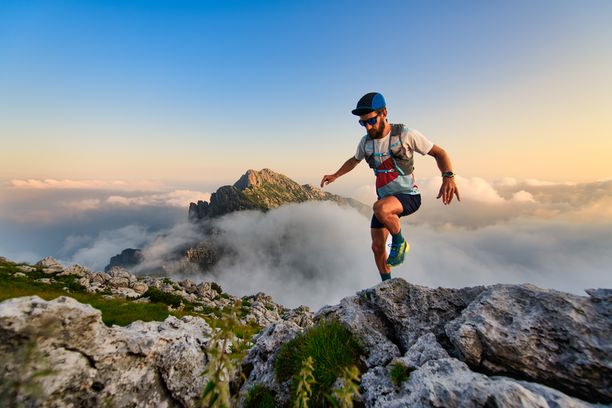
94% of researchers rate our articles as excellent or good
Learn more about the work of our research integrity team to safeguard the quality of each article we publish.
Find out more
ORIGINAL RESEARCH article
Front. Earth Sci., 10 March 2022
Sec. Structural Geology and Tectonics
Volume 10 - 2022 | https://doi.org/10.3389/feart.2022.849181
This article is part of the Research TopicContinental Basin and Orogenic Processes: Tectonic Deformation and Associated Landscape and Environmental EvolutionView all 15 articles
The Cenozoic sediments in marginal basins of East Asia ultimately reflected coupling between the tectonics, landscape evolution, and drainage reorganization. Recently, the provenance of Miocene sediments in the East China Sea Basin (ECSB) and Taiwan has been in hot debate, and several models were proposed to interpret the provenance changes. Most of them are related to river reorganization in East Asia and highly relied on detrital zircon U-Pb dating. In this study, a large number of detrital zircon U-Pb ages of Miocene sediments from the ECSB, Taiwan region, and the potential source areas have been compiled for quantitative provenance analysis. The results suggested that all the early–middle Miocene sediments in Taiwan and the ECSB were closely linked to North China and the Korean Peninsula. Over 80% sediments in Taiwan were delivered from the ECSB whose sediments were predominantly contributed by North China and the Korean Peninsula (70%). However, for the late Miocene to Quaternary sediments in the ECSB, the contribution of the Yangtze River system was 72%, which indicates distinct reorganization of river networks and initial formation of the Yangtze River in the late Miocene. The quantitative provenance analysis together with southward environmental changes from dominantly fluvial sediments in the northern and middle ECSB to shallow marine sediments in Taiwan region suggested that the early–middle Miocene sediments of Taiwan were mainly sourced from the North China and the Korean Peninsula by passing the ECSB. Thus, these sediments in Taiwan region would experience the river–delta–shallow marine route from the ECSB to Taiwan region.
The Cenozoic East Asia has experienced great tectonic movement, especially the uplift of Tibetan Plateau by India–Asia collision and the subsidence of East Asia margin associated with evolving of numerous rift basins, followed by contrasting topography and climatic changes and reorganization of continental river networks (Wang, 2004; Zheng, 2015) (Figure 1). The sedimentary record in marginal basins was believed to ultimately reflect the coupling between these deep earth and surface processes (Clift, 2006; Richardson et al., 2010).
FIGURE 1. (A) Topographic map of East Asia showing major basins and rivers. (B) Tectonic sketch of the East China Sea Basin (modified from Zhu et al., 2019). (C) Tectonic framework of Taiwan (modified from Huang et al., 2012). The basins are modified from Ren et al. (2002). ECSB, East China Sea Basin; JHB, Jianghan Basin.
As one of the ultimate destination of sediments derived from the Tibetan Plateau, the East China margin might preserve crucial evidence associated with the links between Tibetan Plateau and the sea. Recently, the provenance of Miocene sediments of the East China Sea Basin (ECSB) and Taiwan has attracted great interest and been in hot debate. A sharp provenance change was recognized at the beginning of the Miocene in the Taiwan region that the provenance of western foothills of Taiwan changed from the adjacent South China to new source areas characterized by an obvious increase in old zircon ages (Lan et al., 2014; Deng et al., 2017; Zhang et al., 2017; Chen et al., 2019). Lan et al. (2016) claimed that an enlarged paleo-Min River that extended to the Yangtze Block contributed to this change. Zhang et al. (2017) proposed that this provenance change was caused by the establishment of the Yangtze River, and Deng et al. (2017) suggested the North China Craton (NCC) also supplied detritus. In contrast to these large-scale drainage reorganization models, most recently, Chen et al. (2019) suggested that an unknown macroblock that collided with the Asian margin and now covered by thick sediments resulted in this change. Wang et al. (2018) noticed the similarities between Miocene sediments in western Taiwan and Oligocene sediments in Xihu Sag, one of the subordinate subsiding centers in the ECSB, and suggested that erosion and reworking of these Oligocene sediments were responsible for the Miocene provenance changes in western Taiwan.
Although some authors agreed with the view that Miocene sediments in Taiwan were closely linked with the contemporaneous sediments of the ECSB, the routes for sediment derived from the ECSB to paleo-Taiwan region are also under debate. Deng et al. (2017) suggested that longshore current played an important role in sediment transport from shallow marine environments of the east of the present Yangtze Delta to the Taiwan region. Xu et al. (2007) argued that the transport ability of a longshore current is limited. Zhang et al. (2017) and Zhang et al. (2021a) preferred river transport along the ECSB.
Although several different methods have been applied to trace the Miocene provenance change in Taiwan region (Lan et al., 2014; Lan et al., 2016; Chen et al., 2019), the most powerful and widely used one is the detrital zircon U-Pb dating that clearly shows obvious increase in the proportion of zircon grains with ages peaking at 220, 1800, and 2,500 Ma (Lan et al., 2016; Zhang et al., 2017). Advances in quantitative and statistical provenance analysis of detrital zircon U-Pb dating (Vermeesch et al., 2016; Sundell and Saylor, 2017), especially inverse Monte Carlo modeling, can be used to calculate mixing proportions of every source area (Sundell and Saylor, 2017). These quantitative methods together with rapid increase in detrital zircon U-Pb dating of potential areas and Cenozoic sediments in the ECSB and Taiwan region make it possible to re-examine the Miocene provenance change that occurred in Taiwan region.
In this study, we have compiled a large number of literature detrital zircon ages from Cenozoic sedimentary rocks of Taiwan region, the ECSB, and the potential areas including the adjacent rivers in South China and far inland areas of Yangtze block, North China, and Korean Peninsula to determine the proportions of different sources that fed the Miocene sediments in Taiwan region and the ECSB by inverse Monte Carlo modeling. Considering tectonics and changes of sedimentary environment in east China margin, we provide a more comprehensive understanding on drainage reorganization from the sediments in marginal basins and further constraints on sediments transported along the marginal basins.
The Taiwan island is located in the south of the East China Sea and is subducting by South China Sea plate and Luzon arc (Huang et al., 2012). The arc–continent collision between the Luzon arc and Eurasia since 6.5 Ma resulted in the basic tectonic pattern of Taiwan region (Huang, 2017). Consequently, narrow N-S tectonic belts and the high mountain ranges were formed (Chen et al., 2019). The Taiwan island can be divided into five zones: coastal plain, western foothills, the Hsuehshan Range, the Central Range, and the Coastal Range (Figure 1C). The coastal plain, western foothills, and Hsuehshan Range are located in the fold-thrust belt resulted from the deformed and uplifted passive continental margin sediments (Huang et al., 2012). The Central Range and Coastal Range are composed of accretionary prism and forearc basin–volcanic island arc, respectively. Before this collision, Taiwan region received Paleogene sediments of the rift basin and Oligocene to Miocene sediments in the passive margin setting after the opening of South China Sea (Lin et al., 2003).
The ECSB is located in the east of the present Yangtze Estuary (Figure 1). This basin is the largest Cenozoic rift basin in the east Asia margin and deposited relatively complete Cenozoic sediments and thus was believed to be one of the crucial places to trace reorganization of river networks in East Asia (Richardson et al., 2010; Fu et al., 2021). The ECSB roughly consists of two subsequently rifted zones (Figure 1B), the west zone mainly rifted from the late Mesozoic to the Paleocene and the east zone rifted during Eocene to Oligocene (Zhu et al., 2019). Both of them are composed of several sub-basins that are controlled by boundary faults. Since the Miocene, the uplifts in the ECSB were covered by sedimentary strata due to post-rift subsidence, and the ECSB became a broad continental shelf (Lee et al., 2006).
The rift and its associated sediments of the southern ECSB connected with basins around the Taiwan region (Figure 1), and the evolution of these basins have been profoundly affected by the opening of the South China Sea (Huang et al., 2012; Zhu et al., 2019).
We compiled most of the detrital zircon U-Pb ages reported by Lan et al. (2016), which covered Eocene to middle Miocene sedimentary rocks of western foothills in Taiwan. Two sand samples from the Zhuoshui River, two samples from the beach sand in northwestern and southwestern Taiwan Island, respectively (Table 1). In the ECSB, thirteen detrital zircon samples with a total of 1,161 grain ages from the Miocene to Quaternary sediments were compiled from Fu et al. (2021).
Previous studies suggested that the adjacent South China, Yangtze Block, North China Block, and Korean Peninsula could be the potential areas for the Miocene sediments in Taiwan region and the ECSB (Deng et al., 2017; Fu et al., 2021; Zhang J. et al., 2021). We used the fluvial samples that were collected from the rivers covering most part of these potential areas (Table 1; Figure 2) to represent the provenance features of the source areas, rather than the bedrock U-Pb ages, as the previous studies did (e.g., Deng et al., 2017; Fu et al., 2021). The fluvial samples are concise to represent general distribution of U-Pb ages of source areas and efficiently used to provenance analysis in East Asia (Cao et al., 2018; He et al., 2020; Zheng et al., 2020). Despite that, hydraulic sorting, mechanical abrasion, and weathering might bias the detrital zircon ages of source regions (Malusà et al., 2013). For geological time, it is hard to determine the erosion patterns of the paleo rivers, which also have a great influence on the age distributions yielded by source rocks (Wissink and Hoke, 2016).
FIGURE 2. Spectra of detrital zircon ages from the ECSB, Taiwan, and potential areas. The provenance package in R (Vermeesch et al., 2016) was used to make the KDE plots (bin = 20). See Table 1 for source of literature data. MLYR, middle and lower reaches of the Yangtze River trunk stream; TMYR, tributaries in the middle reaches of the Yangtze River.
The qualitative and quantitative statistical provenance analyses were both applied to compare the large detrital data set (Table 1). The R package (Vermeesch et al., 2016) and DZmix (Sundell and Saylor, 2017) were utilized for qualitative and quantitative analyses, respectively. Kernel density estimates (KDE) showed the probability distributions of samples (Vermeesch, 2013). Multidimensional scaling (MDS), which was applied to detrital zircon U-Pb age data sets to measure dissimilarities between samples, was used to highlight differences (Vermeesch, 2013; Vermeesch et al., 2016) and identify source areas which appear to be the most similar to Miocene sedimentary rocks in Taiwan and the ECSB. The DZmix can calculate the contributions of different source areas by Monte Carlo mixture modeling (Sundell and Saylor, 2017) and further assist to determine if the sediments in the ECSB and Taiwan were fed by the same sources.
The kernel density estimates (KDE) using a 20 Ma bandwidth show that a sharp provenance change occurred in Miocene in Taiwan region characterized by obvious increase in zircon age populations with peaks at ∼220 Ma, ∼800 Ma, ∼1800 Ma, and ∼2,500 Ma (Figure 2). This change in the age distribution after Oligocene was first reported by Lan et al. (2016). The similar age distribution also can be observed from the early–middle Miocene sedimentary rocks in the ECSB and present sediments from Coast Plain in Taiwan Island (Figure 2).
The MDS plot with a stress value of 0.056 suggests good quality of the fit (Figure 3). In the MDS plot (Figure 3), three clusters are clearly exhibited. The early–middle Miocene samples from Taiwan, the synchronous deposits in the ECSB, and present sands from Taiwan fall in the same cluster with Korea and North China affinity. The late Miocene–Quaternary samples from the ECSB links best with mainstream of the middle–lower Yangtze River, which is consistent with the view that the present-day Yangtze River initially was formed in the late Miocene (Fu et al., 2021). The Eocene samples from Taiwan are closely linked to the Min River, while the Oligocene samples links with the Qiantang River and the Late Miocene–Quaternary of the ECSB, despite of obviously different age distribution in KDE plots (Figure 2).
FIGURE 3. Non-metric multidimensional scaling (MDS, Vermeesch et al., 2016) plot of detrital zircon data. See Table 1 for source of literature data.
Three contradictory views that enlarged Min River (Lan et al., 2016), concealed microcontinent (Chen et al., 2019), and far inland sources by passing the ECSB (Deng et al., 2017; Zhang et al., 2017; Zhang J. et al., 2021) have been suggested to interpret the provenance change in Miocene. The age features of enlarged Min River and concealed microcontinent cannot be conclusively drawn though associated hypotheses were proposed. If the Miocene provenance change resulted from the sediments that were delivered by passing the ECSB, the contemporaneous deposits in the ECSB and the adjacent rivers in South China would represent the potential sources. According to this, Monte Carlo mixture modeling was constructed by DZmix using the cross-correlation coefficient for finite mixture distributions of probability density plots (Sundell and Saylor, 2017). The mixing model shows source contributions of 88% for the ECSB and 10% for the Min River (Figure 4A). Both contributions of the Jiulong River and Ou River are 1%, suggesting very limited contribution. For the present sediments in the coastal plain of Taiwan, which were believed to be mainly derived from the Miocene sedimentary rocks (Deng et al., 2017), and the mixing model with a mean cross-correlation coefficient of 0.89 and very low standard deviation demonstrates reliable evaluation (Figure 4B). Similarly, source contributions of 81% are from the ECSB, 8% from the Min River, 3% from the Jiulong River, and 7% from the Ou River. All these indicate that the early–middle Miocene sediments in Taiwan mainly were supplied by detrital materials from the ECSB and mixed with a small amount of sediments from the Min River, Jiulong River, and Ou River, and did not require an enlarged Min River or the microcontinent.
FIGURE 4. The mixing modelling showing contribution of source areas for early-middle Miocene sandstones and present sediments from Taiwan. The modelling was generated by the Monte Carlo model (Sundell and Saylor, 2017). The Jiulong River, Min River, Ou River and the early-middle Miocene sediments in ECSB were considered as the potential sources to observe the links between sediments in ECSB and the Taiwan. MLYR, middle and lower reaches of the Yangtze River trunk stream; TMYR, tributaries in the middle reaches of the Yangtze River.
To determine if the early–middle Miocene sediments in Taiwan region were associated with the Yangtze River, samples were taken from middle–lower mainstream of the Yangtze River, branches of the middle Yangtze River, North China, Korea, and other local rivers near Taiwan region to represent the features of the potential source (Figure 5A). Since ∼90% of sediments in mainstream of present middle and lower reaches of the Yangtze River were eroded from the eastern margin of the Tibetan Plateau (Wissink and Hoke, 2016), we compiled samples from the mainstream and branches to represent the Yangtze River and local contributions from middle reaches of the Yangtze River, respectively, to distinguish the contribution of the middle Yangtze River system before the establishment of the present-size Yangtze River. Monte Carlo mixing models of early–middle Miocene sedimentary rocks in Taiwan suggest that the source contributions of 33% were from the Yangtze River and 28% from North China (Figure 5A). Korea and branches of the lower Yangtze River contributed roughly the same sediments (14%). In contrary, present sediments in Taiwan were mainly contributed by North China (22%) and Korea (25%), minor by the Yangtze River (16%), and branches of the middle Yangtze River (12%) (Figure 5B).
FIGURE 5. The mixing modelling showing contribution of source areas for early-middle Miocene sandstones and present sediments from Taiwan, Miocene- Quaternary sandstone from ECSB. The modelling was generated by the Monte Carlo model (Sundell and Saylor, 2017). The fluvial samples in the source areas were compiled to quantitatively determine the ultimate contributions from different sources. Note that the Min River and Jiulong River are not used in the modelling for ECSB. MLYR, middle and lower reaches of the Yangtze River trunk stream; TMYR, tributaries in the middle reaches of the Yangtze River.
For the early–middle Miocene sediments in the ECSB, they were predominantly derived from the North China (40%) and Korea (30%) and the contributions of the Yangtze River system were limited: mainstream (15%) and branches in middle reaches (7%) (Figure 5C). However, for the late Miocene–Quaternary sediments in the ECSB, the Yangtze River contributed the most sediments that the contributions of mainstream were 52% and the branches in middle reaches were 20%, while Korea and North China only contributed a total of 13% sediments (Figure 5D). The cross-correlation coefficient (R2) of modeling for the ECSB sediments was over 0.9, indicating a high level of confidence.
Previous studies suggested that the Miocene provenance changes are closely linked with the Yangtze River system (Zhang et al., 2017; Zhang J. et al., 2021). However, most recent studies suggested that the Yangtze River might not have been established until the late Miocene (12 Ma -10 Ma) (Fu et al., 2021; Sun et al., 2021; Zhang Z. et al., 2021). The ECSB would be one of the most possible places to preserve the crucial evidence for evolution of the Yangtze River (Richardson et al., 2010; Fu et al., 2021). Our MDS plot (Figure 3) clearly shows that the late Miocene–Quaternary sediments in the ECSB were closely linked with the present Yangtze River systems, but the early–middle Miocene sediments in the ECSB and Taiwan region fall into the same cluster with North China and Korea, which was consistent with the most recent view that the Yangtze River was initially formed in the late Miocene (Zhang Z. et al., 2021; Fu et al., 2021; Guo et al., 2021). The modeling also suggested the change in provenance from 70% sediments derived from North China and Korea in the early–middle Miocene to 72% sediments sourced from the Yangtze River system since the late Miocene. Contrasting to our results, Zhang et al. (2021) proposed that about 50% sediments fed by the Yangtze River system in the early Miocene for ECSB sediments. As we have compiled much larger data (Table 1; Figure 5) and obtained mixing models with higher mean cross-correlation coefficient of 0.92 for the early–middle Miocene samples and 0.94 for late Miocene–Quaternary samples than those (0.843) models (Figure 5), our results are more reliable. The contrasting contributions of sediments for the Yangtze River system before and after the late Miocene support the view that the Yangtze River with present-day erosion patterns and similar size of catchment established in the late Miocene, as proposed by Fu et al. (2021).
The provenance of the Miocene sediments in the western foothills of the Taiwan region has been a hot topic of debate, despite a large amount of data obtained (Deng et al., 2017; Lan et al., 2016; Zhang et al., 2017; Chen et al., 2019). The provenance transition from ∼31 to 25 Ma has been inferred from elaborate interpretations of major elements, trace elements, rare elements, and Nd isotopes (Lan et al., 2014). The Miocene sediments show a much higher proportion of old zircons and monazite (∼1850 Ma) (Figure 2) and lower Nd isotope values (Lan et al., 2014; Lan et al., 2016; Chen et al., 2019). It is widely accepted that these sediments were not largely supplied by adjacent small local rivers that derived from the Cathaysia Block (Lan et al., 2016; Deng et al., 2017; Zhang et al., 2017; Chen et al., 2019). However, Chen et al. (2019) claimed that the sediments forming the Miocene strata were from a microcontinent that has been concealed under western Taiwan by overlying strata. Another competitive view is that these sediments were supplied by a large distal river derived from Eurasia, despite the transport routes being a hot debate. Lan et al. (2016) proposed that an enlarged paleo-Min River reached the lower Yangtze craton, resulting in provenance changes. Zhang et al. (2017) suggested that the Yangtze River supplied most of the sediments. Additionally, North China might also have contributed terrigenous clastics (Deng et al., 2017). Wang et al. (2018) noticed similarities in zircon age distribution between Oligocene sediments from the Xihu Sag and Miocene sediments from the Taiwan region. They proposed a mixed model of the Yangtze River and reworked sediment of pre-Miocene sediments of the ECSB.
Several pieces of evidence make the microcontinent model seem impossible. First, in their research, the Yangtze River and rivers derived from the Cathaysia Block were regarded as potential source areas without considering the possibility of North China and Korea, which contributed the most zircons according to our quantitative mixing models (Figures 4, 5); second, seismic sections across the region containing the proposed microcontinental block have revealed that the pre-rift basement is covered by Eocene and Miocene marine strata (Lin et al., 2003). This scenario indicates that even if this “concealed block” existed, it could not have provided a large amount of detritus. Furthermore, it cannot explain why the Eocene sediments in Taiwan are predominantly from nearby South China (Lan et al., 2014; Lan et al., 2016) in the rifting stage (Lin et al., 2003) and why the Miocene sediments sourced from local uplifts concealed the microcontinent as they suggested especially during passive margin subsidence (Huang et al., 2012).
The samples from the Miocene sequences in the western foothills of Taiwan exhibit detrital zircon features similar to the contemporaneous sediments in the ECSB in the KDE plots (Figure 2). The MDS plot also suggests that the Taiwan sediment has a close affinity with that in the ECSB (Figure 3). The mixing modeling clearly shows that approximate 80–90% of zircons were contributed by ECSB sediments (Figure 4). And the 70% of zircons in ECSB sediments were derived from North China and Korea (Figure 5C). Thus, we suggest that the Miocene sediments of western foothills in Taiwan have nearly the same sources with the ECSB and were mainly derived from North China and Korea.
The cross-correlation coefficient is 0.6 for early–middle Miocene sedimentary rocks and 0.89 for present sediments which are mainly derived from these rocks. All the model trails of finite mixture distributions yield a high peak at ∼1.8 Ga with much narrower age range than the counterpart of mixed samples from Miocene sediments in Taiwan but show identical age range with the present sediments from Taiwan and Miocene sedimentary rocks from the ECSB (Figures 4, 5). We noticed that the zircon ages of early–middle Miocene sediments reported by Lan et al. (2016) show obvious variations between samples and the grains for dating per sample between 75 and 91 are also significantly less than the suggested number (120 grains per sample) for provenance analysis proposed by Vermeesch (2004). Therefore, it would produce much better fits for MDS and mixing modelling if high quality of detrital zircon age data can be obtained from the Miocene sedimentary rocks in Taiwan.
Notably, the routes for sediments delivered from the ECSB to paleo-Taiwan region are under debate. Deng et al. (2017) suggested that a longshore current played an important role in sediment transport from shallow marine environments to the east of the present Yangtze Delta to the Taiwan region. Xu et al. (2007) argued that the transport ability of a longshore current is limited. Zhang et al. (2017) preferred river transport along the ECSB.
The depositional environment from northern ECSB to Paleao-Taiwan region suggests southward tilting, which was consistent with southward delivering of the detrital material. The ECSB formed a wide continental shelf (Lee et al., 2006). In the northern ECSB, the strata of early–middle Miocene dominantly consists of sandstone and mudstone conglomerate interbedded by coal, conglomerate, and freshwater limestone, indicating fluvial to lacustrine environments (Kwon and Boggs, 2002). For the middle ECSB, the lithology predominantly composed of fluvial coarse sandstone and red glutenite mudstone with interbred of coal (Fu et al., 2021).
In the southern ECSB, the Miocene strata consist of alternating lacustrine–flood plain and shallow marine sediments, showing frequent fluctuation of the sea level (Yu and Chow, 1997). The sea water deepened to the east of the southern ECSB. In the southern Ryukyu Islands, the Iriomote Island (Figure 1A) is mostly covered by the lower Miocene Iriomote Formation, which was subdivided into A-G member from lower to upper (Saitoh and Masuda, 2004). Iriomote Formation was mainly deposited in a shallow marine setting, but member F containing fossilized roots and coal indicate the terrestrial environment. The well-sorted and roundness sandstone grains combining with framework modes of sandstones that enriched in quartz demonstrate that the siliciclastics were derived from Eurasian continent (Saitoh and Masuda, 2004). In the Taiwan region, the passive continental sediments of early–middle Miocene with well-developed marine microfossils were mainly formed in shallow marine setting (Huang et al., 2012).
Furthermore, back-arc rifting in the northern Okinawa Trough initiated in the middle Miocene and has developed widely since the late Miocene (Letouzey and Kimura, 1985; Letouzey and Kimura, 1986), which was based on observations of the acoustic basement in seismic sections (Gungor et al., 2012). Before that, this region was possibly a zone of uplift, as inferred from the absence of pre-Miocene sediments (Shang et al., 2017), which blocked the sediments of the ECSB to be delivered eastward (Figure 6). Although previous studies did not report the existence of a large Miocene delta, we infer there might be a delta somewhere in the southern ECSB between the river system and the shallow marine sea (Figure 6). The widespread coal and high frequency sea level fluctuations in the southern ECSB (Yu and Chow, 1997; Saitoh and Masuda, 2004) might imply a Miocene delta system. Therefore, from the northern ECSB to the paleo-Taiwan region, a complete sediment delivering system from the river and delta to shallow marine can be suggested (Figure 6). If that was the case, southward delivery by fluvial processes played a major role in the ECSB. The longshore current, wave, and tidal current cannot be neglected, since the Miocene Taiwan region was largely controlled by marine setting (Huang et al., 2012), and the sediments need to be delivered from the delta to the shallow marine.
FIGURE 6. Schematic map showing the sediments delivered from the source areas to the paleo-Taiwan region. PTW, paleo-Taiwan.
Moreover, the early–middle Miocene Paleogeographic characteristics in East Asia might be different from the present, which can be inferred from the predominantly terrestrial deposits in region of the South Yellow Sea (Song et al., 2020). Thus, the river systems which are entirely different from the present might play an important role in the sedimentary transporting system from the North China and Korean Peninsula to the ECSB, but further research is required to trace the detailed routes.
In this study, quantitative and qualitative analyses were applied to determine the provenance of early–middle Miocene sediments in the ECSB and Taiwan region based on a large number of detrital zircon age data, together with regional trend in the sedimentary environment. We have drawn the following conclusions:
1) Early–middle Miocene sediments in the ECSB and Taiwan show close affinity with North China and Korea, and the contribution of 70% was from the two source areas for the ECSB.
2) Over 80% sediments in Taiwan were supplied from the ECSB, possibly by the river in the middle and northern ECSB, and by longshore current, wave, and tidal current from the southern ECSB to paleo-Taiwan region.
3) The contribution of the Yangtze River system was 72% for late Miocene–Quaternary sediments in the ECSB, which was consistent with the late Miocene establishment of the Yangtze River.
The original contributions presented in the study are included in the article/Supplementary Material, further inquiries can be directed to the corresponding author.
XF processed the data processing, wrote the manuscript, and provided funding and helped with conception of idea. LH compiled the data, drew plots and edited the manuscript. WZ contributed to the conception, reviewed and edited the manuscript. XH contributed to data processing and participated in discussion. KF and ZZ reviewed and edited the manuscript.
We declare that this work was supported by Natural Science Foundation of Shanghai (22ZR1463300) and National Natural Science Foundation of China (41702228).
The authors declare that the research was conducted in the absence of any commercial or financial relationships that could be construed as a potential conflict of interest.
All claims expressed in this article are solely those of the authors and do not necessarily represent those of their affiliated organizations, or those of the publisher, the editors, and the reviewers. Any product that may be evaluated in this article, or claim that may be made by its manufacturer, is not guaranteed or endorsed by the publisher.
Cao, L., Shao, L., Qiao, P., Zhao, Z., and van Hinsbergen, D. J. J. (2018). Early Miocene Birth of Modern Pearl River Recorded Low-Relief, High-Elevation Surface Formation of SE Tibetan Plateau. Earth Planet. Sci. Lett. 496, 120–131. doi:10.1016/j.epsl.2018.05.039
Chen, C.-H., Lee, C.-Y., Lin, J.-W., and Chu, M.-F. (2019). Provenance of Sediments in Western Foothills and Hsuehshan Range (Taiwan): A New View Based on the EMP Monazite versus LA-ICPMS Zircon Geochronology of Detrital Grains. Earth-Science Rev. 190, 224–246. doi:10.1016/j.earscirev.2018.12.015
Choi, T., Lee, Y. I., and Orihashi, Y. (2016). Crustal Growth History of the Korean Peninsula: Constraints from Detrital Zircon Ages in Modern River Sediments. Geosci. Front. 7, 707–714. doi:10.1016/j.gsf.2016.05.003
Clift, P. D. (2006). Controls on the Erosion of Cenozoic Asia and the Flux of Clastic Sediment to the Ocean. Earth Planet. Sci. Lett. 241, 571–580. doi:10.1016/j.epsl.2005.11.028
Deng, K., Yang, S., Li, C., Su, N., Bi, L., Chang, Y.-P., et al. (2017). Detrital Zircon Geochronology of River Sands from Taiwan: Implications for Sedimentary Provenance of Taiwan and its Source Link with the east China mainland. Earth-Science Rev. 164, 31–47. doi:10.1016/j.earscirev.2016.10.015
Fu, X., Zhu, W., Geng, J., Yang, S., Zhong, K., Huang, X., et al. (2021). The Present-Day Yangtze River Was Established in the Late Miocene: Evidence from Detrital Zircon Ages. J. Asian Earth Sci. 205, 104600. doi:10.1016/j.jseaes.2020.104600
Gungor, A., Lee, G. H., Kim, H.-J., Han, H.-C., Kang, M.-H., Kim, J., et al. (2012). Structural Characteristics of the Northern Okinawa Trough and Adjacent Areas from Regional Seismic Reflection Data: Geologic and Tectonic Implications. Tectonophysics 522-523, 198–207. doi:10.1016/j.tecto.2011.11.027
Guo, R., Sun, X., Li, C. a., Li, Y., Wei, C., Zhang, Z., et al. (2021). Cenozoic Evolution of the Yangtze River: Constraints from Detrital Zircon U Pb Ages. Palaeogeogr. Palaeoclimatol. Palaeoecol. 579, 110586. doi:10.1016/j.palaeo.2021.110586
He, J., Garzanti, E., Cao, L., and Wang, H. (2020). The Zircon story of the Pearl River (China) from Cretaceous to Present. Earth-Science Rev. 201, 103078. doi:10.1016/j.earscirev.2019.103078
He, M., Zheng, H., Bookhagen, B., and Clift, P. D. (2014). Controls on Erosion Intensity in the Yangtze River basin Tracked by U-Pb Detrital Zircon Dating. Earth-Science Rev. 136, 121–140. doi:10.1016/j.earscirev.2014.05.014
Huang, C.-Y., Yen, Y., Zhao, Q., and Lin, C.-T. (2012). Cenozoic Stratigraphy of Taiwan: Window into Rifting, Stratigraphy and Paleoceanography of South China Sea. Chin. Sci. Bull. 57, 3130–3149. doi:10.1007/s11434-012-5349-y
Huang, C. Y. (2017). Geological Ages of Taiwan Stratigraphy and Tectonic Events (In Chinese). Sci. Sin.-Terrae 47, 394–405. doi:10.1360/N072017-00023
Iizuka, T., Komiya, T., Rino, S., Maruyama, S., and Hirata, T. (2010). Detrital Zircon Evidence for Hf Isotopic Evolution of Granitoid Crust and continental Growth. Geochimica et Cosmochimica Acta 74, 2450–2472. doi:10.1016/j.gca.2010.01.023
Kwon, Y.-I., and Boggs, S. (2002). Provenance Interpretation of Tertiary Sandstones from the Cheju Basin (NE East China Sea): a Comparison of Conventional Petrographic and Scanning Cathodoluminescence Techniques. Sediment. Geology. 152, 29–43. doi:10.1016/S0037-0738(01)00284-6
Lan, Q., Yan, Y., Huang, C.-Y., Clift, P. D., Li, X., Chen, W., et al. (2014). Tectonics, Topography, and River System Transition in East Tibet: Insights from the Sedimentary Record in Taiwan. Geochem. Geophys. Geosyst. 15, 3658–3674. doi:10.1002/2014GC005310
Lan, Q., Yan, Y., Huang, C.-Y., Santosh, M., Shan, Y.-H., Chen, W., et al. (2016). Topographic Architecture and Drainage Reorganization in Southeast China: Zircon U-Pb Chronology and Hf Isotope Evidence from Taiwan. Gondwana Res. 36, 376–389. doi:10.1016/j.gr.2015.07.008
Lee, G. H., Kim, B., Shin, K. S., and Sunwoo, D. (2006). Geologic Evolution and Aspects of the Petroleum Geology of the Northern East China Sea Shelf basin. Bulletin 90, 237–260. doi:10.1306/08010505020
Letouzey, J., and Kimura, M. (1985). Okinawa Trough Genesis: Structure and Evolution of a Backarc basin Developed in a Continent. Mar. Pet. Geology. 2, 111–130. doi:10.1016/0264-8172(85)90002-9
Letouzey, J., and Kimura, M. (1986). The Okinawa Trough: Genesis of a Back-Arc basin Developing along a continental Margin. Tectonophysics 125, 209–230. doi:10.1016/0040-1951(86)90015-6
Lin, A. T., Watts, A. B., and Hesselbo, S. P. (2003). Cenozoic Stratigraphy and Subsidence History of the South China Sea Margin in the Taiwan Region. Basin Res. 15, 453–478. doi:10.1046/j.1365-2117.2003.00215.x
Malusà, M. G., Carter, A., Limoncelli, M., Villa, I. M., and Garzanti, E. (2013). Bias in Detrital Zircon Geochronology and Thermochronometry. Chem. Geology. 359, 90–107. doi:10.1016/j.chemgeo.2013.09.016
Nie, J., Stevens, T., Rittner, M., Stockli, D., Garzanti, E., Limonta, M., et al. (2015). Loess Plateau Storage of Northeastern Tibetan Plateau-Derived Yellow River Sediment. Nat. Commun. 6, 27. doi:10.1038/ncomms9511
Ren, J., Tamaki, K., Li, S., and Junxia, Z. (2002). Late Mesozoic and Cenozoic Rifting and its Dynamic Setting in Eastern China and Adjacent Areas. Tectonophysics 344, 175–205. doi:10.1016/s0040-1951(01)00271-2
Richardson, N. J., Densmore, A. L., Seward, D., Wipf, M., and Yong, L. (2010). Did Incision of the Three Gorges Begin in the Eocene? Geology 38, 551–554. doi:10.1130/G30527.1
Saitoh, Y., and Masuda, F. (2004). Miocene sandstone of 'continental' Origin on Iriomote Island, Southwest Ryukyu Arc, Eastern Asia. J. Asian Earth Sci. 24, 137–144. doi:10.1016/j.jseaes.2003.10.002
Shang, L.-N., Zhang, X.-H., Jia, Y.-G., Han, B., Yang, C.-S., Geng, W., et al. (2017). Late Cenozoic Evolution of the East China continental Margin: Insights from Seismic, Gravity, and Magnetic Analyses. Tectonophysics 698, 1–15. doi:10.1016/j.tecto.2017.01.003
Song, Y., Hu, S., Xu, J., Shen, C., Li, S., Su, P., et al. (2020). Lacustrine Environmental Evolution and Implications on Source Rock Deposition in the Upper Cretaceous-Paleocene of the South Yellow Sea Basin, Offshore Eastern China. Mar. Pet. Geology. 113, 104135. doi:10.1016/j.marpetgeo.2019.104135
Sun, X., Tian, Y., Kuiper, K. F., Li, C. a., Zhang, Z., and Wijbrans, J. R. (2021). No Yangtze River Prior to the Late Miocene: Evidence from Detrital Muscovite and K-Feldspar 40Ar/39Ar Geochronology. Geophys. Res. Lett. 48, e2020GL089903. doi:10.1029/2020GL089903
Sundell, K. E., and Saylor, J. E. (2017). Unmixing Detrital Geochronology Age Distributions. Geochem. Geophys. Geosyst. 18, 2872–2886. doi:10.1002/2016GC006774
Vermeesch, P. (2004). How many Grains Are Needed for a Provenance Study? Earth Planet. Sci. Lett. 224, 441–451. doi:10.1016/j.epsl.2004.05.037
Vermeesch, P. (2013). Multi-sample Comparison of Detrital Age Distributions. Chem. Geology. 341, 140–146. doi:10.1016/j.chemgeo.2013.01.010
Vermeesch, P., Resentini, A., and Garzanti, E. (2016). An R Package for Statistical Provenance Analysis. Sediment. Geology. 336, 14–25. doi:10.1016/j.sedgeo.2016.01.009
Wang, W., Bidgoli, T., Yang, X., and Ye, J. (2018). Source-To-Sink Links Between East Asia and Taiwan From Detrital Zircon Geochronology of the Oligocene Huagang Formation in the East China Sea Shelf Basin. Geochemistry. Geochem. Geophys. Geosyst. 19, 3673–3688. doi:10.1029/2018GC007576
Wang, P. (2004). Cenozoic Deformation and the History of Sea-Land Interactions in Asia. Geophys. Monogr. Ser. 1, 1–22. doi:10.1029/149GM01
Weilin, Z., Kai, Z., Xiaowei, F., Chunfeng, C., Minqiang, Z., and Shunli, G. (2019). The Formation and Evolution of the East China Sea Shelf Basin: A New View. Earth-Science Rev. 190, 89–111. doi:10.1016/j.earscirev.2018.12.009
Wissink, G. K., and Hoke, G. D. (2016). Eastern Margin of Tibet Supplies Most Sediment to the Yangtze River. Lithosphere 8, 601–614. doi:10.1130/L570.1
Wu, F., Yang, J., Wilde, S., Liu, X., Guo, J., and Zhai, M. (2007). Detrital Zircon U-Pb and Hf Isotopic Constraints on the Crustal Evolution of North Korea. Precambrian Res. 159, 155–177. doi:10.1016/j.precamres.2007.06.007
Xu, X., O’Reilly, S. Y., Griffin, W. L., Wang, X., Pearson, N. J., and He, Z. (2007). The Crust of Cathaysia: Age, Assembly and Reworking of Two Terranes. Precambrian Res. 158, 51–78. doi:10.1016/j.precamres.2007.04.010
Xu, Y., Wang, C. Y., and Zhao, T. (2016). Using Detrital Zircons from River Sands to Constrain Major Tectono-thermal Events of the Cathaysia Block, SE China. J. Asian Earth Sci. 124, 1–13. doi:10.1016/j.jseaes.2016.04.012
Yang, J., Gao, S., Chen, C., Tang, Y., Yuan, H., Gong, H., et al. (2009). Episodic Crustal Growth of North China as Revealed by U-Pb Age and Hf Isotopes of Detrital Zircons from Modern Rivers. Geochimica et Cosmochimica Acta 73, 2660–2673. doi:10.1016/j.gca.2009.02.007
Yang, S., Zhang, F., and Wang, Z. (2012). Grain Size Distribution and Age Population of Detrital Zircons from the Changjiang (Yangtze) River System, China. Chem. Geology. 296-297, 26–38. doi:10.1016/j.chemgeo.2011.12.016
Yu, H.-S., and Chow, J. (1997). Cenozoic Basins in Northern Taiwan and Tectonic Implications for the Development of the Eastern Asian continental Margin. Palaeogeogr. Palaeoclimatol. Palaeoecol. 131, 133–144. doi:10.1016/S0031-0182(96)00124-1
Zhang, J., Krijgsman, W., Lu, Y., Liu, J., Li, X., Du, X., et al. (2021a). Detrital Zircon Ages Reveal Yangtze Provenance since the Early Oligocene in the East China Sea Shelf Basin. Palaeogeogr. Palaeoclimatol. Palaeoecol. 577, 110548. doi:10.1016/j.palaeo.2021.110548
Zhang, X., Dalrymple, R. W., Yang, S.-Y., Lin, C.-M., and Wang, P. (2015). Provenance of Holocene Sediments in the Outer Part of the Paleo-Qiantang River Estuary, China. Mar. Geology. 366, 1–15. doi:10.1016/j.margeo.2015.04.008
Zhang, X., Huang, C., Wang, Y., Clift, P. D., Yan, Y., Fu, X., et al. (2017). Evolving Yangtze River Reconstructed by Detrital Zircon U-Pb Dating and Petrographic Analysis of Miocene Marginal Sea Sedimentary Rocks of the Western Foothills and Hengchun Peninsula, Taiwan. Tectonics 36, 634–651. doi:10.1002/2016TC004357
Zhang, Z., Daly, J. S., Li, C. a., Tyrrell, S., Sun, X., Badenszki, E., et al. (2021b). Formation of the Three Gorges (Yangtze River) No Earlier Than 10 Ma. Earth-Science Rev. 216, 103601. doi:10.1016/j.earscirev.2021.103601
Zheng, H. (2015). Birth of the Yangtze River: Age and Tectonic-Geomorphic Implications. Natl. Sci. Rev. 2, 438–453. doi:10.1093/nsr/nwv063
Keywords: Taiwan region, East China Sea Basin, detrital zircon age, Yangtze River (Changjiang River), Miocene provenance changes
Citation: Fu X, Hu L, Zhu W, Huang X, Feng K and Zhou Z (2022) Miocene Provenance Changes in Taiwan Caused by Southward Input of Sediments From East China Sea Basin. Front. Earth Sci. 10:849181. doi: 10.3389/feart.2022.849181
Received: 05 January 2022; Accepted: 04 February 2022;
Published: 10 March 2022.
Edited by:
Xuhua Shi, Zhejiang University, ChinaReviewed by:
Rong Yang, Zhejiang University, ChinaCopyright © 2022 Fu, Hu, Zhu, Huang, Feng and Zhou. This is an open-access article distributed under the terms of the Creative Commons Attribution License (CC BY). The use, distribution or reproduction in other forums is permitted, provided the original author(s) and the copyright owner(s) are credited and that the original publication in this journal is cited, in accordance with accepted academic practice. No use, distribution or reproduction is permitted which does not comply with these terms.
*Correspondence: Weilin Zhu, emh1d2xAdG9uZ2ppLmVkdS5jbg==
Disclaimer: All claims expressed in this article are solely those of the authors and do not necessarily represent those of their affiliated organizations, or those of the publisher, the editors and the reviewers. Any product that may be evaluated in this article or claim that may be made by its manufacturer is not guaranteed or endorsed by the publisher.
Research integrity at Frontiers
Learn more about the work of our research integrity team to safeguard the quality of each article we publish.