Corrigendum: Variability of Sea Breezes Over the Cameroonian Coast and Their Interaction With the West African Monsoon
- 1Brazilian Research Network on Global Climate Change—Rede CLIMA, São José dos Campos, Brazil
- 2International Chair in Mathematical Physics and Applications (ICMPA–UNESCO Chair), Cotonou, Benin
- 3Association of Professionals in Coastal and Aquatics Management—APCAM, Yaoundé, Cameroon
- 4Department of Oceanography, Institute of Fisheries and Aquatic Sciences, University of Douala, Douala, Cameroon
- 5Departamento de Oceanografia, Universidade Federal de Pernambuco—DOCEAN/UFPE, Recife, Brazil
The sea breeze characteristics of over two coastal areas of Cameroon were studied. The study used 3-hourly wind (speed and direction), rainfall, and atmospheric pressure data of 4 years (2006–2009) at Tiko and 5 years (2011–2015) at Ebodje to examine various characteristics of sea breezes. Statistics are presented that describe the occurrence, onset and cessation, and strength of the sea breezes. The frequency of occurrence clearly shows two regimes by two maxima (November and March) at Tiko and (January and July) at Ebodje. The results show that a sea breeze usually starts few hours (about 3–4 h) after the sunrise as a light onshore surface wind, has a mean duration of about 9 h at Tiko, and occurs during all seasons at both coastal areas. The sea breeze occurrence is found to be strongly influenced by the West African monsoon winds, and its onset and cessation times show a pronounced seasonal variation. The sea breeze strength is greater at Tiko than that at Ebodje during the West African monsoon season. Average daily hodographs for Tiko reveals that the sea breeze circulation is in both clockwise and anticlockwise rotation. There would be a significant seasonal effect of large-scale flows on sea breeze hodograph patterns. A wind roses analysis at Ebodje shows a high temporal variability of sea breeze frequencies at 09:00 (Local Standard Time: LST) in the south direction (36%), then at 15:00 LST in the west direction (26%), because of the preponderance of calm atmospheric situations conducive to its onset. Therefore, the circulation of the sea breeze is influenced by local factors (topography and vegetation). This phenomenon in the coastal regions of Cameroon can be the main factor controlling the transport of accidental near shore oil slicks and air pollutants.
Introduction
A sea breeze is a mesoscale meteorological phenomenon based on the thermal contrasts between the land and the sea. It develops along coastal regions when the temperature difference between two adjacent surfaces is large enough and the geostrophic wind is weak. It determines weather and air quality conditions and affects the living conditions of humans in many ways in coastal regions. Numerous studies have been reported on land and sea breezes in respect of air quality through pollutant transport and dispersion (Lu and Turco, 1994), initiation of convective thunderstorms (Simpson, 1995), forecasting of forest fires (Rao and Fuelberg, 2000), and aviation safety (Cooper et al., 1998). Climatological studies of sea breezes in several coastal regions of the world, for instance, over tLower Fraser Valley, B.C. (Steyn and Faulkner, 1986), West Coast of Sweden (Gustavsson et al., 1995; Borne et al., 1998), St. Johns Newfoundland (Banfield, 1991), Northern Adriatic (Maja, 2003), Arabian Gulf region (Eager et al., 2008), Sardinia (Furberg et al., 2002), and Alicante (Azorin-Molina et al., 2009), among others, have shown that sea breezes can induce thunderstorms, bring moisture to clouds, soften oppressive hot weather, and lead to improved or degraded air quality in the coastal area.
Research studies of Frizzola and Fisher (1963), Wexler (1946), Pielke (1974), Bechtold et al. (1991) and Bajamgnigni and Steyn (2012) indicated the influence of prevailing large-scale flow on the structure and evolution of sea breezes associated with the direction (offshore, parallel, and onshore) and strength of a synoptic flow. Pielke (1974), Atkinson (1981), Maja (2003), and Miao et al. (2003) indicated that sea breezes occur most often in spring and summer because of the existence of a temperature gradient between the land and the sea. In their study over Sardinia (middle-latitude), Furberg et al. (2002) observed that sea breezes are more frequent between May and August, and Michael (2003) showed that sea breezes appear most often from spring to autumn. Over the Lower Frazer Valley on the Canadian coast (higher latitude), Steyn and Faulkner (1986) pointed out that maximum number of days of sea breeze were observed in August and minimum in December/January. This shows that sea breezes occur mainly during the summer at middle and high latitudes. However, as observed by Wexler (1946), sea breezes tend to be stronger characterized by high temperatures in the tropics (low latitude) than that in the middle and high latitudes.
In the West and Central Africa, weather and climate are dominated by two major wind systems, the West African southwest monsoon (WAM) and northeasterly trade winds (Harmattan). As a regional (low latitudes) and seasonal climatic phenomenon (about March to September), WAM is forced by a southern gradient of dry and wet static energy between the continent and the Atlantic Ocean (Eltahir and Gong, 1996). Numerous studies on tropical sea breezes (low latitude), in particular along the Guinean coast of West Africa, revealed the influence of WAM on the occurrence of sea and land breezes. For instance, Simpson (1994) showed that tropical sea breezes occur on at least two-thirds of the days of the non-monsoon season (winter). This shows that, in the tropics, the frequency of occurrence of a sea breeze is higher during the winter. Using satellite images and a three-dimensional mesoscale model, Cautenet and Rosset (1989) pointed out two different weather situations, characterized by two contrast wind profiles associated with two types of transitions between WAM and the upper East African jet. In their study, using multiyear meteorological data from several coastline stations, Abatan et al. (2007), Abatan et al. (2013), Bajamgnigni and Steyn (2012), Coulibaly et al. (2019), and Coulibaly et al. (2020) investigated some characteristics of sea and land breezes such as frequency of occurrence, time of onset and cessation, duration, and hodographs rotation over the south coast of Nigeria, the Bight of Benin Republic, and all along the coast of West Africa, respectively. Theses authors reported the existence of two regimes characterized by two peaks in the monthly distribution of sea breeze days, but this is not the case in middle and high latitude regions where the monthly change in sea breeze occurrence exhibits only one maximum (Steyn and Faulkner, 1986; Furberg et al., 2002). None of the aforementioned studies have yet been conducted along the Cameroonian coast.
Issues related to coastal air quality and sea and land breezes are of large interests, as the Atlantic coastline is very rich and characterized by increasing urbanization. The situation is even more accentuated in the industrialized coastal zone because of their situation as an interface between the hinterlands and offshore. This is the case of the Cameroonian coastal zone which opens to the Atlantic Ocean with a coastline of about 402 km (Sayer et al., 1992). This zone is characterized by a growing demographic attractiveness and tourism, and oil and gas activities are booming (MINEP, 2010), particularly in the coastal regions of Ebodje (southern zone) and Tiko (northern zone). The risk of an oil spill is real in these areas. An oil spill accident occurred in January 2007 off the Kribi–Campo region, forming an oil slick on the surface of the sea (Folack and Ngueguim, 2007). This type of disaster affects nursery areas (mangrove estuaries, sea turtle laying area, and seagrass beds), beaches, and recreational areas. Coastal metropolitan areas are often subject to not only the phenomenon of oil spills but also to the extreme events such as storms associated with thermal breezes, as the case of the strong winds that hit Kribi in January 2017, causing material damage and heavy loss of human lives (MEAO, 2017). Several studies have shown the presence of tar balls (indicator of oil pollution) on the beaches of Limbe (Ikome, 1985; Gabche et al., 1998), at the mouth of Lokoundjé, and the mouth of Sanaga (Folack and Ngassa, 1994). The variability of thermal breezes over the Cameroonian coast within the framework of Littoral Conservation and Management has rarely been studied. This is largely due to lack of relevant data. This study presents a multiyear observational study of sea breeze characteristics such as time of onset and cessation, frequency of occurrence, duration, hodographs, and wind roses analysis over the north and south coasts of Cameroon, in particular at Tiko and Ebodje. This objective has the following components:
• To identify sea breezes at Tiko and Ebodje using multiyear meteorological data from near-coastal anemometers, thermometers, and sea breeze detection filters.
• To perform a statistical climatology (frequency of occurrence, mean time of onset and cessation, mean duration, mean wind speed, direction at the time of onset, mean maximum velocity, and mean time of maximum velocity) of sea breezes at Tiko and Ebodje.
• To devise diurnal hodographs of sea breezes at Tiko and wind roses of sea breezes at Ebodje and interpret their diurnal evolution in relation to conservation and management of Cameroonian coastal areas.
Materials and Methods
In this study, the meteorological data utilized consist of a 4-year (2006–2009) wind data for the Tiko zone and a 5-year (2011–2015) wind data for the Ebodje zone. The wind data consist of direction, speed, daily total air temperature, precipitation, relative pressure, and humidity hours over land which are collected at the meteorological stations of Tiko and Ebodje, hosted at the Regional Delegation of Transport, Kribi, Cameroon (Figure 1). These stations have an unobstructed fetch in the near field and are not near any local topographic features. The wind speed and direction are of 3-hourly (Local Standard Time: LST) anemometer measurements (from 6:00 to 18:00 at Tiko; only at 9:00 and 15:00 LST at the Ebodje station) at a height of 10 m above ground level. The daily air temperature is measured at a height of 2 m above ground surface using a mercury thermometer, dry thermometer, and wet thermometer; daily air pressure is measured using a barometer, and rainfalls are measured using a rain gauge.
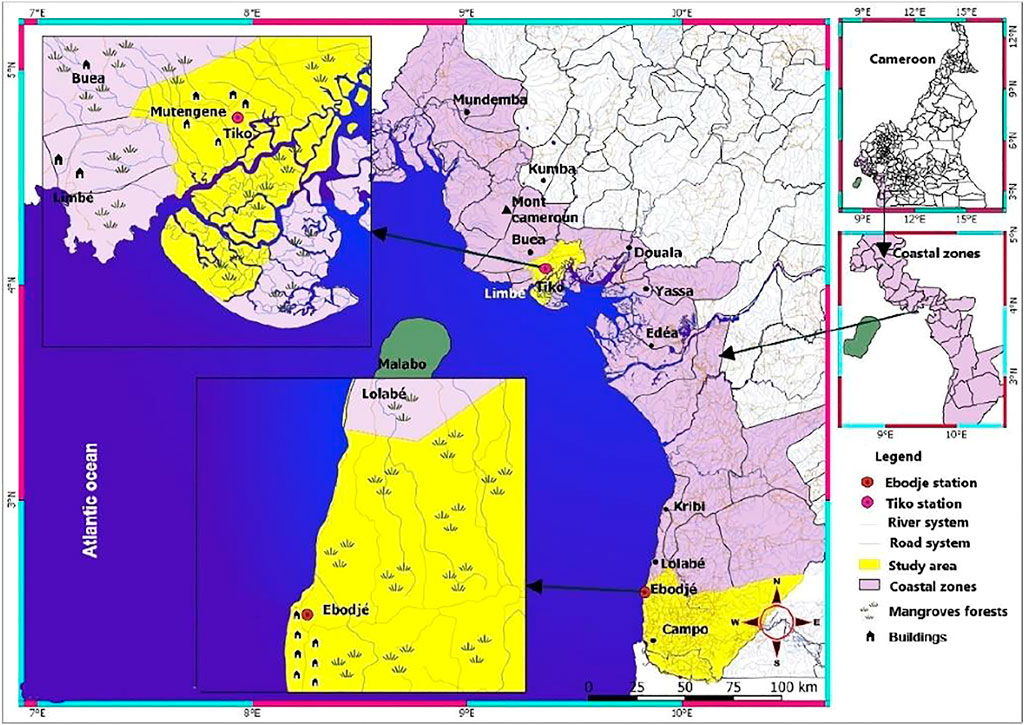
FIGURE 1. Study area showing coastal zones of Cameroon, with the enlarged localization maps of Tiko and Ebodje meteorological stations.
The two stations are considered appropriate for the study because of the availability of observational data for the period. The stations location indicators are given in Table 1. The large data sets were previously examined to exclude some days and months with incomplete and unreliable data. The reduced data sets were, therefore, used in the filters of sea breezes detection in order to extract the sea breeze days.
Our analysis is based first on a sea breeze detection filter, which is the wind reversal criterion developed by Abatan et al. (2007, 2013), Bajamgnigni and Steyn (2012), and Coulibaly et al. (2019). These studies show that the most important and most used strategy for sea breeze identification remains the wind reversal criterion. The thermal gradient criterion developed in their study was not used due to lack of relevant sufficient sea surface temperature data. The wind reversal criterion does not exclude days with sea breeze–like conditions caused by the passage of frontal systems. An attempt to remove such days was made by adding another filter, which is a sea breeze detection algorithm developed by Dudouit (2004) and Dahech (2012 and 2015). Therefore the applied filter is defined as follows:
Wind Reversal Criterion (Filter “a”)
This first filter ensures that the sea breeze has occurred, so whether a change in the wind direction of at least 90° from land to sea during the day associated with an increase in the wind speed of less than 2 m/s has occurred. For that
• most of the 3-hourly wind must be offshore or calm from (sunrise −7 h) to (sunrise +2 h);
• from (sunrise +2 h) to (sunset +2 h) at least two consecutive 3-hourly winds should be onshore.
Sea Breeze Detection Algorithm (Filter “b”)
This second filter follows the previous and exclude days with sea breeze–like conditions caused by the passage of frontal systems. For that, it
• rejects rainy days, corresponding to the days when the total daily rainfall is > 0 mm;
• removes all days with a low-pressure tendency for which the atmospheric pressure is < 1005 hPa at a time of the day;
• eliminates windy days for which the wind speed is too high in the morning for a sea breeze to rise, so when the minimum instantaneous wind speed is > 2 m/s and for which the maximum instantaneous wind speed observed in the middle of the day is > 5 m/s.
Finally, the extracted breeze days in Tiko and Ebodje areas are then validated with records of air total temperature and relative humidity data obtained in both areas.
Rejection statistics are displayed in Figure 2 in order to examine the comparative effect of sea breeze detection filters. The two filters are applied in order, first the filter “a” and then the filter “b”. Steyn and Faulkner (1986) pointed out that the criterion of wind reversal is the most important and the thermal gradient is less important. They characterized the filter “a” as a “phenomenological filter”. We consider the filter “b” as a “mechanistic filter”. A sensitivity analysis reveals that the number of sea breeze days is moderately sensitive to the onshore/offshore wind direction, precipitations, air pressure, temperature, and relative humidity. The set of filters does not consider upper air conditions but exclude days of strong overriding geostrophic winds. Because of the conservative nature of our set of filters, this method will most likely underestimate the sea breeze frequency. Nevertheless, the data subset that would have passed the two filters will provide a sound basis for constructing the sea breeze climatology.
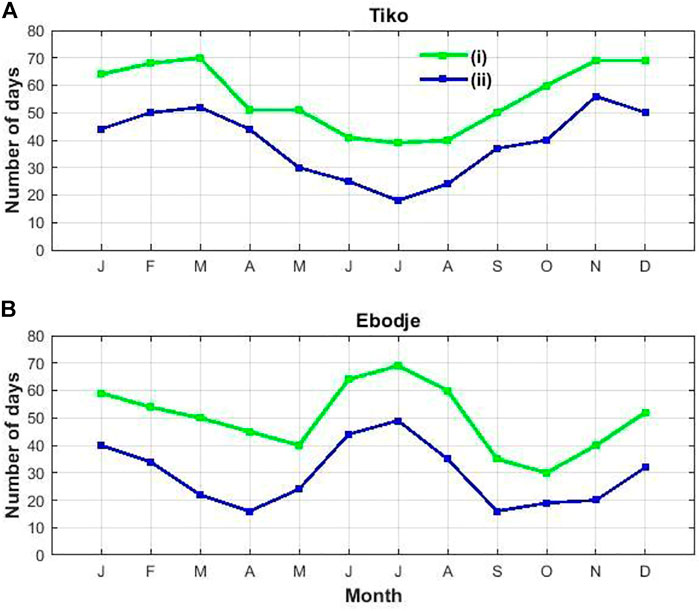
FIGURE 2. Rejection statistics by month for the twos filters of sea breeze detection at (A) Tiko and (B) Ebodje. (i) is the number of days in the whole sample passing the filter a. (ii) is those passing the filter a and b.
Results and Discussion
Characterization of Thermal Breezes in Tiko and Ebodje
Based on the sea breeze day extraction filters described in previous section on the data set, a total of 470 days of sea breeze were detected in Tiko and 351 days of sea breeze in Ebodje. The main parameters of the phenomenon have been determined, and the monthly statistics at Tiko and Ebodje on the frequency of occurrence, mean onset time (and standard deviation), mean cessation time (and standard deviation), and mean sea breeze strength (and standard deviation) are presented in Tables 2, 3, respectively. These statistics are plotted and discussed in the coming sections, in relation to WAM, local coastlines, topography, and conservation and management of Cameroonian coastal areas. The monthly statistics of sea breeze onset and cessation time are not presented in Table 3 due to insufficient data at Ebodje. First, we will show and discuss the results of Tiko plain (located on the northern coast) and then in the Ebodje area (located on the southern coast), comparing them.
One of the interesting characteristics of the sea breeze system is the frequency of occurrence. The mean monthly frequencies of occurrence of sea breeze is shown in Figure 3, which reveal that the sea breeze is a relatively common phenomenon throughout the year in the Tiko and Ebodje areas. This is consistent with the results observed in Abatan et al. (2007) and Abatan et al. (2013) in Nigeria, Bajamgnigni and Steyn (2012) in Benin sea breezes, and Coulibaly et al. (2019) on land and sea breezes along the Guinea Coast of West Africa. The figure shows how the frequency of occurrence varies with month, exhibiting two clear regimes. At the Tiko station, the regime with highest frequency from November to April (>40 days) is characterized by 02 maxima (November and March), and the one with lowest frequency from May to October (<40 days) is characterized by a minimum in July. The Ebodje station data exhibit highest frequencies regime from June to August and from December to February (>30 days), characterized by 02 maxima (January and July), and the lowest frequency regime from March to May and September to November (<30 days) is characterized by 02 minima (April and September). The bimonthly sea breeze variation regimes at Tiko and Ebodje (low latitude) are in contrast with the unique regime observed in the middle and high latitudes (Steyn and Faulkner, 1986; Furberg et al., 2002) and with the two regimes observed in low latitudes by Abatan et al. (2007, 2013), Bajamgnigni and Steyn (2012), and Coulibaly et al. (2019). This characteristic is presumed to be not only due to a seasonal effect and a large-scale synoptic flow but also the position and orientation of each station to the local coastline. Now, we may examine the factor that may be responsible for those features of sea breeze occurrence.
Sea Breeze Occurrence: Interaction With WAM
The coastal regions of Cameroon are crossed by the cool and moist trade winds (monsoon flux) from the St. Helena anticyclone. Its rainfall potential is therefore large, and the slightest obstacle to its path results in stubborn stormy rains on the Cameroonian coast (Tsalefac and Dagorne, 1990). The Tiko and Ebodje areas are characterized by a swamp forest, consisting of fresh water and a mangrove swamp forest, both of which are less influenced by the double rainfall pattern. This makes the environment a wetland region and will consequently affect their land/sea temperature contrasts, which in turn leads to the low frequency of sea breeze occurrences. The plot of the mean monthly rainfall for the period 2006–2009 revealed that Tiko recorded two distinct seasons: a large rainy season of 7 months from April to October (>200 mm) when the cool and moist southwesterly monsoon winds blow from St. Helena and a small dry season of 5 months from November to March (<200 mm) (Figure 4) when the hot and dry northeasterly winds blow from the Sahara Desert; while the resort of Ebodje–Campo, during the period 2011–2015, recorded four distinct seasons: a large dry season from December to February (<200 mm), a small rainy season from March to May (>200 mm), a small dry season from June to August (<200 mm), and a large rainy season from September to November (>200 mm). This is in accordance with Olivry (1986) analyses of the climate patterns in the Cameroonian coastal zone. There is a direct correspondence of seasonality between monthly occurrence of sea breeze days and the synoptic flow of monsoon in central Africa. The regime with highest frequency can be associated with the withdrawal of WAM winds (non-monsoon) during the dry season, resulting in the weakening of intertropical discontinuity (ITD), which is considered the main one on a large scale. This ITD marks the monsoon shear line (Sadler, 1975), which is the front zone between the monsoon and harmattan flows (Frizzola and Fisher, 1963; Bechtold et al., 1991). During the dry period, ITD will be close to the coast, followed by a strong day time heating of the hinterland. The minimum of sea breezes during the low-frequency period (in July at Tiko and April/October at Ebodje) can be associated with the presence of large-scale prevailing winds in this period, which will weaken the sea breeze. Along the Cameroonian coastal zone, the most important synoptic flow is WAM winds, considered as the main driver of coastal circulation (Mounier et al., 2007), which is most intense during the period of mid-May to mid-September (Sultan and Janicot, 2003; Fontaine et al., 2008). First, in order to analyze the connection between the sea breeze occurrences and monsoonal rainfall, a simple linear regression line was constructed between sea breezes and rainfall, according to the regression equations, as shown in Figure 5. Point-polyserial correlation tests performed between sea breezes and rainfall indicate a significant correlation at threshold α = 5%. The two parameters are therefore strongly correlated, with an anticorrelation of a value r = −0.95 (p < 0.05) to Tiko and r = −0.88 (p < 0.05) to Ebodje. The results indicate that 86% of the variation in sea breezes is explained by monsoonal rainfall (r2 = 0.8609) in Tiko and 67% is explained by monsoonal rainfall (r2 = 0.6779) in Ebodje, confirming that the occurrence of sea breezes is related to WAM. A strong correlation (r = −0.96) between the meridional monsoon winds and the number of sea breezes days was confirmed by Bajamgnigni and Steyn (2012) and observed by Coulibaly et al. (2019). Fontaine et al. (2008), using a methodology based on long-wave outgoing radiation, and Dalu et al. (2009), using the hydrological index to detect sea breeze days, pointed out that the mean occurrence of WAM was more frequent at end of June. This is consistent with our observations.
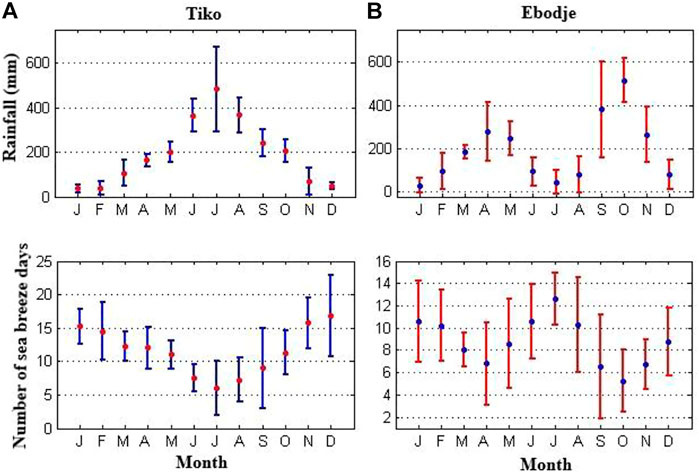
FIGURE 4. Mean and standard deviation monthly frequency of occurrence of sea breeze days and range and mean monthly rainfall and range at Tiko left side (A) and Ebodje right side (B).
Second, in order to analyze the influence of large-scale prevailing winds on the sea breeze occurrences, the wind speed data at 850 hPa from the ERA5 reanalysis were used, which has a horizontal resolution of 0.25° × 0.25°, which represents monsoon winds coming from the ocean and moving toward the continent (https://cds.climate.copernicus.eu/). Only reanalysis values at 6, 12, and 18 UTC were considered when calculating the monthly average large-scale wind speed for each day. Maps of wind speeds at 850 hPa for Tiko and Ebodje over the study period are shown in Figures 6, 7. The reanalysis data show a high seasonal variability in the intensity of the synoptic flow prevailing in both stations. In the northern coastal zone (Figure 6), a maximum wind speed (when the southwesterly wind prevail) of up to 4 m/s from April–July (large rainy season) is observed compared to the rest of the months in the Tiko Plain. In these situations dominated by the strong synoptic winds, the convective enhancement of the sea breeze is weakened or diluted. This explains the minimum in July observed during the period of low frequency of the sea breeze. Then, under weak synoptic conditions, with minimum speeds of up to 2.5 m/s between August–October and January–March, these synoptic winds decelerate, resulting in the appearance and circulation of sea breezes in the Tiko area. This is associated with the maximum observed in March. During the November–January period, the monsoon flow is sheared (speed variation of 2.5–3 m/s), related to the withdrawal of WAM and resulting in the weakening of ITD, which can approach close to the coast and the strong onshore winds associated with the low large-scale flow can intensify the sea breeze. This could explain the maximum observed in November, and thus allow two regimes of sea breeze observed during the rainy and dry period in the Tiko plain. In the southern coastal zone where the direction of synoptic flow is mainly west to southwest, wind maps at 850 hPa in Ebodje show high seasonal variability of large-scale winds. These weak winds (around 2.5 m/s) in January–February (large dry season) get stronger in April (small rainy season), with an average maximum speed of up to 3.5 m/s (Figure 7). Then, these synoptic winds decelerate in July–August (small dry season) to reach minimum speeds in November (large rainy season) with an average minimum speed of <2.5 m/s, which explains the two regimes of variation in the number of sea breeze days marked by two minimum and maximum. The increase in days during the high frequency results in the weakening of the monsoon synoptic flow, which allows the development of the sea breeze at Ebodje. This pattern reverses during the low-frequency period. In the weakening monsoon season (rainy season), Cameroonian central plateaus are constantly swept away by these cool and moist strong trade winds. On the slopes of Mount Cameroon in particular, the in situ observations, at the beginning of the rain season, show that the cumulus clouds that form on the northern and eastern slopes during the day are regularly returned to the south/southwest slopes in an anticyclonic motion (Tsalefac and Dagorne, 1990). At Tiko, the months of November to March, which have the highest number of sea breeze days, correspond to the non-monsoon period (dry season), with the weakening of the monsoon synoptic flow (Figures 6, 7). This period contains 252 days of detected sea breezes, more than half of the total number (470) of sea breeze days detected during the 4-year period (2006–2009). At Ebodje, the months from December to February and June to August, which have the highest number of sea breeze days, correspond to the non-monsoon period (dry season), with monsoon winds having minimum speeds. This period contains 224 days of detected sea breezes, more than half of the total number (351) of sea breeze days detected during the 5-year period (2011–2015). These observations partially support what Simpson (1994) reported on tropical sea breezes that occurred during the monsoon retreat time (dry season). As observed by this author, in our case, sea breezes occurred on about half of days during the non-monsoon season at Tiko and Ebodje.
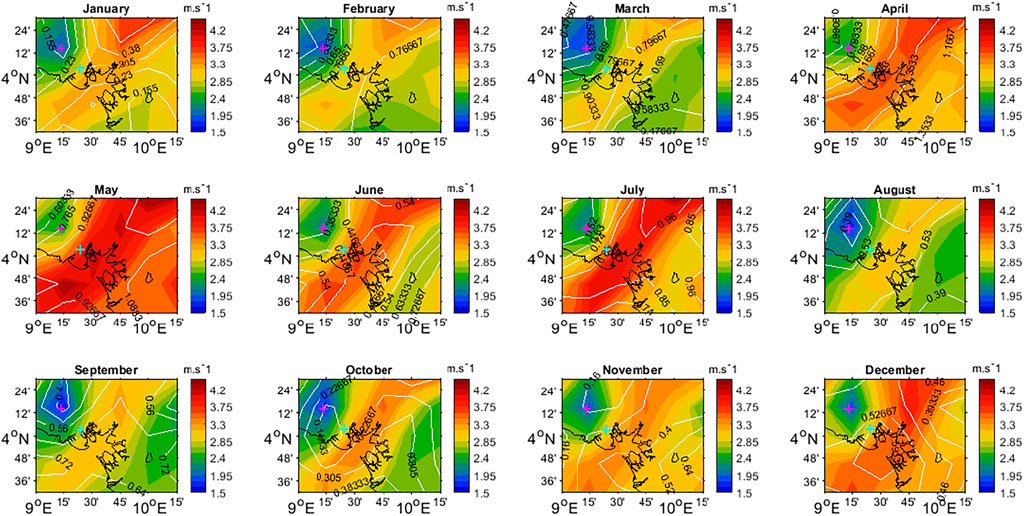
FIGURE 6. Maps of the monthly mean wind speed at 850 hPa at Tiko, from reanalysis data on the study period (2006–2009). The averages are computed from the values at 6, 12, and 18 UTC. The white contours with the labels represent the standard deviation. Cyan and magenta cross correspond to the station and Mount Cameroon location, respectively.
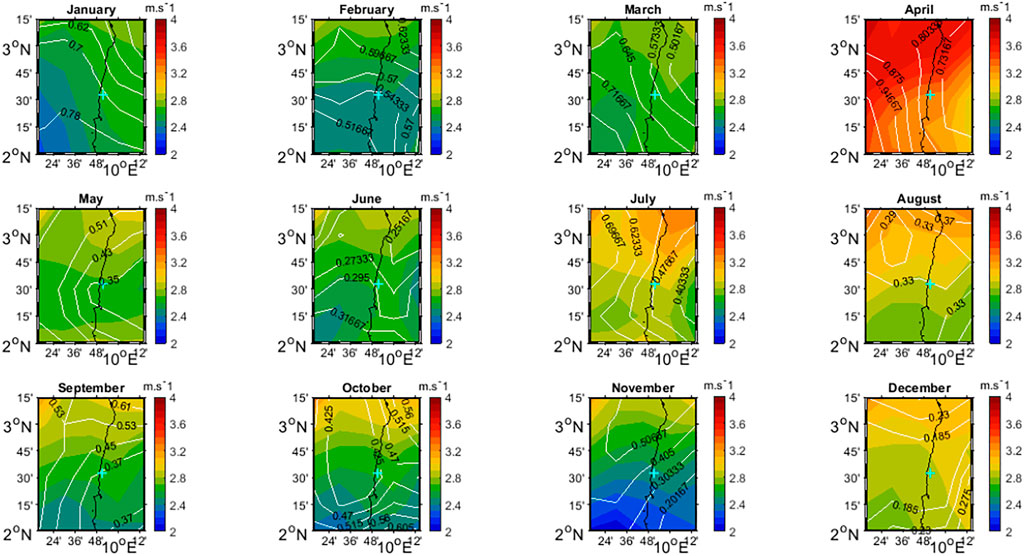
FIGURE 7. Maps of the monthly mean wind speed at 850 hPa at Ebodje, from reanalysis data on the study period (2011–2015). The averages are computed from the values at 6, 12, and 18 UTC. The white contours with the labels represent the standard deviation. Cyan cross correspond to the station location.
Onset, Cessation, and Duration of Sea Breezes
It is of interest to investigate the daily variability of the sea breeze flow. Figure 6 displays the monthly variation in onset and cessation times. For the study period, the sea breeze mean onset time varies between 10:49 (LST) in November and 12:12 (LST) in December, and the sea breeze mean cessation time varies between 17:34 (LST) in June and 19:10 (LST) in February in Tiko. The sea breeze mean cessation time follows roughly similar behavior to that of the onset time (Figure 8). The earliest onset time occurs at 10:49 (LST) in November, while the earliest cessation takes place at 17:34 (LST) in June. Thus, sea breeze onset and cessation times also show a pronounced seasonal variation. During the non-monsoon season (dry season), between November and March, sea breezes occur about 3–4 h after sunrise (6:30 LST) at Tiko, compared to the monsoon season (April to October), which is consistent with the observations of Bajamgnigni and Steyn (2012) at Cotonou, Benin. The occurences of the sea breezes stop about 1.5 h after sunset (18:30 LST). The maximum mean monthly daily duration of the sea breezes at Tiko is 9 h with a minimum being 6 h. This is consistent with the finding of Abatan et al. (2013). The long duration of the observed sea breeze can be related to a very strong onshore synoptic flow. In their study on the influence of the synoptic flow on the evolution of sea breeze in the bay of Alicante (Spain), Azorin-Molina and Chen (2008) found that the onshore synoptic flow favors a longer duration of flow of the sea breeze. Sunrise and sunset times and the duration of sunshine could therefore influence sea breeze times. During the non-monsoon season (November to March), sea breeze onset occurs, on average, later than in the monsoon season. Indeed, the direction of the synoptic wind strongly influences the sea breeze onset times (Leriche et al., 1999). As for the daily duration of the sea breeze, it not only depends on the times of sunrise and sunset but also on the evolution of the speed and direction of the synoptic wind during the development of the sea breeze (Dudouit, 2004). Dahech (2014) reported that the sea breeze mean onset time on the land depends on the season (i.e., sunrise time), distance from the sea, and coastline orientation. The Tiko station is located (near Mount Cameroon) about 19 km from the sea and on a west-northwest/southeast oriented coastline, and the sea breeze mean onset and cessation time in this area is between 11:00–12:00 and 17:00–20:00 (LST), respectively, which is consistent with the finding of Abatan et al. (2013) at the Nigerian coast. The station of Ebodje is still closer to the coast, located about 344 m from the sea and on a north-south oriented coastline. In respect of the characteristics of each station, it is thought that the sea breezes would start, first to blow at Ebodje, with a longer duration, then at Tiko with a delay in the onset and cessation time, and with a shorter duration. However, the wind data were recorded only at two instances (09:00 and 15:00 LST) and do not allow to estimate the sea breeze mean onset and cessation time at Ebodje unlike at Tiko. We can therefore consider that the sea breezes at the Cameroonian coastal region occur 2–3 h after sunrise, at about 09:00 LST, when most of winds blow in an oblique or almost parallel direction to the coastline, with lower minimum speeds in the morning (<1.5 m/s at 09:00 LST), when the majority of the winds blow in a direction almost perpendicular to the coastline, with higher maximum speeds in the early afternoon (<4 m/s at 15:00 LST), and stops blowing 2–3 h after sunset, at about 20:00 LST.
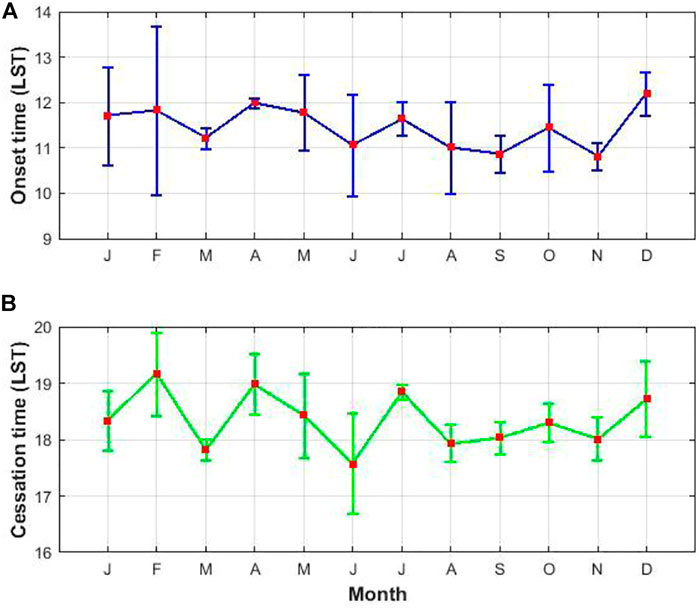
FIGURE 8. Monthly mean and standard deviation of the sea breeze (A) onset and (B) cessation time at Tiko.
Sea Breeze Strength and Evolution Through the Day
Figure 9 shows the mean monthly strengths, or wind speeds, of the sea breezes. At Tiko, the strength of the sea breeze varies between a minimum of 1.90 m/s in July and a maximum of 2.37 m/s in March. The months of December to March (dry season) have higher average wind speeds (>2.1 m/s) compared to the months of April to November (rainy season) which have lower speeds (<2.1 m/s). The one at Ebodje, vary between a minimum in October (1.09 m/s) and a maximum in August (1.52 m/s). In this area the strength of the sea breeze is higher from May to August and in January (>1.30 m/s) when compared to other months (February to April, September to December) which have low energy speeds (<1.30 m/s). Large-scale circulation is characterized by the strong onshore winds that intensify the sea breeze during the dry season. The weak monsoon winds blowing in the same direction as the thermal breeze intensify the sea breeze during the dry season in Tiko and Ebodje. Pushpadas et al. (2010) observed such an effect of the synoptic flow in a numerical model for the west coast of India. The mean strength of the sea breeze speed is 2.1 m/s in the Tiko plain and 1.24 m/s in Ebodje. This may be due to the channelization of the sea toward the station. Recorded values presented herein agree to previous works found in literature along the West African coastline. For example, Abatan et al. (2013), reported the mean breeze wind speeds to vary between 3.0 and 4.8 m/s at the Nigerian coast, and Bajamgnigni and Steyn (2012) registered an average speed of about 4.2 m/s at Cotonou, Benin.
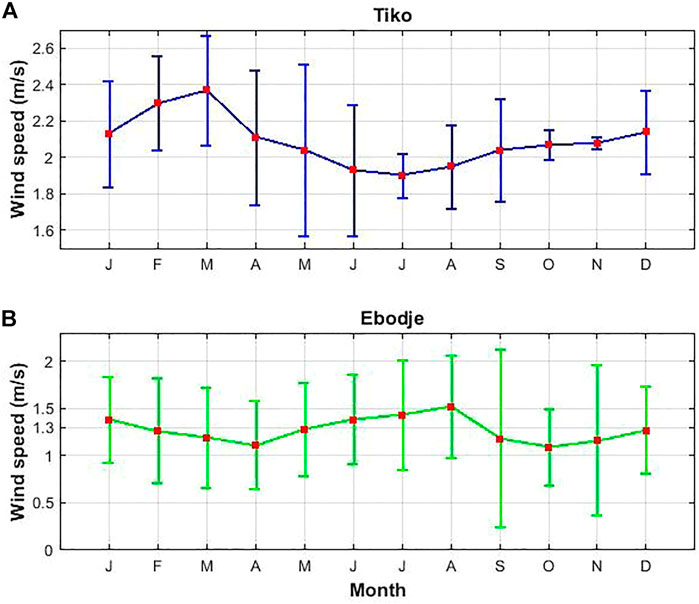
FIGURE 9. Monthly mean and range deviation of wind speed for sea breezes at (A) Tiko and (B) Ebodje.
One of the specific sea breeze characteristics is the clockwise rotation of a theoretical hodograph in the northern hemisphere, due to the Coriolis force veering. In many stations around the world, the deviations from standard hodographs anticlockwise direction were reported. But the effect of Coriolis force would be weak at 4°N. As result at Cameroonian coastal region, one would not expect a systematic sea breeze rotation. Since we have 3-hourly winds data at Tiko and only two instances (09:00 and 15:00 LST) of the winds measured at Ebodje, we will use 3-hourly hodographs to illustrate the sea breeze circulation. The wind data do not allow us to devise the hodographs at Ebodje. Then, we will use the wind roses construct for 09:00 and 15:00 LST (speed and direction) on average to give an idea of the frequency and alternation of the phenomenon at Ebodje.
We plot the 3-hourly hodographs for two individual sea/land breeze days (Figure 10) to study the dynamics of sea breeze circulation in the plain of Tiko. The morning wind is calm for about 3 h of time from 06:00 LST (Figure 10A). It is the diurnal reverse (wind blowing toward the sea at about 06:00 and toward the land at about 09:00 LST, alternating between the land and sea breeze, with the wind now blowing from the sea to the land. And the sea breeze begins to blow around 09:00 LST with a lower speed (1.2 m/s) and westward in direction; then at 12:00 LST its speed increases 2.5 m/s and south-westward in direction, then at 15:00 LST the speed of the sea breeze reaches its maximum (4 m/s) and southward in direction and stops about from 18:00 LST with a calm wind. The sea breeze stops 2–3 h later with a weakening of the wind speed (1 m/s) and the nocturnal reverse (alternation between the sea breeze and the land breeze). Similarly, for the hodograph (Figure 8B), the wind is calm in the morning from 06:00 LST for about 3 h of time. It is the diurnal reverse with alternation between the land breeze and the sea breeze, with the wind now blowing from the sea to the land. And the sea breeze occurs around 09:00 LST with a lower speed (1 m/s) and south-eastward in direction, then at 12:00 LST its speed increases (3 m/s) in southward in direction, then at 15:00 LST the speed of the sea breeze reaches its maximum (5 m/s) and south-westward in direction and stops about from 18:00 (LST) with a calm wind. These hodographs reveal the existence of both clockwise (Figure 10B) and anticlockwise (Figure 10A) wind rotation, as observed by Abatan et al. (2007 and 2013), Bajamgnigni and Steyn, (2012) and Coulibaly et al. (2019). This corresponds to the periods when the main prevailing large-scale flow in Cameroonian coast zone is WAM. Therefore, the sense of rotation of sea breezes on a given day could be the effect of a complex interaction between surface and synoptic pressure gradients, horizontal and vertical diffusion, and advection terms. Coulibaly et al. (2019) on the numerical simulation of land and sea breeze circulation along the Guinean Coast of West Africa, found that the hourly analysis of the rotation term shows over land areas a dominance of diffusion terms. Dahech (2014) reported that the sea breeze reaches its maximum speed (about 5 m/s on average) during the afternoon, and it blows almost perpendicular to the coastline; the breeze continues to blow at a lower speed, varying from 1 to 3 m/s, 2–3 h of time after sunset when the passage between the sea breeze and that of land is triggered (this is the nocturnal reverse). Kusuda and Alpert (1983) have shown that it is possible to rotate both clockwise and anticlockwise in the northern hemisphere. The observed daily hodographs of sea breeze in Tiko are in reasonable accord with these observations.
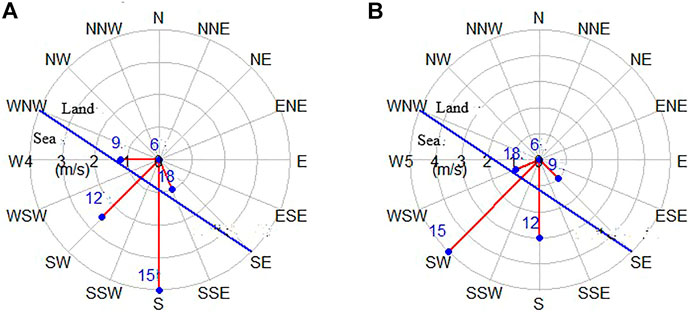
FIGURE 10. (A) Hodograph on 21 April 2008. (B) Hodograph on 23 December 2008 at Tiko. The blue dots and red line indicate the speed and direction. The numbers near the blue dots indicate the hour of the day (LST). The blue line indicates the coastline orientation. All indications are shown on this and all figures that follow.
The mean daily hodographs by seasons have been plotted (Figures 11A–D) to investigate the cause of the two senses of rotation at Tiko station. Two distinct features in the hodograph shape are when months are grouped to build the mean hodographs for different seasons. In contrast to hodographs of individual days plotted above, the average seasonal hodographs (Figures 11C,D) show no very clear diurnal rotation of wind direction for the rainy season associated to the main large-scale dominant flows (WAM), except that for the period from December to February (Figure 11A) which shows a weak rotation anticlockwise related to the dominant diffusion terms over land areas, as observed by Coulibaly et al. (2019). Average seasonal hodographs do not have a systematic rotation. We can have periods when the rotation of the sea breeze is either clockwise, either anticlockwise or sometimes times the rotation is not very clear. This observation is similar with the finding of Abatan et al. (2013), and observations of Bajamgnigni and Steyn (2012) who found that average seasonal hodographs do not show a statistically significant rotation of the sea breeze. As well as the Coriolis force which is weak at this latitude, the lack of rotation is related to the fact that the Tiko region is located close to the mountains of Cameroon and would have a complex local topography. As result, there is not also possible to be any systematic rotation on mean hodograph. Figure 12, displaying the mean hodograph over all sea breeze days for the 4-year study period, is convincing evidence of this. The rotation would be the first clockwise and then anticlockwise, which would be likely related to WAM. Coulibaly et al. (2019) found that the patterns of the diurnal rotation of wind are influenced by the large-scale flow characterized by WAM in the summer. The rotational trends on land are affected by the presence of steep topography and the spatial distribution of land use and cover. Dahech (2015) observed that the coastline orientation strongly changes and influences the direction of the sea breeze. The latter when it is well established, in the early afternoon, blows almost perpendicular to the coastline. Average seasonal hodographs clearly show the offshore and onshore nature of the sea breeze circulation but with indeterminate diurnal rotation of wind direction in Tiko and shows the important contribution of the shape of the local coastline and the influence of the local topography. As found by Coulibaly et al. (2019) on the numerical simulation of land and sea breeze flow, the direction of rotation can be attributed to the variation in the roughness of the land surface due to landscape and urbanization. The influence of the local orientation coastline was investigated with the wind roses devise at Ebodje.
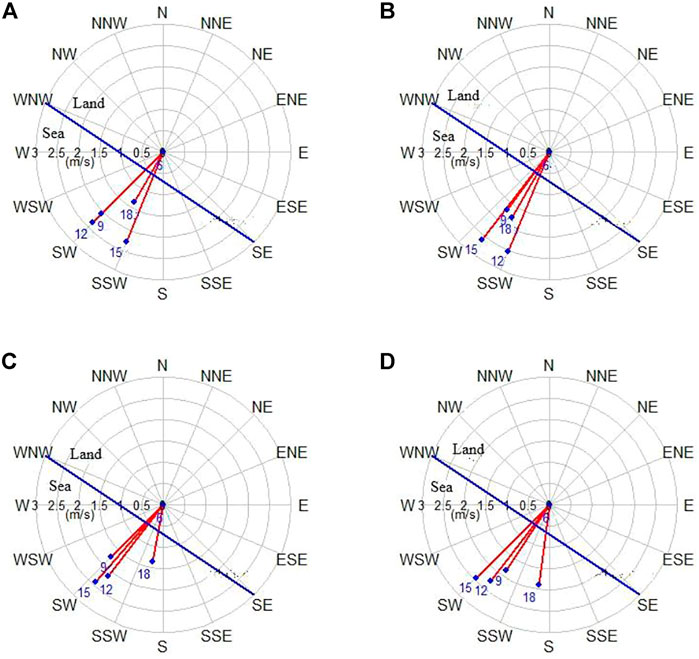
FIGURE 11. Mean hodographs at Tiko, averaged for the periods: (A) December to February (B) March to May; (C) June to August (D) September to November.
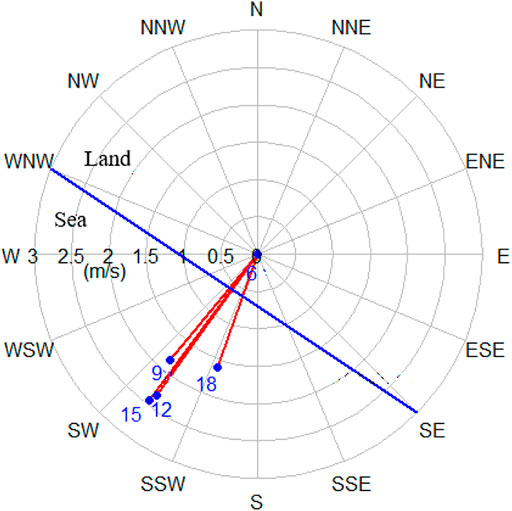
FIGURE 12. Mean hodographs averaged over all sea breeze days for the 4-year 2006–2010 study period at Tiko.
Seasonal wind roses were plotted for wind data collected at Ebodje station to study the temporal dynamics of sea breeze flow (Figure 13). These wind roses were performed for 09:00 LST and 15:00 LST (speed and direction) over a 5-year period (2011–2015), which give an idea of the frequency and strength of sea breezes. It is revealed a predominance of wind direction in the north and south sectors at 09:00 LST and west sector at 15:00 LST in each season; with high frequencies of occurrence of sea breezes at 09:00 LST from December to February in the south (26%) and north (25%) sectors and then at 15:00 LST in the west (23%) sector (Figures 13A,B). Frequencies are higher (21%) in the south sector and (26%) in the north at 09:00 LST from March to May and then at 15:00 LST in the west (16%) sector (Figures 13C,D). Sea breezes are frequent at 09:00 LST from June to August in the south (36%) and north (21%) sectors and then at 15:00 LST in the west (26%) sector (Figures 13E,F). Similarly, winds are frequent at 09:00 LST from September to November in the south (30%) and north (23%) sectors and then at 15:00 LST in the west (18%) sector (Figures 13G,H). These observations show a high temporal variability of sea breezes frequent especially during the dry season and especially during the period from July to August in the south sector (36%) at 09:00 LST and then at 15:00 LST in the west sector (26%) because of the preponderance of calm atmospheric situations conducive to its triggering. The sea breeze begins to blow at 09:00 LST at a slower speed (<1.5 m/s) either in the sectors between the north and north-northwest, or in the sectors between the south and southwest, on the continent in an oblique direction (about 15°) or almost parallel to the coastline, before strengthening sharply and blowing at 15:00 LST at a higher speed (>1.5 m/s) of the sector between the west-southwest and the northwest (Figure 13). The maximum speed of the sea breeze is recorded at 15:00 LST. It reaches an average of 3 m/s in open coastal resorts such as Tiko. The breeze continues to blow at a lower speed, varying from 1 to 3 m/s 2–3 h after sunset when the passage between the sea breeze and the land breeze is triggered, as observed by Dahech (2012 and 2015). The anticlockwise or hourly rotation of the sea breeze at Ebodje during the day is not significantly visible on the wind roses unlike the anticlockwise rotation of the hodographs of the sea breeze at Tiko station because we have only two instances (09:00 and 15:00 LST) of the winds. The sea breeze blows at Ebodje in the sector between the west-southwest and the northwest in all seasons (Figure 13). However, the sea breeze comes from: either the sectors between the north and north-northwest where the coastline orientation is oriented east-northeast/south-southwest to the north-northeast at Ebodje; either the sectors between the south and southwest where the coastline orientation is north/south to the south at Ebodje. Then the sea breeze occurs in the sectors between west-southwest and northwest when the shoreline is north/south or north-northeast/south-southwest on land. These observations could therefore confirm the change in the direction of the sea breeze according to the coastline orientation in the Ebodje area. The sea breeze average speed in this area does not exceed 1.5 m/s, while it can exceed 2 m/s in open environments, as observed by Dahech (2015). The impact of urban morphology, land using, and soil nature on the sea breeze flow would be indisputable, and the breeze is slowed down not only by the buildings in the city but also by the vegetation by the sea. This is the case of the coastal Atlantic forests and mangroves forests along Ebodje and Tiko.
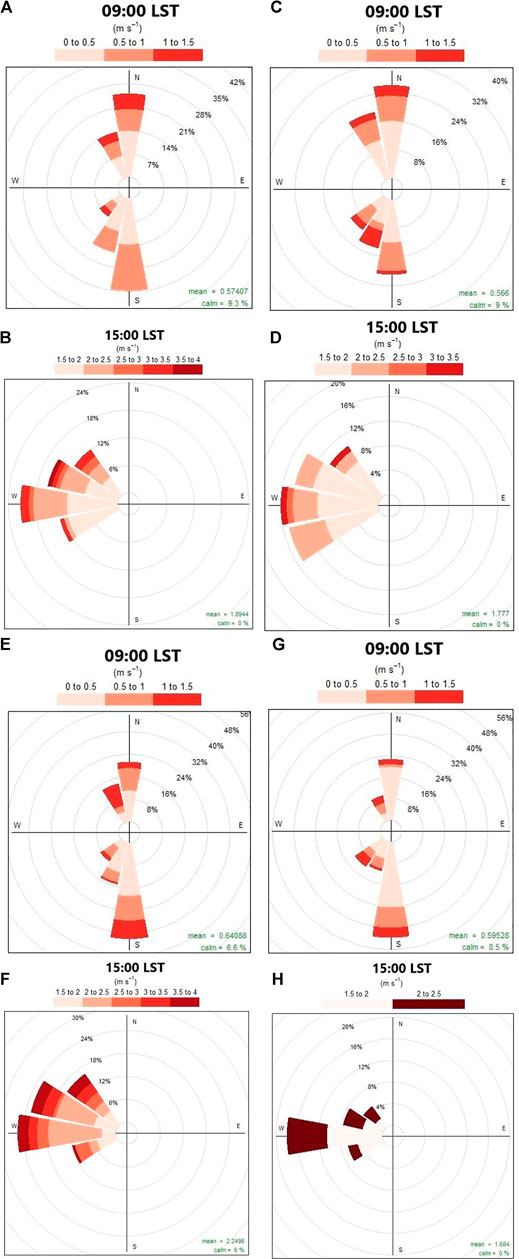
FIGURE 13. Seasonal wind roses with the frequency of occurrence of wind direction and range of wind speed for sea breezes at Ebodje: (A,B) December to February; (C,D) March to May. (E,F) June to August; (G,H) September to November.
Conclusion
The study on the importance of the characteristics of sea breezes in Tiko and Ebodje provides information on their frequency of occurrence highlighting two regimes: the higher frequency regime (November to April at Tiko and June to August and December to February at Ebodje) and the low-frequency regime (May to October at Tiko and March to May and September to November at Ebodje), with a total of 470 (351) days of sea breeze detected in Tiko (in Ebodje). A sea breeze is a relatively common phenomenon throughout the year in the Tiko and Ebodje areas. The sea breeze occurrences depend heavily on the seasons. The point-polyserial correlation test shows a strongly anticorrelation between sea breezes and monsoonal rainfall variability. An analysis of the influence of large-scale prevailing winds, characterized by the WAM on the sea breezes occurrence, shows that sea breezes occurrence is strongly linked to the WAM.
The sea breeze onset and cessation time also indicate a pronounced seasonal variation. The sea breeze average onset time is between 11:00 and 12:00 LST, and cessation time is between 17:00 and 20:00 LST, with a maximum monthly mean duration of 9 h, with a speed of 2.1 m/s in the Tiko area, while the occurrence period of sea breezes is between 9:00 and 15:00 LST, with a speed of 1.24 m/s in the Ebodje area.
The evolution of sea breezes in Tiko shows that the daily hodographs of sea breeze have a systematic rotation clockwise or anticlockwise, and this is related to the details of local and synoptic influences on the given day. Average seasonal hodographs have an indeterminate rotation, or at least if systematic rotation exists, it is usually obscured by daily variation. Examination of the offshore and onshore nature of the sea breeze shows a seasonal variation in the shape of the mean hodographs, presumably due to the influence of synoptic flows, mainly characterized by the WAM. The evolution of sea breezes shows a high temporal variability of sea breezes frequent in Ebodje especially during the dry season from July to August in the south sector (36%) at 9:00 LST and then at 15:00 LST in the west sector (26%) because of the preponderance of calm atmospheric situations conducive to its triggering.
The results and analyses presented herein bring an important contribution to understand sea breeze dynamics in the coastal regions of Cameroon, which can be the main factor in controlling the dynamics of oil slicks, air pollutants, and local wind-driven circulation. Sea breeze winds and induced surface currents can cause oil slicks to move to onshore, which degrades marine protected areas, seagrass beds, and spawning grounds of marine species (e.g., sea turtles). Winds can also, during sea breezes occurrence, transport air pollution to land, which affects the health of coastal populations and their living conditions. Therefore, future studies on winds or thermal breezes could be of great scientific and environmental interest due to their impact on climate, air quality, and human health, especially in the coastal regions of Cameroon.
Data Availability Statement
The original contributions presented in the study are included in the article/Supplementary Material; further inquiries can be directed to the corresponding author.
Author Contributions
FK and AM planned and organized the study. All authors wrote and reviewed the manuscript.
Funding
This work is a contribution of the Brazilian Research Network on Global Climate Change FINEP/ Rede CLIMA Grant 01.13.0353-00.
Conflict of Interest
The authors declare that the research was conducted in the absence of any commercial or financial relationships that could be construed as a potential conflict of interest.
Publisher’s Note
All claims expressed in this article are solely those of the authors and do not necessarily represent those of their affiliated organizations, or those of the publisher, the editors, and the reviewers. Any product that may be evaluated in this article, or claim that may be made by its manufacturer, is not guaranteed or endorsed by the publisher.
Acknowledgments
The authors greatly appreciate the Regional Transport Delegation of Cameroon for the provision of data used for the research. FCKT and ACM thank the support of the Brazilian Research Network on Global Climate Change FINEP/Rede CLIMA Grant 01.13.0353-00.
References
Abatan, A. A., Abiodun, J. B., and Omotosho, J. B. (2013). On the Characteristics of Sea Breezes over Nigeria Coastal Region. Theor. Appl. Climatol. 116, 93. doi:10.1007/s00704013-0931-z
Abatan, A. A., Obodiun, B. J., and Omotosho, B. J. (2007). An Observational Study of Sea Breeze over Nigeria Coastal Region. Res. J. Appl. Sci. 2 (6), 745–751. Available at: https://medwelljournals.com/abstract/?doi=rjasci.2007.745.751.
Azorin-Molina, C., and Chen, D. (2008). A Climatological Study of the Influence of Synoptic-Scale Flows on Sea Breeze Evolution in the Bay of Alicante (Spain). Theor. Appl. Climatol. 96, 249–260. doi:10.1007/s00704-008-0028-2
Azorin-Molina, C., Chen, D., Tijm, S., and Baldi, M. (2011). A Multi-Year Study of Sea Breezes in a Mediterranean Coastal Site: Alicante (Spain). Int. J. Climatol. 31, 468–486. doi:10.1002/joc.2064
Bajamgnigni Gbambie, A. S., and Steyn, D. G. (2012). Sea Breezes at Cotonou and Their Interaction with the West African Monsoon. Int. J. Climatol. 33, 2889–2899. doi:10.1002/joc.3637
Banfield, C. E. (1991). The Frequency of Surface Characteristics of Sea Breeze at St. Johns, New Found Land. Climatol. Bull. 25, 3–20.
Bechtold, P., Pinty, J.-P., and Mascart, F. (1991). A Numerical Investigation of the Influence of Large-Scale Winds on Sea-Breeze- and Inland-breeze-type Circulations. J. Appl. Meteor. 30, 1268–1279. doi:10.1175/1520-0450(1991)030<1268:anioti>2.0.co;2
Borne, K., Chen, D., and Nunez, M. (1998). A Method for Finding Sea Breeze Days under Stable Synoptic Conditions and its Application to the Swedish West Coast. Int. J. Climatol. 18, 901–914. doi:10.1002/(sici)1097-0088(19980630)18:8<901:aid-joc295>3.0.co;2-f
Cautenet, S., and Rosset, R. (1989). Numerical Simulation of Sea Breezes with Vertical Wind Shear during Dry Season at Cape of Three Points, West Africa. Mon. Wea. Rev. 117, 329. doi:10.1175/1520-0493(1989)117<0329:nsosbw>2.0.co;2
Cooper, H. J., Smith, E. A., and Rubes, M. T. (1998). Relevance of Surface Energy Budget within Florida Sea-Breeze Front to Cross-Peninsula Rainwater Runoff Gradient. J. Appl. Meteor. 37, 939–950. doi:10.1175/1520-0450(1998)037<0939:rosebw>2.0.co;2
Coulibaly, A., Omotosho, B. J., Sylla, M. B., Coulibaly, A., and Ballo, A. (2019). Characteristics of Land and Sea Breezes along the Guinea Coast of West Africa. Theor. Appl. Climatol. 138, 953–971. doi:10.1007/s00704-019-02882-0
Dahech, S. (2014). “Brise de mer et dégradation de la qualité de l’air dans les villes in ustrielles: cas de Tunis, Sfax et Gabes,” in Risque climatiques en Tunisie (dir, Hénia L), 26p.
Dahech, S. (2015). “Caractéristiques de la brise littorale dans la région de Sfax (centre tunisien),” in XXVIIIe Colloque de l’Association Internationale de Climatologie, Liège, 6p.
Dahech, S. (2012). Évolution de la répartition spatiale des températures de l'air et de surface dans l'agglomération de Sfax entre 1987 et 2010. Impact sur la consommation d'énergie en été. Climatologie 9, S11–S32. doi:10.4267/climatologie.543
Dalu, G. A., Gaetani, M., and Baldi, M. (2009). A Hydrological Onset and Withdrawal Index for the West African Monsoon. Theor. Appl. Climatol. 96, 179–189. doi:10.1007/s00704-008-0022-8
Dudouit, A. (2004). Une étude de brise de mer dans la région caennaise. Norois 4 (193), 31–45. doi:10.4000/norois.721
Eager, R. E., Raman, S., Wootten, A., Westphal, D. L., Reid, J. S., and Al Mandoos, A. (2008). A Climatological Study of the Sea and Land Breezes in the Arabian Gulf Region. J. Geophys. Res. 113, D15106. doi:10.1029/2007JD009710
Eltahir, E. A. B., and Gong, C. (1996). Dynamics of Wet and Dry Years in West Africa. J. Clim. 9, 1030–1042. doi:10.1175/1520-0442(1996)009<1030:dowady>2.0.co;2
Folack, J., and Ngassa, J. (1994). Environmental Baseline Survey for Offshore Oil Exploration in the Sanaga II Area. Germany: Consultation report Phillips Petroleum Company Cameroon/Gopa consultants Hindenburgring, 11p.
Folack, J., and Ngueguim, J. R. (2007). Rapport de mission sur l’évaluation de la pollution côtière suite à l’incident survenu au terminal flottant de stockage et de déchargement (TFSD) COTCO au large de Kribi, 11p.
Fontaine, B., Louvet, S., and Roucou, P. (2008). Definition and Predictability of an OLR-Based West African Monsoon Onset. Int. J. Climatol. 28, 1787–1798. doi:10.1002/joc.1674
Frizzola, J. A., and Fisher, E. L. (1963). A Series of Sea Breeze Observations in the New York City Area. J. Appl. Meteor. 2, 722–739. doi:10.1175/1520-0450(1963)002<0722:asosbo>2.0.co;2
Furberg, M., Steyn, D. G., and Baldi, M. (2002). The Climatology of Sea Breezes on Sardinia. Int. J. Climatol. 22, 917–932. doi:10.1002/joc.780
Gabche, C. E., Folack, J., and Yongbi, G. C. (1998). Tar Ball Levels on Some Beaches in Cameroon. Mar. Pollut. Bull. 36 (7), 535–539. doi:10.1016/S0025-326X(97)00200-2
Gustavsson, T., Lindqvist, S., Borne, K., and Bogren, J. (1995). A Study of Sea and Land Breezes in an Archipelago on the West Coast of Sweden. Int. J. Climatol. 15, 785–800. doi:10.1002/joc.3370150706
Ikome, E. F. (1985). The Levels of Pollution Due to Hydrocarbons on Some Beaches of the Cameroon West Coast, a Preliminary Survey. Rev.Sci. Techn, Ser. Zoo Tech. 2 (4), 81–83.
Kusuda, M., and Alpert, P. (1983). Anti-clockwise Rotation of the Wind Hodograph. Part I: Theoretical Study. J. Atmos. Sci. 40, 487–499. doi:10.1175/1520-0469(1983)040<0487:acrotw>2.0.co;2
Leriche, P., Kergomard, C., and Chapelet, P. (1999). Etude des brises de mer sur le littoral français de la mer du Nord. Publ. l’Association Int. Climatol. 11, 107–114.
Lu, R., and Turco, R. P. (1994). Air Pollutant Transport in a Coastal Environment. Part I: Two-Dimensional Simulations of Sea-Breeze and Mountain Effects. J. Atmos. Sci. 51 (15), 2285–2308. doi:10.1175/1520-0469(1994)051<2285:aptiac>2.0.co;2
Maja, T. P. (2003). Main Characteristics of Sea/land Breezes along the Eastern Coast of Northern Adriatic. G. eofizika 20, 75–92. Available at: https://hrcak.srce.hr/17198.
Miao, J.-F., Kroon, L. J. M., Vilà-Guerau de Arellano, J., and Holtslag, A. A. M. (2003). Impacts of Topography and Land Degradation on the Sea Breeze over Eastern Spain. Meteorology Atmos. Phys. 84, 157–170. doi:10.1007/s00703-002-0579-1
Michael, W. S. (2003). Sea Breeze Fronts and Their Role in Convective Initiation. Raleigh, North Carolina: North Carolina State University, 22.
MINEP (2010). Plan d’action national de gestion des zones marine et côtière. Yaoundé-Cameroon ENVIREP Cameroon, 140. Available at: http://hdl.handle.net/1834/5227.
Mounier, F., Kiladis, G. N., and Janicot, S. (2007). Analysis of the Dominant Mode of Convectively Coupled Kelvin Waves in the West African Monsoon. J. Clim. 20, 1487–1503. doi:10.1175/JCLI4059.1
Olivry, J. C. (1986). Fleuves et rivières du Cameroun. Paris (FRA): Yaoundé: ORSTOM; MESRES, 745. (Monographies Hydrologiques; 9).
Pielke, R. A. (1974). A Three-Dimensional Numerical Model of the Sea Breezes over South Florida. Mon. Wea. Rev. 102, 115–139. doi:10.1175/1520-0493(1974)102<0115:atdnmo>2.0.co;2
Pushpadas, D., Vethamony, P., Sudheesh, K., George, S., Babu, M. T., and Balakrishnan Nair, T. M. (2010). Simulation of Coastal Winds along the Central West Coast of India Using the MM5 Mesoscale Model. Meteorol. Atmos. Phys. 109 (3–4), 91–106. doi:10.1007/s00703-010-0086-8
Rao, P. A., and Fuelberg, H. E. (2000). An Investigation of Convection behind the Cape Canaveral Sea-Breeze Front. Mon. Wea. Rev. 128, 3437–3458. doi:10.1175/1520-0493(2000)128<3437:aiocbt>2.0.co;2
Sadler, J. C. (1975). “The Upper Tropospheric Circulation over the Global Tropics,” in Atlas UHMET 75 – 05 (Honolulu: Dep. of Meteorol. Univ. of Hawaii), 35.
Sayer, A. J., Harcourt, S. C., and Collins, M. N. (1992). The Conservation Atlas of Tropical Forest Africa. London: IUCN, Macmillan Publishers Ltd, 13–130. doi:10.1007/978-1-349-12961-4
Simpson, J. E. (1995). Notes and Correspondence: Diurnal Changes in Sea-Breeze Direction. J. Appl. Meteorology 35, 1166–1169. Available t: https://www.jstor.org/stable/26188124.
Smithson, P. A. (1982). Meso-scale Atmospheric Circulations. B. W. Atkinson, Academic Press, London, 1981. No. Of Pages: 495, Price: £32.40. J. Climatol. 2, 303–304. doi:10.1002/joc.3370020313
Steyn, D. G., and Faulkner, D. A. (1986). The Climatology of Sea-Breezes in the Lower Fraser Valley, B.C. Climatol. Bull. 20 (3), 21–39.
Sultan, B., and Janicot, S. (2003). The West African Monsoon Dynamics. Part II: The "Preonset" and "Onset" of the Summer Monsoon. J. Clim. 16, 3407–3427. doi:10.1175/1520-0442(2003)016<3407:twamdp>2.0.co;2
Tsalefac, M., and Dagorne, D. (1990). Convection, relief et pluviométrique au Cameroun en mars et octobre. Ministère la coopération. Paris-France: OSTROM, METEO France, 13p.
Keywords: sea breeze characteristics, circulation, surface wind, Cameroonian coast, West African monsoon
Citation: Kouogang Tchuenkam FC, Mama AC, Gah-Muti SY and Araujo M (2022) Variability of Sea Breezes Over the Cameroonian Coast and Their Interaction With the West African Monsoon. Front. Earth Sci. 10:848684. doi: 10.3389/feart.2022.848684
Received: 04 January 2022; Accepted: 25 April 2022;
Published: 31 May 2022.
Edited by:
Matthias Prange, University of Bremen, GermanyReviewed by:
Pak Wai Chan, Hong Kong Observatory, Hong Kong SAR, ChinaMartin Bergemann, German Climate Computing Centre (MPG), Germany
Copyright © 2022 Kouogang Tchuenkam, Mama, Gah-Muti and Araujo. This is an open-access article distributed under the terms of the Creative Commons Attribution License (CC BY). The use, distribution or reproduction in other forums is permitted, provided the original author(s) and the copyright owner(s) are credited and that the original publication in this journal is cited, in accordance with accepted academic practice. No use, distribution or reproduction is permitted which does not comply with these terms.
*Correspondence: Fabius Cédric Kouogang Tchuenkam, ZmFiaXVzLmNlZHJpY0B5YWhvby5mcg==