- 1Sinopec Petroleum Exploration and Production Research Institute, Beijing, China
- 2Southern Company of Exploration, SINOPEC, Chengdu, China
Rock pyrolysis, organic petrology, vitrinite reflectance, gas chromatography-mass spectrometry (GC-MS) analysis, and biomarker compound analysis were performed to comprehensively analyze the organic geochemical characteristics of the Jurassic Da’anzhai Member (J1da) shale strata in Yuanba and Puguang areas in the northeastern Sichuan Basin. Then the organic matter provenance and sedimentary environment were further analyzed. Finally, the significance of oil and gas exploration in J1da shale strata was discussed. Results show that the second section of the Da’anzhai Member (J1da2) has relatively high organic matter abundance (1.24%TOC), type Ⅱ-dominated organic matter type, which is the most favorable section of wells Y1 and T1 in the study area. The organic matter maturity and the hydrocarbon phases are quite different, which is 1.01%Ro dominated by oil generation in Puguang area, while it is 1.67%Ro dominated by gas generation in Yuanba area. Content and chromatograms of biomarkers including n-alkanes, tricyclic terpanes, C24 tetracyclic terpanes, and C27-C28-C29 regular steranes show that the organic matters of J1da shale strata derive from both terrestrial higher plants and lower aquatic organisms, with slightly differentiated mixed ratio of each sublayer. Characteristics of Pr/Ph, γ-cerane and hopanes compounds indicate that the overall depositional environment of J1da is a freshwater lacustrine environment, with saline lake deposits in local areas and intervals in the study area. The rapid changes of sedimentary environment have resulted in obvious stratification of water body, frequent interbeds, and strong heterogeneity of J1da shale strata. Comprehensive analysis shows the shale/mud microfacies in the semi-deep lake subfacies and shale/mud interbedded with siltstone and shell bank microfacies in the shallow lake subfacies are the most favorable sedimentary facies for J1da hydrocarbon enrichment. Deeper burial depth and higher maturity make for oil and gas enrichment with higher gas/oil ratio (GOR); moreover, the thicker intervals with organic-rich shale are favorable targets for geochemical evaluation.
1 Introduction
The Sichuan Basin is one of the important petroliferous basins in China, with several sets of developing hydrocarbon-bearing formations, including Sinian–Middle Triassic marine carbonate rocks and Upper Triassic–Tertiary continental clastic rocks. It contains abundant conventional and unconventional oil and gas resources, showing the characteristics of being “multi-layer, multi-type, multi-evolution, and multi-genesis” (Deng, 1992; Chen et al., 2011; Zou et al., 2014; Tang et al., 2020a; He et al., 2020; Nie et al., 2021). Significant achievements have been made of Silurian marine shale gas reservoirs, and national-level shale gas demonstration areas including Fuling, Changning, Weiyuan, and Zhaotong have been established (Jiang et al., 2015; Xie et al., 2017; Zhang et al., 2020; Zou et al., 2020; Wang et al., 2021). With continuous exploration and development of the Sichuan Basin, oil companies such as China National Petroleum Corporation (CNPC) and Sinopec are actively expanding other shale oil and gas fields, among which the Jurassic continental shale formation is one of the realistic areas (Gao et al., 2018; Long et al., 2020; Cai et al., 2021; Yang et al., 2021). Since year 2008, the Jurassic Formation in Sichuan Basin has successively carried out petroleum exploration works such as old well retesting and exploration well drilling (Zhou et al., 2013; Guo et al., 2016; Zhou et al., 2020). Many wells have been tested and even obtained industrial shale oil and gas flows in the Dongyuemiao Member, Da'anzhai Member, or Qianfoya Formation (Lianggaoshan Formation) (Zhou et al., 2013, 2020; Guo et al., 2021). For example, well YB21 of the Da'anzhai Member in Yuanba area in the northern Sichuan Basin has achieved a maximum daily gas production of 507, 000 m3, and well FY10 of the Dongyuemiao Member in Fuling area in the eastern Sichuan Basin has achieved a maximum daily gas production of 55,800 m3 and oil output of 17.6 m3 (Zhou et al., 2013, 2020; Guo et al., 2021). It indicated that the Jurassic Formation in Sichuan Basin had great potential for shale gas and oil exploration and development. However, bottlenecks such as low initial output or rapid production decline were common in wells aiming at the Jurassic Formation, so large-scale commercial development has not yet been achieved (Liu et al., 2019; Zhou et al., 2020).
Compared with the marine shales, the sedimentary environment of Jurassic continental shale was more complex (Guo et al., 2016; Liu et al., 2019; Zhou et al., 2020). Greatly varying sedimentary microfacies, lateral migration of the sedimentation centers in different periods, and multiple-source supply of provenances from different directions led to a strong heterogeneity and complex enrichment characteristic of Jurassic shale formation; thus, it was difficult for sweet spot/section prediction (Huang et al., 2018a; Liu et al., 2019). In addition, there were few cores in the shale intervals during the old wells, which brought challenges to the basic geological research and exploration of the Jurassic shale in the Sichuan Basin. Previous studies on the Jurassic Da’anzhai Member in the northeastern Sichuan Basin mainly focused on lithology, lithofacies, pore structure, and other characteristics as a reservoir, while there was lack in research on organic geochemical characteristics and biogenic provenance as a source rock, which restricted the overall evaluation of oil and gas exploration potential (Zheng et al., 2013; Xu Q. et al., 2017; Liu et al., 2021).
In this study, based on the core observation and systematic coring of two newly drilled wells (well Y1 in Yuanba area and well T1 in Puguang area), organic geochemical characteristics including organic matter abundance, type, and maturity of the Jurassic Da’anzhai shale member in the Northeast Sichuan Basin were analyzed. Then the characteristics of biomarkers were further analyzed to explain the organic matter biogenesis and sedimentary environment. It is of great significance for accurately evaluating the oil and gas generation potential of the Da’anzhai shale and providing a geochemical basis for the exploration and deployment of the Jurassic shale formations and sweet intervals selection.
2 Geological Settings
Sichuan Basin is a large-scale superimposed basin (Liu et al., 2011; Ma et al., 2019), which can be divided into six regions according to the regional tectonic characteristics, as shown in Figure 1. The northeastern region of Sichuan Basin belongs to the superimposed block of Micangshan arcuate tectonic belts, Dabashan arcuate tectonic belts, and eastern Sichuan arcuate fold belts and contains petroliferous areas of Yuanba, Langzhong, Tongnanba, and Puguang (Zheng et al., 2017; Liu et al., 2021). Among them, Yuanba and Puguang areas are taken as the study area (Figure 1). Since Sinian period, the study area has mainly experienced Caledonian, Hercynian, Indosinian, Yanshan, and Himalayan tectonic movements. Nowadays, the stratigraphic stratum of Yuanba and Puguang area is almost horizontal and in relatively stable tectonic setting, so the reservoir preservation conditions are quite good (Liu et al., 2019). The Jurassic strata are well developed in the study area. From bottom to top, these are the Lower Jurassic Ziliujing Formation, Middle Jurassic Qianfoya Formation (Lianggaoshan Formation), Shaximiao Formation, Upper Jurassic Suining Formation, and Penglaizhen Formation. The Ziliujing Formation can be further divided into Zhenzhuchong Member, Dongyuemiao Member, Ma’anshan Member, and Da’anzhai Member according to the characteristics of lithologic association (Figure 1). In the depositional period of the Da’anzhai Member (J1da), the stratum has undergone a complete lacustrine transgressive–lacustrine regressive cycle, with a sedimentary thickness of about 40–130 m (Zhou et al., 2013, 2020). Due to the frequent oscillations of the water body and the complexity of sediment provenance in the lake basin, the Da’anzhai Member showed obvious heterogeneity among different sections and layers, leading to frequent interbedding of the three lithologies of “sand, mud, and ash,” which created favorable conditions for the shale gas forming and accumulating (Huang et al., 2018a; Wang et al., 2019). The second section of the Da’anzhai Member (J1da2) reached the maximum lacustrine flooding period, developed semi-deep and shallow lake sedimentary facies that contained thick black shales and favorable lithofacies assemblages, and was the main target interval in this study.
3 Samples and Methods
In order to systematically analyze the geochemical characteristics and sediment source of the J1da shales in the northeastern Sichuan Basin, typical shale core samples were collected from the risky exploratory well Y1 in southern Yuanba area and the geological shallow well T1 in southern Puguang area based on detailed core observations. The tectonic locations of sampling wells Y1 and T1 are shown in Figure 1. The sampling depth of shale cores from well Y1 is 3,849.22–3,935.48 m, and the lithology of core samples is mainly gray-black shale, dark-gray calcareous mudstone, dark-gray shell-bearing shale, and dark gray silty shale. The sampling depth of shale cores from well T1 is 12.94–68.87 m, and the lithology of core samples is mainly dark-gray mudstone, shell shale, and gray-black shell-bearing mudstone (Figure 2).
In this study, 73 core samples of well Y1 and 40 core samples of well T1 from J1da stale strata were subjected to laboratory tests including total organic carbon (TOC) content determination, rock pyrolysis, organic petrology, and organic maceral analysis. Then some representative samples were subjected to experimental tests such as chloroform bitumen “A” extraction and quantification, vitrinite reflectance (Ro) detection, kerogen's carbon isotope determination, saturated hydrocarbon gas chromatography (GC) techniques, aromatic hydrocarbon gas chromatography-mass spectrometry (GC-MS) analysis, and rock biomarker compound analysis. The TOC content was obtained using a LECO CS230 carbon-sulfur analyzer, and the experiment was conducted at 20–28°C and a relative humidity of less than 70%. Vitrinite reflectance (Ro, %) was determined using a MPM-80-type micro-spectrophotometer. Five samples were measured, and five Ro data were obtained. Bitumen “A” extractions were performed on the powdered samples (size <0.18 mm) using a XP205 Soxhlet apparatus. The experiment temperature was less than 85°C. The extracts were separated into saturated hydrocarbon, aromatic hydrocarbon, and NSO compound fractions by liquid column chromatography. The saturated fraction of 10 samples were dissolved in petroleum ether and analyzed using GC, and aromatic fraction was analyzed using GC-MS. The GC was reformed using a HP5 column with a temperature 60–310°C at a rate of 6°C/min. The GC-MS analysis was performed on an Agilent 5975C MSD mass spectrometer with a gas chromatograph. Some sample components were separated by thin plates. The fragmentograms for steranes (m/z 217) and triterpanes (m/z 191) were recorded according to the analysis of biomarkers using the Agilent 5977B MSD. All the experimental tests were performed in the Experimental Research Center of Wuxi Institute of Petroleum Geology, Sinopec Petroleum Exploration and Development Research Institute.
4 Conclusions and Discussions
4.1 Organic Geochemical Features
4.1.1 Organic Matter Abundance
Organic matter abundance affected the hydrocarbon generation capacity of source rocks and the scale of a reservoir. Common evaluation indicators of organic matter abundance include TOC content, hydrocarbon generation potential (PG), chloroform bitumen “A,” total hydrocarbon content (HC), and hydrogen index (HI) (Lu and Zhang, 2010). Experimental results show that the average TOC content of the J1da2 shale from well Y1 in Yuanba area are mainly in the two ranges of 1.0–2.0% and 0.5–1.0%. Samples with TOC content between 1.0% and 2.0% account for about 57%, which is significantly higher than that of the J1da1 and J1da3 shale, and is regarded as the main section of high-quality source rock in J1da in Yuanba area. However, TOC content of the J1da shale from well T1 in Puguang area ranges from 0.5% to 2.71%, with an average value of 1.24%, which is slightly higher than that of Yuanba area, and the TOC content increases as the burial depth becomes shallower (Figure 2).
Rock pyrolysis analysis shows that hydrocarbon generation potential (PG = S1 + S2) of the J1da shale from well Y1 in Yuanba area is between 0.78 and 1.98 mg/g, with an average value of only 0.72 mg/g, which is much lower than that of the evaluation standard of a source rock (>2 mg/g). However, PG values of the J1da shale from well T1 in Puguang area range from 0.15 to 10.77 mg/g, with an average value of 3.83 mg/g, which can be evaluated as a good source rock (Figure 2). Combined with the other parameters, it is thought that the above difference is mainly due to the widely varying burial depth and organic matter maturity of shale samples between the two areas. Previous studies indicated that, with the increase of thermal evolution degree, hydrocarbon generation potential of source rocks would decrease significantly (Lu et al., 2003; Pang et al., 2014). Therefore, the hydrocarbon generation potential index PG is more suitable for the immature-low mature shale samples in Puguang area. According to Peters and Cassa (1994), the relative content of TOC and S2 could be used for source rock evaluation, and this method weakened the influence of maturity on a single parameter (TOC or S2) to a certain extent. Figure 3 shows a good correlation between TOC and S2 content of the J1da shale samples in this study; that is, with the increase of TOC, S2 has a corresponding increasing trend. From the overall evaluation, it is thought that the J1da shales in the northeastern Sichuan Basin belong to good-general source rocks.
Chloroform bitumen “A” and total HC reflected the contents of soluble organic matter and hydrocarbons in sedimentary rocks, so they were also important abundance indicators for source rock evaluation (Lu and Zhang, 2010). However, they could be affected by organic matter type and maturity, so they were generally not used alone when evaluating the organic matter abundance of a source rock (Chen et al., 2009). In this study, the content of chloroform bitumen “A” of the shale samples from J1da in Yuanba ranges from 0.008% to 0.066%, with an average value of 0.025%, which gradually increases from bottom to top. However, the chloroform bitumen “A” content of the J1da shale samples in Puguang is between 0.007% and 0.302%, with an average of 0.113%, and the values in the upper part is significantly higher than the lower part, which is consistent with the distribution characteristics of PG. Compared with the Dongyuemiao Member in Fuling area, HC values of the J1da shale are significantly lower, while the asphaltene content is significantly higher, reflecting the differences in kerogen type, thermal evolution degree, and sedimentary environments between different members of Jurassic shale strata in the northeast Sichuan Basin (Shu et al., 2021).
To sum up, the organic matter abundance of J1da shale in Puguang is slightly better than that in Yuanba area. The upper part of the second section of J1da is better than other sections according to well Y1 and well T1, which can be evaluated as a good-general source rock on the whole, which is a favorable sweet spot for the J1da shale in the northeastern Sichuan Basin.
4.1.2 Organic Matter Type
The distributions of organic macerals in source rocks were related to the organic matter provenance and depositional environment (Marynowski, et al., 2007). Identifying the organic macerals and compositional characteristics under the microscope was an effective method to determine the organic matter type of source rocks (Hu and Huang, 1991). In this study, 30 and 20 J1da shale samples were selected for organic petrological analysis from well Y1 and well T1, respectively. Microscopic observation and identification show that the organic macerals of J1da shale samples in northeastern Sichuan Basin consist of vitrinite, fusinite, solid asphalt, and microsomes. Vitrinite are generally developed in all samples. The vitrinite content of samples from well Y1 is 40–92% with an average of 79%, and it ranges from 25% to 90% with an average of 55% in samples from well T1, indicating that the organic matter type of J1da shale in Puguang area is generally better than that in Yuanba area. The vitrinite types in the studied samples are mainly vitrodetrinite and occasionally phyllovitrinite, which are mostly lump-shaped or strip-shaped, with a uniform surface and gray-gray-white color under microscopic observation (Figures 4B,D). It is speculated that they are mainly formed by humification and gelatination from lignocellulosic tissues of higher plants (Raj, 1979). The content of fusinite is second only to vitrinite, and it is mostly bright white with bulges under the microscope (Figures 4A,E), which transform from the lignocellulosic tissue of higher plants by fusainization. Microsomes are dispersed in minerals and clay, so the contents are not easy to estimate. The solid asphalt is distributed only in local intervals and is mainly found along the rock cracks or mineral intergranular filling. It is generally considered that solid asphalt is the product of crude oil cracking, that is, the post-oil asphalt (Figure 4C). Some solid asphalts have orange-yellow fluorescence, which is speculated to be the former-oil asphalt (Figure 4F) (Nishikawa et al., 2009). Mineral matrix asphalt that is uniformly mixed with inorganic minerals is common in J1da samples from well T1, accounting for about 22% of the total organic macerals content. The higher development degree of solid asphalt reflects the better organic matter type of J1da source rock and stronger shale oil and gas generation capacity, to a certain extent (Liu et al., 2021).
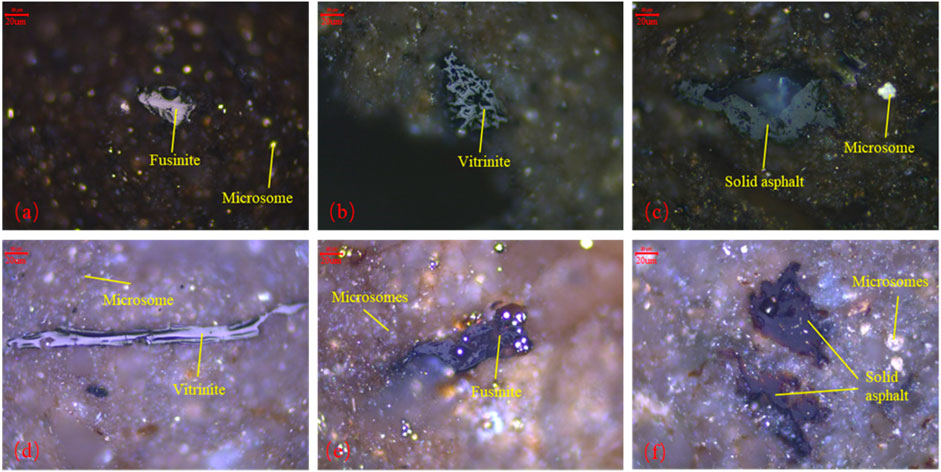
FIGURE 4. Organic macerals characteristics of the typical shale samples under the microscope. (A) Fusinite, well Y1, 3,885 m. (B) Vitrinite, well Y1, 3,907.44 m. (C) Solid asphalt, well Y1, 3,915.82 m. (D) Vitrinite, well T1, 43.91 m. (E) Fusinite, well T1, 53.88 m. (F) Solid asphalt, well T1, 28.2 m.
The carbon isotope of kerogen was related to the depositional environment and the origin of parent materials, so the carbon isotope characteristics inherited from the parent material can be used for the classification of kerogen types (Omokawa, 1982; Beukes et al., 1990). It was generally believed that the higher the content of organic matter in terrestrial higher plants, the heavier the stable carbon isotope in it, while the lower hydrobiont showed just the opposite (Cheng et al., 2008). According to the values of kerogen carbon isotope δ13C, organic matter types of J1da shale are classified, shown in Figure 5. It indicates that organic matter types of J1da shale in the study area are mainly type II1 and II2, reflecting the parent materials are derived from the mixed sources of terrestrial higher plants and lower hydrobionts. The organic matter type of J1da shale in Yuanba is mainly type II2, while it is mainly type II1 in Puguang, both of which show a trend of better types as the burial depth becomes shallower, indicating that the organic matter type in the upper part of the J1da shale strata is better (Figure 5).
4.1.3 Organic Matter Maturity
The value of organic matter maturity determines the stage of thermal evolution degree of a source rock, so as to determine whether it is given priority to generate oil or gas, which is very important for evaluating the exploration potential of shale oil and gas (Garrigues et al., 1988; Lineva et al., 2018). Vitrinite reflectance (Ro) was currently the most effective indicator for determining the organic matter maturity (Qiu et al., 2004). Results show that Ro values of J1da shale in Yuanba area vary in the range of 1.44–1.83%, with an average of 1.67%, based on five samples of well Y1, which indicates the shale strata have reached a mature-high mature evolution stage and is currently in the peak of gas generation period. However, experimental data of 14 J1da shale samples from Puguang area show that Ro values are 0.76–1.18%, with an average value of 1.01%, which is within the oil-generating window.
The maximum pyrolysis peak temperature (Tmax) tends to increase with the increase of burial depth and the age of the formation, so it can also be used as an effective indicator for evaluating the maturity of source rocks (Zhou et al., 2014). Rock-Eval of 40 shale samples of well Y1 shows that the Tmax values range from 472°C to 496°C, with an average of 487°C, indicating the J1da shale in Yuanba is in a high-mature evolution stage, even over-mature stage on the whole. However, the Tmax values of 18 shale samples in Puguang range from 444°C to 453°C, with an average of 449°C, indicating that they are in the mature stage (Figure 6).
Based on the above analysis of geochemical characteristics, it can be concluded that the organic matter content and type of J1da shale in northeastern Sichuan Basin are moderate on the whole, and parameters from well T1 in Puguang are generally better than that from well Y1 in Yuanba. However, the maturity of J1da shale in Yuanba is significantly higher than that in Puguang, resulting in the difference of oil generation or gas generation. Vertically, J1da2 develops the most favorable source rock interval in the J1da shale strata, and the hydrocarbon generation potential of the upper part is better than that of the lower part, so it is the favorable target for the Da’anzhai Member in the northeastern Sichuan Basin.
4.2 Organic Matter Provenance and Depositional Environment
Although many studies believed that the Jurassic Da’anzhai Member in the Sichuan Basin was in a typical terrestrial freshwater lacustrine environment (Zhu et al., 2007; Huang et al., 2018a), some scholars considered that a small scale of transgression occurred during the expansion of the lake basin during this period (Xu W. et al., 2017). In this study, combined with a comprehensive analysis of plenty of biomarker evidence, it is inferred that there exist saline deposits in local areas and intervals, rather than a single freshwater lacustrine environment, of the J1da in the northeastern Sichuan Basin. Moreover, the depositional conditions are of weak reduction, and the rapid change of deposits makes the water body stratified obviously, resulting in the strong heterogeneity of the J1da shale strata.
4.2.1 Organic Matter Provenance
N-Alkanes were widely distributed in organisms such as fungi, algae, and higher plants and were the main components of the saturated hydrocarbon fraction of source rock extracts, so they could be used for indicating the provenance of organic matter (Gelpi et al., 1970; Moldowan et al., 1985; Peters et al., 2005). In this study, GC analysis of saturated hydrocarbon were carried out on 10 shale samples from well Y1 in Yuanba area, and biomarker compound analysis was carried out on shale samples from both well Y1 and well T1. Figure 7 and Table 1 show the statistical results of key parameters based on the laboratory tests. In general, there are similar chromatographic characteristics of n-alkanes among different samples of J1da shale strata in Yuanba area; that is, the carbon number of n-alkanes has a wide distribution range of nC15–nC37, with the main peak carbon number being mainly C19, which agrees with the general compositional characteristics of continental crude oil (Zhu et al., 2013). The chromatograms of J1da2 shale samples from well Y1 show that some samples are pre-single peak with the main peak carbon being nC19 or nC17, reflecting a single provenance of organic matter, and most are derived from plankton such as algae (Jin et al., 2016). However, some samples show double peaks with the main carbon peaks being mostly nC19 and nC25, indicating that the organic matter may be a mixed source of both algae and higher plants (Figure 7). Xu (2015) concluded that the main peak carbon number of n-alkane was mainly nC23 and nC25, which showed the characteristics of post-single peak, by analyzing the saturated hydrocarbon chromatography of shale samples taken from Ziliujing Formation in Puguang area. Based on the above analysis, it is believed that the organic matter of the Jurassic J1da shale in the study area is a typical terrestrial–aquatic mixed input.
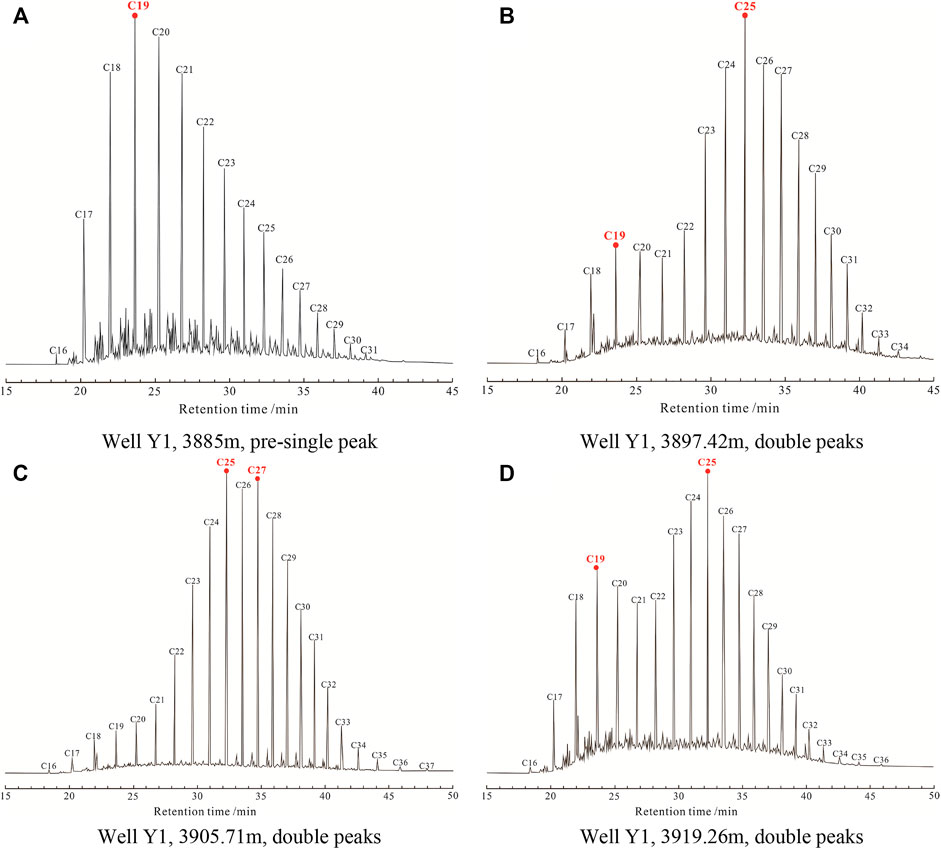
FIGURE 7. Typical saturated hydrocarbon chromatograms of J1da2 shale samples from well Y1. (A) Well Y1, 3,885 m, pre-single peak. (B) Well Y1, 3,897.42 m, double peaks. (C) Well Y1, 3,905.71 m, double peaks. (D) Well Y1, 3,919.26 m, double peaks.
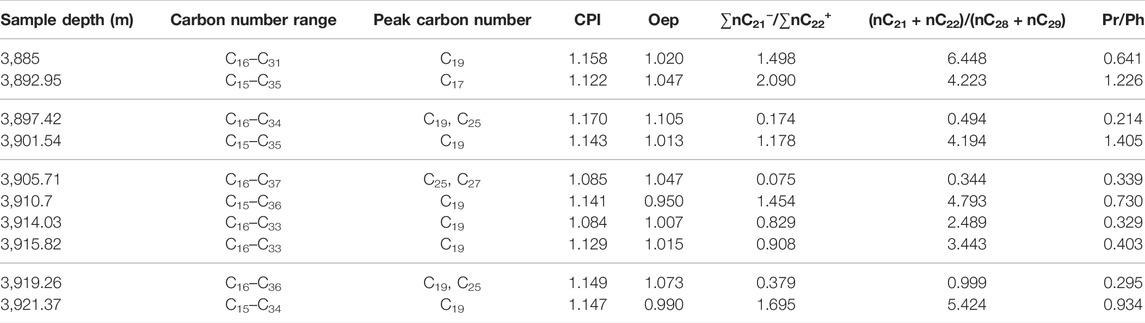
TABLE 1. Statistics of the saturated hydrocarbon chromatographic parameters of shale samples from well Y1.
∑nC21−/∑nC22+ and (nC21 + nC22)/(nC28 + nC29) represented the relative content of light hydrocarbons and heavy hydrocarbons in n-alkanes, which is not only controlled by thermodynamics but also affected by depositional environment and original organic matter (Gelpi et al., 1970; Moldowan et al., 1985; Peters et al., 2005). From Table 1, the value of ∑nC21−/∑nC22+ of the J1da2 shale samples in Yuanba is 0.075–2.09, with an average of 1.028, showing a general equilibrium of C21− and C22+, reflecting that the source of organic matter is a mix of lower aquatic organisms with terrestrial higher plants. Previous study showed that the ∑nC21−/∑nC22+ values of the Ziliujing Formation in Puguang were 0.26–0.37, reflecting a main hydrobiont input (Xu (2015)). Philippi (1974) believed that at the same organic matter maturity, lower hydrobionts had higher (nC21 + nC22) content, while terrestrial higher plants had higher (nC28 + nC29) content. In this study, the values of (nC21 + nC22)/(nC28 + nC29) of J1da2 shale sample from well Y1 ranges from 0.344 to 6.448, with an average of 3.285 (Table 1), indicating that the organic matter provenance is an obvious mixed bio-inputs, and the proportion of different sources vary greatly among layers.
The relative proportion of regular steranes could be used for the analysis of organic matter provenance (Kamp and Leake, 1995). Generally, the predominance of C29-sterane indicated a strong terrigenous input, while lower hydrobionts were rich in C27-sterane (Huang and Meinschein, 1979; Li et al., 2021). Abundant steroids were detected in the J1da shale samples from both wells Y1 and T1 in the study area. As a whole, C27–C29 regular steranes play a dominant role, followed by the rearranged steranes, and the abundance of pregnane is extremely low. The average contents of C27, C28, and C29 regular steranes in samples from Yuanba area are 4.74%, 4.03%, and 6.69%, respectively, and the values in samples from Puguang are 1.84%, 0.99%, and 2.32%, respectively. The typical chromatogram shows that the C27-C28-C29 regular steranes in the ααα-20R configuration show a feature of “V-shaped” or “inverse L-shaped” distribution, that is, C28 < C27 < C29 (Figure 8), further reflecting the J1da shale in the study area has dual biogenic origin of terrestrial higher plants and lower algae and dominated by the former (Seifert and Moldowan, 1986). The previous studies considered the high content of 4α-methyl sterane as a sign of dinoflagellates in the freshwater lake; however, no 4α-methyl sterane was found in the studied samples (Goodwin et al., 1988). Therefore, the existing evidence was not enough to determine the source and cause of algae in the study area. Combined with other scholars' studies, it was speculated that it may originate from planktonic algae or bacteria (Brassell et al., 1986; Chen et al., 1994; Lin, 2017).
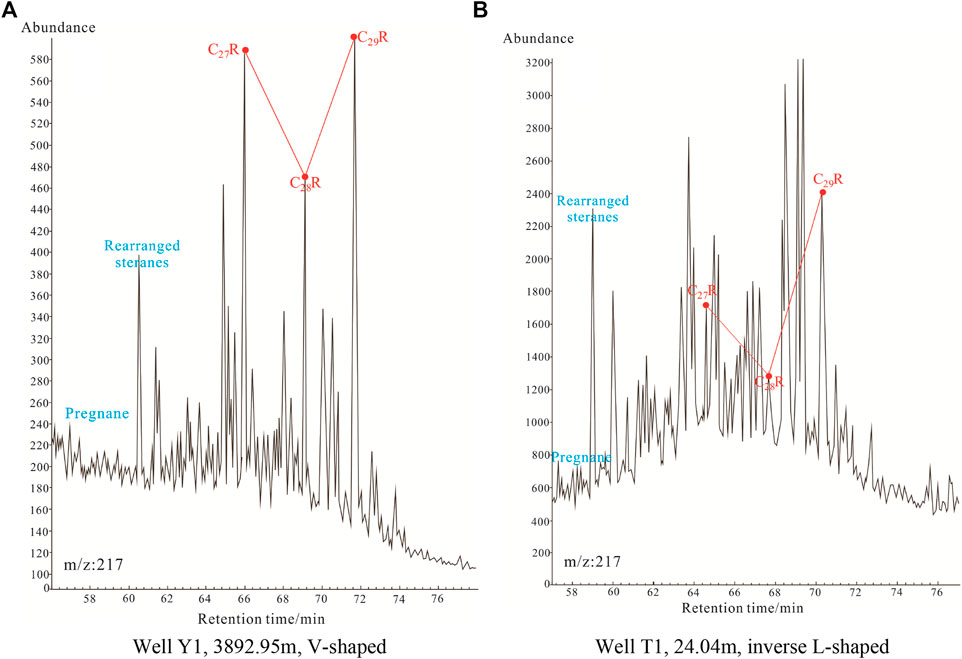
FIGURE 8. Typical sterides chromatograms of J1da shale samples. (A) Well Y1, 3,892.95 m, V-shaped. (B) Well T1, 24.04 m, inverse L-shaped.
In the composition of terpenoids, tricyclic terpenes were generally considered to be derived from microorganisms and algae, whose content and carbon number distribution were quite different among different organic matter types of source rocks (Dutta et al., 2006; Wang et al., 2008), while tetracyclic terpenes may be derived from the degradation of hopane precursors, which indicated terrestrial higher plants (Zhou et al., 2016). According to the experimental tests, the carbon number of tricyclic terpenes in J1da shale samples from well Y1 in Yuanba area is mainly distributed between nC19 and nC29, whose total content is 15.39–24.71% with the mean value of 19.16%, and the content of C24 tetracyclic terpenes is 0.95–1.82% with an average of 1.04%. For J1da shale samples from well T1 in Puguang area, the carbon number of tricyclic terpenes is also distributed in the range of nC19–nC29, whose total content is 8.44–20.88% with a mean value of 11.26% (Figure 9). It shows that in addition to the contribution of algae, there are also certain inputs of terrestrial higher plants during the depositional period of the Jurassic J1da strata in the northeastern Sichuan Basin.
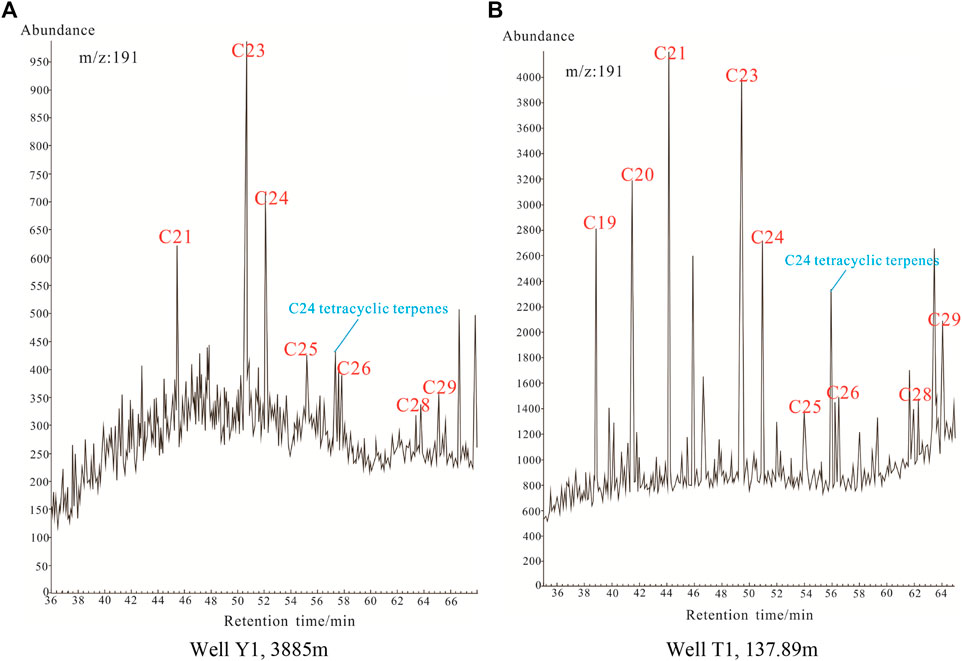
FIGURE 9. Typical terpenoids chromatograms of J1da shale samples. (A) Well Y1, 3,885 m. (B) Well T1, 137.89 m.
4.2.2 Depositional Environment
The ratio of pristane and phytane (Pr/Ph) was usually used to indicate the redox environment where the source rocks and organic matter deposited (Haven et al., 1987). Generally, Pr/Ph value greater than 3.0 reflected an oxidation environment, while less than 1.0 reflected a reducing environment (Wang, 1990; Peters et al., 2005; Tang et al., 2020b). Abundant pristane (Pr) and phytane (Ph) are detected in the J1da2 shale samples from well Y1 in this study. The values of (Pr/Ph) are in the range of 0.214–1.405, with an average of 0.652, shown in Table 1, which shows a characteristic of phytane dominance, indicating that J1da2 shale samples in this study are formed in a reducing or transitional environment. The ratios of isoprenoids to their adjacent n-alkanes, Pr/nC17 and Ph/nC18, can also indicate the redox conditions of water body during paleo-sedimentation (Alias et al., 2012; Yu et al., 2021). The crossplot of the values of Pr/nC17 and Ph/nC18 shows that the depositional environment of J1da2 shale in Yuanba area is mainly a reducing–weak oxidation environment (Figure 10), which is the typical characteristics of semi-deep lake–shallow lake deposition.
Hopanes were common pentacyclic triterpenoids in source rocks, whose carbon number distribution was related to the redox properties of the sedimentary environment (Peters et al., 2005; Lu and Zhang, 2010; Zhu et al., 2021). Hopanes in the studied samples are mainly conventional 17α(H), 21β(H)-hopanes and a small amount of rearranged hopanes, which means that the terrestrial plants in the petrologen are less affected by the bacterial action under the oxidation environment, and the overall hydrocarbon generation condition is in a reducing environment (Zhu et al., 2013). Figure 11 shows two typical hopanes chromatograms of shale samples in the study area. One is shown in Figure 11A; the C31–C35 hopanes show a decreasing distribution pattern with the increase of carbon number, which is common in freshwater lacustrine source rock. This pattern is mainly distributed in the upper section of the J1da shale strata of well Y1 in Yuanba area and also generally seen in the J1da shale strata of well T1 in Puguang area. The other is shown in Figure 11B; the C31–C35 hopanes show a “tail-warped” distribution pattern, which is commonly found in saltwater lacustrine source rock (Zhu et al., 2007). This pattern distributes locally in the middle and lower sections of the J1da shale strata of well Y1 in Yuanba area. From the above analysis, it can be concluded that the J1da shales in the northeastern Sichuan Basin are not deposited in a single water environment, and the sedimentary facies change fast. It is formed in a freshwater lacustrine sedimentary environment in Puguang area; it is dominated by freshwater deposition as a whole in Yuanba area, with salty or brackish sedimentary environment in local intervals.
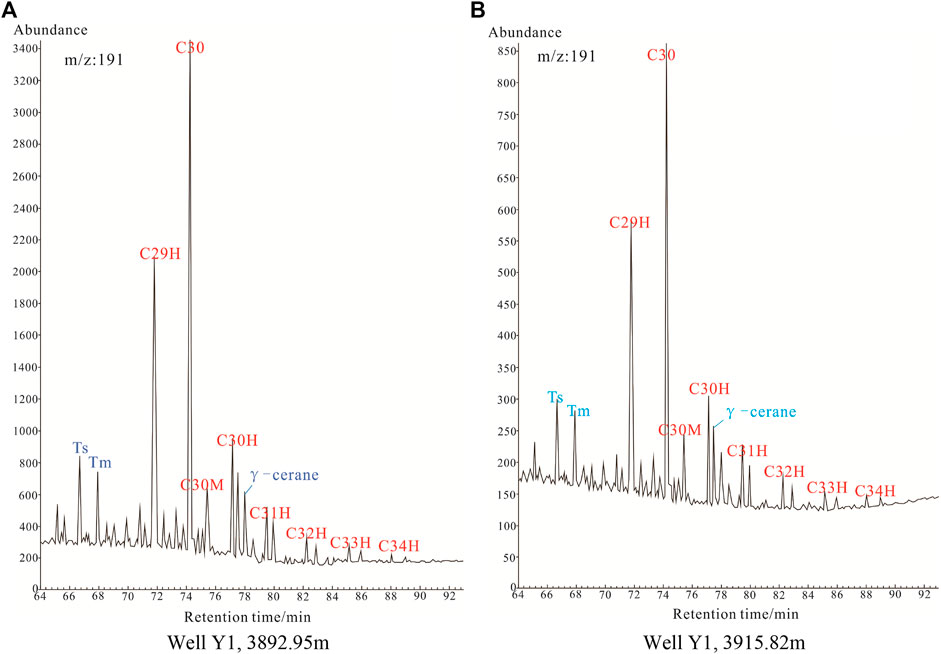
FIGURE 11. Crossplot of Pr/nC17 and Ph/nC18 of J1da2 shale samples from well Y1 (modified from Alias et al., 2012; Yu et al., 2021). (A) Well Y1, 3,892.95 m. (B) Well Y1, 3,915.82 m.
High content of γ-cerane was known as a mark of strong reduction environment in salty or brackish sediments, and the γ-cerane content of source rocks in saline sedimentary environment was usually greater than 1.0 (Zhang et al., 1998; Marynowski et al., 2000). The content of γ-cerane in J1da shale samples in the study area is generally high, which is 1.78–2.50 with an average of 2.11 in well Y1 and 1.06–3.95 with an average of 2.02 in well T1, indicating that the salinity of the water body is high during the deposition period of the Da’anzhai Member. Some scholars believed that high content of γ-cerane did not always exist in the strong reducing and hypersaline environments, and it was essentially a maker of water stratification (Zhang et al., 1999). From this point of view, high content of γ-cerane in the shale samples in this study indicates that the lake water body is stratified during the deposition of the J1da, which leads to the circulation flow stagnation of the bottom water, and the lakebed is in an anoxic and reducing environment, conducive to the preservation of organic matter.
5 Significance of Oil and Gas Exploration
Favorable geochemical conditions are the premise of oil and gas enrichment and also the key elements to find sweet spots/sections. Compared with the Silurian marine shale, the Jurassic continental shale in this study has its own characteristics as a source rock. The sedimentary facies changes rapidly; the shale has strong heterogeneity and thin thickness with medium organic matter abundance, mainly mix typed, as well as widely varying maturity and hydrocarbon phases. It is the basis of clearing shale oil and gas enrichment characteristics and identifying favorable sweet spots of J1da shale strata in the study area.
5.1 Semi-Deep Lacustrine Subfacies is the Most Favorable Sedimentary Facies in J1da
The previous research confirmed that, affected by depth, provenance, and paleomorphology, a small range of extension and a rapid horizontal variation characterized the sedimentary facies of continental strata, and the lithologic assemblages of different sedimentary facies differ significantly (Guo et al., 2016; Zhou et al., 2020). As a result, the organic matter abundance, type, maturity, and reservoir physical properties of different sections in the same set of formations were quite different. Therefore, the sedimentary facies had obvious controls on the enrichment of continental shale oil and gas (Wang et al., 2013; Guo et al., 2021). In the Early Jurassic, the sedimentary and subsidence center of the Sichuan Basin moved from the west to the northeast, due to the beginning of the orogenic crumpling of Dabashan. During the Da’anzhai sedimentary period, the northeastern Sichuan Basin was in a lacustrine depositional system as a whole, and the provenance supply was insufficient, so the J1da member were mainly fine-grained sediments, with local areas being affected by the basin edge delta (Zhang, 2018). According to the lithologic assemblages in Figure 2, the sedimentary environment of well Y1 in Yuanba area can be further divided into shore lake-shallow lake, carbonate shallow lake, clastic shallow lake, and semi-deep lake subfacies, while it can be divided into carbonate shallow lake and semi-deep lake subfacies of well T1 in Puguang area. Combined with previous studies, it is believed that the J1da in the northeastern Sichuan Basin deposit sand flats in shore lake—shell bank in carbonate shallow lake—storm beaches in lake slope-mud in semi-deep lake, from the north to the south, and the center of the lake basin is close to Fuling area in the east of Sichuan Basin (Zhu et al., 2017; Zhang, 2018). Based on the sedimentary facies of two single wells, a schematic diagram of the distribution of J1da sedimentary facies in the northeastern Sichuan Basin is drawn, as shown in Figure 12. Analysis shows that the semi-deep lake subfacies mainly deposit black-dark gray clayey shale. Because the location of well T1 in Puguang area is closer to the lake basin center than well Y1 in Yuanba area during the sedimentary period of J1da, the water body is deeper, the sedimentary facies is more stable, and the shale thickness is thicker for well T1. Affected by the provenance supply from Longmenshan and Micangshan, the water body energy is relatively strong in the shallow lake subfacies for well Y1 in Yuanba area, which brings more fine-grained clastic sediments, predominated by the mud and sand bank microfacies. Shell bank in carbonate shallow lake are large-scale superimposed and contiguous distributions in both Yuanba and Puguang areas (Figure 12). In addition, studies have suggested that sedimentary facies not only affected the macroscopic distribution of shale strata but also determined the microscopic distribution of organic matter in it (Jiang et al., 2013). The development of organic matter was more sensitive to changes in the depositional environment, and the deep, quiet, anoxic, and reducing environment was more conducive to its enrichment (Xiao et al., 2018). Therefore, in this study, the shale/mud microfacies in the semi-deep lake subfacies as well as the shale/mud interbedded with siltstone and shell bank microfacies in the shallow lake subfacies are the most favorable sedimentary facies of the J1da strata in the northeast Sichuan Basin, which are also the favorable factors for shale oil and gas enrichment.
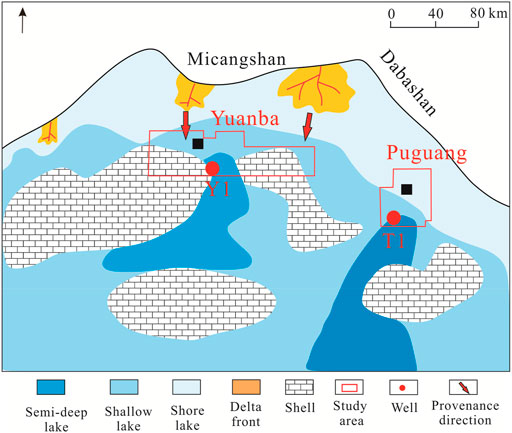
FIGURE 12. Distribution of sedimentary facies of the J1da strata in northeast Sichuan Basin (modified by Zhang, 2018).
5.2 Higher Maturity is More Conducive to Shale Oil and Gas Enrichment in Sichuan Basin
Exploration and development practice showed that most of the shale oil and gas plays that had been put into large-scale commercial development in the United States were in the hydrocarbon-generating depressions in the middle-high maturity stage (Li et al., 2019). The J1da shale maturity in the study area varies greatly (0.76–1.83%Ro), which determines the difference of hydrocarbon phases between Puguang and Yuanba area. By analyzing the fluid properties of several single wells such as well F10, it is concluded that the Dongyuemiao shale stratum is a condensate gas reservoir, the Da’anzhai shale stratum is a volatile oil reservoir, and the Lianggaoshan shale stratum is a condensate gas reservoir in the eastern Sichuan Basin. Due to the difference in maturity, the Jurassic strata in Sichuan Basin have the characteristics of oil and gas symbiosis. From the southwest to the northeast, the hydrocarbon phases are crude oil + condensate oil, condensate gas + wet gas, and wet gas + dry gas, respectively (Li et al., 2017). Complex fluid characteristics of the Jurassic in the study area have brought certain difficulties to the wells deployment, but at the same time, they have verified the diversity of oil and gas exploration, which is to the benefit of large-area contiguous development. The Eagle Ford shale plays in the western Gulf of Mexico Basin shows similar characteristics, where shale oil mainly exploits with Ro of 1.1–1.3%, in the form of light oil and condensate oil associated with wet gas, and are the primary contribution of shale oil and gas growth in the United States (Li et al., 2019). Some scholars verified that the Ro value had an obvious positive correlation with the production of shale oil and gas in the same area, and with the increase of Ro, the gas/oil ratio (GOR) of shale reservoir increased and the hydrocarbons density and viscosity decreased; thus, the flowability increased, which was conducive to petroleum production (Guo et al., 2021; Zhang et al., 2021). Moreover, a higher thermal evolution degree makes the organic pores of shale reservoirs more developed, which increases the storage space of shale oil and gas, beneficial to shale oil and gas enrichment (Guo et al., 2021). Therefore, the exploration potential of the J1da strata in Yuanba area with higher maturity is greater than that in Puguang area; the J1da strata of well Y1 should aim at shale gas, and the J1da strata of well T1 should aim at shale oil.
5.3 Thick Interval of High-Quality Shale is the Exploration Sweet Spot of Wells Y1 and T1
The above organic geochemical analysis shows that the overall organic matter abundance of J1da in the study area is overall general; the average TOC content is only about 1.2%, which is far lower than the standard of marine shale gas enrichment with TOC > 2%. Huang et al. (2018b) determined the lower limit of TOC for the oil generation of J1da2 shale strata as 1.5% by studying the relationship between TOC and S1, indicating that high TOC content was not the only prerequisite for shale oil and gas sweet spot selection (Zhang et al., 2021). For the same area, in the intervals rich in organic matter, the organic matter type and thickness are also important indicators for sweet spot evaluation. Generally speaking, for the same TOC content per unit mass of shale, the overall ranking of hydrocarbon generation potential is typeⅠ > type Ⅱ1 > type Ⅱ2 > type Ⅲ; the better the organic matter type, the greater the oil and gas generation potential, therefore, the upper section of J1da in the study area is better than the lower section. In addition, the thickness of organic-rich shale determines the resource scale of the reservoir. For example, the thickness of high-quality shale in both the upper and lower parts of the Bakken Formation in the Williston Basin are 5–12 m and widely distributed in almost the entire basin. The high-quality shale with thickness 38 m of the lower Silurian Longmaxi Formation in the Fuling Gas Field is known as a favorable target for exploration wells and commercial production. They proved the importance of finding thick intervals in shale strata (Li et al., 2019; Nie et al., 2021; Zhang et al., 2022). However, influenced by the fast-changing sedimentary facies, the distribution and thickness of organic-rich shale in the J1da strata in this study is not as stable as that of marine Silurian shale, and the strong vertical and horizontal heterogeneity makes a poor continuous distribution of high-quality shale (Zhou et al., 2020). Therefore, when selecting the favorable intervals of the J1da shale from the perspective of geochemical characteristics, the superimposed intervals with higher TOC, better organic matter type, and larger thickness should be given priority to consider. In this study, depths of 3,885–3,890 m and 3,911–3,914 m of the J1da2 shale strata for well Y1 in Yuanba area can be selected as favorable intervals, and depths of 15–33 m of the J1da shale strata for well T1 in Puguang area should be considered as the favorable interval. Of course, when selecting the target window through horizontal sections of wells Y1 and T1, more factors should be taken into account besides the favorable sedimentary facies and geochemical favorable intervals discussed in this study, such as reservoir, gas content, and logging display.
6 Conclusions
1) The organic matter abundance of the J1da shale strata in the northeastern Sichuan Basin is medium, the organic matter type is type II, and parameters from well T1 in Puguang are generally better than that from well Y1 in Yuanba area. As the burial depth becomes shallower, TOC content increases, organic matter type becomes better, and the hydrocarbon generation potential becomes better as well for both wells. The upper section of the J1da shale strata is a more favorable layer that can be evaluated as a good-general source rock. The organic matter maturity of the J1da shale strata in the northeastern Sichuan Basin varies greatly, which is in the high mature-over mature stage and priority to generate gas for well Y1 in Yuanba area, while it is in the low mature-early mature stage and preferable to generate oil for well T1 in Puguang area.
2) The organic matter provenance of J1da shale in the northeastern Sichuan Basin has typical characteristics of terrestrial-hydrophilous mixed sediments, with the terrestrial higher plants as the main source, supplemented by the input of lower aquatic organisms, but the mixed ratio of two sources is quite different for each sublayer. The J1da shale strata are formed in a freshwater lacustrine environment as a whole, and the water body during the depositional period is in a reducing-weak oxidation condition, which is favorable for organic matter forming and hydrocarbon generating. Moreover, there is saltwater lacustrine deposition in local areas and intervals. Rapid changes in depositional environment result in obvious stratification of water body and strong heterogeneity of the J1da shale.
3) The shale/mud microfacies in the semi-deep lake subfacies as well as the shale/mud interbedded with siltstone and shell bank microfacies in the shallow lake subfacies are the most favorable sedimentary facies of the J1da strata in the northeast Sichuan Basin. Higher maturity of J1da shale in Yuanba area is more conducive to shale oil and gas enrichment in Sichuan Basin, and thick interval of high-quality shale is the exploration sweet spot of wells Y1 and T1. Depths of 3,885–3,890 m and 3,911–3,914 m of the J1da2 shale strata for well Y1 in Yuanba area and depths of 15–33 m of the J1da shale strata for well T1 in Puguang area should be given priority to consider as favorable geochemical exploration intervals.
Data Availability Statement
The raw data supporting the conclusion of this article will be made available by the authors, without undue reservation.
Author Contributions
QL: Propose ideas, analyze data, and write paper. ZL: Put forward ideas and revise paper. FC: Provide core samples and some experimental data. GL: Make suggestions for some analysis. DZ: Make suggestions for some analysis. PL: Make and analyze some figures. PW: Make and analyze some figures.
Funding
This research is financially supported by the National Science and Technology Major Project (2017ZX05036004) and the China Petroleum and Chemical Corporation Technology Development Project (G5800-20-ZS-HX042).
Conflict of Interest
All authors were employed by SINOPEC and declare that the research was conducted in the absence of any commercial or financial relationships that could be construed as a potential conflict of interest.
Publisher’s Note
All claims expressed in this article are solely those of the authors and do not necessarily represent those of their affiliated organizations or those of the publisher, the editors, and the reviewers. Any product that may be evaluated in this article, or claim that may be made by its manufacturer, is not guaranteed or endorsed by the publisher.
Acknowledgments
We would like to express our sincere gratitude to the Southern Company of Exploration, SINOPEC, for providing shale samples. We are also grateful to the Experimental Research Center of Wuxi Institute of Petroleum Geology, Sinopec Petroleum Exploration and Development Research Institute, for helping us complete the experiments. Last but not least, we wish to thank the reviewers for their valuable comments that significantly improved the quality of this paper.
References
Alias, F. L., Abdullah, W. H., Hakimi, M. H., Azhar, M. H., and Kugler, R. L. (2012). Organic Geochemical Characteristics and Depositional Environment of the Tertiary Tanjong Formation Coals in the Pinangah Area, Onshore Sabah, Malaysia. Int. J. Coal Geol. 104, 9–21. doi:10.1016/j.coal.2012.09.005
Beukes, N. J., Klein, C., Kaufman, A. J., and Hayes, J. M. (1990). Carbonate Petrography, Kerogen Distribution, and Carbon and Oxygen Isotope Variations in an Early Proterozoic Transition from Limestone to Iron-Formation Deposition, Transvaal Supergroup, South Africa. Econ. Geology. 85 (4), 663–690. doi:10.2113/gsecongeo.85.4.663
Brassell, S. C., Eglinton, G., and Mo, F. J. (1986). Biological Marker Compounds as Indicators of the Depositional History of the Maoming Oil Shale. Org. Geochem. 10 (4/6), 927–941. doi:10.1016/s0146-6380(86)80030-4
Cai, X. Y., Zhao, P. R., Gao, B., Zhu, T., Tian, T. L., and Sun, C. X. (2021). Sinopec’s Shale Gas Development Achievements During the “Thirteenth Five-Year Plan” Period and Outlook for the Future. Oil Gas Geology. 42 (01), 16–27. doi:10.3969/j.issn.1672-7703.2021.01.003
Chen, Z. L., Li, S. J., and Alexander, R. (1994). Dinosterane as a Biomarker of Biogenic Origin and Sedimentary Environment. Pet. Exploration Dev. 21 (3), 60–64.
Chen, X., Zhang, M., Huang, G., Hu, G., Wang, X., and Xu, G. (2009). Geochemical Characteristics of Light Hydrocarbons in Cracking Gases from Chloroform Bitumen A, Crude Oil and its Fractions. Sci. China Ser. D-earth Sci. 52, 26–33. doi:10.1007/s11430-009-5014-8
Chen, S., Zhu, Y., Wang, H., Liu, H., Wei, W., and Fang, J. (2011). Shale Gas Reservoir Characterisation: A Typical Case in the Southern Sichuan Basin of China. Energy 36 (11), 6609–6616. doi:10.1016/j.energy.2011.09.001
Cheng, H. Y., Li, A. L., and Gong, J. M. (2008). Analysis on Evaluation Parameters of Terrestrial Source Rocks. Mar. Geology. Front. 24 (2), 7–10. doi:10.3969/j.issn.1009-2722.2008.02.002
Deng, K. L. (1992). Formation and Evolution of Sichuan Basin and Domains for Oil and Gas Exploration. Natural Gas Industry 12 (5), 7–12.
Dutta, S., Greenwood, P. F., Brocke, R., Schaefer, R. G., and Mann, U. (2006). New Insights into the Relationship between Tasmanites and Tricyclic Terpenoids. Org. Geochem. 37 (1), 117–127. doi:10.1016/j.orggeochem.2005.08.010
Gao, Z., Yang, S., Jiang, Z., Zhang, K., and Chen, L. (2018). Investigating the Spontaneous Imbibition Characteristics of Continental Jurassic Ziliujing Formation Shale from the Northeastern Sichuan Basin and Correlations to Pore Structure and Composition. Mar. Pet. Geology. 98, 697–705. doi:10.1016/j.marpetgeo.2018.09.023
Garrigues, P., De Sury, R., Angelin, M. L., Bellocq, J., Oudin, J. L., and Ewald, M. (1988). Relation of the Methylated Aromatic Hydrocarbon Distribution Pattern to the Maturity of Organic Matter in Ancient Sediments from the Mahakam Delta. Geochim. Cosmochim. Acta 52 (2), 375–384. doi:10.1016/0016-7037(88)90093-2
Gelpi, E., Schneider, H., Mann, J., and Oró, J. (1970). Hydrocarbons of Geochemical Significance in Microscopic Algae. Phytochemistry 9 (3), 603–612. doi:10.1016/s0031-9422(00)85700-3
Goodwin, N. S., Mann, A. L., and Patience, R. L. (1988). Structure and Significance of C30 4-methyl Steranes in Lacustrine Shales and Oils. Org. Geochem. 12 (5), 495–506. doi:10.1016/0146-6380(88)90159-3
Guo, X. S., Hu, D. F., Li, Y. P., Wei, X., Liu, R., Liu, Z., et al. (2016). Analyses and Thoughts on Accumulation Mechanisms of Marine and Lacustrine Shale Gas: A Case Study in Shales of Longmaxi Formation and Da’anzhai Section of Ziliujing Formation in Sichuan Basin. Earth Sci. Front. 23 (2), 18–28. doi:10.13745/j.esf.2016.02.003
Guo, X. S., Zhao, Y. Q., Zhang, W. T., Li, Y. P., Wei, X. F., Shen, B. J., et al. (2021). Accumulation Conditions and Controlling Factors for the Enrichment of Shale Oil and Gas in the Jurassic Qianfoya Formation, Yuanba Area, Sichuan Basin. Pet. Geology. Exp. 43 (5), 749–757. doi:10.11781/sysydz202105749
Haven, H. L.T., de Leeuw, J. W., Rullkötter, J., and Damsté, J. S. S. (1987). Restricted Utility of the Pristane/Phytane Ratio as a Palaeoenvironmental Indicator. Nature 330 (6149), 641–643. doi:10.1038/330641a0
He, D. F., Li, Y. Q., and Huang, H. Y. (2020). Formation and Evolution of Sichuan Multi-Cycle Superimposed Basins and Hydrocarbon Accumulation. Beijing: Science Press.
Hu, J. Y., and Huang, D. F. (1991). Theoretical Basis of China's Terrestrial Petroleum Geology. Beijing: Petroleum Industry Press.
Huang, W.-Y., and Meinschein, W. G. (1979). Sterols as Ecological Indicators. Geochim. Cosmochim. Acta 43 (5), 739–745. doi:10.1016/0016-7037(79)90257-6
Huang, D., Duan, Y., Yang, G., Yan, W. P., Wei, T. Q., Zou, J., et al. (2018a). Controlling Effect of Source-Reservoir Configuration Model on Tight Oil Enrichment in Freshwater Lacustrine Sedimentary Area: A Case Study of the Jurassic Da’anzhai Member in Sichuan Basin [J]. Acta Petrolei Sin. 39 (5), 518–527. doi:10.7623/syxb201805003
Huang, D., Duan, Y., Li, Y. C., Chen, H. B., Yan, W. P., and Dai, H. M. (2018b). Study on the TOC Lower Limit of Shale Oil and Gas of Freshwater Lake Facies: A Case Study on the Jurassic Da'anzhai Member in the Sichuan Basin. China Pet. Explor. 23 (6), 38–45. doi:10.3969/j.issn.1672-7703.2018.06.005
Jiang, Z. X., Tang, X. L., Li, W., Ji, W., Gao, X., Yuan, Y., et al. (2015). The Main Control Factors for Shale Gas Accumulation and Exploration Problems in Shale Gas of South China. Acta Geol. Sin. (English Ed.) 89 (S1), 250. doi:10.1111/1755-6724.12304_12
Jiang, Z. X., Guo, L., and Liang, C. (2013). Lithofacies and Sedimentary Characteristics of the Silurian Longmaxi Shale in the Southeastern Sichuan Basin, China. J. Palaeogeogr. 2 (3), 238–251. doi:10.3724/SP.J.1261.2013.00029
Jin, C. F., Li, S. J., Chen, W., and Cao, C. Q. (2016). Climate Changes on the Tibetan Plateau Inferred from Organic Geochemistry Records in Lake Sediments-a Case of Lake Zigetang Co. Bull. Mineral. Petrol. Geochem. 35 (4), 625–633. doi:10.3969/j.issn.1007-2802.2016.04.003
Kamp, P., and Leake, B. E. (1995). Petrology and Geochemistry of Siliciclastic Rocks of Mixed Feldspathic and Ophiolitic Provenance in the Northern Apennines, Italy. Chem. Geol. 122 (1), 1–20. doi:10.1016/0009-2541(94)00162-2
Li, D., Li, J., Zhang, B., Yang, J., and Wang, S. (2017). Formation Characteristics and Resource Potential of Jurassic Tight Oil in Sichuan Basin. Pet. Res. 2 (4), 301–314. doi:10.1016/j.ptlrs.2017.05.001
Li, M. W., Ma, X. X., Jiang, Q. G., Li, Z., Pang, X., and Zhang, C. (2019). Enlightenment from Formation Conditions and Enrichment Characteristics of Marine Shale Oil in North America. Pet. Geology. Recover. Effic. 26 (1), 13–28. doi:10.13673/j.cnki.cn37-1359/te.2019.01.002
Li, F. L., Lv, L., Ma, W. K., Diao, H., Xu, Z. Y., Bai, Y. M., et al. (2021). Geochemical Characteristics of Source Rocks in Liushagang Formation of Wushi Sag. J. China Univ. Pet. (Ed. Nat. Sci.) 45 (1), 31–40. doi:10.3969/j.issn.1673-5005.2021.01.004
Lin, H. M. (2017). Biomarker Characteristics of Lower Paleozoic Source Rocks in Chezhen Sag, Bohai Bay Basin. Pet. Geol. Exp. 39 (2), 230–237.
Lineva, M. D., Malyshev, N. A., and Nikishin, A. M. (2018). Modeling of the Thermal Evolution of the Sedimentary Cover and the Maturity of Organic Matter in the Source-Rock Sequences of Sedimentary Basins of the East Siberian Sea. Mosc. Univ. Geol. Bull. 73 (1), 13–23. doi:10.3103/s0145875218010076
Liu, S. G., Li, Z. W., Sun, W., Deng, B., Luo, Z., Wang, G., et al. (2011). Basic Geological Features of Superimposed Basin and Hydrocarbon Accumulation in Sichuan Basin, China. Sci. Geol. Sin. 46 (1), 233–257.
Liu, Z. B., Liu, G. X., Hu, Z. Q., Feng, D., Zhu, T., Bian, R., et al. (2019). Lithofacies Types and Assemblage Features of Continental Shale Strata and their Significance for Shale Gas Exploration: A Case Study of the Middle and Lower Jurassic Strata in the Sichuan Basin. Nat. Gas Industry 39 (12), 10–21. doi:10.3787/j.issn.1000-0976.2019.12.002
Liu, Z. B., Hu, Z. Q., Liu, G. X., Liu, Z., Liu, H., Hao, J., et al. (2021). Pore Characteristics and Controlling Factors of Continental Shale Reservoirs in the Lower Jurassic Ziliujing Formation, Northeastern Sichuan Basin. Oil Gas Geology. 42 (01), 136–145. doi:10.11743/ogg20210112
Long, S., Cheng, Z., Xu, H., and Chen, Q. (2020). Exploration Domains and Technological Breakthrough Directions of Natural Gas in SINOPEC Exploratory Areas, Sichuan Basin, China. J. Nat. Gas Geosci. 5 (6), 307–316. doi:10.1016/j.jnggs.2020.10.002
Lu, S. F., Xue, H. T., and Zhong, N. N. (2003). Simulating Calculation of the Variations of Organic Matter Abundance and Hydrocarbon-Generating Potential During Geological Processes. Geol. Rev. 3, 292–297.
Ma, X., Yang, Y., Wen, L., and Luo, B. (2019). Distribution and Exploration Direction of Medium- and Large-Sized Marine Carbonate Gas Fields in Sichuan Basin, SW China. Pet. Explor. Dev. 46 (1), 1–15. doi:10.1016/s1876-3804(19)30001-1
Marynowski, L., Narkiewicz, M., and Grelowski, C. (2000). Biomarkers as Environmental Indicators in a Carbonate Complex, Example from the Middle to Upper Devonian, Holy Cross Mountains, Poland. Sediment. Geol. 137 (3), 187–212. doi:10.1016/s0037-0738(00)00157-3
Marynowski, L., Zatoń, M., Simoneit, B. R. T., Otto, A., Jędrysek, M. O., Grelowski, C., et al. (2007). Compositions, Sources and Depositional Environments of Organic Matter from the Middle Jurassic Clays of Poland. Appl. Geochem. 22 (11), 2456–2485. doi:10.1016/j.apgeochem.2007.06.015
Moldowan, J. M., Seifert, W. K., and Gallegos, E. J. (1985). Relationship between Petroleum Composition and Depositional Environment of Petroleum Source Rocks. AAPG Bull. 69 (8), 1255–1268. doi:10.1306/ad462bc8-16f7-11d7-8645000102c1865d
Nie, H., Chen, Q., Zhang, G., Sun, C., Wang, P., and Lu, Z. (2021). An Overview of the Characteristic of Typical Wufeng-Longmaxi Shale Gas Fields in the Sichuan Basin, China. Nat. Gas Industry B 8 (3), 217–230. doi:10.1016/j.ngib.2021.04.001
Nishikawa, E., Yamamoto, H., and Toyoguchi, M. (2009). Estimation of Solid Paraffins Contained in Asphalts. J. Jpn. Pet. Inst. 3 (7), 547–551. doi:10.1627/jpi1958.3.547
Omokawa, M. (1982). Carbon Isotope Variations in Kerogen Types. Res. Org. Geochem. 3, 39–45. doi:10.20612/rog.3.0_39
Pang, X. Q., Li, Q. W., Chen, J. F., Li, M. W., and Pang, H. (2014). Recovery Method of Original TOC and its Application in Source Rocks at High Mature-Over Mature Stage in Deep Petroliferous Asins. J. Palaeogeogr. (Chinese Edition) 16 (06), 769–789. doi:10.7605/gdlxb.2014.06.062
Peters, K. E., and Cassa, M. R. (1994). “Applied Source Rock Geochemistry. Memoirs,” in The Petroleum System – From Source to Trap. Editors L. B. Magoon, and D. G. Dow (American Association of Petroleum Geologists Memoir), Vol. 60, 93–120.
Peters, K. E., Walters, C. C., and Moldowan, J. M. (2005). The Biomarkerguide (second edition) (II): Biomarkers and Isotopes in Petroleum Exploration and Earth History. (New York: Cambridge University Press).
Philippi, G. T. (1974). The Influence of Marine and Terrestrial Source Material on the Composition of Petroleum. Geochim. Cosmochim. Acta 38 (6), 947–966. doi:10.1016/0016-7037(74)90067-2
Qiu, N. S., Hu, S. B., and He, L. J. (2004). Theory and Application of Research on Thermal Regime in Sedimentary Basin. Beijing: Petroleum Industry Press.
Raj, S. (1979). Structural Characteristics of Vitrinites. Am. Chem. Soc. Div. Fuel Chem. Prepr. (United States), Vol. 24, 3.
Seifert, W. K., and Moldowan, J. M. (1986). Use of Biological Makers in Petroleum Exploration. Methods Geochem. Geophys. 24, 261–290.
Shu, Z. G., Zhou, L., Li, X., Liu, H., Zeng, Y., Xie, H., et al. (2021). Geological Characteristics of Gas Condensate Reservoirs and their Exploration and Development Prospect in the Jurassic Continental Shale of the Dongyuemiao Member Of Ziliujing Formation, Fuxing Area, Eastern Sichuan Basin. Oil Gas Geology. 42 (01), 212–223. doi:10.11743/ogg20210118
Tang, L., Song, Y., Jiang, S., Jiang, Z., Li, Z., Yang, Y., et al. (2020a). Organic Matter Accumulation of the Wufeng-Longmaxi Shales in Southern Sichuan Basin: Evidence and Insight from Volcanism. Mar. Pet. Geology. 120 (5), 104564. doi:10.1016/j.marpetgeo.2020.104564
Tang, L., Song, Y., Pang, X., Jiang, Z., Guo, Y., Zhang, H., et al. (2020b). Effects of Paleo Sedimentary Environment in Saline Lacustrine Basin on Organic Matter Accumulation and Preservation: A Case Study From the Dongpu Depression, Bohai Bay Basin, China. J. Pet. Sci. Eng. 185, 106669. doi:10.1016/j.petrol.2019.106669
Wang, X. L., Gao, G., Yang, H. B., Liu, G. D., Huang, Z. L., and Wei, H. Y. (2008). Research on Relation between Oil Properties and Petroleum Pool Formation of Permian in the 5th & 8th Districts, Northwestern Margin of Junggar Basin. Geol. J. China Univ. 14 (2), 256–261. doi:10.3969/j.issn.1006-7493.2008.02.017
Wang, W., Huang, D., Yi, H. Y., Zhao, R. R., Cen, Y. J., and Li, Y. C. (2019). Stratigraphic Division and Geochemical Characteristics of Freshwater Lacustrine Shale: A Case Study of Jurassic Da’anzhai Section, Sichuan Basin. Pet. Geology. Exp. 41 (05), 724–730.
Wang, R., Liu, H., Dou, L., Bai, H., and Zhang, N. (2021). Effect of Adsorption Phase and Matrix Deformation on Methane Adsorption Isotherm of Fuling Shale. J. Nat. Gas Sci. Eng. 95 (6), 104018. doi:10.1016/j.jngse.2021.104018
Wang, Q. B., Liu, R. B., Wei, X. F., Ni, K., and Gao, H. X. (2013). Geologic Condition of Shale Gas Accumulation in Continental Facies and Main Controlling Factors of Enrichment and High Production: Taking Yuanba District as an Example. Fault-Block Oil & Gas Field 20 (6), 698–703. doi:10.6056/dkyqt201306005
Xu, Q., Liu, B., Ma, Y., Song, X., Wang, Y., Xin, X., et al. (2017). Controlling Factors and Dynamical Formation Models of Lacustrine Organic Matter Accumulation for the Jurassic Da'anzhai Member in the Central Sichuan Basin, Southwestern China. Mar. Pet. Geology. 86, 1391–1405. doi:10.1016/j.marpetgeo.2017.07.014
Xu, W., Ruhl, M., Jenkyns, H. C., Hesselbo, S. P., Riding, J. B., Selby, D., et al. (2017). Carbon Sequestration in an Expanded Lake System During the Toarcian Oceanic Anoxic Event. Nat. Geosci. 10 (2), 129–134. doi:10.1038/ngeo2871
Xu, Y. L. (2015). Geochemical Characteristics and Resource Potential Evaluation of the Hydrocarbon Source Rocks in Jurassic in Puguang Region [D]. Chengdu University of Technology.
Xiao, J. L., Wei, X. F., and Li, H. J. (2018). Difference of Accumulation Conditions between Fuling Marine Shale Gas and Yuanba-Xinglongchang Lacustrine Shale Gas. Nat. Gas Exploration Dev. 41 (4), 8–17.
Xie, J., Zhang, H. M., She, C., Li, Q., Fan, Y., and Yang, Y. (2017). Practice of Geology-Engineering Integration in Changning State Shale Gas Demonstration Area. China Pet. Explor. 22 (1), 21–28.
Yang, Z., Tang, Z. X., Li, G. H., Jia, X. Y., Wu, Y. X., Chen, X., et al. (2021). Optimization of Enrichment Plays, Evaluation of Sweet Area & Section and Application of Key Technologies for the Continental Shale Strata Oil in China. Acta Geol. Sin. 95 (8), 2257–2272. doi:10.3969/j.issn.0001-5717.2021.08.001
Yu, J. Q., Liu, R., Zhang, K., and Yan, X. (2021). Organic Geochemical Characteristics and Metallogenic Conditions of Oil Shale of Lower Cretaceous Bayingebi Formation in Yin'e Basin. Glob. Geology. 40 (2), 1–13. doi:10.3969/j.issn.1004-5589.2021.02.010
Zhang, Z. H., Yang, F., Li, D. M., and Fang, C. L. (1998). Biomarker Assemblage Characteristics of Source Rocks and Associated Crude Oils in Saline Lake Facies of Cenozoic in China. Acta Sedimentol. Sin. 16 (3), 119–131.
Zhang, L. P., Huang, D. F., and Liao, Z. Q. (1999). Gammacerane-Geochemical Indicator of Water Column Stratification. Acta Sedimentol. Sin. 7 (1), 136–141.
Zhang, K., Jia, C., Song, Y., Jiang, S., Jiang, Z., Wen, M., et al. (2020). Analysis of Lower Cambrian Shale Gas Composition, Source and Accumulation Pattern in Different Tectonic Backgrounds: A Case Study of Weiyuan Block in the Upper Yangtze Region and Xiuwu Basin in the Lower Yangtze Region. Fuel 263, 115978. doi:10.1016/j.fuel.2019.115978
Zhang, Y., Du, Y., Liu, G., Li, W. H., He, S., Wang, X. Z., et al. (2021). Characteristics and Exploration Direction of Lacustrine Shale Oil and Gas in Da’ Anzhai Member of Jurassic in Sichuan Basin. Geology. China.
Zhang, K., Jiang, S., Zhao, R., Wang, P., Jia, C., and Song, Y. (2022). Connectivity of Organic Matter Pores in the Lower Silurian Longmaxi Formation Shale, Sichuan Basin, Southern China: Analyses from Helium Ion Microscope and Focused Ion Beam Scanning Electron Microscope. Geol. J., 1–13. doi:10.1002/gj.4387
Zhang, M. Z. (2018). The Development Model of Shale and the Conditions of Shale Gas Accumulation in Lower Jurassic Ziliujing Formation in Northeastern Sichuan Basin. Beijing: China University of Petroleum-Beijing.
Zheng, R. C., He, L., Liang, X. W., and Xu, W. L. (2013). Forming Conditions of Shale Gas (oil) Plays in the Lower Jurassic Da'anzhai Member in the Eastern Sichuan Basin. Nat. Gas Industry 33 (12), 30–40.
Zheng, Z. H., Li, D. H., Bai, S. S., Jia, J., Zan, X., Liu, Z. Y., et al. (2017). Resource Potentials of Natural Gas in Sichuan Basin. China Pet. Explor. 22 (3), 12–20.
Zhou, D. H., Jiao, F. Z., Guo, X. S., Guo, T., and Wei, Z. (2013). Geologic analysis of Middle-Lower Jurassic Shale Reservoirs in Yuanba Area, Northeastern Sichuan Basin. Pet. Geology. Exp. 35 (6), 596–600.
Zhou, Q., Zhang, Y., Chen, X., and Zhang, Y. M. (2014). Kinetic Study of Pyrolysis Characteristics of Coal from Inner Mongolia Region. Asian J. Chem. 26 (23), 8011–8014. doi:10.14233/ajchem.2014.16906
Zhou, X., He, S., Chen, Z. Y., Liu, P., and Wang, F. (2016). Characteristics and Controlling Factors of Source Rocks in Yanchang Formation Sequence Framework, Ordos Basin. Earth Sci. 41 (6), 1055–1066. doi:10.3799/dqkx.2016.087
Zhou, D. H., Sun, C. X., Liu, Z. B., and Nie, H. K. (2020). Geological Characteristics of Continental Shale Gas Reservoir in the Jurassic Da'anzhai Member in the Northeastern Sichuan Basin. China Pet. Explor. 25 (05), 32–42.
Zhu, Y., Hao, F., Zou, H., Cai, X., and Luo, Y. (2007). Jurassic Oils in the Central Sichuan Basin, Southwest China: Unusual Biomarker Distribution and Possible Origin. Org. Geochem. 38 (11), 1884–1896. doi:10.1016/j.orggeochem.2007.06.016
Zhu, Y. M., Hu, W., Li, Y., Hao, F., Tanglou, G., and Zou, H. (2013). Geochemical Characteristics and Origins of Typical Marine and Terrestrial Oils in the Northeastern Sichuan Basin. Acta Petrolei Sin. 34 (2), 255–262. doi:10.7623/syxb201302007
Zhu, T., Yu, L. J., and Wang, F. (2017). Comparative Analysis of the Accumulation Conditions and Development Strategies of the Marine and Lacustrine Shale Gas from the Sichuan Basin, China. Nat. Gas Geosci. 28 (4), 633–641. doi:10.11764/j.issn.1672-1926.2017.02.003
Zhu, Y. M., Hao, F., Zou, H. Y., Li, P. P., and Hu, D. F. (2021). Compositional Variations and Oil-Source Implication of Rearranged Hopanes from the Middle-lower Jurassic Source Rocks in the Northern Sichuan Basin. Acta Sedimentol. Sin. doi:10.14027/j.issn.1000-0550.2020.118
Zou, C., Du, J., Xu, C., Wang, Z., Zhang, B., Wei, G., et al. (2014). Formation, Distribution, Resource Potential, and Discovery of Sinian-Cambrian Giant Gas Field, Sichuan Basin, SW China. Pet. Explor. Dev. 41 (3), 306–325. doi:10.1016/s1876-3804(14)60036-7
Keywords: geochemistry, organic matter provenance, depositional environment, shale, Da’anzhai Member, Northeastern Sichuan Basin
Citation: Li Q, Liu Z, Chen F, Liu G, Zhang D, Li P and Wang P (2022) Geochemical Characteristics and Organic Matter Provenance of Shale in the Jurassic Da’anzhai Member, Northeastern Sichuan Basin. Front. Earth Sci. 10:860477. doi: 10.3389/feart.2022.860477
Received: 23 January 2022; Accepted: 21 February 2022;
Published: 11 April 2022.
Edited by:
Kun Zhang, Southwest Petroleum University, ChinaReviewed by:
Yangyang Wang, Ministry of Emergency Management, ChinaJunwen Peng, China University of Petroleum, Beijing, China
Ling Tang, CNOOC Research Institute Ltd., China
Copyright © 2022 Li, Liu, Chen, Liu, Zhang, Li and Wang. This is an open-access article distributed under the terms of the Creative Commons Attribution License (CC BY). The use, distribution or reproduction in other forums is permitted, provided the original author(s) and the copyright owner(s) are credited and that the original publication in this journal is cited, in accordance with accepted academic practice. No use, distribution or reproduction is permitted which does not comply with these terms.
*Correspondence: Qianwen Li, 15810434649@163.com