- 1Oregon State University and NOAA Pacific Marine Laboratory, Hatfield Marine Science Center, Newport, OR, United States
- 2Bubbleology Research International, Goleta, CA, United States
- 3Ocean Networks Canada, University of Victoria, Victoria, BC, Canada
- 4Department of Marine Sciences, University of Georgia, Athens, GA, United States
Editorial on the Research Topic
Recent Advances in Natural Methane Seep and Gas Hydrate Systems
Marine hydrocarbon seep emissions of methane, oil, and other trace gases can profoundly impact the marine, regional, and global environment. Cold seeps arise from petroleum hydrocarbon fields (thermogenic), biogenic sources, and gas hydrates (thermogenic and biogenic). Deeply sourced, energy-laden fluids percolate through the sediment column, undergoing continual alteration (Figure 1). Seeps come in many flavors and varieties, ranging from highly active mud volcanoes to relict hardgrounds of authigenic carbonates (Joye, 2020). This global geological methane source is estimated to be 63–80 Tg CH4 year−1, with marine seepage contributing 20–30 Tg CH4 year−1 (Etiope et al., 2019) or 5–10 Tg CH4 year−1 (Saunois et al., 2020). An estimate of pre-industrial CH4 emissions—which are not confounded with fossil fuel production emissions - from ice core 14CH4 suggests an emission rate of 1.6 Tg CH4 year−1 (Hmiel et al., 2020). This lower estimate appears inconsistent with several recently published emissions from larger seep fields.
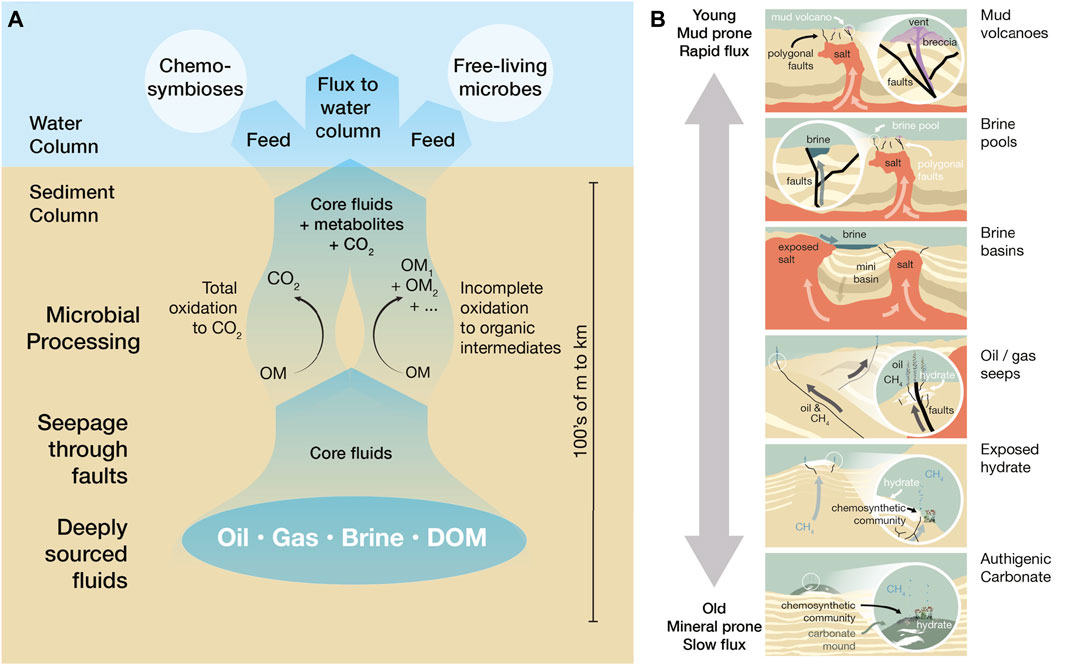
FIGURE 1. (A) The fluids that fuel hydrocarbon seeps fall into four categories: oil, gas, brine, and dissolved organic matter (DOM), and are arranged like this: oil + gas and oil + gas + brine + DOM. During transit, fluids are altered by biotic and abiotic processes. Upon discharge, these organic rich substrates fuel activity free-living and chemosymbiotic associations. (B) A range of seep environments from mud-prone systems—including mud volcanoes, brine pools, and brine basins—to oil and gas seeps with gas hydrate and low flow systems dominated by authigenic carbonates. Adapted from Joye (2020).
Underlying this debate is the paucity of quantitative studies, with challenges arising from the known spatial and temporal heterogeneity in seepage and the difficulty of doing seep science in some areas (e.g., the Arctic). Geological controls underlie these heterogeneities—particularly geological structures such as anticlines and faults that allow hydrocarbon accumulation and faults that allow migration to the seabed and atmosphere.
Another key question is what is the contribution of bioavailable chemosynthetic energy to higher trophic levels (Leifer et al., 2017). Methane seepage at the seafloor drives whole ecosystems through chemosynthetic primary production that is fueled by methane and sulfide oxidation and which induces authigenic carbonate formation (e.g., Levin et al., 2016). Although chemosynthetic production provides nutrition to a host of species at the bottom of the food chain, precipitated carbonate provides hardground for attachment and shelter. The extent of the seafloor and water-column ecosystem impacts of seepage remains unclear with further advances needed. It is timely to understand the full extent of seep ecosystem interactions as potential rises in their disturbance due to changes in the gas hydrate stability zone (GHSZ) become more common.
In this special issue, studies investigated the state and fate of methane from the sediments in the ocean and atmosphere (Becker et al., Chen et al., Grilli et al., Michel et al., and Meurer et al.). Others utilized seafloor mapping to quantify seepage and identify structural controls, and presented novel approaches (Ayoama and Maeda, Li et al., Merle et al., Riedel et al., Römer et al., and Vrolijk et al.). Several groups studied environmental, geochemical, and biological impacts (Sert et al., Yao et al.). Below we describe these studies.
In recent years, the awareness of cold seeps along active and passive margins has grown due to better scientific tools for locating and quantifying seepage and its signatures. This is well illustrated in Merle et al. who used multibeam sonar to survey the seep spatial distribution along the U.S. Cascadia Margin. This elevated the known number of Cascadian seeps significantly and provided insights into the seep distribution along the whole margin from its southern to northern boundaries and from the coast to the base of the accretionary prism. Ayoama and Maeda propose a methodology to quantify the amount of methane seepage by understanding the correlation between plumes and originating seeps. Vrolijk et al. developed a decision-making tool to assist in seep exploration and evaluation, providing a methodical query structure to identify the required information needed to find and characterize a seep site.
Marine cold seep methane flux quantification remains uncertain because the underlying geological controls are poorly understood. Riedel et al. advance the knowledge on emission controls by combining an acoustic multibeam water column, bathymetry, backscatter, and sub-bottom profiler data to determine linkages between sub-seafloor structures, seafloor gas seeps, and gas discharge into the water column. They also classify depositional, erosional, and tectonic factors as mainly responsible for gas emission control. Li et al. show an example of tectonic controls wherein multiple gas emissions were identified near the fault complex along the western slope of the Mid-Okinawa Trough. They provide new evidence of the role tectonic stresses play in determining the sites of seepage. Furthermore, changing GHSZ boundaries also controls seafloor gas seepage. These new findings underscore these deposits’ importance as a methane source with implications for habitat formation and energy sources. For example, Yao et al. present carbonate formation evidence through anaerobic methane oxidation during intense methane seepage likely associated with gas hydrate destabilization. Chen et al. shed light on the transition between gas hydrate and free gas occurrence and the zone where gas bubbles and hydrate crystals co-exist in the same aqueous solution. Becker et al. present 4 years of discrete and continuous temperature logging in an IODP hole on the Vancouver Margin accretionary prism that constrains possible pore-fluid flow in the prism associated with the bottom-simulating reflector and the base of the GHSZ.
Understanding methane’s water column fate, including whether seep-derived methane reaches the sea surface and atmosphere, has become a pressing issue. Meurer et al. use a glider equipped for simultaneous measurements of currents and methane concentration to determine the dissolved plume spatial distribution and to quantify water column methane. The study findings suggest biological seep impacts occur over longer distances than previously appreciated. Michel et al. and Grilli et al. demonstrate elevated methane concentrations throughout the whole water column at their study sites at the Cascadia Margin and the Western Black Sea and also observe areas of elevated dissolved methane concentrations at the surface, suggesting that these shallow seep sites contribute methane to the atmosphere. Similar observations were made by Römer et al. from shallow methane seepage related to salt diapirism in the German North Sea where bubble plumes reached close to the sea surface causing a slight methane oversaturation in surface waters, also indicating seabed seepage contributions to atmospheric methane inventories. Most of the methane dissolves into the shallow water column and provides bioavailable chemosynthetic energy. The extent of seep impacts on marine ecosystems remains poorly understood, addressed by Sert et al., who found that seep-related biogeochemical processes in Arctic seeps modify the composition of dissolved organic matter to higher diversity, but that distributions of nutrients, chlorophyll, and particulate matter were governed by the water-column hydrography and primary production.
These studies fill key knowledge gaps with respect to understanding the seep contribution to global methane budgets and thus climate change by introducing new survey tools, by identifying geological controls to enable more informed extrapolations, and by demonstration of conditions where transport to the atmosphere occurs. Unlike lower latitudes, the Arctic gas hydrates are sufficiently shallow to allow an atmospheric impact, with the magnitude of increases due to warming seabed and changes in the GHSZ very uncertain. Marine and terrestrial seepage currently are estimated to contribute ∼14% of the natural (non-regulatable) budget (Saunois et al., 2020). This estimate has significant uncertainty and likely will increase as seep emissions that are co-mingled with production in oil fields, which predate the fields and will persist after oil fields become uneconomic and are eventually abandoned, are reassigned from production. Seep emissions will also increase as Arctic warming destabilizes submerged permafrost and hydrates. First evidence is from the Barents Sea, where methane concentration growth, sea ice retreat, and warming are the fastest on the globe (Yurganov et al., 2016; Yurganov et al., 2021). However, Arctic seepage processes, from hydrate and subsea permafrost destabilization, to seasonal sea ice, to mixed-layer depth changes and the resulting impacts on seep methane transport to the atmosphere remain poorly characterized. Given that current global estimates are from a handful of snapshot emissions of a highly dynamic process and extrapolation to vast geological settings (Leifer, 2019), the potential for significant budget mis-estimation argues for many more quantified studies, particularly in the Arctic.
Author Contributions
The authors listed have made a substantial, direct, and intellectual contribution to the work and approved it for publication.
Conflict of Interest
Author IL is employed by Bubbleology Research International, which has no related commercial or financial interests that could be construed as a potential conflict of interest.
The remaining authors declare that the research was conducted in the absence of any commercial or financial relationships that could be construed as a potential conflict of interest.
Publisher’s Note
All claims expressed in this article are solely those of the authors and do not necessarily represent those of their affiliated organizations, or those of the publisher, the editors and the reviewers. Any product that may be evaluated in this article, or claim that may be made by its manufacturer, is not guaranteed or endorsed by the publisher.
Acknowledgments
The authors are immensely grateful to all the contributors and in particular the reviewers of the manuscripts, especially in light of the COVID-19 pandemic that suddenly emerged and impacted everyone and required additional patience with the entire process toward publication. This also extends to Frontiers Publishing who provided generous support and many necessary extensions of deadlines where required.
References
Etiope, G., Ciotoli, G., Schwietzke, S., and Schoell, M. (2019). Gridded Maps of Geological Methane Emissions and Their Isotopic Signature. Earth Syst. Sci. Data 11 (1), 1–22. doi:10.5194/essd-11-1-2019
Hmiel, B., Petrenko, V. V., Dyonisius, M. N., Buizert, C., Smith, A. M., Place, P. F., et al. (2020). Preindustrial 14CH4 Indicates Greater Anthropogenic Fossil CH4 Emissions. Nature 578 (7795), 409–412. doi:10.1038/s41586-020-1991-8
Joye, S. B. (2020). The Geology and Biogeochemistry of Hydrocarbon Seeps. Annu. Rev. Earth Planet. Sci. 48, 205–231. doi:10.1146/annurev-earth-063016-020052
Leifer, I., Judd, A., and Hildebrand, J. A. (2017). Life Aquatic Chemosynthetic in the Photic Zone -Up the Food Chain? Ocean. Fish. Open Access J. 4, 555636. Under Review. doi:10.19080/OFOAJ.2017.04.555636
Leifer, I. (2019). A Synthesis Review of Emissions and Fates for the Coal Oil Point marine Hydrocarbon Seep Field and California marine Seepage. Geofluids 2019, 1–48. doi:10.1155/2019/4724587
Levin, L. A., Baco, A. R., Bowden, D. A., Colaco, A., Cordes, E. E., Cunha, M. R., et al. (2016). Hydrothermal Vents and Methane Seeps: Rethinking the Sphere of Influence. Front. Mar. Sci. 3, 72. doi:10.3389/fmars.2016.00072
Saunois, M., Stavert, A. R., Poulter, B., Bousquet, P., Canadell, J. G., Jackson, R. B., et al. (2020). The Global Methane Budget 2000-2017. Earth Syst. Sci. Data 12 (3), 1561–1623. doi:10.5194/essd-2019-128
Yurganov, L. N., Leifer, I., Leifer, I., and Lund Myhre, C. (2016). Seasonal and Interannual Variability of Atmospheric Methane over Arctic Ocean from Satellite Data. Curr. Probl. Remote Sensing Earth Space 13, 107–119. doi:10.21046/2070-7401-2016-13-2-107-119
Keywords: methane seeps, gas hydrates, brine seeps, gas venting, petroleum, hydrocarbon seepage, chemosymbiotic seep environments
Citation: Baumberger T, Leifer I, Scherwath M and Joye S (2022) Editorial: Recent Advances in Natural Methane Seep and Gas Hydrate Systems. Front. Earth Sci. 10:868609. doi: 10.3389/feart.2022.868609
Received: 03 February 2022; Accepted: 25 March 2022;
Published: 27 April 2022.
Edited and reviewed by:
Timothy Ian Eglinton, ETH Zürich, SwitzerlandCopyright © 2022 Baumberger, Leifer, Scherwath and Joye. This is an open-access article distributed under the terms of the Creative Commons Attribution License (CC BY). The use, distribution or reproduction in other forums is permitted, provided the original author(s) and the copyright owner(s) are credited and that the original publication in this journal is cited, in accordance with accepted academic practice. No use, distribution or reproduction is permitted which does not comply with these terms.
*Correspondence: Martin Scherwath, bXNjaGVyd2FAdXZpYy5jYQ==