- 1Key Laboratory of Sustainable Forest Ecosystem Management-Ministry of Education, School of Forestry, Northeast Forestry University, Harbin, China
- 2State Key Laboratory of Frozen Soils Engineering, Da-Xing’anling Observatory and Research Station of Permafrost Engineering and Environment, Northwest Institute of Eco-Environment and Resources, Chinese Academy of Sciences, Lanzhou, China
- 3School of Civil Engineering, Institute of Cold-Regions Science and Engineering, Northeast-China Observatory and Research-Station of Permafrost Geo-Environment (Ministry of Education PRC), Northeast Forestry University, Harbin, China
Carbon storage is an important component of ecosystem services. Under climate warming and human activities, land use/land cover (LULC) have been undergoing tremendous change, leading to spatio-temporal variations in carbon storage. Based on seven series of LULC data and combined with carbon module of Integrated Valuation of Ecosystem Services and Tradeoffs (InVEST) model, spatial-temporal changes in LULC types and carbon storage were analyzed and estimated for Heilongjiang Province, Northeast China. Results show varied carbon storage among different types of LULC. Forest and cropland are the dominant LULC types in Heilongjiang Province, Northeast China, accounting for 46–49% (20.90 × 104–22.12 × 104 km2) and 30–37% (13.56 × 104–16.70 × 104 km2) of the total area. Areal extents of forest, grassland, and unused land declined from 1980 to 2015, by 1.22, 0.84, and 1.11 × 104 km2, respectively; while those of cropland and construction land expanded, by 3.14 and 0.08 × 104 km2, respectively. From 1980 to 2015, carbon storage displayed consistent change trends with those of LULC types: carbon storage of forest, grassland, and unused land decreased by 236.22, 116.61 and 21.82 × 106 Mg C, respectively; and those of cropland and construction land increased by 414.65 and 0.99 × 106 Mg C, respectively. The total carbon storage in the study region was 6,863.06 × 106–6,907.64 × 106 Mg C, for which the forest, cropland, and grassland were the major contributor (6,778.75 × 106–6,840.57 × 106 Mg C). Due to the conversion of large extents of forest, grassland, and unused land to cropland, which facilitated the formation of carbon sinks and thus enlarged the carbon storage by 45.36 × 106 Mg C from 1980 to 2015. Frequent forest fires, urban expansion, farmland reclamation, and engineering construction were the important factors of changes in the LULC, accelerating permafrost degradation and leading to obvious changes in the total carbon storage in the Heilongjiang Province, Northeast China. Therefore, the estimation of carbon storage in different LULC types can provide important data support and have important implications for evaluation of ecosystem services and carbon cycle.
Introduction
Ecosystem services refer to the benefits that derive from the structure and function of ecosystems and are major contributors to human well-being (Adelisardou et al., 2021). However, these services are currently under great pressure due to anthropogenic activities and climate change, such as continuous growth of population, industrialization, urbanization, and wildfire (Tolessa et al., 2017; Xie et al., 2017; Li et al., 2020a). Thus, protecting natural ecosystems and enhancing their services have become an urgent global challenge (Ouyang et al., 2016). Carbon storage is a key indicator of ecosystem services function since its closely related with climate regulation and productivity of terrestrial ecosystems (Houghton, 2003; Zhao et al., 2019). Climate and land use/land cover (LULC) changes are the major influencing factors for ecosystem carbon storage, due to fundamental changes in the structure and functions of ecosystems over time (Adelisardou et al., 2021). Climate change alters ecosystem carbon storage by controlling the balance between carbon inputs from plant productivity and carbon outputs from soil carbon decomposition (Wang et al., 2019). Under a warming climate, boreal ecosystems are experiencing continuous and severe LULC changes, affecting the environment, biodiversity, and human health (Viana et al., 2019; Serban et al., 2021). Changes in LULC types are usually accompanied by a large amount of carbon exchange and alter the carbon cycle process by modifying the ecosystem’s structure and function. (Noble et al., 2000; Chen and Tian, 2007).
The Integrated Valuation of Ecosystem Services and Tradeoffs (InVEST) model is a suite of free, open-source software used to map and assess the changes in carbon storage due to LULC changes (Zhao et al., 2019). Carbon storage module of InVEST is a simplified carbon cycle that maps and quantifies how much carbon is stored in a landscape, as well as how much is sequestered or lost over time (Dida et al., 2021). The InVEST model is more effective in assessing and studying the impact of climate and LULC change on ecosystem carbon storage (Nie et al., 2020). Under a warming climate, understanding the relationship between LULC systems and carbon stock is essential since every LULC system has either a positive or negative impact on the carbon balance (Toru and Kibret, 2019). Carbon dynamics are highly influenced by the alteration of forest cover and different land cover types that store varying amounts of carbon (Dida et al., 2021).
In permafrost regions, the LULC change has significant consequences on ecological environment and engineered systems (Serban et al., 2021). The area of all soils in the northern circumpolar permafrost region is approximately 1.8782 × 107 km2, or approximately 16% of the global soil area (Tarnocai et al., 2009). However, this region contains approximately 1,672 Pg C, of which 1,466 Pg C is stored in permafrost terrains (Tarnocai et al., 2009). This 1,672 Pg C accounts for approximately 50% of the estimated global belowground organic carbon pool (Tarnocai et al., 2009). Heilongjiang Province in Northeast China is on the southern margin of both the boreal coniferous forest and the Eurasia permafrost body, which is more sensitive to the LUCC and climate change. Moreover, Heilongjiang Province is a major province of agriculture, industry and forestry in China, and an important grain production base and primary forest distribution region. It has the largest natural forest in China, with strong carbon sink reserves and carbon sink production capacity (Guo, 2011), and has the highest plant carbon storage and carbon density in China (Wang et al., 2001). Heilongjiang Province is located in the mid-high latitude region, with low temperature all the year round, and vegetation grows slowly. However, the increase in population and human activity (e.g., deforestation) had led to significant changes in LULC before 2000s. Therefore, this study aims at exploring the changes of LULC and their effects on carbon storage in Heilongjiang Province in Northeast China during the past 35 years (1980–2015) under a changing climate and human activities. The quantitative assessment of ecosystem carbon storage is vital for sustainable development and utilization of natural resources and proper management of ecological environment. The main objectives were: 1) to analyze the spatio-temporal patterns of LULC and carbon storage; 2) to evaluate the impact of climate change, forest fire and human activities on the LULC and the response characteristics of carbon storage to LULC changes.
Study Region and Methods
Study Region
This study region (Heilongjiang Province) (43.4167°—53.05°N; 121.1833°—135.0833°E; 0-1,692 m a. s. l.), Northeast China (Figure 1) is rich in water resources, with four water systems: the Heilongjiang-Amur River and tis three tributaries (Songhua, Suifen and Ussuri rivers). The Songhua River is the largest tributary of the Heilongjiang-Amur River. The Wudalianchi and Xingkai lakes are the main inland lakes. Heilongjiang Province is bestowed with the most important state-owned forest area and largest timber production base in China. The rich forest vegetation types mainly include Larix gmelinii (Rupr.) Kuzen, Pinus koraiensis Sieb. et Zucc., Pinus sylvestris var. mongolica Litv., Picea asperata Mast., Abies fabri (Mast.) Craib, Betula platyphylla Suk., Quercus mongolica Fisch. ex Ledeb., Acer saccharum Marsh, and Fraxinus mandshurica Rupr. The soil type in the study region is brown coniferous forest soil.
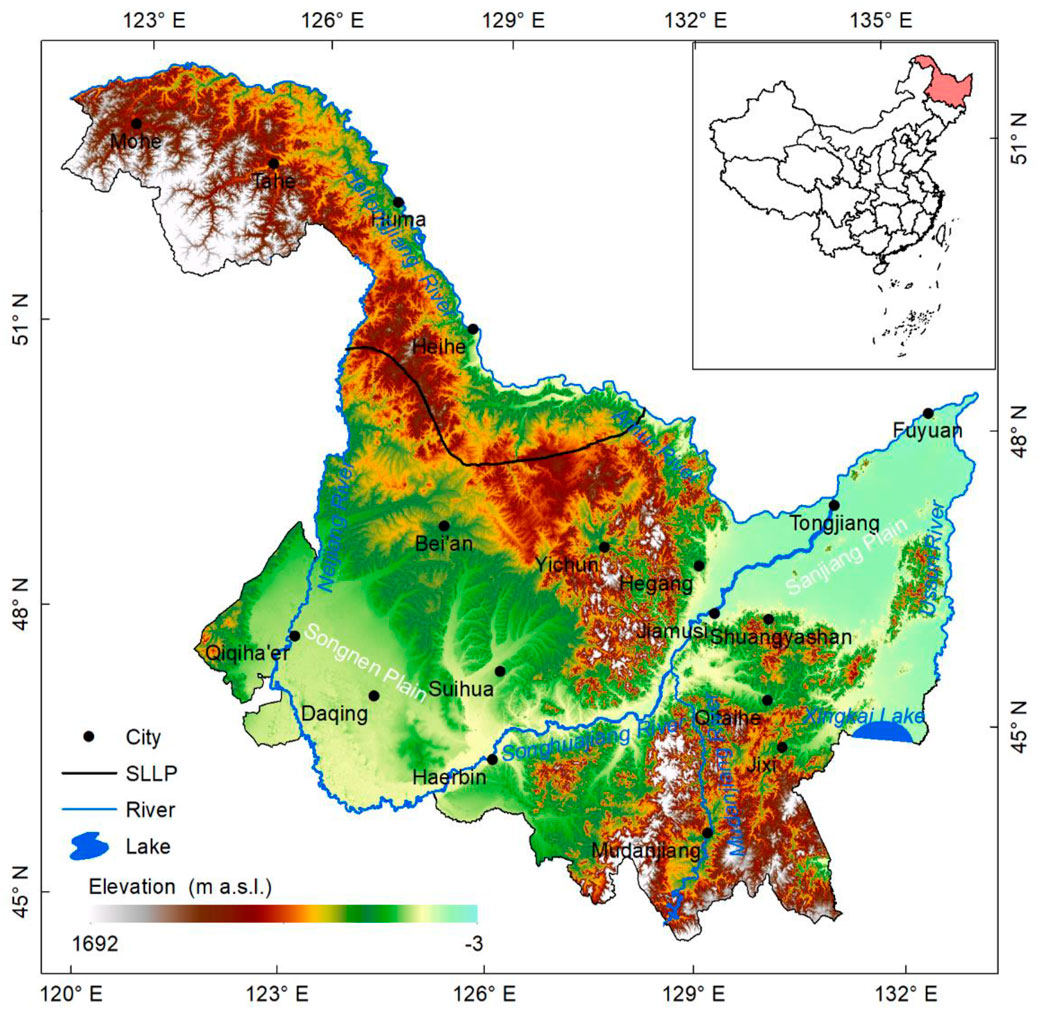
FIGURE 1. The study region (Heilongjiang Province) in Northeast China. The SLLP means Southern limit of latitudinal permafrost.
The region is characterized by a cold temperate continental monsoon climate with mean annual air temperature (MAAT) of 2.22°C (from 1961 to 2019), at a rising rate of 0.25°C/10a, and with a mean annual precipitation of 518 mm, at a rate of 8.3 mm/10a (Figure 2). Compared with the MAAT during 1961–1987 (1.67°C), the MAAT increased by 1.01°C during 1988–2019 (2.68°C). The increasing trend of MAAT is very significant (p < 0.001).
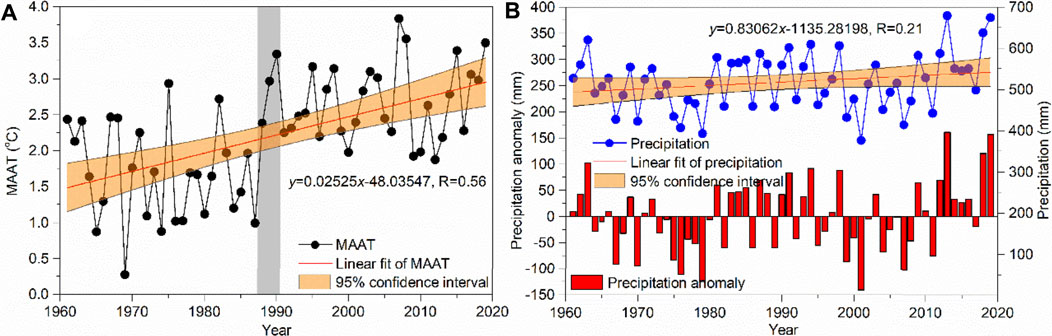
FIGURE 2. Changes in the mean annual air temperature (MAAT) and mean annual precipitation during 1961–2019 in Heilongjiang Province, Northeast China during 1961–2019.
Data Sources
The meteorological data were obtained from the National Meteorological Information Center of China (http://www.nsmc.cma.gov.cn). The LULC data were downloaded from the Resource and Environment Science and Data Center (https://www.resdc.cn/?aspxerrorpath=/Datalist1.aspx), with the resolution of 1 km. The digital elevation map (DEM) was collected from Geospatial Data Cloud (http://www.gscloud.cn/home), and the resolution is 90 m.
Carbon Module of InVEST Model
The InVEST model is based on the ArcGIS or as a stand-alone to transformed into software tools using Python as the scripting language (Liu. et al., 2018). The carbon module of InVEST uses a simplified carbon cycle to estimate the amount of static carbon storage and dynamic sequestration for each cell in a specific region (Tallis et al., 2013; He et al., 2016; Adelisardou et al., 2021). The carbon module consists of four carbon pools: aboveground carbon, belowground carbon, dead biological carbon (litter carbon), and soil carbon. The calculation formula is as follows:
where
Carbon density data at depths of 0–1 m were obtained from literature and National Science and Technology Infrastructure (Table 1) (Li et al., 2003; Xie et al., 2004; Chuai et al., 2013; Yang et al., 2021).
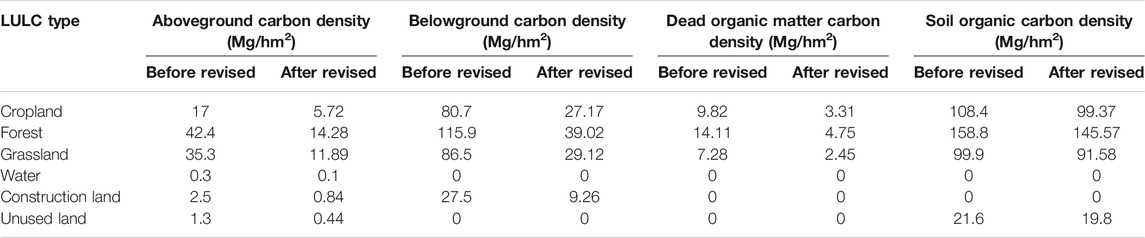
TABLE 1. Carbon density of different LULC type (Yang et al., 2021).
Biomass carbon density (aboveground and belowground carbon) and soil carbon density are closely related to air temperature and precipitation (Raich and Nadelhoffer, 1989). Therefore, we use the MAAT and mean annual precipitation (MAP) during 1980–2019 in Heilongjiang Province and in China to revise the carbon density. The revised formula is as follows (Giardina and Ryan, 2000; Chen et al., 2007; Alam et al., 2013; Yang et al., 2021):
where
Results
Changes in LULC Types From 1980 to 2015
In Heilongjiang Province, forest was the main LULC type, accounting for 46–49% of the total area (Figure 3, Supplementary Figure S1 and Table 2). It was followed by cropland, accounting for 30–37% of the total area. Grassland and unused land accounted for 6–8% of the total area. The area of water (3%) and construction land (1–2%) were less than 3% of the total area. From 1980 to 2015, the areal extents of forest, grassland, unused land, and water decreased by 1.22 × 104, 0.84 × 104, 1.11 × 104 and 0.05 × 104 km2, respectively. Cropland and construction land increased by 3.14 × 104 and 0.08 × 104 km2, respectively (Tables 2 and 3).
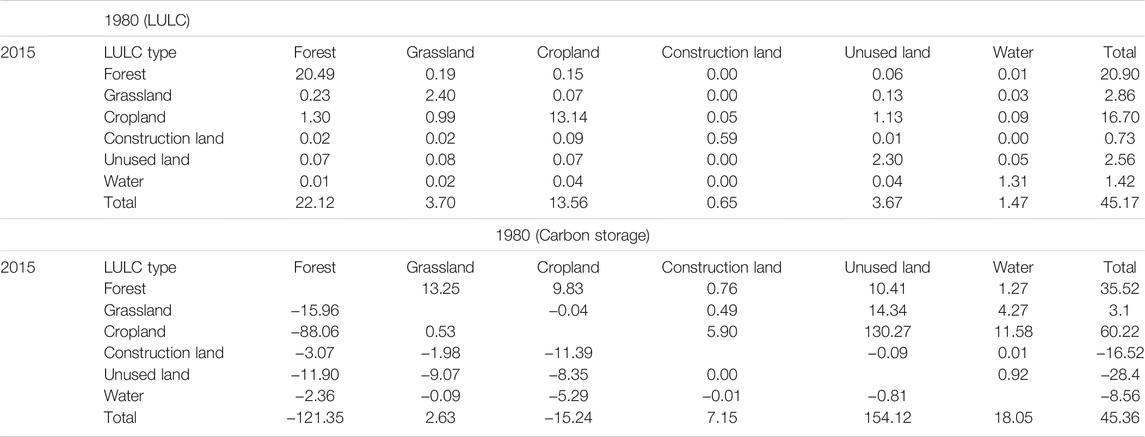
TABLE 2. LULC and carbon storage transfer matrix during 1980–2015 in Heilongjiang Province, Northeast China (104km2, 106 Mg).

TABLE 3. Changes in LULC types during 1980–1990, 1990–1995, 1995–2000, 2000–2005, 2005–2010, 2010–2015 in Heilongjiang Province, Northeast China (104 km2).
From 1980 to 2015 (Table 2), forest was mainly converted to cropland and grassland. Grassland and unused land were mainly converted to cropland. Water was mainly converted to unused land. The increase in cropland was mainly due to the conversion from forest, grassland, and unused land. The increased construction land was mainly due to the conversion from cropland. During 1980 to 2000, the decreasing areas of forest, grassland, and unused land were the largest. The increasing areas of water and cropland were the largest (Table 3).
Changes of Carbon Storage From 1980 to 2015
From Figure 4, Supplementary Figure S2 and Table 4, carbon was mainly stored in forest, cropland, and grassland. Changes in LULC have led to increases or decreases in carbon storage of different LULC types. From 1980 to 2015, the carbon storage of forest, grassland, and unused land decreased gradually, while that of cropland and construction land increased gradually. The total carbon storage of Heilongjiang Province increased from 1980 to 2015, with the lowest in 1980 and the highest in 1995.

TABLE 4. Carbon storage in different LULC type from 1980 to 2015 in Heilongjiang Province, Northeast China (×106 Mg).
From 1980 to 2015, the total carbon storage of Heilongjiang Province increased by 45.36 × 106 Mg C (Table 2), since the conversion of large areas of forest, grassland, and unused land to cropland. Due to the low carbon density of the unused land, the conversion to cropland facilitated the formation of carbon sinks and thus increases carbon storage. However, the change of carbon storage varied with changes in different LULC types: the carbon storage in forest and cropland types decreased after transformation, and those of grassland, water, construction land, and unused land increased (Table 2).
Discussions
Impacts on the LULC Types
There are many driving factors of LULC changes, which can be divided into anthropogenic and natural factors. With rising global temperatures, the belt of boreal forest is moving northward, and temperate forests would continue to migrate closer or into the positions of current boreal forests, and possibly at an unprecedented moving rate (Leithead et al., 2010). Then, the coniferous forest would transfer to broadleaf forest, shrubs, grassland, or even wetlands. Due to its marginal location at the southern edge of the boreal forest, the LULC in Northeast China are more sensitive to climate warming (Serban et al., 2021). Moreover, Northeast China underwent the largest increase in air temperature and a significant decline in summer-autumn precipitation (Zhang et al., 2020; Li X. et al., 2021). In Heilongjiang Province, Northeast China, persistent climate warming was recorded from 1961 to 2019, especially after 1988 (Figure 2). The MAAT during 1988–2019 was 1.01°C higher than that during 1961–1987, and; it rose by 1.06°C from 1961 to 2019 at an average rate of 0.25°C/10a. However, the average rising rate of global MAAT was 0.12°C/10a from 1951 to 2012 (Zhang et al., 2018). Under a climate change, the permafrost in northeast China was undergoing large-scale degradation and the southern limit of permafrost was moving northward significantly (Li X. et al., 2021). And compared with that in the 1960s, the extent of Xing’an permafrost in Northeast China had decreased by 40.6% by the 2010s (Li X. et al., 2021). And the permafrost degradation had caused large areas of coniferous forest moved northward significantly (Jin et al., 2007). Consequently, the pronounced shrub expansion was occurring in the circumpolar Arctic tundra driven by climate warming and permafrost degradation (Myers-Smith et al., 2011).
The increases in cropland and construction land were mainly concentrated around roads and major settlements. Thus, the main causes for unused land, grassland, and forest disturbances were deforestation and clearing for agricultural cultivation, construction of public facilities, resource exploitation, lumbering, airport construction, and others. Since 1893, a large number of people emigrated from the south to northeast China, the population grew exponentially and land was reclaimed and cultivated on a large scale. LULC had undergone significant changes, and which of Heilongjiang Province has the most intense (Jiang, 2017). After 1904, the implementation of the open-land reclamation policy and the construction of the Middle-East railway in Heilongjiang Province, as well as the activities of going and working in the countryside and mountainous areas in 1949, resulted in drastic changes to LULC, which mainly focused on the expansion of cropland and the reclamation of grassland and wetland (Cheng and Fang, 2010). For example, in Figure 3, the areas of cropland in Sanjiang Plain and Songnen Plain increased significantly from 1980 to 2015. In Mo’he County in northern Heilongjiang Province, rapid population growth and the demand for residence and factories forced the clearance of natural vegetation, resulting in the expansion of urbanization area and increase of road from 1985 to 2020 (Figures 5A–F). Changes of LULC types were concentrated in the Hola Basin for coal mining, airport construction, and along the roads in the south for transporting coal after 2005 (Figures 5A–F); those all contributed to the rapid expansion of the anthropic class (Serban et al., 2021). From Table 3, compared with that during 1980–1990, 1990–1995 and 1995–2000, the forest decreased and cropland increased significantly during 2000–2005, 2005–2010 and 2010–2015, and the grassland conversion area was 0 km2 during 2000–2005. This may be explained by the implementation of policy of returning the cultivated croplands to forest and grasslands in 2003.
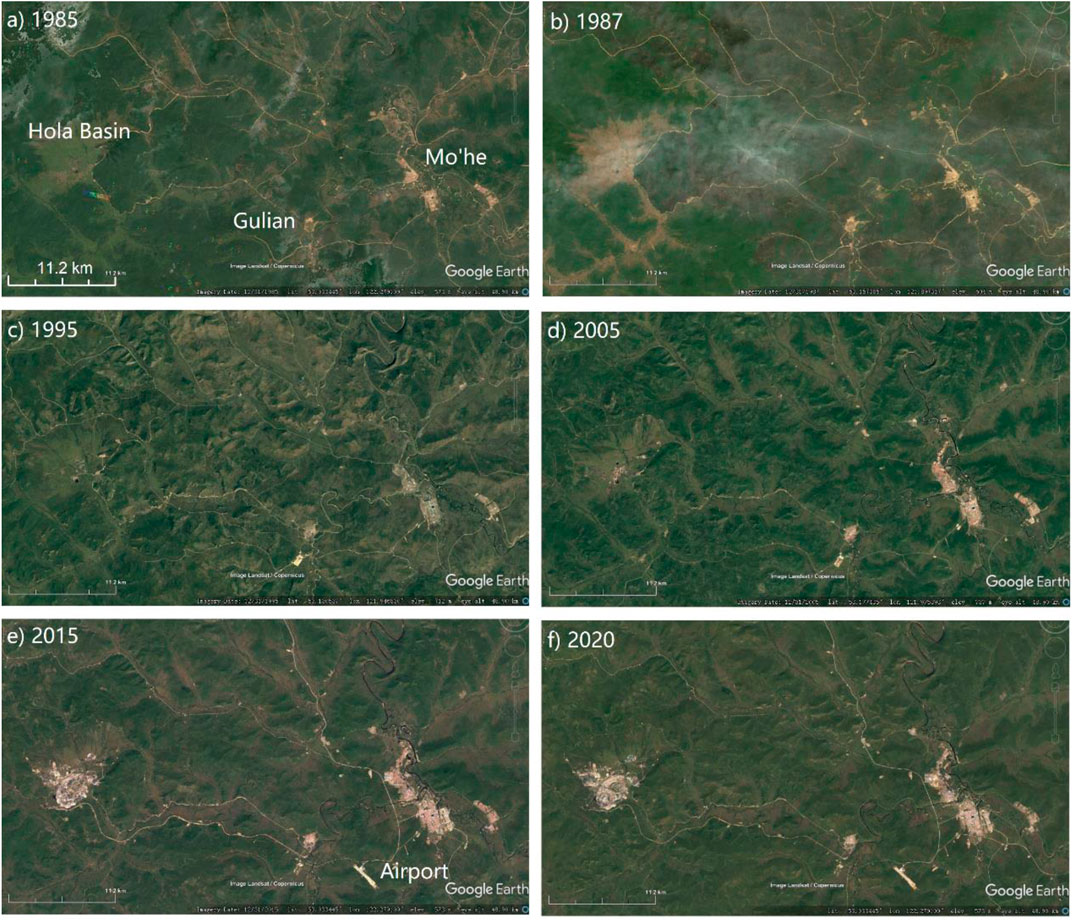
FIGURE 5. Landscape changes in Mo’he, Gulian and Hola basins in Mo’he County in northern Heilongjiang Province, Northeast China from 1985 to 2020. Panel (A–F) is the expansion of Mo’he and Gulian towns, panel (A–D) is the expansion of coal mines at Hola and basins and roads, panel (E,F) represents the construction of airport, and panel (B) represents the forest fire in 1987.
Wildfires is also an important driver for changes in the LULC types. Under a warming climate and human activities, the fire frequency, magnitude, and severity gradually increase, influencing the ecosystem. Importantly, wildfires were caused not only by climatic factors, but also by Anthropocene changes in landscape (Bowman et al., 2020). In the western United States, sedimentary charcoal records showed a primary control of fire activity by temperature and drought over the last 3,000 years (Marlon et al., 2012). Moreover, the effect of climate change is becoming apparent in the increasing numbers of extreme fire events (Cruz et al., 2012; Bowman et al., 2017; Ndalila et al., 2018). Such as in Australia, a globally anomalous fire season in 2019–2020 burned over 5×106 hm2 of Eucalyptus forests (Boer et al., 2020). The wildfire occurred in the northern Da Xing’anling (Hinggan) Mountains, Northeast China, burned more than 1 × 106 hm2 of forests on 6 May-2 June 1987 (Li X. et al., 2021). Wildfires could result in an irreversible permafrost degradation, successions of vegetation, rapid losses of soil carbon, and formation and development of thermokarst (Cong et al., 2020; Li X. et al., 2021). After wildfires, ground temperatures and active layer thickness increase; the release of soil carbon enhances, and; vegetation changes from coniferous forests to broad-leaved forests, shrublands or grasslands. It may take decades or even centuries for the fire-disturbed ecosystems and permafrost environment to return to pre-fire conditions (Holloway et al., 2020; Li X. et al., 2021). For example, in Figure 5B, the black and brown areas showed the burned area causing by the fire in 1987, which resulted in extensive vegetation destruction. The high fire frequency and severity could cause the conversion of coniferous forest to meadow, shrub, deciduous broadleaf forest, or tundra, and expansion of shrubs across the Arctic tundra, it may even take hundreds of years to recover to the pre-fire conditions (Li X. et al., 2021; Chen et al., 2021).
Changes in LULC and Effects on Carbon Storage
Under a warming climate, more severe and frequent boreal forest fires had driven and shifted boreal ecosystem into a positive net carbon balance (from a net carbon sink to a net carbon source over a fire cycle), resulting in a positive climate feedback (Bond-Lamberty et al., 2007; Walker et al., 2019). It is mainly manifested in increasing emissions of CO2 directly through the combustion of forest biomass (LULC changes) and soil carbon stocks (e.g., wetlands and peatlands) and emissions of CH4 and N2O indirectly through thawing of permafrost and changing thermokarst hydrology (O'Connor et al., 2010; Gibson et al., 2018; Loranty et al., 2018; Walker et al., 2019; Bowman et al., 2020). Boreal forests store 30–40% of terrestrial carbon; 70–80% of which is stored in organic soil, and; consequently, this shift from a net carbon sink to a carbon source could impact the global carbon cycle (Lorenz and Lal, 2010). In the northern permafrost region, organic soils (peatlands) and cryoturbated permafrost-affected mineral soils have the highest mean soil organic carbon contents (32.2–69.6 kg/m2) (Tarnocai et al., 2009).
The change of LULC after fire (removal of vegetation and organic layer) increases exposure to solar radiation, result in the diminishing or even disappearance of thermal insulation and reducing of surface albedo, while the ground heat influx increases (Nossov et al., 2013; Holloway et al., 2020). Furthermore, because of heat accumulation, permafrost degrades rapidly in the form of deepening active layer and rising soil temperature (Brown et al., 2015; Li X. et al., 2021). In addition to forest fires and climate warming directly induced soil carbon emissions, permafrost degradation can alter microbial activity and result in more carbon being released into the atmosphere in the form of CH4 and CO2 effluxes that contribute to further warming (Loranty et al., 2018). The thawing of ice-rich permafrost could lead to the occurrence of thermokarst phenomenon, such as thermokarst lakes and ponds, surface subsidence, and thaw slumps (Holloway et al., 2020). Thermokarst development can lead to landscape wetting by promoting the transition from permafrost plateau forest to wetlands (Baltzer et al., 2014). Thermokarst lakes in permafrost regions are sources of the strong CH4 emissions and their expansion may have significantly contributed to the rise of the atmospheric CH4 concentrations (van Huissteden et al., 2011).
Heilongjiang Province is located at the southern edge of boreal forest and Eurasian permafrost, with forest area accounting for 46.3–49.0% of the province area from 1980 to 2015, and permafrost area accounting for 21.6% of the province area (Figure 1). The research at the Xiao Xing’anling Mountains in Heilongjiang Province, Northeast China, also showed the rapidly degrading permafrost under marsh-wetlands since 2004. The organic matter and CH4 stored in the permafrost soil were gradually released to the atmosphere (Shan et al., 2020). The studies in Northeast China show that forest fire and permafrost degradation not only lead to the change of LULC, but also to the reduction of carbon storage (Li et al., 2020b; Li et al., 2022). Furthermore, in Northeast China, a more rapid permafrost degradation has been occurring under the expanding urban regions and engineering infrastructures, such as the Mo’he County and airport and in the right-of-way along the China-Russia Crude Oil Pipeline (Li et al., 2018; Mao et al., 2019). Under a warming climate and thermal disturbances of airport construction and operation, the warming rate of permafrost at depth of 10 m reached by 0.03–0.15°C/a; the permafrost table lowered by 7.5 m from depths of 4.5–12 m in ten year (Mao et al., 2019). Due to the construction of the China-Russia Crude Oil Pipeline and the removal of vegetation, active layer thickness increased by 2.7 m and permafrost temperature at depth of 15–20 m rose by 0.2°C from 2014 to 2017 (Li et al., 2018). Under the influence of urbanization, the area of construction land in Mo’he County increased by 39.3% in the last decade. From the edge of the urban area to the center of Mo’he County, ground temperature increased from −0.9 to 3.0°C at the depth of 15 m and the permafrost table lowered from 6.1 to 22.3 m (Lu, 2018). In Northeast China, a severe burn led to a rising of soil temperatures (2.3°C) and substantial reduction of soil carbon (67.5%) in the active layer, which were not recovered seven years after forest fire (Li et al., 2020b).
Therefore, under the combined effects of climate warming, urbanization expansion, high frequency forest fires, rapid permafrost degradation, and engineering construction, significant changes in LULC and soil carbon storage have occurred in Heilongjiang Province, Northeast China.
Conclusions
The changes of LULC types and soil carbon storages were analyzed based on InVEST modeling from 1980 to 2015 in Heilongjiang Province, Northeast China, with the following conclusions:
Forest and cropland are the dominant LULC types in Heilongjiang Province, Northeast China, accounting for 46%-49%and 30–37% of the total area. From 1980 to 2015, forest, grassland, unused land, and water decreased by 1.22 × 104, 0.84 × 104, 1.11 × 104, and 0.05 × 104 km2, respectively. Cropland and construction land increased by 3.14 × 104 and 0.08 × 104 km2, respectively. This was due to the conversion of forest to cropland and grassland, that of grassland and unused land to cropland, and that of water surfaces to unused land from 1980 to 2015. The changes of LULC types were the most obviously during 1980–2000. The carbon storage of forest, grassland, and unused land decreased gradually by 236.22, 116.61 and 21.82 × 106 Mg C, respectively, while those of cropland and construction land increased gradually by 414.65 and 0.99 × 106 Mg C, respectively. From 1980 to 2015, due to the conversion of large areas of forest, grassland, and unused land to cropland. The unused land has a low carbon density, and the conversion of the unused land to cropland facilitated the formation of carbon sinks and thus increased carbon storage. Therefore, the total carbon storage of Heilongjiang Province increased by 45.36 × 106 Mg C from 1980 to 2015.
Under a warming climate and human activities, such as frequent wildfires, urban expansion, farmland reclamation, and engineering construction had led to changes in LULC, accelerating permafrost degradation. Under the combined action of these factors, soil carbon storages were significantly changed. Forest and grassland areas and their carbon storage decreased obviously, even though the total carbon storage of Heilongjiang Province increased. However, forest and grassland are important component of ecosystem service function and carbon pools in Northeast China. Therefore, evaluation of ecosystem carbon storage in boreal forest and permafrost regions are of great significance for decision-making in ecosystem service, ecological environmental protection and sustainable development.
Data Availability Statement
The original contributions presented in the study are included in the article/Supplementary Material, further inquiries can be directed to the corresponding authors.
Author Contributions
XL and HJ contributed to the conceptualization and writing of the manuscript. XL, LS and CH contributed to the theory and methodology. CH, HC, TH, GY, HY, YH, SK and JL contributed to the resources and field data collection and data compilation. All authors contributed to the article and approved the submitted version.
Funding
This research is funded by the Natural Science Foundation of China (Grant No. 42001052); Startup Fund of Northeast Forestry University for Chengdong Outstanding Youth Scholarship (YQ 2020-10); the State Key Laboratory of Frozen Soils Engineering Open Fund (Grant No. SKLFSE202008), and; Fundamental Research Fund for the Central Universities (Grant No. 2572021BA03).
Conflict of Interest
The authors declare that the research was conducted in the absence of any commercial or financial relationships that could be construed as a potential conflict of interest.
Publisher’s Note
All claims expressed in this article are solely those of the authors and do not necessarily represent those of their affiliated organizations, or those of the publisher, the editors and the reviewers. Any product that may be evaluated in this article, or claim that may be made by its manufacturer, is not guaranteed or endorsed by the publisher.
Supplementary Material
The Supplementary Material for this article can be found online at: https://www.frontiersin.org/articles/10.3389/feart.2022.846456/full#supplementary-material
References
Adelisardou, F., Zhao, W., Chow, R., Mederly, P., Minkina, T., and Schou, J. S. (2021). Spatiotemporal Change Detection of Carbon Storage and Sequestration in an Arid Ecosystem by Integrating Google Earth Engine and InVEST (The Jiroft plain, Iran). Int. J. Environ. Sci. Technol. 71, 1279–1290. doi:10.1007/s13762-021-03676-6
Alam, S. A., Starr, M., and Clark, B. J. F. (2013). Tree Biomass and Soil Organic Carbon Densities across the Sudanese woodland savannah: A Regional Carbon Sequestration Study. J. Arid Environments 89, 67–76. doi:10.1016/j.jaridenv.2012.10.002
Baltzer, J. L., Veness, T., Chasmer, L. E., Sniderhan, A. E., and Quinton, W. L. (2014). Forests on Thawing Permafrost: Fragmentation, Edge Effects, and Net forest Loss. Glob. Change Biol. 20, 824–834. doi:10.1111/gcb.12349
Boer, M. M., Resco de Dios, V., and Bradstock, R. A. (2020). Unprecedented Burn Area of Australian Mega forest Fires. Nat. Clim. Chang. 10, 171–172. doi:10.1038/s41558-020-0716-1
Bond-Lamberty, B., Peckham, S. D., Ahl, D. E., and Gower, S. T. (2007). Fire as the Dominant Driver of central Canadian Boreal forest Carbon Balance. Nature 450 (7166), 89–92. doi:10.1038/nature06272
Bowman, D. M. J. S., Kolden, C. A., Abatzoglou, J. T., Johnston, F. H., van der Werf, G. R., and Flannigan, M. (2020). Vegetation Fires in the Anthropocene. Nat. Rev. Earth Environ. 1, 500–515. doi:10.1038/s43017-020-0085-3
Bowman, D. M. J. S., Williamson, G. J., Abatzoglou, J. T., Kolden, C. A., Cochrane, M. A., and Smith, A. M. S. (2017). Human Exposure and Sensitivity to Globally Extreme Wildfire Events. Nat. Ecol. Evol. 1, 58. doi:10.1038/s41559-016-0058
Brown, D. R. N., Jorgenson, M. T., Douglas, T. A., Romanovsky, V. E., Kielland, K., Hiemstra, C., et al. (2015). Interactive Effects of Wildfire and Climate on Permafrost Degradation in Alaskan lowland Forests. J. Geophys. Res. Biogeosci. 120, 1619–1637. doi:10.1002/2015jg003033
Chen, G., and Tian, H. (2007). Land Use/cover Change Effects on Carbon Cycling in Terrestrial Ecosystems. Chin. J. Plant Ecol. 31, 189–204 [in Chinese]. doi:10.1111/gcb.15069
Chen, G., Yang, Y., Liu, L., Li, X., Zhao, Y., and Yuan, Y. (2007). Research Review on Total Belowground Carbon Allocation in Forest Ecosystems. J. Subtrop. Res. Environ. 2, 34–42 [in Chinese]. doi:10.19687/j.cnki.1673-7105.2007.01.005
Chen, Y., Hu, F. S., and Lara, M. J. (2021). Divergent Shrub‐cover Responses Driven by Climate, Wildfire, and Permafrost Interactions in Arctic Tundra Ecosystems. Glob. Change Biol. 27, 652–663. doi:10.1111/gcb.15451
Cheng, Y., and Fang, Y. (2010). Morphology Change and its Influencing Factors of Agricultural Terrain Structure in Northeast China. Econ. Geogr. 30 (8), 1349–1353. doi:10.15957/j.cnki.jjdl.2010.08.017
Chuai, X., Huang, X., Lai, L., Wang, W., Peng, J., and Zhao, R. (2013). Land Use Structure Optimization Based on Carbon Storage in Several Regional Terrestrial Ecosystems across China. Environ. Sci. Pol. 25, 50–61. doi:10.1016/j.envsci.2012.05.005
Cong, J., Gao, C., Han, D., Li, Y., and Wang, G. (2020). Stability of the Permafrost Peatlands Carbon Pool under Climate Change and Wildfires during the Last 150 Years in the Northern Great Khingan Mountains, China. Sci. Total Environ. 712, 136476. doi:10.1016/j.scitotenv.2019.136476
Cruz, M. G., Sullivan, A. L., Gould, J. S., Sims, N. C., Bannister, A. J., Hollis, J. J., et al. (2012). Anatomy of a Catastrophic Wildfire: The Black Saturday Kilmore East Fire in Victoria, Australia. For. Ecol. Manag. 284, 269–285. doi:10.1016/j.foreco.2012.02.035
Dida, J. J. V., Tiburan, C. L., Tsutsumida, N., and Saizen, I. (2021). Carbon Stock Estimation of Selected Watersheds in Laguna, Philippines Using InVEST. Philippine J. Sci. 150, 501–513.
Giardina, C. P., and Ryan, M. G. (2000). Evidence that Decomposition Rates of Organic Carbon in mineral Soil Do Not Vary with Temperature. Nature 404, 858–861. doi:10.1038/35009076
Gibson, C. M., Chasmer, L. E., Thompson, D. K., Quinton, W. L., Flannigan, M. D., and Olefeldt, D. (2018). Wildfire as a Major Driver of Recent Permafrost Thaw in Boreal Peatlands. Nat. Commun. 9, 3041. doi:10.1038/s41467-018-05457-1
Guo, S. (2011). Analysis on Carbon Stock and Potential Carbon Sequestration in Heilongjiang Province. For. Eng. 27 (3), 9–16. doi:10.16270/j.cnki.slgc.2011.03.022
He, C., Zhang, D., Huang, Q., and Zhao, Y. (2016). Assessing the Potential Impacts of Urban Expansion on Regional Carbon Storage by Linking the LUSD-Urban and InVEST Models. Environ. Model. Softw. 75, 44–58. doi:10.1016/j.envsoft.2015.09.015
Holloway, J. E., Lewkowicz, A. G., Douglas, T. A., Li, X., Turetsky, M. R., Baltzer, J. L., et al. (2020). Impact of Wildfire on Permafrost Landscapes: A Review of Recent Advances and Future Prospects. Permafrost and Periglac Process 31, 371–382. doi:10.1002/ppp.2048
Houghton, R. A. (2003). Revised Estimates of the Annual Net Flux of Carbon to the Atmosphere from Changes in Land Use and Land Management 1850-2000. Tellus B 55, 378–390. doi:10.1034/j.1600-0889.2003.01450.x
Jiang, L. (2017). Effect and Mechanism of Land Cover Change on Climate Change in Heilongjiang Province during 1900s-2010s. Doctoral Dissertation. Heilongjing (China): Harbin Normal University.
Jin, H., Yu, Q., Lü, L., Guo, D., He, R., Yu, S., et al. (2007). Degradation of Permafrost in the Xing'anling Mountains, Northeastern China. Permafrost Periglac. Process. 18, 245–258. doi:10.1002/ppp.589
Leithead, M. D., Anand, M., and Silva, L. C. R. (2010). Northward Migrating Trees Establish in Treefall Gaps at the Northern Limit of the Temperate-Boreal Ecotone, Ontario, Canada. Oecologia 164, 1095–1106. doi:10.1007/s00442-010-1769-z
Li, G., Wang, F., Ma, W., Fortier, R., Mu, Y., Zhou, Z., et al. (2018). Field Observations of Cooling Performance of Thermosyphons on Permafrost under the China-Russia Crude Oil Pipeline. Appl. Therm. Eng. 141, 688–696. doi:10.1016/j.applthermaleng.2018.06.005
Li, K., Wang, S., and Cao, M. (2003). Carbon Storage of Vegetation and Soil in China. Sci. China (Series D). 33, 72–80. doi:10.1002/ecs2.3341
Li, X., Jin, H., Wang, H., Marchenko, S. S., Shan, W., Luo, D., et al. (2021b). Influences of forest Fires on the Permafrost Environment: A Review. Adv. Clim. Change Res. 12, 48–65. doi:10.1016/j.accre.2021.01.001
Li, X., Jin, H., He, R., and Huang, Y. (2020a). Effects of forest Fires on Ecological Service in Permafrost Regions. Clim. Chang. Res. 16, 545–554 [in Chinese]. doi:10.12006/j.issn.1673-1719.2020.019
Li, X., Jin, H., Sun, L., Wang, H., He, R., Huang, Y., et al. (2021a). Climate Warming over 1961-2019 and Impacts on Permafrost Zonation in Northeast China. J. For. Res. doi:10.1007/s11676-021-01403-y
Li, X., Jin, H., Wang, H., Jin, X., Bense, V. F., Marchenko, S. S., et al. (2022). Effects of Fire History on thermal Regimes of Permafrost in the Northern Da Xing'anling Mountains, NE China. Geoderma 410, 115670. doi:10.1016/j.geoderma.2021.115670
Li, X., Jin, H., Wang, H., Wu, X., Huang, Y., He, R., et al. (2020b). Distributive Features of Soil Carbon and Nutrients in Permafrost Regions Affected by forest Fires in Northern Da Xing'anling (Hinggan) Mountains, NE China. Catena 185, 104304. doi:10.1016/j.catena.2019.104304
Liu, S., Hu, N., Zhang, J., and Lv, Z. (2018). Spatiotemporal Change of Carbon Storage in the Loess Plateau of Northern Shaanxi, Based on the InVEST Model. Sci. Cold Arid Reg. 10, 0240–0250. doi:10.3724/SP.J.1226.2018.00240
Loranty, M. M., Abbott, B. W., Blok, D., Douglas, T. A., Epstein, H. E., Forbes, B. C., et al. (2018). Reviews and Syntheses: Changing Ecosystem Influences on Soil thermal Regimes in Northern High-Latitude Permafrost Regions. Biogeosciences 15, 5287–5313. doi:10.5194/bg-15-5287-2018
Lorenz, K., and Lal, R. (2010). “Carbon Dynamics and Pools in Major Forest Biomes of the World”, in Carbon sequestration in Forest Ecosystems (Dordrecht: Springer), 159–205.
Lu, Y. (2018). Study on the Change of Permafrost in Urban Environment: A Case Study of Mohe County. Northwest Institute of Eco-Environmental and Resources Chinese Academy of Sciences, Doctoral thesis. (Lanzhou, China: University of Chinese Academy of Sciences), 1–141 (in Chinese).
Mao, Y., Li, G., Ma, W., Mu, Y., Wang, F., Miao, J., et al. (2019). Field Observation of Permafrost Degradation under Mo'he Airport, Northeastern China from 2007 to 2016. Cold Regions Sci. Tech. 161, 43–50. doi:10.1016/j.coldregions.2019.03.004
Marlon, J. R., Bartlein, P. J., Gavin, D. G., Long, C. J., Anderson, R. S., Briles, C. E., et al. (2012). Long-term Perspective on Wildfires in the Western USA. Proc. Natl. Acad. Sci. 109, E535–E543. doi:10.1073/pnas.1112839109
Myers-Smith, I. H., Forbes, B. C., Wilmking, M., Hallinger, M., Lantz, T., Blok, D., et al. (2011). Shrub Expansion in Tundra Ecosystems: Dynamics, Impacts and Research Priorities. Environ. Res. Lett. 6, 045509. doi:10.1088/1748-9326/6/4/045509
Ndalila, M. N., Williamson, G. J., and Bowman, D. M. J. S. (2018). Geographic Patterns of Fire Severity Following an Extreme Eucalyptus Forest Fire in Southern Australia: 2013 Forcett-Dunalley Fire. Fire 1, 40. doi:10.3390/fire1030040
Nie, X., Lu, B., Chen, Z., Yang, Y., Chen, S., Chen, Z., et al. (2020). Increase or Decrease? Integrating the CLUMondo and InVEST Models to Assess the Impact of the Implementation of the Major Function Oriented Zone Planning on Carbon Storage. Ecol. Indicators 118, 106708. doi:10.1016/j.ecolind.2020.106708
Noble, I. R., Bolin, B., Ravindranath, N. H., Verardo, D. J., and Dokken, D. J. (2000). Land Use, Land Use Change, and Forestry. Environ. Conserv. 28, 284–293.
Nossov, D. R., Torre Jorgenson, M., Kielland, K., and Kanevskiy, M. Z. (2013). Edaphic and Microclimatic Controls over Permafrost Response to Fire in interior Alaska. Environ. Res. Lett. 8, 035013. doi:10.1088/1748-9326/8/3/035013
O'Connor, F. M., Boucher, O., Gedney, N., Jones, C. D., Folberth, G. A., Coppell, R., et al. (2010). Possible Role of Wetlands, Permafrost, and Methane Hydrates in the Methane Cycle under Future Climate Change: A Review. Rev. Geophys. 48, RG4005. doi:10.1029/2010rg000326
Ouyang, Z., Zheng, H., Xiao, Y., Polasky, S., Liu, J., Xu, W., et al. (2016). Improvements in Ecosystem Services from Investments in Natural Capital. Science 352, 1455–1459. doi:10.1126/science.aaf2295
Raich, J. W., and Nadelhoffer, K. J. (1989). Belowground Carbon Allocation in forest Ecosystems: Global Trends. Ecology 70, 1346–1354. doi:10.2307/1938194
Serban, R.-D., Serban, M., He, R., Jin, H., Li, Y., Li, X., et al. (2021). 46-Year (1973-2019) Permafrost Landscape Changes in the Hola Basin, Northeast China Using Machine Learning and Object-Oriented Classification. Remote Sensing 13, 1910. doi:10.3390/rs13101910
Shan, W., Xu, Z., Guo, Y., Zhang, C., Hu, Z., and Wang, Y. (2020). Geological Methane Emissions and Wildfire Risk in the Degraded Permafrost Area of the Xiao Xing'an Mountains, China. Sci. Rep. 10, 21297. doi:10.1038/s41598-020-78170-z
Tallis, H. T., Ricketts, T., Guerry, A. D., Wood, S. A., Sharp, R., Nelson, E., et al. (2013). Capital Project: Stanford. InVEST 2.5.6 User’s Guide. Stanford, CA, USA: Nat. Cap. Proj.
Tarnocai, C., Canadell, J. G., Schuur, E. A. G., Kuhry, P., Mazhitova, G., and Zimov, S. (2009). Soil Organic Carbon Pools in the Northern Circumpolar Permafrost Region. Glob. Biogeochem. Cycles 23, GB2023. doi:10.1029/2008gb003327
Tolessa, T., Senbeta, F., and Kidane, M. (2017). The Impact of Land Use/land Cover Change on Ecosystem Services in the central highlands of Ethiopia. Ecosystem Serv. 23, 47–54. doi:10.1016/j.ecoser.2016.11.010
Toru, T., and Kibret, K. (2019). Carbon Stock under Major Land Use/land Cover Types of Hades Sub-watershed, Eastern Ethiopia. Carbon Balance Manage 14, 7. doi:10.1186/s13021-019-0122-z
van Huissteden, J., Berrittella, C., Parmentier, F. J. W., Mi, Y., Maximov, T. C., and Dolman, A. J. (2011). Methane Emissions from Permafrost Thaw Lakes Limited by lake Drainage. Nat. Clim Change 1, 119–123. doi:10.1038/nclimate1101
Viana, C. M., Oliveira, S., Oliveira, S. C., and Rocha, J. (2019). Land Use/land Cover Change Detection and Urban Sprawl Analysis. Spat. Model. GIS R Earth Environ. Sci. 2019, 621–651. doi:10.1016/b978-0-12-815226-3.00029-6
Walker, X. J., Baltzer, J. L., Cumming, S. G., Day, N. J., Ebert, C., Goetz, S., et al. (2019). Increasing Wildfires Threaten Historic Carbon Sink of Boreal forest Soils. Nature 572, 520–523. doi:10.1038/s41586-019-1474-y
Wang, X., Feng, Z., and Ouyang, Z. (2001). Vegetation Carbon Storage and Density of Forest Ecosystems in China. Chin. J. Appl. Ecol. 12 (1), 13–16 [in Chinese]. doi:10.13287/j.1001-9332.2001.0003
Wang, X., Li, Y., Gong, X., Niu, Y., Chen, Y., Shi, X., et al. (2019). Storage, Pattern and Driving Factors of Soil Organic Carbon in an Ecologically Fragile Zone of Northern China. Geoderma 343, 155–165. doi:10.1016/j.geoderma.2019.02.030
Xie, G., Zhang, C., Zhen, L., and Zhang, L. (2017). Dynamic Changes in the Value of China's Ecosystem Services. Ecosystem Serv. 26, 146–154. doi:10.1016/j.ecoser.2017.06.010
Xie, X., Sun, B., Zhou, H., Li, Z., and Li, A. (2004). Organic Carbon Density and Storage in Soils of China and Spatial Analysis. Acta Pedologica Sin 41, 35–43 [in Chinese]. doi:10.11766/trxb200301140106
Yang, J., Xie, B., and Zhang, D. (2021). Spatio-temporal Evolution of Carbon Stocks in the Yellow River Basin Based on InVEST and CA-Markov Models. Chin. J. Eco-agric. 29, 1018–1029 [in Chinese]. doi:10.13930/j.cnki.cjea.200746
Zhang, X., Liu, X., Wang, W., Zhang, T., Zeng, X., Xu, G., et al. (2018). Spatiotemporal Variability of Drought in the Northern Part of Northeast China. Hydrological Process. 32, 1449–1460. doi:10.1002/hyp.11503
Zhang, Y., Liang, S., and Xiao, Z. (2020). Observed Vegetation Greening and its Relationships with Cropland Changes and Climate in China. Land 9, 274. doi:10.3390/land9080274
Keywords: carbon storage, LULC types, InVEST model, spatio-temporal changes, permafrost degradation, ecosystem services
Citation: Li X, Huang C, Jin H, Han Y, Kang S, Liu J, Cai H, Hu T, Yang G, Yu H and Sun L (2022) Spatio-Temporal Patterns of Carbon Storage Derived Using the InVEST Model in Heilongjiang Province, Northeast China. Front. Earth Sci. 10:846456. doi: 10.3389/feart.2022.846456
Received: 31 December 2021; Accepted: 02 March 2022;
Published: 24 March 2022.
Edited by:
Sizhong Yang, GFZ German Research Centre for Geosciences, GermanyReviewed by:
Shuying Zang, Harbin Normal University, ChinaChuanyu Gao, Northeast Institute of Geography and Agroecology (CAS), China
Copyright © 2022 Li, Huang, Jin, Han, Kang, Liu, Cai, Hu, Yang, Yu and Sun. This is an open-access article distributed under the terms of the Creative Commons Attribution License (CC BY). The use, distribution or reproduction in other forums is permitted, provided the original author(s) and the copyright owner(s) are credited and that the original publication in this journal is cited, in accordance with accepted academic practice. No use, distribution or reproduction is permitted which does not comply with these terms.
*Correspondence: Xiaoying Li, lixiaoying@nefu.edu.cn; Long Sun, sunlong365@126.com