- 1Central-Asian Institute for Applied Geosciences (CAIAG), Bishkek, Kyrgyzstan
- 2Key Laboratory of Tibetan Plateau Environment and Land Surface Processes, Institute of Tibetan Plateau Research, Chinese Academy of Sciences, Beijing, China
- 3Program of Field Research in the Environmental Sciences, Niigata University, Niigata, Japan
The northern Tien Shan Mountains are one of the most hazardous areas in Kyrgyzstan due to frequent glacial lake outburst floods (GLOFs). The current status of glacial lakes in this region has not been reported in recent years. Therefore, this study investigates the variations of glacial lakes in the Kyrgyz and Teskey ranges using Sentinel-2 and PlanetScope optical satellite images from 2017 to 2019. Results confirm that there are 800 glacial lakes, of which 351 were in the Kyrgyz and 449 were in the Teskey Range. We found seasonality in the character of glacial lakes, most of which have been found to be unstable during our observation period. 242 glacial lakes showed significant variations in area. We found 46 newly emerging glacial lakes, five of which have rapidly expanded in the year since they formed, while 18 were found to have vanished. Thirteen lakes were found to be short-lived or unstable. In this study, 14 lakes showed a significant expansion of area. Such large variations in the glacial lakes are closely linked to local geomorphological conditions, local climate and glacier melt. We also identified a significant shift in the number of glacial lakes at altitudes over 3,500 m asl. It is associated with the mountain permafrost zone and variations of the glacier terminus. The current study recommends detailed investigation of glacial lakes in each season with the use of remote sensing and field surveys.
Introduction
The northern Tien Shan contains hundreds of glacial lakes (Jansky et al., 2010; Bolch et al., 2011; Narama et al., 2015; Daiyrov et al., 2018) and it is considered one of the most hazardous areas in Kyrgyzstan due to frequent glacial lake outburst floods (GLOFs) (Kubrushko and Staviskiy, 1978; Kubrushko and Shatravin, 1982; Baimoldaev and Vinohodov, 2007; Erokhin, 2008, 2017; Narama et al., 2009, 2010a, 2018; Janský et al., 2010; Daiyrov et al., 2020).
The majority of glacial lakes in the 2000s in the region developed mainly since the 1980s (Narama et al., 2009). These lakes are smaller than those in the Himalaya (Ageta et al., 2000; Komori et al., 2004; Bajracharya et al., 2007), and Andes mountains (Emmer and Vilmek, 2013, 2016a, 2016b; Cook et al., 2016; Wilson et al., 2018). However, the effects of lake draining were not proportional to their size because many residents lived near the river affected by glacier hazards (Narama et al., 2010a, 2018). They resulted in significant economic losses for local populations in Kyrgyzstan.
In recent years, the unstable situation of glacial lakes in the region has posed major risks to local communities. Several researchers have investigated the general status of glacial lakes and their characteristics of area changes in the northern Tien Shan using satellite imagery (Bolch et al., 2011; Wang et al., 2013; Kattel et al., 2020), field-based observations (Erokhin, 2008; Erokhin and Cerny, 2009; Janský et al., 2010), and mixed methods (Narama et al., 2010a, 2018; Daiyrov et al., 2018; Daiyrov and Narama, 2021). Several studies have been verified the increasing area (Bolch et al., 2011; Wang et al., 2013) and seasonal large variability (Daiyrov et al., 2018) of glacial lakes across the northern Tien Shan. This increasing trend is related to the warming of the climate and glacier shrinkage (Bolch et al., 2011; Wang et al., 2013; Kapitsa et al., 2017). In addition, the large variability of glacial lakes in terms of number is also linked to local geomorphological conditions (Daiyrov et al., 2018).
All the above-stated factors tend to increase the number of GLOF events in the area (Narama et al., 2018; Daiyrov et al., 2020). Their drainage processes have been identified by field-based observations (Erokhin, 2008; Narama et al., 2010a, 2018; Daiyrov et al., 2020; Kattel et al., 2020; Daiyrov and Narama, 2021). For recent GLOFs in the Teskey Range, a triggering mechanism for a sudden large drainage was caused by the opening of tunnel closure, which was caused by the freezing of stored water or the deposition of ice-debris mixture (Narama et al., 2010a, 2018). For another case of GLOF, triggering factors for a large drainage were an intense precipitation, a high and rapid air temperature rise and higher inflow of glacier meltwater (Erokhin et al., 2017; Daiyrov et al., 2020).
Glacial lake inventories were conducted by several researchers in the Kyrgyz and Teskey ranges to update the database of glacial lakes (Erokhin, 2008; Janský et al., 2006, 2010; Daiyrov and Narama, 2014; Narama et al., 2015; Kattel et al., 2020). Janský et al. (2006, 2010) and Erokhin. (2008) described the genetic types (morainic-glacial, supraglacial, etc.) of glacial lakes. Other researchers assessed their hazard levels based on the lake formation process (Jansky´ et al., 2010; Bolch et al., 2011; Narama et al., 2010a, 2018) and the criterion (e.g., the overflow of the lake basin to a critical (outburst) level, the character of the runoff from the lake, the character of the glacial lake dam, etc.) (Erokhin, 2008, 2009). Recent research (Kattel et al., 2020) also highlighted the importance of creating a spatial database inventory for glacial lakes in the Kyrgyz Range. The inventory results have indicated that the area of many lakes has been changing. Several glacial lakes have recently emerged, and their areas have been rapidly expanding (Erokhin, 2008, 2010; Daiyrov and Narama, 2014; Kattel et al., 2020).
It is important that the current state of glacial lakes be updated, and their characteristics evaluated to understand the causes of their area variation and potential for outbursts, as well as to assist the government in minimizing GLOF risks in the region. The main objective of this study is not only to examine the current status of glacial lakes but also to evaluate their spatial distribution throughout the Kyrgyz and Teskey ranges. The additional aim of this research is to evaluate the annual behavior, particularly year-by-year change, of glacial lake areas throughout 2017–2019 (update of Daiyrov et al., 2018) using satellite images. In this study, we classified the types of identified glacial lakes based on their area changing in order to understand their potential risks.
Study area
The study areas are on both flanks of the Kyrgyz Range and the northern flank of the Teskey Range (Figure 1). These ranges are located in the northern Тien Shan, cover the northern portion of Kyrgyzstan, and extend approximately 800 km from west to east. The mountains in the northern Tien Shan range in elevation from 3,000 to 4,000 m asl. These mountain rocks are mostly Precambrian, Paleozoic, and Ordovician granitoids, as well as gneisses and marbles (Geology Map of the Kyrgyz SSR of 1:500,000 Scale, 1987; Mountains of Kyrgyzstan, 2001; De Batist et al., 2002). The dissected mountainous topography has a major effect on the regional climate regime (Climate of Kyrgyz SSR, 1965). The study regions are primarily affected by the continental climate system and have spatial differences in seasonal and annual precipitation among the Tien Shan mountains (Climate of Kyrgyz SSR, 1965; Narama et al., 2010b). The annual precipitation ranges from 300 to 700 mm (Nature of the Issyk-Kul and Naryn regions, 1991; Nature of Kyrgyzstan, 2004; Daiyrov et al., 2018).
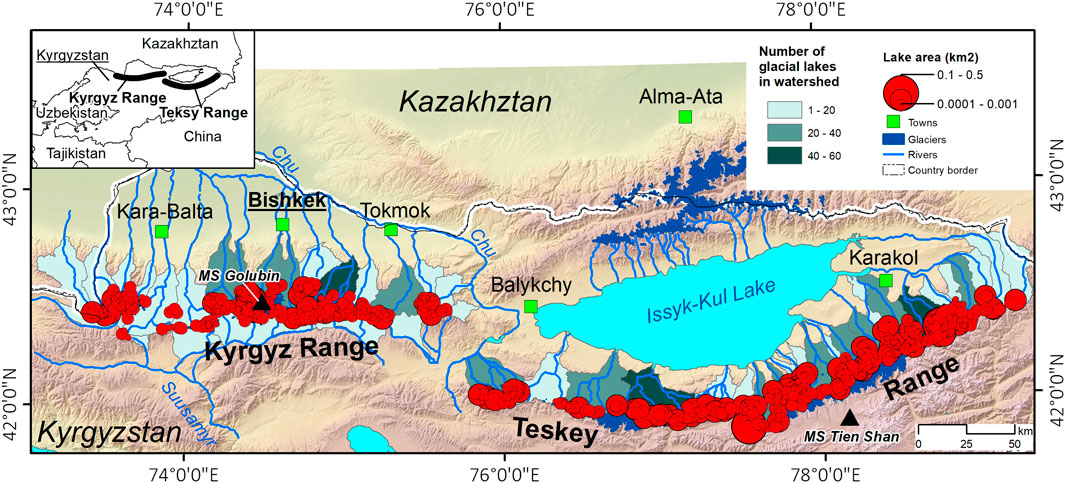
FIGURE 1. The study area and the spatial distribution of glacial lakes in the Kyrgyz and Teskey ranges.
The glacier zones are located above 3,100 m above sea level (m a.s.l.) in the northern Tien Shan. Total glacier coverage on both flanks of the Kyrgyz Range is about 520 km2 (Nature of Kyrgyzstan, 2004; Usubaliev et al., 2013) and in the northern parts of the Teskey Range is approximately 510 km2 (Usubaliev et al., 2013). Rapid atmospheric warming has had a significant impact on the decrease of glacier coverage in Kyrgyzstan in recent decades (Aizen et al., 2006; Narama et al., 2006, 2010b; Aizen et al., 2007; Bolch, 2007; Kutuzov and Shahgedanova, 2009; Kattel et al., 2020). Several studies estimate that the Teskey Range lost between 8 and 12 percent of its glacier area between 1970 and 2000 (Narama et al., 2006; Kutuzov and Shahgedanova, 2009). Furthermore, according to Bolch (2015), the area of glacier cover in the Ala-Archa river basin of the Kyrgyz Range has been reduced by 18% from 1964 to 2010 (Bolch, 2015). As a result, several recent studies have showed that many glacial lakes have developed at glacier fronts and on debris surfaces in the Kyrgyz and Teskey ranges (Narama et al., 2015; Daiyrov et al., 2018; Kattel et al., 2020), which is a major concern of this study. As many towns and villages have been developed along the alluvial fan of these glacial lakes, they are now very susceptible to outburst floods (Narama et al., 2010b).
Data and methods
In this study, Sentinel-2 and PlanetScope satellite images were used to investigate the interannual area changes of glacial lakes on both flanks of the Kyrgyz Range and the northern flank of the Teskey Range between 2017 and 2019. For the study, 43 Sentinel-2 images (21 for Kyrgyz and 22 for Teskey ranges) and 62 PlanetScope images (11 for Kyrgyz and 51 for Teskey) from the months of July, August, September, and October were utilized (Table 1). PlanetScope images have spatial resolutions ranging from 3 to 5 m, with a frame size of ∼25.5 km × 8.5 km. The time intervals of these satellites are varied. The daily nadir is also affected by the terrain. It is worth noting that the above-mentioned image resolution enables the mapping of glacial lakes with an extent of less than 0.001 km2. Due to a lack of data (all data for Teskey Range for 2018) and a limited occupancy area, the majority of the data used in this study are retrieved from Sentinel-2 (10 m resolution). It is because all the data from this satellite is publicly accessible, and the product sizes vary from 100 km × 100 km.
When comparing the two Sentinel-2 and PlanetScope image sources, the pixel differences of small lakes (0.0001–0.001 km2) were found to have a value of ±24 m2 on the same acquisition day. This value is acceptable to extract glacial lakes primarily from Sentinel-2 with area values of less than 0.001 km2. However, the extraction of micro-glacial lakes with area values ranging from 0.0001 to 0.0002 km2 from the same Sentinel-2 source is unsatisfactory due to high uncertainty. Therefore, we utilized PlanetScope Satellite data to extract all micro-glacial lakes. This research used similar polygon data from Sentinel-2 to make annual assessments of certain micro-lakes, like those that were shown in PlanetScope images.
All polygons for each lake were manually delineated on bands 1, 2, and 3 (RGB) images. This color is also better for extracting the surface water. Glacial lakes, which are found on moraine complexes including ice at glacier fronts, were chosen for this study. These glacial lakes have developed at the glacier terminus or on the moraine complex and have a significant potential to cause GLOFs (Narama et al., 2010a, 2018; Daiyrov et al., 2020). In this study, we did not consider lakes on the clean glacier surfaces and supraglacial ponds because there are only two in the whole study region.
ALOS/PRISM DSMs (2006–2011) and WorldView DSMs data were used to determine the elevation of the lakes. Please note that the DEMs data set that we have used in this study has already been processed by the provider. The Ministry of Economy, Trade and Industry (METI), Japan and the Japan Aerospace Exploration Agency (JAXA), are the data providers. We extracted elevation information of the lakes in ArcGIS using the Spatial Analyst Tools package after conversion from polygon data to point data. These datasets have a resolution of 12 and 8 m, respectively. However, since the WorldView DSM data only covers one-third of the study area, we utilized the ALOS/PRISM DSMs data set primarily for the Teskey Range, as well as for the whole study area. In this study, information about glacial lakes includes the lake ID, which is based on the inventory of the Ministry of Emergency Situations of the Kyrgyz Republic (MES), as well as the lake area size and coordinates (latitude and longitude). The area of glacial lakes in this study is divided into four classes: 0.0001–0.001, 0.001–0.01, 0.01–0.1 and 0.1–0.5 km2.
We analyzed the annual areal changes for each lake in this study to identify the actual differences between 2017 and 2019. The variation of glacial lakes over a 3-year period was used to classify the identified lakes in this study into six primary categories (e.g., stable, increasing, decreasing, appearing, vanishing, and short-lived types) based on Daiyrov et al. (2018) guidelines. The present study considered evaluating year-to-year changes in the area of glacial lakes. Each lake type was assigned based on its change in character from August 2017 to August 2018, and August 2018 to August 2019. We used a threshold of 30% of the 2017 lake area to distinguish between “change” and “no change.” For example, if the lake area remains the same or changes from 0 to 30% of its initial extent, it is considered “no change”. If the lake area grows or decreases by more than 30% of its original extent, we count it “differently”.
If there are no changes in the lake area during a 3-year period, we classify it as “stable”. We classify this type of lake as “growing” or “increasing” when the lake area continues to grow. If the area of glacial lakes identified declines or disappears over a 3-year period, we refer to these as “decreasing” or “vanishing” types. The newly appearing lakes during the 3-year observation period were classified as “appearing” types. A “short-lived” type was classified according to its seasonal living period. This means that a lake’s formation, growth, and disappearance may occur over the course of several months in a single year.
All glacial lakes in this study were manually digitized. Glacial lakes whose areas are too small (e.g., 0.0001–0.0002 km2) to delineate may have large errors. The reason is due to the difficulty in distinguishing water from wetlands (wet area) or water from shadows. The pixel size of the image does not define the lake area outline, so we counted this as uncertainty. Therefore, for uncertainty estimation, we considered the relationship between the area of the glacial lake and the spatial resolution of the image using the method proposed by Hanshaw and Bookhagen (2014).
In this method, we calculated the number of pixels that comprise the area measurements outline by dividing the perimeter (P) by the grid-cell size (G). Using an assumption of ∼69% of peripheral pixels with errors, multiply this number by 0.6872 (Error
As shown in Eq. 1
In Eq. 2, D is the relative error in percent, and A is the total area of the glacial lake in square kilometers. There were, however, fewer such uncertainty cases in our study [2.07% (17 small lakes) of the total 800 lakes], which accounts for the average relative area error of 29%. Overall, the observed relative errors for all glacial lakes in this study range from 0.41 to 20%. The relative error of the glacial lakes in this study decreased as the area of the glacial lakes increased, which is also consistent with earlier observations of Li et al. (2022) from the Hindu Kush, Karakoram, and the Himalayan mountainous region.
We analyzed the summer temperature anomaly and precipitation from May to October of each year (2017–2019), using 11-days running means (Daiyrov et al., 2018) to examine the effects of local climate on lake expansions. For the Kyrgyz Range, we used data from the “Golubin” meteorological station (3,305 m asl.) and for the Teskey Range, we used the “Tien Shan” meteorological station data (3,614 m asl.).
Results
Glacial lake inventory
In this study, we extracted 800 glacial lakes. Furthermore, we have developed diagrams to explain the number of each lake type as well as to clearly illustrate their distribution by elevation. Out of 800, 351 glacial lakes were found on both flanks of the Kyrgyz Range and 449 on the northern flank of the Teskey Range (Figure 1). The glacial lakes are evenly distributed among them. On the other hand, the glacial lakes in the Teskey Range are larger in size than the lakes in the Kyrgyz Range. The evaluations of satellite images over the whole period (2017–2019) indicate a modest increase in the number of glacial lakes. For example, the number of glacial lakes was 772 in 2017, but it increased to 800 in 2019 (Figure 2A). Moreover, in 2017, 347 glacial lakes were identified in the Kyrgyz Range, with five new lakes added, and 425 in the Teskey Range, with 29 new lakes added.
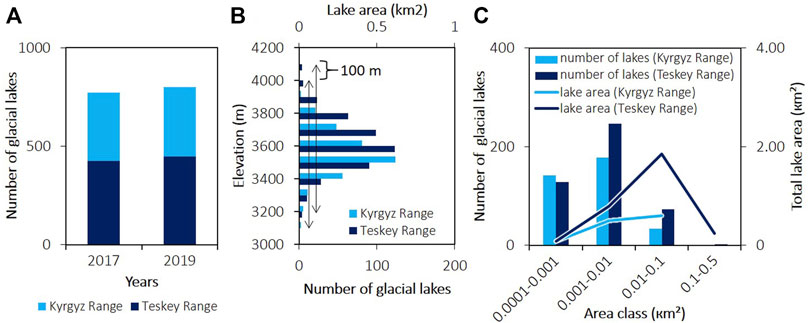
FIGURE 2. A static comparison of glacial lakes between the Kyrgyz and Teskey ranges. The Figure shows the number of glacial lakes between 2017 and 2019 (A), the distribution of glacial lakes with elevations (B), and the number and area class of glacial lakes (C).
The elevation overlaps of glacial lakes in 2019 between the Kyrgyz and Teskey ranges is less than or equal to 100 m (Figure 2B). The lowest elevation range of glacial lakes in the study region starts at 3,100 m asl., and they are spread up to 4,200 m asl. The majority of glacial lakes in the Kyrgyz Range are located between 3,500 and 3,600 m asl., while the Teskey Range has the greatest concentration of glacial lakes between 3,600 and 3,700 m asl., which is 100 m higher.
In 2019, the total area of all 800 glacial lakes in the region is estimated to be 4.13 km2. Approximately 87% of the glacial lakes in these areas are micro (0.0001–0.001 km2) and small (0.001–0.01 km2) in size (Figure 2C). In the same year (2019), 269 micro (34% of total lake number) and 423 small-type (53% of total lake number) lakes were identified, accounting for approximately 35% of the glacial lake area coverage in the study region. The Kyrgyz Range has 33 (9% out of 351) and the Teskey Range has 73 (16% out of 449) large lakes, each with an extent ranging from 0.01 to 0.1 km2. The size of these two lakes is the largest in the Teskey Range, with values ranging from 0.1 to 0.5 km2. Despite the fact that there are fewer large lakes (0.01–0.1 km2) than other types of lakes, the overall area of large lakes (59% of the total area of all lakes) is the greatest in both ranges when compared to the total area of all other types of lakes.
Consider the distribution of glacial lakes by watersheds; the majority of lakes in the Kyrgyz Range are located on the northern flank (Figure 3A). There are 304 glacial lakes on the northern flank of the Kyrgyz Range. Along the Kyrgyz Range, 47 glacial lakes were identified in the Issyk-Ata river basin in 2019, the most ever reported among other river basins in this range. The number of lakes in each of the five river basins is between 20 and 40. The remaining 17 river basins in the region have at least one glacial lake each, with a maximum of 20 glacial lakes. All the glacial lakes are distributed uniformly along the northern flank of the Teskey Range (Figure 3B). In contrast to the other river basins in this range, the Ton river basin has the most glacial lakes (57). Ten river basins include 20–50 glacial lakes in the Teskey Range. The number of glacial lakes in the other thirteen river basins of this range ranges from one in some basins to twenty in others.
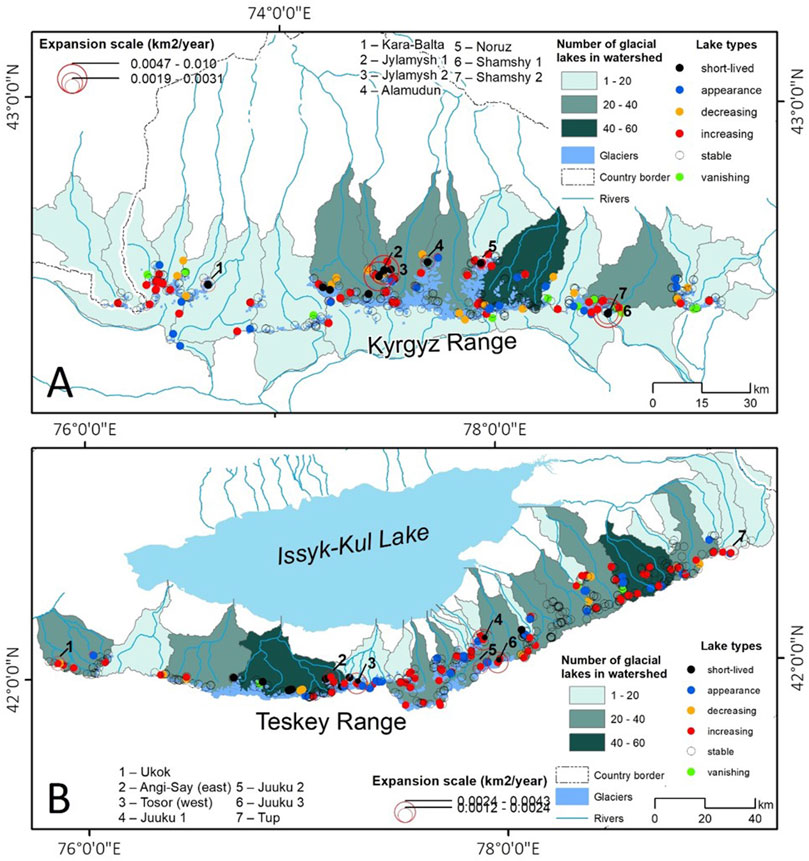
FIGURE 3. Lake type distribution by watershed in Kyrgyz (A) and the Teskey (B) ranges. A circle in red color shows the location of 14 large expansion lakes.
Variations of glacial lakes
Data assessments during the past 3 years (2017–2019) show that the extent of the majority of glacial lakes (576) in both ranges is stable, accounting for 72% of all lake types (Figure 4). However, there was a significant shift in the dynamics of 242 glacial lakes, accounting for 30% in both areas. The area of 136 glacial lakes has increased significantly, while the area of 29 other lakes has decreased. Forty-six new glacial lakes appeared between 2017 and 2019, while 18 glacial lakes disappeared (note: vanished lakes were not counted in the total of 800 lakes in 2019 due to had already disappeared). This study also identified 13 short-lived types of glacial lakes throughout this time period.
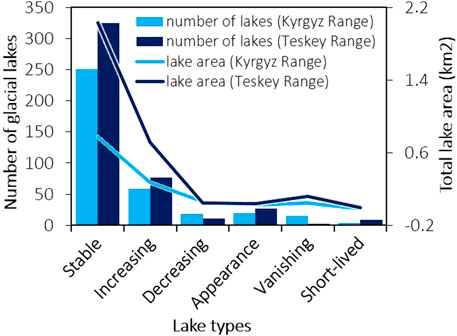
FIGURE 4. Lake types by number based on lake area changes from 2017 to 2019, and the total area of each type.
This study also evaluates the areal expansion of glacial lakes in both mountain ranges between 2017 and 2019. The findings indicate that even in a short period of time, the 14 glacial lakes in both study areas have seen significant areal expansion (Figure 5). We investigated the expansion scale of these lakes in order to get a better understanding of their precise information.
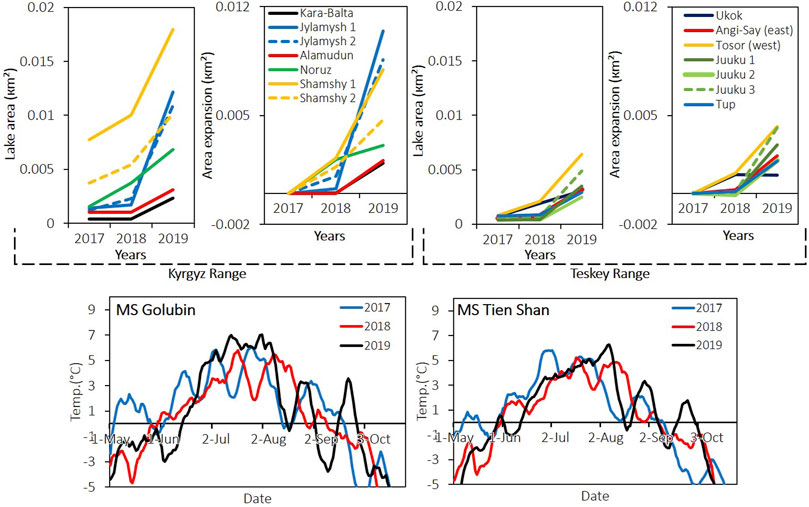
FIGURE 5. The fourteen glacial lakes’ largest area changes, as well as their rate of expansion, from 2017 to 2019. In Figures 3A,B, their locations are depicted. The below panels show an 11-day running mean of summer temperature (May–October) anomaly in 2017–2019 at the Golubin (Kyrgyz Range) and Tien Shan (Teskey Range) meteorological stations (for locations, see Figure 1).
Our results show that the rate of expansion between 2018 and 2019 was rapid for nine lakes. The area of Jylamysh 1 and 2 glacial lakes, as well as Shamshy 1 glacial lake, has rapidly expanded in comparison to the other lakes in the region. Jylamysh 1 glacial lake experienced the fastest rate of expansion (0.010 km2/year) between 2018 and 2019 (largest rate in study area). From 2017 to 2018, the increased area of Jylamysh 2 lake was recorded as 0.0011 km2, but from 2018 to 2019, it has grown even more, reaching a value of 0.0086 km2. Shamshy 1 glacial lake in the Kyrgyz Range, expanded by 0.0022 km2 in 2018 compared to its original area and continued to grow until 2019, when it reached 0.0079 km2. The extended area of Shamshy 2 lake was 0.0017 km2 in 2017–2018, but this area has increased by 0.0047 km2 in 2018–2019. In the Teskey Range, between 2018 and 2019, the area of six glacial lakes (Angi-Say east, Juuku 1, 2 and 3, Tosor-west, and Tup) was significantly expanded. The glacial lakes Tosor-west (0.0043 km2) and Juuku 3 (0.0042 km2) showed the greatest expansion in their areas between 2018 and 2019.
In this study, we also identified sudden expansions of five newly appeared lakes within a year. Since their emergence, these lakes have grown in size. However, their expansion scale was significantly smaller than compared to above 14 glacial lakes. The areas of two short-lived lakes in the Sokuluk and one in the Ala-Archa river basins from the Kyrgyz Range, as well as five short-lived lakes in the Ton and one in the Kichi-Kyzylsu river basin from the Teskey Range, have changed dramatically between 2017 and 2019.
The local short-term summer temperature anomaly of MS Golubin station was higher in 2019 (Figure 5) than those of the Teskey Range (Figure 5, MS Tien Shan). However, the role of precipitation in this phenomenon was not pronounced in the study region.
Discussion
Change of variation type in lake area
The characteristics of variations in lake area in the Kyrgyz and Teskey ranges are seasonal, and they were comparable during the entire period of observation (2017–2019). Daiyrov et al. (2018) and Kattel et al. (2020) also reported that the extent of many lakes in this range has been changing with their types by season every year.
The causes of the seasonal variation in the regional glacial lakes are linked to the local geomorphological conditions (Daiyrov et al., 2018; Narama et al., 2018; Daiyrov and Narama, 2021). Glacier recessions because of rising air temperatures (Bolch et al., 2011; Wang et al., 2013; Kapitsa et al., 2017; Daiyrov et al., 2018) are thought to be a contributing factor, particularly for the variations of some lake types (e.g., stable and increasing). These also contribute elements to the emergence of new glacial lakes, as well as their expansion in quantity and size in the region, in a very short period of time (2017–2019). There is also a strong possibility of vanished glacial lakes re-emerging in the future in the region. Our findings indicate that the area has seen multiple outbursts of re-appearing short-lived type glacial lakes. A good illustration of this is Angi-Say (Kubrushko and Staviskiy, 1978; Kubrushko and Shatrabin, 1982; Yerokhin, 2008; Narama et al., 2010a) and Toguz-Bulak (Daiyrov et al., 2020) glacial lakes.
Our study also shows that numerous stable-type glacial lakes in the region are likely to be drained. For example, the Chelektor lake was stable for a long period, but its area grew substantially in 2017, resulting in a rapid outburst that caused devastating flooding downstream. Although the majority of glacial lakes are small in size, they may cause large drainages (Narama et al., 2010a, 2018). Specifically, our observation suggests that the drainage from the glacial lakes through the moraines underground may be more dangerous (e.g., a short-lived type reported by Narama et al. (2010a, 2018), Daiyrov et al. (2018), and Daiyrov and Narama (2021), because it is more susceptible to outbursts than other types of lakes. They reappear after the outbursts, which are more sensitive to drainage repetition. Thus, it is important to use the above factors to evaluate regional glacial lake outburst hazards, like the existence of a lake basin, buried ice, and ice-tunnel in the moraine complex.
Therefore, the current effort will continue to investigate all types of glacial lakes in the future, even those that are empty or have disappeared in the Tien Shan of Kyrgyzstan. We should also look at their area changes in detail each season and how they change within the melting season in the future.
High expansion scale of glacial lakes on moraine complex
We found that the area of several glacial lakes grew significantly between 2018 and 2019. In this study, the ratio of lake expansion to size was found to be relatively large in both the Kyrgyz and Teskey ranges, and it occurred in only one to 3 years. Even some glacial lakes in the study area showed sudden expansion for a short period until they emptied (Narama et al., 2018). The annual area expansion rate for three glacial lakes, Jylamysh 1 (0.010 km2/year), Jylamysh 2 (0.0086 km2/year) and Shamshy 1 (0.0079 km2/year) were large expansion. Between 2010 and 2014, the Jylamysh 1 lake, which is classified as ID_K35 in the Glacial Lake Inventory (Narama et al., 2015), showed higher areal growth, and the whole water body of this lake was nearly drained in 2014. Since then, the area of the nearly empty Jylamysh 1 lake has increased significantly.
Large drainage cases of short-lived glacial lakes are linked to local geomorphological conditions (moraine complex) and rapid glacier melting (Narama et al., 2010a, 2018). Daiyrov et al. (2018) showed that local geomorphological factors are the main factors that cause the expansion of glacial lakes over a short period of time, rather than air temperature or glacier shrinkage in the Teskey Range. Therefore, we also agree that the reason for the significant variation in the glacial lake in the region within a short period (2017–2019) is above-mentioned factors, like local geomorphological conditions, local climate, and glacier melting. Our climate data analysis can be a supplement to the above statement.
Figure 6 also demonstrates that over 3,500 m asl, glacial lakes shifted significantly in the variations. In particular, the increasing and newly appeared types of glacial lakes were identified in a high number from 3,500 to 4,000 m asl. This is explained by the fact that glacial lakes above 3,500 m asl. are located on the moraine complex, including ice, and some are located in the glacial terminus, i.e., there are favorable conditions for glacial lake formations. The moraine complex under mountain permafrost zone are the main sources of glacial lake formation and growth in the study region. This was also confirmed by Daiyrov et al. (2018) in their research. In addition, many glaciers terminus are located around the elevation.
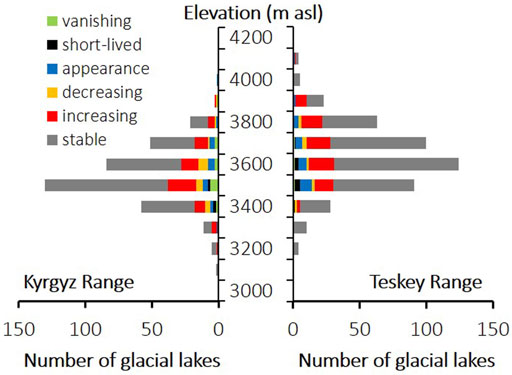
FIGURE 6. A variation in glacial lake dynamics by elevation between 2017 and 2019 in the study region.
Glaciers are susceptible to melting under atmospheric warming (Narama et al., 2006, 2010b; Bolch, 2015), which increases the area of glacial lakes. In the terminal moraines, the ground ice also melts, subsides, and further forms a lake basin (Daiyrov et al., 2018) and enlarges it by thermal erosion (Kääb and Haeberli, 2001; Kropáček et al., 2015), which results in appearance of a glacial lake during the summer season.
Conclusion
This study evaluates variations of glacial lakes in the Kyrgyz and Teskey ranges of the northern Tien Shan, as well as the potential risk hazards of glacial lakes identified in the region based on their spatial distribution and annual changes in area. The number of glacial lakes in Kyrgyzstan has increased from 772 in 2017 to 800 in 2019. The Teskey Range has more glacial lakes (449) than the Kyrgyz Range (351).
The highest number of glacial lakes is found between 3,500 and 3,600 m asl in Kyrgyz and 3,600–3,700 m asl in the Teskey ranges. Most glacial lakes are of micro and small sizes. The majority of the glacial lakes in the Kyrgyz and Teskey ranges are stable. However, the change of the 242 lakes found in both areas revealed a significant areal variation. This study identified 13 glacial lakes of the short-lived type.
The study identified large variation in the size of existing lakes in both ranges over a short period, but the Teskey Range showed the most variation, particularly in 2019. The area of glacial lakes has increased significantly (136 out of 242) in the Kyrgyz Range. In this study, 14 lakes have seen significant areal expansion in both ranges, but the areas of three lakes in the Kyrgyz Range have expanded dramatically, posing an outburst risk. A total of five new lakes appeared during our study period, expanding rapidly the same year they appeared.
The identified lakes in this study cover a total of 4.13 km2. Although larger lakes (0.01–0.10 km2) are rarer, their total area (59%) exceeds the area of all other types of lakes. The result showed that glacial lake expansion in the northern Tien Shan is faster than in the Himalaya, which is associated with the local geomorphological characteristics as well as rapid glacier retreat. More research on lake basin hydrology is needed, using both remote sensing and in situ observational techniques, to better understand the specific causes of lake area expansion and to assess the outburst potential.
Data availability statement
Publicly available datasets were analyzed in this study. This data can be found here: https://apps.sentinel-hub.com; https://www.planet.com; https://earthexplorer.usgs.gov (Further inquiries can be directed to the corresponding author).
Author contributions
All authors listed have made a substantial, direct, and intellectual contribution to the work and approved it for publication.
Funding
The study is supported by the Second Tibetan Plateau Scientific Expedition and Research Program (STEP) (2019QZKK0208), the Strategic Priority Research Program of the Chinese Academy of Sciences (XDA20100300), and National Natural Science Foundation of China (Grant No. 41771088). This study used ALOS satellite image data from ALOS Research Announcement (RA) in the framework of JAXA EORC.
Acknowledgments
We also thank the two reviewers and the editor for their constructive comments to improve the quality of this manuscript.
Conflict of interest
The authors declare that the research was conducted in the absence of any commercial or financial relationships that could be construed as a potential conflict of interest.
Publisher’s note
All claims expressed in this article are solely those of the authors and do not necessarily represent those of their affiliated organizations, or those of the publisher, the editors and the reviewers. Any product that may be evaluated in this article, or claim that may be made by its manufacturer, is not guaranteed or endorsed by the publisher.
References
Ageta, Y., Iwata, S., Yabuki, H., Naito, N., Sakai, A., Narama, C., and Karma, (2000). Expansion of glacier lakes in recent decades in the Bhutan Himalayas. Wallingford, UK: IAHS Publication, 165–175.
Aizen, V., Aizen, E. M., and Kuzmichonok, V. A. (2007). Glaciers and hydrological changes in the Tien Shan: Simulation and prediction. Environ. Res. Lett. 2, 045019. doi:10.1088/1748-9326/2/4/045019
Aizen, V., Kuzmichenok, V. A., Surazakov, A. B., and Aizen, E. M. (2006). Glacier changes in the central and northern Tien Shan during the last 140 years based on surface and remote-sensing data. Ann. Glaciol. 43, 202–213. doi:10.3189/172756406781812465
Baimoldaev, T., and Vinohodov, B. (2007). Kazselezaschita—safety measures before and after the disasters. Almaty, Kazakhstan: National Academy of Sciences of the Republic of Kazakhstan. (In Russian).
Bajracharya, S. R., Mool, P. K., and Shrestha, B. R. (2007). Impact of climate change on Himalayan glaciers and glacial lakes; case studies on GLOF and associated hazards in Nepal and Bhutan. Kathmandu, Nepal: ICIMOD.
Bolch, T. (2007). Climate change and glacier retreat in northern Tien Shan (Kazakhstan/Kyrgyzstan) using remote sensing data. Glob. Planet. Change 56, 1–12. doi:10.1016/j.gloplacha.2006.07.009
Bolch, T. (2015). Glacier area and mass changes since 1964 in the Ala-Archa valley, Kyrgyz Ala-Too, northern Tien Shan. Led. I. Sneg. 129 (28), 28–39. doi:10.15356/2076-6734-2015-1-28-39
Bolch, T., Peters, J., Yegorov, A., Pradhan, B., Buchroithner, M., and Blagoveshchensky, V. (2011). Identification of potentially dangerous glacial lakes in the northern Tien Shan. Nat. Hazards 59, 1691–1714. doi:10.1007/s11069-011-9860-2
Climate of Kyrgyz, S. S. R. (1965). Editors Z. A Ryazantseva, Kyrgyzstan (Frunze city: Publisher Academy of Sciences of the Kyrgyz SSR), 290.
Cook, S. J., Kougkoulos, I., Edwards, L. A., Dortch, J., and Hoffmann, D. (2016). Glacier change and glacial lake outburst flood risk in the Bolivian Andes. Cryosphere 10, 2399–2413. doi:10.5194/tc-10-2399-2016
Daiyrov, M., and Narama, C. (2014). Proceedings of international conference “Remote- and Ground based Earth observations in Central Asia”. Kyrgyzstan: Bishkek, 250–253.Glacier lake inventory in the southern slope of Kungoy-Alatoo Range, in the Tien Shan Mountains, using ALOS and Landsat satellite data
Daiyrov, M., Narama, C., Yamanokuchi, T., Tadono, T., Kääb, A., and Ukita, J. (2018). Regional geomorphological conditions related to recent changes of glacial lakes in the Issyk-Kul basin, northern Tien Shan. Geosciences 8, 99. doi:10.3390/geosciences8030099
Daiyrov, M., Narama, C., Kääb, A., and Tadono, T. (2020). Formation and outburst of the Toguz-Bulak glacial lake in the northern Teskey Range, Tien Shan, Kyrgyzstan. Geosciences 10 (11), 468. doi:10.3390/geosciences10110468
Daiyrov, M., and Narama, C. (2021). Formation, evolution, and drainage of short-lived glacial lakes in permafrost environments of the northern Teskey Range, Central Asia. Nat. Hazards Earth Syst. Sci. 21, 2245–2256. doi:10.5194/nhess-21-2245-2021
De Batist, M., Imbo, Y., Vermeesch, P. M., Klerkx, J., Giralt, S., Delvaux, D., et al. (2002). “Bathymetry and sedimentary environments of Lake Issyk-Kul, Kyrgyz Republic (Central Asia): A large, high altitude, tectonic lake,” in book: Lake Issyk-Kul: Its Natural Environment. Editors J. Klerkx, and B. Imanackunov (Dordrecht, Netherlands: Kluwer Academic Publishers), 101–123.
Emmer, A., and Vilimek, V. (2013). Review article: Lake and breach hazard assessment for moraine-dammed lakes: An example from the Cordillera Blanca (Peru). Nat. Hazards Earth Syst. Sci. 13, 1551–1565. doi:10.5194/nhess-13-1551-2013
Emmer, A., Vilímek, V., and Zapata, M. L. (2016a). Hazard mitigation of glacial lake outburst floods in the Cordillera Blanca (Peru); the effectiveness of remedial works. J. Flood Risk Manag. 11, 489–501. doi:10.1111/jfr3.12241
Emmer, A., Klimes, J., Mergili, M., Vilimek, V., and Cochachin, A. (2016b). 882 lakes of the Cordillera Blanca: An inventory, classification, evolution and assessment of susceptibility to outburst floods. Catena 147, 269–279. doi:10.1016/j.catena.2016.07.032
Erokhin, S. (2008). “Data report of glacial lakes in 2000–2008,” in book: Inventory of glacial lakes, Ministry of Emergency Situations of the Kyrgyz Republic, Bishkek, Kyrgyzstan. (In Russian).
Erokhin, S., and Cerny, M. (2009). “Monitoring of outbursting lakes of Kyrgyzstan,” in Material of the international conference “Mitigation of natural hazards in mountain areas”, Bishkek, 30–34.
Erokhin, S., Zaginaev, V., Meleshko, A., Ruiz-Villanueva, V., Petrakov, D. A., Chernomorets, S. S., et al. (2017). Debris flows triggered from non-stationary glacier lake outbursts: The case of the Teztor Lake complex (Northern Tian Shan, Kyrgyzstan). Landslides 15, 83–98. doi:10.1007/s10346-017-0862-3
Geological Map of the Kyrgyz SSR of 1:500,000 Scale (1987). Bishkek, Kyrgyzstan: Academy of Sciences of the Kyrgyz SSR, 38–39.
Hanshaw, M. N., and Bookhagen, B. (2014). Glacial areas, lake areas, and snow lines from 1975 to 2012: Status of the Cordillera Vilcanota, including the Quelccaya ice cap, northern central Andes, Peru. Cryosphere 8, 359–376. doi:10.5194/tc-8-359-2014
Janský, B., Šobr, M., and Engel, Z. (2010). Outburst flood hazard: Case studies from the Tien-Shan Mountains, Kyrgyzstan. Limnologica 40, 358–364. doi:10.1016/j.limno.2009.11.013
Janský, B., Šobr, M., and Yerokhin, S. A. (2006). Typology of high mountain lakes of Kyrgyzstan with regard to the risk of their rupture. Limnol. Rev. 6, 135–140.
Kääb, A., and Haeberli, W. (2001). Evolution of a high-mountain thermokarst lake in the Swiss Alps. Arct. Antarct. Alp. Res. 33, 385–390. doi:10.1080/15230430.2001.12003445
Kapitsa, V., Shahgedanova, M., Machguth, H., Severskiy, I., and Medeu, A. (2017). Assessment of evolution and risks of glacier lake outbursts in the Djungarskiy Alatau, Central Asia, using Landsat imagery and glacier bed topography modelling. Nat. Hazards Earth Syst. Sci. 17, 1837–1856. doi:10.5194/nhess-17-1837-2017
Kattel, D. B., Mohanty, A., Daiyrov, M., Wang, W., Mishra, M., Kulenbekov, Z., et al. (2020). Evaluation of glacial lakes and catastrophic floods on the northern slopes of the Kyrgyz Range. Mt. Res. Dev. 40 (3), R37–R47. doi:10.1659/MRD-JOURNAL-D-19-00068.1
Komori, J., Gurung, D. R., Iwata, S., and Yabuki, H. (2004). Variation and lake expansion of Chubda Glacier, Bhutan Himalayas, during the last 35 years. Bull. Glaciol. Res. 21, 49–55.
Kropáček, J., Neckel, N., Tyrna, B., Holzer, N., Hovden, A., Gourmelen, N., et al. (2015). Repeated glacial lake outburst flood threatening the oldest Buddhist monastery in North-Western Nepal. Nat. Hazards Earth Syst. Sci. 15, 2425–2437. doi:10.5194/nhess-15-2425-2015
Kubrushko, S. S., and Shatravin, V. I. (1982). Long-term prediction of glacial mudflows in Tien Shan. Data Glaciol. Stud. 43, 60–62. (In Russian).
Kubrushko, S. S., and Staviskiy, Y. S. (1978). Glacier lakes of Kyrgyzstan and their role in mud flow formation. Data Glaciol. Stud. 32, 59–62. (In Russian).
Kutuzov, S., and Shahgedanova, M. (2009). Glacier retreat and climatic variability in the eastern Teskey-Alatoo, inner Tien Shan between the middle of the 19th century and beginning of the 21st century. Glob. Planet. Change 69, 59–70. doi:10.1016/j.gloplacha.2009.07.001
Li, W., Wang, W., Gao, X., Wang, X., and Wang, R. (2022). Inventory and spatiotemporal patterns of glacial lakes in the HKH-TMHA region from 1990 to 2020. Remote Sens. 14, 1351. doi:10.3390/rs14061351
Mountains of Kyrgyzstan (2001). Editors I. Aitmatov, I. Togoev, G. Hughes, and Zh Jeenbaev (Bishkek, Technology: Publisher: Kyrgyz National University), 14, 56–57.
Narama, C., Shimamura, Y., Nakayama, D., and Abdrakhmatov, K. (2006). Recent changes of glacier coverage in the western Terskey-Alatoo Range, Kyrgyz Republic, using Corona and Landsat. Ann. Glaciol. 43, 223–229. doi:10.3189/172756406781812195
Narama, C., Severskiy, I., and Yegorov, A. (2009). Current state of glacier changes, glacial lakes, and outburst floods in the Ile Ala-Tau and Kungöy Ala-Too ranges, northern Tien Shan Mountains. Chiriga Kronshud. 84, 22–32. doi:10.7886/hgs.84.22
Narama, C., Duishonakunov, M., Kääb, A., Daiyrov, M., and Abdrakhmatov, K. (2010a). The 24 July 2008 outburst flood at the western Zyndan glacier lake and recent regional changes in glacier lakes of the Teskey Ala-Too Range, Tien Shan, Kyrgyzstan. Nat. Hazards Earth Syst. Sci. 10, 647–659. doi:10.5194/nhess-10-647-2010
Narama, C., Kääb, A., Duishonakunov, M., and Abdrakhmatov, K. (2010b). Spatial variability of recent glacier area changes in the Tien Shan Mountains, Central Asia, using Corona (∼1970), Landsat (∼2000), and ALOS (∼2007) satellite data. Glob. Planet. Change 71, 42–54. doi:10.1016/j.gloplacha.2009.08.002
Narama, C., Daiyrov, M., Kazehare, S., Yamamoto, M., and Tadono, T. (2015). Glacier Lake Inventory of the northern Tien Shan – Kyrgyz, Kungoy, and Teskey Ala‐Too Ranges. Niigata, Niigata Printing, Japan: Report of Mountain Research Group of Niigata University.
Narama, C., Daiyrov, M., Duishonakunov, M., Tadono, T., Sato, H., Kääb, A., et al. (2018). Large drainages from short-lived glacial lakes in the Teskey Range, Tien Shan Mountains, Central Asia. Nat. Hazards Earth Syst. Sci. 18, 983–995. doi:10.5194/nhess-18-983-2018
Nature of Kyrgyzstan (2004). in book: Geography of Kyrgyzstan. Editor O. Barataliev (Asanov, U. PublisherBishkek: Kyrgyz National University), 720.
Nature of the Issyk-Kul and Naryn regions (1991). In book: Encyclopedia of the Issyk-Kul and Naryn regions. Editor M. Borbugulov (Frunze city: Academy of Sciences of Kyrgyzstan), 56–62.
Usubaliev, R., Chen, X., and Osmonov, A. (2013). “The geography of glaciation of the mountains of Kyrgyzstan,” in book: Physical geography of Kyrgyzstan (Bishkek: Academy of Sciences of Kyrgyzstan), 133–210.
Wang, X., Ding, Y., Liu, S., Jiang, L., Wu, K., Jiang, Z., et al. (2013). Changes of glacial lakes and implications in Tian Shan, Central Asia, based on remote sensing data from 1990 to 2010. Environ. Res. Lett. 8, 1–11.
Keywords: glacial lakes, area change, remote sensing, Sentinel-2, PlanetScope
Citation: Daiyrov M, Kattel DB, Narama C and Wang W (2022) Evaluating the variability of glacial lakes in the Kyrgyz and Teskey ranges, Tien Shan. Front. Earth Sci. 10:850146. doi: 10.3389/feart.2022.850146
Received: 07 January 2022; Accepted: 27 June 2022;
Published: 08 August 2022.
Edited by:
Shuhab Khan, University of Houston, United StatesReviewed by:
Leigh A. Stearns, University of Kansas, United StatesRobert McNabb, Ulster University, United Kingdom
Copyright © 2022 Daiyrov, Kattel, Narama and Wang. This is an open-access article distributed under the terms of the Creative Commons Attribution License (CC BY). The use, distribution or reproduction in other forums is permitted, provided the original author(s) and the copyright owner(s) are credited and that the original publication in this journal is cited, in accordance with accepted academic practice. No use, distribution or reproduction is permitted which does not comply with these terms.
*Correspondence: Dambaru Ballab Kattel, a2F0dGVsZGJAZ21haWwuY29t, a2F0dGVsZGJAaXRwY2FzLmFjLmNu