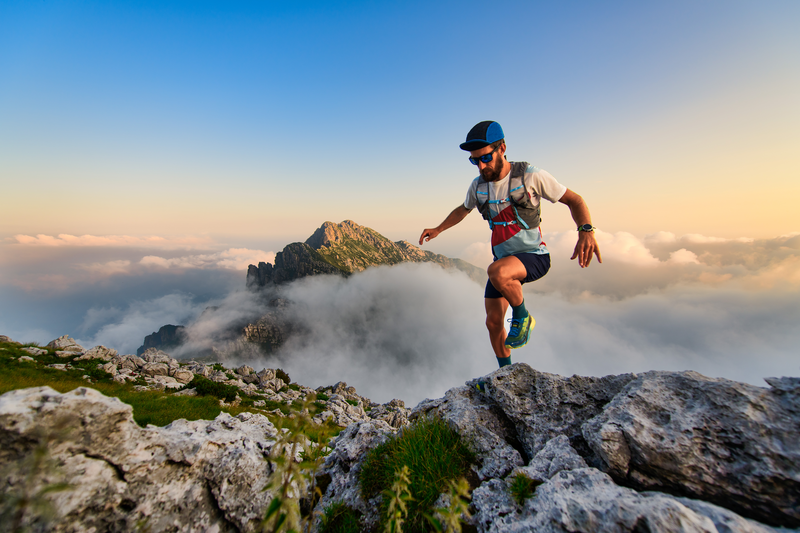
94% of researchers rate our articles as excellent or good
Learn more about the work of our research integrity team to safeguard the quality of each article we publish.
Find out more
ORIGINAL RESEARCH article
Front. Earth Sci. , 07 March 2022
Sec. Geohazards and Georisks
Volume 10 - 2022 | https://doi.org/10.3389/feart.2022.863357
This article is part of the Research Topic Advances in Modeling, Assessment, and Prevention of Geotechnical and Geological Disasters View all 44 articles
In order to study the influence of microbial content on the engineering properties of expansive soil im-proved by microbially induced calcium carbonate (MICP) method, and to clarify its influence law and mechanism of action, and to provide some theoretical reference for the application of MICP method to expansive soil treatment. Microbial reproduction and their mineralization in expansive soils have been studied. The problems such as moisture content controlling and low calcium content in the process of treating expansive soil with MICP method have been solved by means of adding calcium salt and using optimum moisture content as the control standard of the total amount of bacterial solution and cementation solution. The tests such as compaction, swelling rates and triaxial shear are carried out. The hydrophilicity, compaction, expansibility and strength characteristics of improved expansive soil under different microbial content are analyzed. The results demonstrate that with the increasing of microbial content, the dry density and shear strength of the improved soil are first increased significantly and then tend to be stable gradually. At the same time, the hydrophilicity and expansibility of improved soil are all decreased significantly. The microstructures of improved expansive soil are imaged by scanning electron microscope. Analytic results show that soil particles have been cemented and the pores in the soil have been filled due to microbially induced calcite precipitation. As a result, microstructure characteristics of the soil have been improved. From the microscopic point of view, the improvement mechanism of MICP method on expansive soil has been revealed. The conclusions above laid a theoretical foundation for in-tensive study on the improvement of expansive soil by MICP method. It also provides a new idea for perfecting and expanding the engineering application of expansive soil improvement technology.
Expansive soil is a special kind of soft clay with obvious swelling and shrinking properties, multiple fissures and superconsolidation which is extremely widely distributed in China (Xu et al., 2017). Montmorillonite and illite are the two highest proportion of clay minerals in expansive soil, are extremely water absorbent. As a consequence, with changes in water content, significant swelling and shrinkage and generates a large number of fissures are exhibits in soil, which results in a significant reduction in strength when the soil is exposed to water. Especially under extreme weather conditions, the moisture content of the soil is changed with the changing of ambient humidity. While the repeated cycles of moisture absorption–drying occur, the engineering properties of the expansive soil are deteriorated gradually, and the strength is decreased significantly and the deformation is increased, which seriously affects engineering safety (Liu et al., 2020a).
For a long time, domestic and foreign scholars have conducted a lot of research on the engineering properties of improved expansive soils, and many research results have been also obtained. Traditional methods for improving expansive soil are classed into physical improvement and chemical improvement. (Fu et al., 2019; Bian 2016, Bian 2020; Elkady and Shaker, 2018). studied the feasibility of improving expansive soil with lime through expansiveness and water stability experiments. (Wu et al., 2021a; Wu et al., 2021b). mixed steel slag powder into expansive soil, which effectively improved the expansion and shrinkage properties of expansive soil. (Guo et al., 2020). carried out a composite improvement of expansive soil with iron tailing sand-calcium carbide slag. Direct shear tests are conducted to determine the optimum slag mixing rate and optimum sand mixing rate for the improving expansive soil. (Shafiqu and Hasan, 2018). used polymethacrylate (PMA) polymer to improve expansive soils and the optimum admixture of PMA is determined through tests of compaction, swelling rate and unconfined compressive strength. (Wu et al., 2020). mixed calcium lignosulfonate (calcium wood) into expansive soils, it is found that the porosity of the expansive soils can be reduced by calcium wood and the water stability of this soil is also improved. Therefore, the poor engineering properties of expansive soil can be improved with above methods. However, among these improved methods, not only the environment will be caused greater pollution by using the materials such as lime and PMA polymer, but also these improved methods are expensive and a long construction period is needed. Thus, seeking a more economical, eco-friendly and efficient method for improving expansive soil is an urgent problem to be solved in the academic and engineering circles.
Microbially Induced Calcite Precipitation (MICP) is a kind of microbial mineralization process ubiquitous in nature. In the MICP method, high-producing alkaline urease bacteria are uesd to promote the hydrolysis of urea and to interact with calcium sources provided by the outside or some metal ions in the soil (Bai et al., 2017; Bai et al., 2019), calcium carbonate (calcite) precipitation by mineralization is produced. Microbes act as nucleation nodes of calcium carbonate and calcium carbonate crystals are induced the aggregation (Sun et al., 2021; Liu et al., 2019). The main process of MICP method is to mixing the soil with a bacterial solution and a cementation solution (calcium source). In the presence of the above two liquids, calcium carbonate suspended particles are adhered to the surface of soil particles or are penetrated into the pores of soil particles with the flow of liquid (Bai et al., 2020; Bai et al. 2021a; Bai et al. 2021b). In recent years, MICP method has been become a new green technology that is widely used in crack repairing (Yuan et al., 2020; He et al., 2019; Liu et al., 2020b), concrete self-healing (Feng et al., 2021; Bai et al., 2021c), heavy metal pollutant repairing (Lu et al., 2021; Xu et al., 2020), and wind protection and sand fixation (Mwandira et al., 2019; Li et al., 2020) and other engineering fields. (Cheng et al., 2021). applied MICP method to the modification of loess, it is found that the strength of this improved soil has been increased significantly. (Cai et al., 2020). explored the action mechanism of MICP method to cure silty soils, and the maintenance days needed and nutrient solution concentration are determined. (Liang et al., 2020). used MICP method to improve sandy soils, and eggshells are creatively used as a calcium source for the mineralisation process in MICP method. (Hao et al., 2021). improved the weathered sand based on MICP method, and the cementation of calcium carbonate produced in the mineralisation reaction are confirmed through scanning electron microscopy.
Considering the economy and environmental protection for engineering construction, MICP method is used to improve expansive soil, which has broad engineering application prospects. However, there is less literature related MICP method is used to improve expansive soil. Therefore, based on the current studies (Qin et al., 2020; Jiang et al., 2021; Li et al., 2021; Yao et al., 2021; Yu et al., 2021; Yu et al. 2021; Yu et al., 2022), the effect of different microbial contents on the mechanical properties, expansibility and shrinkage of expansive soil improved by MICP method has been investigated. The influence mechanism of MICP method on the microstructure of improved expansive soil has been revealed through the testing of scanning electron microscope imaging.
The soil samples used in the test are taken from the Huancheng road project in Nanning, Guangxi, these are mainly composed of clay minerals such as montmorillonite, illite and kaolinite. The soil is shown scaly, predominantly yellowish and off-white and has a slippery and gritty feel to the touch. The physical properties of expansive soil are shown in Table 1.
The microorganism used in the experiment is Bacillus pasteurii (ATCC1185-9), acquired from the China General Microbial Strain Conservation and Management Centre (CGMCC). This bacterium is a nonpathogenic Gram isolated from natural soil. It has a strong ability to produce urease (Whiffin 2004) and is widely used in related research on the improvement of soft soil by the MICP method. Before the test, the bacteria are inoculated into the slant medium. After activated and expanded, the cultured bacteria liquid is obtained. Then, the bacterial liquid is put into a constant temperature intelligent precision shaker with a rotation speed of 150 r/min and a temperature of 30°C for about 36 h. Finally, a spectrophotometer is used to determine the concentration of the bacterial solution. When the absorbance OD600 = 1, the concentration of the bacterial solution is considered to meet the test requirements. The absorbance OD600 values of the bacterial solutions used during the test are kept at 1.
The main role of the cementation solution is to provide a sufficient source of calcium and nitrogen for bacterial mineralisation and to provide a source of energy for the metabolism of microorganisms. In order to prevent the higher chloride ion concentration from inhibiting the activity of urease and affecting the improvement effect of the MICP method on the expansive soil, a lower concentration of the cementation solution was used in the experiment, generally 0.2 mol/L (Kejun et al., 2019). The mixing ratio of the cementation solution is 978.4 ml of distilled water, 43.8 g of CaCl2.6H2O crystals, and 43.8 g of urea.
Expansive soil sample retrieved is dried and crushed first, and then passed through a 2 mm sieve. In order to achieve the maximum compaction of the soil sample and to ensure sufficient nutrient solution for bacterial growth, the optimum moisture content ω = 16.2% corresponding to the maximum compaction is used as the control standard for the total amount of cementing solution and bacterial solution. The cementation solution and the bacterial solution are sprayed into the soil sample for 2, 4, 6, and 8 different times and them are mixed well at every time. At the same time, unimproved expansive soil with the same moisture content is configured as a control group to compare the improvement effects of expansive soil.
In order to ascertain the influence of microbial content on the engineering properties of improved expansive soils, according to the “Standard for Geotechnical Test Methods” (GBT50123-2019), tests for swelling rate and triaxial compression are carried out on different improved expansive soils. Improved expansive soils are air-dried at different treatment times and approximately 5 kg of each of the air-dried soil samples are taken for the preparation of compaction soil samples. For improved expansive soil with different treatment times, two parallel test groups have been made. The air-dried soil samples are crushed and through a 0.5 mm sieve, then a free swelling rate test is performed. For both the unloaded swelling rate and the 100 kPa loaded swelling rate tests, the optimal moisture content ω = 16.2% is used as the control standard for the moisture content of the soil samples.
Specimens of improved soil and unimproved soil with a height of 80 mm, a diameter of 39.1 mm are prepared for triaxial compression test. To ensure the accuracy of the test, three parallel samples are taken for each group of tests. Soil samples are put in the vacuum saturation apparatus to saturate for 12 h. Then they are put into the GDS triaxial instrument to saturate again under the action of negative pressure. Finally, soil samples are subjected to consolidation and drainage shear tests at a rate of 0.04 mm/min under a confining pressure of 50 kPa, 100 kPa, 200 kPa and 300 kPa respectively. According to the requirements of the test specification, when the axial strain is reached to 15%, the specimen is considered damaged and the test can be terminated.
For explore the influence of MICP method on microstructure changes of soil samples, using JSM-IT500LV scanning electron microscope and JEC-3000FC ion sputtering instrument, scanning electron microscopy imaging tests are carried out for unimproved expansive soil and six times treated improved expansive soil. Finally, soil sample has been imaged by scanning electron microscope.
According to the Geotechnical Test Method Standard (GBT50123-2019), compaction tests for improved expansive soil have been carried out under standard conditions, optimal moisture content and maximum dry density of expansive soil with different treatment times have been obtained. Tested results by compaction test of improved expansive soil, as shown in Figure 1.
It can be found from Figure 1 that the optimal moisture content of unimproved expansive soil is 16.2% and its maximum dry density is 1.94 g/cm3. With the increasing of the number of MICP treatment (microbial content), the optimal moisture content of expansive soils first is increased and then is decreased. This is due to the fact that microbially induced calcium carbonate precipitation firstly are cemented with soil particles, soil particles are changed to bigger. Secondly, soil pores are filled with calcium carbonate precipitation, which led to soil pore structure has been changed. The permeability of soil is reduced and its compactness is increased, which led to its optimal moisture content is increased. In addition, due to the action of charge force, calcium carbonate precipitates are adsorbed and are wrapped on the surface of the clay minerals, the hydrophilicity of soil has been reduced. Therefore, the optimal moisture content required to achieve the same density is increase inevitably.
It can also be seen from Figure 1 that the maximum dry density of expansive soil is tends to decrease and then to increase with the increasing of the number of MICP treatment. The maximum dry density is decreased from 1.94 g/cm3 to 1.84 g/cm3 and then is started to increase again. Combined with the analysis of the microstructure of expansive soils improved by MICP method, it is clear that calcium carbonate precipitation is increased with the increasing of treatment times. So that soil particles are changed to bigger and the frictional resistance between soil particles is increased. At the same time, with the increasing of soil particle diameters, the volume of soil pore is increased slightly. As a result, it becomes more difficult to compact soil sample, making it somewhat dry density is reduced. However, as the number of improvements continues to increase, the greater the content of calcium carbonate precipitation, more soil pores are filled by calcium carbonate precipitation, soil porosity is decreases gradually, and soil compactness is increased. As a result, the maximum dry density of soil is increased gradually instead.
According to the Geotechnical Test Method Standard (GBT50123-2019), the free swelling rate, unloaded swelling rate and 100 kPa loaded swelling rate tests have been carried out on the soil samples. Tested results of swelling ratios of improved soil samples, as shown in Figure 2.
As can be seen from Figure 2, free swelling rate, unloaded swelling rate and 100 kPa loaded swelling rate are decreased gradually with the increasing of times of MICP treatments increases (the microbial content increases). When soil is treated by microbial liquid for more than 6 times, the above three indexes all are closed to a certain fixed value. Compared with unimproved expansive soil, when treatment times of microbial liquid is 6, free swelling rate of improved soil sample is reduced from 61.3 to 14.9%, unloaded swelling rate is reduced from 14.2 to 2.80%, and 100 kPa loaded swelling rate is decreased from 1.8 to 0.4%. This shows that the expansibility of expansive soil can be suppressed effectively by MICP method.
From the changes in the microstructure of improved expansive soil, it can be considered that microbially induced calcite precipitation is existed in the form of spherical particles or spherical aggregates of calcite. It is adhered on the surface of soil particles, so that the frictional resistance between soil particles is increased, and the contact area between expansive soil particles and water is reduced, and the hydrophilicity of expansive soil is reduced, and the expansibility of expansive soil is inhibited. At the same time, water film in the soil is become thinner significantly, swelling potential of expansive soil is reduced, due to the ions between the highly-priced calcium ions in cementation solution and expansive soil are exchanged (Ma et al., 2019). With constantly increasing of improvement times, the total amounts of calcium carbonate precipitation are increased, which resulting in soil particles sizes and soil pore ratio all are increased constantly. Therefore, when the improvement times is exceeded 6, the hydrophilicity of expansive soil is tended to be stable, and the three expansibility indexes are all close to stable values. This means when the improvement times is exceeded 6, increasing the improvement number will no longer have a significant effect on reducing the expansibility of expansive soil.
The relations between deviatoric stress and axial strain of unmodified expansive soil and MICP method improved expansive soil have been obtained through triaxial consolidation and drainage shear tests. The relations between deviatoric stress and axial strain of expansive soil with different improvement times, as shown in Figure 3 respectively.
From Figure 3, it can be found when confining pressure is 50 kPa, 100 kPa, 200 kPa, and 300 kPa respectively, no significant peak is occurred in deviator stress (q = σ1−σ3) of improved expansive soil. With the increasing of axial strain, the increase rate of deviatoric stress is decreased gradually, and finally it is tended to be stable. It is reflected that its stress-strain relations is a typical strain-hardening type. Under the same confining pressure, with the increasing of treatment number by microbial, when soil sample is sheared failure the deviatoric stress value (ε1 = 15%) is increased gradually, that is, its shear strength is increased gradually. Taking confining pressure σ3 = 100 kPa as an example, comparing with unimproved expansive soil samples, the failure deviatoric stress of improved soil samples after it is treated by MICP method for 2, 4, 6, and 8 times is increased by 59.76%, respectively. 79.19, 109.85 and 76.2% respectively.
At the same time, it can be found from Figure 3 that the axial strain
Through the test of scanning electron microscope imaging, the microstructure of expansive soil improved by MICP method can be visual analysis. Scanning electron microscope imaging of expansive soil is shown in Figure 4.
It can be found from Figure 4A that in unimproved expansive soil, the spatial distribution of soil particles is in sheet structure, and the particles are mainly connected in the form of face-to-face connection. According to the lattice expansion theory (Hensen and Smit 2002), the highly directional arrangement structure presented by expansive soil is extremely beneficial to its swelling and shrinkage. When water molecules is entered the interstices to form a water film, lattice expansion is occurred, the spacing between lattice faces is increased gradually, which thus causes expansive soil to swell. On the contrary, after the loss of water, the water film in the gap is become thinner, the distance between lattice faces is reduced, which causes expansive soil to shrink.
As it can be seen in Figure 4B, the microstructure of expansive soil after 6 times of treatments is shown that the lamellar soil particles are filled with granular and flaky crystals of different sizes and with irregular surfaces. The essence of these crystals is calcium carbonate precipitation produced by microbial mineralization, these precipitation with the effect of “cementation” and “filling”.
It can also be seen from Figure 4 that some calcium carbonate precipitates are attached to the surface of soil particles, and soil particles are condensed into aggregates under the cementation of calcium carbonate precipitates. At the same time, the pores between the soil particles are filled by some calcium carbonate deposits. Due to the increasing of aggregates and the filling of pores, the pore volume is reduced significantly, soil hydrophilicity to a certain extent is reduced and water stability of the soil is improved. With the increase of irregular crystals on aggregate surface, friction resistance and cohesion between soil particle faces are increased, shear strength of soil sample is improved (Yao et al., 2022). This is consistent with the results obtained from triaxial test, that the shear strength of expansive soil is increased with the increasing of treatment times.
Experimental study on Nanning expansive soil improved by MICP method have been carried out. Through indoor tests such as compaction, swelling rate and triaxial compression etc., improvement effect of the MICP method is studied comparatively, and microstructure mechanism of improved expansive soil by MICP method has been revealed. The following conclusions have been obtained.
(1) Optimal moisture content required for expansive soil to reach the maximum compaction degree is used as the control standard for total amount of bacteria solution and cementation solution. Thus, the problem that physical state of soil can be changed due to excessive moisture content during improvement of expansive soils by MICP method is solved. In the process of improving expansion soil by MICP method, another problem that low calcium content in natural expansive soil is also solved by adding calcium salt.
(2) Due to microbially induced calcium carbonate precipitation, soil particles are cemented and soil pores are filled and microstructure characteristics of soil are improved, thereby hydrophilicity, compaction, swelling and strength properties of soil have been improved.
(3) Swelling characteristics of expansive soils are suppressed effectively by MICP method. Free swelling rate, unloaded swelling rate and 100 kPa loaded swelling rate are all lower than those of unimproved expansive soil. After improvement, the lowest values of above three swellability indices are 14.9, 2.80 and 0.4% respectively, which are 75.7, 80.3 and 77.8% lower than the unimproved expansive soil.
(4) After improvement, the shear strength of expansive soil has been increased significantly. With treatment times, cohesion and internal friction angle both are increased first and then are become stable. After six treatment times, cohesion and internal friction angle are reached the maximum value, which are 117.04 kPa and 24.48° respectively.
(5) Analysis of microstructure imaging of expansive soil by scanning electron microscope indicate that microstructure of soil is changed due to microbial mineralisation process. Furthermore, improvement mechanism of expansive soils by MICP method is revealed at the microscopic level. Calcium carbonate precipitation is caused in the process of microbial mineralisation, from which soil particles are cemented and soil pores are filled, the swelling, hydrophilicity and the strength of expansive soil thereby are improved.
The above conclusions have laid a theoretical foundation for in-depth research on expansive soils improved by MICP method. It also provides a new idea to prefect and expand engineering application for improvement technology of expansive soil.
The original contributions presented in the study are included in the article/Supplementary Material, further inquiries can be directed to the corresponding author.
Conceptualization, QO and HX; methodology, QO and HX; software, QO and HX; validation, QO and HX; formal analysis, QO, HX, ZL, WJ, HS, XT, and MO; investigation, QO, HX, ZL, WJ, HS, XT, and MO; resources, QO and HX; data curation, QO, HX and WJ; writing-original draft preparation, QO and HX; writing-review and editing, QO, HX and ZL; visualization, QO, HX, ZL, WJ, HS, XT, and MO; supervision, QO, HX, and ZL; project administration, HX; funding acquisition, HX All authors have read and agreed to the published version of the manuscript.
The work described in this paper is supported by a grant from the National Natural Science Foundation of China (Project No. 50978097) and the research Foundation of Education Bureau of Hunan Province of China (Project No. 19B581).
Author WJ was employed by Sinohydro Foundation Engineering Co, Ltd.
The remaining authors declare that the research was conducted in the absence of any commercial or financial relationships that could be construed as a potential conflict of interest.
All claims expressed in this article are solely those of the authors and do not necessarily represent those of their affiliated organizations, or those of the publisher, the editors, and the reviewers. Any product that may be evaluated in this article, or claim that may be made by its manufacturer, is not guaranteed or endorsed by the publisher.
The assistance and guidance of the steering group members is gratefully acknowledged.
Bai, B., Long, F., Rao, D., and Xu, T. (2017). The Effect of Temperature on the Seepage Transport of Suspended Particles in a Porous medium'The Effect of Temperature on the Seepage Transport of Suspended Particles in a Porous Medium '. Hydrol. Process. 31 (2), 382–393. doi:10.1002/hyp.11034
Bai, B., Rao, D., Chang, T., and Guo, Z. (2019). A Nonlinear Attachment-Detachment Model with Adsorption Hysteresis for Suspension-Colloidal Transport in Porous media'A Nonlinear Attachment-Detachment Model with Adsorption Hysteresis for Suspension-Colloidal Transport in Porous media'. J. Hydrol. 578, 124080. doi:10.1016/j.jhydrol.2019.124080
Bai, B., Xu, T., Nie, Q., and Li, P. (2020). Temperature-driven Migration of Heavy Metal Pb2+ along with Moisture Movement in Unsaturated soils'Temperature-Driven Migration of Heavy Metal Pb2+ along with Moisture Movement in Unsaturated Soils'. Int. J. Heat Mass Transfer 153, 119573. doi:10.1016/j.ijheatmasstransfer.2020.119573
Bai, B., Nie, Q., Zhang, Y., Wang, X., and Hu, W. (2021a). Cotransport of Heavy Metals and SiO2 Particles at Different Temperatures by seepage'Cotransport of Heavy Metals and SiO2 Particles at Different Temperatures by Seepage'. J. Hydrol. 597, 125771. doi:10.1016/j.jhydrol.2020.125771
Bai, B., Jiang, S., Liu, L., Li, X., and Wu, H. (2021b). The Transport of Silica Powders and lead Ions under Unsteady Flow and Variable Injection concentrations'The Transport of Silica Powders and lead Ions under Unsteady Flow and Variable Injection Concentrations'. Powder Tech. 387, 22–30. doi:10.1016/j.powtec.2021.04.014
Bai, B., Zhou, R., Cai, G., Hu, W., and Yang, G. (2021c). Coupled Thermo-Hydro-Mechanical Mechanism in View of the Soil Particle Rearrangement of Granular Thermodynamics. Comput. Geotechnics 137 (8), 104272. doi:10.1016/j.compgeo.2021.104272
Bian, J. (2016). 'Water Stability of Lime-Treated Expansive Soil'. J. Yangtze River Scientific Res. Inst. 33, 77–82. doi:10.11988/ckyyb.20140013
Bian, J. (2020). 'Remoulded Lime-Treated Expansive Soil: Shear Strength and Application'. J. Yangtze River Scientific Res. Inst. 37, 103–109. doi:10.11988/ckyyb.20190973
Cai, H., Xiao, J., Wang, Z., and Li, J. (2020). 'Experimental Study on Solidification of Soft clay Based on MICP'. Chin. J. Geotechnical Eng. 42, 249–253. doi:10.11779/CJGE2020S1049
Cheng, Y., Tang, C., Xie, Y., Liu, B., and Pan, X. (2021). 'Experimental Study on Structure Strength of Loess Improved by Microbial Induced Calcite Precipitation'. J. Eng. Geology. 29, 44–51.doi:10.13544/j.cnki.jeg.2020-359
Elkady, T. Y., and Shaker, A. A. (2018). 'Role of Cementation and Suction in the Swelling Behavior of Lime-Treated Expansive Soils'. J. Mater. Civil Eng. 30, 1–8. doi:10.1061/(ASCE)MT.1943-5533.0002140
Feng, J., Chen, B., Lu, S., Hu, W., Xu, X., and Zhang, Y. (2021). 'Preparation and Characterization of Self-Healing concrete Based on Biomineralization Mechanism'. J. Funct. Mater. 52, 3026–3033+3042. doi:10.3969/j.issn.1001-9731.2021.03.004
Fu, C., Zeng, Z., Mo, H., and Huang, Y. (2019). 'Experimental Study on the Engineering Characteristics of the Improved Expansive Soils with Lime'. J. Guangxi Univ. (Nat Sci Ed) 44, 524–533.
Guo, K., Chu, C., Ye, H., Li, D., and Zhao, H. (2020). 'Study on Direct Shear Strength of Expansive Soil Improved by Iron Tailing Sands and Calcium Carbide Slag'. J. Hefei Univ. Tech. (Natural Science) 43, 1263–1268. doi:10.3969/j.issn.1003-5060.2020.09.020
Hao, M., Gao, Y., He, J., Qi, Y., and Hang, L. (2021). 'Microbially Induced Carbonate Precipitation for Wind Erosion Control of Desert Soil: Field-Scale Tests'. Geoderma 383, 1–9.doi:10.1016/j.geoderma.2020.114723
He, J., Guo, H., Tan, Q., and Cheng, X. (2019). 'Experiment Research on the Restoration of white marble Beams Using Microbially-Induced Carbonate Precipitation'. Sci. Conservation Archaeology 31, 46–53.
Hensen, E. J. M., and Smit, B. (2002). 'Why Clays Swell'. J. Phys. Chem. B 106, 12664–12667. doi:10.1021/jp0264883
Jiang, W., Zhang, C., Xiao, H., Luo, S., Li, Z., and Li, X. (2021). 'Preliminary Study on Microbially Modified Expansive Soil of Embankment'. Geomechanics Eng. 26 (3), 301–310. doi:10.12989/gae.2021.26.3.301
Kejun, W., Li, Y., and Shihui, L. (2019). ‘Development of an Improved Immersing Method to Enhance Microbial Induced Calcite Precipitation Treated sandy Soil through Multiple Treatments in Low Cementation media Concentration'. Geotechnical Geol. Eng. 37 (2), 1015–1027. doi:10.1007/s10706-018-0669-6
Li, S., Li, C., Yao, D., and Wang, S. (2020). 'Feasibility of Microbially Induced Carbonate Precipitation and Straw Checkerboard Barriers on Desertification Control and Ecological Restoration'. Ecol. Eng. 152, 1–8. doi:10.1016/j.ecoleng.2020.105883
Li, X., Zhang, C., Xiao, H., Jiang, W., Qian, J., and Li, Z. (2021). 'Reducing Compressibility of the Expansive Soil by Microbiological-Induced Calcium Carbonate Precipitation'. Adv. Civil Eng. 2021, 1–12.doi:10.1155/2021/8818771
Liang, S., Chen, J., Niu, J., Gong, X., and Feng, D. (2020). Using Recycled Calcium Sources to Solidify sandy Soil through Microbial Induced Carbonate precipitation'Using Recycled Calcium Sources to Solidify sandy Soil through Microbial Induced Carbonate Precipitation'. Mar. Georesources Geotechnology 38, 393–399. doi:10.1080/1064119x.2019.1575939
Liu, H., Xiao, P., Xiao, Y., and Chu, J. (2019). 'State-of-the-art Review of Biogeotechnology and its Engineering Applications'. J. Civil Environ. Eng. 41, 1–14.
Liu, K., Ye, W., Gao, H., and Dong, Q. (2020a). 'Multi-scale Effects of Mechanical Property Degradation of Expansive Soils under Drying-Wetting Environments'. Chin. J. Rock Mech. Eng. 39, 2148–2159. doi:10.1080/1064119x.2020.1823535
Liu, S., Yu, J., Zeng, W., Peng, X., Cai, Y., and Tu, B. (2020b). ' Repair Effect of Tabia Cracks with Microbially Induced Carbonate Precipitation '. Chin. J. Rock Mech. Eng. 39, 191–204. doi:10.13722/j.cnki.jrme.2019.0421
Lu, Y., Chen, L., Yu, Q., Cheng, C., and Cheng, Y. (2021). 'Fusarium Oxysporum Induces Mineralization Recovery Rare Earth Ions Lanthanum (Ⅲ)'. Acta Microbiologica Sinica 61, 1621–1631. doi:10.13343/j.cnki.wsxb.20210104
Ma, P., Zhuang, Y., and Liu, Z. (2019). 'Experimental Study on Expandability of Montmorillonite Modified by Electrochemical Method'. Chin. J. Geotechnical Eng. 41, 900–907. doi:10.11779/CJGE201905013
Mwandira, W., Nakashima, K., Kawasaki, S., Ito, M., Sato, T., Igarashi, T., et al. (2019). Solidification of Sand by Pb(II)-tolerant Bacteria for Capping Mine Waste to Control Metallic Dust: Case of the Abandoned Kabwe Mine, Zambia. Chemosphere 228, 17–25. doi:10.1016/j.chemosphere.2019.04.107
Qin, Y., Wang, L., Yuan, M., Shen, T., Li, X., and Wang, S. (2020). 'An Experimental Study on Handan Strong Expansive Soil Enhanced by Bacillus Megaterium'. J. Southwest China Normal Univ. (Natural Sci. Edition) 45, 87–95.doi:10.13718/j.cnki.xsxb.2020.08.013
Shafiqu, Q. S. M., and Hasan, S. H. (2018). 'Improvement an Expansive Soil Using Polymethacrylate Polymer'. Conf. Ser. Mater. Sci. Eng. 454, 1–10. doi:10.1088/1757-899x/454/1/012138
Sun, D., Xu, W., Liu, K., Ouyang, J., and Wang, A. (2021). 'Research Progress of the Application of MICP in Architecture'. Mater. Rep. 35, 382–393. doi:10.11896/cldb.20100019
Whiffin, V. S. (2004). 'Microbial CaCO3 Precipitation for the Production of Biocement'. Int. J. Heat Mass Transfer 153, 119573.
Wu, D., She, W., Wei, L., Zuo, W., Hu, X., Hong, J., et al. (2020). Stabilization Mechanism of Calcium Lignosulphonate Used in Expansion Sensitive Soil'Stabilization Mechanism of Calicium Lignosulphonate Used in Expansion Sensitive Soil'. J. Wuhan Univ. Technol.-Mat. Sci. Edit. 35, 847–855. doi:10.1007/s11595-020-2329-y
Wu, Y., Miao, S., Li, X., Wang, H., Cao, Y., and Hu, X. (2021a). 'Experimental Study on Physical and Mechanical Properties of Expansive Soil Improved by Steel Slag Powder Cement under Freeze-Thaw Cycle'. J. Eng. Geology. 29, 851–861. doi:10.13544/j.cnki.jeg.2019-464
Wu, Y., Qiao, X., Li, D., Han, T., Miao, S., and Cao, Y. (2021b). 'Experimental Study on Expansive Soil Improved by Steel Slag Powder Cement under Dry-Wet Cycle'. J. Xi'an Univ. Architecture Tech. (Natural Sci. Edition) 53, 319–329.
Xu, L., Yang, L., Yang, X., Yinjun, S., and Qi, Y. (2017). 'Physico-mechanical Properties of Cement-Modified Expansive Soil under Freeze-Thaw Cycles'. J. Yangtze River Scientific Res. Inst. 34, 87–91+103.
Xu, Z., Zhang, H., Yang, H., and Xu, N. (2020). 'The Remediation Effect of MICP Technology on Mn(Ⅱ) and Cr(Ⅵ) Contaminated Soil'. J. Yangzhou University(Natural Sci. Edition) 23, 73–78.
Yao, Y., Ni, J., and Li, J. (2021). Stress-dependent Water Retention of Granite Residual Soil and its Implications for Ground Settlement. Comput. Geotechnics 129, 103835. doi:10.1016/j.compgeo.2020.103835
Yao, Y., Li, J., Ni, J., Liang, C., and Zhang, A. (2022). Effects of Gravel Content and Shape on Shear Behaviour of Soil-Rock Mixture: Experiment and DEM modelling'Effects of Gravel Content and Shape on Shear Behaviour of Soil-Rock Mixture: Experiment and DEM Modelling'. Comput. Geotechnics 141, 104476. doi:10.1016/j.compgeo.2021.104476
Yu, M., Zhang, J., Zhou, Y., and Sun, K. (2021). 'Experimental Study on the Modification of Expansive Soil by MICP Technology'. J. Yangtze River Scientific Res. Inst. 38, 103–108+122. doi:10.11988/ckyyb.20200096
Yu, X., Xiao, H., Li, Z., Qian, J., Luo, S., and Su, H. (2022). 'Experimental Study on Microstructure of Unsaturated Expansive Soil Improved by MICP Method'. Appl. Sci. 12, 342. doi:10.3390/app12010342
Keywords: expansive soil, microbially induced calcite precipitation method, swell-shrink characteristics, mineralization process, microstructure
Citation: Ouyang Q, Xiao H, Li Z, Jiang W, Su H, Tian X and Ouyang M (2022) Experimental Study on the Influence of Microbial Content on Engineering Characteristics of Improved Expansive Soil. Front. Earth Sci. 10:863357. doi: 10.3389/feart.2022.863357
Received: 27 January 2022; Accepted: 17 February 2022;
Published: 07 March 2022.
Edited by:
Xiaodong Fu, Institute of Rock and Soil Mechanics (CAS), ChinaReviewed by:
Tao Li, Beijing Jiaotong University, ChinaCopyright © 2022 Ouyang, Xiao, Li, Jiang, Su, Tian and Ouyang. This is an open-access article distributed under the terms of the Creative Commons Attribution License (CC BY). The use, distribution or reproduction in other forums is permitted, provided the original author(s) and the copyright owner(s) are credited and that the original publication in this journal is cited, in accordance with accepted academic practice. No use, distribution or reproduction is permitted which does not comply with these terms.
*Correspondence: Hongbin Xiao, dDIwMDkwMTY5QGNzdWZ0LmVkdS5jbg==
Disclaimer: All claims expressed in this article are solely those of the authors and do not necessarily represent those of their affiliated organizations, or those of the publisher, the editors and the reviewers. Any product that may be evaluated in this article or claim that may be made by its manufacturer is not guaranteed or endorsed by the publisher.
Research integrity at Frontiers
Learn more about the work of our research integrity team to safeguard the quality of each article we publish.