- 1Research Group for Terrestrial Palaeoclimates, Max Planck Institute for Chemistry, Mainz, Germany
- 2Department of Geosciences, University of Tübingen, Tübingen, Germany
- 3Faculty of Environmental Sciences and Engineering, Babes Bolyai University, Cluj-Napoca, Romania
- 4Interdisciplinary Research Institute on Bionanoscience, Babes Bolyai University, Cluj-Napoca, Romania
- 5Instituto de Geociências e Ciências Exatas, Universidade Estadual Paulista (UNESP), Rio Claro, Brazil
- 6Department of Geology, Tajik National University, Dushanbe, Tajikistan
Trapped charge characteristics in quartz are of increasing interest for their utility as indicators of sediment provenance. These include sensitivity of optically stimulated luminescence (OSL) and thermoluminescence (TL) signal and paramagnetic E1’ defect centre in quartz. Up until now, these methods have largely been used independently in provenance investigations, especially in aeolian systems. Variations in quartz OSL and TL signal sensitivity in loess-palaeosol sequences has been linked to shifts in sediment source corresponding to climatic fluctuations. However, the processes responsible for observed variation in OSL and TL sensitivity in quartz, including its link to the original rock source and/or its sedimentary history, remains a topic of contention. On the other hand, the intensity of E1’ defect centre in quartz is known to reflect the provenance of quartz, and is also routinely utilised as a provenance indicator in aeolian studies. In this study we aim to understand the variations in E1’ intensity and OSL (and TL) sensitivity by direct comparison of these characteristics in fine-grained quartz from a c. 60 m-thick loess-palaeosol sequence in Tajikistan. In doing so, we investigate the natural processes that may have led to variability in trapped-charge characteristics over the c. 500 ky timespan covered by the sequence. Our results suggest that apart from the inherent link to its provenance signature, the observed luminescence sensitivity of quartz especially in loess-palaeosol sequences are largely influenced by depositional and post-depositional processes during soil formation.
Introduction
Deposits of aeolian dust, or loess, have long been recognised as excellent terrestrial archives of past environmental change (Liu, 1985; Kukla et al., 1988; Schaetzl et al., 2018). These deposits form stacked sequences of primary loess and buried soils—commonly known as loess-palaeosol sequences - that largely reflect colder, drier, more windy phases, and more humid, warmer, less windy periods respectively (Marković et al., 2015). Whilst in situ evidence of past climatic conditions can be extracted from the physical and chemical characteristics of loess sediments (e.g., Fitzsimmons et al., 2012 and references therein), identifying the source of loess—its provenance—is equally valuable for elucidating dust transport pathways, thereby providing insights into past wind dynamics and atmospheric circulation in a region (Ding et al., 2000; Muhs et al., 2008). A number of provenance techniques have been applied to distinguish source change in loess, including various isotopic (Sr, Nd; Smith et al., 2003; Rao et al., 2006) and geochemical (major and trace elements; Sun, 2002; Buggle et al., 2008) approaches. However, these methods are derived from bulk sediments, which can lead to indeterminate results (Újvári et al., 2012). The limitations of radiogenic and elemental approaches in loess provenance studies have led to an increased interest in targeting specific minerals, such as zircon (Stevens et al., 2010; Fenn et al., 2018) and quartz (Nagashima et al., 2007; Sun et al., 2008). Provenance techniques based on detrital zircon U-Pb ages in loess are, however, often limited by the low concentrations of zircon grains (Fenn et al., 2018). By contrast, quartz is ubiquitous, and its resistance to weathering provides an excellent material for provenance analysis in loess sediments.
In recent years, lattice defects and impurities in quartz have been increasingly investigated as potential indicators of sedimentary history and transport processes (Pietsch et al., 2008; Fitzsimmons, 2011; Sawakuchi et al., 2012) and provenance (Nagashima et al., 2007; Toyoda et al., 2016; Nagashima et al., 2017; Sawakuchi et al., 2018). A range of characteristics are exploited for this purpose. These involve various components of the optically stimulated luminescence (OSL) and thermoluminescence (TL) signals (Tsukamoto et al., 2011; Jeong and Choi, 2012), including its sensitivity characteristics (Zheng et al., 2009; Lü and Sun, 2011; Sawakuchi et al., 2012; Zular et al., 2015), or specific intrinsic or impurity-related defect centres in quartz such as E1’, Al-hole and Ti-Li centres using electron spin resonance (ESR; Nagashima et al., 2007; Shimada et al., 2013; Tissoux et al., 2015; Wei et al., 2020).
Luminescence sensitivity, conventionally defined as the luminescence emitted per unit dose per unit mass of the sample, is an intrinsic property of the quartz crystal lattice. The luminescence sensitivity of quartz is often linked to 1) the thermal history of the quartz-bearing source rocks, which forms the basis of its use as a provenance indicator (Fitzsimmons, 2011; Sawakuchi et al., 2011); and 2) the sedimentary history of the grain, whereby quartz is sensitised by repeated processes of erosion, transport, storage and burial (Pietsch et al., 2008; Fitzsimmons et al., 2010). As such, the technique shows promise for application in long profiles of aeolian loess. Recent investigations of quartz OSL and TL sensitivity down loess profiles in Tajikistan (Li and Zhou, 2021), as well as from the Chinese loess plateau (CLP; Lü et al., 2014; Lü et al., 2021) yield distinctions in the sensitivity of quartz between loess and palaeosol units. The variability observed within individual profiles has been attributed to the impact of climatic fluctuations on geomorphic processes, such as the expansion and contraction of nearby deserts leading to sensitivity change related to transport distance from the source (deserts) to the sink (loess) (Lü et al., 2014); increased glacial erosion in mountain regions during cold phases leading to increased production of low sensitivity quartz (Lü et al., 2014); and preferential weathering of certain rock types in response to long-term climate fluctuations, thereby leading to availability of quartz of different origin for loess deposition (Li and Zhou, 2021).
One of the most commonly used ESR-based quartz provenance technique utilises the heat-treated E1’ centre (or HT-E1’ centre) in combination with the crystallinity index of quartz (Nagashima et al., 2007; Toyoda et al., 2016). This method has been applied to distinguish between quartz from different inland Asian desert sources (Sun et al., 2007; Sun et al., 2008) and ocean sediment cores (Nagashima et al., 2011; Wang et al., 2020). The use of HT-E1’ centre as a provenance indicator is based on the premise that HT-E1’ reflects the total number of oxygen vacancies (Si=Si) in a quartz crystal and increases with rock age, a quantity which is characteristic of the source rock (Toyoda and Hattori, 2000; Toyoda et al., 2016). In a recent study (Dave et al., 2022), an alternate provenance technique is proposed based on the measurement of natural (not heat-treated) intensity of E1’ and peroxy defect centres in detrital quartz, both these parameters are known to increase with the age of the host rock (Odom and Rink, 1989). This study was successful in differentiating loess deposits from two different basins in Central Asia, as well as identified temporal variations in provenance in response to climatic fluctuations at a long loess sequence (Karamaidan) in Tajikistan.
Ultimately, the aim of sediment provenance analysis is to identify the parent rock from which sediments are derived. However, the process of sediment cycling is complicated by multiple factors that affect the fate of the material from source to sink (Weltje and von Eynatten, 2004). With this in mind, one of the first considerations for the application of a provenance technique lies in its sensitivity to detect changes in sediment composition, and therefore source, at a site. In this study, we compare two different approaches based on trapped charge within fine-grained aeolian quartz which appear to reflect different aspects of the sediment cycling process. The natural E1’ signal from quartz measures a single defect type and largely reflects the signature of the host rock (through its age: Odom and Rink, 1989; Dave et al., 2022). By contrast, the OSL and TL signals are more complex and may reflect either the signature of the parent rock (through its thermal history: Fitzsimmons, 2011; Sawakuchi et al., 2011; Sawakuchi et al., 2018), and/or its sedimentary and transport history (Pietsch et al., 2008; Fitzsimmons et al., 2010). Consequently, at any given point in a sedimentary setting, the quartz OSL and TL sensitivity may record the influence of both, and the degree of influence of either depends on which parameters act as a dominating factor at that time (Fitzsimmons, 2011; Lü and Sun, 2011; Sawakuchi et al., 2011). Recent studies based on multi-spectroscopic techniques (Sharma, 2017), as well as laboratory-based experiments on repeated irradiation and bleaching of natural quartz (Bartyik et al., 2021; Li and Zhou, 2021) show that its OSL signal exhibits preferential sensitisation, the magnitude of which may vary depending on the quartz source. Thus, at this stage, understanding the reasons for sensitisation of quartz remain poorly understood. Therefore, a combined approach based on measurement of both the natural E1’ intensity and luminescence sensitivity of the OSL and TL signals, offers an alternative approach to interpret luminescence sensitivity data in loess records, as well as assess the influence of depositional and post-depositional processes in aeolian environments on luminescence sensitivity.
Materials and Methods
Site Setting and Sampling
The site of Karamaidan (KAR; 38° 34′ 33.47″ N, 69° 16′ 40.79″ E, 1,629 m a.s.l.) lies in the foothills of the Gissar Range, on the northern margins of the Afghan-Tajik depression (Figure 1). The Afghan-Tajik depression is a seismically active sedimentary basin, the northern part of which is interrupted by a series of north-south oriented folds and ridges (McNab et al., 2019) that are traversed by a number of rivers arising from the glaciated Alai, Trans-Alai and Pamir ranges. These drainage systems are tributaries to the Amu Darya River and provides vast glacio-/fluvial outwash plain that acts as local dust source area (Li et al., 2019). The farther west and northwest located, Karakum and Kyzylkum deserts, act as additional dust sources for the Afghan-Tajik depression (Li et al., 2019). Given this setting, the margins of this depression preserve some of the thickest loess deposits in Eurasia, several of which represent quasi-continuous deposition over the last million years (Forster and Heller, 1994; Dodonov and Baiguzina, 1995). The loess deposits at KAR provide one such palaeoclimatic archive, which is known to preserve a record of climate change over the past 1 million years (Ma; Forster and Heller, 1994) and thus, provides an ideal test site to investigate long term variations in dust source and their relationship to changing climatic conditions.
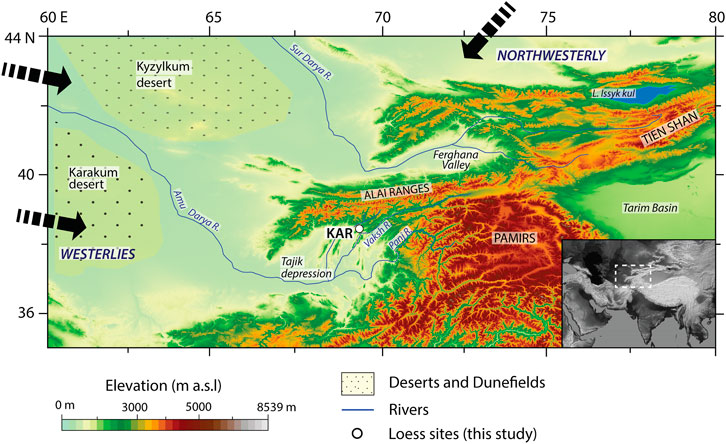
FIGURE 1. Regional setting and location of study site KAR within western Central Asia. The elevation map was created using Shuttle Radar Topography Mission (SRTM) data from AW3D of the Japan Aerospace Exploration Agency.
The KAR profile represents a >100 m thick package of alternating primary loess and buried soils (Forster and Heller, 1994), exposed as a result of a recent earthquake-induced landslide. In this study we investigate a c. 56 m stratigraphic interval from the lower part of the KAR section, comprising of six loess and five palaeosol units, with three additional weak to moderately developed soils observed within the primary loess intervals (Figure 2). The relative chronology of the c. 56 m subsection sampled for this study is described and interpreted to span marine isotope stages (MIS) 9-19 (Dave et al., 2022), based on the correlation of in-situ magnetic susceptibility with the global marine isotope stack (Lisiecki and Raymo, 2005; Figure 2) as well as the previous chronostratigraphy obtained by Forster and Heller (1994) (Supplementary Figure S1). A description of the stratigraphy of the subsection, magnetic susceptibility measurements and relative chronology can be found in the Supplementary Material S1.
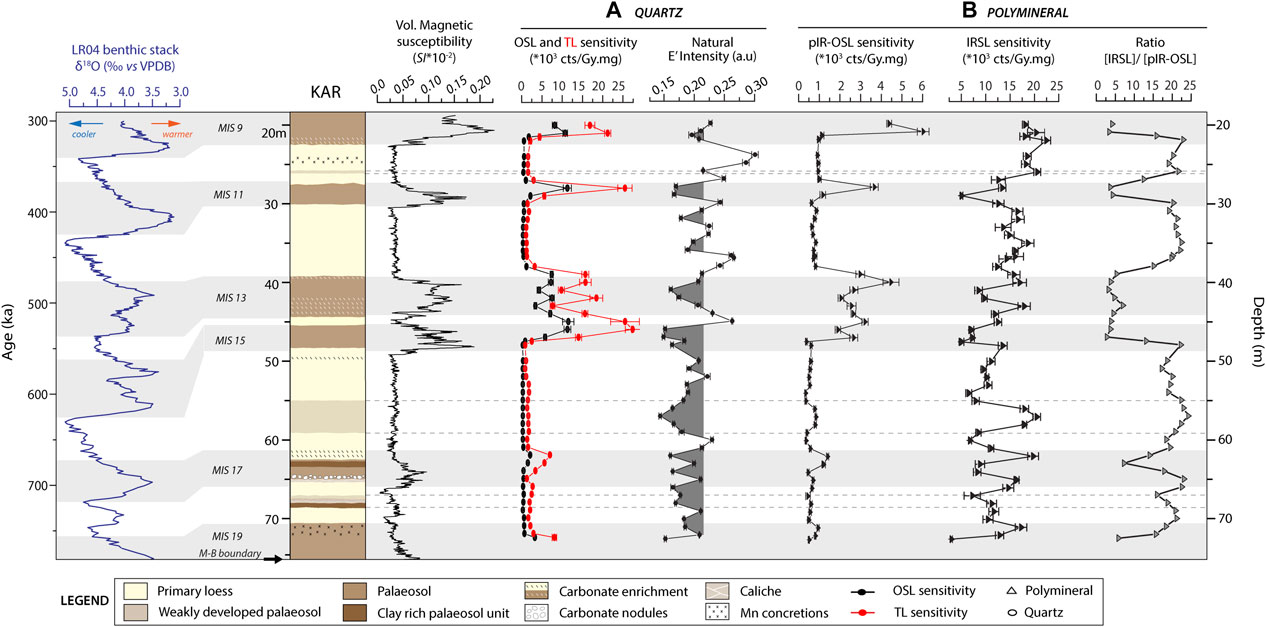
FIGURE 2. Down-profile variations in (A) the sensitivity of the OSL and 110°C TL signal and the natural E1’ intensity from fine grain quartz and (B) the sensitivity of the pIR-OSL signal, the IRSL signal and the ratio of [IRSL]/[pIR-OSL] signal from fine grain polymineral samples for the KAR loess sequence. Stratigraphy of KAR and its correlation to the global benthic curve (Lisiecki and Raymo, 2005) is based on in-situ magnetic susceptibility measurements at the site (modified after Dave et al., 2022).
Sampling at KAR was carried out by abseiling down the exposed vertical cliff to ensure continuous down-profile observation and sample collection. Prior to sampling, more than 1 m of the overlying sediment on the cliff-face was removed to prevent contamination by recent displacement and to expose undisturbed stratigraphy. We collected a total of 55 samples every 0.5–1 m by hammering opaque tubes into the cleaned and exposed vertical section.
Luminescence Measurements
All samples were processed under subdued red-light conditions in dedicated laboratories at the Johannes Gutenberg University and Max Planck Institute for Chemistry in Mainz to extract fine-grained (4–11 µm) quartz and polymineral grains using published procedures (Frechen et al., 1996). Details of sample preparation, instrumentation and luminescence sensitivity measurements are given in the supplementary. The protocols for measurement of OSL and TL sensitivity of fine-grained quartz and the infrared stimulated luminescence (IRSL) and post infrared-optically stimulated luminescence (pIR-OSL) of polymineral grains are outlined in Table 1. Since luminescence sensitivity entails the measurement of luminescence emitted (counts, cts) per unit dose (Gy) per unit mass (mg), the luminescence measurements from all aliquots were normalised to the given laboratory dose and the corresponding aliquot mass. The sensitivity is expressed as cts/Gy.mg and uncertainties as 1σ standard deviation.
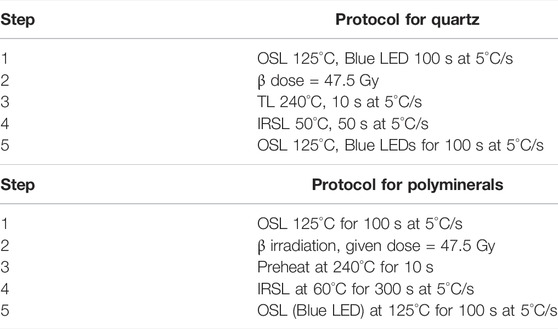
TABLE 1. Protocol for luminescence sensitivity measurements from fine-grained quartz and polymineral samples (modified after Sawakuchi et al., 2018; Li and Zhou, 2021).
In addition, we conducted preliminary experiments to assess the effect of repeated laboratory- irradiation and bleaching cycles (80 cycles) on four fine-grained quartz samples from loess and soil units respectively. The protocol for these experiments is briefly described and outlined in Supplementary Table S1 in the Supplementary Materials S1.
Electron Spin Resonance Measurements
ESR allows the measurement of paramagnetic (containing an unpaired electron) species in a material. In this study we focus on the natural intensity of two paramagnetic defect centres in fine-grained quartz from KAR: the E1’ centre and the peroxy centre. The measurement of these centres was carried out at Babeș-Bolyai-University in Cluj-Napoca and is reported in Dave et al. (2022). A summarised account of sample measurement procedures and parameters is presented in the Supplementary Materials S1.
Results
Figure 2 illustrates the down-profile variations in luminescence sensitivity of the OSL and 110°C TL (hereon referred to as TL) signal and E1’ defect centre intensity from fine-grained quartz, and the pIR60-OSL, IR60 (here 60°C refers to temperature of IRSL wash and is hereon referred to as pIR-OSL and IRSL respectively) and the ratio [IRSL]/[pIR-OSL] from polymineral fine grains. We observe that the quartz luminescence sensitivity of the TL and OSL signals is higher in the palaeosol horizons compared to primary loess units, and correlates with the variability in magnetic susceptibility (Figure 2A). In addition, Figure 3A shows the correlation between the 110°C TL and OSL signal, which is stronger in fine-grained quartz from soils as compared to that extracted from loess samples. This may be a result of either a difference in the inherent properties of quartz related to its origin and/or to the sensitisation of quartz during its sedimentary and depositional history. Jain et al. (2003) observed that not all components of the OSL signal in quartz sensitise in an identical manner, which implies that the degree of correlation between the 110°C TL and the different OSL components may vary between samples. Albeit at this stage, making a distinction between the sensitisation process of the different OSL components is not possible.
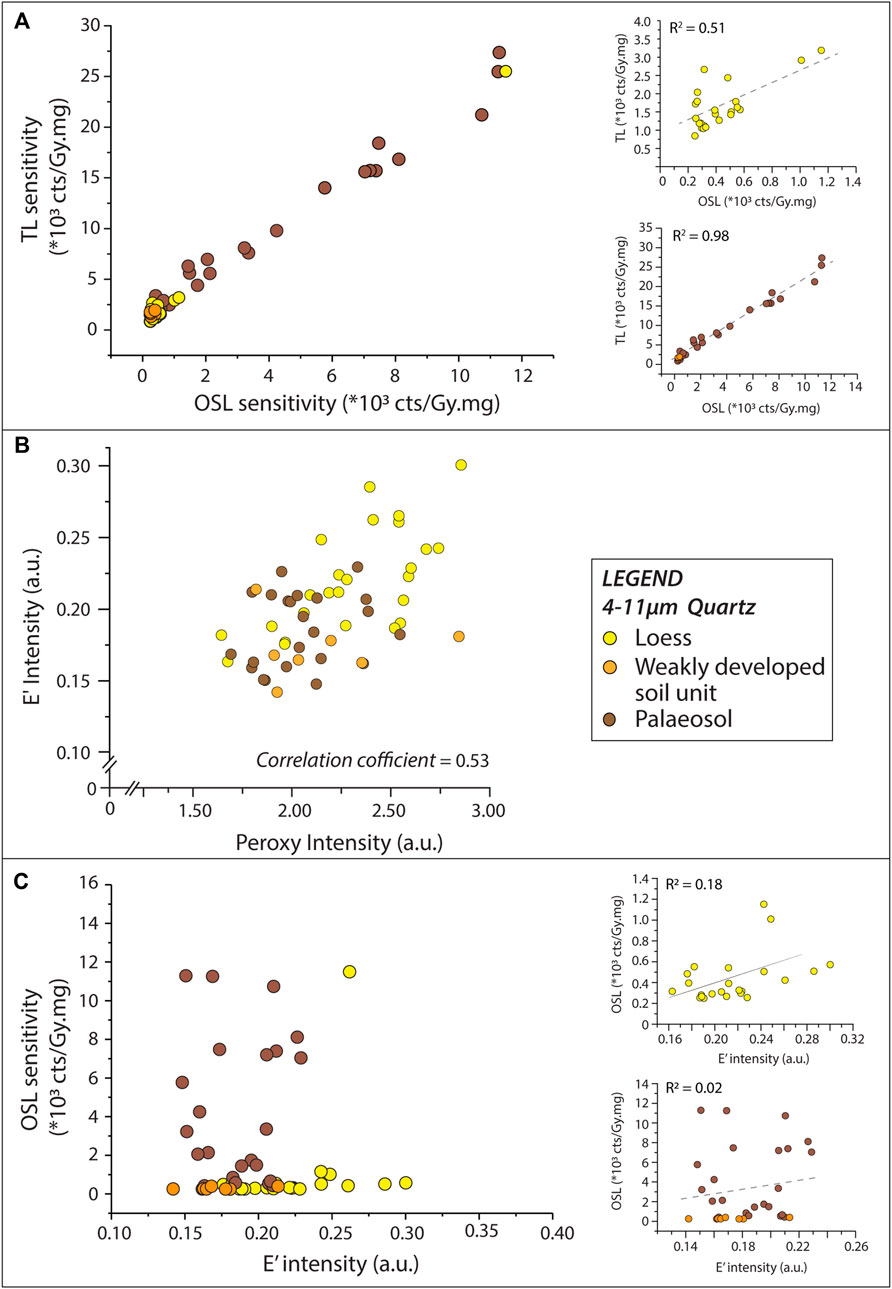
FIGURE 3. (A) Plot comparing the sensitivity of OSL and 110°C TL signal from fine-grained quartz with respect to different stratigraphic units (loess, palaeosol, weakly developed soil) (B) Cross-plot of the E1’ and peroxy centre intensity from fine grain quartz with respect to stratigraphic units (modified from Dave et al., 2022) (C) Plot comparing E1’ intensity and sensitivity of the OSL signal from fine-grained quartz with respect to stratigraphic units. Note: The anomalous value of the loess sample (A0301, at depth c. 45 m), that occurs as an “outlier” in the main plots of (A) and (C), is not plotted in the inset plots to avoid rescaling of the y-axis.
The ratio [IRSL]/[pIR-OSL] in polymineral grains has been proposed as a proxy for weathering intensity in loess-palaeosol sequences in the CLP (Wang and Miao, 2006). This ratio was proposed to indicate the relative enrichment of quartz in palaeosols due to weathering of feldspar minerals in these units. However, it must be noted that this is a “relative feldspar-content” driven interpretation and does not consider the inherent sensitivity of feldspar due to source change and/or its variation due to its sedimentary history. Our results on down-profile variability in the pIR-OSL and IRSL signal from polymineral fine-grains show that the pIR-OSL signal increases in soils and decreases in loess units while the IRSL signal does not show any apparent trend down the loess-palaeosol section (Figure 2B). Thus, the ratio [IRSL]/[pIR-OSL] from fine-grained polymineral samples at KAR shows a decrease within palaeosol horizons and an increase in loess horizons (Figure 2B), suggesting that in this case, the ratio is predominantly driven by the strong variation of the pIR-OSL signal from the quartz fraction as compared to the IRSL signal from the feldspar fraction in the polymineral.
Down-profile variation in the intensity of the natural E1’ signal of fine-grained quartz in general, indicates lower intensity in the palaeosol units and higher values in the primary loess horizons (Figure 2A). The variation in peroxy intensity of fine-grained quartz with depth correlates with the variations in natural E1’ values (Supplementary Figure S2) and hence, only the E1’ trend is shown in Figure 2A. Also, the variations in E1’ are more pronounced above c. 60 m depth. While, below c. 60 m (prior to MIS 16), the natural E1’ intensity is generally lower but fluctuates at a higher frequency and appears to be inconsistent with the observed stratigraphy of loess and soil units (Figure 2A). Nonetheless, the plot comparing natural E1’ and peroxy intensities in fine-grained quartz highlights the difference between loess and soil units (Figure 3B). Additionally, a brief look at Figures 3A, B suggests that the TL and OSL sensitivity is higher while the E1’ and peroxy intensity is lower in soils and vice versa for loess horizons. However, the comparison of the E1’ intensity with the OSL sensitivity of fine-grained quartz in Figure 3C does not show any correlation. Thus, the fundamental relationship between E1’ intensity and OSL sensitivity of quartz as yet remains elusive (Poolton et al., 2000; Schilles et al., 2001).
Discussion
Climate modelling studies and down-profile grain size variations in loess-palaeosol sequences in southern Tajikistan suggest stronger Westerly winds during glacial periods as compared to interglacials; thereby indicating dust transport from distal sources during glacial periods (Vandenberghe et al., 2006). Based on these studies, we suggest that the low natural E1’ intensities observed at KAR in the warmer, more humid soil units (interglacial conditions) is linked to proximal source signature. There are also phases within loess accumulating glacial stages, particularly during interstadial periods which oversaw weak pedogenesis, when E1’ intensity values were also low and which likewise indicate a net input of proximal source material (Figure 2A). At the same time, throughout the KAR profile, natural E1’ intensities, while variable, are relatively low, which suggests that proximal source sediments dominate the net background sediment contribution to the site throughout its depositional history. This observation is in tune with recent observations made by Li et al. (2019) that propose an increased likelihood of proximally sourced dust in loess deposits in the Tajik basin based on elemental geochemistry data from loess and modern meteorological analysis. Nevertheless, during glacial phases there is a stronger shift towards quartz with higher E1’ intensities (Figure 2A), particularly above c. 60 m depth. Below this depth the distinction between primary loess and palaeosols based on E1’ intensity is less pronounced. This is most likely due to the presence of multiple palaeosol horizons, low sedimentation rates, and compaction of the sediment, which may have contributed to over-printing of the quartz E1’ signature. Furthermore, the c. 60 m depth marker at KAR coincides with the end of the mid-Pleistocene transition (MPT; Clark et al., 2006), after which the Earth’s climate shifted to lower-frequency (c. 100 kyr), higher-amplitude glacial-interglacial cycles. The MPT transition has been observed to manifest in other loess regions as more distinct loess-palaeosol oscillations with higher sedimentation rates (e.g., Fitzsimmons et al., 2012; Marković et al., 2015), which may explain the more distinct high E1’ intensity values within the glacial loess above c. 60 m at KAR.
The natural E1’ intensity values from fine-grained quartz add nuance to, and raises questions about, the interpretation derived from the OSL and TL sensitivity record at KAR. Within the primary loess and weak palaeosol layers accreted during the glacial and interstadial periods, OSL and TL sensitivity remains consistently low in relation to quartz from the more strongly developed interglacial palaeosols with high sensitivity values (Figure 2A). The net background input of proximally sourced material to the site, as proposed from the E1’ results, accounts for the comparatively constant sensitivity of the quartz OSL and TL signal observed in the loess units at KAR. However, it is unclear why the OSL and TL sensitivity shows minimal variation during loess accumulating glacial periods, which is unlike the variations shown by the E1’ signal. We hypothesise that the net OSL and TL sensitivity remains unaffected by the source shifts recorded by the E1’ during loess accumulating (glacial) stages because E1’ is a more sensitive proxy which measures a specific defect centre in quartz, whereas luminescence sensitivity is emitted from multiple traps as a result of recombination in both electron and hole centres (Aitken, 1998; Yukihara and McKeever, 2011). As a result, the proximal signal dominates the OSL and TL sensitivity values and masks more subtle changes due to input from a distal source.
It is likewise unclear what caused the increase in OSL and TL sensitivity observed during the formation of interglacial palaeosols. We propose this to be due to depositional and post-depositional processes associated with soil formation. Most of these processes may be linked to factors like the effect of dose rate from the surrounding sediment, repeated cycles of bleaching and irradiation during extended periods of soil formation and the possible effect of diagenesis during burial on the sensitivity of quartz. Of these factors, firstly, previous studies (e.g., Lü et al., 2014; Li and Zhou, 2021; Lü et al., 2021) demonstrate no correlation between the dose rate (i.e., ionising radiation received during burial) and OSL and TL sensitivity in long loess-palaeosol sequences and therefore, we suggest that downcore variability in dose rate is unlikely to explain the observed sensitivity changes. Secondly, it is well known that quartz is a stable mineral and least susceptible to in-situ weathering, however not much is known about the effect of diagenesis of quartz during burial on luminescence sensitivity. However, we envisage that these changes (if any) are likely to be insignificant over Quaternary timescales. This brings us to the role of repeated cycles of bleaching and irradiation associated with extended and intense periods of pedogenesis, aided by reduced sedimentation rates as the most likely factor contributing to enhanced sensitivity values observed in soil units. Since, the sensitisation of quartz via such a process is unlikely to be consistent, it would also explain the high degree of scatter observed in quartz OSL and TL sensitivity within the palaeosol horizons as compared to primary loess (Figure 3A). Our premise is also supported by the recent study from Li and Zhou (2021), who observed a three-fold increase in OSL sensitivity of fine-grained quartz from Tajik loess, after repeated laboratory induced irradiation and bleaching cycles. Additionally, our experiments based on limited (80 cycles) but repeated cycles of laboratory induced irradiation and bleaching in quartz from KAR loess, shows a 15–25% increase in OSL and TL sensitivity (Supplementary Figure S3), which further supports our hypothesis.
We also observe that the fine-grained quartz from the weakly developed soil units within the loess exhibits similar natural E1’ and peroxy characteristics to quartz from the well-developed palaeosol units (Figure 3B), suggesting a proximal source signature as observed during interglacial stages. However, the same quartz from weakly developed soils yields lower OSL and TL sensitivities similar to that observed in loess (Figure 3A). This agrees with our premise that repeated irradiation and bleaching cycles during soil formation aids the sensitisation of quartz, since quartz from weakly developed soils which represent weak pedogenesis under higher sedimentation rate are less likely to experience prolonged and repeated cycles of irradiation and bleaching post-deposition and therefore, do not exhibit increased sensitivity unlike the well-developed palaeosols.
At this stage, it must be noted from the work of previous studies that the inherent sensitivity of quartz as well as its preferential ability to sensitise (i.e., the degree and rate of sensitisation) related to its origin (Sharma, 2017; Sawakuchi et al., 2018; Bartyik et al., 2021; Li and Zhou, 2021) are crucial factors in dictating the OSL and TL sensitivity of quartz. Nonetheless, during formation of loess-palaeosol sequences, additional external factors like extended periods of irradiation and bleaching during pedogenesis aided by the low sedimentation rates during soil formation can lead to sensitisation of the OSL and TL signals in quartz. This post-depositional sensitisation of quartz in soil horizons can overprint the inherent luminescence sensitivity characteristics of the quartz.
Conclusion
This study utilises the natural E1’ and peroxy characteristics of quartz as a provenance indicator to assess the observed variations in luminescence (OSL and TL) sensitivity down a long loess-palaeosol sequence in Tajikistan. Previous studies link down-profile variations in luminescence sensitivity with climatic fluctuations that occur over glacial-interglacial timescales (Lü et al., 2014; Li and Zhou, 2021; Lü et al., 2021). However, the cause for the observed luminescence sensitivity variations in quartz is still debated (Lü et al., 2014; Li and Zhou, 2021; Lü et al., 2021). Our comparison of luminescence characteristics with E1’ and peroxy centres in quartz suggest that the high OSL and TL sensitivity in the soil horizons is likely to reflect sensitisation of quartz due to repeated irradiation and bleaching cycles during pedogenesis. At the same time, luminescence sensitivity variations that may have otherwise occurred due to changes in sediment source - for instance, as a result of input of some distal material at the loess site during glacial periods, is likely to be masked by the dominant input of proximally derived material. Nevertheless, the OSL and TL sensitivity of quartz from aeolian environments holds great potential for identifying spatial variability in luminescence characteristics between sites.
Data Availability Statement
The raw data supporting the conclusion of this article will be made available by the authors, without undue reservation.
Author Contributions
AD, AT-G and KF conceptualized the research. AD processed the samples, designed and performed the experiments, analysed the results and wrote the original draft of the manuscript. GS carried out the magnetic susceptibility measurements in the field. AD, KF, GS and NS carried out fieldwork and sample collection. All authors contributed to the manuscript revision and read and approved the submitted manuscript.
Funding
This research was supported by the Independent Max Planck Research grant awarded to KF. AT-G acknowledges the financial support of UEFISCDI PN-III-P4-ID-PCE-2020-0542 no 114/2021. We acknowledge open access publication support by Open Access Publishing Fund of the University of Tübingen, Germany.
Conflict of Interest
The authors declare that the research was conducted in the absence of any commercial or financial relationships that could be construed as a potential conflict of interest.
Publisher’s Note
All claims expressed in this article are solely those of the authors and do not necessarily represent those of their affiliated organizations, or those of the publisher, the editors and the reviewers. Any product that may be evaluated in this article, or claim that may be made by its manufacturer, is not guaranteed or endorsed by the publisher.
Acknowledgments
AD would like to thank the Luminescence Laboratory at the Max Planck Institute for Evolutionary Anthropology, Leipzig, Germany for access to the HF etching laboratory, and R Schiebel at the Max Planck Institute for Chemistry (MPIC) for access to a high precision mass balance. We would also like to thank our colleagues for their assistance during fieldwork: S Yusupov, A Safarov, ZN Nadjmudinov and RS Pavlatov (Tajik National University); C Prud’homme, A Engström Johansson, L Marquer, Z Perić, S de Graaf, M Notwatzki and K Reetz (MPIC).
Supplementary Material
The Supplementary Material for this article can be found online at: https://www.frontiersin.org/articles/10.3389/feart.2022.835281/full#supplementary-material
References
Aitken, M. J. (1998). An Introduction to Optical Dating: The Dating of Quaternary Sediments by the Use of Photon-Stimulated Luminescence. Oxford: Oxford University Press.
Bartyik, T., Magyar, G., Filyó, D., Tóth, O., Blanka-Végi, V., Kiss, T., et al. (2021). Spatial Differences in the Luminescence Sensitivity of Quartz Extracted from Carpathian Basin Fluvial Sediments. Quat. Geochronol. 64, 101166. doi:10.1016/j.quageo.2021.101166
Buggle, B., Glaser, B., Zöller, L., Hambach, U., Marković, S., Glaser, I., et al. (2008). Geochemical Characterization and Origin of Southeastern and Eastern European Loesses (Serbia, Romania, Ukraine). Quat. Sci. Rev. 27 (9), 1058–1075. doi:10.1016/j.quascirev.2008.01.018
Clark, P. U., Archer, D., Pollard, D., Blum, J. D., Rial, J. A., Brovkin, V., et al. (2006). The Middle Pleistocene Transition: Characteristics, Mechanisms, and Implications for Long-Term Changes in Atmospheric pCO2. Quat. Sci. Rev. 25 (23-24), 3150–3184. doi:10.1016/j.quascirev.2006.07.008
Dave, A. K., Timar-Gabor, A., Kabacińska, Z., Scardia, G., Safaraliev, N., Nigmatova, S., et al. (2022). A Novel Proxy for Tracking the Provenance of Dust Based on Paired E1’-Peroxy Paramagnetic Defect Centres in Fine-Grained Quartz. Earth Space Sci. Open Archive. [Preprint]. doi:10.1002/essoar.10510943.1
Ding, Z. L., Rutter, N. W., Sun, J. M., Yang, S. L., and Liu, T. S. (2000). Re-arrangement of Atmospheric Circulation at about 2.6Ma over Northern China: Evidence from Grain Size Records of Loess-Palaeosol and Red Clay Sequences. Quat. Sci. Rev. 19 (6), 547–558. doi:10.1016/S0277-3791(99)00017-7
Dodonov, A. E., and Baiguzina, L. L. (1995). Loess Stratigraphy of Central Asia: Palaeoclimatic and Palaeoenvironmental Aspects. Aeolian Sediments Quat. Rec. 14 (7), 707–720. doi:10.1016/0277-3791(95)00054-2
Fenn, K., Stevens, T., Bird, A., Limonta, M., Rittner, M., Vermeesch, P., et al. (2018). Insights into the Provenance of the Chinese Loess Plateau from Joint Zircon U-Pb and Garnet Geochemical Analysis of Last Glacial Loess. Quat. Res. 89 (3), 645–659. doi:10.1017/qua.2017.86
Fitzsimmons, K. (2011). An Assessment of the Luminescence Sensitivity of Australian Quartz with Respect to Sediment History. Geochronometria 38 (3), 199–208. doi:10.2478/s13386-011-0030-9
Fitzsimmons, K. E., Rhodes, E. J., and Barrows, T. T. (2010). OSL Dating of Southeast Australian Quartz: A Preliminary Assessment of Luminescence Characteristics and Behaviour. Quat. Geochronol. 5 (2), 91–95. doi:10.1016/j.quageo.2009.02.009
Fitzsimmons, K. E., Marković, S. B., and Hambach, U. (2012). Pleistocene Environmental Dynamics Recorded in the Loess of the Middle and Lower Danube Basin. Quat. Sci. Rev. 41, 104–118. doi:10.1016/j.quascirev.2012.03.002
Forster, Th., and Heller, F. (1994). Loess Deposits from the Tajik Depression (Central Asia): Magnetic Properties and Paleoclimate. Earth Planet. Sci. Lett. 128 (3–4), 501–512. doi:10.1016/0012-821X(94)90166-X
Frechen, M., Schweitzer, U., and Zander, A. (1996). Improvements in Sample Preparation for the Fine Grain Technique. Anc. TL 14 (2), 15–17. Available at: http://ancienttl.org/ATL_14-2_1996/ATL_14-2_Frechen_p15-17.pdf.
Jain, M., Murray, A. S., and Bøtter-Jensen, L. (2003). Characterisation of Blue-Light Stimulated Luminescence Components in Different Quartz Samples: Implications for Dose Measurement. Radiat. Meas. 37 (4), 441–449. doi:10.1016/S1350-4487(03)00052-0
Jeong, G. Y., and Choi, J.-H. (2012). Variations in Quartz OSL Components with Lithology, Weathering and Transportation. Quat. Geochronol. 10, 320–326. doi:10.1016/j.quageo.2012.02.023
Kukla, G., Heller, F., Ming, L. X., Chun, X. T., Sheng, L. T., and Sheng, A. Z. (1988). Pleistocene Climates in China Dated by Magnetic Susceptibility. Geol 16 (9), 811–814. doi:10.1130/0091-7613(1988)016<0811:pcicdb>2.3.co;2
Li, Y., Song, Y., Kaskaoutis, D. G., Chen, X., Mamadjanov, Y., and Tan, L. (2019). Atmospheric Dust Dynamics in Southern Central Asia: Implications for Buildup of Tajikistan Loess Sediments. Atmos. Res. 229, 74–85. doi:10.1016/j.atmosres.2019.06.013
Li, Y., and Zhou, L. (2021). Variations of Thermally and Optically Stimulated Luminescence Sensitivity of Loess and Pedocomplex Samples from Southern Tajikistan, Central Asia. Geochronometria 48 (1), 242–252. doi:10.1515/geochr-2015-0118
Lisiecki, L. E., and Raymo, M. E. (2005). A Pliocene-Pleistocene Stack of 57 Globally Distributed Benthic δ18O Records. Paleoceanography 20 (1), a–n. doi:10.1029/2004PA001071
Lü, T., Sun, J., Li, S.-H., Gong, Z., and Xue, L. (2014). Vertical Variations of Luminescence Sensitivity of Quartz Grains from Loess/paleosol of Luochuan Section in the Central Chinese Loess Plateau since the Last Interglacial. Quat. Geochronol. 22, 107–115. doi:10.1016/j.quageo.2014.04.004
Lü, T., Sun, J., Feathers, J. K., and Sun, D. (2021). Spatiotemporal Variations and Implications of Luminescence Sensitivity of Quartz Grains on the Chinese Loess Plateau since the Last Interglaciation. Quat. Res. 99, 190–203. Cambridge Core. doi:10.1017/qua.2020.53
Lü, T., and Sun, J. (2011). Luminescence Sensitivities of Quartz Grains from Eolian Deposits in Northern China and Their Implications for Provenance. Quat. Res. 76 (2), 181–189. doi:10.1016/j.yqres.2011.06.015
Marković, S. B., Stevens, T., Kukla, G. J., Hambach, U., Fitzsimmons, K. E., Gibbard, P., et al. (2015). Danube Loess Stratigraphy - towards a Pan-European Loess Stratigraphic Model. Earth-Science Rev. 148, 228–258. doi:10.1016/j.earscirev.2015.06.005
McNab, F., Sloan, R. A., and Walker, R. T. (2019). Simultaneous Orthogonal Shortening in the Afghan-Tajik Depression. Geology 47 (9), 862–866. doi:10.1130/G46090.1
Muhs, D. R., Bettis, E. A., Aleinikoff, J. N., McGeehin, J. P., Beann, J., Skipp, G., et al. (2008). Origin and Paleoclimatic Significance of Late Quaternary Loess in Nebraska: Evidence from Stratigraphy, Chronology, Sedimentology, and Geochemistry. Geol. Soc. Am. Bull. 120 (11–12), 1378–1407. doi:10.1130/B26221.1
Nagashima, K., Tada, R., Tani, A., Toyoda, S., Sun, Y., and Isozaki, Y. (2007). Contribution of Aeolian Dust in Japan Sea Sediments Estimated from ESR Signal Intensity and Crystallinity of Quartz. Geochem. Geophys. Geosyst. 8 (2), a–n. doi:10.1029/2006GC001364
Nagashima, K., Nishido, H., Kayama, M., Kurosaki, Y., Ohgo, S., and Hasegawa, H. (2017). Composition of Asian Dust from Cathodoluminescence Spectral Analysis of Single Quartz Grains. Geology 45 (10), 879–882. doi:10.1130/G39237.1
Nagashima, K., Tada, R., Tani, A., Sun, Y., Isozaki, Y., Toyoda, S., et al. (2011). Millennial-scale Oscillations of the Westerly Jet Path during the Last Glacial Period. J. Asian Earth Sci. 40 (6), 1214–1220. doi:10.1016/j.jseaes.2010.08.010
Odom, A. L., and Rink, W. J. (1989). Natural Accumulation of Schottky-Frenkel Defects: Implications for a Quartz Geochronometer. Geol 17 (1), 55–58. doi:10.1130/0091-7613(1988)017<0055:naosfd>2.3.co;2
Pietsch, T. J., Olley, J. M., and Nanson, G. C. (2008). Fluvial Transport as a Natural Luminescence Sensitiser of Quartz. Quat. Geochronol. 3 (4), 365–376. doi:10.1016/j.quageo.2007.12.005
Poolton, N. R. J., Smith, G. M., Riedi, P. C., Bulur, E., Bøtter-Jensen, L., Murray, A. S., et al. (2000). Luminescence Sensitivity Changes in Natural Quartz Induced by High Temperature Annealing: A High Frequency EPR and OSL Study. J. Phys. D. Appl. Phys. 33 (8), 1007–1017. doi:10.1088/0022-3727/33/8/318
Rao, W., Yang, J., Chen, J., and Li, G. (2006). Sr-Nd Isotope Geochemistry of Eolian Dust of the Arid-Semiarid Areas in China: Implications for Loess Provenance and Monsoon Evolution. Sci. Bull. 51 (12), 1401–1412. doi:10.1007/s11434-006-2008-1
Sawakuchi, A. O., Blair, M. W., DeWitt, R., Faleiros, F. M., Hyppolito, T., and Guedes, C. C. F. (2011). Thermal History versus Sedimentary History: OSL Sensitivity of Quartz Grains Extracted from Rocks and Sediments. Quat. Geochronol. 6 (2), 261–272. doi:10.1016/j.quageo.2010.11.002
Sawakuchi, A. O., Guedes, C. C. F., DeWitt, R., Giannini, P. C. F., Blair, M. W., Nascimento, D. R., et al. (2012). Quartz OSL Sensitivity as a Proxy for Storm Activity on the Southern Brazilian Coast during the Late Holocene. Quat. Geochronol. 13, 92–102. doi:10.1016/j.quageo.2012.07.002
Sawakuchi, A. O., Jain, M., Mineli, T. D., Nogueira, L., Bertassoli, D. J., Häggi, C., et al. (2018). Luminescence of Quartz and Feldspar Fingerprints Provenance and Correlates with the Source Area Denudation in the Amazon River Basin. Earth Planet. Sci. Lett. 492, 152–162. doi:10.1016/j.epsl.2018.04.006
Schaetzl, R. J., Bettis, E. A., Crouvi, O., Fitzsimmons, K. E., Grimley, D. A., Hambach, U., et al. (2018). May)Approaches and Challenges to the Study of Loess—Introduction to the LoessFest Special Issue. Quat. Res. 89, 563–618. doi:10.1017/qua.2018.15
Schilles, T., Poolton, N. R. J., Bulur, E., Bøtter-Jensen, L., Murray, A. S., Smith, G. M., et al. (2001). A Multi-Spectroscopic Study of Luminescence Sensitivity Changes in Natural Quartz Induced by High-Temperature Annealing. J. Phys. D. Appl. Phys. 34 (5), 722–731. doi:10.1088/0022-3727/34/5/310
Sharma, S. K. (2017). Understanding the Reasons for Variations in Luminescence Sensitivity of Natural Quartz Using Spectroscopic and Chemical Studies. Proc. Indian Natl. Sci. Acad. 92 (0), 9024. doi:10.16943/ptinsa/2017/49024
Shimada, A., Takada, M., and Toyoda, S. (2013). Characteristics of ESR Signals and TLCLs of Quartz Included in Various Source Rocks and Sediments in Japan: A Clue to Sediment Provenance. Geochronometria 40 (4), 334–340. doi:10.2478/s13386-013-0111-z
Smith, J., Vance, D., Kemp, R. A., Archer, C., Toms, P., King, M., et al. (2003). Isotopic Constraints on the Source of Argentinian Loess – with Implications for Atmospheric Circulation and the Provenance of Antarctic Dust during Recent Glacial Maxima. Earth Planet. Sci. Lett. 212 (1), 181–196. doi:10.1016/s0012-821x(03)00260-7
Stevens, T., Palk, C., Carter, A., Lu, H., and Clift, P. D. (2010). Assessing the Provenance of Loess and Desert Sediments in Northern China Using U-Pb Dating and Morphology of Detrital Zircons. Geol. Soc. Am. Bull. 122 (7–8), 1331–1344. doi:10.1130/B30102.1
Sun, J. (2002). Provenance of Loess Material and Formation of Loess Deposits on the Chinese Loess Plateau. Earth Planet. Sci. Lett. 203 (3), 845–859. doi:10.1016/S0012-821X(02)00921-4
Sun, Y., Tada, R., Chen, J., Chen, H., Toyoda, S., Tani, A., et al. (2007). Distinguishing the Sources of Asian Dust Based on Electron Spin Resonance Signal Intensity and Crystallinity of Quartz. Atmos. Environ. 41 (38), 8537–8548. doi:10.1016/j.atmosenv.2007.07.014
Sun, Y., Tada, R., Chen, J., Liu, Q., Toyoda, S., Tani, A., et al. (2008). Tracing the Provenance of Fine-Grained Dust Deposited on the Central Chinese Loess Plateau. Geophys. Res. Lett. 35 (1), L01804. doi:10.1029/2007GL031672
Tissoux, H., Voinchet, P., Lacquement, F., and Despriée, F. (2015). ESR as a Method for the Characterization of Alluvial Sediments. Radiat. Meas. 81, 2–8. doi:10.1016/j.radmeas.2015.05.010
Toyoda, S., and Hattori, W. (2000). Formation and Decay of the E1′ Center and of its Precursor. Appl. Radiat. Isotopes 52 (5), 1351–1356. doi:10.1016/S0969-8043(00)00094-4
Toyoda, S., Nagashima, K., and Yamamoto, Y. (2016). ESR Signals in Quartz: Applications to Provenance Research - A Review. Quat. Int. 397, 258–266. doi:10.1016/j.quaint.2015.05.048
Tsukamoto, S., Nagashima, K., Murray, A. S., and Tada, R. (2011). Variations in OSL Components from Quartz from Japan Sea Sediments and the Possibility of Reconstructing Provenance. Quat. Int. 234 (1), 182–189. doi:10.1016/j.quaint.2010.09.003
Újvári, G., Varga, A., Ramos, F. C., Kovács, J., Németh, T., and Stevens, T. (2012). Evaluating the Use of Clay Mineralogy, Sr-Nd Isotopes and Zircon U-Pb Ages in Tracking Dust Provenance: An Example from Loess of the Carpathian Basin. Chem. Geol. 304-305, 83–96. doi:10.1016/j.chemgeo.2012.02.007
Vandenberghe, J., Renssen, H., van Huissteden, K., Nugteren, G., Konert, M., Lu, H., et al. (2006). Penetration of Atlantic Westerly Winds into Central and East Asia. Quat. Sci. Rev. 25 (17), 2380–2389. doi:10.1016/j.quascirev.2006.02.017
Wang, K., Tada, R., Zheng, H., Irino, T., Zhou, B., and Saito, K. (2020). Provenance Changes in Fine Detrital Quartz in the Inner Shelf Sediments of the East China Sea Associated with Shifts in the East Asian Summer Monsoon Front during the Last 6 Kyrs. Prog. Earth Planet Sci. 7 (1), 5. doi:10.1186/s40645-019-0319-5
Wang, X., and Miao, X. (2006). Weathering History Indicated by the Luminescence Emissions in Chinese Loess and Paleosol. Quat. Sci. Rev. 25 (13), 1719–1726. doi:10.1016/j.quascirev.2005.11.009
Wei, C., Yin, G., Li, Y., Liu, C., Li, W., and Guo, R. (2020). Quartz Electron Spin Resonance Signal Intensity of Al and Ti-Li Centers as a Provenance Indicator: An Example from the Yangtze River Basin. Quat. Int. 562, 76–84. doi:10.1016/j.quaint.2020.06.023
Weltje, G. J., and von Eynatten, H. (2004). Quantitative Provenance Analysis of Sediments: Review and Outlook. Sediment. Geol. 171 (1), 1–11. doi:10.1016/j.sedgeo.2004.05.007
Yukihara, E. G., and McKeever, S. W. S. (2011). Optically Stimulated Luminescence: Fundamentals and Applications. John Wiley & Sons. doi:10.1002/9780470977064
Zheng, C. X., Zhou, L. P., and Qin, J. T. (2009). Difference in Luminescence Sensitivity of Coarse-Grained Quartz from Deserts of Northern China. Radiat. Meas. 44 (5), 534–537. doi:10.1016/j.radmeas.2009.02.013
Keywords: provenance, luminescence sensitivity, defect centres, Tajikistan, Central Asia, loess
Citation: Dave AK, Timar-Gabor A, Scardia G, Safaraliev N and Fitzsimmons KE (2022) Variation in Luminescence Characteristics and Paramagnetic Defect Centres in Fine-Grained Quartz From a Loess-Palaeosol Sequence in Tajikistan: Implications for Provenance Studies in Aeolian Environments. Front. Earth Sci. 10:835281. doi: 10.3389/feart.2022.835281
Received: 14 December 2021; Accepted: 02 May 2022;
Published: 31 May 2022.
Edited by:
David K. Wright, University of Oslo, NorwayReviewed by:
Sumiko Tsukamoto, Leibniz Institute for Applied Geophysics (LIAG), GermanyManoj Kumar Jaiswal, Indian Institute of Science Education and Research Kolkata, India
Copyright © 2022 Dave, Timar-Gabor, Scardia, Safaraliev and Fitzsimmons. This is an open-access article distributed under the terms of the Creative Commons Attribution License (CC BY). The use, distribution or reproduction in other forums is permitted, provided the original author(s) and the copyright owner(s) are credited and that the original publication in this journal is cited, in accordance with accepted academic practice. No use, distribution or reproduction is permitted which does not comply with these terms.
*Correspondence: Aditi K. Dave, aditi-krishna.dave@uni-tuebingen.de
†Present address:Aditi K. Dave, Department of Geosciences, University of Tübingen, Tübingen, Germany