at different times.
The effect of treatment times on the calcium carbonate content using different carbon-capturing bacteria is shown in Figure 3. Increasing the number of sprays led to the increase in calcium carbonate content. The calcium carbonate content of sand samples treated by Streptomyces microflavus and Ca(NO3)2 system were varied from 1.06, 3.11, 4.67, and 5.58% corresponding to 1, 3, 5, and 7 times of treatments. In the Paenibacillus mucilaginosus and Ca(NO3)2 system, the calcium carbonate content were 1.19, 2.59, 3.40, and 4.26% after 1, 3, 5, and 7 times of treatment, which were lower than those obtained by Streptomyces microflavus and Ca(NO3)2 system. This also can be explained by the characteristic of anaerobic Paenibacillus mucilaginosus, which shows weaker carbonization efficiency than aerobic bacterium of Streptomyces microflavus at high CO2 level. The calcium carbonate contents results were in line with the previous hardness results, where the calcium carbonate content increased as the increase of the number of sprays, consequently contributed to the greater hardness. Therefore, the Streptomyces microflavus could better capture and transform CO2 than the Paenibacillus mucilaginosus to precipitate calcium carbonate crystals in the carbonization box.
The microstructure of precipitates induced from two types of carbon-capturing bacteria was analyzed by FESEM, as shown in Figures 4A,B, respectively. FESEM showed that the morphology of the mineralized sediments by Streptomyces microflavus was typical rhombohedral-shaped crystals with a particle size varied from 10–30 μm (Figure 4A), known as calcite (Reddy, 2013). This is the most stable polymorph of CaCO3 due to its lowest solubility (Van Paassen, 2009). Unlike the regular CaCO3 crystals precipitated by Streptomyces microflavus, the morphology of the Paenibacillus mucilaginosus precipitated CaCO3 was mainly spherical crystals with a single crystal size of 1–10 μm, (Figure 4B). The spherical shape of CaCO3 is consistent with the shape of vaterite (Chu et al., 2013), which can be transformed into a more stable polymorph of CaCO3, such as calcite, via the dissolution and reprecipitation process (Van Paassen, 2009). Although both shapes of CaCO3 crystals were able to connect sand particles and provide mechanical strength during the carbonization process, the Shore hardness of the consolidated layer using Streptomyces microflavus was higher than Paenibacillus mucilaginosus from previous results. This is because the shapes, sizes, and stabilities of CaCO3 precipitates are critical factors that control its engineering properties, similar observations were made by Al Qabany et al. (2012). For example, the crystal transformed by Streptomyces microflavus was stable calcite, while Paenibacillus mucilaginosus generated unstable vaterite. However, the final formation of crystals could undergo phase transformation under the influence of other parameters, such as temperature, treatment times, and concentration of cementation solution (Wang et al., 2019).
FIGURE 4. FESEM images of the microbial carbonized samples using (A) Streptomyces microflavus; (B) Paenibacillus mucilaginosus.
This paper presented a method to consolidate sand using Streptomyces microflavus for dust control. The proposed microbial carbonization method provided an effective, low-cost, environmentally friendly, and publicity acceptable method for dust control in the desert area, which could effectively accelerate the conversion of CO2 into HCO3− and drive biomineralization through microbial carbonization pathway. The following conclusions can be drawn from this study:
1) Under the enzymatic action of Streptomyces microflavus, CO2 was absorbed and transformed, consequently producing carbonate ions to mineralize calcium ions into calcium carbonate precipitation. In this process, sand was cemented and formed a consolidated sand layer, which had adequate mechanical strength for dust control.
2) The Shore hardness and calcium carbonate content were measured on the consolidated layer using Streptomyces microflavus, which had higher Shore hardness and calcium carbonate content than the samples treated by Paenibacillus mucilaginosus under the same treatment conditions. The comparison indicates that using Streptomyces microflavus is a more efficient and sustainable method for dust control.
3) The SEM images show effective cementation among sand grains by both carbon-capturing bacteria. The Streptomyces microflavus mainly precipitated rhombohedral-shaped calcite, which is the most stable polymorph than the Paenibacillus mucilaginosus generated unstable vaterite. This resulted in the variance of the engineering properties of the consolidated sand layer through the microbial carbonization process.
The raw data supporting the conclusion of this article will be made available by the authors, without undue reservation.
XY: Conceptualization, Methodology, Formal analysis, Investigation, Data curation, and Writing—original draft. QM: Methodology, Investigation, and Data curation. YY: Conceptualization, Methodology, Validation, Resources, Writing—review and editing, and Funding acquisition. WZ: Validation and Writing—review and editing. LC: Conceptualization, Validation, and Writing—review and editing.
The research described in this technical note was financially supported by the Opening Funds of Jiangsu Key Laboratory of Construction Materials of Southeast University (Grant No. CM2018-02) and the National Natural Science Foundation of China (Grant Nos. 51702238 and 52108300). Their financial support is gratefully acknowledged.
The authors declare that the research was conducted in the absence of any commercial or financial relationships that could be construed as a potential conflict of interest.
All claims expressed in this article are solely those of the authors and do not necessarily represent those of their affiliated organizations, or those of the publisher, the editors and the reviewers. Any product that may be evaluated in this article, or claim that may be made by its manufacturer, is not guaranteed or endorsed by the publisher.
Al Qabany, A., Soga, K., and Santamarina, C. (2012). Factors Affecting Efficiency of Microbially Induced Calcite Precipitation. J. Geotech. Geoenviron. Eng. 138 (8), 992–1001. doi:10.1061/(asce)gt.1943-5606.0000666
Benhelal, E., Zahedi, G., Shamsaei, E., and Bahadori, A. (2013). Global Strategies and Potentials to Curb CO2 Emissions in Cement Industry. J. Clean. Prod. 51, 142–161. doi:10.1016/j.jclepro.2012.10.049
Cheng, L., Cord-Ruwisch, R., and Shahin, M. A. (2013). Cementation of Sand Soil by Microbially Induced Calcite Precipitation at Various Degrees of Saturation. Can. Geotech. J. 50 (1), 81–90. doi:10.1139/cgj-2012-0023
Cheng, L., and Cord-Ruwisch, R. (2014). Upscaling Effects of Soil Improvement by Microbially Induced Calcite Precipitation by Surface Percolation. Geomicrobiology J. 31 (5), 396–406. doi:10.1080/01490451.2013.836579
Cheng, L. (2012). Innovative Ground Enhancement by Improved Microbially Induced CaCO3 Precipitation Technology. Murdoch University.
Chu, D. H., Vinoba, M., Bhagiyalakshmi, M., Hyun Baek, I., Nam, S. C., Yoon, Y., et al. (2013). CO2 Mineralization into Different Polymorphs of CaCO3 Using an aqueous-CO2 System. RSC Adv. 3 (44), 21722–21729. doi:10.1039/c3ra44007a
DeJong, J. T., Mortensen, B. M., Martinez, B. C., and Nelson, D. C. (2010). Bio-mediated Soil Improvement. Ecol. Eng. 36 (2), 197–210. doi:10.1016/j.ecoleng.2008.12.029
DeJong, J. T., Soga, K., Kavazanjian, E., Burns, S., Van Paassen, L. A., Al Qabany, A., et al. (2013). Biogeochemical Processes and Geotechnical Applications: Progress, Opportunities and Challenges. Géotechnique 63 (4), 287–301. doi:10.1680/geot.sip13.p.017
Grantz, D. A., Vaughn, D. L., Farber, R., Kim, B., Zeldin, M., VanCuren, T., et al. (1998). Seeding Native Plants to Restore Desert farmland and Mitigate Fugitive Dust and PM10. Wiley Online Library.
Hamdan, N., and Kavazanjian, E. (2016). Enzyme-induced Carbonate mineral Precipitation for Fugitive Dust Control. Géotechnique 66 (7), 546–555. doi:10.1680/jgeot.15.p.168
Harkes, M. P., Van Paassen, L. A., Booster, J. L., Whiffin, V. S., and van Loosdrecht, M. C. M. (2010). Fixation and Distribution of Bacterial Activity in Sand to Induce Carbonate Precipitation for Ground Reinforcement. Ecol. Eng. 36 (2), 112–117. doi:10.1016/j.ecoleng.2009.01.004
Liu, H., and Lian, B. (2019). Quantitative Evaluation of Different Fractions of Extracellular Polymeric Substances Derived from Paenibacillus Mucilaginosus against the Toxicity of Gold Ions. Colloids Surf. B: Biointerfaces 175, 195–201. doi:10.1016/j.colsurfb.2018.11.075
Lo, C.-Y., Tirkolaei, H. K., Hua, M., De Rosa, I. M., Carlson, L., Kavazanjian, E., et al. (2020). Durable and Ductile Double-Network Material for Dust Control. Geoderma 361, 114090. doi:10.1016/j.geoderma.2019.114090
Mousavi, F., Abdi, E., Ghalandarayeshi, S., and Page-Dumroese, D. S. (2021). Modeling Unconfined Compressive Strength of fine-grained Soils: Application of Pocket Penetrometer for Predicting Soil Strength. Catena 196, 104890. doi:10.1016/j.catena.2020.104890
Mujah, D., Shahin, M. A., and Cheng, L. (2017). State-of-the-art Review of Biocementation by Microbially Induced Calcite Precipitation (MICP) for Soil Stabilization. Geomicrobiology J. 34 (6), 524–537. doi:10.1080/01490451.2016.1225866
Raymond, A., Purdy, C., Fox, T., Kendall, A., DeJong, J., Kavazanjian, E., et al. (2019). Life Cycle Sustainability Assessment of Enzyme-Induced Carbonate Precipitation (EICP) for Fugitive Dust Control. Acad. J. Civil Eng. 37 (2), 600–607.
Reddy, M. S. (2013). Biomineralization of Calcium Carbonates and Their Engineered Applications: a Review. Front. Microbiol. 4, 314.
Ruan, S., and Unluer, C. (2017). Influence of Mix Design on the Carbonation, Mechanical Properties and Microstructure of Reactive MgO Cement-Based concrete. Cement and Concrete Composites 80, 104–114. doi:10.1016/j.cemconcomp.2017.03.004
Shi, G., Qi, J., Teng, G., Wang, Y., and Shen, H. (2021). Influence of Coal Properties on Dust Suppression Effect of Biological Dust Suppressant. Advanced Powder Technology.
Shi, G., Qi, J., Wang, Y., and Liu, S. (2021). Experimental Study on the Prevention of Coal Mine Dust with Biological Dust Suppressant. Powder Technology.
Sun, X., Miao, L., Wang, H., Yuan, J., and Fan, G. (2021). Enhanced Rainfall Erosion Durability of Enzymatically Induced Carbonate Precipitation for Dust Control, 791. Science of The Total Environment, 148369. doi:10.1016/j.scitotenv.2021.148369
Wang, Y., Soga, K., DeJong, J. T., and Kabla, A. J. (2019). Microscale Visualization of Microbial-Induced Calcium Carbonate Precipitation Processes. J. Geotech. Geoenviron. Eng. 145 (9), 04019045. doi:10.1061/(asce)gt.1943-5606.0002079
Whiffin, V. S. (2004). Microbial CaCO3 Precipitation for the Production of Biocement. Murdoch University.
Xu, Y., Yu, C., and Yu, X. (2021). Microbial Mineralization and Carbonation Consolidation of Dredger Fill and its Mechanical Properties. J. Mater. Civil Eng. 33 (7), 04021144. doi:10.1061/(asce)mt.1943-5533.0003769
Yu, X., Qian, C., and Jiang, J. (2019). Desert Sand Cemented by Bio-Magnesium Ammonium Phosphate Cement and its Microscopic Properties. Construction Building Mater. 200, 116–123. doi:10.1016/j.conbuildmat.2018.12.046
Yu, X., and Rong, H. (2022). Seawater Based MICP Cements Two/one-phase Cemented Sand Blocks. Appl. Ocean Res. 118, 102972. doi:10.1016/j.apor.2021.102972
Zhan, Q., Qian, C., and Yi, H. (2016). Microbial-induced Mineralization and Cementation of Fugitive Dust and Engineering Application. Construction Building Mater. 121, 437–444. doi:10.1016/j.conbuildmat.2016.06.016
Zhan, Q., Yu, X., Pan, Z., and Qian, C. (2021). Microbial-induced Synthesis of Calcite Based on Carbon Dioxide Capture and its Cementing Mechanism. J. Clean. Prod. 278, 123398. doi:10.1016/j.jclepro.2020.123398
Keywords: calcium carbonate precipitation, microbial carbonization, carboncapturing bacteria, dust control, shore hardness
Citation: Yu X, Meng Q, Yang Y, Zhang W and Cheng L (2022) Microstructural and Geomechanical Study on Microbial-Carbonized Sand Using Streptomyces Microflavus for Dust Control. Front. Earth Sci. 10:875010. doi: 10.3389/feart.2022.875010
Received: 13 February 2022; Accepted: 07 March 2022;
Published: 11 April 2022.
Edited by:
Chaojun Jia, Central South University, ChinaReviewed by:
Bai Siriguleng, Inner Mongolia University of Technology, ChinaCopyright © 2022 Yu, Meng, Yang, Zhang and Cheng. This is an open-access article distributed under the terms of the Creative Commons Attribution License (CC BY). The use, distribution or reproduction in other forums is permitted, provided the original author(s) and the copyright owner(s) are credited and that the original publication in this journal is cited, in accordance with accepted academic practice. No use, distribution or reproduction is permitted which does not comply with these terms.
*Correspondence: Yang Yang, eXl5b3VuZ0BjcXUuZWR1LmNu
Disclaimer: All claims expressed in this article are solely those of the authors and do not necessarily represent those of their affiliated organizations, or those of the publisher, the editors and the reviewers. Any product that may be evaluated in this article or claim that may be made by its manufacturer is not guaranteed or endorsed by the publisher.
Research integrity at Frontiers
Learn more about the work of our research integrity team to safeguard the quality of each article we publish.