- 1Cluj-Napoca Department, Emil Racovita Institute of Speleology, Romanian Academy, Cluj-Napoca, Romania
- 2Romanian Institute of Science and Technology, Cluj-Napoca, Romania
- 3Centro Nacional de Investigación Sobre la Evolución Humana, CENIEH, Burgos, Spain
- 4Institute for Environmental Studies, Charles University Prague, Prague, Czechia
- 5Faculty of Geology and Geophysics, University of Bucharest, Bucharest, Romania
- 6Institute of Geological Sciences, Polish Academy of Sciences, Warsaw, Poland
- 7Department of Geospeleology and Paleontology, Emil Racovita Institute of Speleology, Romanian Academy, Bucharest, Romania
- 8Department of Biosciences, Centre for Ecological and Evolutionary Synthesis, University of Oslo, Oslo, Norway
Over the last thousands of years, human impact led to significant changes in the landscape, with impacts on the environment and the functioning of the ecosystems. We assumed that even small human settlements developed around water bodies might have a substantial impact that surpasses the natural cycles of climate change in an area, especially linked to forest cutting. We have chosen a temporary lake in the Romanian Carpathians as temporary lakes are overlooked in paleoclimatic studies, although they have potential as hotspots for paleoenvironmental research studies. Zăton Lake has exposed riverbank sediments dated with radioactive lead and optical stimulated luminescence techniques, analyzed for sediments and paleomagnetism, and sampled for fossil Oribatida mites. The results show that for the last 2,200 years, natural cycles of warm and cold periods changed the oribatid communities around the lake, with warmer cycles of rich fauna alternating with poor fauna during colder periods. However, in the last few centuries, the increased human occupation in the area replaced the ecologically diverse fauna with eurytopic taxa, more similar to the colder periods of the environmental evolution around the lake. Even if the human occupation around the lake was negligible, probably a few hundred to ∼2,500 people as in the present, the impact on the area is drastic, with ecologically nonspecific species becoming dominant. In conclusion, even small and temporary water bodies can contain unique and valuable information on past histories of climate change and human impacts. At regional scales, the combined effects of climate change and long-term human impacts in local areas can have deleterious effects on invertebrate species and communities.
Introduction
Human land use during the Holocene has led to some drastic changes in soil stability and productivity, the functioning of river ecosystems, and lateral transfer of sediments and carbon, with possible effects also on the local climate (Montgomery, 2007; Borrelli et al., 2017; Wang et al., 2017). Vegetation clearance and burning and agricultural and settlement expansion have led to increasing regional soil erosion rates (Arnaud et al., 2012; Roberts et al., 2018). Jenny et al. (2019) have found that a significant portion of the Earth’s surface had already shifted to human-driven soil erosion 4,000 years ago, following land deforestation. Land-use changes also impact the aquatic ecosystems that provide unique communities and numerous services. Their vulnerability is also enhanced because most of the world’s human populations develop around water bodies (Williamson et al., 2008). Lake sediments have the potential of providing excellent archives for long-term human impacts, although only recently, research on lake sediments switched from generating climate records to detecting human impacts caused by the exploitation of both land and water (Dubois et al., 2018). For many European lakes, the pristine conditions pre-date the forest clearance and introduction of agriculture between the Neolithic and Bronze Age with an intensification caused by industrialization and population growth in the 19th century (Bennion et al., 2010). The paleolimnological analysis in northwestern Europe considered human impact minimal before the pre-industrial period (1800–1850 A.D.), a period which is a reference for the lack of impacts (Bennion et al., 2010; Rose et al., 2011; Battarbee et al., 2012; Bennion et al., 2012; Bennion et al., 2012). However, more recently, research has shown that significant metal pollutions from 600 BCE onward occurred in the Balkans, with gradually increasing values in metals of anthropogenic origin until a peak at ∼1,620 CE (Longman et al., 2018). This pollution includes a prominent, likely medieval lead peak and extended periods of metal production in the region after the Roman occupation. These early peaks were followed by recent (1850–present) enrichment in all elements.
Nevertheless, the separation of human impacts from other natural causes, such as climate change or other environmental signals, varies significantly between sites and can be subject to false interpretation (Bennion et al., 2010). For example, climate change impacts on freshwaters can be similar to the effects of eutrophication (Jeppesen et al., 2014). The use of lake proxies to cover at least some of the important climate changes that preceded the industrial impacts of the 19th century can overcome such erroneous interpretation and other constraints of paleolimnological studies given by a poor dating control and low preservation of the appropriate proxies. By studying sediments from caves using a multi-proxy approach, we found that these can provide paleoclimatic and paleoenvironmental archives for periods poorly covered by the surface sediments and offer biological proxies in a good state of preservation (Moldovan et al., 2011, 2016).
Temporary lakes are a neglected category of water bodies because they are not stable water and food sources. They are also neglected by studies on climate change and human impact, even if they have been recognized important in carbon processing (Morris et al., 2013; Catalan et al., 2014; Bartolotti et al., 2016). Temporary lakes develop in shallow depressions with varying surfaces and experience dry periods of various lengths, with occasionally predictable onset and duration.
Zăton Lake (Southern Carpathians, Romania) has exposed riverbank sediments during periods of low water and a small and stable human population in the surrounding basin that dates to at least the 14th century. A preliminary investigation on short sequences of sediments deposited in the meanders of Bulba Cave (Southwestern Carpathians) provided calibrated radiocarbon ages from 1671 to 1995 A.D. (unpublished data). As the cave belongs to the karstic system connected to Zăton Lake, we decided to concentrate our attention on the latter to understand the evolution of the subterranean system. Zăton Lake is temporary, with alternating regimes of water accumulation and slow drainage, and it is a source of sediments for the cave system. The scope of this article was to study the temporal dynamics and resilience of Zăton Lake by the combined effects of human impacts and climatic changes using sediments and fossil mites (Acari: Oribatida) as paleoenvironmental indicators. We have chosen this simple system for our investigation with few known environmental data to verify the assumption that human impacts prevail over climate change, at least in small systems with low resilience. The stratigraphic and quantitative studies on oribatid mites are fewer than those on insects and mollusks due to their more recent recognition as paleoecological indicators and the much lower number of specialists (Solhøy & Solhøy 2000). Nevertheless, recent studies have shown their presence even in very old karstic sediments and their important paleoenvironmental information when cross-correlated with coeval proxies (Moldovan et al., 2011, 2016).
There are studies showing changes in the lake sediments worldwide caused by nutrient enrichment starting with the Bronze Age but mostly of increased acidification after the 19th century and decreased total organic carbonic carbon over the last 200 years (Bennion et al., 2010). Renberg (1990) showed that natural acidification over the early and middle Holocene was followed by a period of elevated pH associated with forest clearance and early agriculture during the Bronze Age and two phases of recent acidification associated with re-acidification caused by land abandonment, forest regeneration, and acid deposition. In a high-altitude lake (above 2000 m a.s.l.) in the Southern Carpathians, Vincze et al. (2017) found evidence of human impact only after 4,200 cal yr B.P., during the Bronze Age. At a lower altitude, in a northern lake in Romania, Peters et al. (2020) found the first anthropogenic impacts after 5,000 cal yr B.P., slightly stronger between 2,300 cal yr B.P. and the 12th century A.D., with large scale forest clearings in the lowlands, foothills, and intensified agriculture. The impact intensified during the Iron Age and the Medieval Period, around 2,600 cal yr B.P. For the last 100–150 years, several natural and anthropogenic drivers of the changes observed in lake sediments or peat bogs were mentioned for sites in Romania (Feurdean et al., 2013, 2015; Haliuc et al., 2019, 2020) in northern, northeastern, and northwestern Romania.
Material and Methods
Study Area
Located in the northeastern part of the Mehedinți Plateau, in the Southern Carpathians (44°58′24.6″N, 22°45′20.8″E; Figure 1), the Zăton basin (19.5 km2) is the largest temporary karst lake in Romania. Its watershed lies at elevations ranging between 810 and 327 m a.s.l. The lower point corresponds to a sink located at the limit between the impervious and karstic deposits. It is a temporary lake formed in a semi-closed depression, flooded during periods with high precipitation or snow melting, and formed at the contact between impervious and karstic deposits. Its slopes are used for agriculture and cattle farming. The lake can extend more than 2.5 km, while its depth may exceed 20 m. Granitic and metamorphic formations of the Danubian Unit and impervious sedimentary deposits (Upper Cretaceous; Miocene), especially including shales and siliciclastic rocks, form the lake bed.
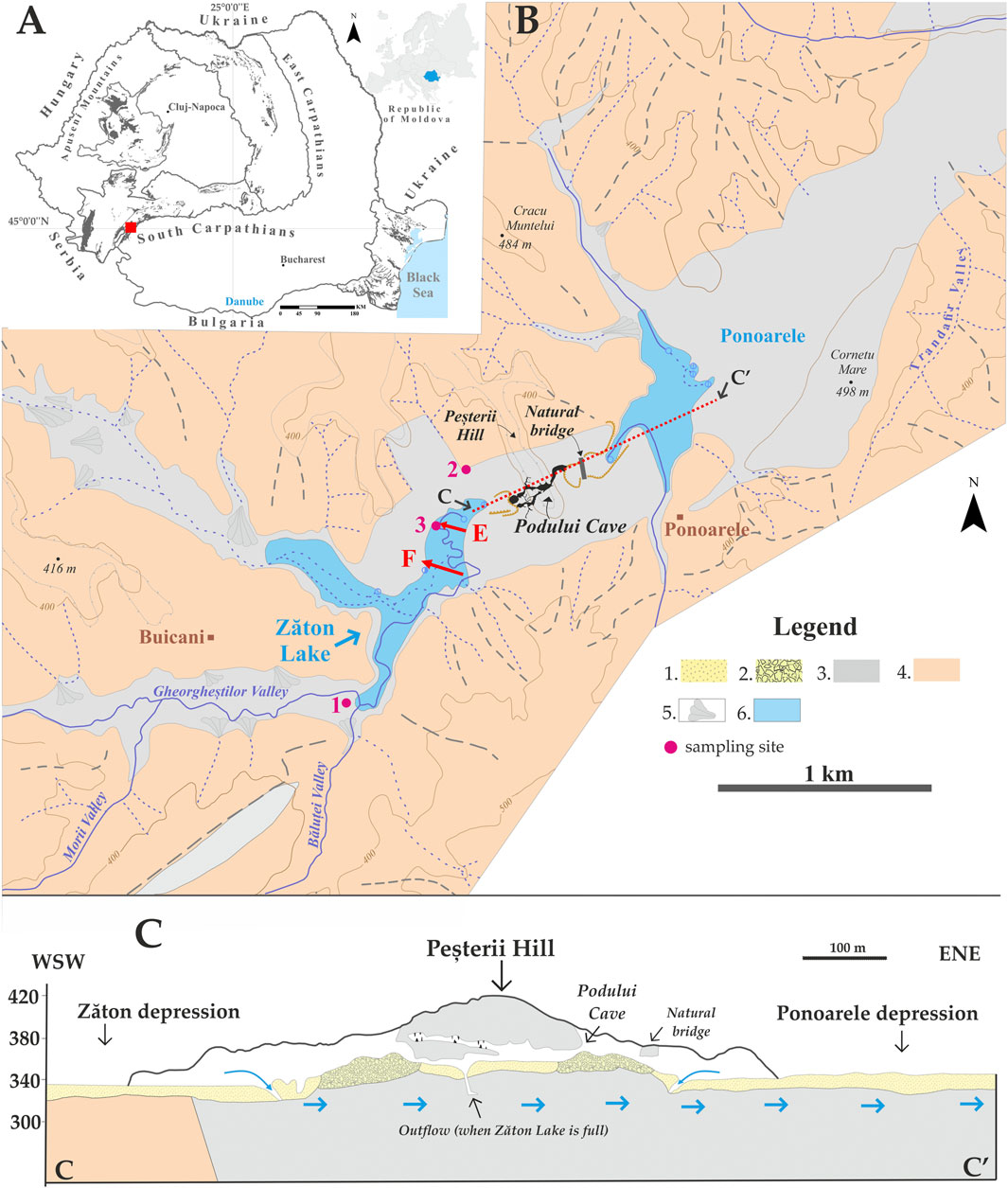
FIGURE 1. (A) Location of the Zăton Basin in the S.W. part of the Romanian Carpathians. (B) Zăton Lake and the connected cave system. (C) Schematic representation of the long section of the cave system and its functioning when Zăton depression fills with water. The E and F positions of the two geophysical profiles are marked with red arrows. 1 = quaternary sediments; 2 = scree debris; 3 = limestone; 4 = impervious rocks; 5 = talus fan; and 6 = lake. (B,C) Modified from Goran, 1978.
The lake is supplied by the Valea Mare and Valea Gheorgheştilor streams and small tributaries. During the dry season, the lake dries down, and the scarce stream waters sink into one or more swallet holes on contact with a stripe of massive limestone of the Upper Jurassic–Lower Cretaceous. The waters drain through karst conduits in the lower level of the Podului Cave (∼750 m long) toward the west to further reach the passages of Bulba Cave (>5 km-long). The sink and karst conduits cannot drain all the flow during the wet season, and a reservoir forms upstream.
The water accumulation strongly depends on the amount of precipitation and the drainage capacity of the karst conduits, with years when the lake does not form at all. Maximum water levels are usually recorded during the spring and late autumn. The main stream has a meandering course incised within the terrace deposits at low water levels. Short tributaries have steeper and more rugged profiles, typical for ravines (Figure 2).
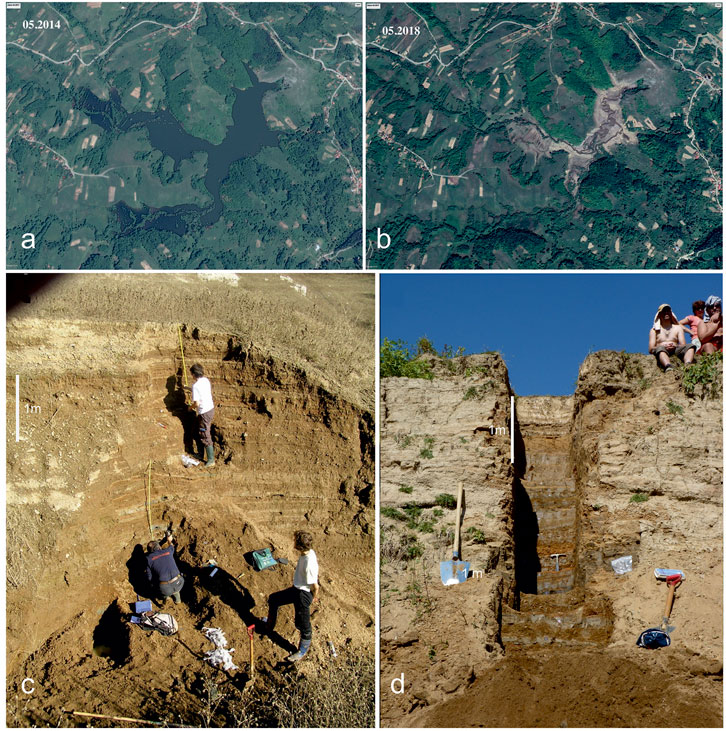
FIGURE 2. Google Earth images of Zăton Lake taken in May 2014 (A) and May 2018 (B), with and without water, respectively. The pictures shown as follows were taken in November 2010 of the Zaton2 profile (C) and in June 2013 of the Zaton3 profile (D).
The climate is temperate continental with a mean annual temperature of 9.1°C, a mean summer temperature of 19.2°C, and a mean winter temperature of −1.1°C. Annual mean precipitations are 670 mm (Fick and Hijmans, 2017). Southwestern Romania is located in a transition zone with a regional climate dominated by the influence of the Carpathian mountain topography and westerly driven circulation patterns (Micu et al., 2015). Most studies display substantial inter-site variability to indicate the complexity of climate in the Romanian Carpathians in southeastern Europe (Roberts et al., 2012; Longman et al., 2017).
The vegetation around the lake is represented by scarce patches of forest and shrubs with rocky surfaces covered with grass and agricultural fields. The forest is represented mainly by deciduous trees with some planted coniferous.
Analyzed Sections
Three profiles (Figures 1, 2) were sampled in the Zăton basin. Zaton1 was a ∼4 m-long core drilled on a small terrace located between Valea Mare and Valea Gheorgheștilor. The borehole intercepted the local aquifer, and part of the samples (mainly sands) were lost and highly disturbed. The retrieved samples were very recent sediments; hence, Zaton1 was used as a reference for modern sediments, but it will not be used in this study.
Zaton2 was a trench excavated within a natural outcrop on a left-hand side tributary of the main stream, immediately upstream of the sink of the lake. It is mainly composed of coarse sediments and has a thickness of ∼4 m.
Zaton3 was another trench excavated within a meander inner bank of the main stream. This 4.8 m-thick section includes various sediments ranging from clays through gravels. Both Zaton2 and Zaton3 were sampled during the dry season at low water levels.
Sedimentary Facies Analysis
In the field, Zaton2 and Zaton3 sections were described (Figures 2C,D), following the principles of facies analysis (Walker and James, 1992; Miall, 1985, 1996). These included the separation and description of the facies, interpretation of sedimentary processes, and separation and interpretation of facies associations in terms of the geometry of the fluvial and lake sedimentary architecture.
Geophysical Measurements
Resistivity methods calculate the electrical apparent resistivity distribution in the subsoil (Daily and Lytle, 1983). Integrated resistivity and ground-penetrating radar (GPR) surveys have been widely used to highlight, at different scales, the heterogeneity and morphology of fluvial deposits (Kostic and Aigner, 2007; Wozniak et al., 2018; Yogeswar et al., 2019), a key factor for groundwater flow modeling in valley-fill deposits. The GPR method is a high-resolution technique that uses high-frequency electromagnetic pulses to map shallow subsurface contrasts in dielectric properties (Jeffy et al., 2019). Both methods allow a noninvasive investigation of the sedimentary units in the near subsurface.
Vertical electrical soundings undertaken in profiles E and F (Figure 1B) were performed to characterize the resistivity variation within the Zăton infill deposits. For both profiles, the maximum depth of investigation was about 26.67 m. An ABEM SAS 1000 resistivity-meter with a Schlumberger type array was used for data recording. Along with profile E, a GPR profile was recorded using a GSSI Sir 3,000 system with a 200-Mhz antenna. The acquisition parameters for the resistivity survey were carefully established to increase the signal/noise ratio. The error limit between the repeated measurements was set to 0.2%, and for each measurement, a number between minimum 1 and maximum 4 repetitions was automatically performed in case the error limit was not reached. The GPR acquisition parameters were chosen after performing several tests on site. A total of 1024 samples/scans were used to increase the vertical resolution and a time window of 50 ns. The chosen value for the dielectric constant was 8, and the number of scans/units was set to 60. The calibration of the wheel was realized at a distance of 20 m.
In order to emphasize the resistivity distribution in the subsurface, the acquired resistivity data were inverted by using Res2DInv (Loke and Dahlin, 2002). The inversion routines are based on the smoothness-constrained least-squares method (Silvester and Ferrari, 1990; Loke and Barker, 1995), and the forward resistivity calculations were executed by applying an iterative algorithm based on the finite element method.
GPR data were processed with RADAN 6.6 (radar data analysis) provided by GSSI, and after the time-zero offset correction, the usage of a horizontal high pass filter (background removal) was involved in order to remove the low-frequency features. The multiple reflections caused by radar energy reverberations were attenuated using a deconvolution filter. The penetration depth was limited to 3m, but features below the saturated zone could not be detected.
Paleomagnetism of the Zaton3 Section
Paleomagnetic analysis was possible only on the Zaton3 profile due to its fine sediments in opposition to the coarser sediments of Zaton2.
For rock-magnetism, samples were collected in plastic cylinders (∼11 cm3) specially designed to avoid the rotation of the sample during sampling or measurements. The cylinders were oriented using a magnetic compass corrected for the local declination, excavated, capped, and packaged to avoid the loss of humidity during the transport. We sampled only undisturbed horizontal fine sediments (clay or silt), which allowed us to press the cylinders into the clean face of the outcrop. In the laboratory, the samples were kept in a refrigerator to preserve the original humidity as much as possible.
In the Paleomagnetic Laboratory of the University of Bucharest, we analyzed the structure of natural remanent magnetization by alternating field (AF) demagnetization. AF demagnetization was performed using an LDA-3A–A.F. demagnetizer (AGICO) in several steps up to a maximum of 100 mT. After each demagnetization step, remanent magnetization was measured using a JR6 spinner magnetometer (AGICO). Demagnetization results were analyzed on orthogonal demagnetization diagrams (Zijderveld, 1967), and magnetization components were isolated by principal component analysis (Kirschvink, 1980) by Remasoft 3.0 software (Chadima and Hrouda, 2006). The line fits were based on the following restraints: (1) a minimum of 4 demagnetization steps; (2) the line fit was anchored to the origin; and (3) the maximum angular deviation was <10°. From 30 samples, 26 samples gave results that fit these criteria. The typical results are presented in the Supplementary Material.
Dating of the Profiles
Optically stimulated luminescence (OSL) and 210 Pb methods were used for age determination of the sediments of Zaton2 and Zaton3, respectively. 210Pb isotope with a half-life of 22.2 years was used to estimate sediment rates for the last 100 years on the Zaton2 section. The OSL method was used for sediments that were presumed to be older than 100 years.
For 210Pb, samples taken from section Zaton2 were sealed in plastic bags and stored for at least 28 days prior to measurements. The measurements were performed at the Institute of Geological Sciences, Polish Academy of Sciences, Warsaw, Poland. The activity of unsupported (allogenic) 210Pb was calculated by extraction of the supported 210Pb activity from the total activity of 210Pb. The constant rate of supply (CRS) model was applied to age calculation (Appleby, 2001). An age-depth function was calculated using the randomization method and was fitted using the LOESS procedure (Clevland and Devlin, 1988).
Sediment samples for OSL dating were collected by hammering 25 cm-long, opaque plastic tubes (20 cm) into the sediment sections under no-light conditions. The measurement protocol was the single aliquot regeneration (SAR) applied on quartz (Murray and Wintle, 2003; Wintle and Murray, 2006). The OSL dating was performed at the Dating and Luminescence Dosimetry Laboratory, Babes-Bolyai University, Cluj-Napoca, Romania.
Fauna Sampling
For fossil invertebrates, a total of 22 samples were taken from Zaton2 and 15 samples from Zaton3 sections and analyzed. The selection of samples was made according to the succession of sedimentary layers by sampling each possible layer. Approximately 1 kg of sediment was taken from each sampling point and placed in sealed and labeled plastic bags. In the laboratory, the samples were kept in 10% KOH for 30 min and washed successively through sieves of 250 μm, 125 μm, and 40 μm with filtered water. Sub-samples for each sieve dimension were examined separately under an Olympus SZX2 stereomicroscope in 90° alcohol, and each specimen was identified under an Olympus BX51 microscope. Identification of the species was carried out following the specific methods for Oribatida mites (Weigmann, 2006).
For the ecological characterization of the identified oribatids, the following references were used: Weigmann and Kratz (1982), Lytle (1983), Schatz (1983), and Miko (2016).
Mites have been used in paleoclimatic and paleoenvironmental studies, especially in the last decades (Krivolutsky and Druk, 1986; Erickson, 1988; Elias, 1994; Coetzee and Brink, 2003; Erickson et al., 2003; Krivolutsky and Sidorchuk, 2003; Solhøy, 2001; Erickson and Platt, 2007; Moldovan et al., 2011, 2016). The choice of oribatid mites for paleoenvironmental studies has several rationalities: (i) abundant in karstic sites, such as the Zăton Lake basin; (ii) narrow ecological niches reflecting the paleoenvironments thoroughly; (iii) flightless, reflecting the structure of past in situ communities; (iv) trophically diverse and, thus, have a high species diversity; and (v) very sensitive to habitat evolution and various kinds of disturbance, including chemical pollution by metals or synthetic products, land use, management, and fires (Van Straalen, 1998; Behan-Pelletier, 1999; Behan-Pelletier & Newton, 1999; Solhøy & Solhøy, 2000; Fountain & Hopkin, 2001; Henig-Sever et al., 2001; Lindo & Visser, 2004; Migliorini et al., 2005; Migliorini, 2009; Erdmann et al., 2007).
Results
Sediment Thickness and Distribution
The thickness of the sedimentary filling and its boundary with the bedrock were estimated using the inverted resistivity sections, which are displayed and described in Supplementary Figure S1.
Facies and Facies Associations, Processes, and Environmental Evaluation
Following the principles of facies analysis, lithological logs were drawn for Zaton2 and Zaton3 sections, allowing for the description of five sedimentary facies and two types of facies associations (Figure 3) based on grain-size characteristics (Supplementary Material), sedimentary textures/structures, and thickness.
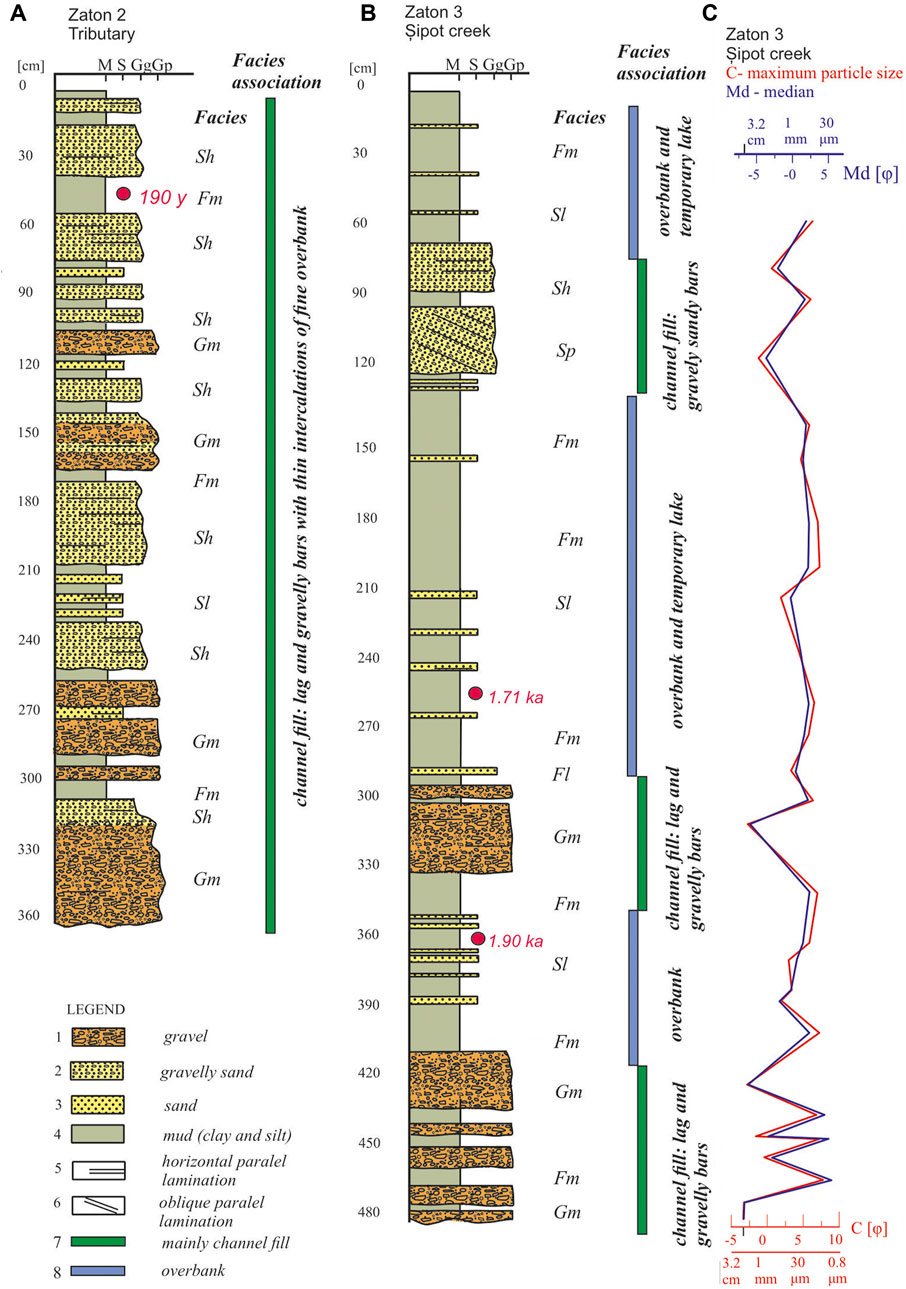
FIGURE 3. Sedimentological logs of Zăton Lake. (A) Zaton2 (see Figure 1E for location). (B) Zaton3. (C) The distribution graph of depth and the granulometric parameters C (maximum particle size) and median (Md). The grain size categories on the top of logs: M–mudstone, S–sand, Gg–gravel (granules: 2–4 mm), and Gp–gravel (pebbles: 4–64 mm). The facies codes on the right side of the log are described in the text: Gm, Sh, Sp, Fl, and Fm.
Massive or crudely stratified gravel (Gm) is characteristic of the Zaton2 profile but was also found in the lower part of the Zaton3 section. It comprises a layer of decimeter and centimeter thickness of poorly sorted gravel. The largest clasts reach 7–10 cm. The shape of the clasts is mainly sub-angular or sub-rounded. The internal structure is massive, sometimes with normal grading or horizontal parallel stratification. Imbrication of larger clasts has rarely been observed. Occasional changes from clast-supported to matrix-supported have been encountered. The matrix consists of sand or, sometimes, mud. This facies suggests a bed load of higher density tractive stream flows. They are typical for alluvial bars (Miall, 1985, 1996) but also for the distal parts of the alluvial fans.
Horizontally stratified sand and gravelly sand (Sh) were abundant in the section of Zaton2. In Zaton3, it was encountered as decimetric layers toward the top. It includes sand, sometimes with granular gravels (2–4 mm). The clasts rarely exceed 1 cm in diameter. It shows an organized character, laminated or stratified parallel to the general stratification of the sedimentary filling of the basin. This facies suggests the bottom load of tractive currents, characteristic of sandy river bars (Miall, 1985). Planar stratified gravelly sand (Sp) was found only once in the upper part of the Zaton3 section. It has a thickness of 20 cm. It consists of coarse, poorly sorted sand and coarse clasts of gravel. The internal structure was organized and tabularly oblique stratified. In a lower flow regime, it is a tractive facies specific to river bars. Small scale massive and laminated sand (Sl): this facies subordinated as the frequency was met in the median part of the basin Zaton3 section. It is associated with fine facies (Fm). It consists of sub-centimeter layers of sand from coarse to fine with different structures, massive or parallel laminated, and rarely with ripples on the top. These are sandy sheets in the overbank or alluvial plain deposits formed during floods (Miall, 1985). Massive silty mud or mud (Fm) is a facies that dominates the middle part of the Zaton3 section. It is composed of clays and silt with a massive structure. The layers are usually thick in centimeters but sometimes may exceed 10 cm. The facies suggest suspension settling processes on the alluvial plain during occasional floods.
The described facies can be associated in two categories. Association I consists of coarser facies (Gm, Sh, and Sp). It constitutes the main part of the Zaton2 profile and the lower part of the Zaton3 section. It is interpreted in terms of gravelly and sandy bars of river channels. Association II consists of the fine facies (Fm and Sl). The association dominates the middle and upper sectors of the Zaton3. It suggests flooding and overflow processes and suspension settling during lake stages (Supplementary Figure S2).
Sedimentary Evolution and Depositional Conditions
From both macroscopic geomorphological observations and analyses, it is clear that in the history of sediment filling of the Zăton depression, the fluvial phases alternated with lacustrine phases. Thus, periods of accumulation alternated with periods of erosion, and for the last 4 m analyzed in the vicinity of the top, next to the Zaton3 section, fluvial facies, canals, ridges (overbank), and finer lake facies were identified (Figure 3). The control factors must have been the climatic and hydrological regime, the position, and the width of the cave system siphons.
The GPR survey (Supplementary Figure S3) proved to be a helpful tool for characterizing the spatial distribution of the depositional elements, their geometry, and their bounding surface within the vadose zone. The high conductivity saturated zone of the sediment filling of the lake acted as a shield for the radar waves; therefore, the features below the vadose zone could not be detected due to the high attenuation of the signal.
The high amplitude reflectors, which can be vaguely observed also below the saturated zone, are present on the radargram between X.T. = 0 m and X.T. = 25 m in the southeastern part of the profile and between X.T. = 80 m and X.T. = 100 m in the northwestern part of the profile, having a concave-up shape, and can be associated with buried channels filling depositional elements. These high amplitude reflectors bound packages of parallel or oblique reflectors having low lateral continuity, which may be associated with different cycles of the channel filling. The middle part of the radargram shows reflectors having a low inclination and moderate amplitude. We can remark the alternation in the depth of continuous reflectors with reflectors having poor lateral continuity. The latter is being faded by the presence of other reflectors and may be associated with finer overbank, alluvial, or lacustrine facies, characterized by low angle cross-stratification alternation of gravel to fine grain, thus highlighting the alternation of fluvial phases with lacustrine phases.
Age Control of the Sections
Zaton2
The total specific activity of 210Pb ranged from 54.8+/−1.2 Bq kg-1 at the sediment surface to 0.14 +/– 0.28 Bq kg-1 at 56 cm (Figure 4A). The calculated activity of supported 210Pb was 0.30+/−0.25 Bq kg-1, and the supported lead level was reached at 40 cm. The age-depth model (Figure 4B) showed that the uppermost 50 cm of the sediment sequence was deposited during the last 190 years.
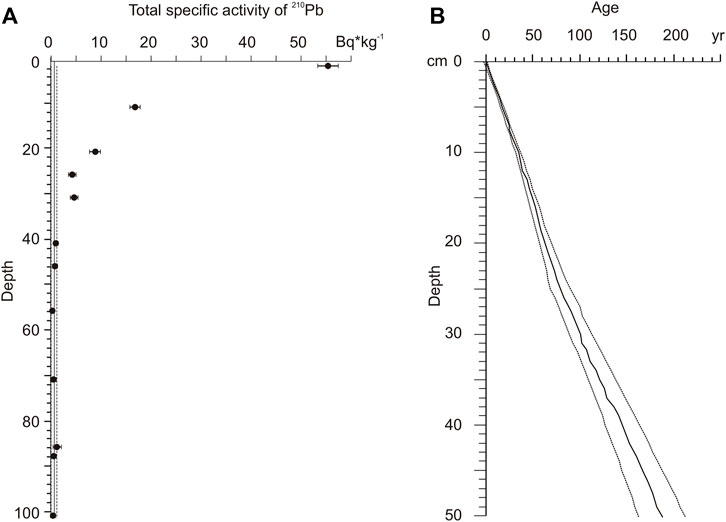
FIGURE 4. (A) Total specific activity of 210 Pb in sediments of Zaton2; the solid line is a calculated value of supported 210 Pb. (B) Age-depth model (solid line) with the 2SD confidence band (dashed lines) for the Zaton2 sediment sequence based on 210Pb dating.
Zaton3
For further information on the age of the sediments, we used a paleomagnetic record (Figure 5). We have obtained two reliable OSL ages at a depth of 265 cm (1.71 ± 0.17 ka) and 360 cm (1.90 ± 0.20 ka).
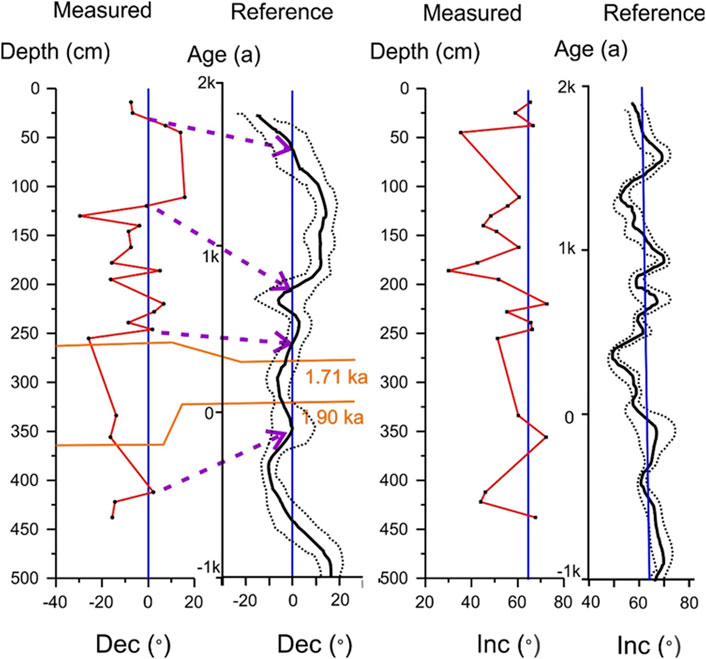
FIGURE 5. Paleomagnetic dating of sediments in the Zaton3 section using the correlation of the measured declinations and inclinations (Dec; Inc.; red lines) with the reference paleosecular variation curve for Bulgaria (Kovacheva et al., 2014) based on the Bayesian approach (black line = reference curve; dotted lines = confidence limits). Blue lines mark expected declination and inclination based on the geocentric axial dipole hypothesis. OSL ages are marked with orange lines. Dotted purple lines mark suggested correlation points.
Paleomagnetic Dating
The characteristic paleomagnetic directions at the sampling site are presented in Figure 5, separately for declination and inclination. The typical demagnetization results are presented in Supplementary Figure S4. These data were compared for paleomagnetic dating with the paleosecular variation curve from Bulgaria (Kovacheva et al., 2014), the nearest regional reference curve (∼300 km south of the study site). Comparing the measured inclination and the expected declination, it is evident that the measured inclination is shallower than the expected inclination based both on the reference curve and the geocentric axial dipole hypothesis. Such inclination shallowing can happen during sediment deposition related to factors such as particle shape, viscosity, pH, and current shear or during compaction of sediments (Li and Kodana, 2016). Due to this inclination shallowing, we mainly used the declination values to date the sediments. Assuming quasi-continuous sedimentation produced by seasonal flooding episodes, we have identified similar oscillations on the measured and reference declination curves. The correlation of inflection points around zero declination between these two curves is marked with dotted arrows in Figure 5. Such correspondence agrees with the two available OSL ages. Since the sampling density was not uniform along the section due to the presence of coarse sediments, this time model must be regarded as a crude one. Based on this preliminary time model, we estimated that the age of the sediments is between the present and ∼ 2.2 ka for the sampled section.
Fossil Oribatid Mites
The Zaton2 section had 136 individuals belonging to 40 species (Supplementary Table S1), with only three species having more than 10 individuals (Table 1). Two layers were richer in individuals, located in the lower part of the section with Zygoribatula exarata as the most abundant species. Along with the profile, the layers with fossils were cyclically intercalated with layers without fossils (Supplementary Table S1).

TABLE 1. Identified Oribatida in the Zaton2 section (Supplementary Table S1) for species with more than 10 individuals; most abundant depths are vertically marked in gray. Color legend: yellow = dry/xerophile, dark blue = halotolerant, and light blue = open area.
The Zaton3 section had 742 individuals belonging to 89 species of oribatid mites, with 23 species represented by more than 10 individuals (Table 2; Supplementary Table S2; Figure 6). Two principal successions of layers in the section were especially rich in fauna, between 116 and 200 cm and between 290 and 439 cm in depth. Tectocepheus velatus, a cosmopolitan and eurytopic species, was the most abundant species, with 182 individuals in the entire section. In abundance was also Microppia minus, a forest generalist present in most of the section, Opiella nova, eurytopic, and Punctoribates hexagonus, living in moist habitats and which was only found in the lower half of the section.
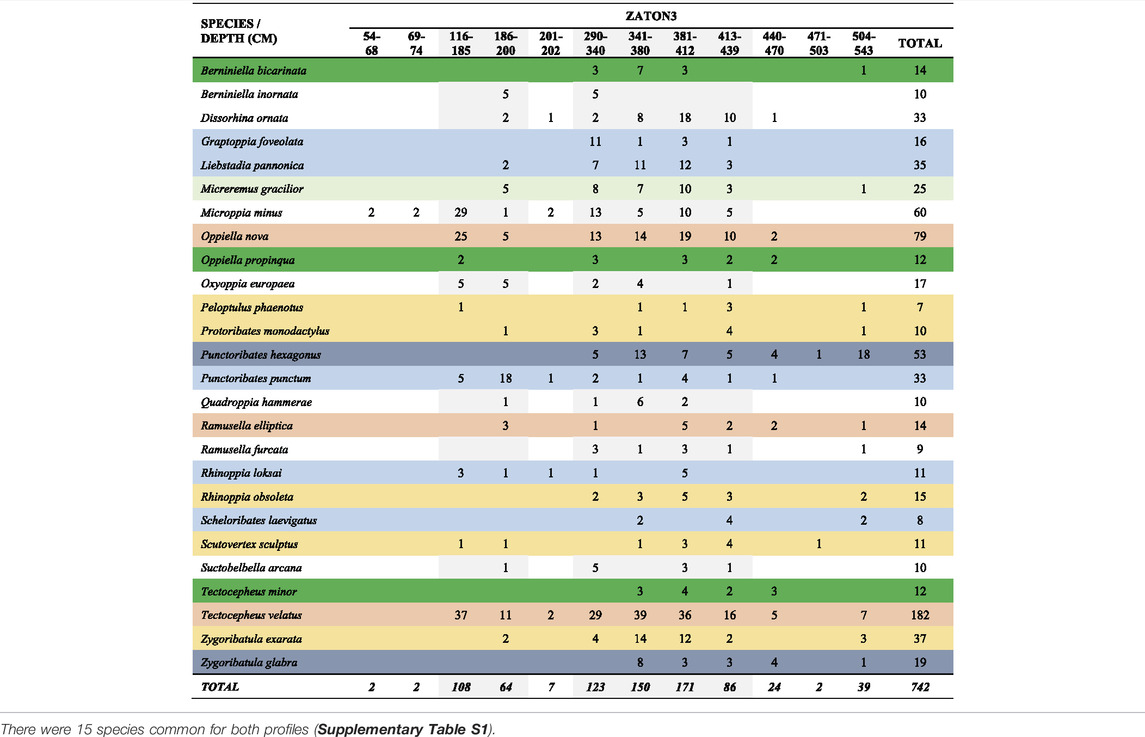
TABLE 2. Identified Oribatida in the Zaton3 section (Supplementary Table S2) for species with more than 10 individuals; most abundant depths are vertically marked in gray. Color legend: dark green = forest, light green = shrub, light red = eurytopic, yellow = dry/xerophilic, dark blue = halotolerant, light blue = open area, and white = nonspecific.
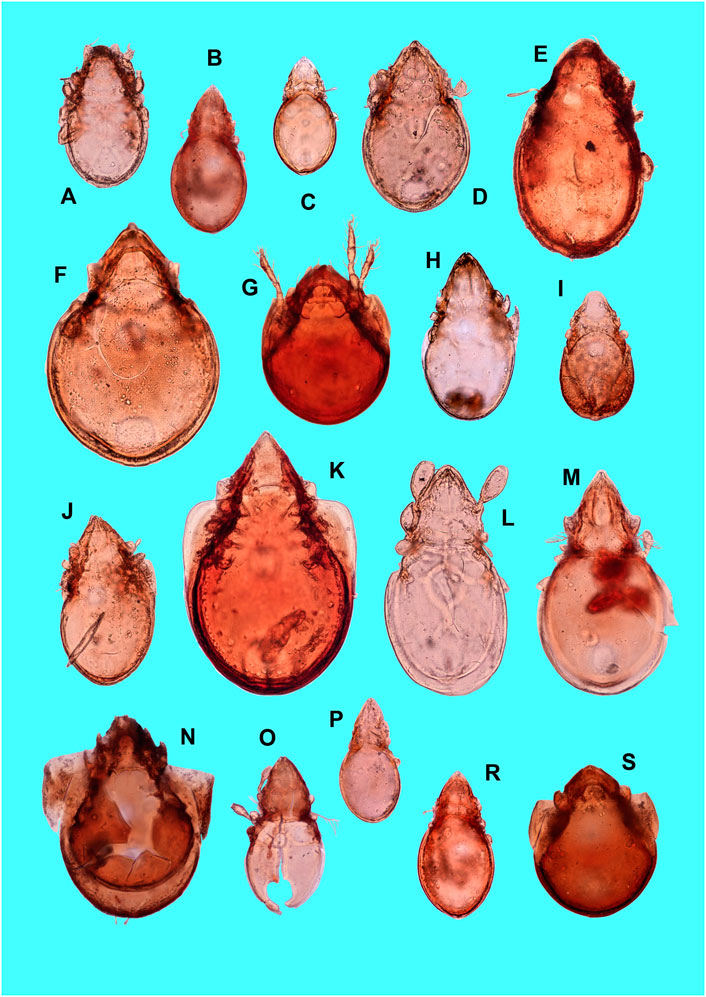
FIGURE 6. Some of the identified oribatid fossils in Zăton Lake sediments. (A) Tectocepheus velatus; (B) Oppiella nova; (C) Dissorhina ornata; (D) Zygoribatula exarata; (E) Scutovertex minutus; (F) Zygoribatula undulata; (G) Punctoribates hexagonus; (H) Protoribates monodactylus; (I) Micreremus brevipes; (J) Liebstadia pannonica; (K) Scheloribates laevigatus; (L) Eueremaeus sp.; (M) Ctenobelba sp.; (N) Peloptulus phaenotus; (O) Ramusella insculpta; (P) Confinoppia confinis; (R) Oppiella uliginosa; (S) Punctoribates punctum.
Some of the levels in the Zaton3 section were very poor in fossils, that is, the upper part down to 75 cm, a fine clay layer at −200 cm, and a layer between 470 and 500 cm in depth. The section was dominated by eurytopes, a species with large ecological spectra, while their dominance increased drastically in its upper part. At depths of ∼190 cm, 450 cm, and 520 cm, the dominance of species characteristic for open areas and of those halotolerant was visible. Forest and shrub species were mainly below −290 cm, with the highest representation at ∼450 cm depth.
Zaton2 is contemporaneous with the upper part of Zaton3 (Figure 7). From the ecological point of view, most of the species in Zaton2 are in the lower part of the section (between 220 and 265 in depth) and are represented by xerophytic or open area species (Figure 7). Above −220 cm, in the upper section, very few species are characteristic for open areas. In the lower part of Zaton3 (between 300 and 450 cm in depth), eurytopic species are abundant, with species characteristics for grassland and open areas and forest and shrub species. In the middle part of the profile (between 116 and 200 cm in depth), the forest species became less abundant, with a reduction of xerophilous and dry habitat species. Eurytopic species became dominant at this depth. The upper part of the section (0–75 cm in depth) is almost depleted of mites, with only three species represented in a very low number, Micreremus brevipes, Microppia minus (forest generalist), and Phauloppia sp. (soil inhabitant) species.
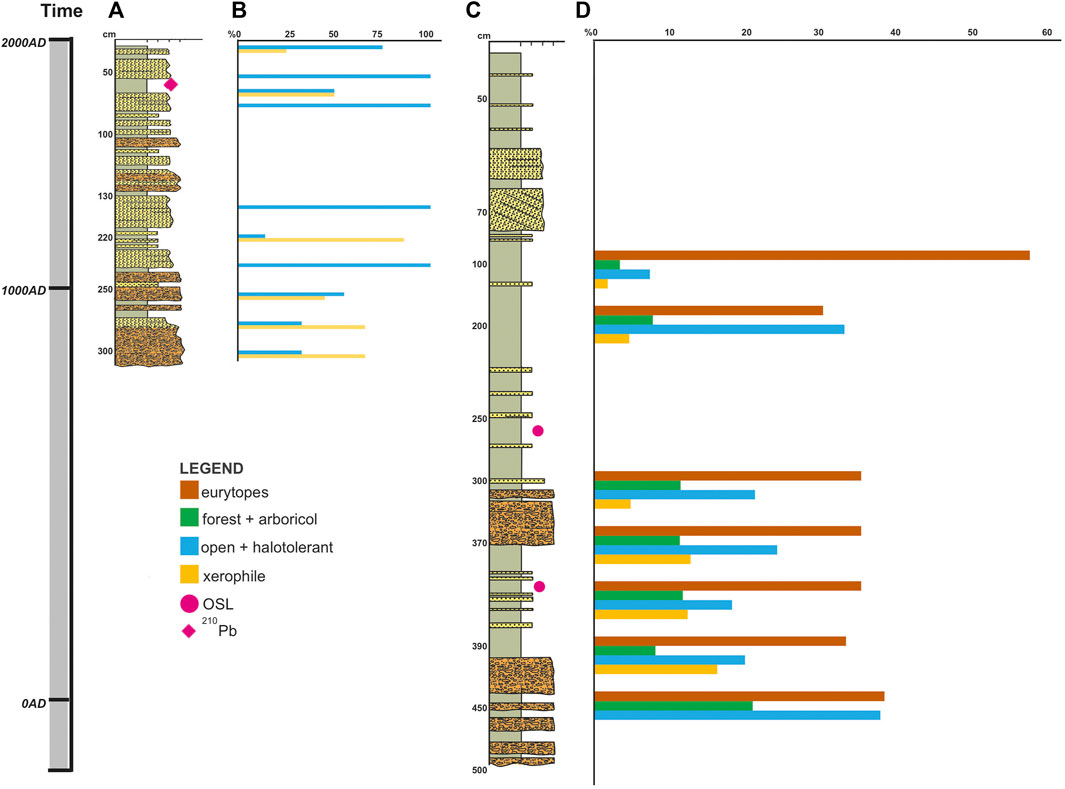
FIGURE 7. Age and the corresponding sedimentary profiles (A,C) in Zăton Lake with the distribution of oribatid mites (only species with more than 10 individuals) in Zaton2 (B) and Zaton3 (D) according to their environmental requirements.
Discussion
Sedimentary Environment Changes in the Last 2,200 Years
The facies and grain size analysis suggested a variability of the sediment characteristics from the median section, Zaton3, dominated by fine sediments of alluvial plain (facies association II) and secondary by channel fill and gravelly bars (facies association I) toward the marginal section, Zaton2, dominated by the coarse channel fill of the tributary systems alternating with the fine sediments of the alluvial plain of the main Șipot Creek (Supplementary Figures S1, S2). The tractive and suspension processes could also be interrupted by mass flows forming alluvial cones from the torrential tributary systems, which are reflected in the Gm facies and lack the internal structure, especially in the marginal sectors. Throughout the last 2,200 years, during which the last 4.5 m of the sedimentary filling of the Zăton basin was accumulated, the periods of channel fill bars accumulation alternated with periods of sedimentation of fine facies, overbank, or lake. These changes could have been possible due to the change of sedimentation regime in this type of basin. The periods of total flooding and drying have led to the alternation of the accumulative and erosional processes. Even though the flood periods are dicyclic, the number of flood days per year must also be variable. Other control factors are the drainage system and the communication with the cave where the stream flows. The position of the siphon and the drainage capacity controlled the accumulation and erosion processes and the style of the fluvial systems. If, at present, the streams are meandering (Figure 8B), in 1976, the basin included two streams with a relatively linear flow (Figure 8A). As it appears recorded on the columns shown in Figure 3, this sedimentation style has certainly worked since the beginning of the filling history of the basin. All these factors of the hydrodynamic regime and sedimentation regime must have been affected by the vegetation around the lake and the Oribatida mites’ communities.
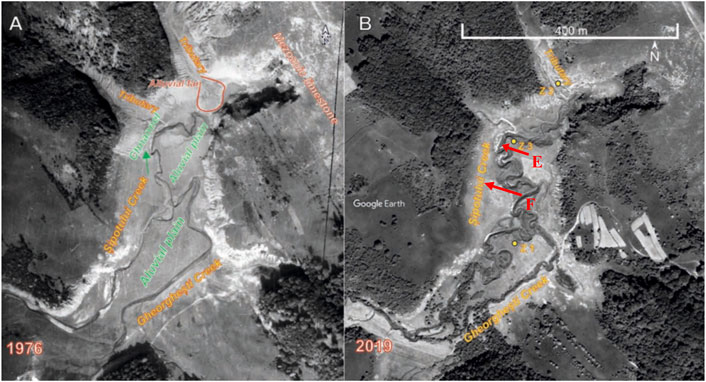
FIGURE 8. Aerial images of the Zăton Basin taken in 1976 (A); source: The Emil Racovita Institute of Speleology archive); and in 2019 (B); source: Google Earth). It shows a variable morphology through time from a relatively straight fluvial style in 1976 to a meandering style in 2019. The difference in the forest cover around the lake from 1976 to 2019 should be noted, with a slight increase more recently.
Paleoenvironmental Inferences
The two analyzed sections were different in abundance and in the diversity of Oribatida. Zaton2, which covered the last ∼ 600 years, was generally poor in species, and its abundances were from zero (in nine layers) to very low (with only three layers with more than five specimens). The sediment accumulation in this profile is evidence of changes in the lake surroundings, with increased soil erosion in the last six centuries. The Zaton2 profile is characterized by the cyclical alternation of layers with few species and no fauna. The layers without fauna in Zaton2 are those with higher granulometry and those resulting from the cyclic soil erosion of the adjacent slope. Zaton2 is similar in environmental information to the upper part of Zaton3.
The Zaton3 section covers more than the last 2,200 years and shows a change from a mixture of eurytopic, forest, and grassland with open areas in the lower part to dominant eurytopic species in the middle part of the profile and almost lack species in the upper, most recent part of the section. The increased abundance and diversity for oribatid mites in the Zaton3 section are associated with warmer periods in the last 2,200 years (Figure 9). Colder periods are scarce in species and have a very low number of individuals, except for the colder period around 250 A.D., with no species. Another exception is the Modern Warm Period (1870 A.D.—present), when no fossils could be extracted. The correspondent upper layers of Zaton2 contained a mixture of a low number of halotolerant, open space, and xerophilic species (Punctoribates hexagonus, P. punctum, and Zygoribatula exarata) and a new species with no known ecology (Ramusella cf. sengbushi).
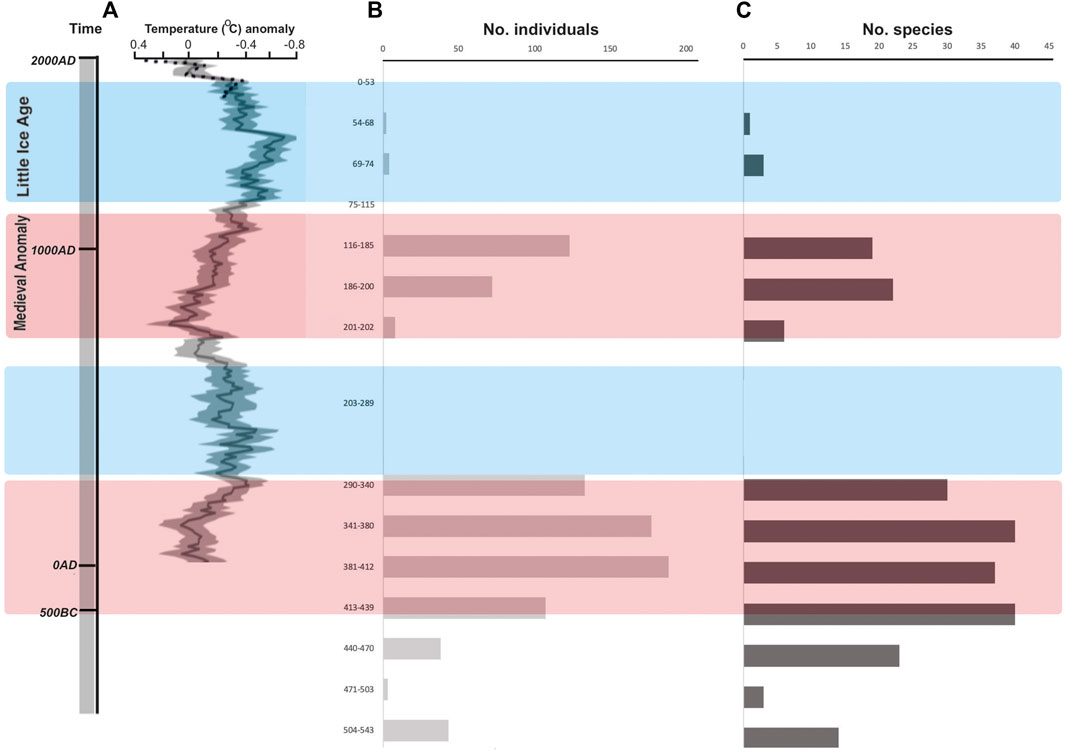
FIGURE 9. Distribution of the oribatid mites’ abundances (B) and diversity (C) in the Zaton3 profile and the corresponding temperature anomaly (A) from PAGES 2k Consortium, 2013). Warmer (red) and colder (blue) periods alternate along time.
The lower part of the profile has particular species often found in soils with salinization, probably due to the presence of fine sediments with high capillarity overflown by water in spring and then drying on hot summer sunny weather, much similar to the dynamic of the lake in the present. However, the species association in the lower part of Zaton3 suggested a more humid environment with more water in the system, perhaps even swampy areas. Eurytopic species dominance in the middle part of the profile indicated a change from the lower part of the section surrounded by trees to a more open landscape that is less humid, maybe with some shrublands and individual trees, but indeed not a period with larger forested areas. The almost depleted fauna layers correspond to colder periods, except for the upper part of the section (Figure 9). A scarcity of species in the upper, more recent part of the section resembles the colder periods more and can be explained by the alteration of the forest cover, which at present is only maintained in small areas with planted trees. For the Zaton3 profile, the thickest layer with no oribatid fossils was around 250 A.D. with a colder climate and possibly more extended periods of water accumulation in the lake, as suggested by the fine sediments, especially the mixture of clay and silt.
Thus, five periods could be identified in the evolution of the lake environments from sediment granulometry and facies, together with Oribatida mites’ communities (Table 3). These periods can be related to the area’s less or more dense human presence:
1. ∼250 B.C.–∼ 200 A.D. Neolithic and Bronze Age sites in the area (data from the National Archeologic Repertoire) attest to human settlements in the region even before the deposition of the studied sediments in the lake. The Roman occupation that lasted in the region between 96 B.C. and 275 A.D. has left no archeological trace. However, if human settlements were established around the lake before and during the Roman occupation, they were probably formed from small or temporary populations with less impact on the area. Oribatida species were abundant and specific for a mixture of forest and open areas in this period of warmer climate. We found a different age limit for the historically established climatic events when considering the Oribatid fauna in Zaton3. For example, the Roman Warm Period in the Zăton basin started earlier than ∼250 B.C. and extended to earlier than ∼250 A.D., which is in contrast to the attributed limits to this climatic period, 250 B.C.–400 A.D. (Cambell et al., 1998).
2. Around 250 A.D. A strong signal of colder weather was observed in our data, with significant deposition of sediments and almost no oribatids. Loehle (2007) also mentioned this climatic anomaly from 18 multi-proxy long series in different regions on Earth.
3. ∼950 A.D.–∼1250 A.D. Human settlements were probably present, but we have no archeological data on the occupation in the area. The effects of a change in the environment are already attested by almost exclusively eurytopic species, characteristic of disturbed environments with some shrubs and trees, corresponding to the Medieval Warm Period.
4. ∼1300 A.D.–∼1870 A.D. The cold period called the “Little Ice Age” in Europe is observed in the scarcity of oribatids in the lake sediments, with taxa characteristic for a mixture of trees, shrubs, and open areas, much more like the landscape in the present (Figure 2). A more significant human population in the area can be traced back to 1766 and 1792, the foundation dates of two wooden churches (now historical monuments). Local information also mentions a former wooden church dating back to the 14th century. These are time landmarks for more organized human settlements and agricultural practices in the very proximity of Zăton Lake. Alterations of the forest cover were probably contemporaneous with the need for more land for agricultural purposes in a generally rocky and dry karstic area. The Little Ice Age also appeared differently in our study on Zaton2. It started around 1300 but ended sooner, in the 17th century, not during the 15th–19th centuries, as in other parts of Europe. However, such differences can be caused by a poor age control for the two analyzed profiles.
5. ∼1870 A.D.–present. The modern period has a warmer climate, and one would expect a relatively abundant oribatid fauna as in other warm periods of the last millennia. We did not expect high diversity of taxa but at least many specimens due to a favorable climate and better chances of preservation of fossils of such young ages. On the contrary, we obtained no abundant or diversified oribatid representatives. The growing human population has, even more, impacted the already installed degradation of the initial environment around the lake in the last two centuries, with intense use of the land around the lake and in the region. More substantial anthropogenic impacts in the last 100–150 years were also documented for other northern, northeastern, and northwestern Romanian lake sediments or peat bogs (Feurdean et al., 2013, 2015; Haliuc et al., 2019, 2020).
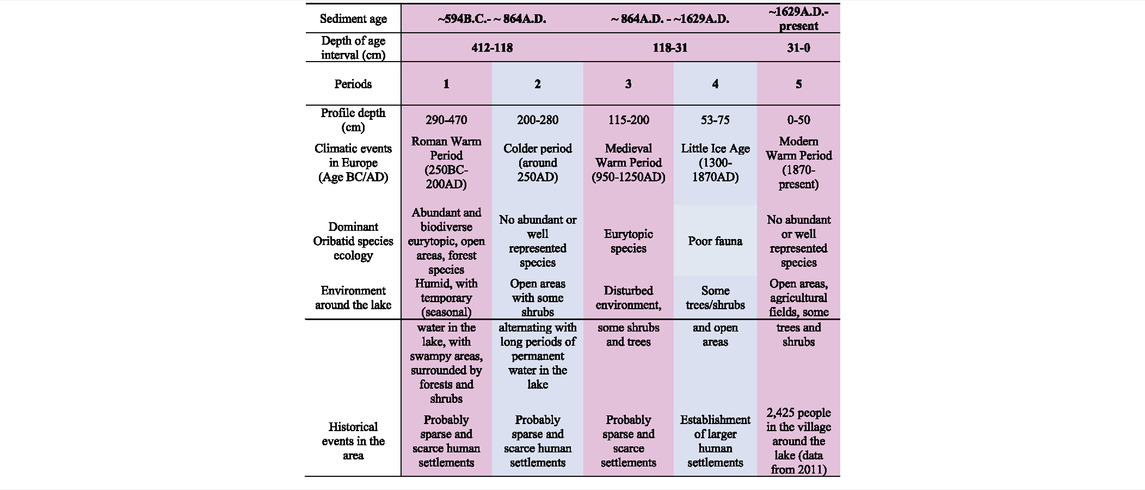
TABLE 3. Tentative correlation of historical time events and paleoenvironment during the last 2,200 years (Wikipedia, 2021) in the Zăton Lake area, as inferred from fossil oribatids and sediment data. Blue = colder periods; red = warmer periods.
Conclusion
Climate has an essential role in lakes’ water availability and evolution, especially for temporary lakes such as Zăton, whose evolution depends on the precipitation amount and duration. However, vegetation, especially forests, can play an essential role in water availability, protection from desiccation, and buffering the impact of climate. For Zăton Lake, this natural cycle was interrupted by establishing human settlements around the lake, the forest cutting in the region, and the use of land for agriculture and cattle. Soil erosion is also enhanced due to reduced vegetation on the lake slopes, especially the northern, steeper slopes. The Zaton2 profile, formed in the last 600 years, might have resulted from forests cut around the lake during the Little Ice Age combined with establishing more significant human settlements in the area, beginning with the 14–18th century. Most probably, forest cuttings were more intense at the beginning of the human occupation, and disturbances continue to be strong at present with more populations in the area (2,425 in the village around the lake in 2011, with more than double the populations in the area after the WWII), whose living is partly based on agriculture.
Temporary lakes, such as Zăton Lake, can be a good model for paleoenvironmental studies, where natural and anthropic processes can drastically influence the shape, annual dynamics, and sediment deposition. Zăton Lake has become an important site for paleoenvironmental reconstruction covering the last 2,200 years, a period poorly documented for the Romanian Carpathians. As a biological proxy, Oribatida mites have proven once again to be a good indicator of past environments and indirectly assess the human presence in a relationship or decoupled from the rhythmic climate changes. Minor differences in the past climate at local levels are of utmost importance to understand regional climatic influences and can be used to model future regional impacts for the sustainable use of local natural resources.
Data Availability Statement
The original contributions presented in the study are included in the article/Supplementary Material, further inquiries can be directed to the corresponding author.
Author Contributions
OM and SC designed the research. OM, CP, R-DR, MK, IM, AP, and MR collected the field data. LM made the identification of oribatid mites. CP made the paleomagnetic analyses. R-DR made the sedimentologic analysis. MG and HH made the 210Pb analysis. RO made the resistivity measurements. MK and IM made the maps, and OM, LM, CP, R-DR, and SC wrote the first draft. All authors corrected and approved the final version.
Funding
The Ministry of Research, Innovation and Digitization grant, CNCS/CCCDI – UEFISCDI, project no. 2/2019 (DARKFOOD), within PNCDI III and the EEA Financial Mechanism 2014-2021 under the project contract no. 3/2019 (KARSTHIVES 2) financially supported the different teams for the analyses and result interpretation.
Conflict of Interest
The authors declare that the research was conducted in the absence of any commercial or financial relationships that could be construed as a potential conflict of interest.
Publisher’s Note
All claims expressed in this article are solely those of the authors and do not necessarily represent those of their affiliated organizations, or those of the publisher, the editors, and the reviewers. Any product that may be evaluated in this article, or claim that may be made by its manufacturer, is not guaranteed or endorsed by the publisher.
Acknowledgments
We are grateful to Mihai Terente, Daniel Vereș, and Marius Vlaicu for the help with sampling, Alida Gabor for the OSL dates, and Ioana Meleg for the sorting of the sediments in the laboratory; all these activities were financially supported by the KARSTHIVES Project—“Climate archives in karst”. We acknowledge the reviewers for improving the clarity of the manuscript.
Supplementary Material
The Supplementary Material for this article can be found online at: https://www.frontiersin.org/articles/10.3389/feart.2022.856685/full#supplementary-material
References
Appleby, P. G. (2001). “Chronostratigraphic Techniques in Recent Sediments,”. Tracking Environmental Changes Using Lake Sediments: Basin Analysis, Coring, and Chronological Techniques. Editors W. M. Last, and J. P. Smol (Dordrecht: Kluwer Academic Publishers), 1, 171–203. doi:10.1007/0-306-47669-X_9
Arnaud, F., Révillon, S., Debret, M., Revel, M., Chapron, E., Jacob, J., et al. (2012). Lake Bourget Regional Erosion Patterns Reconstruction Reveals Holocene NW European Alps Soil Evolution and Paleohydrology. Quat. Sci. Rev. 51, 81–92. doi:10.1016/j.quascirev.2012.07.025
Battarbee, R. W., Anderson, N. J., Bennion, H., and Simpson, G. L. (2012). Combining Limnological and Palaeolimnological Data to Disentangle the Effects of Nutrient Pollution and Climate Change on lake Ecosystems: Problems and Potential. Freshw. Biol. 57, 2091–2106. doi:10.1111/j.1365-2427.2012.02860.x
Behan-Pelletier, V. M. (1999). Oribatid Mite Biodiversity in Agroecosystems: Role for Bioindication. Agric. Ecosyst. Environ. 74, 411–423. doi:10.1016/s0167-8809(99)00046-8
Behan-Pelletier, V., and Newton, G. (1999). Linking Soil Biodiversity and Ecosystem Function: The Taxonomic Dilemma. BioScience 49 (2), 149–153. doi:10.2307/1313540
Bennion, H., Battarbee, R. W., Sayer, C. D., Simpson, G. L., and Davidson, T. A. (2010). Defining Reference Conditions and Restoration Targets for lake Ecosystems Using Palaeolimnology: a Synthesis. J. Paleolimnol 45, 533–544. doi:10.1007/s10933-010-9419-3
Bennion, H., Carvalho, L., Sayer, C. D., Simpson, G. L., and Wischnewski, J. (2012). Identifying from Recent Sediment Records the Effects of Nutrients and Climate on Diatom Dynamics in Loch Leven. Freshw. Biol. 57, 2015–2029. doi:10.1111/j.1365-2427.2011.02651.x
Borrelli, P., Robinson, D. A., Fleischer, L. R., Lugato, E., Ballabio, C., Alewell, C., et al. (2017). An Assessment of the Global Impact of 21st century Land Use Change on Soil Erosion. Nat. Commun. 8, 2013. doi:10.1038/s41467-017-02142-7
Bortolotti, L. E., St. Louis, V. L. V. L., Vinebrooke, R. D., and Wolfe, A. P. (2016). Net Ecosystem Production and Carbon Greenhouse Gas Fluxes in Three Prairie Wetlands. Ecosystems 19, 411–425. doi:10.1007/s10021-015-9942-1
Cambell, I. D., Campbell, C., Apps, M. J., Rutter, N. W., and Bush, A. B. G. (1998). Late Holocene Similar to 1500 Yr Climatic Periodicities and Their Implications. Geology 26, 471–473.
Catalán, Núria, N., von Schiller, Daniel, D., Marcé, Rafael, R., Koschorreck, Matthias, M., Gomez-Gener, Lluís, L., and Obrador, Biel, B. (2014). Carbon Dioxide Efflux during the Flooding Phase of Temporary Ponds. Limnetica 33, 349–360. doi:10.23818/limn.33.27
Chadima, M., and Hrouda, F. (2006). Remasoft 3.0 - a User-Friendly Paleomagnetic Data Browser and Analyzer. Travaux Géophysiques XXVII, 20–21.
Cleveland, W. S., and Devlin, S. J. (1988). Locally Weighted Regression: An Approach to Regression Analysis by Local Fitting. J. Am. Stat. Assoc. 83, 596–610. doi:10.1080/01621459.1988.10478639
Coetzee, L., and Brink, J. S. (2003). Fossil Oribatid Mites (Acari, Oribatida) from the Florisbad Quaternary Deposits, South Africa. Quat. Res. 59, 246–254. doi:10.1016/s0033-5894(02)00024-8
Daily, W., and Lytle, J. (1983). Geophysical Tomography. J. Geomagn. Geoelec 35, 423–442. doi:10.5636/jgg.35.423
Dubois, N., Saulnier-Talbot, É., Mills, K., Gell, P., Battarbee, R., Bennion, H., et al. (2018). First Human Impacts and Responses of Aquatic Systems: A Review of Palaeolimnological Records from Around the World. Anthropocene Rev. 5 (1), 28–68. doi:10.1177/2053019617740365
Elias, S. A. (1994). Quaternary Insects and Their Environments. Washington D. C.: Smithsonian Institution Press, 284.
Erdmann, G., Otte, V., Langel, R., Scheu, S., and Maraun, M. (2007). The Trophic Structure of Bark-Living Oribatid Mite Communities Analysed with Stable Isotopes (15N, 13C) Indicates strong Niche Differentiation. Exp. Appl. Acarol 41, 1–10. doi:10.1007/s10493-007-9060-7
Erickson, J. M. (1988). “Fossil Oribatid Mites as Tools for Quaternary Paleoecologists: Preservation Quality, Quantities, and Taphonomy,” in Late Pleistocene and Early Holocene Paleoecology and Archaeology of the Eastern Great Lakes Region. Editors R. S. Laub, N. G. Miller, and D. W. Steadman (New York: Bulletin of the Buffalo Society of Natural Sciences), 33, 207–226.
Erickson, J. M., Platt, R. B., and Jennings, D. H. (2003). Holocene Fossil Oribatid Mite Biofacies as Proxies of Palaeohabitat at the Hiscock Site, Byron, New York. Bull. Buffalo Soc. Nat. Sci. 37, 176–189.
Erickson, J. M., and Platt, R. B. (2007). “Oribatid Mites,” in Encyclopedia of Quaternary Science. Editor S. A. Elias (Oxford: Elsevier), 1547–1566. doi:10.1016/b0-44-452747-8/00290-8
Feurdean, A., Galka, M., Kuske, E., Tantau, I., Lamentowicz, M., Florescu, G., et al. (2015). Last Millennium Hydro-Climate Variability in Central-Eastern Europe (Northern Carpathians, Romania). The Holocene 25, 1179–1192. doi:10.1177/0959683615580197
Feurdean, A., Liakka, J., Vannière, B., Marinova, E., Hutchinson, S. M., Mosburgger, V., et al. (2013). 12,000-Years of Fire Regime Drivers in the Lowlands of Transylvania (Central-Eastern Europe): a Data-Model Approach. Quat. Sci. Rev. 81, 48–61. doi:10.1016/j.quascirev.2013.09.014
Fick, S. E., and Hijmans, R. J. (2017). WorldClim 2: New 1‐km Spatial Resolution Climate Surfaces for Global Land Areas. Int. J. Climatol 37, 4302–4315. doi:10.1002/joc.5086
Fountain, M. T., and Hopkin, S. P. (2001). Continuous Monitoring of Folsomia candida (Insecta: Collembola) in a Metal Exposure Test. Ecotoxicology Environ. Saf. 48, 275–286. doi:10.1006/eesa.2000.2007
Goran, C. (1978). Le Karst du plateau du Mehedinţi. I. Partie nord. Travaux de L’institut de Spéologie “E. Racovitza” XVII, 165–183.
Haliuc, A., Buczkó, K., Hutchinson, S. M., Ács, É., Magyari, E. K., Korponai, J., et al. (2020). Climate and Land-Use as the Main Drivers of Recent Environmental Change in a Mid-altitude mountain lake, Romanian Carpathians. PLoS ONE 15 (10), e0239209. doi:10.1371/journal.pone.0239209
Haliuc, A., Feurdean, A., Mîndrescu, M., FrantiucHutchinson, A. S. M., and Hutchinson, S. M. (2019). Impacts of forest Loss in the Eastern Carpathian Mountains: Linking Remote Sensing and Sediment Changes in a Mid-altitude Catchment (Red Lake, Romania). Reg. Environ. Change 19, 461–475. doi:10.1007/s10113-018-1416-5
Henig-Sever, N., Poliakov, D., and Broza, M. (2001). A Novel Method for Estimation of Wild Fire Intensity Based on Ash Ph and Soil Microarthropod Community. Pedobiologia 45, 98–106. doi:10.1078/0031-4056-00072
Jenny, J.-P., Koirala, S., Gregory-Eaves, I., Francus, P., Niemann, C., Ahrens, B., et al. (2019). Human and Climate Global-Scale Imprint on Sediment Transfer during the Holocene. Proc. Natl. Acad. Sci. U.S.A. 116, 22972–22976. doi:10.1073/pnas.1908179116
Jeppesen, E., Meerhoff, M., Davidson, T. A., Trolle, D., Sondergaard, M., Lauridsen, T. L., et al. (2014). Climate Change Impacts on Lakes: an Integrated Ecological Perspective Based on a Multi-Faceted Approach, with Special Focus on Shallow Lakes. J. Limnology 73, 84–107. doi:10.4081/jlimnol.2014.844
Kirschvink, J. L. (1980). The Least-Squares Line and Plane and the Analysis of Palaeomagnetic Data. Geophys. J. Int. 62, 699–718. doi:10.1111/j.1365-246x.1980.tb02601.x
Kostic, B., and Aigner, T. (2007). Sedimentary Architecture and 3D Ground-Penetrating Radar Analysis of Gravelly Meandering River Deposits (Neckar Valley, SW Germany). Sedimentology 54, 789–808. doi:10.1111/j.1365-3091.2007.00860.x
Kovacheva, M., Kostadinova-Avramova, M., Jordanova, N., Lanos, P., and Boyadzhiev, Y. (2014). Extended and Revised Archaeomagnetic Database and Secular Variation Curves from Bulgaria for the Last Eight Millennia. Phys. Earth Planet. Interiors 236, 79–94. doi:10.1016/j.pepi.2014.07.002
Krivolutsky, D. A., and Druk, A. Y. (1986). Fossil Oribatid Mites. Annu. Rev. Entomol. 31, 533–545. doi:10.1146/annurev.en.31.010186.002533
Krivolutsky, D. A., and Sidorchuk, E. A. (2003). Subfossil Oribatid Mites in the Holocene Deposits of the Arkhangel'sk Region. Doklady Akademii Nauk 392, 421–425.
Li, Y.-X., and Kodama, K. P. (2016). Detecting and Correcting for Paleomagnetic Inclination Shallowing of Sedimentary Rocks: A Review. Front. Earth Sci. 4, 7. doi:10.3389/feart.2016.00007
Lindo, Z., and Visser, S. (2004). Forest Floor Microarthropod Abundance and Oribatid Mite (Acari: Oribatida) Composition Following Partial and clear-cut Harvesting in the Mixed wood Boreal forest. Can. J. For. Research-Revue Canadienne de Recherche Forestiere 34, 998–1006. doi:10.1139/x03-284
Loehle, C. (2007). A 2000-Year Global Temperature Reconstruction Based on Non-treering Proxies. Energ. Environ. 18, 1049–1058. doi:10.1260/095830507782616797
Loke, M. H., and Barker, R. D. (1995). Least‐squares Deconvolution of Apparent Resistivity Pseudosections. Geophysics 60, 1682–1690. doi:10.1190/1.1443900
Loke, M. H., and Dahlin, T. (2002). A Comparison of the Gauss-Newton and Quasi-Newton Methods in Resistivity Imaging Inversion. J. Appl. Geophys. 49, 149–162. doi:10.1016/s0926-9851(01)00106-9
Longman, J., Veres, D., Finsinger, W., and Ersek, V. (2018). Exceptionally High Levels of lead Pollution in the Balkans from the Early Bronze Age to the Industrial Revolution. Proc. Natl. Acad. Sci. U S A. 115, E5661–E5668. doi:10.1073/pnas.1721546115
Longman, J., Ersek, V., Veres, D., and Salzmann, U. (2017). Detrital Events and Hydroclimate Variability in the Romanian Carpathians during the Mid-to-late Holocene. Quat. Sci. Rev. 167, 78–95. doi:10.1016/j.quascirev.2017.04.029
Miall, A. D. (1985). Architectural-element Analysis: A New Method of Facies Analysis Applied to Fluvial Deposits. Earth-Science Rev. 22, 261–308. doi:10.1016/0012-8252(85)90001-7
Miall, A. D. (1996). The Geology of Fluvial Deposits: Sedimentary Facies, Basin Analysis and Petroleum Geology. New York: Springer-Verlag, 582.
Micu, D. M., Dumitrescu, A., Cheval, S., and Birsan, M.-V. (2015). Climate of the Romanian Carpathians. Springer-Verlag.
Migliorini, M. (2009). “Oribatid Mite (Arachnida: Oribatida) Coenoses from SW Sardinia,”. Research on the Terrestrial Arthropods of Sardinia. Editors P. Cerretti, F. Mason, A. Minelli, G. Nardi, and D. Whitmore (Italy: Zootaxa), 2318, 8–37. doi:10.11646/zootaxa.2318.1.4
Migliorini, M., Pigino, G., Caruso, T., Fanciulli, P. P., Leonzio, C., and Bernini, F. (2005). Soil Communities (Acari Oribatida; Hexapoda Collembola) in a clay pigeon Shooting Range. Pedobiologia 49, 1–13. doi:10.1016/j.pedobi.2004.06.009
Miko, L. (2016). Oribatid Mites (Acarina: Oribatida) of the Czech Republic. Revised Check–List with a Proposal for Czech Oribatid Nomenclature. Klapalekiana 52 (Suppl. l), 1–302.
Moldovan, O. T., Constantin, S., Panaiotu, C., Roban, R. D., Frenzel, P., and Miko, L. (2016). Fossil Invertebrates Records in Cave Sediments and Paleoenvironmental Assessments - a Study of Four Cave Sites from Romanian Carpathians. Biogeosciences 13, 483–497. doi:10.5194/bg-13-483-2016
Moldovan, O. T., Mihevc, A., Miko, L., Constantin, S., Meleg, I. N., Petculescu, A., et al. (2011). Invertebrate Fossils from Cave Sediments: a New Proxy for Pre-quaternary Paleoenvironments. Biogeosciences 8, 1825–1837. doi:10.5194/bg-8-1825-2011
Montgomery, D. R. (2007). Soil Erosion and Agricultural Sustainability. Proc. Natl. Acad. Sci. U.S.A. 104, 13268–13272. doi:10.1073/pnas.0611508104
Morris, E. P., Flecha, S., Figuerola, J., Costas, E., Navarro, G., Ruiz, J., et al. (2013). Contribution of Doñana Wetlands to Carbon Sequestration. PLoS ONE 8, e71456. doi:10.1371/journal.pone.0071456
Murray, A. S., and Wintle, A. G. (2003). The Single Aliquot Regenerative Dose Protocol: Potential for Improvements in Reliability. Radiat. Measurements 37, 377–381. doi:10.1016/s1350-4487(03)00053-2
PAGES 2k Consortium (2013). Continental-scale Temperature Variability during the Past Two Millennia. Nat. Geosci. 6, 339–346. doi:10.1038/ngeo1797
Peters, M., Friedmann, A., Stojakowits, P., and Metzner-Nebelsick, C. (2020). Holocene Vegetation History and Environmental Change in the Lăpuş Mountains, north-west Romania. Palynology 44, 441–452. doi:10.1080/01916122.2019.1615567
Renberg, I. (1990). A 12,600 Year Perspective of the Acidification of Lilla Oresjon, Southwest Sweden. Philosophical Trans. R. Soc. B 327, 357–361.
Roberts, N., Fyfe, R. M., Woodbridge, J., Gaillard, M.-J., Davis, B. A. S., Kaplan, J. O., et al. (2018). Europe's Lost Forests: a Pollen-Based Synthesis for the Last 11,000 Years. Sci. Rep. 8, 716. doi:10.1038/s41598-017-18646-7
Roberts, N., Moreno, A., Valero-Garcés, B. L., Corella, J. P., Jones, M., Allcock, S., et al. (2012). Palaeolimnological Evidence for an East-West Climate See-Saw in the Mediterranean since AD 900. Glob. Planet. Change 84-85, 23–34. doi:10.1016/j.gloplacha.2011.11.002
Rose, N. L., Morley, D., Appleby, P. G., Battarbee, R. W., Alliksaar, T., Guilizzoni, P., et al. (2011). Sediment Accumulation Rates in European Lakes since AD 1850: Trends, Reference Conditions and Exceedence. J. Paleolimnol 45, 447–468. doi:10.1007/s10933-010-9424-6
Schatz, H. (1983). U.-Ordn.: Oribatei, Hornmilben. Catalogus Faunae Austriae, Teil IXi. Wien: Verlag der Österreichischen Akademie der Wissenschafter, 118.
Silvester, P. P., and Ferrari, R. L. (1990). Finite Elements for Electrical Engineers. 2nd. ed. Cambridge University Press.
Solhøy, I. W., and Solhøy, T. (2000). The Fossil Oribatid Mite Fauna (Acari: Oribatida) in Late-Glacial and Early-Holocene Sediments in Kråkenes Lake, Western Norway. J. Paleolimnology 23, 35–47.
Solhøy, T. (2001). “Oribatid Mites,” in Tracking Environmental Change Using lake Sediments. 4: Zoological Indicators. Editors J. P. Smol, H. J. B. Birks, and W. M. Last (Dordrecht: Kluwer Academic Publishers), 81–104.
Van Straalen, N. M. (1998). Evaluation of Bioindicator Systems Derived from Soil Arthropod Communities. Appl. Soil Ecol. 9, 429–437. doi:10.1016/s0929-1393(98)00101-2
Vincze, I., Orbán, I., Birks, H. H., Pál, I., Finsinger, W., Hubay, K., et al. (2017). Holocene Treeline and Timberline Changes in the South Carpathians (Romania): Climatic and Anthropogenic Drivers on the Southern Slopes of the Retezat Mountains. The Holocene 27, 1613–1630. doi:10.1177/0959683617702227
R. G. Walker, and N. P. James (Editors) (1992). Facies Models Response to Sea Level Change. St. John’s, Canada: Geological Association of Canada, 119–142.
Wang, Z., Hoffmann, T., Six, J., Kaplan, J. O., Govers, G., Doetterl, S., et al. (2017). Human-induced Erosion Has Offset One-Third of Carbon Emissions from Land Cover Change. Nat. Clim Change 7, 345–349. doi:10.1038/nclimate3263
Weigmann, G. (2006). “Hornmilben (Oribatida),” in Die Tierwelt Deutschlands. Editor F. Dahl (Keltern: Goecke and Evers), 76, 1–520.
Weigmann, G., and Kratz, W. (1982). Die deutschen Hornmilbenarten und ihre ökologische Charakteristik. Zool. Beitr. 27, 259–489.
Wikipedia (2021). Wikipedia.com. Avilableat: https://en.wikipedia.org/wiki/List_of_periods_and_events_in_climate_history (Accessed on October2021 4.
Williamson, C. E., Dodds, W., Kratz, T. K., and Palmer, M. A. (2008). Lakes and Streams as Sentinels of Environmental Change in Terrestrial and Atmospheric Processes. Front. Ecol. Environ. 6, 247–254. doi:10.1890/070140
Wintle, A. G., and Murray, A. S. (2006). A Review of Quartz Optically Stimulated Luminescence Characteristics and Their Relevance in Single-Aliquot Regeneration Dating Protocols. Radiat. Measurements 41, 369–391. doi:10.1016/j.radmeas.2005.11.001
Wozniak, T., Bania, G., Moscicki, W., J., and Cwiklik, M. (2018). Electrical Resistivity Tomography (ERT) and Sedimentological Analysis Applies to Investigation of Upper Jurassic Limestones from the Krzeszowice Graben (Krakow Upland, Southern Poland). Geol. Quaterly 62, 287–302.
Yogeshwar, P., Hamacher, S., Reçi, H., Hauck, T., Onuzi, K., and Tezkan, B. (2019). Investigating Sedimentological Architecture Using Electrical Resistivity Tomography: A Case Study from the Archaeological Open-Air Site Shën Mitri, Southern Albania. Pure Appl. Geophys. 176, 843–856. doi:10.1007/s00024-018-1987-6
Keywords: Oribatida, climate change, human impact, paleoenvironments, indicator species
Citation: Moldovan OT, Miko L, Panaiotu C, Roban R-D, Gąsiorowski M, Hercman H, Orza R, Kenesz M, Mirea IC, Petculescu A, Robu M and Constantin S (2022) Small Human Population Drastic Impact, as Inferred From Multi-Proxies of a Temporary Carpathian Lake. Front. Earth Sci. 10:856685. doi: 10.3389/feart.2022.856685
Received: 17 January 2022; Accepted: 28 March 2022;
Published: 27 April 2022.
Edited by:
Xibin Tan, China Earthquake Administration, ChinaReviewed by:
Jiawei Fan, China Earthquake Administration, ChinaHu Wang, Southwest Jiaotong University, China
Copyright © 2022 Moldovan, Miko, Panaiotu, Roban, Gąsiorowski, Hercman, Orza, Kenesz, Mirea, Petculescu, Robu and Constantin. This is an open-access article distributed under the terms of the Creative Commons Attribution License (CC BY). The use, distribution or reproduction in other forums is permitted, provided the original author(s) and the copyright owner(s) are credited and that the original publication in this journal is cited, in accordance with accepted academic practice. No use, distribution or reproduction is permitted which does not comply with these terms.
*Correspondence: Oana Teodora Moldovan, b2FuYW1vbDM1QGdtYWlsLmNvbQ==
†These authors have contributed equally to this work