1 Introduction
The assumption that the majority of the Ediacaran fossil taxa on the iconic Mistaken Point E lived erect in the water column underpins inferences concerning: 1) paleoecology of early macrofossil assemblages; 2) how they reproduced; 3) importance of tiering and 4) controls on community dynamics (Mitchell et al., 2015; Mitchell et al., 2019; Mitchell and Butterfield, 2018; Mitchell and Kenchington, 2018). Recent work has cast some doubt on the erect mode of life of some elements of the Mistaken Point biota (namely Beothukis mistakensis, Charnia masoni, Charniodiscus procerus and Gigarimaneta samsoni; McIlroy et al., 2020; McIlroy et al., 2021; Taylor et al., 2021). Careful consideration of morphology, taphonomy and sedimentology have led to the proposal that the fractal-like Rangeomorpha in particular could have harboured sulfur-reducing symbionts and lived as soft sediment recliners (Dufour and McIlroy, 2017; McIlroy et al., 2020, McIlroy et al., 2021). Critical to this type of palaeobiological assessment is determining life attitude. To this end, it has been proposed that—since it seems that rangeomorphs and relatives could feasibly live in the reclining position like Fractofusus—the null hypothesis for interpreting the mode of life of the organisms in this biota should be that they lived as they are found, flat upon the ancient seafloor (McIlroy et al., 2021). This challenge to the Ediacaran palaeobiological community included recommendations for evidence that might be sought to demonstrate an erect mode of life (McIlroy et al., 2021) such as the presence of associated scratch circles (Jensen et al., 2018). Those methodologies have been used to good effect in demonstrating that Charniodiscus concentricus and Arborea spinosus lived with the frond somewhat erect (sediment-parallel recumbent) in the water column, whereas C. procerus was probably a recliner (Pérez-Pindeo et al., 2022).
2 Competing Tuffite Depositional Models for Mistaken Point E Surface
The work of Vixseboxse et al. (2021) aims to demonstrate using orientation data that all of the fronds on the Mistaken Point E surface apart from Fractofusus misrai lived erect in the water column thereby validating all the assumptions in their recent works (Mitchell et al., 2015; Mitchell and Butterfield, 2018; Mitchell and Kenchington, 2018; Mitchell et al., 2019). The iconic images of the E surface with current-aligned fronds have fuelled the idea that the overlying tuffite was deposited from a turbidity current (Benus, 1988; Seilacher, 1999; Matthews et al., 2020). Many authors have also considered that it may have also been a water-lain ash-fall tuff (Anderson and Conway Morris, 1982; Jenkins, 1992; Wood et al., 2003; Bamforth et al., 2008; Brasier et al., 2012). The evidence for tuffite deposition from turbidity currents is compelling at several levels in the Ediacaran successions of Mistaken Point Ecological Reserve (Matthews et al., 2020). However, the E Surface tuff/tuffite does not include a partial bouma sequence and is simply a normally graded bed with the composition of a volcanic ash that could be a water-lain ash-fall tuff. Arguments in favor of deposition of the E Surface tuffite by a turbidity current tend to lean heavily on the common orientation of stemmed organisms, which may impart a degree of circular reasoning if applied to this study.
The typically close-cropped image of “Seilacher’s Corner” gives the impression that all fronds have a common orientation (Figure 1A). Some high aspect ratio fronds are oriented 90o to or 180o to the inferred paleocurrent direction, an orientation that is inconsistent with felling by a turbidity current (McIlroy et al., 2021; Figure 1B). This up-current or transverse frond direction is formally documented on the E surface by Vixseboxse et al. (2021), who argue that turbulent eddies (vortices) in the heads of passing turbidity currents were able to fell their studied rangeomorphs in an upslope direction. This inference that turbidity currents can fell fronds in an upslope direction underpins the conclusions drawn from this study.
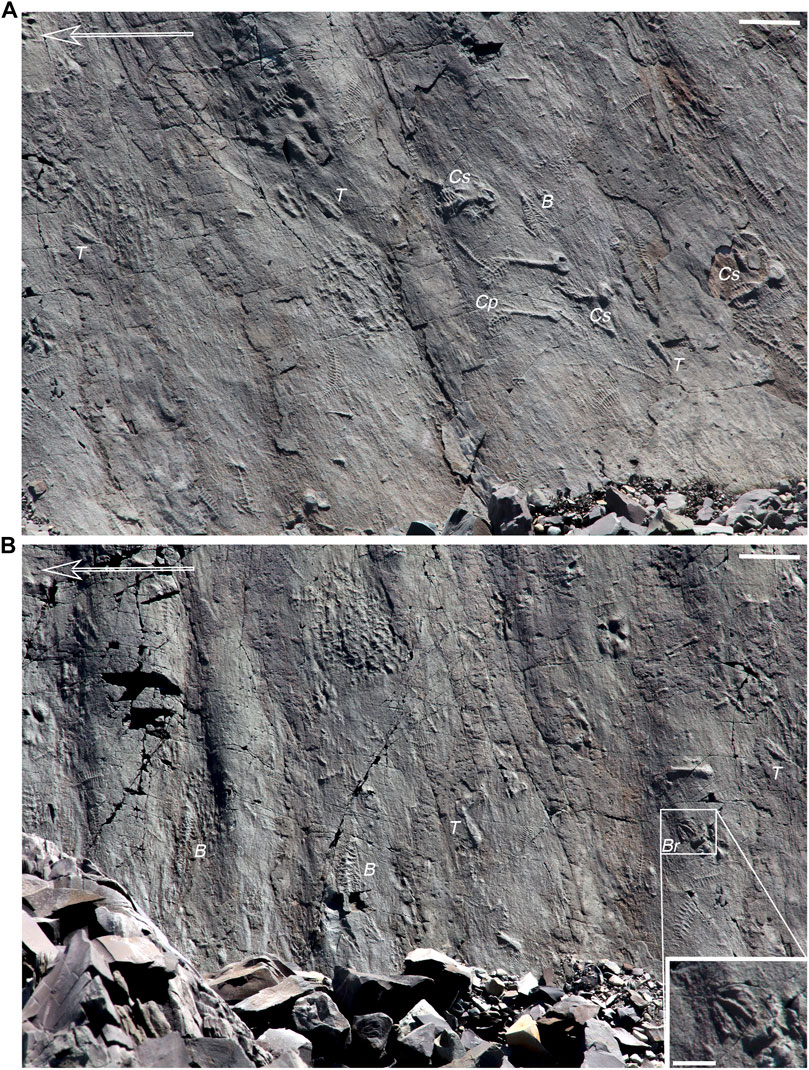
FIGURE 1. (A) Mistaken Point E Surface immediately surrounding “Seilacher’s Corner,” including several presumptively current-aligned Charniodiscus fronds (B, Beothukis; Cs, Charniodiscus spinosus; Cp, Charniodiscus procerus; T, Thectardis avalonensis); arrow indicates inferred paleocurrent direction (scale bar = 10 cm). (B) Mistaken Point E Surface immediately east of “Seilacher’s Corner,” with multiple aligned specimens of Beothukis sp. (B) and Thectardis avalonensis (T) as well as a single specimen of Bradgatia (Br) which is aligned opposite to inferred paleocurrent direction; arrow indicates inferred paleocurrent direction (scale bar = 10 cm). (B) inset, magnification of Bradgatia specimen which is aligned opposite to inferred paleocurrent direction (scale bar = 3 cm).
2.1 Turbulence in Turbidity Currents Causes Fluctuations in Strength of Downstream Velocity Not Upcurrent Flow
We note here that near-bed, upslope-directed instantaneous velocities in turbidity currents, relative to the substrate, are unknown from experiments (e.g., Baas et al., 2011), field studies (e.g., Kostaschuk et al., 2018), and bed-scale facies models including the set of models preferred by Vixseboxse et al. (2021) (i.e., Haughton et al., 2009, their Figure 1A). Instead, stream-wise turbulent fluctuations simply account for variations in the downstream velocity (e.g., Baas et al., 2011, their Figure 9; Kostaschuk et al., 2018, their figures 2, 3), with instantaneous values remaining positive (i.e., downslope) and rarely approaching zero.
“Roll waves” (Brock, 1969; Allen, 1984) such as are invoked by Vixseboxse et al. (2021) do not lead to upslope pulses in near-bed velocity. They occur at high Froude numbers (>∼2) wherever Kelvin-Helmholtz interfacial waves break and rush downslope. This process can occur along the top of the flow’s body, along density discontinuities in a stratified flow (e.g., Kneller and McCaffrey, 2003), but likely not in the head region which is thoroughly mixed by strong upward-directed turbulence. Cartigny et al. (2014); cited regarding this issue by Vixseboxse et al. (2021) only mention roll waves ‘in passing’; they do not implicate roll waves in creating near-bed, upslope-directed velocity pulses in the heads of density currents. There really is no support in the gravity-current literature for the proposal of Vixseboxse et al. (2021) that vortices in the heads of flows might account for bimodal felling directions.
The absence of sediment below the current felled fronds is also inconsistent with fronds having been held aloft by the turbidity current for a period before preservation in an up-current direction. If not adpressed onto the seafloor prior to the passage of sediment-laden currents then erect fronds could not lie on the E Surface, but would instead be present in the overlying tuffite, rendering them un-preservable except perhaps for their basal discs. The assemblage is thus best considered to be an obrution deposit that smothered the seafloor biomass and necromass (Liu et al., 2011) in its life position, probably due to ash-fall rather than felling and burial by an ash-rich turbidity current.
2.2 Up-Current Orientated Fronds Demonstrate Rheotropism in a Clear Water Current Prior to Tuffite Deposition
The excellent fossil orientation data collected by Vixseboxse et al. (2021) thus needs some reconsideration given that felling by a turbidity current cannot account for the upstream orientation of multifoliate rangeomorph frond Bradgatia and problematic sponge-like Thectardis avalonensis (see Sperling et al., 2007, but also Antcliffe et al., 2014). The data seem to support our assertion that Bradgatia grew along the seafloor and that the bimodal orientation is likely a function of rheotropic/chemotactic epifaunal growth relating to a pre-ash paleocurrent (McIlroy et al., 2021). This is in need of verification by the collection of frond orientation data that is supported by independent current indicators (i.e., physical sedimentary structures—work in progress at Memorial) in order to avoid issues with circular reasoning. The bimodal orientation of Thectardis avalonensis is consistent with the inferred filter-feeding mode of life of a sponge-like organism in which the mouth of the cone could be orientated into the current in order to funnel water, or be orientated in the down-current direction to draw water through surficial pores by Bernoulli effect. Sponges living on sediment substrates have non-feeding pinacocytes on their lower surfaces to mitigate the effects of sulfide toxicity that would otherwise cause cell death (Dufour and McIlroy 2017). The interpretation of Thectardis as a sponge-grade reclining conical suspension feeder does not preclude it also having erect growth, especially if it is not monospecific.
The orientation data of the erect-stemmed taxa do show remarkably consistent orientations among C. spinosus (Pérez-Pinedo et al., 2022), as do the closely related taxa Plumeropriscum hofmanni and Primodandelabrum sp., which meet the criteria for erect mode of life identified by Pérez-Pindeo et al. (2022). The erect taxa and some recliners (Bradgatia sp. and Charniodiscus procerus) do have a common orientation, which could simply be due to all those taxa living in response to, or falling in the direction of, a persistent clear-water bottom current (Figure 1).
2.3 The Problem of “Binning” and the Danger of Creating “Wastebasket Taxa”
The data pertaining to the binned category “Beothukis” is literally a wastebasket taxon containing several Beothukis-like taxa (i.e., Beothukis spp.). The authors note that the holotype of Beothukis has an anomalous orientation. The issue with binning taxa in this manner in statistical analyses is that while it makes for convenient treatment of lots of fossil organisms, it likely hides taxonomic, morphological and palaeobiological diversity. The authors seem to have taken some liberties with this bin, in that the paratype and three directly comparable specimens appear to have been disregarded without explanation [there are three specimens like the paratype of B. mistakensis, all of which have the same orientation as the holotype (Figure 1B)]. While it is true that Hawco et al. (2020) recovered a number of E Surface specimens in the same morphometric cluster as the holotype from the E-Surface, the same authors clearly state that the methodology can only identify morphogroups and cannot determine the taxonomic rank of those groups. We would assert from knowing the material on the E surface that the 17 “binned Beothukis” the authors report from the E Surface include “beothukids” not conspecific with the holotype, and as such do not necessarily share its reclining mode of life, living oriented transverse to their inferred paleocurrent direction. We imagine that the other “beothukids” apart from the type material did have a broadly current-parallel orientation and some may have lived erect in the water-column, perhaps accounting for the “cohorts”, but given their poor preservation and indeterminate taxonomy it would be premature to comment further. We consider that there are only two definite B. mistakensis on the E Surface, and the taxonomic status of the paratypes remains tenuous. It is clear however that the mode of life of the holotype of Beothukis mistakensis cannot be inferred to have been erect from the orientation of the multiple cohorts of binned “beothukids.”
3 Discussion
Authors of Vixseboxse et al. (2021) have previously presented a range of sophisticated paleoecological, spatial and morphometric studies on the Ediacaran biota of Mistaken Point. Recent work undermines some of the assumptions made by Mitchell and Kenchington (2018) due to reinterpretation of some key taxa as recliners (e.g., McIlroy et al., 2020; Charniodiscus procerus, Pérez-Pindeo et al., 2022), the lack of taxonomic rigor is reflected in their unexplained “binning” of taxa. The newly inferred reclining mode of life for some (but not all) taxa is a reflection of the improving palaeobiological understanding of the Mistaken Point biota, but is problematic to pre-published work quantifying epibenthic tiering and the wide-ranging inferences concerning feeding and dispersal based thereon. This earlier work has several other unexplained assumptions (e.g. 5 cm tall Fractofusus and 30 cm tall Charniodiscus) as well as the same binning issues as this work.
To explain anomalous frond orientations, Vixseboxse et al. (2021) invoke unrealistic turbidity current dynamics to explain how erect fronds were felled into the prevailing current. There is no precedent—that we know of—for tool marks or other paleocurrent indicators having a counter-current orientation in the published literature. We consider that the anomalous orientation of taxa is much better explained by growth of reclining organisms into and away from weak “blue-water” currents (McIlroy et al., 2021) rather than invoking unprecedented fluid dynamics in putative turbidity currents to explain problematic frond orientations.
Author Contributions
All authors listed have made a substantial, direct, and intellectual contribution to the work and approved it for publication.
Funding
Funding was provided to DM by Discovery Grant and 1321 Discovery Accelerator Supplement awards from the Natural Sciences and Engineering Research Council.
Conflict of Interest
The authors declare that the research was conducted in the absence of any commercial or financial relationships that could be construed as a potential conflict of interest.
Publisher’s Note
All claims expressed in this article are solely those of the authors and do not necessarily represent those of their affiliated organizations, or those of the publisher, the editors and the reviewers. Any product that may be evaluated in this article, or claim that may be made by its manufacturer, is not guaranteed or endorsed by the publisher.
References
Allen, P. A. (1984). Reconstruction of Ancient Sea Conditions with an Example from the Swiss Molasse. Mar. Geology. 60, 455–473. doi:10.1016/0025-3227(84)90162-2
Anderson, M. M., and Conway Morris, S. (1982). “A review, With Descriptions of Four Unusual Forms, of the Soft-Bodied Fauna of the Conception and St. John’s Groups (Late Precambrian),” in Proceedings of the Third North American Paleontological Convention, Avalon Peninsula, Newfoundland 1, 1–8.
Antcliffe, J. B., Callow, R. H., and Brasier, M. D. (2014). Giving the Early Fossil Record of Sponges a Squeeze. Biol. Rev. Camb Philos. Soc. 89, 972–1004. doi:10.1111/brv.12090
Baas, J. H., Best, J. L., and Peakall, J. (2011). Depositional Processes, Bedform Development and Hybrid Bed Formation in Rapidly Decelerated Cohesive (Mud-sand) Sediment Flows. Sedimentology 58, 1953–1987. doi:10.1111/j.1365-3091.2011.01247.x
Bamforth, E. L., Narbonne, G. M., and Anderson, M. M. (2008). Growth and Ecology of a Multi-Branched Ediacaran Rangeomorph from the Mistaken Point Assemblage, Newfoundland. J. Paleontol. 82, 763–777. doi:10.1666/07-112.1
Benus, A. P. (1988). “Sedimentological Context of a Deep-Water Ediacaran Fauna (Mistaken Point, Avalon Zone, Eastern Newfoundland),” in Trace Fossils, Small Shelly Fossils and the Precambrian-Cambrian Boundary. Editors E. Landing, G. M. Narbonne, and P. M. Myrow (New York: New York State Museum and Geological Survey Bulletin) 463, 8–9.
Brasier, M. D., Antcliffe, J. B., and Liu, A. G. (2012). The Architecture of Ediacaran Fronds. Palaeontology 55, 1105–1124. doi:10.1111/j.1475-4983.2012.01164.x
Brock, R. R. (1969). Development of Roll-Wave Trains in Open Channels. J. Hydr. Div. 95, 1401–1427. doi:10.1061/JYCEAJ.0002132
Cartigny, M. J. B., Eggenhuisen, J. T., Hansen, E. W. M., and Postma, G. (2014). Concentration-dependent Flow Stratification in Experimental High-Density Turbidity Currents and Their Relevance to Turbidite Facies Models. J. Sediment. Res. 83, 1046–1064. doi:10.2110/jsr.2013.71
Dufour, S. C., and McIlroy, D. (2017). Ediacaran Pre-placozoan Diploblasts in the Avalonian Biota: the Role of Chemosynthesis in the Evolution of Early Animal Life. Geol. Soc. Lond. Spec. Publications 448, 211–219. doi:10.1144/SP448.5
Haughton, P., Davis, C., McCaffrey, W., and Barker, S. (2009). Hybrid Sediment Gravity Flow Deposits - Classification, Origin and Significance. Mar. Pet. Geology. 26, 1900–1918. doi:10.1016/j.marpetgeo.2009.02.012
Hawco, J. B., Kenchington, C. G., Taylor, R. S., and Mcilroy, D. (2020). A Multivariate Statistical Analysis of the Ediacaran Rangeomorph Taxa Beothukis and Culmofrons. PALAIOS 35, 495–511. doi:10.2110/palo.2020.049
Jenkins, R. J. F. (1992). “Functional and Ecological Aspects of Ediacaran Assemblages,” in Origin and Early Evolution of the Metazoa. Editors J. H. Lipps, and P. W. Signor (Boston, MA: Springer US), 131–176. doi:10.1007/978-1-4899-2427-8_5
Jensen, S., Högström, A. E. S., Almond, J., Taylor, W. L., Meinhold, G., Høyberget, M., et al. (2018). Scratch Circles from the Ediacaran and Cambrian of Arctic Norway and Southern Africa, with a Review of Scratch circle Occurrences. Bull. Geosci. 93, 287–304. doi:10.3140/bull.geosci.1685
Kostaschuk, R., Nasr‐Azadani, M. M., Meiburg, E., Wei, T., Chen, Z., Negretti, M. E., et al. (2018). On the Causes of Pulsing in Continuous Turbidity Currents. J. Geophys. Res. Earth Surf. 123, 2827–2843. doi:10.1029/2018JF004719
Kneller, B. C., and McCaffrey, W. D. (2003). The Interpretation of Vertical Sequences in Turbidite Beds: The Influence of Longitudinal Flow Structure. J. Sediment. Res. 73, 706–713.
Liu, A. G., Mcilroy, D., Antcliffe, J. B., and Brasier, M. D. (2011). Effaced Preservation in the Ediacara Biota and its Implications for the Early Macrofossil Record. Palaeontology 54, 607–630. doi:10.1111/j.1475-4983.2010.01024.x
Matthews, J. J., Liu, A. G., Yang, C., McIlroy, D., Levell, B., and Condon, D. J. (2020). A Chronostratigraphic Framework for the Rise of the Ediacaran Macrobiota: New Constraints from Mistaken Point Ecological Reserve, Newfoundland. Geol. Soc. Am. Bull. 133, 13. doi:10.1130/b35646.1
McIlroy, D., Dufour, S. C., Taylor, R., and Nicholls, R. (2021). The Role of Symbiosis in the First Colonization of the Seafloor by Macrobiota: Insights from the Oldest Ediacaran Biota (Newfoundland, Canada). Biosystems 205, 104413. doi:10.1016/j.biosystems.2021.104413
McIlroy, D., Hawco, J., McKean, C., Nicholls, R., Pasinetti, G., and Taylor, R. (2020). Palaeobiology of the Reclining Rangeomorph Beothukis from the Ediacaran Mistaken Point Formation of southeastern Newfoundland. Geol. Mag. 1, 1–15. doi:10.1017/S0016756820000941
Mitchell, E. G., and Butterfield, N. J. (2018). Spatial Analyses of Ediacaran Communities at Mistaken Point. Paleobiology 44, 40–57. doi:10.1017/pab.2017.35
Mitchell, E. G., Harris, S., Kenchington, C. G., Vixseboxse, P., Roberts, L., Clark, C., et al. (2019). The Importance of Neutral over Niche Processes in Structuring Ediacaran Early Animal Communities. Ecol. Lett. 22, 2028–2038. doi:10.1111/ele.13383
Mitchell, E. G., Kenchington, C. G., Liu, A. G., Matthews, J. J., and Butterfield, N. J. (2015). Reconstructing the Reproductive Mode of an Ediacaran Macro-Organism. Nature 524, 343–346. doi:10.1038/nature14646
Mitchell, E. G., and Kenchington, C. G. (2018). The Utility of Height for the Ediacaran Organisms of Mistaken Point. Nat. Ecol. Evol. 2, 1218–1222. doi:10.1038/s41559-018-0591-6
Pérez-Pindeo, D., McKean, C., Taylor, R. S., and McIlroy, D. (2022). Charniodiscus and Arborea Are Separate Genera within the Arboreomorpha: Using the Holotype of C. Concentricus to Resolve a Taxonomic Tangle. Front. Earth Sci. 9, 785929. doi:10.3389/feart.2021.785929
Seilacher, A. (1999). Biomat-Related Lifestyles in the Precambrian. Palaios 14, 86–93. doi:10.2307/3515363
Sperling, E. A., Pisani, D., and Peterson, K. J. (2007). “Poriferan Paraphyly and its Implications for Precambrian Palaeobiology,” in The Rise and Fall of the Ediacaran Biota. Editors P. Vickers-Rich, and P. Komarower (London: Geological Society of London Special Publications), 286, 355–368. doi:10.1144/sp286.25Geol. Soc. Lond. Spec. Publications
Taylor, R. S., Matthews, J. J., Nicholls, R., and McIlroy, D. (2021). A Re-assessment of the Taxonomy, Palaeobiology and Taphonomy of the Rangeomorph Organism Hapsidophyllas flexibilis from the Ediacaran of Newfoundland, Canada. Paläontol. Z. doi:10.1007/s12542-020-00537-4
Vixseboxse, P. B., Kenchington, C. G., Dunn, F. S., and Mitchell, E. G. (2021). Orientations of Mistaken Point Fronds Indicate Morphology Impacted Ability to Survive Turbulence. Front. Earth Sci. 9, 762824. doi:10.3389/feart.2021.762824
Keywords: mistaken point, ediacaran, rheotropism, density currents, orientation, rangeomorph
Citation: McIlroy D, Pérez-Pinedo D, Pasinetti G, McKean C, Taylor RS and Hiscott RN (2022) Rheotropic Epifaunal Growth, Not Felling by Density Currents, Is Responsible for Many Ediacaran Fossil Orientations at Mistaken Point. Front. Earth Sci. 10:849194. doi: 10.3389/feart.2022.849194
Received: 05 January 2022; Accepted: 26 January 2022;
Published: 14 February 2022.
Edited by:
Allison Daley, University of Lausanne, SwitzerlandReviewed by:
Olev Vinn, University of Tartu, EstoniaCopyright © 2022 McIlroy, Pérez-Pinedo, Pasinetti, McKean, Taylor and Hiscott. This is an open-access article distributed under the terms of the Creative Commons Attribution License (CC BY). The use, distribution or reproduction in other forums is permitted, provided the original author(s) and the copyright owner(s) are credited and that the original publication in this journal is cited, in accordance with accepted academic practice. No use, distribution or reproduction is permitted which does not comply with these terms.
*Correspondence: D. McIlroy, dmcilroy@mun.ca