- 1Centro de Estudios Avanzados en Zonas Áridas (CEAZA), La Serena, Chile
- 2Departamento de Biología Marina, Universidad Católica del Norte, Coquimbo, Chile
- 3Instituto Argentino de Nivología, Glaciología y Ciencias Ambientales (IANIGLA), CCT CONICET Mendoza, Mendoza, Argentina
- 4Instituto de Ciencias de La Tierra, Facultad de Ciencias, Universidad Austral de Chile, Valdivia, Chile
- 5Centro de Investigación en Ecosistemas de la Patagonia (CIEP), Coyhaique, Chile
- 6Centro de Estudios del Hombre Austral, Instituto de la Patagonia, Universidad de Magallanes, Punta, Arenas
Deglaciation modeling of the Patagonian Ice Field since the Last Glacial Maximum has been a topic of intensive research in Central West Patagonia (44°–49°S). However, the chronology of deglaciation onset, acceleration, and the subsequent thinning and recession of the different ice lobes as well as the timing and extension of large proglacial systems are still a matter of discussion. The maximum eastward extension the Lago Cochrane/Pueyrredón ice lobe was around ∼20,000–27,000 cal yrs BP; its associated proglacial lake drained toward the Pacific between 12,600 and 8,000 cal yrs BP. This study presents the first two pollen and charcoal records from the Lago Cochrane/Pueyrredón valley, spanning the last 11,650 cal yrs BP. The Laguna Maldonado record spans between 11,650 and 8,500 cal yrs BP, while the Laguna Anónima record the last 8,500 cal yrs BP, thereby overlapping chronologically. The lithological record of Laguna Maldonado shows that organic sedimentation began at the onset of the Holocene (11,650 cal yrs BP), once the site was free of glacio-lacustrine influence. Between 11,650 and 10,500 cal yrs BP, an open Nothofagus forest developed associated with high fire occurrence/frequency, followed by a transitional phase to a closer forest associated with a shift from low to high fire activity up to 9,400 cal yrs BP. Between 9,400 and 8,500 cal yrs BP, the Laguna Maldonado record suggests the development of an open forest or probably scattered patches of forest of variable size in a steppe matrix, probably related to geomorphological and paraglacial dynamics as well as climatic forcings, while the Laguna Anónima record indicates the development of a closed Nothofagus forest and high to low fire occurrence/frequency from 8,200 to 3,800 cal yrs BP. Since 3,800 cal yrs BP, a highly dynamic open forest or forest patches scattered in a grass/shrub–grass steppe matrix occurred around Laguna Anónima associated with high fire occurrence/frequency synchronous with an important increase in the presence of hunter-gatherers during the last 3,000 years. The results from the Lago Cochrane/Pueyrredón valley are integrated and discussed at the regional scale alongside other records from Central West Patagonia.
Introduction
During the Last Glacial Maximum (LGM), Central West Patagonia (44–49°S; CWP, Figure 1A) was completely covered by the Patagonian Ice Sheet (PIS) which consisted of terrestrial lobes that retreated into large paleolakes on the east and is inferred to have reached the continental shelf on the west coast (e.g., Caldenius, 1932; Coronato and Rabassa, 2011; Harrison and Glasser, 2011; Davies et al., 2020). The PIS retreated into the Andes after the LGM, but the chronology of the onset of deglaciation, its acceleration, the subsequent thinning and recession of the different ice lobes, and the timing and age of large proglacial systems are still a matter of discussion, even though intensive research has been undertaken recently (e.g., Bendle et al., 2017; García et al., 2019; Thorndycraft et al., 2019; Davies et al., 2020; Leger et al., 2021).
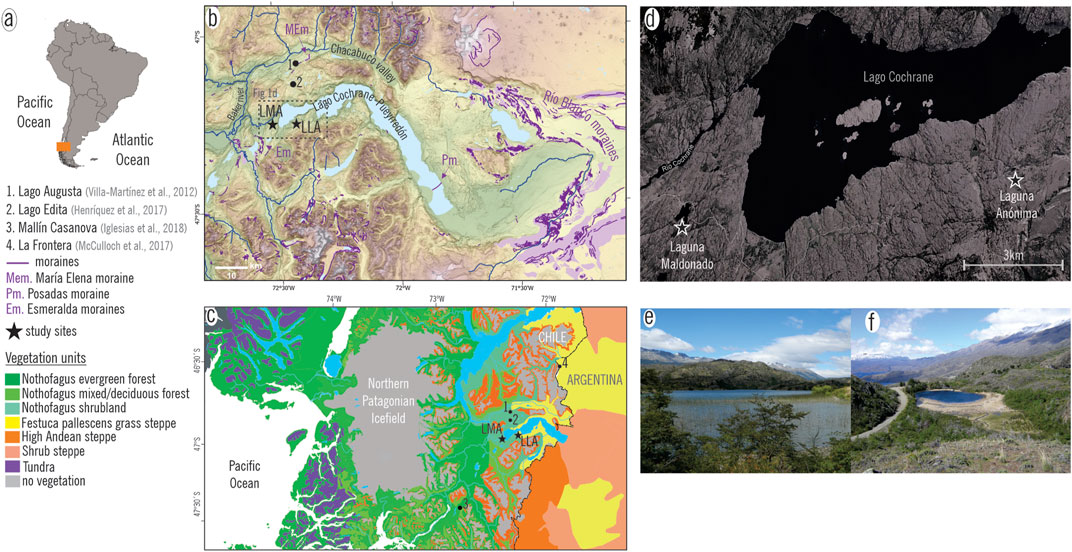
FIGURE 1. (A) Map of South America showing the study area location; (B) digital elevation model (DEM) showing the location of Laguna Maldonado (LMA) and Laguna Anónima (LLA), main glacial geomorphological features (moraines; modified from Bendle et al., 2017; Thorndycraft et al., 2019; Davies et al., 2018, 2020), and sites mentioned in the text; (C) vegetation map (taken from Luebert and Pliscoff, 2017) showing the steep west–east gradient and the locations of the paleoenvironmental records reported in this paper and previously published; (D) satellite image of the western and southern coasts of Lago Cochrane showing the location of LMA and LLA; photographs of (E) LMA and (F) LLA.
The Lago Cochrane–Pueyrredón lobe (47°S) occupied the homonymous and the Chacabuco valleys and reached its eastward maximum extension in the Patagonian steppe around ∼20,000–27,000 yrs BP (Figure 1B; Turner et al., 2005; Hein et al., 2009; Hein et al., 2010; Hein et al., 2011; Boex et al., 2013; Bendle et al., 2017; Thorndycraft et al., 2019; Davies et al., 2020). Large proglacial lakes developed in these valleys after the onset of deglaciation ca. 18,000 cal yrs BP, until they drained toward the Pacific between 12,600 and 8,000 cal yrs BP (Thorndycraft et al. (2019) and references therein). Large areas were exposed due to these major landscape transformations, offering a wide range of environments to be colonized by biota. Only three paleoenvironmental records, two located in the Chacabuco valley and one in the low Baker River valley, provide insights into the environmental dynamics of CWP (Figures 1B,C; Villa-Martínez et al., 2012; Henríquez et al., 2017; Iglesias et al., 2018). On one hand, Lago Edita and Lago Augusta records date back to Termination 1 (19,400 and 15,600 cal yrs BP, respectively) when a mosaic of cold-resistant hygrophilous conifers and rainforest trees along with alpine herbs developed in the Chacabuco valley (Villa-Martínez et al., 2012; Henríquez et al., 2017), followed by the development of a Nothofagus forest up to the present (Villa-Martínez et al., 2012). On the other hand, the Mallín Casanova record spans the last 9,950 cal yrs BP and revealed the development of Nothofagus/Pilgerodendron rainforests until the Late Holocene, when a major vegetation change led to the development of a more open forest in a grass steppe matrix (Iglesias et al., 2018). As recorded in several records from CWP, the Late Holocene presented vegetation changes of moderate magnitude related to an increased fire event frequency (Markgraf et al., 2007; de Porras et al., 2012; de Porras et al., 2014; Nanavati et al., 2019). This is synchronous with an increase in the human presence of hunter-gatherer groups who occupied the Chacabuco valley at least since 8,600 cal yrs BP, as indicated by a colonization process rooted in the extra-Andean steppes (Mena and Blanco, 2017; Borrero et al., 2019; Nuevo-Delaunay et al., 2022). CWP and supraregional archeological records show marked increases in the number of radiocarbon ages dating to the Late Holocene, which are indicative of human demographic growth and are supported by other archeological evidence (e.g., artifact deposition rates, changes in landscape use), suggesting greater anthropogenic pressure on the ecosystems (García Guraieb et al., 2015; Pérez et al., 2016).
As the geomorphological data indicate, the different glacial lobes in CWP may have undergone independent dynamics during Termination 1 and even well into the Holocene (e.g., Kaplan et al., 2004; Douglass et al., 2006; García et al., 2019; Thorndycraft et al., 2019; Davies et al., 2020; Leger et al., 2021). At the time that cold-resistant hygrophilous conifers and rainforest trees occurred in the Chacabuco valley up to 11,800 cal yrs BP, the Lago Cochrane–Pueyrredón valley was still occupied by a moraine-dammed lake. Hence, the environmental evidence could not be extrapolated to large spatial scales (e.g., other valleys) in CWP, since it may just provide a local and biased picture of past environments. Although landscapes in CWP (47°S) were still under configuration (e.g., proglacial lake drainage, Neoglacial advances) well into the Holocene, most paleoecological records fail to show changes, probably due to their location and sensitivity to track moderate vegetation changes. Thus, the present study aims to 1) reconstruct the environmental dynamics of the Lago Cochrane/Pueyrredón valley since 11,650 cal yrs BP by analyzing the first pollen and charcoal records in this valley, Laguna Maldonado and Laguna Anónima, two small, closed-basin lakes located in the forest-steppe ecotone (Figure 1C); 2) relate those environmental changes to the associated drivers (e.g., glacial/postglacial dynamics, climate, humans); and 3) discuss the results in a broader context in order to shed light on the Holocene environmental and climate dynamics of CWP (47°S). The location of Laguna Maldonado and Laguna Anónima at the forest-steppe ecotone in the Lago Cochrane/Pueyrredón valley implies that both pollen records are highly sensitive to track vegetation changes of moderate/slight magnitude associated with Holocene geomorphological, climatic, and/or potentially human-induced changes. The Laguna Maldonado record spans between 11,650 and 8,500 cal yrs BP, while the Laguna Anónima record encompasses the last 8,500 cal yrs BP; the records are correlated stratigraphically by the presence of the conspicuous Hudson volcano (H1) tephra layer (Stern et al., 2016).
Modern Setting
The landscape of Central West Patagonia has been modified by glacial action during the whole Quaternary and particularly since the LGM, when the PIS covered the whole region. At present, the Northern Patagonian Ice Field (Figure 1C) and other important but smaller glaciers remain after the PIS recession and the accelerated glacial retreat due to the ongoing global warming. The Lago Cochrane/Pueyrredón valley (47°S, Figure 1B) is a west–east oriented basin, once occupied by the Lago Cochrane/Pueyrredón ice lobe during the LGM, which reached its maximum eastward extension between 20,000 and 27,000 cal yrs BP (Río Blanco moraine; Hein et al., 2010). The onset of deglaciation of the Lago Cochrane/Pueyrredón ice lobe, as well as most ice lobes in the southern CWP (Glasser et al., 2012; Boex et al., 2013), is dated around 18,000 cal yrs BP (Hein et al., 2010); it was followed by an acceleration of ice thinning and recession that enabled the formation and growth of a large proglacial lake system called Lago Chalenko (e.g., Turner et al., 2005; Bell, 2008; Thorndycraft et al., 2019). Altitudinal, geochronological and isostatic shoreline data indicate that Lago Chalenko drained (at the Bayo level, 350 m a.s.l.) to the Pacific Ocean through the Baker valley between 12,000 and 10,000 cal yrs BP (Thorndycraft et al., 2019) when the Lago Cochrane/Pueyrredón valley was completely free of glacial influence. However, the Lago Cochrane/Pueyrredón valley landscape was similar to that at present ca. 8,000 cal yrs BP, given that a moraine-dammed lake (>120 m a.s.l.) prevailed in the valley until then, as suggested by glacial lake outburst flood (GLOF) records (e.g., flood bars) and stepped deltas (e.g., Turner et al., 2005; Thorndycraft et al., 2019).
The Lago Cochrane/Pueyrredón valley is under the yearlong influence of cold fronts associated with the Southern Westerlies system (SWs) that bring precipitation to southernmost western South America (Garreaud, 2009; Garreaud et al., 2013; Viale et al., 2019). As the cold fronts migrate from the Pacific coast to the east and are intercepted by the Andes, a rain shadow effect is produced by the forced subsidence of surface air masses, producing a steep west–east decreasing precipitation gradient (Garreaud et al., 2013; Viale et al., 2019). Vegetation distribution is conditioned by the W–E precipitation gradient from the Donatia fascicularis–dominated tundra and evergreen Nothofagus betuloides forests to the Nothofagus antarctica and Berberis ilicifolia shrubland and the Festuca pallescens and Mulinum spinosum steppe (Figure 1C) (Luebert and Pliscoff, 2017). Evergreen forests are dominated by Nothofagus betuloides and the deciduous Nothofagus pumilio along with Berberis ilicifolia, Gaultheria mucronata, and herbs in the understory strata. The Nothofagus antarctica and Berberis ilicifolia shrubland is a transitional vegetation unit between the forests and the grass steppes (forest-steppe ecotone), which has small (<2 m) Nothofagus trees along with shrubs such as Berberis microphylla, Ovidia andina, and Ribes magellanicum, herbs (e.g., Geranium berteroanum, Osmorhiza chilensis), and ferns (Blechnum penna-marina). The easternmost vegetation unit in this steep gradient is the grass steppe dominated by Festuca pallescens associated with Mulinum spinosum, Acaena splendens, and Baccharis patagonica.
Laguna Maldonado (LMA; 47,26°S; 72,51°W; 324 m a.s.l.; 207 ha; Figures 1D,E) and Laguna Anónima (LLA; 47,25°S; 72,37°W; 484 m a.s.l.; 7 ha; Figures 1D,F) are small closed lakes located at the western and southern margins of Lago Cochrane, respectively, and 10 km away from each other (Figures 1B–D). Both are located in the “ecotonal” N. antarctica shrublands, an ideal and extremely sensitive location to trace past precipitation changes based on vegetation (pollen record) changes. Given the proximity of the lakes along with their location in the same vegetation unit, their pollen records might be similar in vegetation and climatic terms.
Materials and Methods
Two independent series of overlapped cores were retrieved from the wetland areas located on the present shores of Laguna Maldonado (LMA0608B; 193 cm) and Laguna Anónima (LLA 0607A; 745 cm) using a modified Livingstone piston corer. The uppermost part of the Laguna Maldonado core was the present surface of the coastal wetland, underlied by the uppermost reported tephra layer in this paper that dates back to the middle Holocene (see Results; Stern et al., 2016). Laguna Anónima yielded a thick volcanic ash layer at 745 cm depth, which impeded coring deeper to a base (potentially glacial clays or an organic sedimentation contact) in this lake.
The sedimentary sequences of both cores were characterized by naked-eye lithological description and X-ray analysis. Loss-on-ignition analysis at 1–2 cm intervals was used to determine organic and inorganic (carbonate and clastic fraction) content (Bengtsson and Enell, 1986; Heiri et al., 2001). The main volcanic ash layers from both records were analyzed and published by Stern et al. (2016). Their origin and eruption were based on age, tephra glass color, morphology, mineralogy, and both bulk and glass chemical analysis (petrology, geochemical composition, and chronology; Stern et al., 2016).
The chronology of cores LMA0608B and LLA0607A was based on seven radiocarbon dates each (Table 1), which were calibrated with the Southern Hemisphere calibration curve (SHCal20; Hogg et al., 2020) using the CALIB 8.0.1 program (Stuiver et al., 2020). A Bayesian age–depth model was produced for each composite core with the “rbacon” package (Blaauw et al., 2021) in R platform (R core team, 2021). The basal age and the two uppermost ages of the LMA0608B core from Laguna Maldonado were excluded from the age–depth model. The basal age showed to be an outlier, considering the other ages. The two uppermost ages were excluded since they were younger than the basal H1 tephra layer age ∼7,663 ± 35 14C years, according to Stern et al. (2016). This is probably related to the coring site location and the fact that this is the uppermost part of the core, so it might have been contaminated by younger material from the modern surface of the shore of the lake. We included the average maximum (lake core) basal age of the H1 tephra layer (Stern et al., 2016) to build the Laguna Maldonado age–depth model. Adjusted depths were used to construct an age model assuming instantaneous deposition of tephra layers.
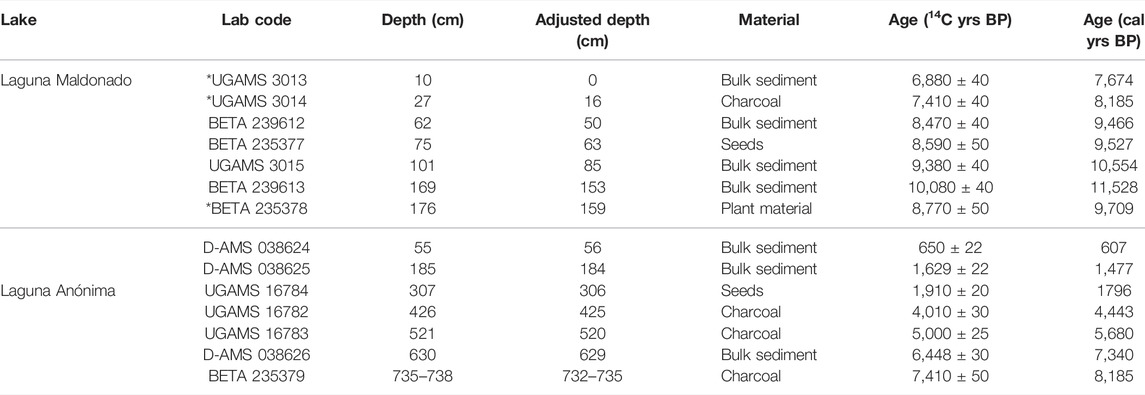
TABLE 1. AMS radiocarbon ages from cores LMA0608B and LLA 0607A from Laguna Maldonado and Laguna Anónima, respectively. The data marked with * were not included in the age–depth model (see Materials and Methods).
Pollen analysis was performed from 1 cm3 of sediment samples which were obtained at 4–5 cm intervals and treated with standard laboratory techniques for pollen extraction from the sediments (Faegri and Iversen, 1989). Tablets of the exotic spore Lycopodium clavatum were added to each sample to calculate pollen concentration (grains cm−3) (Stockmarr, 1971) and pollen accumulation rates (PARs; grains cm−2 yr−1). Pollen grains were identified at 400–1,000 × magnification under a light microscope. The basic pollen sum included at least 300 terrestrial pollen grains per sample. Pollen percentages of terrestrial taxa were based on the sum of trees, shrubs, herbs, and grasses. Aquatic/paludal and fern taxa were calculated based on a sum that included the basic pollen sum and the sum of aquatic and paludal taxa or the sum of ferns, respectively. The pollen diagram was divided into zones using unconstrained CONISS cluster analysis (Grimm, 1987), considering all local terrestrial pollen taxa >2%. The interpretation of LMA and LLA records was aided by comparison with the uppermost pollen samples of both records. The LMA superficial pollen assemblages have ∼45% of Nothofagus dombeyi type and ∼25–12% Poaceae along with Podocarpaceae, Fitzroya/Pilgerodendron, Saxegothaea conspicua, and Weinmannia trichosperma (forest); Escallonia and Ribes (understory); and Asteraceae subf. Asteroideae, Fabaceae, Chenopodiaceae, and Rumex (steppe) with percentages under 5% (data not shown).
Macroscopic charcoal particles (>125 um) were counted in both records to infer local fire history in the LLA and LMA sediment cores. 2 cm3 of the sediment was sampled at contiguous 1 cm intervals, deflocculated in 10% KOH, and sieved through a 125 μm screen (Whitlock and Larsen, 2001). The residual particles were counted in gridded Petri dishes using a stereomicroscope at 10–40 × magnification. The charcoal series of each core was interpolated at its median sample resolution (10 yrs sample−1 in LLA and 18 yrs sample−1 in LMA) using CHARanalysis software (Higuera et al., 2009). The interpolated charcoal accumulation rates (CHARinterpol; cm−2 yr−1) were separated into their background (CHARback) and peak accumulations (CHARpeak) (Long et al., 1998), which detects significant charcoal peaks interpreted as local fire events.
The series of CHARback of LLA and LMA were determined using a locally weighted regression (LOWESS) robust to outliers, using a window width of 1,000 years. This window selection allows maximizing the signal-to-noise index and the goodness-of-fit between the empirical and modeled noise distributions (Kelly et al., 2011). The CHARpeak values were calculated by subtracting CHARback from CHARinterpol, and their noise distributions were determined by a Gaussian mixture model using the 99th percentile threshold. The charcoal peaks that exceeded this threshold were considered fire events. Later, the fire peak magnitude (charcoal particles cm−2 peak−1) and fire event frequency (peaks 1,000 years−1) were estimated. Finally, the raw charcoal series of both sites (and their respective age–depth models) were homogenized using bootstrap resampling (min–max rescaling, Box–Cox, and Z-score transformation) to compute one composite curve (binned per 100 years) that was calculated with the “paleofire” R package (Blarquez et al., 2014) as an approach to biomass burning and to summarize the main fire trends through time in the study area.
Results
Lithology and Chronology
The Laguna Maldonado sequence of 193 cm depth is composed of laminated gray clays (193–172 cm) with low organic (2–9%) and carbonate (∼2%) content and high values of inorganic density (>1 g cm−3) (Figure 2A). Between 172 and 105 cm, the sequence presents a greenish-brown gyttja with variable values of organic matter up to 42% and a concomitant decline of inorganic density (0.15–0.3 g cm−3). From 105 to 10 cm, the occurrence of a brown organic gyttja is associated with the rise of organic matter (up to 90%) at the expense of inorganic density, which had its lowest values (<0.1 g cm−3), except for abrupt increments ranging from 0.5 to 1.1 g cm−3 related to volcanic ash layer deposition at the top 10 cm and a silty sand/sand layer observed at 76–81 and 28 cm. Carbonate percentages remained below 4% throughout the sequence, with minimum values from 80 cm to the top of the record.
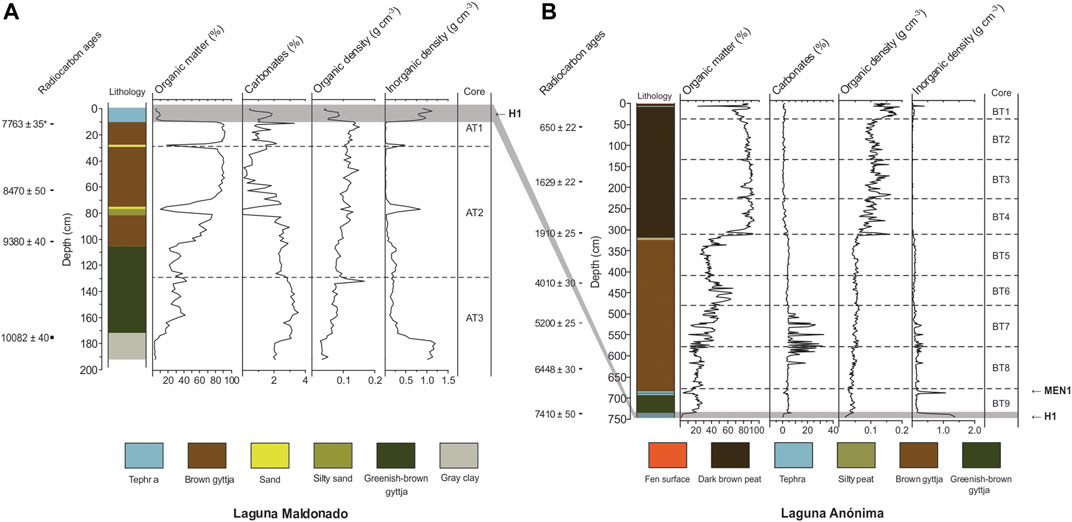
FIGURE 2. Radiocarbon ages, lithology, and loss-on-ignition results of (A) LMA0608B and (B) LLA 0607A cores. Letters represent tephra layers described by Stern et al. (2016), and dashed horizontal lines indicate the core boundaries.
The Laguna Anónima record is a 747 cm long core including a basal volcanic ash layer of 9 cm at the bottom with high inorganic density values (>1 g cm−3; Figure 2B). Between 738 and 689 cm, a layer of greenish-brown gyttja is present, with organic matter values that fluctuate between 21 and 25%. This is overlaid by a 2 cm tephra layer (689–687 cm). Between 687 and 325 cm, the sediment is brown gyttja with highly variable values of organic content (20–60%), particularly between 600 and 510 cm where peaks of carbonates reach up to 31%. A silty peat layer occurs between 325 and 319 cm, overlaid by a dark brown peat associated with a major increase of organic matter (from 30 to 90%) and density (0.5 to >1 g cm−3) from 319 cm to the top. Between 319 and 0 cm, dark brown peat shows low inorganic density (below 0.1 g cm−3), except for a volcanoclastic deposit at 5–6 cm.
Based on previous tephrochronological studies by Stern et al. (2016), the volcanic ash layers were attributed to different Holocene eruptions of the Hudson or Mentolat volcanos. Thus, the tephra layer at 687–689 cm in LLA corresponds to the MEN1 eruption (Mentolat volcano) dated at ca. 7,689 cal yrs BP. The tephra layers located in the top 10 cm of the LMA sedimentary sequences and at 736–747 cm of the LLA sedimentary sequences belong to the large explosive H1 eruption (Hudson volcano) dated ca. 8,440 cal yrs BP (median probability).
The record of LMA ranges from 11,650 to 8,500 cal yrs BP (Figure 3A; Table 1). The sediment deposition time is about 19 years cm−1 between 11,650 and 10,500 cal yrs BP and increases up to 25–40 yrs cm−1 between 10,500 and 9,700 cal yrs BP (Figure 4A). After 9,700 and up to 8,500 cal yrs BP, it dropped to 20 yrs cm−1. The sedimentary record of LLA covers the last 8500 cal yrs BP (Figure 3B; Table 1). The sediment deposition time overlying the H1 tephra layer gradually increased from 10 to 25 yrs cm−1 between 8,200 and 4,200 yrs cm−1 and remained relatively constant until 2,100 cal yrs BP (Figure 4B). During the last two millennia, the sedimentation rates declined and fluctuated around 5–14 yrs cm−1. This drop in deposition time is associated with a lithological shift from gyttja to peat that indicates the transition of the deposition environment from a lake to a wetland, given the core location at the current position of the lake’s shoreline.
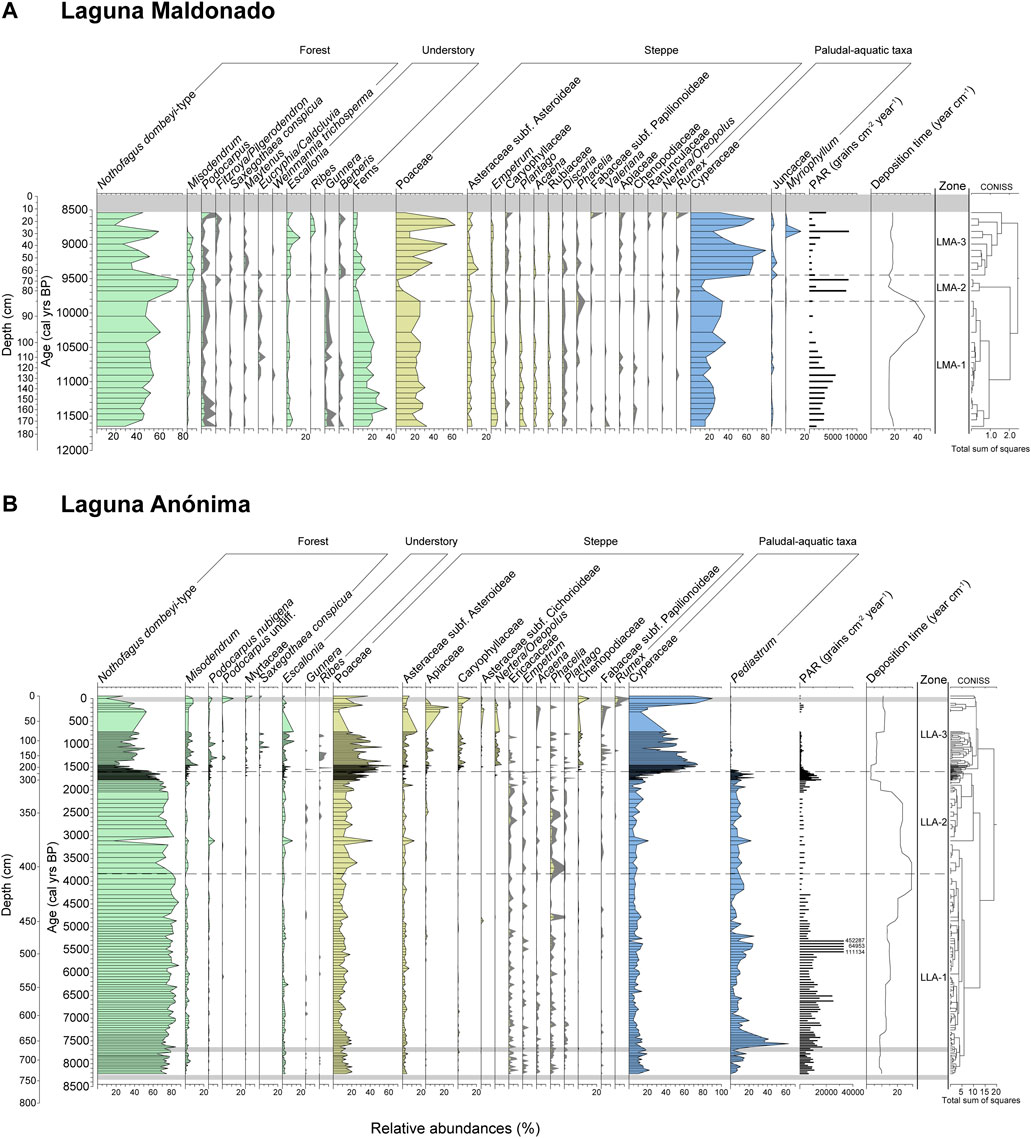
FIGURE 4. Pollen diagrams of (A) Laguna Maldonado and (B) Laguna Anónima in percentages including the total pollen accumulation rate (PAR; grains cm−3 year−1), the deposition time (year cm−1), and the CONISS analysis. Dashed lines denote the pollen zones, and gray horizontal lines denote the position of tephra layers. Gray shadows in some curves of pollen types indicate an exaggeration factor of 4x.
Pollen Records of Laguna Maldonado and Laguna Anónima
The pollen record of LMA (ca. 11,650–8,500 cal yrs BP, Figure 4A) is dominated mainly by Nothofagus dombeyi type and Poaceae. The cluster analysis divided the pollen assemblages into three major zones.
LMA-1 (174–85 cm; 11,650–9,830 cal yrs AP): It is dominated by Nothofagus dombeyi type which rapidly increases from 30 to 50% in the first 200 years of the record. Poaceae as secondary taxa fluctuates around 25%. Other forest taxa occur in traces, such as Podocarpus, Escallonia, and Gunnera. Poaceae and other steppe taxa represented by Asteraceae subf. Asteroideae, Rubiaceae, Acaena, Plantago, and Empetrum show percentages below 10%. Fern spores display the highest values (20–30%), whereas the paludal Cyperaceae remains around 30%.
LMA-2 (85–64 cm; 9,830–9,450 cal yrs BP): Nothofagus dombeyi type has the highest values (80–85%) in the record, while Misodendrum increases after 9,600 cal yrs BP. Poaceae declines to its lowest values, and the steppe taxa are only recorded in low abundance. Ferns and Cyperaceae also show their lowest values.
LMA-3 (64–11 cm; 9,450–8,500 cal yrs BP): It is characterized by a decline of Nothofagus dombeyi type (20–50%) and an increase of Poaceae, which fluctuates between 62 and 8%. Among the forest and understory taxa, Misodendrum remains stable, while Escallonia, Ribes, and ferns increase. Asteraceae subf. Asteroideae shows a slight increase (5–10%) similar to Juncaceae and Myriophyllum, whereas Cyperaceae reaches its highest values (up to 80%).
The total pollen accumulation rate (PAR) ranges from ∼1,500 to 5,500 grains cm3 yr−1 from 11,650 to 10,400 cal yrs BP (Figure 4A) with the highest rates for the whole record of pioneer and cold steppe taxa (Supplementary Figure S1). Around 9,700–9,500 cal yrs BP, peaks in PAR (up to 8,100 grains cm3 yr−1; Figure 4A) are mainly associated with a significant increase of Nothofagus dombeyi–type PAR (Supplementary Figure S1), resembling the percentage diagram. Between 9,400 and 7,700 cal yrs BP, the PAR is the lowest (<1,100 grains cm3 yr−1; Figure 4A) for the whole record except for two minor peaks at 8,800 and 8,500 cal yrs BP which are related to a generalized decrease in the PAR of most taxa (Supplementary Figure S1).
The record pollen of LLA encompassed the last 8,200 years and was divided into three zones, where the dominant taxon is Nothofagus dombeyi type, accompanied by Poaceae (Figure 4B).
LLA-1 (731–404 cm; 8,200–3,830 cal yrs BP): Nothofagus dombeyi type dominates the pollen assemblages (∼80%, with maxima around 7,200–3,830 cal yrs BP), whereas other forest and understory taxa values such as Misodendrum and Escallonia, respectively, are present under 5%. Poaceae (∼15%) followed by Asteraceae subf. Asteroideae (<10%) is the main representative of the steppe, while Ericaceae, Empetrum, Acaena, and Phacelia only occur in very low percentages (>3%) or have intermittent presence. Among the aquatic and paludal taxa, Cyperaceae and the non-pollen palynomorph Pediastrum show values below 20%. Nevertheless, Pediastrum displays two increments, around 7,600–7,100 cal yrs BP (up to 60%) and 5,600–5,100 cal yrs BP (up to 25%).
LLA-2 (404–236 cm; 3,830–1,600 cal yrs BP): It is characterized by a slight decrease of Nothofagus dombeyi type (∼70%) and the concomitant increase of Poaceae (20–40%). The other taxa remain with abundances similar to the previous zone. Nothofagus dombeyi type shows an abrupt decrease around 3,200 cal yrs BP, while Escallonia, Poaceae, and Asteraceae subf. Asteroideae increase.
LLA-3 (236–0 cm; last 1,600 cal yrs BP): Nothofagus dombeyi type shows an important and rapid decline (from 70% to 30%) followed by a slight increase up to 50% after 1,400 cal yrs BP but with highly fluctuating values. Poaceae pollen varies in an opposite way to Nothofagus dombeyi type, reaching maximum values above 60%. Misodendrum, Podocarpus nubigenus, and Saxegothaea conspicua increase their percentages, similar to steppe taxa such as Asteraceae subf. Asteroideae, Apiaceae, Caryophyllaceae, Asteraceae subf. Cichioroideae, and Nertera/Oreopolus. Exotic taxa such as Rumex (<10%) occur in the last ∼150 cal yrs BP of the record. Cyperaceae values rise close to 90%, while Pediastrum decreases almost to zero.
PAR values are highly variable, ranging between 3,700 and 450,000 grains cm3 yr−1 (Figure 4B), but are much higher than those in the LMA record. Nothofagus dombeyi type and Poaceae are the two most abundant pollen types in the total PAR throughout the record (Supplementary Figure S2). The PAR remains under 20,000 grains cm3 yr−1 from 8,200 to 4,500 cal yrs BP, interrupted by a major PAR peak between 5,600 and 5,200 cal yrs BP which is associated with a generalized PAR increase of most of the pollen types (Figure 4B; Supplementary Figure S2). Between 4,500 and 1900 cal yrs BP, the PAR remains stable around 5,000 grains cm3 yr−1, mostly associated with a steady PAR of Nothofagus dombeyi type (∼1,000–5,000 grains cm3 yr−1). Around 1900 cal yrs BP, a second minor peak in PAR is recorded, probably associated with a change in the deposition time as a consequence of the clogging of the shore of LLA (wetland). Nothofagus dombeyi type, ferns, Poaceae, and Asteraceae subf. Asteroideae show this peak in PAR individually (Supplementary Figure S2). A dramatic reduction in total PAR, as well as in the individual pollen type PAR, occurs around 1,600 cal yrs BP associated with the development of the wetland, implying a higher (lower) sedimentation rate (deposition time) (Figure 4B).
The Macro-Charcoal Particle Records of Laguna Maldonado and Laguna Anónima
The charcoal records of LMA and LLA show high variability on millennial and multi-centennial scales (Figure 5). The LMA record displays the highest CHAR around 11,650–10,500, 9,700–9,500, and 8,800–8,500 cal yrs BP (Figure 5A), while the highest CHAR values in LLA occur around 8,200–7,500 cal yrs BP, 6,600 cal yrs BP, and 1,100–700 cal yrs BP (Figure 5B). The global signal-to-noise index of LMA and LLA records reaches 5.8 and 6.6, respectively, indicating that both records are suitable for peak detection (Kelly et al., 2011).
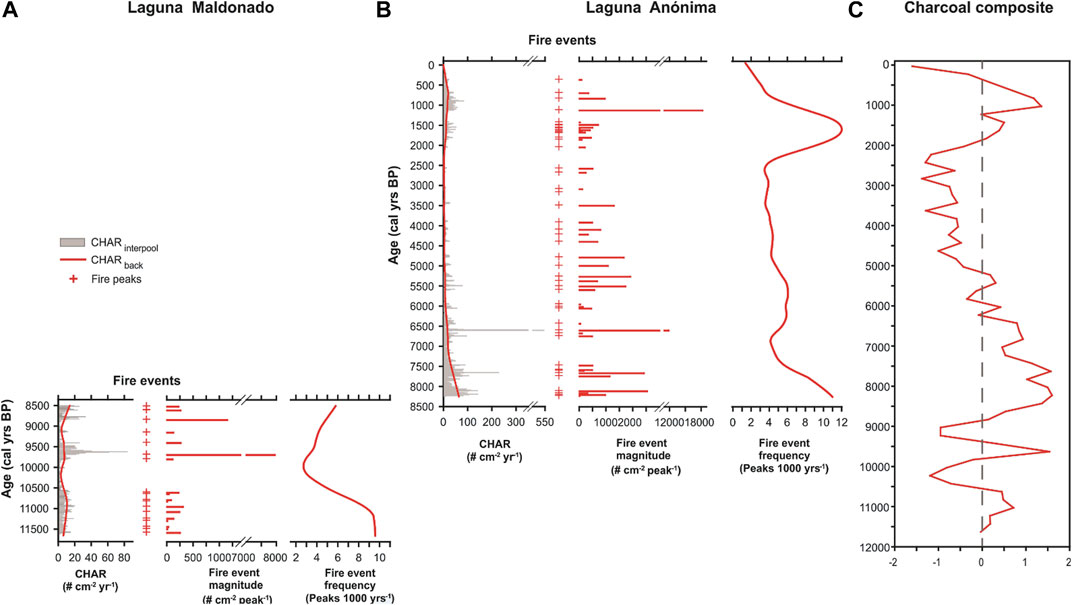
FIGURE 5. Charcoal accumulation rates (CHARinterpol and CHARback), inferred fire events, fire peak magnitudes, and fire event frequency of (A) Laguna Maldonado and (B) Laguna Anónima. (C) Composite curve of the charcoal series of Laguna Maldonado and Laguna Anónima records summarizing biomass burning over time. The gray shadow indicates the 95% confidence interval.
Eighteen significant fire events were inferred in the LMA record (11,700–8,500 cal yrs BP). Periods with high fire event frequency are around 11,700–10–500 cal yrs BP and after 9,700 cal yrs BP (4–9.5 fire events 1,000 years−1), whereas low incidence occurs between 10,500 and 9,700 cal yrs BP. The largest fires may have occurred around 9,700 cal yrs BP, where a fire event magnitude reached up to 8,000 particles cm−2 peak−1.
In the LLA record (8,500 cal yrs BP), 47 significant fire events were inferred, reaching the highest frequency prior to 7,500 cal yrs BP, and between 2000 and 1,000 cal yrs BP (up to 12 fire events 1,000 years−1). In the last millennia, the decline of CHAR values and the fire frequency suggest that fire activity decreases. The maximum fire peak magnitudes occur around ∼8,200–7,600, ∼6,600, 5,500–4,800, and ∼1,100 cal yrs BP, reaching up to 18,400 particles cm−2 peak−1 in the last one.
The composite charcoal curve summarizes the main patterns of the charcoal deposition of the LMA and LLA records that can be interpreted as biomass burning over time (Figure 5C). Positive trends of biomass burning are noted at ∼11,650–10,500, ∼9,500, ∼8,700–5,100, and 1800–600 cal yrs BP, intercalated with periods with negative values at ∼10,500, ∼9,300–8,700, and 5,100–1900 cal yrs BP and during the last 600 years.
Discussion
Paleoenvironmental Interpretation
The pollen and charcoal records of Laguna Maldonado and Laguna Anónima (Figures 4A,B, 5A–C) provide a quite complete picture of the paleoenvironmental dynamics of the Lago Cochrane/Pueyrredón valley during the Holocene, once the westernmost area of Lago Cochrane was free of ice influence. Once the Chalenko lake was drained through the Baker River valley (<350 m a.s.l.), organic sedimentation began in Laguna Maldonado (∼324 m a.s.l.) around 11,650 cal yrs BP, as reflected by the transition between lacustrine gray clays and greenish-brown gyttja (Figure 2A). Between 11,650 and 10,500 cal yrs BP, an open Nothofagus forest developed, as suggested by the percentages of Nothofagus dombeyi type reaching up to 50% associated with Escallonia, Berberis, and ferns in the understory (Figure 4A). This forest was intermingled with a cold steppe dominated by Poaceae along with Empetrum, Acaena, Plantago, and Rubiaceae, most pioneer and/or shade-intolerant species, indicating primary colonization stages of new ecological niches in the postglacial landscapes (de Porras et al., 2012; Markgraf et al., 2007; Moreno, 2020, Heusser, 2003). This vegetation assemblage suggests colder conditions than present, although under similar precipitation. The high CHAR values, frequency fire events, and positive anomaly of the CHAR composite curve (Figure 5A) are synchronous with relatively high PAR values between 11,650 and 10,400 cal yrs BP (Supplementary Figure S1), which suggests an increment of burnable fuel but also a marked dry season (precipitation seasonality), hence ideal conditions for fire to occur in ecotone areas (Holz and Veblen, 2012). From 10,500 to 9,800 cal yrs BP, Nothofagus dombeyi–type percentages increase slightly, while Escallonia, Gunnera, and fern values decrease along with cold steppe taxon percentages (Figure 4A), thus suggesting a transitional phase to a more closed forest associated with a shift to low fire activity (Figure 5A). Thus, the pollen record suggests the onset of an increasing precipitation trend synchronous with a temperature increase up to those similar to the present values, while the charcoal record points to a reduction in seasonality.
By 9,800 cal yrs BP and up to 9,400 cal yrs BP, an increase in Nothofagus dombeyi–type percentages at the expense of understory pollen types (Escallonia, Gunnera, fern spores), Poaceae, and Empetrum indicates the development of a closed Nothofagus forest which is followed by an increment of fire activity at 9,600–9,400 cal yrs BP. This may suggest a rise in precipitation (even over modern values) with a completely reduced seasonal and/or interannual (wet winters and dry summers) variability that may have promoted the colonization of Nothofagus species after fire disturbances (e.g., Mallín El Embudo, de Porras et al., 2014). Locally, the decrease in Cyperaceae values (<20%) supports the latter, since a yearlong high lake level would have reduced coastal areas to be colonized by species of this family (Figure 4A; Heusser, 2003; de Porras et al., 2012).
Between 9,400 and 8,500 cal yrs BP, a decrease of Nothofagus dombeyi–type percentages (even under the present ones) along with highly variable values associated with an increase of understory taxon percentages such as Escallonia and Ribes and the rise of the values of the exclusive Nothofagus parasite Misodendrum (more frequent in periodically disturbed forests; Haberle et al., 2000) as well as steppe pollen types such as Poaceae and Asteraceae subf. Asteroideae (Figure 4A) suggest not just an open forest but probably scattered patches of forest of variable size developed in a steppe matrix. This may indicate a reduction in precipitation except for a short period between ∼8,900 and 8,800 cal yrs BP when Nothofagus dombeyi–type percentages increased. The latter might be indicating a reversal in moisture availability (precipitation), similar to the period between 9,800 and 9,400 cal yrs BP associated with high charcoal accumulation rate and the deposition of clastic layers. Cyperaceae percentages support both inferences (Figure 4A): first, the reduction in precipitation as paludal vegetation is favored by larger coastal areas, and second, the 200-year precipitation increase is reflected as a Cyperaceae percentage decrease due to a reduction of areas around the lake to be colonized. However, it is key to highlight that the western Lago Cochrane/Pueyrredón valley was still undergoing large landscape transformations related to the postglacial and Neoglacial processes. On the one hand, three moraine-dammed lakes that occupied the Lago Cochrane/Pueyrredón valley collapsed, as shown by the GLOFs and their associated geoforms as flood bars, between ∼11,700 and 8,000 cal yr BP (Thorndycraft et al., 2019), while Neoglacial advances were recorded ∼10,900 ± 1,300 and ∼7,900 ± 1,100 years BP in the Lago General Carrera/Buenos Aires basin (Bourgois et al., 2016). On the other hand, the Lago Cochrane/Pueyrredón valley and most CWP experienced the fall of volcanic ash of two explosive eruptions from the Mentolat (MEN1) and Hudson (H1) volcanos (Stern et al., 2016). Since the opening of the forest predates either glacial or volcanic processes, it is likely that this change responds to a climatic forcing (precipitation decrease), though it cannot be excluded that the high variability of pollen assemblages (and therefore vegetation) during this time is associated with a combination of forcings. The high fire activity between 9,400 and 8,500 cal yrs BP is just based on the fire frequency but not on biomass burning due to the low magnitude of fire events.
Unlike the record of Laguna Maldonado (LMA), Laguna Anónima (LLA) reflects the development of a closed Nothofagus forest at least from 8,200 until 3,800 cal yrs BP, as suggested by steady percentages of Nothofagus dombeyi type around 70–80% (Figure 4B). It is likely that the location of LLA 10 km eastward and 150 m higher in altitude than LMA (Figure 1B) provided more stable environmental conditions and lesser magnitude geomorphological changes than those occurring further west in the Lago Cochrane/Pueyrredón valley; hence, vegetation was less disturbed. Besides, ash fall of both explosive eruptions (MEN1 and H1) did not affect vegetation in any significant manner around LLA (Figure 4B). Thus, the presence of a closed Nothofagus forest around LLA associated with low fire occurrence frequency, particularly between 7,100 and 3,800 cal yrs BP, suggests higher than present precipitation and reduced seasonality.
Since 3,800 cal yrs BP, a slight decrease in Nothofagus dombeyi–type percentages (65–50%) and the concomitant increase of Poaceae indicate a gradual opening of the forest up to 1,600 cal yrs BP, when a major change is recorded at both the local (basin) and regional scales (Figure 4B). The coastal areas of Laguna Anónima became a wetland, as revealed by the changes in lithology (shift to peat sediment), organic matter percentages (80%; Figure 2B), and the Cyperaceae (Pediastrum) percentages increasing (decrease) up to 70% (almost 0%) (Figure 4B). A dramatic increase of Poaceae and other steppe taxa (Asteraceae subf. Asteroideae, Apiaceae, and Caryophyllaceae) at the expense of Nothofagus dombeyi type from 1,600 up to 150 cal yrs BP (Figure 4B) occurred later, associated with the highest fire occurrence frequency for the whole record. Even though there is synchronicity of local and regional vegetation changes, we think it has no relationship to the changes in the deposition environment, as it is supported by the PAR results (Supplementary Figure S2), as well as similar and synchronous changes recorded in other sites of CWP (Iglesias et al., 2018; de Porras et al., 2014; see Regional Discussion). This implies the development of a highly dynamic open forest or forest patches scattered in a grass/shrub–grass steppe matrix, suggesting a decrease in precipitation but under strong seasonality, as revealed by the high fire occurrence frequency.
The last 150 cal yrs BP reflects a decrease in Nothofagus dombeyi type, interpreted as Euro-Chilean settlement–related changes as a consequence of logging and/or opening areas for cattle breeding as well as the appearance of Rumex, an exotic and invasive herb, related to the introduction of livestock (Figure 4B; Cárdenas et al., 1993; Martinic, 2005).
Regional Discussion
After the Lago Cochrane/Pueyrredón ice lobe reached its LGM extension into the steppe around 20,000–27,000 cal yrs BP, as indicated by the Río Blanco moraines, the deglaciation of this ice lobe began at around 20,000–18,000 cal yrs BP (Figure 6A; Hein et al., 2009; Hein et al., 2010; Hein et al., 2011, Thorndycraft et al., 2019). Consequently, the Chacabuco and Lago Cochrane/Pueyrredón valleys experienced ice thinning and recession and the development of large proglacial lakes that drained to the Atlantic above 500–600 m a.s.l. (Stage 1; Figure 6A; Thorndycraft et al., 2019). Once proglacial lakes ceased to inundate deglaciated areas, a mosaic of cold-resistant hygrophilous conifers and rainforest trees and a scrubland dominated by Poaceae and accompanied by cold steppe taxa developed above the modern tree line in the Chacabuco valley (570 m a.s.l.) between 19,000 and 16,800 cal yrs BP (Figure 6B; Henríquez et al., 2017). Similar vegetation was recorded around Lago Augusta by 16,000–15,000 cal yrs BP (Figure 6C; Villa-Martínez et al., 2012) when the Atlantic drainage of the proglacial lakes in the Chacabuco and Lago Cochrane/Pueyrredón valleys (∼460 m a.s.l.) was abandoned and the ice front was westward of the easternmost part of both valleys (Stages 2 and 3; Figure 6A; Thorndycraft et al., 2019). By 15,000 cal yrs BP, the Barrancos spillway opened (440 m a.s.l.) and the proglacial lakes drained to the Pacific Ocean through the Baker River valley (Stage 4; Figure 6A; Thorndycraft et al., 2019). After glaciers re-advanced during the Antarctic Cold Reversal (ACR; Stage 5; Figure 6A), a large proglacial lake called Lago Chalenko formed, inundating the Lago General Carrera/Buenos Aires, Chacabuco, and Lago Cochrane/Pueyrredón basins below 350 m a.s.l. between 14,200 and 12,600 cal yrs BP (Stage 6; Figure 6A; Davies et al., 2018; Thorndycraft et al., 2019). The mosaic of hygrophilous Fitzroya/Pilgerodendron conifers and scrubland prevailed in the Chacabuco valley under millennial-scale fluctuations until 11,800 cal yrs BP (Figures 6B,C; Villa-Martínez et al., 2012; Henríquez et al., 2017) even though no vegetation changes occurred synchronous to the ACR and/or the Lago Chalenko formation.
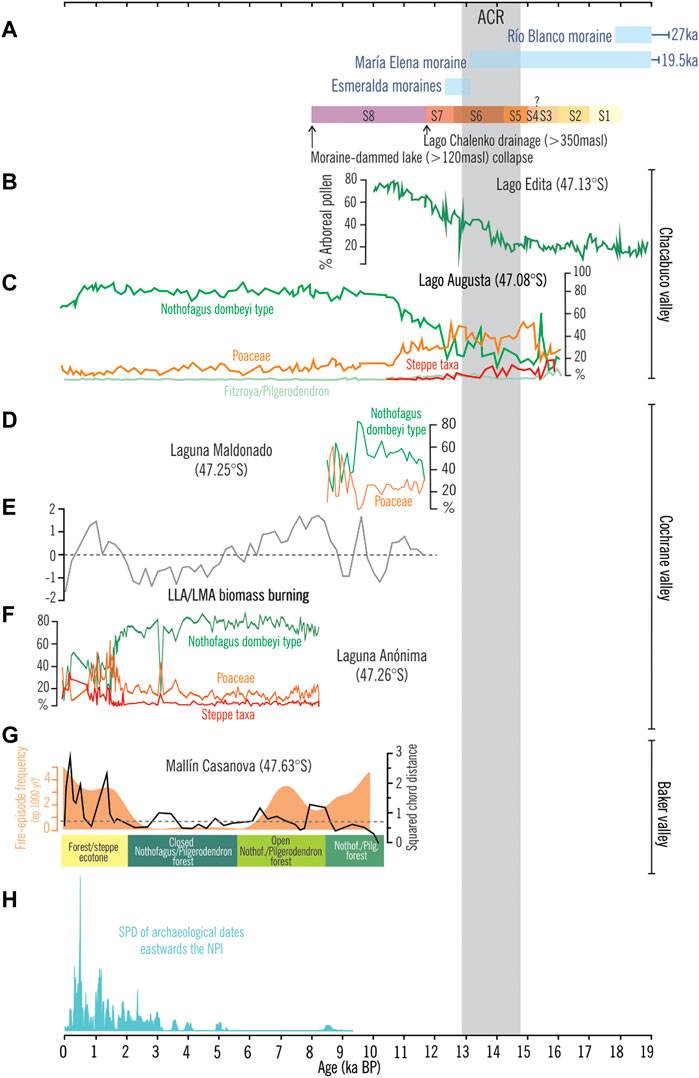
FIGURE 6. Integration of selected paleoenvironmental data from CWP since the LGM showing (A) the chronology of the Chacabuco and Lago Cochrane/Pueyrredón main moraines and the deglaciation stages of Lago Buenos Aires/General Carrera and Lago Cochrane/Pueyrredón proposed by Hein et al., 2009, 2010; Villa-Martínez et al., 2012; Boex et al., 2013; Turner et al., 2005; Glasser et al., 2012; Davies et al., 2018, 2020; Thorndycraft et al., 2019; (B) Lago Edita arboreal pollen percentages (Henríquez et al., 2017); (C) Lago Augusta summarized pollen diagram (Villa-Martínez et al., 2012); (D) a summarized pollen diagram (%) of Laguna Maldonado; (E) raw composite charcoal series as a proxy of biomass burning from LMA and LLA; (F) a summarized pollen diagram (%) of Laguna Anónima; (G) Mallín Casanova vegetation changes, fire-episode frequency, and square chord distance as a proxy of vegetation change magnitude (Iglesias et al., 2018); (H) summed probability distribution curve of archeological 14C ages from the eastern side of the NPI (Nuevo Delaunay et al., 2022).
The drainage of Lago Chalenko (at the Bayo level, 350 m a.s.l.) to the Pacific Ocean through the Baker valley occurred around 12,000–10,000 cal yrs BP (Stage 7; Figure 6A; Thorndycraft et al., 2019). After 11,800 and up to 9,800 cal yrs BP, a dense Nothofagus forest established at the expense of the decline in hygrophilous and cold-resistant trees, herbs, and shrubs associated with high fire frequency in the Chacabuco valley (Figures 6B,C; Villa-Martínez et al., 2012; Henríquez et al., 2017). In the Lago Cochrane/Pueyrredón valley, organic sedimentation began in Laguna Maldonado at 11,650 cal yrs BP when an open Nothofagus forest intermingled with a cold steppe (Figure 6D) developed up to 10,400 cal yrs BP, suggesting colder conditions but similar precipitation to the present. These colder conditions could be related to a glacial re-advance recorded in CWP (Bourgois et al., 2016; Davies et al., 2020; Glasser et al., 2012; Harrison et al., 2012). The pollen record of Laguna Maldonado also shows low percentages (<5%) of hygrophilous and cold-resistant trees (e.g., Podocarpus, Fitzroya/Pilgerodendron) similar to that recorded in the Chacabuco valley during Termination 1 (18,000–11,000 cal yrs BP). However, based on the comparison of the LMA pollen assemblages and modern pollen assemblages from westernmost CWP rainforests (Haberle and Bennett, 2001), we interpret the low percentages of hygrophilous and cold-resistant trees as indicating azonal conditions restricted to the border of the lake rather than reflecting zonal vegetation, and/or long-distance dispersal of pollen grains from rainforests located westward (Haberle and Bennett, 2004). The occurrence of positive anomalies of CHAR in the Lago Cochrane/Pueyrredón (Figure 6E) and Chacabuco valleys between ca. 11,700–10,500 and 11,800–9,800 cal yrs BP, respectively, is synchronous with a shift in the fire regime of CWP (de Porras et al., 2012, 2014; Nanavati et al., 2019) and most Patagonian records south of 40°S (e.g., Markgraf et al., 2007; Whitlock et al., 2007; Abarzúa and Moreno, 2008; Power et al., 2008; Nanavati et al., 2019; Villa-Martínez and Moreno, 2021). This may have been related to the increase in Nothofagus abundance, as well as to warmer winters which extended the growing season and effectively drier summers which increased fire activity (Renssen et al., 2005; Whitlock et al., 2007). This environmental setting occurs broadly in southern most South America, linked to a decline of the SWs wind strength between ∼11,300 and 7,500 cal yrs BP (Moreno et al., 2021).
It was not until 8,000 cal yrs BP that the landscape of the Lago Cochrane/Pueyrredón valley became similar to the present, given that a moraine-dammed lake (>120 m a.s.l.) developed and collapsed sometime between 11,000 and 8,000 cal yrs BP (Stage 8; Figure 6A; Thorndycraft et al., 2019). A transitional phase to a closed forest and the associated fire regime shift to low fire activity that occurred from 10,500 to 9,800 cal yrs BP in the Lago Cochrane/Pueyrredón valley (Figures 6D,E), while closed Nothofagus forests were established in the Chacabuco and Coyhaique valleys showing little variation from 9,800 and 9,400 cal yrs BP to recent, respectively (Figures 6B,C; Villa-Martínez et al., 2012) (Villa-Martínez and Moreno, 2021). Unlike the Chacabuco and Coyhaique valleys, the Laguna Maldonado record suggests the development of an open forest or probably scattered patches of forest of variable size in a steppe matrix between 9,400 and 8,500 cal yrs BP, probably related to climatic forcings and alternatively geomorphological and paraglacial dynamics. The Laguna Anónima record, located at a more distal position regarding the periglacial influence, indicates the development of a closed-canopy Nothofagus forest (peaking ∼7,100 cal yrs BP) and low fire occurrence frequency from ∼8,250 to 3,800 cal yrs BP. Further south and west, alternating open-to-closed evergreen Nothofagus and Pilgerodendron forests developed around Mallín Casanova since the Early Holocene to 2000 cal yrs BP without major changes (Figure 6G; Iglesias et al., 2018). This sustained forest development since the Early Holocene to the Late Holocene associated with a low fire occurrence/frequency was recorded regionally in CWP and was related to increased precipitation but under reduced seasonality (Markgraf et al., 2007; de Porras et al., 2012; de Porras et al., 2014; Nanavati et al., 2019; Villa-Martínez and Moreno, 2021). Neoglacial advances do not seem to have strongly affected the dynamics of vegetation, except for a strong increase of PAR in Laguna Anónima between ∼5,500 and 5,000 yrs BP, which may be an expression of a more dense forest, consistent with glacial advances recorded in CWP and South West Patagonia (Davies et al., 2020; Harrison et al., 2012; Kaplan et al., 2016; Sagredo et al., 2018). These Neoglacial advances were associated with a strengthening of the Southern Westerlies winds and a negative phase of SAM-like to millennial–submillenial time scale (Kaplan et al., 2016; Kaplan et al., 2020). A mild decreasing trend in Nothofagus percentages began at 3,800 cal yrs BP around Laguna Anónima, followed by a major change from a closed to an open Nothofagus forest peaking at 1900–1,600 cal yrs BP (Figure 6F) associated with an increased fire occurrence/frequency and magnitude since 2000 cal yrs BP (Figure 6E). A synchronous vegetation and fire regime change occurred around Mallín Casanova, where a closed Nothofagus/Pilgerodendron forest changed to a forest/steppe ecotone, also associated with a rise in the fire occurrence frequency (Figure 6G; Iglesias et al., 2018), which suggests regional (rather than local) forcing as a driver of such important vegetation changes. Late Holocene changes were also recorded in other sites of CWP at 44°S, with retraction of Nothofagus forest and steppe expansion. Forest around Lago Shaman began retraction at 3,000 cal yrs BP, peaking between 2000 and 1,500 cal yrs BP, while the Mallín El Embudo record shows forest retraction between 4,200 and 2000 cal yrs BP (de Porras et al., 2012, 2014). At 47°S, La Frontera shows a Nothofagus forest retraction between 4,000 and 2,100 cal yrs BP (McCulloch et al., 2017). Other records, such as Lago Augusta (47°S) (Figure 6C; Villa-Martínez et al., 2012), Lago Mellizas and Lago Churrasco at 45°S (Moreno et al., 2019; Villa-Martinez and Moreno, 2021), do not show any vegetation changes until recent times (last 150 cal yrs BP) when the European settlement started in this part of Patagonia (Nuevo-Delaunay, 2015).
The fact that some records show Late Holocene vegetation changes while some others fail to do so could be related to their location and/or the sensitivity of the surrounding vegetation to respond to moderate magnitude and/or sudden climatic changes. Late Holocene vegetation and fire regime changes could be the result of a summer insolation anomaly increase regarding the present levels during the Late Holocene (Alder and Hostetler, 2015; Nanavati et al., 2019), superimposed on an intensified seasonal and/or interannual precipitation variability, and therefore highly variable Southern Westerlies winds, in response to the increased frequency at annual to secular timescale of the Southern Annular Mode-like (SAM-like) conditions as reported at the regional scale for Sudamerica (Dätwyler et al., 2018), James Ross Island (58°S; Abram et al., 2014), and at the local scale for Lago Cipreses (49°S; Moreno et al., 2014; Moreno et al., 2018).
The pollen record of Laguna Anónima does not show conclusive evidence related to glacial advances in the Late Holocene which have been recorded along Patagonia, especially between ∼2000–1,000 cal yrs BP and ∼500–200 cal yrs BP (e.g., Davies et al., 2020 and references therein). Those advances were of a lesser magnitude and extension than those that occurred during the Early Holocene and Mid-Holocene. Thus, pollen records within the forest such as Lago Augusta (Villa-Martínez et al., 2012) and Lago Mellizas (Villa-Martinez and Moreno, 2021) or near the modern forest-steppe ecotone such as Lago Shaman (de Porras et al., 2012), Mallín El Embudo (de Porras et al., 2014) and Laguna Anónima are not sensitive to variations of this magnitude. However, records such as La Frontera (McCulloch et al., 2017), in the forest-steppe ecotone but very close to mountains up to 2000 m high, and Mallín Casanova (Iglesias et al., 2018) in the Baker River watershed very close to NPI show changes in concordance with the main Neoglacial advances occurred during the Late Holocene.
Similar to the glacial advances/retreats, changes in vegetation have been associated with greater or lesser influence of the SWs (e.g., de Porras et al., 2012; Glasser et al., 2012; Kaplan et al., 2016; Davies et al., 2020; Villa-Martinez and Moreno, 2021). However, the changes recorded are very dissimilar throughout the latitudinal range of Southern Westerlies’ influence, from subtropical to Antarctic zones (e.g., Villa-Martínez et al., 2003; Maldonado and Villagrán, 2006; Moreno et al., 2018; Nanavati et al., 2019; Davies et al., 2020; Kaplan et al., 2020). Particularly in Patagonia, the differences between records have been associated with latitudinal expansions or retractions of the SWs, greater or lesser strength of the SWs, and/or latitudinal displacement of this climatic belt (e.g., Villa-Martínez et al., 2012; de Porras et al., 2014; Quade and Kaplan, 2017; Moreno et al., 2019). Our records, like most CWP pollen records, show changes in pollen assemblages associated with greater or lesser influence of SWs, with greater influence of SWs during times of expansion of the forests. In this sense, the differences with other records of this nature in CWP (e.g., Markgraf et al., 2007; de Porras et al., 2012; Villa-Martínez et al., 2012; Henríquez et al., 2017; McCulloch et al., 2017; Nanavati et al., 2019; Villa-Martínez and Moreno, 2021) are related with a different sensitivity of the records. Thus, a more complete view of the behavior of SWs, particularly during the Holocene, where the magnitude of change is smaller than during the last glacial termination, requires the integration of information from different records at the regional scale.
Besides to the climatic forcing, the archeological record eastward the Northern Patagonian Ice Field reveals a marked increase in dated occupational events, indicating a change from a discontinuous human presence from 8,600 to 3,000 cal yrs BP to a continuous one from 3,000 cal yrs BP onward (Figure 6H; Nuevo-Delaunay et al., 2022). This trend has been acknowledged for Late Holocene archeological records of the wider west central Santa Cruz area (Argentina), encompassing several neighboring basins to the east of the study area (Sacchi et al., 2016; Goñi et al., 2019; Mengoni Goñalons et al., 2019). Groups residing more permanently in the eastern steppes have been interpreted as part of the same mobile populations occupying western valleys less intensely, for instance, as indicated by the use of geochemically traced toolstones procured in eastern sources (Borrero, 2004; Méndez et al., 2018). Thus, humans occupying the broader area cannot be discarded as potential ignition agents forcing vegetation change, as has been suggested for other valleys in CWP (Méndez et al., 2016).
Conclusion
The Laguna Maldonado and Laguna Anónima lake sediment cores are the first paleoenvironmental records from the Lago Pueyrredón/Cochrane valley. They provide key evidence to constrain the deglaciation dynamics of CWP. The contact of glacio-lacustrine clays and organic sediments in the Laguna Maldonado record indicates a minimum age for the drainage of Lago Chalenko (below 324 m a.s.l.) through the Baker River valley at the onset of the Holocene.
The establishment of open Nothofagus forests associated with cold steppes between 11,650 and 10,400 cal yrs BP followed by a transitional phase to closed Nothofagus forests by 10,400–9,400 cal yrs BP suggests increasing trends in temperature and precipitation up to 10,400 and 9,400 cal yrs BP, respectively. Centennial-scale fluctuating scattered patches of Nothofagus forest of variable size in a steppe matrix from 9,400 to 8,500 cal yrs BP were related to geomorphological and paraglacial dynamics as well as climatic forcing. The Laguna Anónima record, located in an upper/eastward and therefore more stable position, indicates the development of a closed Nothofagus forest and low fire occurrence frequency from 8,200 to 3,800 cal yrs BP, thus suggesting higher than present precipitation and reduced seasonality. Since 3,800 cal yrs BP, a decreasing trend in Nothofagus percentages is recorded, followed by a major change from a closed to an open Nothofagus forest that peaked between 1900 and 1,600 cal yrs BP, associated with increased fire occurrence frequency and magnitude since 2000 cal yrs BP. The synchronicity and direction of Late Holocene vegetation changes in the Lago Cochrane/Pueyrredón valley with other records from CWP reveal a regional climatic driver, probably associated with a summer insolation anomaly increase superimposed on SAM-like short-scale climatic variability. However, the synchronous sudden increase of local/regional hunter-gatherer presence since 3,000 cal yrs BP led us to hypothesize that humans could have also played a key role in the fire regime and vegetation changes.
To conclude, our results indicate that far from being stable, the western Lago Cochrane/Pueyrredón valley was still undergoing large landscape transformations during the Early-to-Middle Holocene and that these were related to the last stages of deglaciation associated with the dynamics of the SWs. It is key therefore to continue pursuing the search for sensitive sites, for instance, sites revealing changes of lesser intensity than those of the Holocene-Pleistocene transition, to show a robust and complete picture of landscape and environmental and climate change, and to shed light on the past scenarios of CWP since the Last Glacial Maximum.
Data Availability Statement
The original contributions presented in the study are included in the article/Supplementary Material, and further inquiries can be directed to the corresponding author.
Author Contributions
AM designed the study, performed fieldwork, obtain the funding and wrote the manuscript with contributions from all coauthors. MEdP and AM-C analyzed the data and participated in writing and editing the manuscript. OR designed the study, performed fieldwork and contributed with archaeological data and ideas. CM and AN-D obtained funding and participated in writing and editing the manuscript.
Funding
Funded by ANID FONDECYT 1180306; ANID FONDECYT 1210042; ANID FONDECYT 1210045; ANID Regional R20F0002; National Geographic Grant HJ-150R-17.
Conflict of Interest
The authors declare that the research was conducted in the absence of any commercial or financial relationships that could be construed as a potential conflict of interest.
Publisher’s Note
All claims expressed in this article are solely those of the authors and do not necessarily represent those of their affiliated organizations, or those of the publisher, the editors, and the reviewers. Any product that may be evaluated in this article, or claim that may be made by its manufacturer, is not guaranteed or endorsed by the publisher.
Acknowledgments
To Varyl Thorndycraft for Figure 1A, Dra. Ana M. Abarzúa for fieldwork assistance, Francisco Mena and Víctor Lucero.
Supplementary Material
The Supplementary Material for this article can be found online at: https://www.frontiersin.org/articles/10.3389/feart.2022.833637/full#supplementary-material
References
Abarzúa, A. M., and Moreno, P. I. (2008). Changing Fire Regimes in the Temperate Rainforest Region of Southern Chile over the Last 16,000 Yr. Quat. Res. 69, 62–71. doi:10.1016/j.yqres.2007.09.004
Abram, N. J., Mulvaney, R., Vimeux, F., Phipps, S. J., Turner, J., and England, M. H. (2014). Evolution of the Southern Annular Mode during the Past Millennium. Nat. Clim Change 4, 564–569. NATURE. doi:10.1038/NCLIMATE2235
Alder, J. R., and Hostetler, S. W. (2015). Global Climate Simulations at 3000-year Intervals for the Last 21 000 Years with the GENMOM Coupled Atmosphere-Ocean Model. Clim. Past 11, 449–471. doi:10.5194/cp-11-449-2015
Bell, C. M. (2008). Punctuated Drainage of an Ice‐dammed Quaternary lake in Southern South america. Geografiska Annaler: Ser. A, Phys. Geogr. 90, 1–17. doi:10.1111/j.1468-0459.2008.00330.x
Bendle, J. M., Thorndycraft, V. R., and Palmer, A. P. (2017). The Glacial Geomorphology of the Lago Buenos Aires and Lago Pueyrredón Ice Lobes of central Patagonia. J. Maps 13, 654–673. doi:10.1080/17445647.2017.1351908
Bengtsson, L., and Enell, M. (1986). “Chemical Analysis,” in Handbook of Holocene Palaeoecology and Palaeohydrology. Editor B. E. Berglund (Chichester: Wiley), 423–445.
Blaauw, M., Christen, J. A., and Aquino-López, M. A. (2021). Rbacon: Age-Depth Modelling Using Bayesian Statistics. R package version 2.5.7. https://CRAN.R-project.org/package=rbacon.
Blarquez, O., Vannière, B., Marlon, J. R., Daniau, A.-L., Power, M. J., Brewer, S., et al. (2014). Paleofire: An R Package to Analyse Sedimentary Charcoal Records from the Global Charcoal Database to Reconstruct Past Biomass Burning. Comput. Geosciences 72, 255–261. doi:10.1016/j.cageo.2014.07.020
Boex, J., Fogwill, C., Harrison, S., Glasser, N. F., Hein, A., Schnabel, C., et al. (2013). Rapid Thinning of the Late Pleistocene Patagonian Ice Sheet Followed Migration of the Southern Westerlies. Sci. Rep. 3, 2118. doi:10.1038/srep02118
Borrero, L. A., Nuevo Delaunay, A., and Méndez, C. (2019). Ethnographical and Historical Accounts for Understanding the Exploration of New Lands: The Case of Central Western Patagonia, Southernmost South America. J. Anthropological Archaeology 54, 1–16. doi:10.1016/j.jaa.2019.02.001
Borrero, L. A. (2004). “The Archaeozoology of the Andean 'Dead Ends' in Patagonia: Living Near the continental Ice Cap,” in Colonisation, Migration, and Marginal Areas: A Zooarchaeological Approach. Editors M. Mondini, S. Muñoz, and S. Wickler (Havertown: Oxbow Books), 55–61.
Bourgois, J., Cisternas, M. E., Braucher, R., Bourlès, D., and Frutos, J. (2016). Geomorphic Records along the General Carrera (Chile)-Buenos Aires (Argentina) Glacial Lake (46°-48°S), Climate Inferences, and Glacial Rebound for the Past 7-9 Ka. J. Geology. 124, 27–53. doi:10.1086/684252
Caldenius, C. (1932). Las Glaciaciones Cuaternarias en Patagonia y Tierra del Fuego, Dirección Genera de Minas y Geología, 95. Ministerio de Agricultura de la Nación, 148.
Cárdenas, R., Vera, D., and Hall, C. G. (1993). Los Chono y Los Veliche de Chiloé. Santiago: Olimph.
Coronato, A., and Rabassa, J. (2011). “Pleistocene Glaciations in Southern Patagonia and Tierra del Fuego,” in Developments in Quaternary Sciences. Editors J. Ehlers, P. L. Gibbard, and P. D. Hughes (Elsevier), 715–727. Chapter. doi:10.1016/b978-0-444-53447-7.00051-9
Dätwyler, C., Neukom, R., Abram, N. J., Gallant, A. J. E., Grosjean, M., Jacques-Coper, M., et al. (2018). Teleconnection Stationarity, Variability and Trends of the Southern Annular Mode (SAM) during the Last Millennium. Clim. Dyn. 51 (5), 2321–2339. doi:10.1007/s00382-017-4015-0
Davies, B. J., Darvill, C. M., Lovell, H., Bendle, J. M., Dowdeswell, J. A., Fabel, D., et al. (2020). The Evolution of the Patagonian Ice Sheet from 35 Ka to the Present Day (PATICE). Earth-Science Rev. 204, 103152. doi:10.1016/j.earscirev.2020.103152
Davies, B. J., Thorndycraft, V. R., Fabel, D., and Martin, J. R. V. (2018). Asynchronous Glacier Dynamics during the Antarctic Cold Reversal in central Patagonia. Quat. Sci. Rev. 200, 287–312. doi:10.1016/j.quascirev.2018.09.025
de Porras, M. E., Maldonado, A., Abarzúa, A. M., Cárdenas, M. L., Francois, J. P., Martel-Cea, A., et al. (2012). Postglacial Vegetation, Fire and Climate Dynamics at Central Chilean Patagonia (Lake Shaman, 44°S). Quat. Sci. Rev. 50, 71–85. doi:10.1016/j.quascirev.2012.06.015
de Porras, M. E., Maldonado, A., Quintana, F. A., Martel-Cea, A., Reyes, O., and Méndez, C. (2014). Environmental and Climatic Changes in central Chilean Patagonia since the Late Glacial (Mallín El Embudo, 44° S). Clim. Past 10, 1063–1078. doi:10.5194/cp-10-1063-2014
Douglass, D., Singer, B., Kaplan, M., Mickelson, D., and Caffee, M. (2006). Cosmogenic Nuclide Surface Exposure Dating of Boulders on Last-Glacial and Late-Glacial Moraines, Lago Buenos Aires, Argentina: Interpretive Strategies and Paleoclimate Implications. Quat. Geochronol. 1, 43–58. doi:10.1016/j.quageo.2006.06.001
García Guraieb, S., Goñi, R., and Tessone, A. (2015). Paleodemography of Late Holocene hunter-gatherers from Patagonia (Santa Cruz, Argentina): An Approach Using Multiple Archaeological and Bioarchaeological Indicators. Quat. Int. 356, 147–158.
García, J.-L., Maldonado, A., de Porras, M. E., Nuevo Delaunay, A., Reyes, O., Ebensperger, C. A., et al. (2019). Early Deglaciation and Paleolake History of Río Cisnes Glacier, Patagonian Ice Sheet (44°S). Quat. Res. 91, 194–217. doi:10.1017/qua.2018.93
Garreaud, R. D. (2009). The Andes Climate and Weather. Adv. Geosci. 22, 3–11. doi:10.5194/adgeo-22-3-2009
Garreaud, R., Lopez, P., Minvielle, M., and Rojas, M. (2013). Large-scale Control on the Patagonian Climate. J. Clim. 26, 215–230. doi:10.1175/JCLI-D-12-00001.1
Glasser, N. F., Harrison, S., Schnabel, C., Fabel, D., and Jansson, K. N. (2012). Younger Dryas and Early Holocene Age Glacier Advances in Patagonia. Quat. Sci. Rev. 58, 7–17. doi:10.1016/j.quascirev.2012.10.011
Goñi, R., Re, A., García Guraieb., S., Cassiodoro, G., Tessone, A., Rindel, D., et al. (2019). Climate Changes, Human Peopling and Regional Differentiation during Late Holocene in Patagonia. Quat. Int. 505, 4–20.
Grimm, E. C. (1987). CONISS: a FORTRAN 77 Program for Stratigraphically Constrained Cluster Analysis by the Method of Incremental Sum of Squares. Comput. Geosciences 13, 13–35. doi:10.1016/0098-3004(87)90022-7
Haberle, S., and Bennett, K. D. (2004). Postglacial Formation and Dynamics of North Patagonian Rainforest in the Chonos Archipelago, Southern Chile. Quat. Sci. Rev. 23, 2433–2452. doi:10.1016/j.quascirev.2004.03.001
Haberle, S. G., and Bennett, K. D. (2001). Modern Pollen Rain and lake Mud-Water Interface Geochemistry along Environmental Gradients in Southern Chile. Rev. Palaeobotany Palynology 117, 93–107. doi:10.1016/S0034-6667(01)00079-3
Haberle, S. G., Szeicz, J. M., and Bennett, K. D. (2000). Late Holocene Vegetation Dynamics and lake Geochemistry at Laguna Miranda, XI Region, Chile. Revista Chilena de Historia Nat. 73, 655–669. doi:10.4067/s0716-078x2000000400010
Harrison, S., Glasser, N. F., Duller, G. A. T., and Jansson, K. N. (2012). Early and Mid-holocene Age for the Tempanos Moraines, Laguna San Rafael, Patagonian Chile. Quat. Sci. Rev. 31, 82–92. doi:10.1016/j.quascirev.2011.10.015
Harrison, S., and Glasser, N. F. (2011). “The Pleistocene Glaciations of Chile,” in Developments in Quaternary Sciences. Editors J. Ehlers, P. L. Gibbard, and P. D. Hughes (Elsevier), 739–756. doi:10.1016/b978-0-444-53447-7.00054-4
Hein, A. S., Dunai, T. J., Hulton, N. R. J., and Xu, S. (2011). Exposure Dating Outwash Gravels to Determine the Age of the Greatest Patagonian Glaciations. Geology 39, 103–106. doi:10.1130/g31215.1
Hein, A. S., Hulton, N. R. J., Dunai, T. J., Schnabel, C., Kaplan, M. R., Naylor, M., et al. (2009). Middle Pleistocene Glaciation in Patagonia Dated by Cosmogenic-Nuclide Measurements on Outwash Gravels. Earth Planet. Sci. Lett. 286, 184–197. doi:10.1016/j.epsl.2009.06.026
Hein, A. S., Hulton, N. R. J., Dunai, T. J., Sugden, D. E., Kaplan, M. R., and Xu, S. (2010). The Chronology of the Last Glacial Maximum and Deglacial Events in central Argentine Patagonia. Quat. Sci. Rev. 29, 1212–1227. doi:10.1016/j.quascirev.2010.01.020
Heiri, O., Lotter, A. F., and Lemcke, G. (2001). Loss on Ignition as a Method for Estimating Organic and Carbonate Content in Sediments: Reproducibility and Comparability of Results. J. Paleolimnology 25, 101–110. doi:10.1023/A:1008119611481
Henríquez, W. I., Villa-Martínez, R., Vilanova, I., De Pol-Holz, R., and Moreno, P. I. (2017). The Last Glacial Termination on the Eastern Flank of the central Patagonian Andes (47 ° S). Clim. Past 13, 879–895. doi:10.5194/cp-13-879-2017
Heusser, C. J. (2003). Ice Age Southern Andes: A Chronicle of Paleoecological Events. Amsterdam: Elsevier.
Higuera, P. E., Brubaker, L. B., Anderson, P. M., Hu, F. S., and Brown, T. A. (2009). Vegetation Mediated the Impacts of Postglacial Climate Change on Fire Regimes in the South-central Brooks Range, Alaska. Ecol. Monogr. 79, 201–219. doi:10.1890/07-2019.1
Hogg, A. G., Heaton, T. J., Hua, Q., Palmer, J. G., Turney, C. S., Southon, J., et al. (2020). SHCal20 Southern Hemisphere Calibration, 0-55,000 Years Cal BP. Radiocarbon 62, 759–778. doi:10.1017/RDC.2020.59
Holz, A., and Veblen, T. T. (2012). Wildfire Activity in Rainforests in Western Patagonia Linked to the Southern Annular Mode. Int. J. Wildland Fire 21, 114–126. doi:10.1071/WF10121
Iglesias, V., Haberle, S. G., Holz, A., and Whitlock, C. (2018). Holocene Dynamics of Temperate Rainforests in West-Central Patagonia. Front. Ecol. Evol. 5 (177). doi:10.3389/fevo.2017.00177
Kaplan, M. R., Ackert, R. P., Singer, B. S., Douglass, D. C., and Kurz, M. D. (2004). Cosmogenic Nuclide Chronology of Millennial-Scale Glacial Advances during O-Isotope Stage 2 in Patagonia. Geo. Soc. Am. Bull. 116, 308–321. doi:10.1130/b25178.1
Kaplan, M. R., Schaefer, J. M., Strelin, J. A., Denton, G. H., Anderson, R. F., Vandergoes, M. J., et al. (2016). Patagonian and Southern South Atlantic View of Holocene Climate. Quat. Sci. Rev. 141, 112–125. doi:10.1016/j.quascirev.2016.03.014
Kaplan, M. R., Strelin, J. A., Schaefer, J. M., Peltier, C., Martini, M. A., FloresWinckler, E. G., et al. (2020). Holocene Glacier Behavior Around the Northern Antarctic Peninsula and Possible Causes. Earth Planet. Sci. Lett. 534, 116077. doi:10.1016/j.epsl.2020.116077
Kelly, R. F., Higuera, P. E., Barrett, C. M., and Hu, F. S. (2011). Short Paper: A Signal-To-Noise index to Quantify the Potential for Peak Detection in Sediment-Charcoal Records. Quat. Res. 75, 11–17. doi:10.1016/j.yqres.2010.07.011
Leger, T. P. M., Hein, A. S., Bingham, R. G., Rodés, Á., Fabel, D., and Smedley, R. K. (2021). Geomorphology and 10Be Chronology of the Last Glacial Maximum and Deglaciation in Northeastern Patagonia, 43°S-71°W. Quat. Sci. Rev. 272, 107194. doi:10.1016/j.quascirev.2021.107194
Luebert, F., and Pliscoff, P. (2017). Sinopsis bioclimática y vegetacional de Chile. Santiago, Chile: Editorial Universitaria.
Maldonado, A., and Villagrán, C. (2006). Climate Variability over the Last 9900 Cal Yr BP from a Swamp forest Pollen Record along the Semiarid Coast of Chile. Quat. Res. 66, 246–258. doi:10.1016/j.yqres.2006.04.003
Markgraf, V., Whitlock, C., and Haberle, S. (2007). Vegetation and Fire History during the Last 18,000 Cal Yr B.P. In Southern Patagonia: Mallín Pollux, Coyhaique, Province Aisén (45°41′30″ S, 71°50′30″ W, 640 M Elevation). Palaeogeogr. Palaeoclimatol. Palaeoecol. 254, 492–507. doi:10.1016/j.palaeo.2007.07.008
McCulloch, R. D., Figuerero Torres, M. J., Mengoni Goñalons, G. L., Barclay, R., and Mansilla, C. (2017). A Holocene Record of Environmental Change from Río Zeballos, central Patagonia. The Holocene 27, 941–950. doi:10.1177/0959683616678460
Mena L., F., and Blanco J., J. F. (2017). Estado De La Investigación Arqueológica Del Valle Del Chacabuco. Andes Centro Patagónicos. Xi Region De Aisén, Chile. Magallania 45, 199–217. doi:10.4067/s0718-22442017000200199
Méndez, C., de Porras, M. E., Maldonado, A., Reyes, O., Nuevo Delaunay, A., and García, J.-L. (2016). Human Effects in Holocene Fire Dynamics of Central Western Patagonia (∼44° S, Chile). Front. Ecol. Evol. 4 (100). doi:10.3389/fevo.2016.00100
Méndez, C., Stern, C. R., Nuevo Delaunay, A., Reyes, O., Gutiérrez, F., and Mena, F. (2018). Spatial and Temporal Distributions of Exotic and Local Obsidians in Central Western Patagonia, Southernmost South America. Quat. Int. 468, 155–168. doi:10.1016/j.quaint.2017.08.062
Mengoni Goñalons, G. L., Figuerero Torres, M. J., Fernández, M. V., Rocco, M., and Dekmak, W. (2019). “Persistencia y hiatos en el área de Los Antiguos, Monte Zeballos y Paso Roballos, NO de Santa Cruz, Patagonia: nuevas dataciones para la cronología regional,” in Arqueología de la Patagonia: el Pasado en las Arenas. Editors J. Gómez Otero, S. Ariadna, and A. Banegas (Puerto Madryn: Instituto de Diversidad y Evolución Austral), 327–338.
Moreno, P. I., Henríquez, W. I., Pesce, O. H., Henríquez, C. A., Fletcher, M. S., Garreaud, R. D., et al. (2021). An Early Holocene westerly Minimum in the Southern Mid-latitudes. Quat. Sci. Rev. 251, 106730. doi:10.1016/j.quascirev.2020.106730
Moreno, P. I., Simi, E., Villa-Martínez, R. P., and Vilanova, I. (2019). Early Arboreal Colonization, Postglacial Resilience of Deciduous Nothofagus Forests, and the Southern Westerly Wind Influence in central-east Andean Patagonia. Quat. Sci. Rev. 218, 61–74. doi:10.1016/j.quascirev.2019.06.004
Moreno, P. I. (2020). Timing and Structure of Vegetation, Fire, and Climate Changes on the Pacific Slope of Northwestern Patagonia since the Last Glacial Termination. Quat. Sci. Rev. 238, 106328. doi:10.1016/j.quascirev.2020.106328
Moreno, P. I., Vilanova, I., Villa-Martínez, R., Dunbar, R. B., Mucciarone, D. A., Kaplan, M. R., et al. (2018). Onset and Evolution of Southern Annular Mode-like Changes at Centennial Timescale. Sci. Rep. 8 (1), 3458. doi:10.1038/s41598-018-21836-6
Moreno, P. I., Vilanova, I., Villa-Martínez, R., Garreaud, R. D., Rojas, M., and De Pol-Holz, R. (2014). Southern Annular Mode-like Changes in Southwestern Patagonia at Centennial Timescales over the Last Three Millennia. Nat. Commun. 5, 4375. doi:10.1038/ncomms5375
Nanavati, W. P., Whitlock, C., Iglesias, V., and de Porras, M. E. (2019). Postglacial Vegetation, Fire, and Climate History along the Eastern Andes, Argentina and Chile (Lat. 41-55°S). Quat. Sci. Rev. 207, 145–160. doi:10.1016/j.quascirev.2019.01.014
Nuevo Delaunay, A. (2015). Transformación del paisaje arqueológico rural en el centro-oeste de la provincia de Santa Cruz, siglo XX. Unpublished PhD dissertation, Universidad de Buenos Aires.
Nuevo-Delaunay, A., Méndez, C., Reyes, O., Seelenfreund, A., and Belmar, C. (2022). La ocupación humana antigua de los callejones sin salida de los Andes de Patagonia: midiendo la intensidad de uso del espacio en los márgenes del Campo de Hielo Norte (Aisén, Chile). Chungara Revista de Antropología Chilena In press. doi:10.4067/S0717-73562022005000203
Pérez, S. I., Postillone, M. B., Rindel, D., Gobbo, D., Gonzalez, P. N., and Bernal, V. (2016). Peopling Time, Spatial Occupation and Demography of Late Pleistocene–Holocene Human Population from Patagonia. Quat. Int. 425, 214–223.
Power, M. J., Marlon, J., Ortiz, N., Bartlein, P. J., Harrison, S. P., Mayle, F. E., et al. (2008). Changes in Fire Regimes since the Last Glacial Maximum: an Assessment Based on a Global Synthesis and Analysis of Charcoal Data. Clim. Dyn. 30, 887–907. doi:10.1007/s00382-007-0334-x
Quade, J., and Kaplan, M. R. (2017). Lake-level Stratigraphy and Geochronology Revisited at Lago (Lake) Cardiel, Argentina, and Changes in the Southern Hemispheric Westerlies over the Last 25 Ka. Quat. Sci. Rev. 177, 173–188. doi:10.1016/j.quascirev.2017.10.006
R Core Team (2021). R: A Language and Environment for Statistical Computing. Vienna, Austria: R Foundation for Statistical Computing. URL https://www.R-project.org/.". (Viena).
Renssen, H., Goosse, H., Fichefet, T., Masson-Delmotte, V., and Koç, N. (2005). Holocene Climate Evolution in the High-Latitude Southern Hemisphere Simulated by a Coupled Atmosphere-Sea Ice-Ocean-Vegetation Model. The Holocene 15, 951–964. doi:10.1191/0959683605hl869ra
Sacchi, M., Bozzuto, D., Horta, L., Fernández, N., De Nigris, M., Civalero, T., et al. (2016). Dataciones y circulación humana: influencia de las fluctuaciones del sistema lacustre Pueyrredón-Posadas durante el Holoceno. Andes 27, 1–17.
Sagredo, E. A., Kaplan, M. R., Araya, P. S., Lowell, T. V., Aravena, J. C., and Moreno, P. I. (2018). Trans-pacific Glacial Response to the Antarctic Cold Reversal in the Southern Mid-latitudes. Quat. Sci. Rev. 188, 160–166. doi:10.1016/j.quascirev.2018.01.011
Stern, C., Henríquez, W., Villa-Martínez, R., Sagredo, E., Aravena, J., and De Pol-Holz, R. (2016). Holocene Tephrochronology Around Cochrane (∼47° S), Southern Chile. Andean Geology. 43, 1–19. doi:10.5027/andgeoV43n1-a01
Stockmarr, J. (1971). Tablets with Spores Used in Absolute Pollen Analysis. Pollen spores 13, 615–621.
Stuiver, M., Reimer, P. J., and reimer, R. W. (2020). CALIB. 8.0.1 (http://calib.org).
Thorndycraft, V. R., Bendle, J. M., Benito, G., Davies, B. J., Sancho, C., Palmer, A. P., et al. (2019). Glacial lake Evolution and Atlantic-Pacific Drainage Reversals during Deglaciation of the Patagonian Ice Sheet. Quat. Sci. Rev. 203, 102–127. doi:10.1016/j.quascirev.2018.10.036
Turner, K. J., Fogwill, C. J., McCulloch, R. D., and Sugden, D. E. (2005). Deglaciation of the Eastern Flank of the north Patagonian Icefield and Associated continental‐scale lake Diversions. Geografiska Annaler: Ser. A, Phys. Geogr. 87, 363–374. doi:10.1111/j.0435-3676.2005.00263.x
Viale, M., Bianchi, E., Cara, L., Ruiz, L. E., Villalba, R., Pitte, P., et al. (2019). Contrasting Climates at Both Sides of the Andes in Argentina and Chile. Front. Environ. Sci. 7. doi:10.3389/fenvs.2019.00069
Villa-Martínez, R., and Moreno, P. I. (2021). Development and Resilience of Deciduous Nothofagus Forests since the Last Glacial Termination and Deglaciation of the central Patagonian Andes. Palaeogeogr. Palaeoclimatol. Palaeoecol. 574, 110459. doi:10.1016/j.palaeo.2021.110459
Villa-Martínez, R., Moreno, P. I., and Valenzuela, M. A. (2012). Deglacial and Postglacial Vegetation Changes on the Eastern Slopes of the central Patagonian Andes (47°S). Quat. Sci. Rev. 32, 86–99. doi:10.1016/j.quascirev.2011.11.008
Villa-Martínez, R., Villagrán, C., and Jenny, B. (2003). The Last 7500 Cal Yr B.P. Of westerly Rainfall in Central Chile Inferred from a High-Resolution Pollen Record from Laguna Aculeo (34 °S). Quat. Res. 60, 284–293. doi:10.1016/j.yqres.2003.07.007
Whitlock, C., and Larsen, C. (2001). “Charcoal as a Proxy Fire,” in Tracking Environmental Change Using Lake Sediments: Terrestrial, Algal, and Siliceous Indicators. Editors J. P. Smol, H. J. B. Birks, and W. M. Last (Dordrecht, Netherlands: Kluwer Academic Publishers), 75–97.
Keywords: Holocene, pollen records, human–environment interaction, environmental dynamics, Central West Patagonia
Citation: Maldonado A, de Porras ME, Martel-Cea A, Reyes O, Nuevo-Delaunay A and Méndez C (2022) Holocene Environmental Dynamics of the Lago Cochrane/Pueyrredón Valley, Central West Patagonia (47°S). Front. Earth Sci. 10:833637. doi: 10.3389/feart.2022.833637
Received: 11 December 2021; Accepted: 07 March 2022;
Published: 05 April 2022.
Edited by:
Michael R. Kaplan, Columbia University, United StatesReviewed by:
Claudia Mansilla, University of Magallanes, ChileBethan Joan Davies, University of London, United Kingdom
Copyright © 2022 Maldonado, de Porras, Martel-Cea, Reyes, Nuevo-Delaunay and Méndez. This is an open-access article distributed under the terms of the Creative Commons Attribution License (CC BY). The use, distribution or reproduction in other forums is permitted, provided the original author(s) and the copyright owner(s) are credited and that the original publication in this journal is cited, in accordance with accepted academic practice. No use, distribution or reproduction is permitted which does not comply with these terms.
*Correspondence: Antonio Maldonado, antonio.maldonado@ceaza.cl