Corrigendum: Thermo-microstratigraphy of shells reveals invisible fire use and possible cooking in the archaeological record
- Interdisciplinary Center for Archaeology and Evolution of Human Behaviour (ICArEHB), Universidade do Algarve, Faro, Portugal
The archaeological visibility of hearths related to shellfish cooking methods is limited, particularly in pre-ceramic shell midden contexts. Important evidence for use of fire is the thermal alteration of components, namely the identification of burnt shells. Mollusk shells that mineralize as aragonite are particularly indicative of burning due to the conversion of aragonite to calcite through recrystallization at known temperature thresholds. However, roasting temperatures needed to open bivalves, do not necessarily cause thermal alterations in the cooked shell. This complicates the significance of shell mineralogy by itself to recognize cooking, and discerning pre-depositional from in situ heating. To distinguish between cooking and burning, we combine micromorphological analyses with microscopic Fourier transformed infrared spectroscopy to investigate mineralogical thermo-alterations alongside microstratigraphic formation studies. Experimentally heated specimens of Cerastoderma edule and Scrobicularia plana are used to identify the temperature thresholds of biogenic calcium carbonate phase alteration at the micro-scale. These results are then used to interpret mineral alterations in deposits from two Mesolithic shell midden contexts from Portugal. Micro-stratigraphically controlled mineralogy proved to be particularly useful to distinguish between pre-depositional heating from in situ heating, configuring a novel methodology for recognition of traces of cooking shellfish versus traces of fire used for other purposes. Mapping the mineral phase conversion at a micro stratigraphic scale also allows us to identify instances of in situ fire events that were invisible macroscopically. This combined microstratigraphic and mineralogical methodology considerably increases our capacity of deciphering intricate shell midden stratigraphy and occupational events.
Introduction
Mollusk shells are common components in archaeological deposits and important indicators of paleoenvironment, coastal foraging ranges, and diet. While the use of shells to identify exploited resources and reconstruct coastal paleoenvironments in prehistory are relatively common (Mannino and Thomas, 2002; Mannino et al., 2007; Colonese et al., 2009; Andrus, 2011; Mannino et al., 2011; Prendergast et al., 2016; Canti, 2017; Prendergast and Schöne, 2017; García-Escárzaga et al., 2019), few studies have focused on how mollusks have been processed and potentially cooked in prehistoric settings, particularly in the absence of ceramic containers. This is relevant since most archaeological shells relate to dietary waste (Erlandson, 1988; Erlandson and Moss, 2001; Bailey and Milner, 2002; Craig et al., 2007; Diniz, 2016; García-Escárzaga and Gutiérrez-Zugasti, 2021). The evolution of cooking and associated pyrotechnology is a crucial behavioral trait that may vary in time and space (Wrangham and Conklin-Brittain, 2003; Wrangham and Carmody, 2010). Currently, to what extent fire was used to process shellfish in prehistoric times through roasting techniques is largely understudied.
Archaeological evidence for the use of fire relies on the recognition of combustion by-products and traces in the archaeological record. The identification of ashes, charcoal, burned components, and underlying thermally altered substrates (Mentzer, 2014; Aldeias et al., 2016; Goldberg et al., 2017; Mallol et al., 2017) are proxies for the presence of combustion. However, intact combustion features can be difficult to discern due to both post-depositional processes, such as dissolution, or preservation issues. This is particularly true for open-air shelly deposits where small components like ash can easily be blown away or migrate down the profiles due to the high level of sedimentary porosity (March et al., 2014). Furthermore, the construction of fires on top of sandy shelly deposits often do not present an indicative rubified substrate (Villagran, 2018; Aldeias et al., 2019). Another important aspect, however, is that fires used for shellfish roasting might not entail the preservation of intact combustion features–which are, at its simplest, an intact ash layer with charcoals and burned components overlying a baked substrate. This is because, as ethnographic records show, shellfish roasting fires frequently involving the removal of fire residues, since hearths can be done either above the shellfish or using rocks previously heated by fires (Meehan, 1975; Waselkov, 1987). In these cases, the ashes and charcoals are pushed aside to retrieve the thermally opened mollusks. Such activities entail the dismantlement of the combustion products, with the dispersion of ashes and charcoals, to retrieve the cooked mollusks, resulting in the loss of the stratigraphic signatures of intact hearths (Aldeias et al., 2019). Our ability to identify and differentiate these–function-related–syn-depositional disturbances from naturally reworked combustion residues is currently challenging. Even so, important evidence for use of fire is the thermal alteration of components, namely the identification of burnt shells. Mollusk shells that mineralize as aragonite are particularly indicative of burning due to the conversion of metastable biogenic aragonite to calcite through recrystallization at known temperature thresholds (Lécuyer, 1996; Pokroy et al., 2007; Parker et al., 2010; Toffolo, 2021).
Here we argue that burning and cooking should be differentiated not by simply identifying burned archaeological shells, but by assessing the associated burned components in their original sedimentary context. For this, we need to understand two related aspects: first, the identification of what are the traces left by roasting (not boiling) of shellfish in pre-ceramic contexts; second, the contextual association of burned shells and other components to differentiate the primary or secondary–potentially unintentional–burning of previously deposited shells.
This paper focuses on understanding burning in shells mineralized as aragonite. For that, we use experimentally heated specimens of Cerastoderma edule (common cockle) and Scrobicularia plana (peppery furrow) to discern biogenic calcium carbonate (CaCO3) phase alteration at the micro-scale. These results are then used to calibrate mineral alterations in deposits of two Mesolithic shell midden contexts from Portugal (Figure 1). Micromorphological analyses (Courty et al., 1989; Macphail and Goldberg, 2017; Nicosia and Stoops, 2017) of microfacies and their interpreted formation processes are combined with microscopic Fourier transformed infrared spectroscopy (microFTIR) (Goldberg and Berna, 2010; Berna, 2017) with twofold objectives: 1) to reconstruct the microstratigraphic histories of deposition in shell-rich contexts, and 2) to discern between microstratigraphic traces of fire for shellfish processing versus secondary, post-depositional, burning of shells, according to our expectations, synthesized in Table 1.
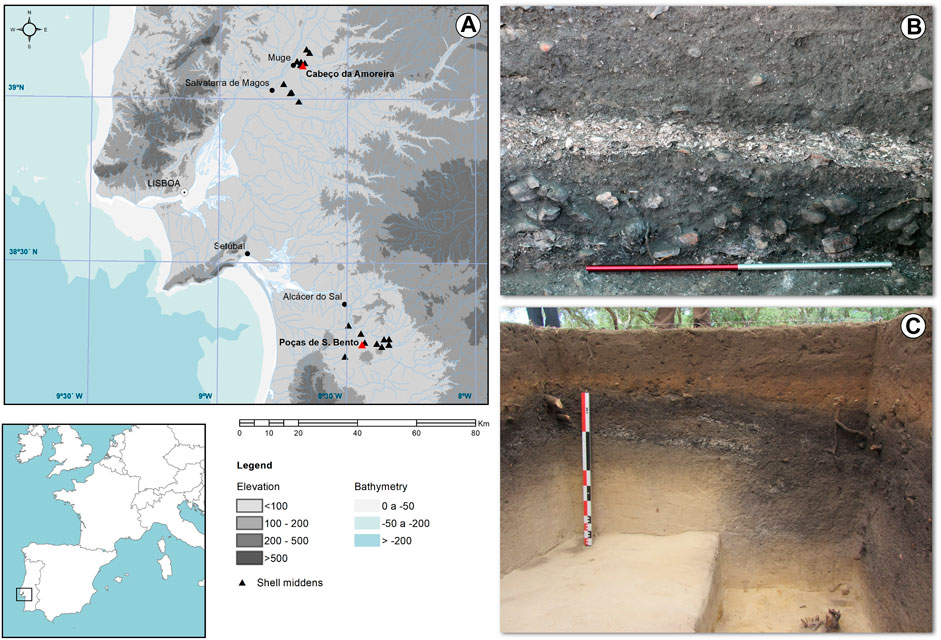
FIGURE 1. (A) Geographic location of the Mesolithic shell middens analyzed. (B) Aspect of the stratigraphy at Cabeço da Amoreira, in the Muge Valley. (C) Aspect of the stratigraphy at Poças de São Bento, in the Sado Valley.
Materials and methods
Experimentally heated shells
We used the collection of modern shells of C. edule and S. plana that were heated in a muffle at controlled temperatures by Aldeias et al. (2019). The shells were inserted into ceramic crucibles and heated at increasing temperatures from 100 to 900°C, for 5 and 20 m heating durations. Mineralogical analyses reported by Aldeias et al. (2019) were done by hand grinding a portion of the heated shells and obtaining infrared transmission and ATR spectra on powdered shell material. The collected results showed that calcitic phases start to appear at slightly different temperatures in each species: 200°C in C. edule and only at 350°C in S. plana. They also observed that the complete transformation of the original biogenic aragonite to calcite occurred at slightly different temperatures for each species: at 500°C for samples of C. edule and at 400°C for S. Plana (Aldeias et al., 2019).
In the present study, we further expand on this previous work by using microFTIR on thin sections produced from the same shells of C. edule and S. plana used by Aldeias et al. (2019) heated for 5 min at the threshold of the transformation interval previously observed, that is, at 250, 350, 400, and 450°C (Figure 2). Since the carbonate phase conversion in C. edule was shown to start at lower temperature than S. plana (Aldeias et al., 2019), two specimens of C. edule heated at 200°C were also used, though one of them was heated for 20 min, a difference that Aldeias et al. (2019) observed to have no effect on the FTIR-ATR results. Two specimens of each shell species were heated to enable double tests at each temperature (repetition one and repetition two in Figures 2, 3), resulting in 18 individual experimental shell samples used in this study (10 samples of C. edule and eight samples of S. plana) (Figures 2, 3). The experimental shells were impregnated with a polyester resin, cut along their growth axis, and produced into 2.7 × 4.6-cm thin sections by Spectrum Petrographics (Vancouver, United States).
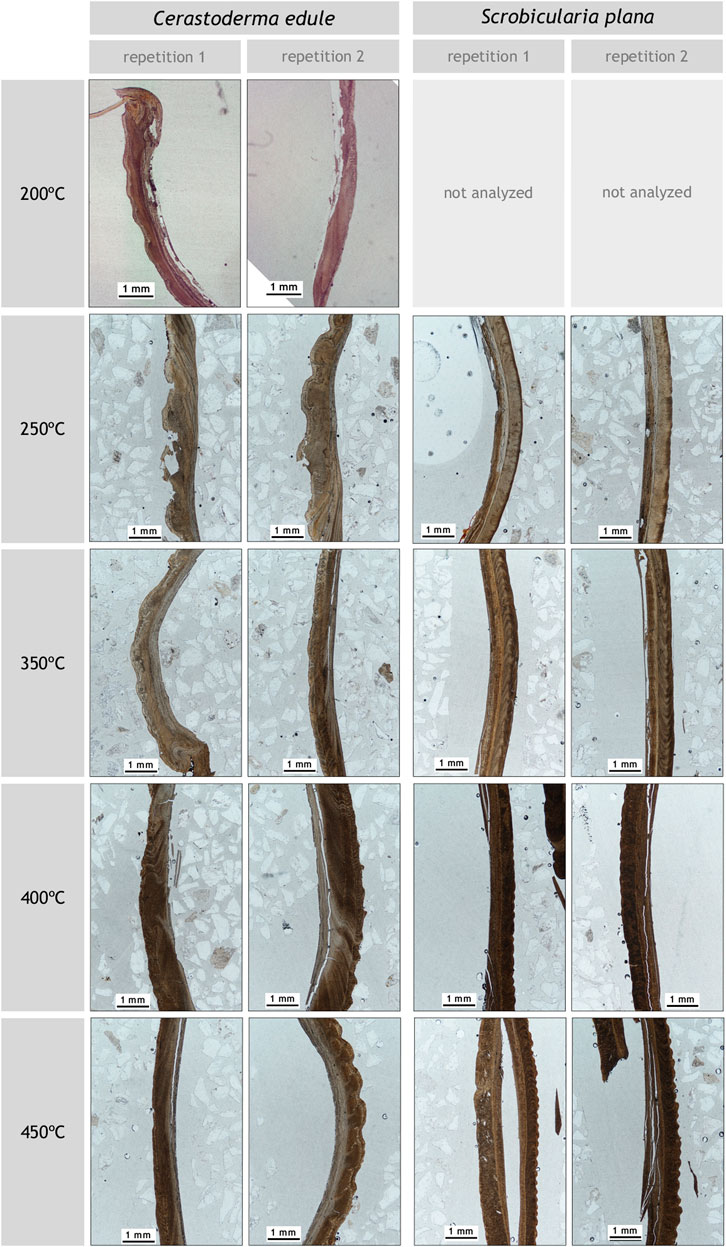
FIGURE 2. Petrographic images of the 18 thin experimentally heated shells used in this study, showing the observed effects of thermo-alteration: darkening and cracking increase with higher temperatures, though the crystalline structures are still visible.
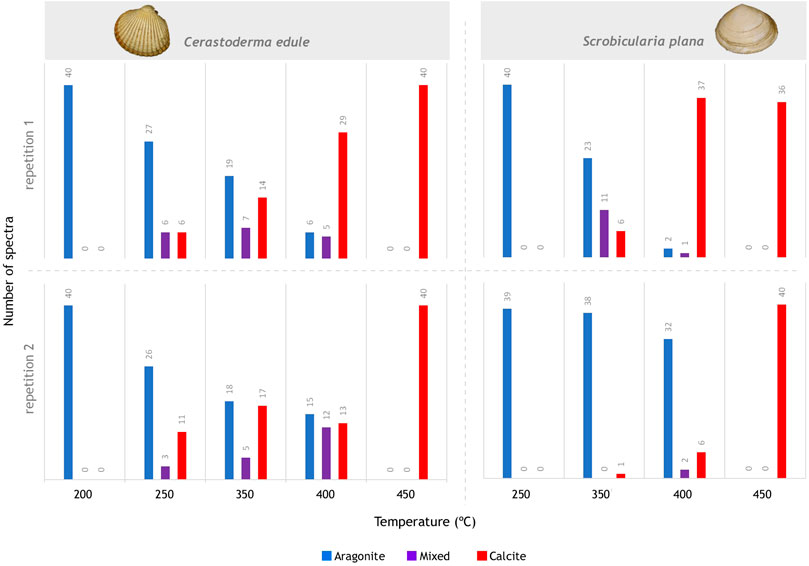
FIGURE 3. Aragonite-calcite conversion in the 18 experimentally heated shells; each individualized graph represents a single specimen of the two repetitions at each temperature; note the gradual conversion observed in Cerastoderma edule and the abrupt conversion in Scrobicularia plana. Shell species image credits: Natural History Museum Rotterdam, adapted from original file at WoRMS Database (https://www.marinespecies.org/index.php) under a CC BY-NC-SA license.
Archaeological thin sections from mesolithic shell middens
To assess the context of heated components in archaeological deposits, we investigate two Mesolithic shell midden sites from Portugal (Figure 1): Cabeço da Amoreira and Poças de São Bento. Both sites are among the largest shell middens in the Muge and the Sado valleys, respectively. These are two major paleoestuaries with brackish conditions formed during the Early Holocene sea-level rise (van der Schriek et al., 2007; Vis et al., 2008; Costa et al., 2019). The beginning of Mesolithic occupations of both the Muge and Sado valleys is characterized by the onset of human burials dated to 8,500–8,000 cal BP, in simple hollows opened in the sandy substrates of both sites (Bicho et al., 2010; Diniz and Arias, 2012; Bicho et al., 2013; Peyroteo-Stjerna, 2020; Arias et al., 2021). The following cumulative anthropogenic accretion of shelly deposits (and further human and animal inhumations) in several sites in the estuaries’ margins spanned until c. 7,400 cal BP, when the first Neolithic farmers were populating the surrounding regions (Bicho et al., 2017; Diniz et al., 2021). In the case of Cabeço da Amoreira and Poças de São Bento, pit features and putative post-holes at times cross-cut the complex interbedded shelly deposits.
Previous micromorphological studies have focused on reconstructing the depositional histories and the range of activities leading to the formation of these shell middens of Cabeço da Amoreira (Aldeias and Bicho, 2016) and at Poças de São Bento (Duarte et al., 2019). These studies stressed human-driven accumulations as the main agents of deposition, with the identification of three main types of deposits (sedimentary microfacies): 1) deposits in primary position, related to single events of direct shell tossing, 2) reworked shelly sediments resulting from mass reallocation of previously discarded debris, and 3) active layers related to more stable occupation surfaces. Table 2 describes the characteristics of the microfacies based on the micromorphological studies of those sites, alongside the indication of the thin sections that are used in the current paper.
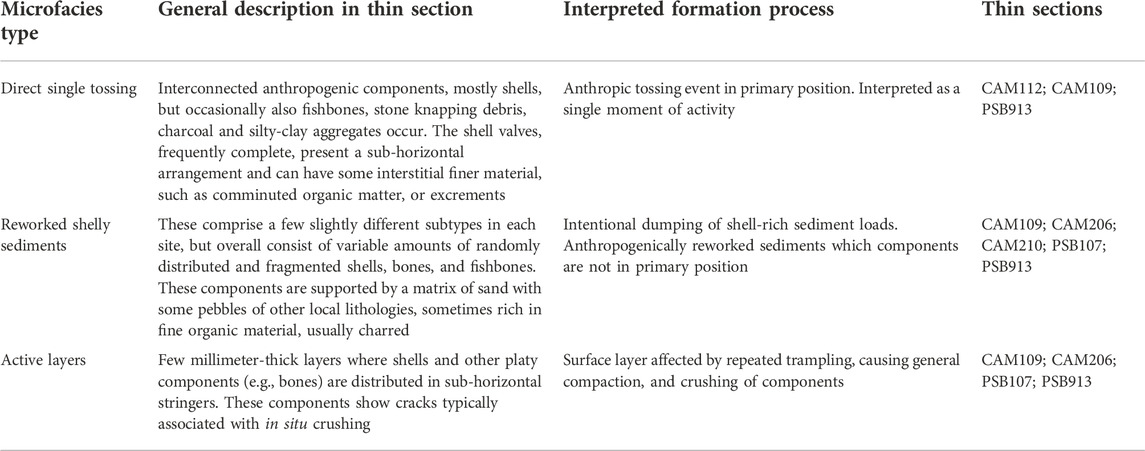
TABLE 2. Types of sedimentary microfacies identified in Cabeço da Amoreira and Poças de São Bento shell middens used in this work.
The experimentally heated shells can be directly compared to these archaeological sites since C. edule and S. plana are the predominant species at both sites. Micromorphological samples were collected at the two sites through the removal of undisturbed sediment blocks from the excavation profiles and carefully wrapped either with soft paper or with pre-plastered bandages. The blocks were then dried for several days and impregnated with a polyester resin mix. For Cabeço da Amoreira, large 5,7x13,5 cm thin sections were produced by the Servei de Micromofologia i Anàlisi d’Imatges at the University of Lleida (Spain). For Poças de São Bento, 5x7 cm thin sections were produced at the Departamento de Ciencia y Engenieria del Terreno y de los Materiales of the University of Cantabria (Santander, Spain). Thin sections from Cabeço da Amoreira are labelled CAM followed by an unique number identifier, whereas thin sections from Poças de São Bento bear the label PSB, also followed by an unique number identifier (e.g., CAM112 or PSB107). The microscopic observations were carried out under petrographic microscopes with plane- and cross-polarized light at magnifications ranging from 20x to 400x in plane polarized light (PPL) and cross-polarized light (XPL).
Microscopic fourier transform infrared spectroscopy mapping
MicroFTIR spectra were collected directly on the thin sections (of both experimental shells and archaeological micromorphology samples) using a Thermo Scientific Nicolet iN10 MX microscope. Spectra were collected in attenuated total reflectance (ATR) mode using a germanium crystal tip at a 4 cm−1 resolution in 256 and 64 scans, for experimental shells and archaeological ones, respectively, both within the 675–4,000 cm−1 range, and were compared with available libraries. The germanium tip has 150 x 150 µm diameter, though the measured area can be less than 150 µm depending on the degree of contact between the tip and the thin sections.
In the 18 samples of experimentally heated shells, a standard number of 40 individual measurements were collected in each shell (39 in one of them–see Figure 3), equally distributed in the shells’ thickness (Figure 4), resulting in 1,438 individual measurements (see Supplementary Material). In the archaeological thin sections, several mapping areas of 1 cm2 were selected throughout the slides’ surface (Figure 5) to analyze the shells that were visibly specimens of S. plana and C. edule. Depending on the preservation degree and size of the shell fragments, a variable number of measurements were placed in each shell within each map (Figure 5), in the most similar way possible as in the experimental shells, covering the specimens’ thickness along their section. For archaeological shells in six thin sections, a total of 9,038 individual measurements were collected (see Supplementary Material).
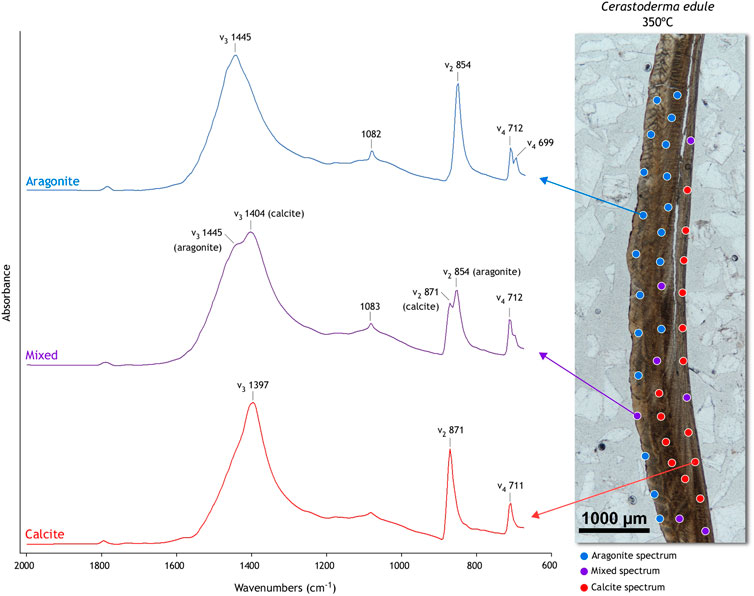
FIGURE 4. (A) Examples of microFTIR spectra of aragonite, calcite, and mixing both, obtained in experimentally heated specimen of Cerastoderma edule. (B) Petrographic microphotograph of the analyzed specimen with an example of the regular distribution of points to obtain microFTIR measurements; note the distribution of calcite spectra along the inner surface and aragonite spectra in the outer surface, showing a heterogenous conversion.
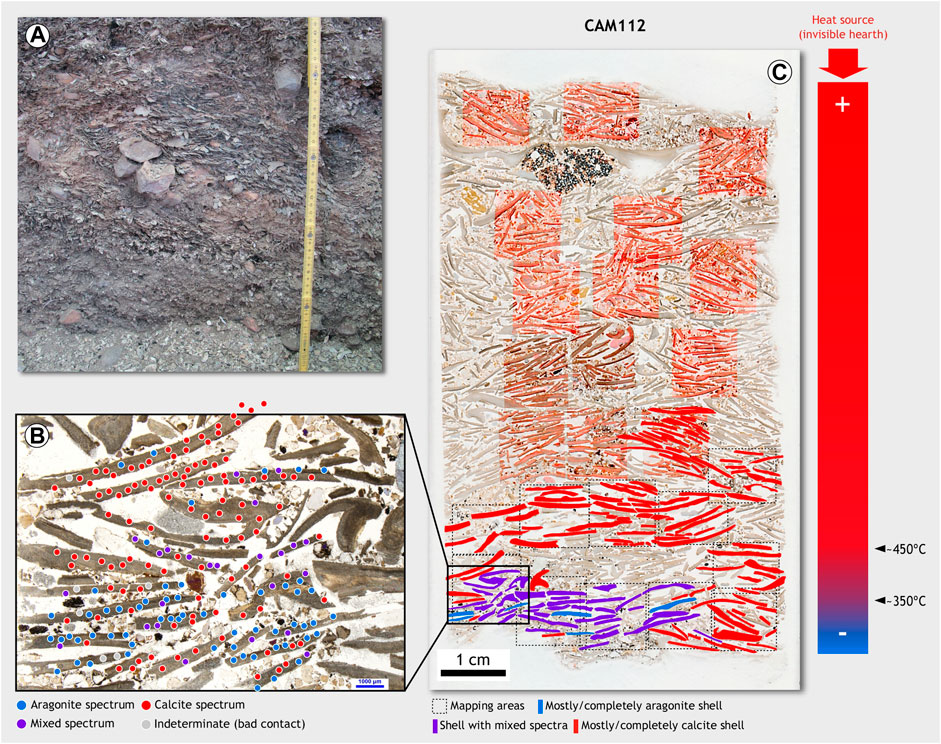
FIGURE 5. Sample CAM112. (A) Close-up of the field profile of the direct tossing deposits; note the absence of any evidence of structured combustion feature (B) Petrographic microphotograph showing an example of how the points to obtain FTIR measurements were distributed through the archaeological shells in the mapping areas; note the distribution of heated (calcitic) shells in the top of the image, while the shells in the bottom are not completely thermo-altered, with mainly aragonitic mineralogy, and the measurements of mixed mineralogy concentrate in the transitional area. (C) Thin section scan showing the mapping areas of ∼1 cm2 (dashed rectangles) and individual shells’ mineralogy obtained; the not-dashed rectangle indicates the location of the microphotograph A, and the rectangles with reddish filter indicate mappings where all shells are calcitic; the vertical bar to the right shows the approximate paleotemperature gradient experienced by the shells when subjected to post-depositional fire placed on top of the deposit, and invisible at the macroscopic scale.
Results
Experimentally heated shells
The microFTIR mapping on the oven-heated shells yielded the spectra correspondent to the CaCO3 polymorphs of biogenic aragonite and calcite (Figure 4). Biogenic aragonite spectra present the same peak position for shells heated above 200°C as reported by Aldeias et al. (2019) in ATR: the ν3 asymmetric stretch at 1,445 cm−1, the ν1 symmetric stretch at 1,082 cm−1, the ν4 peak at 856 cm−1, and the ν2 doublet at 712 and 700 cm−1. Calcite is represented by the ν3 peak at 1,397 cm−1, the ν4 peak at 871 cm−1, and the ν2 peak at 711 cm−1, in accordance with reference data (Weiner, 2010). A third type of spectrum exhibits mixture of both aragonite and calcite polymorphs in the specimens heated at the transitional temperature phases. In the mixed spectra, the peaks of both polymorphs in the same band overlap, usually with one appearing as a shoulder (Figure 4), depending on the relative proportions, as described elsewhere (Loftus et al., 2015; Toffolo et al., 2019).
We observed that the occurrence of all three types of spectra occur in a same shell during the mineral transformation temperature interval (Figure 3). The microFTIR mapping revealed a slightly different pattern between both species in the aragonite conversion to calcite. In S. plana the total conversion is faster since the shells remain mostly aragonitic at 250 and 350°C. At 400°C nearly all measurements provided calcite spectra, with only a few mixed ones, and exclusively calcite spectra at 450°C. In the case of C. edule, the conversion is more protracted, with mixed spectra already at 250°C, co-existence of aragonite and calcite spectra in similar proportions at 350 and 400°C, and only at 450°C all measured points correspond to calcite. These results generally match the trends identified by FTIR-ATR previously obtained by Aldeias et al. (2019).
Both shell species revealed similar petrographic transformations in terms of darkening and longitudinal fissures development with increasing temperature. At 250°C the shells are practically intact, and at 350°C the inner surface exhibits fissures along the different layers, that are progressively intensified at 400 and 450°C. In general, the effects of these alterations are more intense in S. plana than in C. edule. In Figure 2, it can be observed that at 450°C, C. edule exhibits only slightly darkened patches, following the carbonate growth lines, whereas S. plana is homogenously darkened, although the growth lines are still visible.
Archaeological thin sections
In CAM112 the areas mapped with micro-FTIR reveal that most shells are calcitic, except at the bottom, where they remain aragonitic or with a mixture of both polymorphs. This shift is progressive, and it is observed also within the mapping areas in the bottom of the thin section, where the totally calcitic shells are above, aragonitic shells are in the bottom, while mixed spectra occur in greater amounts the transitional middle area (Figure 5).
In CAM109, the bottom layer of single tossed shells reveals a totally aragonitic mineralogy in all mapping areas. In the overlying layer of reworked shells, the shells are predominantly aragonitic, except for rare random shell fragments that yielded mixed spectra and calcitic mineralogy, especially towards the top of the deposit. The microFTIR mapping in the active layer at the top of this thin section revealed also mainly aragonitic shells. Overall, in CAM109 the shells are not thermo-altered, being only the layer of reworked sediments that includes few thermo-altered shells (Figure 6).
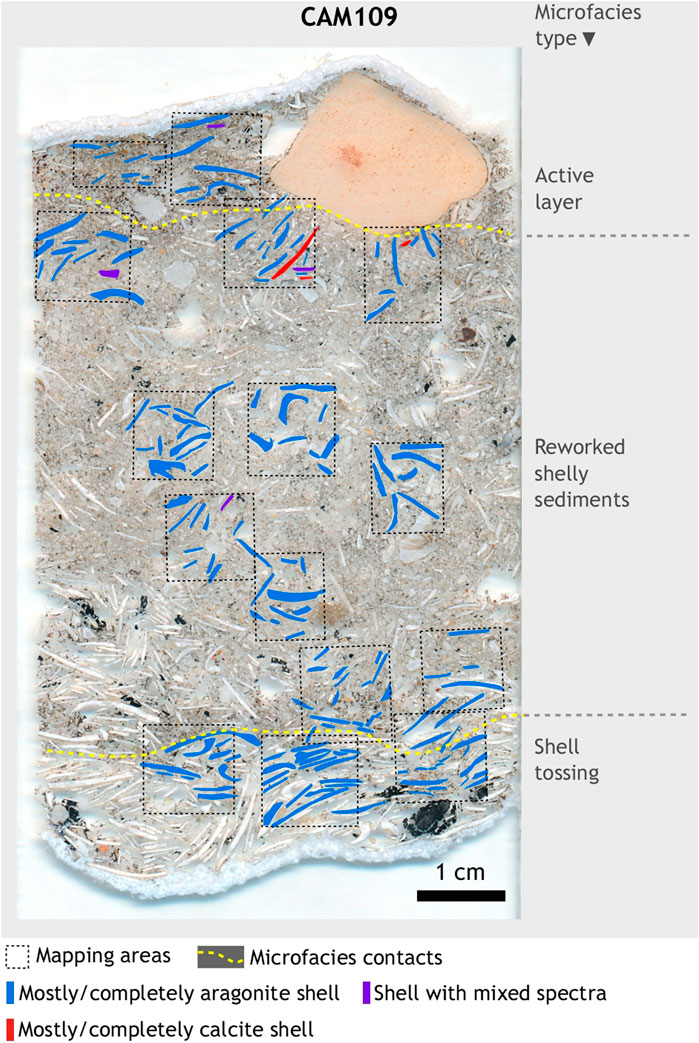
FIGURE 6. Thin section scan of sample CAM109 showing individual shells’ overall mineralogy obtained through microFTIR.
In contrast to CAM109, in the thin section CAM206, the mapped areas in the active layer revealed completely calcitic shells (Figure 7). In the reworked deposits both under- and overlying the active layer, the shells in the mapped areas exhibit similar proportions of all mineralogical spectra (Figure 7). Thin section CAM210, a sample of reworked shelly sediments, revealed mostly aragonitic shells with rare calcitic fragments (Figure 8).
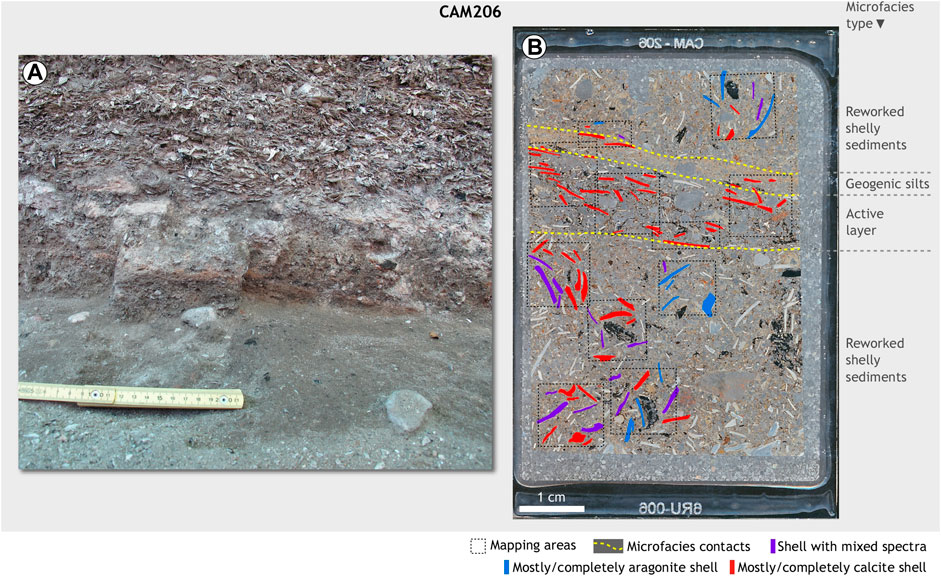
FIGURE 7. Sample CAM206: (A) Field photograph of the sample in profile, prior to block extraction. (B) Thin section scan of the samples showing individual shells’ overall mineralogy obtained through microFTIR.
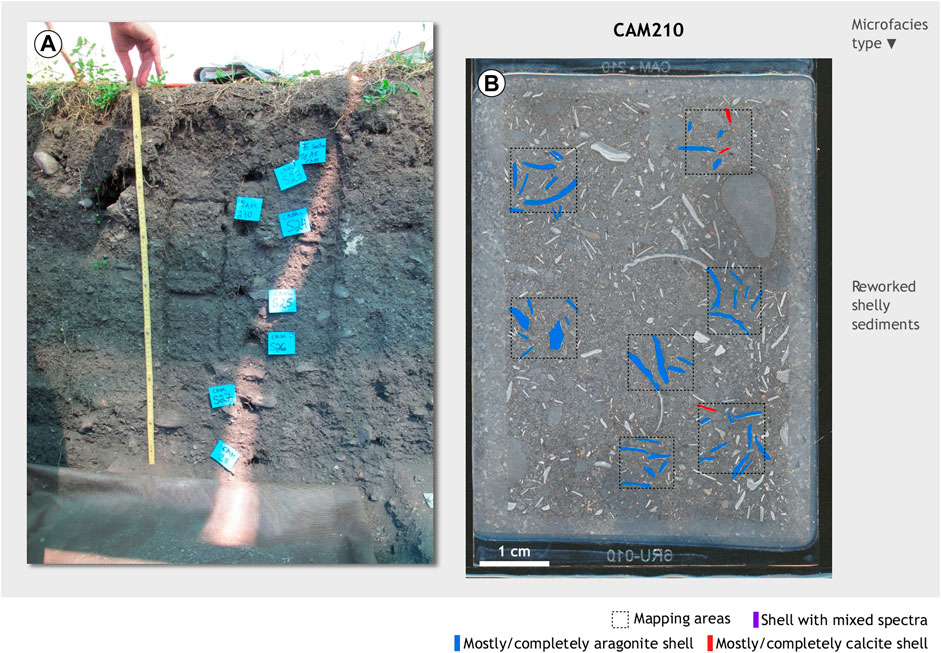
FIGURE 8. Sample CAM210: (A) Field photograph of the sample in profile, prior to block extraction. (B) Thin section scan of the samples showing individual shells’ overall mineralogy obtained through microFTIR.
The sample PSB107 mapping exhibits mostly calcitic shells in the active layer and a mix of all mineralogical spectra in the underlying reworked deposit, though aragonitic ones predominate (Figure 9).
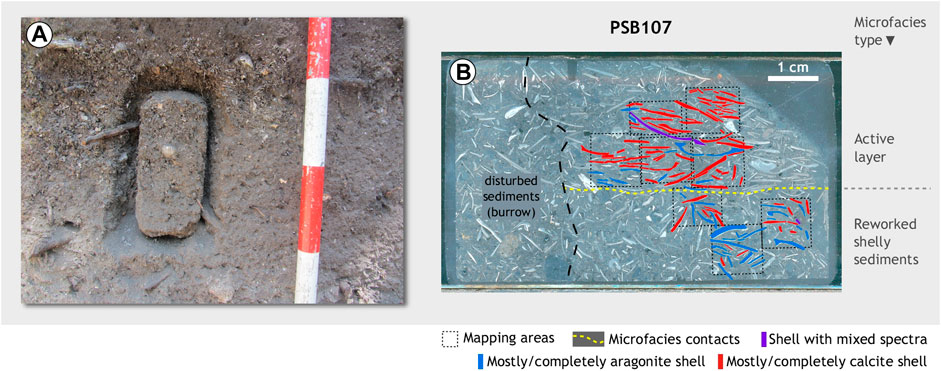
FIGURE 9. Sample PSB107. (A) Field photograph of the sample in profile, prior to block extraction; note the general homogeneity that the shelly deposit exhibits in the field, though different depositional and postdeposional events are revealed by microstratigraphy and mineralogy. (B) Thin section scan with individual shells’ overall mineralogy obtained through micro FTIR.
All of the thin sections produced from sample PSB913 provided a mainly aragonitic mineralogy of the shells in all represented layers (Figure 10). Rare fragments of calcitic shells were documented in the direct shell tossing deposit as well as in the reworked shelly sediments deposit (Figure 10).
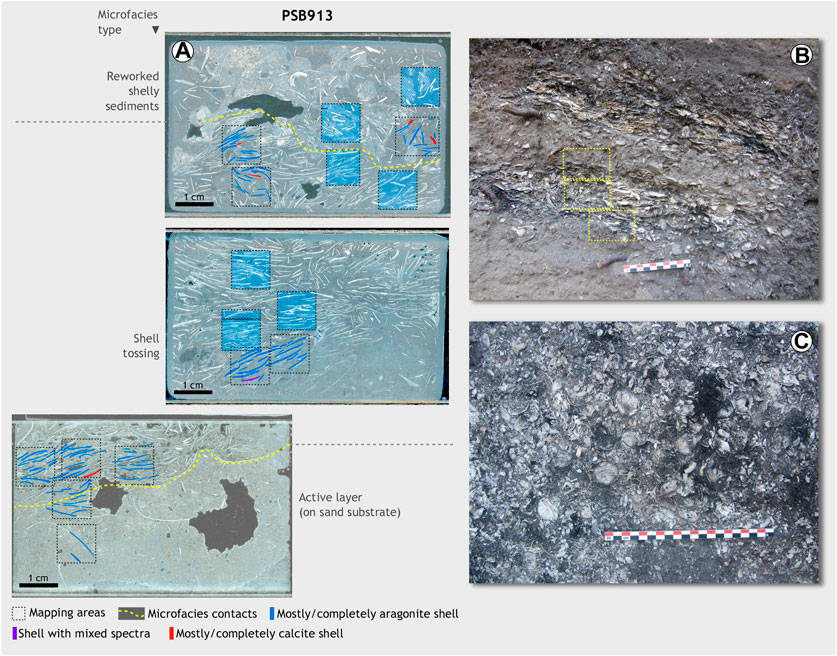
FIGURE 10. Sample PSB913. (A) Scans of three thin sections produced from the sample, showing individual shells’ overall mineralogy obtained through micro FTIR; rectangles with bluish filter indicate that all shells in the map are aragonitic. (B) Close-up of the field profile with the direct tossing deposits and reworked deposits interstratified; note the absence of heated substrate or any containment features for combustion; the dashed yellow rectangles indicated the approximate provenience of the thin sections. (C) Plane aspect of the direct tossing deposit interpreted as immediate discard of roasting residues; note the abundance of charcoal and the integrity of shells that in general revealed no mineralogical thermal alteration.
As it can be observed in Table 3, these results reveal very diverse situations for each type of sedimentary microfacies identified micromorphologically, with several meaningful implications for the circumstances in which the thermo-alteration of shells and their discarding are related in the shell midden formation.
Discussion
Burning of shells in the archaeological record does not exclusively equate to cooking, as it can occur simply because fire was used for any other purpose: for warmth, light, cooking or smoking of other food items besides shellfish. The construction of a fire on top of shelly deposits previously accumulated can also lead to (unintentional) thermal alteration of those shells (March et al., 2014). In such cases, the shells constitute previous debris unrelated to the fire event and not indicative of hearth use or function. One should be cautious, therefore, in relating shells burned at high temperatures (above 200°C) with shellfish cooking, since those higher temperatures imply the burning of the edible mollusk. How to recognize the intentional use of fire to cook shellfish has been challenging through analysis of thermal alterations of individual shells by themselves (Milano et al., 2016; Müller et al., 2017; Milano et al., 2018; Staudigel et al., 2019). Here we integrate the thermo-stratigraphic and contextual factors to build upon those previous attempts.
Mineral phase conversion and CaCO3 diagenesis
Like other bivalves, S. plana and C. edule are formed by two and three layers, respectively, composed of aragonite crystals that exhibit different crystalline microstructures and grow by precipitating incremental lines controlled by environmental conditions during the mollusk life (tides, temperature, stress) (Schöne, 2008). During shell growth, organic matter is also secreted, filling the space between the aragonite crystals and can represent 0.05–5% of weight (Weiner, 2010).
Milano et al. (2016) demonstrated a progressive microstructure expansion with increasing temperatures in C. edule, associated with the organic matrix loss. Heating is one of the possible causes for disappearance of mollusk shell’s organic matrix pointed out in the literature, which has been recorded at temperatures >300°C and a total replacement of aragonite to calcite at around 500°C (Milano et al., 2016; Milano and Nehrke, 2018). Our results overall agree with previous studies but show that the aragonite to calcite conversion starts at slightly different temperatures in the different species (Figure 3), namely at 250°C for C. edule and 350/400°C for S. plana. However, the temperature of complete mineralogical recrystallization of the shells was recorded at 450°C for both S. plana and C. edule.
Biogenic aragonite is known to reprecipitate as calcite in chemically unstable archaeological contexts (Weiner, 2010; Toffolo, 2021), but not always through recrystallization as it occurs upon heating. Meteoric water induces dissolution of biogenic aragonite and subsequent neoformation of calcite, which means a progressive loss of shell material, eventually until complete disappearance of the shell (Toffolo, 2021). This phenomenon is observed at the Mesolithic shell middens analyzed here by the existence of pendants composed of calcite spar in the underside of components (shells and pebbles) which most probable source are shells in upper layers that were dissolved (Aldeias and Bicho, 2016; Duarte et al., 2019). Previous studies on the transformation of biogenic aragonite into calcite suggest that diagenetic recrystallization observed in the geologic record is likely to be a much slower process than the timespan occurred at the Mesolithic archaeological contexts under study (Curtis and Krinsley, 1965; Brand, 1989; Ritter et al., 2017; Peacock et al., 2020; Toffolo, 2021). The presence of aragonitic shells in the older layers of the studied shell middens further indicates that the alteration from biogenic aragonite to calcite is not time dependent at these sites.
Another cause for aragonite-calcite recrystallization in shells is biological post-depositional activity induced by microorganisms that feed on the shells’ carbonate through micro-boring (James and Jones, 2015). These originate secretion of micritic calcite within micro-tunnels that, with time, replace entirely the skeletal clast microstructure. We can rule out this agent in our archaeological shells, since no micro-boring is observed in thin section, as shells preserve larger crystals in their different layers. Biologically induced calcite at both sites was observed too, in the form of alveolar septal and needle fabric cements hardening some layers, usually at the bottom of the shell mounds, where shells are still well preserved (Aldeias and Bicho, 2016; Duarte et al., 2019).
In our specimens experimentally heated between 250 and 450°C, microFTIR yielded aragonite, calcite and mixed spectra (Figure 3). The previous FTIR-ATR experiments by Aldeias et al. (2019) showed the existence of a mixed stage during the temperature threshold in powdered shell material. Here, the coexistence of areas where aragonite remains unchanged and others where aragonite was completely converted to calcite in the same shell was revealed by microFTIR mapping. This evidence shows that the aragonite to calcite conversion within a same shell can be heterogenous (Figures 3, 4). The reason for this is unknown, but we can tentatively suggest that it might be related to the different aragonite crystal microstructures that constitute each layer of these bivalves. Different crystalline microstructures had been demonstrated to react differently to temperature increase (Milano et al., 2016). Our experimentally heated shells showed that the complete calcite conversion starts from the inner surface and progressively expands to the outer surface in all shells of both species, as exemplified in Figure 4. This suggests a correlation with the different microstructures in the shell layers but needs future targeted research to be confirmed.
Experimental studies on infrared spectra with aragonite and calcite shows that mixtures of both polymorphs is discernable in the ν2 and the ν3 peaks of CaCO3 where the relative intensities correspond to the predominance of one over the other (Loftus et al., 2015; Toffolo et al., 2019). We observed spectra with these different relative peak intensities in our experimental heated shells (e.g., Figure 4), indicating variable proportions of both aragonite and calcite phases in the measured point in the shell. Therefore, the aragonite-calcite conversion by heating occurs heterogeneously and at different temperatures in each species. The coexistence of aragonite, mixed aragonite-calcite, and calcite spectra in the same shell, was also observed in the archaeological thin sections (Figure 5), a correlation that strongly suggests a thermal cause for their CaCO3 phase conversion.
Thus, we assume that the recrystallization in the archaeological shell middens analyzed here was not cause by diagenetic processes. This is further supported by the preservation of shell’s aragonite, which strongly indicates preservation of the original ontogenically precipitated aragonite, thus absence of major diagenesis (Weiner, 2010). Furthermore, a diagenetic cause for the conversion is incompatible with the existence of aragonitic and calcitic shells in deposits at different depths throughout the sites’ lateral stratigraphies. All these factors strongly indicate that the aragonite-calcite conversion in the archaeological shells analyzed here is caused by thermal alteration related to fire use by humans at the sites.
Tracing depositional histories through mineralogical alterations
Previous micromorphological analysis of these shell middens distinguished several different deposition modes that include: direct single-tossing events of shells, reworked load-dumped shelly deposits, and active layers interpreted as occupational surfaces (see Aldeias and Bicho (2016) and Duarte et al. (2019) for details). In this work, we add data on shell’s in situ mineralogical composition to the microfacies analysis.
Our results show that aragonitic shells predominate in microfacies related to reworked shelly deposits. These reworked deposits result, almost exclusive, from accumulation of S. plana specimens, thus indicating that most shells were not subjected to temperatures above 250°C. Therefore, it is more plausible to assume that most reworked deposits are composed by shells generally not subjected to high temperatures (i.e. above 250-350°C) before their deposition. Only in thin section CAM206 aragonite and calcite occur in similar proportions (Figure 7). In this case, about half of the measured shells were exposed to temperatures above 400°C prior to being reworked. The fact that heated shells are still in close association to each other, but not in their primary position, could be indicative of a low degree of mixing, or a reworking within smaller distances. The occurrence in the same microfacies of other components, namely large charcoals and pebbles (Figure 7), further supports this hypothesis, and is in contrast with the lack of charcoals associated with fully aragonitic shells in other reworked deposits analyzed. The fact that shells in CAM206 deposit are very fragmented can be a consequence of heating, which makes shells more brittle, and not necessarily intense reworking.
The microfacies interpreted as having functioned as surface active layers, thus subjected to physical pressure due to intense occupational activity and trampling, showed different cases of shell mineralogy. The shells within the active layer represented in CAM206 are fully calcitic and supported by a charcoal-rich matrix (Figure 7). In PSB107, the shells in a trampled layer are also fully calcitic (except rare aragonitic ones) (Figure 8), but here no charcoals are present in the matrix. These active layers, unlike reworked deposits, do not show mixing of aragonite and calcite polymorphs, indicating that heating in active layers might be syn-depositional, thus related to the occupation activities responsible for their formation. In turn, CAM109 and PSB913 represent occupational debris not affected by high temperatures, thus fire activity was more limited in its formation, as anticipated by the lack of other charred components.
Micro-stratigraphic shell mineralogy suggests that the trampled shell debris in CAM206 resulted from occupation activities involving fire, strong enough to burn shells, although not in situ, but resulting from short-distance dispersion originated by occupation actions such as trampling or sweeping. In sample PSB107, regardless of the microstratigraphic contact between the active layer and the reworked shelly deposit, the thermal alteration of the shells exhibits a gradual vertical pattern through that contact, better observed in CAM112, as discussed below.
Differentiating cooking from burning
Ethnographic accounts suggest that shellfish cooking does not require high temperatures or long exposures to fire, since the main goal is to open the valves and retrieve the eatable mollusk without burning it (Meehan, 1975; Waselkov, 1987). These sources describe that most techniques used for cooking shellfish imply roasting by simply exposing the shellfish to hot embers. The roasting experiments of Aldeias et al. (2019) basically generate aragonitic shells that experience temperatures always below 200°C, which agrees with other experiments (Müller et al., 2017). However, Aldeias et al. (2019) noticed some exceptions in the case of roasting with fire above or directly on embers. With these cooking methods, localized hot spots may entail the exposure of individual shells to temperatures higher than 200°C, producing spatially concentrated conversions to calcite. Still, most shells cooked this way remain aragonitic. Therefore, both ethnographic and experimental data point to low temperature exposure of shellfish, even when using roasting techniques. Thus, archaeological specimens cooked this way should retain their aragonitic structure. Archaeological assemblages of shells exposed to temperatures above 450°C, showing a complete conversion to calcite, are difficult to explain as resulting from cooking and require other explanations. In turn, this raises the question of how can we infer cooking shellfish in archaeological sites?
Contextual data from micromorphology helps in identifying deposits in primary position and is thus optimal to look for traces of activities that formed them. In our studied thin sections, examples of deposits in primary position consist in those formed by direct single tossing of shells and likely relate to the immediate discard of the shell right after the consumption of the mollusk. The mineralogy of tossing deposits analyzed by microFTIR mapping present twofold situations. In samples CAM109 and PSB913, shells within direct tossing deposits are fully aragonitic, with very residual calcitic ones in the latter (Figure 10). The bulk of these deposits are composed of S. plana specimens, with the retention of an aragonitic mineralogy clearly indicating that, when tossed away, the shells were probably not heated to temperatures higher than 350/400°C and definitely not above 450°C.
The deposit in sample CAM112 showed a completely different situation. Here, the mineralogical analysis revealed a gradient, with a progressive conversion of aragonitic to calcitic shells as we move upwards in the deposit (Figure 5). This suggests that the shells in the upper part of CAM112 were affected by homogenous heating at higher temperatures, that progressively decreased downwards. As this sample is composed almost entirely by S. plana, the palaeotemperature experienced by the shells in the deposit can be more precisely pinpointed (Figure 5). The mixed spectra of the lowest shells shows exposures to temperatures mainly below 350°C, with some shells heated to above 400°C. Above this area, all shells are calcitic (that is, heated to more than 450°C). This vertical gradient strongly suggests that the aragonite-calcite conversion occurred in situ. Since the integrity of the deposit has not changed, this evidence is better understood not as the result of cooking of that specific shellfish but interpreted as the effect of a later fire placed on top of the shell tossing deposit. Therefore, the sequence of events would have been an action of discarding shellfish followed by an unrelated fire activity in this locale. No significant charcoals are observed in CAM112, which supports the hypothesis of this deposit having functioned as a combustion substrate. A similar interpretation of burning–and not cooking–can be applied to the gradient conversion observed in PSB107 (Figure 9), where the burned shells were subsequently affected by trampling, causing structural compaction, and crushing.
Our expectations for prehistoric shellfish roasting in the archaeological record involve the presence of mainly aragonitic shells alongside combustion remains, such as abundant charcoals and ashes, which may not preserve the typical microstratigraphy of in situ fires (Table 1). A set of evidence such as this was identified in superimposed deposits represented in sample PSB913 (Figure 10). Spatially, this feature resembled an intact combustion feature, however, after micromorphological analysis, the components here were interpreted as related to direct single tossing event of abundant charcoals and well-preserved shells (Figure 10). The microFTIR mapping revealed fully aragonitic shells in these deposits, with residual exceptions. This led us to consider this context a result of immediate discard of debris directly from roasting activities. The occurrence of few shells that have experienced exceptionally higher temperatures due to localized hotspots occurring in roasting features confirms the expectation formulated in the experiments by Aldeias et al. (2016).
Implications for prehistoric shellfish cooking and archaeological site formation
In our approach, micromorphology coupled with microFTIR mapping allowed us to overcome two issues raised by previous works on shellfish cooking. The fact that aragonite-calcite conversion occurs above the burning threshold makes mineralogy by itself not suited to detect pre-depositional heating (Müller et al., 2017). Our investigation shows that, with contextual control and spatial and micro-stratigraphic precision, mineralogical alterations can be useful not only to infer pre-depositional heating, but also for distinguish it from in situ heating.
Staudigel et al. (2019) applied clumped isotopes to specifically distinguish boiling from roasting. They found that a “nonuniform distribution of ∆47 values between shell midden constituents suggests a nonuniform thermal history for shell midden constituents” (Staudigel et al., p. 4), in agreement with data obtained by Müller et al. (2017). Both studies conclude that this may be an effect of the technique used for cooking that consisted either in using only one of the valves or heating the entire bivalve from below, causing an uneven heating of valves, being the lower one significantly more affected than the top. Our results concur that boiling was unlikely and that roasting might have been a preferential cooking technique in the absence of hot stones or organic containers. This is suggested by the existence of some shells heated above 450°C, hence fully calcitic, among unheated shells and charcoals in the remains of interpreted roasting activity (Figure 10). With boiling, one would expect no mineralogical change at all in shells mineralogy (Milano et al., 2016; Milano et al., 2018). Staudigel et al. (2019) raise several effects specific of shell midden formation that hamper interpretation of pre-depositional heating, such as the discarding of uncooked shells, different thermal histories for different shells, or cooking techniques where heating is unevenly distributed. Our approach, by including micromorphological analysis that allow to study the thermal history of shells in their undisturbed archaeological context, considerably overcomes these limitations. We advocate for future integration of the contextual data from sedimentary microfacies to discern cooking from burning in the archaeological record.
Conclusion
Micro-stratigraphically controlled mineralogy proved to be particularly useful to distinguish between pre-depositional heating from in situ heating, configuring a novel methodology for recognition of traces of cooking shellfish versus traces of fire used for other purposes. One of the strengths of this approach was enabling the identification of in situ fire events that were invisible in the macroscopic archaeological record lacking structural features containing them, by mapping the mineral phase conversion stratigraphically. This advantage, combined with the potential of distinguishing between (primary and reworked) depositional events related to shelly sediments and post-depositional events related to fire use, considerably increases our capacity of deciphering intricate shell midden stratigraphy and occupational events.
Future work combining micromorphology and in situ mineralogy mapping would benefit from integrating other shellfish species and exploring contexts with evidence of in situ fires, such as features structured with pebbles, since ashes are rarely found in open-air shell middens and charcoals easily disperse. The combined micromorphological and mineralogical approach to originally aragonitic shells from such contexts would perhaps elucidate if the shells immediately below and above fire features are burnt or not, to infer pre-depositional heating of the combustion substrates. If it is only in the combustion-related layers that shells are burnt, it could perhaps relate to tossing shells to an active burning fire for other purposes than cooking them and correlate with the array of cultural activities documented in prehistoric shell middens. The application of the methodology we propose is not limited to shell middens, on the contrary, it has a high informative potential in any prehistoric context that contains shells mineralized as aragonite and subjected to burning to better characterize their depositional and pre-depositional thermal histories.
Data availability statement
The original contributions presented in the study are included in the article/Supplementary Material, further inquiries can be directed to the corresponding author.
Author contributions
VA designed the study, CS carried out the analysis and both equally contributed to data interpretation and writing the manuscript.
Funding
CS is currently funded by the European Union’s Horizon 2020 research and innovation programme through a MSCA Widening Fellowship under Grant Agreement nr. SEArch–101003409, and during the early stages of this research by a doctoral fellowship BES-2012-053695 granted by the Spanish Government. VA is funded by the Portuguese Fundação paraa Ciência e Tecnologia (FCT), grant numbers PTDC/HAR 20 ARQ/29606/2017 and IF/01157/2015/CP1308/CT0002. Research at Cabeço da Amoreira was funded by FCT projects PTDC/HAH/64185/2006) and PTDC/HIS-ARQ/112156/2009 awarded to Nuno Bicho (University of Algarve). Research at Poças de São Bento was funded by the Spanish Government grants HAR2011-29907-C03-00 and HAR2014-51830-P, both awarded to PabloArias (University of Cantabria) and by FCT through grant PTDC/HIS-ARQ/121592/2010 awarded to MarianaDiniz (Universidade de Lisboa). Open Access to this article is financed by FCT—Fundação para a Ciência e a Tecnologia, within the scope of the project ICArEHB UIDP/04211/2020 IHC PROGRAMATICO.
Acknowledgments
We wish to thank Nuno Bicho, Mariana Diniz and Pablo Arias, the directors of the field research at the archaeological sites where the samples used in this study were obtained, for allowing us to use the field data and for comments on the manuscript; Raquel Maria for the training and helping CS in the microFTIR preliminary tests, conducted at the Max Planck Institute for Evolutionary Anthropology; and José Paulo da Silva for support in technical issues with the FTIR microscope at Algarve Centre of Marine Sciences (CCMAR) used to obtain the data presented in the paper. We would also like to especially thank Shira Gur-Arieh, who was involved in the shell experiments reported previously, and the reviewers whose comments helped improving the manuscript.
Conflict of interest
The authors declare that the research was conducted in the absence of any commercial or financial relationships that could be construed as a potential conflict of interest.
Publisher’s note
All claims expressed in this article are solely those of the authors and do not necessarily represent those of their affiliated organizations, or those of the publisher, the editors and the reviewers. Any product that may be evaluated in this article, or claim that may be made by its manufacturer, is not guaranteed or endorsed by the publisher.
Supplementary material
The Supplementary Material for this article can be found online at: https://www.frontiersin.org/articles/10.3389/feart.2022.869487/full#supplementary-material
References
Aldeias, V., and Bicho, N. (2016). Embedded Behavior: Human Activities and the Construction of the Mesolithic Shellmound of Cabeço da Amoreira, Muge, Portugal. Geoarchaeology 31, 530–549. doi:10.1002/gea.21573
Aldeias, V., Dibble, H. L., Sandgathe, D., Goldberg, P., and Mcpherron, S. J. (2016). How heat alters underlying deposits and implications for archaeological fire features: A controlled experiment. J. Archaeol. Sci. 67, 64–79. doi:10.1016/j.jas.2016.01.016
Aldeias, V., Gur-Arieh, S., Maria, R., Monteiro, P., and Cura, P. (2019). Shell we cook it? An experimental approach to the microarchaeological record of shellfish roasting. Archaeol. Anthropol. Sci. 11, 389–407. doi:10.1007/s12520-016-0413-1
Andrus, C. F. T. (2011). Shell midden sclerochronology. Quat. Sci. Rev. 30, 2892–2905. doi:10.1016/j.quascirev.2011.07.016
Arias, P., Diniz, M., Araújo, A. C., Armendariz, Á., and Teira, L. C. (2021). “New perspectives on the mesolithic of the Sado valley (southern Portugal): Preliminary results of the SADO MESO project,” in Foraging assemblages volume 1. Serbian archaeological society, belgrade, republic of Serbia; the Italian academy for advanced studies in America. Editors D. Boric, D. Antonovic, and B. Mihailovic (New York, USA: Columbia University).
Bailey, G., and Milner, N. (2002). Coastal hunter-gatherers and social evolution: Marginal or central? Before farming 2002, 1–22. doi:10.3828/bfarm.2002.3-4.1
Berna, F. (2017). “FTIR microscopy”, in Archaeological Soil and Sediment Micromorphology. Editors C. Nicosia, and G. Stoops (Hoboken, Chichester, Oxford: Wiley Blackwell).
Bicho, N., Cascalheira, J., Gonçalves, C., Umbelino, C., Rivero, D. G., and André, L. (2017). Resilience, replacement and acculturation in the Mesolithic/Neolithic transition: The case of Muge, central Portugal. Quat. Int. 446, 31–42. doi:10.1016/j.quaint.2016.09.049
Bicho, N., Cascalheira, J., Marreiros, J., Gonçalves, C., Pereira, T., and Dias, R. (2013). Chronology of the Mesolithic occupation of the Muge valley, central Portugal: The case of Cabeço da Amoreira. Quat. Int. 308–309, 130–139. doi:10.1016/j.quaint.2012.10.049
Bicho, N., Umbelino, C., Detry, C., and Pereira, T. (2010). The emergence of Muge mesolithic shell middens in Central Portugal and the 8200 cal yr BP cold event. J. Isl. Coast. Archaeol. 5, 86–104. doi:10.1080/15564891003638184
Brand, U. (1989). Aragonite-calcite transformation based on Pennsylvanian molluscs. Geol. Soc. Am. Bull. 101, 377–390. doi:10.1130/0016-7606(1989)101<0377:actbop>2.3.co;2
Canti, M. G. (2017). “Burnt carbonates,” in Archaeological Soil and Sediment micromorphology. Editors C. Nicosia, and G. Stoops (Hoboken, Chichester, Oxford: Wiley Blackwell).
Colonese, A. C., Troelstra, S., Ziveri, P., Martini, F., Lo Vetro, D., and Tommasini, S. (2009). Mesolithic shellfish exploitation in SW Italy: Seasonal evidence from the oxygen isotopic composition of osilinus turbinatus shells. J. Archaeol. Sci. 36, 1935–1944. doi:10.1016/j.jas.2009.04.021
Costa, A. M., Freitas, M. D. C., Leira, M., Costas, S., Costa, P. J., Andrade, C., et al. (2019). The role of climate, marine influence and sedimentation rates in late-Holocene estuarine evolution (SW Portugal). Holocene 29, 622–632. doi:10.1177/0959683618824768
Courty, M., Goldberg, P., and Macphail, R. (1989). Soils and micromorphology in archaeology. Cambridge: Cambridge University Press.
Craig, O. E., Forster, M., Andersen, S. H., Koch, E., Crombé, P., Milner, N. J., et al. (2007). Molecular and isotopic demonstration of the processing of aquatic products in northern European prehistoric pottery. Archaeometry 49, 135–152. doi:10.1111/j.1475-4754.2007.00292.x
Curtis, C. D., and Krinsley, D. (1965). The detection of minor diagenetic alteration in shell material. Geochimica Cosmochimica Acta 29, 71–84. doi:10.1016/0016-7037(65)90118-3
Diniz, M., Arias Cabal, P., Araújo, A. C., and Peyroteo Stjerna, R. (2021). “Debating neolithization from a mesolithic point of view: The Sado valley (Portugal) experience,” in Foraging assemblages volume 2. Serbian archaeological society, belgrade, republic od Serbia; the Italian academy for advanced studies in America. Editors D. Boric, D. Antonovic, and B. Mihailovic (New York, USA: Columbia University).
Diniz, M. (2016). “Between land and sea: Assessing hunter-gatherer subsistence practices and cultural landscapes in southern Portugal during the final mesolithic,” in Archaeology of maritime hunter-gatherers. From settlement function to the organization of the coastal zone. Actes de la séance de la Société préhistorique française de Rennes, 10-11 avril 2014. Editors C. Dupont, and G. Marchand (Paris: Société préhistorique française).
Diniz, M. T., and Arias, P. (2012). “O povoamento humano do paleo-estuário do Sado (Portugal): Problemáticas em torno da ocupação dos concheiros mesolíticos,” in Environmental changes and human interaction along the Western Atlantic edge/Mundanças ambientais e interação humana na fachada atlântica ocidental. Editors A. C. Almedida, A. M. S. Bettencourt, D. Moura, S. Monteriro-Rodrigues, and M. I. C. Alves (Coimbra: APEQ-CITCEM-CEGOT-CGUP-CCT).
Duarte, C., Iriarte, E., Diniz, M., and Arias, P. (2019). The microstratigraphic record of human activities and formation processes at the Mesolithic shell midden of Poças de São Bento (Sado Valley, Portugal). Archaeol. Anthropol. Sci. 11, 483–509. doi:10.1007/s12520-017-0519-0
Erlandson, J. M., and Moss, M. L. (2001). Shellfish feeders, carrion eaters, and the archaeology of aquatic adaptations. Am. Antiq. 66, 413–432. doi:10.2307/2694242
Erlandson, J. M. (1988). The role of shellfish in prehistoric economies: A protein perspective. Am. Antiq. 53, 102–109. doi:10.2307/281156
García-Escárzaga, A., Gutiérrez-Zugasti, I., Cobo, A., Cuenca-Solana, D., Martín-Chivelet, J., Roberts, P., et al. (2019). Stable oxygen isotope analysis of Phorcus lineatus (da Costa, 1778) as a proxy for foraging seasonality during the Mesolithic in northern Iberia. Archaeol. Anthropol. Sci. 11, 5631–5644. doi:10.1007/s12520-019-00880-x
García-Escárzaga, A., and Gutiérrez-Zugasti, I. (2021). The role of shellfish in human subsistence during the Mesolithic of Atlantic Europe: An approach from meat yield estimations. Quat. Int. 584, 9–19. doi:10.1016/j.quaint.2020.03.003
Goldberg, P., and Berna, F. (2010). Micromorphology and context. Quat. Int. 214, 56–62. doi:10.1016/j.quaint.2009.10.023
Goldberg, P., Miller, C. E., and Mentzer, S. M. (2017). Recognizing fire in the paleolithic archaeological record. Curr. Anthropol. 0, S175–S190. doi:10.1086/692729
James, N. P., and Jones, B. (2015). Origin of carbonate sedimentary rocks. Oxford, Chichester, Hoboken: Wiley.
Lécuyer, C. (1996). Effects of heating on the geochemistry of biogenic carbonates. Chem. Geol. 129, 173–183. doi:10.1016/0009-2541(96)00005-8
Loftus, E., Rogers, K., and Lee-Thorp, J. (2015). A simple method to establish calcite: Aragonite ratios in archaeological mollusc shells. J. Quat. Sci. 30, 731–735. doi:10.1002/jqs.2819
Macphail, R. I., and Goldberg, P. (2017). Applied soils and micromorphology in archaeology. Cambridge: Cambridge University Press.
Mallol, C., Mentzer, S. M., and Miller, C. E. (2017). “Combustion features,” in Archaeological soil and sediment micromorphology. Editors C. Nicosia, and G. Stoops (Hoboken, Chichester, Oxford: Wiley Blackwell).
Mannino, M. A., and Thomas, K. D. (2002). Depletion of a resource? The impact of prehistoric human foraging on intertidal mollusc communities and its significance for human settlement, mobility and dispersal. World Archaeol. 33, 452–474. doi:10.1080/00438240120107477
Mannino, M. A., Thomas, K. D., Leng, M. J., di Salvo, R., and Richards, M. P. (2011). Stuck to the shore? Investigating prehistoric hunter-gatherer subsistence, mobility and territoriality in a mediterranean coastal landscape through isotope analyses on marine mollusc shell carbonates and human bone collagen. Quat. Int. 244, 88–104. doi:10.1016/j.quaint.2011.05.044
Mannino, M. A., Thomas, K. D., Leng, M. J., Piperno, M., Tusa, S., and Tagliacozzo, A. (2007). Marine resources in the mesolithic and neolithic at the grotta dell’uzzo (sicily): Evidence from isotope analyses of marine shells. Archaeometry 49, 117–133. doi:10.1111/j.1475-4754.2007.00291.x
March, R. J., Lucquin, A., Joly, D., Ferreri, J. C., and Muhieddine, M. (2014). Processes of formation and alteration of archaeological fire structures: Complexity viewed in the light of experimental approaches. J. Archaeol. Method Theory 21, 1–45. doi:10.1007/s10816-012-9134-7
Mentzer, S. M. (2014). Microarchaeological approaches to the identification and interpretation of combustion features in prehistoric archaeological sites. J. Archaeol. Method Theory 21, 616–668. doi:10.1007/s10816-012-9163-2
Milano, S., Lindauer, S., Prendergast, A. L., Hill, E. A., Hunt, C. O., Barker, G., et al. (2018). Mollusk carbonate thermal behaviour and its implications in understanding prehistoric fire events in shell middens. J. Archaeol. Sci. Rep. 20, 443–457. doi:10.1016/j.jasrep.2018.05.027
Milano, S., and Nehrke, G. (2018). Microstructures in relation to temperature-induced aragonite-to-calcite transformation in the marine gastropod Phorcus turbinatus. PLOS ONE 13, e0204577. doi:10.1371/journal.pone.0204577
Milano, S., Prendergast, A. L., and Schöne, B. R. (2016). Effects of cooking on mollusk shell structure and chemistry: Implications for archeology and paleoenvironmental reconstruction. J. Archaeol. Sci. Rep. 7, 14–26. doi:10.1016/j.jasrep.2016.03.045
Müller, P., Staudigel, P. T., Murray, S. T., Vernet, R., Barusseau, J.-P., Westphal, H., et al. (2017). Prehistoric cooking versus accurate palaeotemperature records in shell midden constituents. Sci. Rep. 7, 3555. doi:10.1038/s41598-017-03715-8
Nicosia, C., and Stoops, G. (2017). Archaeological Soil and Sediment Micromorphology. Hoboken, Chichester, Oxford: Wiley Blackwell.
Parker, J. E., Thompson, S. P., Lennie, A. R., Potter, J., and Tang, C. C. (2010). A study of the aragonite-calcite transformation using Raman spectroscopy, synchrotron powder diffraction and scanning electron microscopy. CrystEngComm 12, 1590. doi:10.1039/b921487a
Peacock, E., Mitchell, J., and Kirkland, B. (2020). Investigating freshwater mussel (Unionidae) shell diagenesis at an archaeological site on the Tombigbee River, Mississippi, southeastern U.S.A. J. Archaeol. Sci. Rep. 31, 102350. doi:10.1016/j.jasrep.2020.102350
Peyroteo-Stjerna, R. (2020). Chronology of the burial activity of the last hunter-gatherers in the southwestern Iberian Peninsula, Portugal. Radiocarbon 63, 265–299. doi:10.1017/rdc.2020.100
Pokroy, B., Fitch, A. N., and Zolotoyabko, E. (2007). Structure of Biogenic Aragonite (CaCO3). Cryst. Growth & Des. 7, 1580–1583. doi:10.1021/cg060842v
Prendergast, A. L., and Schöne, B. R. (2017). Oxygen isotopes from limpet shells: Implications for palaeothermometry and seasonal shellfish foraging studies in the Mediterranean. Palaeogeogr. Palaeoclimatol. Palaeoecol. 484, 33–47. doi:10.1016/j.palaeo.2017.03.007
Prendergast, A. L., Stevens, R. E., O'Connell, T. C., Fadlalak, A., Touati, M., Al-Mzeine, A., et al. (2016). Changing patterns of eastern Mediterranean shellfish exploitation in the Late Glacial and Early Holocene: Oxygen isotope evidence from gastropod in Epipaleolithic to Neolithic human occupation layers at the Haua Fteah cave, Libya. Quat. Int. 407, 80–93. doi:10.1016/j.quaint.2015.09.035
Ritter, A.-C., Mavromatis, V., Dietzel, M., Kwiecien, O., Wiethoff, F., Griesshaber, E., et al. (2017). Exploring the impact of diagenesis on (isotope) geochemical and microstructural alteration features in biogenic aragonite. Sedimentology 64, 1354–1380. doi:10.1111/sed.12356
Schöne, B. R. (2008). The curse of physiology—challenges and opportunities in the interpretation of geochemical data from mollusk shells. Geo-Mar. Lett. 28, 269–285. doi:10.1007/s00367-008-0114-6
Staudigel, P. T., Swart, P. K., Pourmand, A., Laguer-Díaz, C. A., and Pestle, W. J. (2019). Boiled or roasted? Bivalve cooking methods of early Puerto Ricans elucidated using clumped isotopes. Sci. Adv. 5, eaaw5447. doi:10.1126/sciadv.aaw5447
Toffolo, M. B., Regev, L., Dubernet, S., Lefrais, Y., and Boaretto, E. (2019). FTIR-Based Crystallinity Assessment of Aragonite–Calcite Mixtures in Archaeological Lime Binders Altered by Diagenesis. Minerals 9, 121. doi:10.3390/min9020121
Toffolo, M. B. (2021). The significance of aragonite in the interpretation of the microscopic archaeological record. Geoarchaeology 36, 149–169. doi:10.1002/gea.21816
van der Schriek, T., Passmore, D. G., Stevenson, A. C., and Rolao, J. (2007). The palaeogeography of Mesolithic settlement-subsistence and shell midden formation in the Muge valley, Lower Tagus Basin, Portugal. Holocene 17, 369–385. doi:10.1177/0959683607075839
Villagran, X. S. (2018). The Shell Midden Conundrum: Comparative Micromorphology of Shell-Matrix Sites from South America. J. Archaeol. Method Theory 26, 344–395. doi:10.1007/s10816-018-9374-2
Vis, G.-J., Kasse, C., and Vandenberghe, J. (2008). Late Pleistocene and Holocene palaeogeography of the Lower Tagus Valley (Portugal): effects of relative sea level, valley morphology and sediment supply. Quat. Sci. Rev. 27, 1682–1709. doi:10.1016/j.quascirev.2008.07.003
Waselkov, G. A. (1987). Shellfish Gathering and Shell Midden Archaeology. Adv. Archaeol. Method Theory 10, 93–210. doi:10.1016/b978-0-12-003110-8.50006-2
Weiner, S. (2010). Microarchaeology: Beyond the visible archaeological record. New York: Cambridge University Press.
Wrandham, R., and Conklin-Brittain, N. (2003). Cooking as a biological trait. Comp. Biochem. Physiology Part A Mol. Integr. Physiology 136, 35–46. doi:10.1016/s1095-6433(03)00020-5
Keywords: prehistoric cooking, shell midden, mesolithic, microstratigraphy, mineralogy, aragonite, calcite, palaeotemperature
Citation: Simões CD and Aldeias V (2022) Thermo-microstratigraphy of shells reveals invisible fire use and possible cooking in the archaeological record. Front. Earth Sci. 10:869487. doi: 10.3389/feart.2022.869487
Received: 04 February 2022; Accepted: 18 July 2022;
Published: 01 September 2022.
Edited by:
Manuel Will, University of Tübingen, GermanyReviewed by:
Emma Loftus, Ludwig Maximilian University of Munich, GermanyMatthew Canti, Historic England, United Kingdom
Copyright © 2022 Simões and Aldeias. This is an open-access article distributed under the terms of the Creative Commons Attribution License (CC BY). The use, distribution or reproduction in other forums is permitted, provided the original author(s) and the copyright owner(s) are credited and that the original publication in this journal is cited, in accordance with accepted academic practice. No use, distribution or reproduction is permitted which does not comply with these terms.
*Correspondence: Vera Aldeias, dmxhbGRlaWFzQHVhbGcucHQ=