- 1School of Highway, Chang’an University, Xi’an, China
- 2Shandong Express Engineering Inspection Co. Ltd., Jinan, Shandong, China
Coarse-grained soil is widely used in the seasonal frozen soil region as subgrade filler. However, substantial frost heave has been observed in coarse filler in high-speed railway embankments. To investigate the frost heave characteristics of the coarse-grained soil in a deep seasonal frozen soil zone, indoor tests were carried out under water supply and no water supply conditions. The effect of water, fineness, and temperature on frost heave behavior is studied experimentally. The relationship between the freezing rate and frost heave of coarse-grained soils was analyzed. The results show that the freezing process of the filler can be divided into the rapid cooling stage, phase transition stage, slow freezing stage, and freezing stability stage. In the closed system, the increase of the fine-grained soil does not affect the cooling process, while the moisture content significantly affects the whole process. In the opened system, both the fine-grained soil content and the ambient temperature affect the cooling process. When the ambient temperature decreased from −5°C to −15°C, the duration of the phase transition stage decreased by almost 17 h, whereas when the fine-grained soil content increased from 2.7 to 16%, the duration of the phase transition stage increased by only 5 h. In both opened and closed systems, the development of the frost heave is closely related to the cooling process. Approximately 95% of frost heave occurs during the phase transition stage and slow freezing stage. Frost heave develops most rapidly in the phase transition stage, with approximately 85% of the frost heave amount occurring in this stage.
1 Introduction
In recent years, China has built a number of high-speed railways in seasonal frozen soil regions. Compare with ordinary subgrade, higher smoothness and stability of track surface conditions are needed by high-speed railway subgrade. Although there may be less frost heave in the deep season frozen soil regions possibly due to larger freezing rates, some railway lines still face severe frost deformation (Shi et al., 2014; Niu et al., 2016; Lin et al., 2018; Niu et al., 2020). So as to ensure the speed and safety of train operation, a series of measures have been proposed to mitigate the deformation caused by frost heave. Coarse-grained soil (CGS) is widely used as subgrade filler due to insensitivity to frost heave and high water-permeability, and it is one of the most common anti-frost heave measures. However, according to the actual condition, frost heave still exists in coarse-grained soil filler (Cai et al., 2016; Niu et al., 2020). In some areas frost heave amount even reached 20 mm (Lin et al., 2018; Niu et al., 2020), far exceeding the design value (TB 10035 (AuthoreAnonymous, 2006). Therefore, it is significant to study the frost heave characteristics of coarse-grained soil in deep seasonal frozen soil.
Zhang (2013) investigated the occurrence, development, and variation of frost heave of high-speed railway subgrade in cold regions through field tests. Niu et al. (2017) analyzed the distribution law of frost heave along with the freezing depth of high-speed railway subgrade in cold regions by field monitoring and concluded that frost heave of subgrade mainly appeared in the bottom of the foundation bed. Tai et al. (2017) (Tai et al., 2018) systematically studied the variation characteristics of the temperature and deformation fields of subgrade engineering of the Ha-Qi high-speed railway in the deep seasonal freezing soil zone. With a continuous understanding of the frost heave mechanism of frozen soil, some scholars began to study the influence of different factors on the frost heave of coarse-grained soil (Konrad, 2008; Du et al., 2014; she et al., 2019). Through laboratory tests, some scholars found that augments with fine particles and clay minerals would improve the frost susceptibility of coarse-grained soil (Vinson et al., 1986; Ye et al., 2007; Xu et al., 2011; Wang and Yue, 2013), and some scholars considered that water content, porosity, negative temperature, dry density, and compaction level affect the frost heave of subgrade (Nie et al., 2013; Wang et al., 2016; Luo et al., 2019). Sheng et al. (2014a) found that frost deformation in a closed system may be offset by the consolidation deformation of unfrozen soil based on laboratory test results and that the frost heave mechanism of coarse-grained soil is determined by a combination of multi-factor (Sheng et al., 2014b). Although scholars have conducted considerable research and have achieved results on the frost-heaving characteristics of the coarse-grained soil, most of the experiments have been focused on the factors influencing the development of frost heaving in seasonal frozen soil regions. Few studies have concentrated on the frost deformation of coarse-grained soil in the deep seasonal frozen soil zone.
In this article, according to the actual conditions that the railway may encounter in the deep seasonal frozen soil region, indoor tests were carried out in both closed and opened systems. The effect of water, fineness, and temperature on frost heave behavior are studied experimentally, respectively, and the relationship between the freezing rate and frost heave of coarse-grained soils was analyzed. The main objective of this study is to reveal the frost heave characteristics of the coarse-grained soil in a deep seasonal frozen soil zone.
2 Test apparatus and materials
2.1 Material
The soil, used in freezing procedure experiments, is angular pebbles, which was all taken from the filler of the embankment at the Harbin–Mudanjiang high-speed rail. The grain size distribution and physical properties of the soil are shown in Figure 1 and Table 1. According to the particle analysis results, the fine-grained fraction (particle size less than 0.075 mm) accounts for 2.7% of the total mass. The non-uniformity coefficient
2.2 Test apparatus
The test device is shown in Figure 2, which is composed of a specimen model, an environmental box (precision: ±0.5°C), a temperature sensor (precision: ±0.1°C, quantity: 3), a displacement sensor (precision: ±0.01 mm, quantity: 1), and a water supply device. The specimen model consists of two layers of Plexiglas barrels with diameters of 150 and 250 mm. The inner barrel is placed on a base plate and the glass barrel is filled with insulating foam. The sensor layout is shown in Figure 2.
2.3 Test procedure
The tests contain closed and opened system tests. Test schemes are shown in Table 2 and Table 3. Before the test, the filler was added with water depending upon the required moisture content, and then it was enclosed for 24 h. At the beginning of the test, 1 cm thick soil was filled at the bottom of the barrel and compacted, and then the remaining soil samples were placed into the Plexiglass barrel three times. The temperature sensors were placed at the fixed position of soil samples respectively. After the soil sample was filled, the top plate was covered, and the dial indicator was installed. The procedure for the freeze heave test under the opened system is essentially the same as earlier. The difference was that in the opened system, a 2-cm thick layer of gravel needed to be laid before filling the soil sample, to the water to be in full contact with the bottom of the sample.
3 Results and discussion
3.1 Test results under closed system
3.1.1 Temperature development of sample
Figure 3 plots the change curve of temperature with time at different fine-grained content during freezing. In Figure 3A, the temperature from top to bottom is always from low to high during the whole freezing process and the curve slope changes with the time of freezing. Based on the curve slope, the whole process is divided into the following four stages: 1) rapid cooling stage, 2) phase transition stage, 3) slow freezing stage, and 4) freezing stability stage.
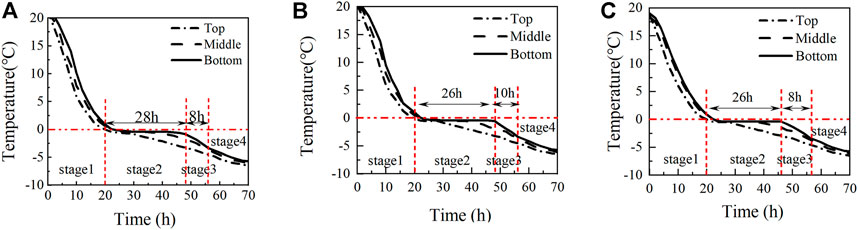
FIGURE 3. Temperature development under different fine-grained content of sample: (A) 2.7%; (B) 8%; (C) 16%.
The rapid cooling stage mainly occurs in the first 20 h. In this stage, the temperature of the sample decreases at a larger cooling rate, and the temperature at the top is always lower than the temperature at the bottom. The duration of the phase transition stage is mainly between 26 and 28 h, and this process does not almost change with the increase of fine-grained content. At this stage, the temperature of each part of the sample tends to be consistent and fluctuates around the freezing point, but the duration of this stage is different in the sample, the middle and lower parts of the sample lasts longer than the upper part. With the end of the phase transition stage, the temperature of samples goes downward to negative and continues to decrease. This part is called the slow freezing stage. Finally, as the temperature of the sample approaches the ambient temperature, the cooling rate of the sample slows down rapidly, which is the stage of freezing stability.
It can be seen that the fine-grained content has little effect on the filler temperature. Therefore, to explore whether the moisture content has an impact on the temperature, the temperature development of fillers under different moisture content is given as follows, as shown in Figure 4.
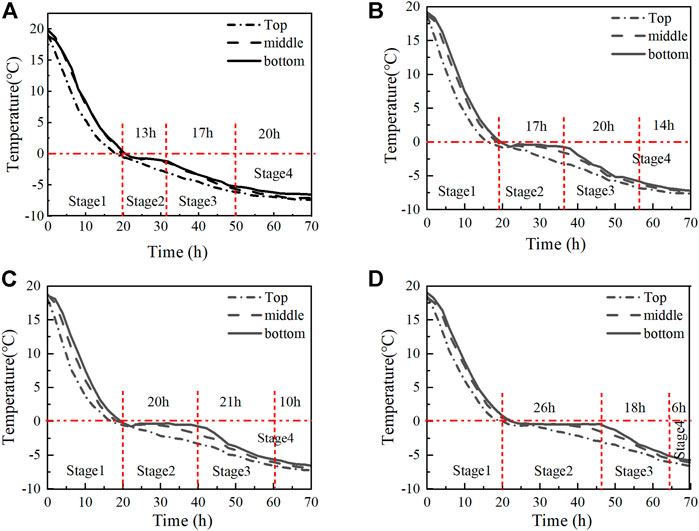
FIGURE 4. Temperature development under different moisture contents: (A) 4%; (B) 6%; (C) 7%; and (D) 8%.
Figure 4 shows the temperature development under different moisture content when fine-grained content is 2.7%. It can be seen that the temperature curve is almost the same, but the duration of different stages is different with the change in the moisture content. The duration of stage 1 almost does not change, whereas, the duration of stage 2 increases with the increase of the moisture content. When the moisture content is 4%, the duration is 13 h. The duration is 17 h when the moisture content is 8%, which is an increase of almost 75% compared to the water content of 4%. Stage 3 changed with the increase of the moisture content but maintained at about 20 h. Stage 4 decreases with the increase of the water content. The development of stage 1 under different factors is almost similar because the change of the fine-grained soil content and moisture content has little effect on the thermal conductivity of the soil at positive temperature. After the sample enters the negative temperature, the moisture content will have a greater impact on the temperature change. This is closely related to the cold energy absorbed in the sample and the latent heat released by water turning into ice, as shown in Figure 5.
In the phase transition stage, the water in the soil begins to freeze. At this time, the latent heat released by the water turned into ice can be balanced with the cold energy absorbed by the soil sample, so the temperature fluctuates at the freezing point. In Figure 3 and Figure 4, It can be found that the duration of this stage in the lower part of the soil sample is significantly longer than that in the upper part because the temperature gradient between the upper part and the lower part of the soil sample is different. The temperature gradient in the upper part of the sample is always large, the cold energy absorbed is much higher than the latent heat released, so the upper temperature always shows a downward trend. In addition, the duration of this stage is also prolonged as the moisture content increases. The aforementioned illustrates that the water content and temperature gradient will affect the duration of the phase change. The smaller the temperature gradient and the higher the water content, the longer will be stage 2.
In the slow freezing stage, soil temperature began to decline gradually. This is because the cold energy absorbed is greater than the latent heat released. At this stage, the cold energy absorbed by the soil and the latent heat released are decreasing over time. The unfrozen water content decreases rapidly with the decrease of temperature, so the latent heat released is less. The temperature gradient of soil samples gradually decreases with the decrease of soil temperature, which makes the cold energy absorbed by soil samples gradually decrease. However, the absorbed cold energy is always greater than the released latent heat, so the temperature of the soil sample begins to decrease gradually.
In the freezing stability stage, the temperature difference between the sample and the external environment is getting smaller, and the unfrozen water content is not changing, so the temperature of the soil sample gradually tends to be stable.
3.1.2 Frost heave of sample
Figure 6 shows the frost heave deformation process and Figure 7 plots the comparison of frost heave results.
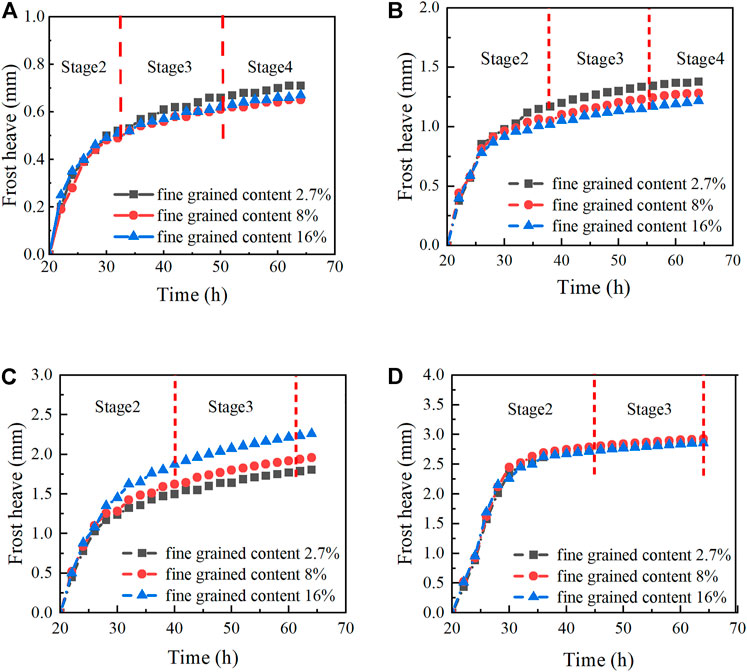
FIGURE 6. Frost heave of samples under different moisture contents: (A) 4%; (B) 6%; (C) 7%; and (D) 8%.
The proportion to the temperature change and frost-heaving results of the samples can be found that the development of frost heaving is closely related to stage 2 and stage 3. Table 4 indicates that stage 2 and stage 3 contribute more than 95% to frost heaving, and stage 2 contributes more than 80%. With the moisture content increasing, the proportion of frost heaving generated by stage 2 increases in the total amount. When the moisture content is 8%, the proportion reaches 93%. In the early stage of freezing, due to the temperature gradient, the liquid water in the soil is quickly frozen so that the frost heave rate of soil samples is larger, and the frost heave deformation increases rapidly; after entering the slow freezing stage over time, the unfrozen water content decreases with the decrease of temperature (Leng et al., 2010), so the frost-heaving rate of soil samples decreases gradually, and the growth trend of frost-heaving deformation becomes slow. After reaching the stage of freezing stability, the unfrozen water in the filler has basically no longer changed, so the frost-heaving rate drops gradually and finally reaches stability. Therefore, it is necessary to pay attention to the influence of frost-heaving deformation on the structure at the early stage of freezing, especially the influence of the phase transition stage on frost-heaving deformation.
1) Influence of water content on frost heave
It can be seen from Figure 7 that the frost heave ratio of the sample increases with the increase of the water content. When the water content is lower than 6%, the increase of the frost heave rate of the sample is small, and then the increasing trend accelerates. The influence of the water content on frost heave is mainly related to the volume increase of ice. When the water content is low, the volume increment after water freezing can be filled into the pores, so no frost heave occurs. However, with the increase of water content, the volume increment cannot be completely offset by pores, so the frost-heaving amount of filler increases.
2) Influence of fine-grained soil content
Figure 7 shows the fine-grained soil content almost does not affect the frost-heaving ratio of coarse-grained soil under the closed system, because there is almost no water migrating under the closed system. It is well-known that the frost heave of frozen soil is caused by water migration and accumulation to the freezing front. Under closed systems, water cannot freely migrate and gather, so frost heaving does not manifest. Under the closed system, subgrade engineering filler containing fine particles will freeze under the action of negative temperature when the moisture content is high, which will produce a large frost heave. Under the same moisture content, the frost-heaving amount of filler does not increase with the increase of the fine-grained content.
3.2 Test results under opened system
3.2.1 Temperature development of Sample
The temperature development curve of each sample at the upper, middle, and lower positions under different fine-grained content is displayed in Figures 8A–C.
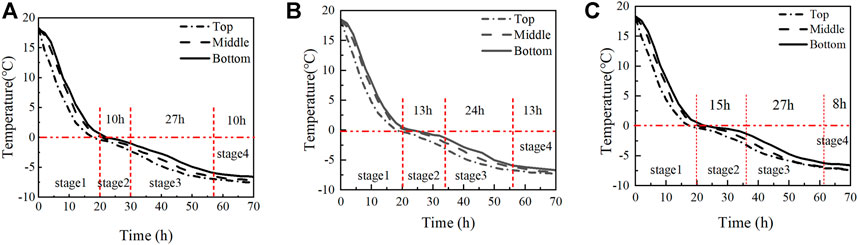
FIGURE 8. Temperature development under different fine-grained contents: (A) 2.7%; (B) 8%; and (C) −16%.
Figure 8 plots the temperature curve of different fillers at −10°C, in which the initial moisture content is 4%. It can be seen that the cooling process under the opened system can also be divided into four stages: 1) rapid cooling stage, 2) phase transition stage, 3) slow freezing stage, and 4) freezing stability stage. However, different from the closed system, due to the supply of water, the development of each stage is different with the fine-grained content. The duration of the phase transition stage increases with the increase of the fine-grained content. The change of the slow freezing stage is not manifested. The time to reach stage 4 increases with the increase of the fine-grained soil content. These changes are related to the migration of water in the filler. The bigger the fine-grained content, the more water can migrate to the freezing front during freezing. The more energy is released when water becomes ice, so the whole process will change.
Figure 9 shows the temperature curve of filler at different temperatures. The whole cooling process is also divided into four stages. However, it is obvious that the ambient temperature will have a huge impact on the whole cooling process. The lower the ambient temperature, the shorter is the phase transition stage, the larger is the slope of the slow freezing stage curve, and the less time it takes to reach the freezing stability stage. This is because the temperature gradient increases with the temperature drops, which makes the cold energy transfer more quickly and blocks the process of moisture migration to the freezing front.
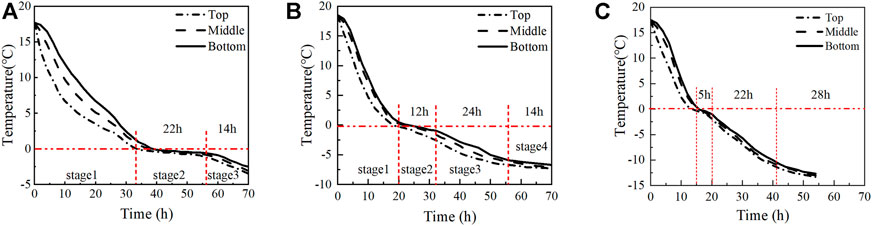
FIGURE 9. Temperature development under different ambient temperatures: (A) −5°C; (B) −10°C; and (C) −15°C.
3.2.2 Frost heave of sample
Figure 10 indicates that the frost-heaving development of the samples under different temperatures is very different. The start time of frost heaving under −5°C, −10°C, and −15°C is about 33, 20, and 13 h, respectively, while the duration of frost-heaving development is 30, 20, and 10 h, respectively. Meanwhile, the frost-heaving amount of the sample is also significantly different under different temperature conditions. The frost-heaving amount is the largest at −10°C and the smallest at −5°C. In addition, under the same temperature, the higher the content of fine-grained soil, the greater is the frost heave. According to Figure 10, combined with Figure 8 and Figure 9, it can be found that the frost-heaving amount of the phase transition stage accounts for about 85% of the total. This is because lots of liquid water was frozen. In the slow freezing stage, due to the decrease of the unfrozen water content in the soil, the growth trend of frost-heaving rate and frost-heaving deformation of soil samples is slowed down. In the freezing stability stage, owing to the unfrozen water content almost no longer changing, the frost heaving rate of soil samples decreases and tends to be stable.
1) Influence of fine-grained content on frost heave under open system
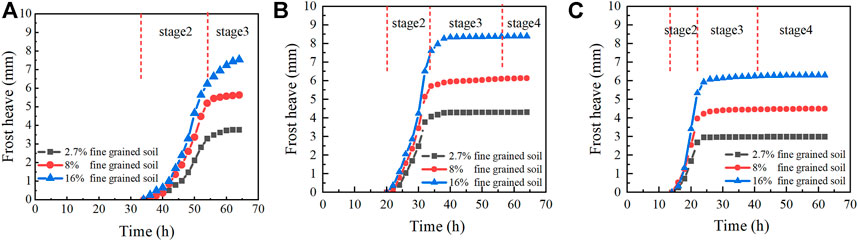
FIGURE 10. Frost heave of different samples under 8% moisture content: (A) −5°C; (B) −10°C; and (C) −5°C.
As can be seen from Figure 11, the frost heave ratio of the sample grows with the increase of the fine-grained soil content, and the frost heave ratio of all soil samples is beyond 1%, which means that the frost grade is weak frost at least (TB 10035 (2006)). Figure 11 indicates the fine-grained soil in filler will exert a huge effect on the frost heave. As we all know, there is little bound water in coarse-grained soil, and there is almost no frost heaving during freezing. When fine-grained content in coarse-grained soil gradually increases, on the one hand, the bound water content increases, so that the bound water in the lower soil layer migrates to the freezing front under the action of molecular gravity and osmotic pressure. On the other hand, the capillary phenomenon began to appear, which make the water of the lower part migrate to the freezing front through the capillary channel. Fine-grained content is small because the capillary phenomenon is not significant. The frost heave rate is consistent with the result under the closed system. However, with fine-grained content increases, the capillary phenomenon begins to become obvious, so the frost heave rate begins to increase significantly. Therefore, in the design and construction of high-speed railway subgrade frost-proof layer filler in large rainfall and cold regions, the content of fine particles in the filler should be controlled, especially for low embankment, zero-section replacement subgrade and cutting. Cement modified coarse particle filler can be filled when needed.
2) Influence of ambient temperature on frost heave
Figure 11 displays the frost heave ratio is the largest at −10°C, followed by −5°C, and the smallest at −15°C. However, Figure 8 shows that when the ambient temperature is −5°C, the corresponding stage at the end of the test is stage 3, and the frost heave at this time is almost the same as that at −10°C. Therefore, the final frost heave of the soil sample at −5°C may be greater than the frost heave at −10°C. This is similar to the findings of Peng and Liu, 2010) and Xu et al. (2010), the lower the freezing temperature, the smaller is the frost heave. The cooling rate is related with freezing temperature, the temperature is lower and the cooling rate is bigger. When the cooling rate is large, the soil freezing surface moves downward rapidly. At this time, weak bound water and capillary water in the soil cannot migrate to the freezing area and freeze to ice in situ, and the capillary channel is blocked by ice crystals. Therefore, the migration and accumulation of water will not occur, and ice interlayer cannot be seen in the soil layer. Only ice crystals scattered in the soil pores, and the frozen soil formed at this time generally has no obvious frost heaving. In contrast, when cooling rate is small, the freezing duration is increased, which can promote the migration and accumulation of water in the unfrozen area to the frozen area. Migration and accumulation of water can form ice interlayer in the soil, resulting in obvious frost heave.
3.3 Analysis of results
The frost heave results of the opened system and the closed system are compared, and the results are shown in Figure 12.
Figure 12 shows the frost heave ratio under opened system conditions greater than the closed system, which means the external water source as one of the important factors, leading to the frost heave. In the closed system, the freezing process is greatly affected by the moisture content. In the opened system, fine-grained content, ambient temperature, and water migration will have a greater impact on the freezing process of filler. Combined with the temperature development of filler, it can be found that in the four stages divided, the aforementioned factors have the greatest impact on stage 2, and 85% of frost-heaving deformation is completed at this stage. In other words, the development of stage 2 has a huge influence on the frost heave of filler, and the development of stage 2 is closely related to the absorbed cold energy and the latent heat released by the phase change. In the closed system, when the ambient temperature is constant, the greater the moisture content, the longer is the duration of stage 2. This is because the greater the moisture content, the more latent heat is released during freezing. In the opened system, the smaller the fine-grained content is, the shorter is the stage 2 duration when the ambient temperature is lower. This is because the content of fine particles affects the migration of water, and the ambient temperature affects not only the migration of water but also the absorption of cold energy per unit time.
The frost heave rate under the opened system is much larger than that under the closed system, and the values are all greater than 1%, ranging from 1.2 to 3.4%. This indicates that the recharge of moisture is an important factor in the occurrence of frost heave. Therefore, in order to be able to further analyze the relationship between temperature and frost heave of the soil itself, the effect of moisture migration on temperature needs to be further considered. Subsequent work will focus on the effect of moisture migration on soil temperature and frost heave and the link between temperature and frost heave.
4 Conclusion
Based on the detailed analyses of the experimental results, the following conclusions can be made:
1) According to the change process of the test temperature, the cooling process of the sample can be divided into four areas according to the development of time: 1) rapid cooling stage; 2) phase transition stage; 3) slow freezing stage; and 4) freezing stability stage.
2) In the closed system, the variation of the fine-grained soil has a small effect on the cooling process, while the change in the moisture content significantly affects the whole process. The phase transition stage is the most affected stage and the higher the water content the longer is the duration of the stage.
3) In the opened system, both the fines content and the ambient temperature affect the cooling process, but the ambient temperature has a greater effect on the cooling process than the fines content. When the ambient temperature was reduced from −5°C to −15°C, the phase transition stage was shortened by nearly 17 h, whereas when the fines content was increased from 2.7 to 16%, the phase transition stage was increased by only 5 h.
4) In both opened and closed systems, the development of the frost heave is closely related to the cooling process. Freezing occurs mainly at the phase transition stage and slow freezing stage, and approximately 95% of the freezing occurs at these two stages. The most rapid development of frost heaving occurs at the phase transition stage, where approximately 85% of the frost heaving is generated.
5) The frost heave rate under the opened system is much larger than that under the closed system, and the values are all greater than 1%, ranging from 1.2 to 3.4%, which indicates that moisture migration is an important factor in the occurrence of frost heave. Therefore, subsequent work needs to focus on the role of moisture migration in the overall frost heaving.
Data availability statement
The original contributions presented in the study are included in the article/Supplementary Files; further inquiries can be directed to the corresponding author.
Author contributions
XM and QW conceived this research. WH wrote the first draft of the manuscript and contributed to data analysis. XM and SZ contributed to conception and field investigation.
Funding
This research was supported by the National Natural Science Foundation of China (51878064) and the Natural Science Basic Research Program of Shaanxi (Grant No. 2021JQ-244).
Acknowledgments
The authors acknowledge the financial support from the National Natural Science Foundation of China (51878064).
Conflict of interest
The author SZ was employed by Shandong Express Engineering Inspection Co. Ltd.
The remaining authors declare that the research was conducted in the absence of any commercial or financial relationships that could be construed as a potential conflict of interest.
Publisher’s note
All claims expressed in this article are solely those of the authors and do not necessarily represent those of their affiliated organizations, or those of the publisher, the editors, and the reviewers. Any product that may be evaluated in this article, or claim that may be made by its manufacturer, is not guaranteed or endorsed by the publisher.
References
AuthorAnonymous (2006). Code for design of special railway subgrade. China: China Railway Publishing House.TB 10035
Du, X., Ye, Y., Zhang, Q., and Cheng, A. (2014). Experimental research on frost heaving characteristics of coarse-grained soil filler of High-speed railway subgrade in cold region. Int. Conf. Mech. Civ. Eng. 12 (2), 12–23. doi:10.1155/2018/5682619
Konrad, J.-M. (2008). Freezing-induced water migration in compacted base-course materials. Can. Geotech. J. 45 (7), 895–909. doi:10.1139/t08-024
Lin, Z., Niu, F., Li, X., Li, A., Liu, M., Luo, J., et al. (2018). Characteristics and controlling factors of frost heave in high-speed railway subgrade, Northwest China. Cold Regions Sci. Technol. 153, 33–44. doi:10.1016/j.coldregions.2018.05.001
Luo, Q., Wu, P., and Wang, T. (2019). Evaluating frost heave susceptibility of well-graded gravel for HSR subgrade based on orthogonal array testing. Transp. Geotech. 21, 100283. doi:10.1016/j.trgeo.2019.100283
Nie, Z., Liu, Y., and Wang, X. (2013). Experimental study on the factors influencing the frost heave of graded gravel on the surface layer of passenger dedicated line[J]. J. Railw. Sci. Eng. 10, 59–62. (in Chinese). doi:10.1061/9780784478509.010
Niu, F., Li, A., Luo, J., Lin, Z., Yin, G., Liu, M., et al. (2017). Soil moisture, ground temperatures, and deformation of a high-speed railway embankment in Northeast China. Cold Regions Sci. Technol. 133, 7–14. doi:10.1016/j.coldregions.2016.10.007
Niu, F., Lin, Z., and Wu, X., 2016.Characteristics of subgrade's temperature, moisture and frost heave deformation in Haomen, passenger railway line from Lanzhou to Xinjiang, [J].J. Glaciol. Geocryol. 38(4): 1074–1082. doi:10.7522/j.issn.1000-0240.2016.0125
Niu, F., Zheng, H., and Li, A. (2020). The study of frost heave mechanism of high-speed railway foundation by field-monitored data and indoor verification experiment. Acta Geotech. 15 (3), 581–593. doi:10.1007/s11440-018-0740-8
Peng, L., and Liu, J. (2010). Research on deformation characteristics of positive melting silty clay under cyclic loading[J]. Geotech. Eng. 32 (04), 567–572. (in Chinese).
She, W., Wei, L., Zhao, G., Yang, G., Jiang, J., and Hong, J. (2019). New insights into the frost heave behavior of coarse grained soils for high-speed railway roadbed: Clustering effect of fines. Cold Regions Sci. Technol. 167, 102863. doi:10.1016/j.coldregions.2019.102863
Sheng, D., Zhang, S., Niu, F., and Cheng, G. (2014b). A potential new frost heave mechanism in high-speed railway embankments. Géotechnique 64 (2), 144–154. doi:10.1680/geot.13.p.042
Sheng, D., Zhao, G., Zhang, S., and Niu, F. (2014a). “Possible frost heave mechanisms in an unsaturated high-speed railway formation,” in Proceedings of the sixth international conference on unsaturated soils, unsaturated soil: research and applications (at Sydney, London: CRC Press), 3–14. doi:10.1201/b17034-3Vol. 1
Shi, G., Zhao, S., Li, X., and Niu, Y. 2014.The frost heaving deformation of high-speed railway subgrades in cold regions: Monitoring and analyzing, [J].J. Glaciol. Geocryol.36(2):360–368.doi:10.7522/j.issn.1000-0240.2014.0044
Tai, B., Liu, J., Wang, T., Shen, Y., and Li, X. (2017). Numerical modelling of anti-frost heave measures of high-speed railway subgrade in cold regions. Cold Regions Sci. Technol. 141, 28–35. doi:10.1016/j.coldregions.2017.05.009
Tai, B., Liu, J., Yue, Z., Liu, J., Tian, Y., and Wang, T. (2018). Effect of sunny-shady slopes and strike on thermal regime of subgrade along a high-speed railway in cold regions, China. Eng. Geol. 232, 182–191. doi:10.1016/j.enggeo.2017.09.002
Vinson, T. S., Ahmad, F., and Rieke, R. (1986). Factors important to the development of frost heave susceptibly criteria for coarse-grained soils[J]. Transp. Res. Rec., 124–131. doi:10.1016/0148-9062(88)92819-7
Wang, Q., Liu, J., Zhu, X., Liu, J., and Liu, Z. (2016). The experiment study of frost heave characteristics and gray correlation analysis of graded crushed rock. Cold Regions Sci. Technol. 126, 44–50. doi:10.1016/j.coldregions.2016.03.003
Wang, T. L., and Yue, Z. R. (2013). Influence of fines content on frost heaving properties of coarse-grained soil[J]. Rock Soil Mech. 34 (02), 359–364. (in Chinese).
Xu, J., Niu, F., Niu, Y., and Liu, H. (2011). Analysis of the effect of replacement method on suppressing frost heave of railway subgrade in seasonal frozen soil area[J]. China Railw. Sci. 32 (05), 1–7. (in Chinese).
Xu, J., Niu, F., Niu, Y., Lin, Z., and Xu, Z. (2010). Experimental analysis on frost heave sensitivity of remodeled clayey loess [J]. Civ. Eng. Environ. Eng. 32 (01), 24–30. (in Chinese). doi:10.3969/j.issn.1674-4764.2010.01.005
Ye, Y., Wang, Z., Cheng, A., and Luo, M. (2007). Frost heave classification of railway subgrade filling material and the design of anti-freezing layer[J]. China Railway Sci. 28 (1), 1–7. (in Chinese). doi:10.3321/j.issn:1001-4632.2007.01.001
Keywords: frost heave, temperature, coarse-grained soils, fine-grained content, moisture content
Citation: Huang W, Mao X, Wu Q, Zhao S and Zhang J (2022) Experimental and analytical investigation on frost heave characteristics of coarse-grained soils under closed and open systems. Front. Earth Sci. 10:854586. doi: 10.3389/feart.2022.854586
Received: 14 January 2022; Accepted: 27 June 2022;
Published: 22 July 2022.
Edited by:
Qingbai Wu, Chinese Academy of Sciences (CAS), ChinaReviewed by:
Fujun Niu, South China University of Technology, ChinaZhanju Lin, Lanzhou Branch of Chinese Academy of Sciences, China
Copyright © 2022 Huang, Mao, Wu, Zhao and Zhang. This is an open-access article distributed under the terms of the Creative Commons Attribution License (CC BY). The use, distribution or reproduction in other forums is permitted, provided the original author(s) and the copyright owner(s) are credited and that the original publication in this journal is cited, in accordance with accepted academic practice. No use, distribution or reproduction is permitted which does not comply with these terms.
*Correspondence: Qian Wu, d3VxaWFuQGNoZC5lZHUuY24=