- 1Centro de Estudios Avanzados en Zonas Áridas (CEAZA), La Serena, Chile
- 2Departamento de Biología, Universidad de La Serena, La Serena, Chile
- 3Departamento de Biología Marina, Universidad Católica del Norte, Coquimbo, Chile
The effects of climate change may be more evident in mountainous areas. In these areas, an increase in temperature and a decrease in precipitation can reduce the amount of snow, which represents a source of water for human consumption and vegetation. To analyze the effects of climate change on vegetation, it is possible to examine the climate–vegetation relationship in the past and observe the influence of variation in temperature and precipitation on the dynamics of plant communities. The aim of the present work was to describe the climate–vegetation dynamics of the last 4,500 years in the high subtropical Andes of Chile (30°S). The paleoclimatic reconstruction was carried out through the analysis of fossil pollen and macroscopic carbon obtained from sediment cores from two high Andean lakes. The dynamics of the vegetation was analyzed taking into account the alpha and beta diversity. The pollen and carbon records showed three contrasting periods during the last 4,500 years. From the beginning of the sequences until ∼1900 cal. yr BP, relatively dry climatic conditions are suggested, with a slight trend toward more humid conditions after 2,700 cal. yr BP. Pollen records from ∼1900 to ∼600 cal. yr BP suggest wetter conditions than today. Finally, relatively arid conditions have reappeared in the last ∼600 years. The diversity analysis showed that the climate mainly influences the composition of taxa in the communities (beta diversity) and not the richness (alpha diversity). In periods of dry/wet transition and vice versa, beta diversity changes. On the other hand, richness remains relatively constant throughout the record.
1 Introduction
Although climate change is a global phenomenon, arid and semiarid areas may be more vulnerable (World Water Council, 2009); one of these areas is located from 27 to 32°S in Chile (Sarricolea et al., 2016). This area is a transition between arid regions such as the Atacama Desert (∼18–27°S) and semiarid Mediterranean areas (∼31–37°S) (Kaiser et al., 2008). At this latitude, precipitation occurs in winter and is generated by the interaction of two systems: the southern westerlies and the South Pacific anticyclone (Montecinos and Aceituno, 2003). In summer, the anticyclone blocks the storms associated with the westerly winds; in winter, the anticyclone is less intense, moves north, and allows the generation of rainfall associated with the system of westerly winds (Quintana, 2012). The area includes high-mountain ecosystems, which are important for the water balance of the watersheds, since they store it in the form of snow and glaciers. Their melting is important for human consumption and the functioning of these ecosystems (Pellicciotti et al., 2014). The effects of climate change may be more evident in mountainous areas, due to temperature increases and increases in drought which diminish the amount of snow, impacting the plant communities and ecosystem functioning (Schickhoff et al., 2016). To understand better the relationship between climate change and ecosystem impact, studies may be performed to analyze the effects of variation in precipitation and temperature patterns on past plant communities (Birks et al., 2010).
Plant community dynamics may be affected by changes in precipitation and temperature, influencing the composition and abundance of species (Woodward and Williams, 1987); additionally, glacier retreats leave ice-free areas that may be colonized (Losapio et al., 2021). The region located between ∼30 and ∼36° is a transition zone and presents different types of vegetation. The region is dominated by deciduous shrubs and succulents, but there are evergreen sclerophyllous trees (Armesto et al., 2007). Pollen studies have been performed in the Andean region of northern Chile, estimating the composition of plant communities and climate by pollen analysis (Martel-Cea et al., 2016; de Porras and Maldonado, 2018). As well as by providing information on climate conditions, pollen records may also be used to study aspects related to plant ecology at a scale of thousands of years; pollen analysis may be used to relate changes in diversity to the environment (Blarquez et al., 2014). Many concepts and theories generated in areas such as community ecology, such as alpha, beta, and gamma diversity, may be applied in paleoecological studies (Jackson and Blois, 2015). However, it must be taken into account that the taxonomic resolution obtained through pollen is low, and this could influence the results of diversity analysis (Mander and Punyasena, 2014). Some studies in the mountain zone of north–central Chile show that there is lower alpha and beta diversity at higher altitudes, related to climate conditions less favorable for plant development (López-Angulo et al., 2018).
Paleoclimate studies in north–central Chile indicate that during the Late Holocene there were variations in the climate conditions (Martel-Cea et al., 2016; Tiner et al., 2018). For example, in the last ∼700 years, a relatively dry period began, which continues until now (Martel-Cea et al., 2016). However, how this variation may influence the diversity of plant communities has not been analyzed. This study presents the results of pollen records obtained in lakes formed by glacial retreat in the high mountains of subtropical Chile (30°S) for the last 4,500 years, complemented with macroscopic carbon analysis and the changes in alpha and beta diversity in the plant communities during this period.
2 Study Area
The study area is located on the western side of the subtropical Andes, in the north–central region of Chile, specifically in lakes Corralito (30° 22′ S; 70° 28′ W; 3,800 m above sea level (a.s.l.)) and Quebrada Parada (30° 24′ S; 70° 25′ W; 3,889 m a.s.l.). Both are contained in the Área Protegida Privada y Santuario de la Naturaleza Estero Derecho (APPSNED). The altitude of the area ranges from 2,000 m to above 4,000 m a.s.l.; the local climate varies with the altitude. There is a cold desert climate between 2,000 and 3,000 m a.s.l., with an annual mean temperature of 11°C and mean precipitation of 30 mm, which occurs mainly in winter (CAEED, 2017). From 3,000 to 4,000 m a.s.l., there is a cold mountain steppe climate with high solar radiation, mean annual temperature of 8°C, and mean annual precipitation of 300 mm, which falls mainly as snow in winter. Above 4,000 m a.s.l., there are areas covered with ice all year round; the temperature fluctuates around 0°C and usually does not exceed 10°C (Cepeda and Novoa, 2006). Rock glaciers are present in this zone (Valois et al., 2020). Precipitation is controlled by the interaction between the system of westerly winds and the Southeast Pacific subtropical anticyclone (Montecinos and Aceituno, 2003). The anticyclone is less intense during winter; it moves northward and allows storms associated with westerly winds to arrive, generating precipitation (Quintana, 2012). The study area is sensitive to interannual variations associated with the El Niño Southern Oscillation (ENSO). The negative phase of ENSO (El Niño) produces abnormally warm and rainy winters, while the opposite occurs in the positive phase (La Niña) (Aceituno, 1988). There are small lakes of glacial origin above 3,800 m a.s.l. in the area, including Laguna Corralito with an area of 0.02 Km2 and Laguna Quebrada Parada, which covers 0.01 km2 (Figure 1; Supplementary Material S1). The sediment cores were obtained from these small lakes.
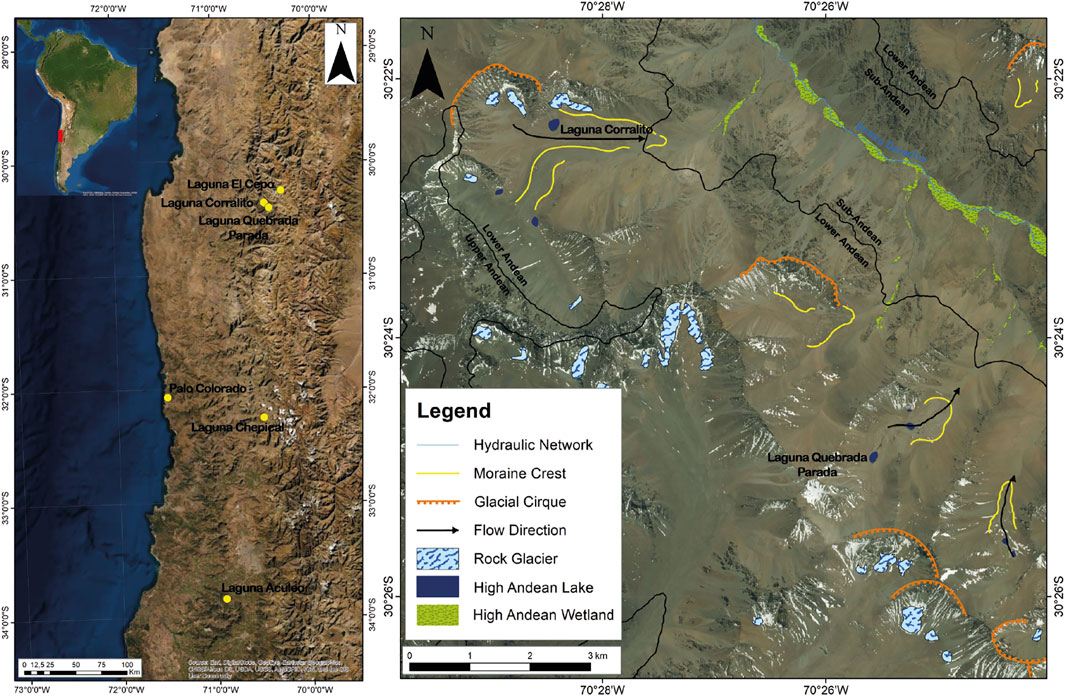
FIGURE 1. Map of the study area showing the location of lakes analyzed, the main geomorphological characteristics of the area, and sites discussed in the text.
The vegetation in this area is Andean steppe, which covers xerophytic herbs, shrubs, and subshrubs. There are four vegetation belts in the area: below 2,700 m a.s.l. is the pre-Andean belt, with desert shrubs (e.g., Atriplex desertícola) and trees (e.g., Prosopis chilensis); the sub-Andean belt from 2,700 to 3,500 m a.s.l., with shrubby vegetation and grasslands dominated by Stipa chrysophylla, Viviana marifolia, and Cristaria andicola; the lower Andean zone from 3,500 to 4,250 m a.s.l., with cushion plants of Adesmia subterranea, Calceolaria pinifolia, and Azorella cryptantha; and the upper Andean belt from 4,250 to 4,450 m a.s.l., with species such as Menonvillea cuneata (Squeo et al., 2009). The study area has patchy vegetation, and there are many places prone to landslides that make the vegetation scarce in some places (Squeo et al., 2009). The lakes from which sediment cores were obtained are in the lower Andean belt.
3 Methods
3.1 Sampling, Lithology, and Dating
During the summer of 2018, two sediment cores were extracted from Laguna Corralito (LCO) and two from Quebrada Parada (LQP) using a UWITEC gravity corer. The LCO core was 83 cm and that of LQ Parada was 183 cm. The sediment description of the cores includes texture, color, photographs, X-ray images, and loss on ignition. The chronology of the cores was obtained using eight dates obtained by AMS 14C analysis for each lake. The dates were corrected for the reservoir effect considering the age obtained at the surface sediments of lake LCO (244 calendar years before present “cal. yr BP” (BP = ad 1950)); this correction was applied to both lakes given that the deposition environment and the geology of the lakes are equivalent (Velásquez et al., 2021). Temporal variations of the reservoir effect during the last 4,000 years do not seem to have importance according to studies close to the study area (Tiner et al., 2018).
The ages were calibrated with the SHCal20 curve (Hogg et al., 2020); the age–depth model was generated using the Bacon package version 2.5.7 (Blaauw and Christen, 2011). Two cores from LCO were used, namely LCO-shc1 (73 cm) and LCO-shc2 (66 cm); the former covers a time scale of ∼3,000 years and the latter of ∼4,500 years. We analyzed the lithological characteristics, loss on ignition, and dates of both cores to obtain a composite sequence that covered the last ∼4,500 years. Centimeter 73 of core LCO-shc1 was assembled with centimeter 48–58 of core LCO-shc2. The cores have different sediment accumulation, and thus the union of these cores generated an artificial inflection in the age–depth curve of the sediments. The possibility of a hiatus in the bottom part of core LCO-shc2 (with a lower sedimentation rate) is unlikely, considering the sedimentary structures seen in the X-ray images and the sedimentation rate of the complete core.
3.2 Analysis of Pollen and Macroscopic Carbon Particles
The sediment samples were taken every 2 cm of the cores for the pollen study. Pollen extraction was performed using routine protocols (Faegri and Iversen, 1989), including deflocculation and elimination of humic acids (KOH 10%), removal of carbonates (HCL 10%), removal of silicates (HF 40%), and acetolysis to eliminate the organic material. Lycopodium tablets were included in the extraction to calculate the concentration (Stockmarr, 1971) and later pollen accumulation rate. At least 300 pollen grains of terrestrial taxa were counted in each level, using an optical microscope under 400x and 1,000x. Pollen grains were identified using bibliography (Heusser, 1971; Markgraf and D’Antoni, 1978) and reference samples from the Laboratorio de Paleoecología y Paleoclimatología of the Centro de Estudios Avanzados en Zonas Áridas (CEAZA). Pollen diagrams, cluster analysis using restricted incremental sums of squares, (CONISS) and calculation of the rate of pollen accumulation were performed in the TILIA 1.7.16 program (Grimm, 2011). Pollen diagrams and cluster analysis were performed using the pollen types with more than two percent. The number of zones was determined using the “broken stick” test implemented in the Rioja package of R version 3.4.4 (O’Reilly et al., 2014). Only the main terrestrial taxa described for the study area (Squeo et al., 2009; Villagrán et al., 1983) were graphed in the pollen diagrams.
Carbon macroparticles from both lakes were analyzed, using sediment samples of 2 cm3 from each centimeter of the cores. KOH 10% was added to each sample for 6 h, after which they were sifted using 250- and 125-µm sieves. Carbon particles were counted on a stereoscopic microscope and were quantified as the number of particles per cm3 of sediment (Whitlock and Larsen, 2002). Carbon data were analyzed with the program CharAnalysis, which uses the accumulation of macroscopic carbon particles in sediment cores to estimate a number of parameters such as local fire episodes, magnitude and frequency of the events, recurrence time, and others (Higuera et al., 2009).
3.3 Analysis of Alpha and Beta Diversity
Alpha diversity was calculated as the number of pollen types found in each sample in the sediment core (Gosling et al., 2018). Detrended correspondence analysis (DCA) was performed on the square root-transformed pollen abundance, obtaining values of changes in the vegetation composition (beta diversity) over time from the fossil pollen (Correa-Metrio et al., 2014). Euclidean distances between ordination scores in the first axis (DCA 1) were measured for each sample to estimate the dissimilarity in the composition of the communities among the samples and to be used as a value of “ecological change,” which is also a proxy of beta diversity (Correa-Metrio et al., 2014). The comparison of alpha and beta diversity values over time was performed for the two lakes, thus allowing us to estimate whether or not the behavior of the two nearby lakes changed synchronically with the climate changes in the last 4,500 years. DCA was calculated in the vegan package of R (Oksanen et al., 2015).
4 Results
4.1 Laguna Corralito
4.1.1 Lithological Description, Loss due to Ignition, and Chronology
The composite sequence of LCO had 83 cm; the first 73 cm was from the first core and 10 cm from the second. The first 6 cm of LCO-shc2 from the base was composed of gray clay with 95% inorganic material; from 58 to 51 cm, the core had a yellowish color with a silty texture, with 33.19% organic matter and 0.09 g/cc organic density, while 50–48 cm had a brown color with a clayey texture, with 31.48% organic matter and 0.06 g/cc organic density (Figure 2B). The LCO-shc1 between 73 and 68 centimeters has a dark brown color and a silty texture, with 30.33% of organic matter and 0.07 g/cc of organic density. The sequence from 68 to 23 cm was dark brown with a silty texture, with 29.97% organic matter and 0.06 g/cc organic density. The sequence from 23 to 18 cm had a yellowish brown color with a silty/clayey texture, with 27.71% organic matter and 0.04 g/cc density. The sequence from 18 to 3 cm had a brown color with a clayey texture, with 28.98% organic matter and 0.05 g/cc density. The sequence from 3 to 2 cm had light brown color and silty texture, with 30.33% organic matter and 0.05 g/cc density. The upper 2 cm was dark brown with a silty/clayey texture, with 47.17% organic matter and 0.06 g/cc density (Figure 2A). The percentage of inorganic matter was relatively constant in both cores.
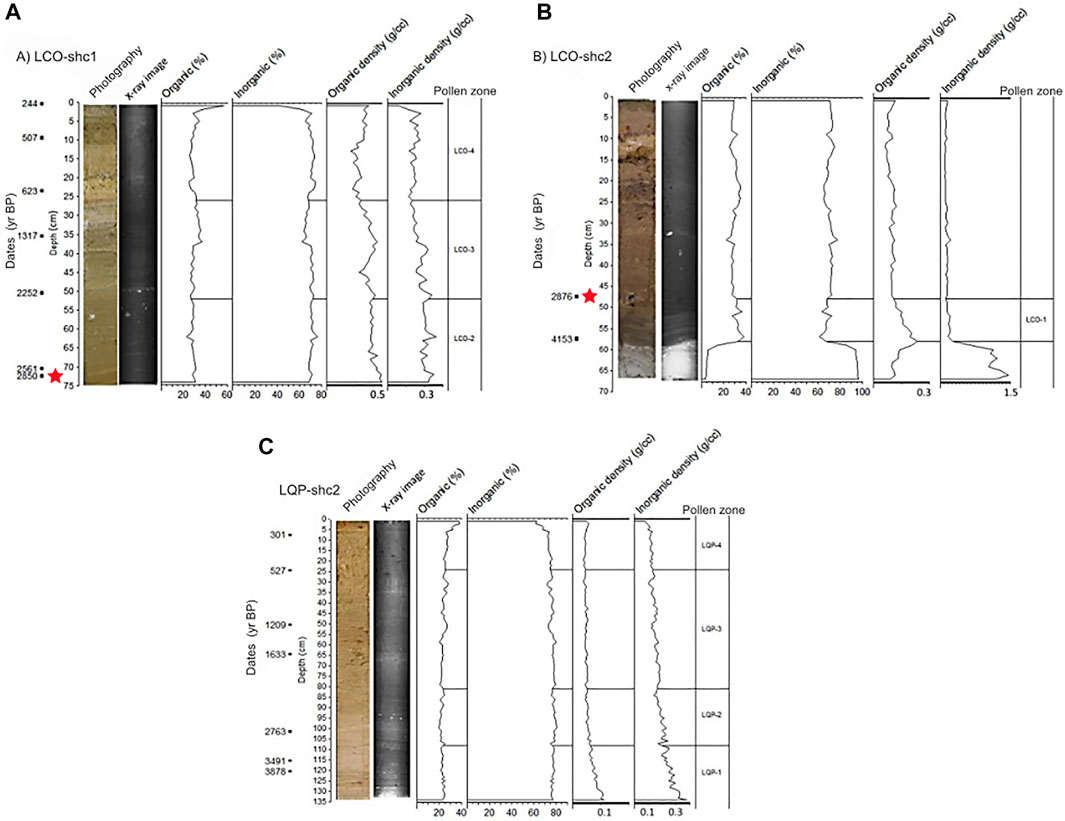
FIGURE 2. Photographs, X-ray images, percentage and density of organic and inorganic matter, and radiocarbon ages of the cores from (A) Laguna Corralito LCO-shc1, and (B) Laguna Corralito LCO-shc2 (The LCO-shc2 is attached to the LCO-shc1 base from 48 cm). (C) Laguna Quebrada Parada LQP-shc2. The red star denotes the tie point between LCO-shc1 and LCO-shc2.
A total of eight 14C dates were obtained, from the later sediments to the gray inorganic clays, which were corrected for the reservoir effect, considering the surface age as reservoir (Table 1). Thus, the sequence includes a range from ∼4,300 cal. yr BP to the present. The change of slope in the age–depth curve is due to the change of core because of the difference in sedimentation rates of the cores. From 83 to 73 cm, the mean sedimentation rate was 0.003 cm/year, after which it increased to a mean of 0.03 cm/year (Figure 3A).
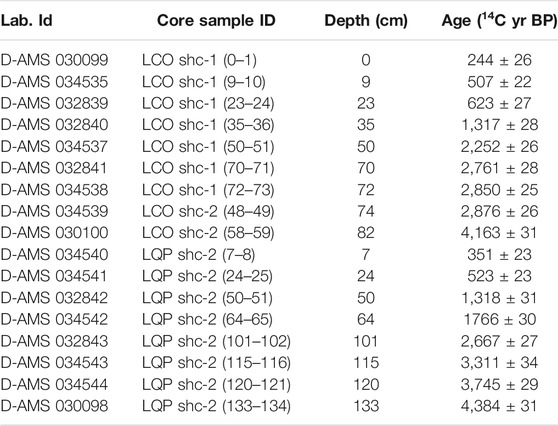
TABLE 1. Radiocarbon-dated from cores of Laguna Corralito (LCO) and Laguna Quebrada Parada (LQP). All dates were obtained from bulk sediments, and all dates were adjusted by 244 ± 2614C years.
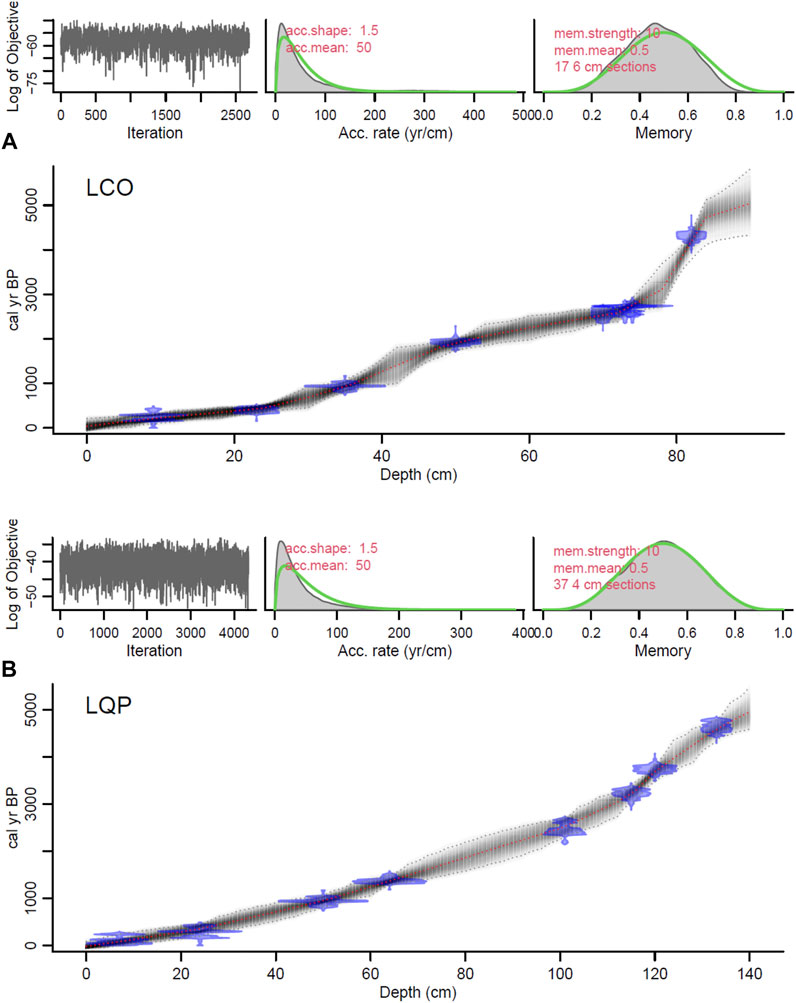
FIGURE 3. Age–depth model of the core of (A) Laguna Corralito (LCO) and (B) Laguna Quebrada Parada (LQP). The top panels show, from left to right, Markov chain Monte Carlo iteration, prior (green curves)/posterior (gray histograms) distributions of the accumulation rate and memory of LCO and LQP. In the main panel, calibrated radiocarbon ages are shown in blue, and the age–depth model is represented by the gray dots, with a darker gray indicating more probable calendar ages. The gray dotted lines show 95% confidence intervals, and the red curve indicates the best model that was used for the study of both lakes.
4.1.2 Analysis of Pollen and Macroscopic Carbon Particles
The pollen diagram was divided into four pollen zones (LCO-1, LCO-2, LCO-3, and LCO-4). The most abundant pollen type was Ephedra, which reached up to ∼30%. Other types of pollen were generally less than 10% (Figure 4).
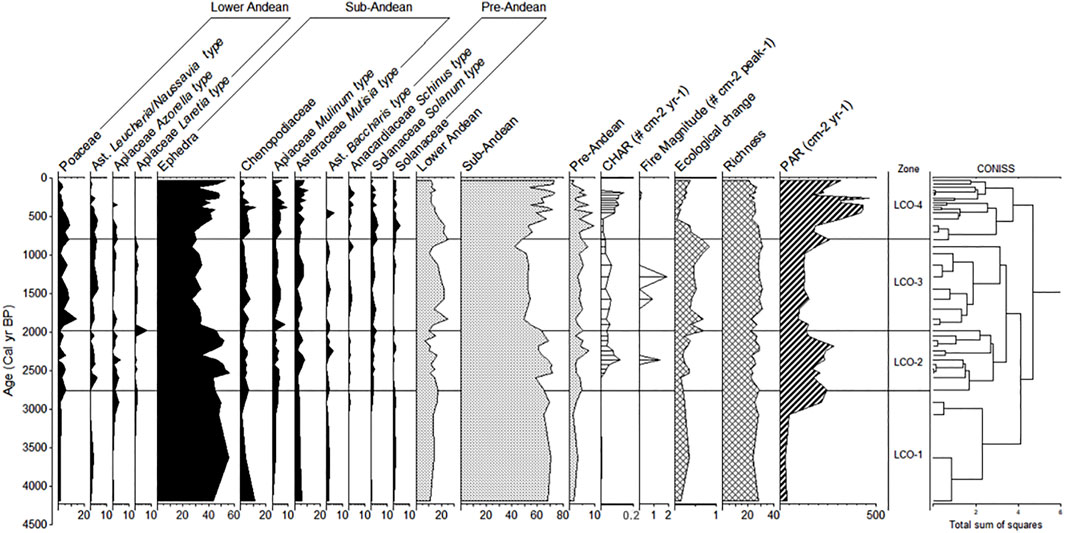
FIGURE 4. Pollen diagram of the percentage of the main taxa of Laguna Corralito. The carbon accumulation rate “CHAR” (# particles cm-2 years-1), the magnitude of the fire (# particles cm-2 peak-1), and the pollen accumulation rate “PAR” (# pollen grains cm-2 years-1) are shown. The values of the ecological change and the pollen richness recorded throughout the record are also shown.
LCO-1: From ∼4,300 to ∼2,760 cal. yr BP, Ephedra dominates (around 50%). Companion taxa are Asteraceae Mutisia-type (around 4%), Apiaceae Mulinum-type (around 2%), Chenopodiaceae (around 5%), and Poaceae (around 2%). The greatest abundance of pollen in this area is from taxa of the sub-Andean belt, reaching up to 70%. The rate of pollen accumulation was the lowest of all records in almost all the periods. No carbon particles were recorded in this zone.
LCO-2: From ∼2,760 to ∼1,980 cal. yr BP, Ephedra continued to be the most abundant pollen type (57%). Other pollen types, present in less than 10%, were Asteraceae Mutisia-type (4%), Poaceae (3%), Asteraceae Leucheria/Nassauvia-type (1.8%), and Apiaceae Azorella-type (1.7%). There was a small increase of Solanaceae Solanum-type (2%) pollen. Sub-Andean pollen continued to dominate in this period (up to 71%), but with fluctuations. The percentage of lower Andean taxa decreased (to 9%) but not considerably. Pre-Andean taxa maintained low percentages (7%). The content of macroscopic carbon particles increased considerably; a fire event about ∼2,400 cal. yr BP is inferred.
LCO-3: From ∼1,980 to ∼790 cal. yr BP, there was a decrease in the percentage of Ephedra (33%) and an increase in Poaceae (14%), Asteraceae Leucheria/Nassauvia-type, and Apiaceae Azorella-type (3%). Apiaceae Laretia-type was recorded for the last time about 800 cal. yr BP. This period had a reduced sub-Andean pollen percentage (42%), reaching the lowest percentage in the entire record. There was an increase in pollen of the lower Andean belt (22%). The record of carbon particles suggests two fire events at ∼1,500 and ∼1,300 cal. yr BP; the second event was larger.
LCO-4: This period from ∼790 cal. yr BP to the present was dominated by Ephedra (40%), with the presence of pollen types of sub-Andean vegetation: Asteraceae Mutisia-type (6%) and Apiaceae Mulinum-type (5%). The pollen of the higher zones (lower Andean zone) such as Poaceae decreased to 3%. The record of carbon particles from ∼500 to ∼200 cal. yr BP was not enough to infer fire events.
4.2 Laguna Quebrada Parada
4.2.1 Lithological Description, Loss due to Ignition, and Chronology
Core LQP-shc2 had a total of 134 cm. From 134 to 124 cm, the core was brown with a clayey texture, with 23.96% organic material and 0.07 g/cc organic density. From 124 to 107 cm, the core was brown with a silty texture, with 11.76% organic matter and 0.05 g/cc density. From 107 to 105 cm, the core was dark brown with a silty/clayey texture, with 22.96% organic matter and 0.04 g/cc density. From 105 to 4 cm, the core was olive brown with a silty texture, with 23.68% organic matter and 0.04 g/cc. From 4 to 2 cm, the core was yellowish brown with a silty texture, with 31.98% organic matter and density of 0.04 g/cc. The first 2 cm of the core was brown with a silty texture, with 37.81% organic matter and 0.04 g/cc density. The X-ray images show greater presence of inorganic material from 134 to 129 cm and clasts in 100–95 cm. The percentage of inorganic matter was relatively constant from 134 to 5 cm, with a mean of 76%. The percentage of inorganic matter decreased to 65% in the last 5 cm (Figure 2C).
A total of eight 14C dates were obtained, which were corrected for the reservoir effect and calibrated in cal. yr BP the same way as in LCO (Table 1). Thus, the period that the core includes is from ∼4,500 cal. yr BP. Between 134 and 102 cm, the sedimentation rate was 0.02 cm/year; from 102 to 26 cm, the rate was 0.03 cm/year, and from cm 26 to 0 the sedimentation rate increased to 0.07 cm/year (Figure 3B).
4.2.2 Analysis of Pollen and Macroscopic Carbon Particles
A total of four pollen zones (LQP-1, LQP-2, LQP-3, and LQP-4) were identified from the analysis of the conglomerate. The most abundant type of pollen was Ephedra, which reached 53%, followed by Asteraceae Mutisia-type with 16%, Poaceae and Asteraceae Leucheria/Nassauvia-type with 12%, and Apiaceae Azorella-type with 6% (Figure 5).
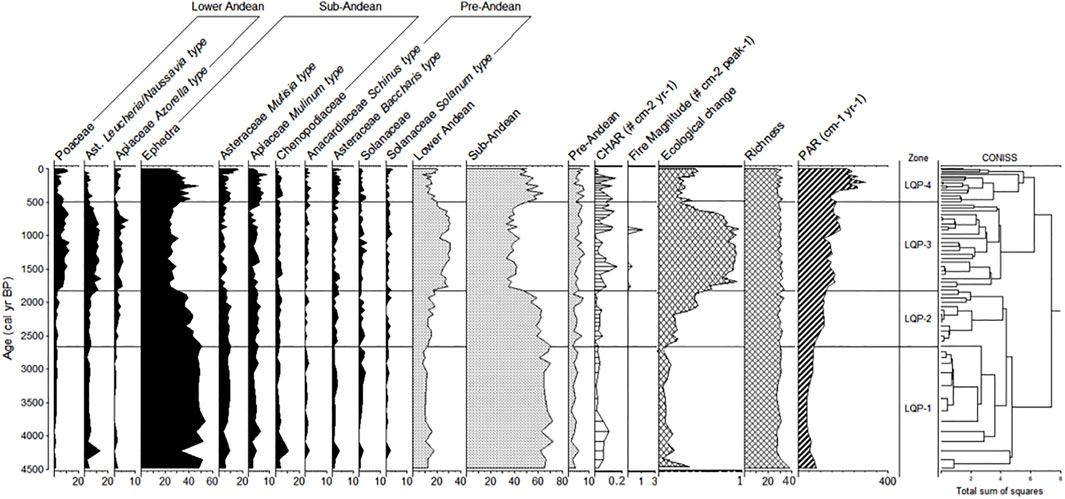
FIGURE 5. Pollen diagram of the percentage of the main taxa of Laguna Quebrada Parada. The carbon accumulation rate “CHAR” (# particles cm-2 years-1), the magnitude of the fire (# particles cm-2 peak-1), and the pollen accumulation rate “PAR” (# pollen grains cm-2 years-1) are shown. The values of the ecological change and the pollen richness recorded throughout the record are also shown.
LQP-1: The period from ∼4,500 to ∼2,660 cal. yr BP was dominated by Ephedra, which reached 53%. Lesser amounts of Apiaceae Mulinum-type (3%), Asteraceae Mutisia-type (7%), Poaceae (1%), Asteraceae Leucheria/Nassauvia-type (5%), and Apiaceae -Azorella-type (1%) were found. The pollen types of the pre-Andean belt were 2% or less. Species of the sub-Andean belt were dominant in this period (up to 72%); the carbon accumulation rate was low (mean 0.03 particles cm−2 year−1), and no fire events were inferred.
LQP-2: This period from ∼2,660 to ∼1,820 cal. yr BP showed dominance of Ephedra (around 42%). The other pollen types were Asteraceae Mutisia-type (8%), Apiaceae Mulinum-type (4%), Poaceae (2%), Apiaceae Azorella-type (2%), and Asteraceae Leucheria/Nassauvia-type (5%). This period is dominated by pollen of sub-Andean species (up to 70%). The carbon accumulation rate was low, and no fire events were inferred.
LQP-3: Between ∼1,820 and ∼490 cal. yr BP, Ephedra (26%), Asteraceae Mutisia-type (4%), and Apiaceae Mulinum-type (3%) all reduced their percentages. Poaceae pollen increased (8%), as did Asteraceae Leucheria/Nassauvia-type (10%) and Apiaceae Azorella-type (3%). Although Ephedra continued to be the dominant taxon, the percentage of pollen of the sub-Andean belt decreased (34%), which was the lowest value of the entire record. Pollen of species of the lower Andean belt increased up to 31%, which is the highest value of the record. The carbon accumulation rate reached its highest value (0.14 particles cm−2 year−1) but was not constant; it oscillated during the period. Three fire events were inferred in this zone, and the largest around ∼1,000 cal. yr BP.
LQP-4: Between ∼490 cal. yr BP and the present, there was an increase in the percentages of Ephedra (34%), Asteraceae Mutisia-type (8%), and Apiaceae Mulinum-type (5%). Poaceae decreased (5%), as did Asteraceae Leucheria/Nassauvia-type (3%), which disappeared after 190 cal. yr BP. The lowest rate of carbon accumulation of the last ∼4,500 years was recorded, and no fire events were detected.
4.2.3 Alpha and Beta Diversity
The results of the DCA analysis in LCO showed 17.74% of the variation explained by the first component (Table 2). LCO-4, LCO-2, and LCO-1 showed mostly negative values, while values were positive in the LCO-3 zone (Figure 6). The first component explained 39.59% of the variation in LQP. Again, LQP-4, LQP-2, and LQP-1 showed mostly negative values. LQP-4 showed positive values beginning with ∼1970 years AD. The values were positive in the LQP-3 zone on this axis.
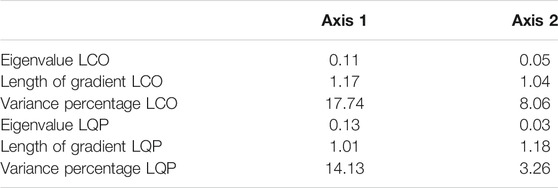
TABLE 2. DCA results of the abundance of fossil pollen from Laguna Corralito (LCO) and Laguna Quebrada Parada (LQP).
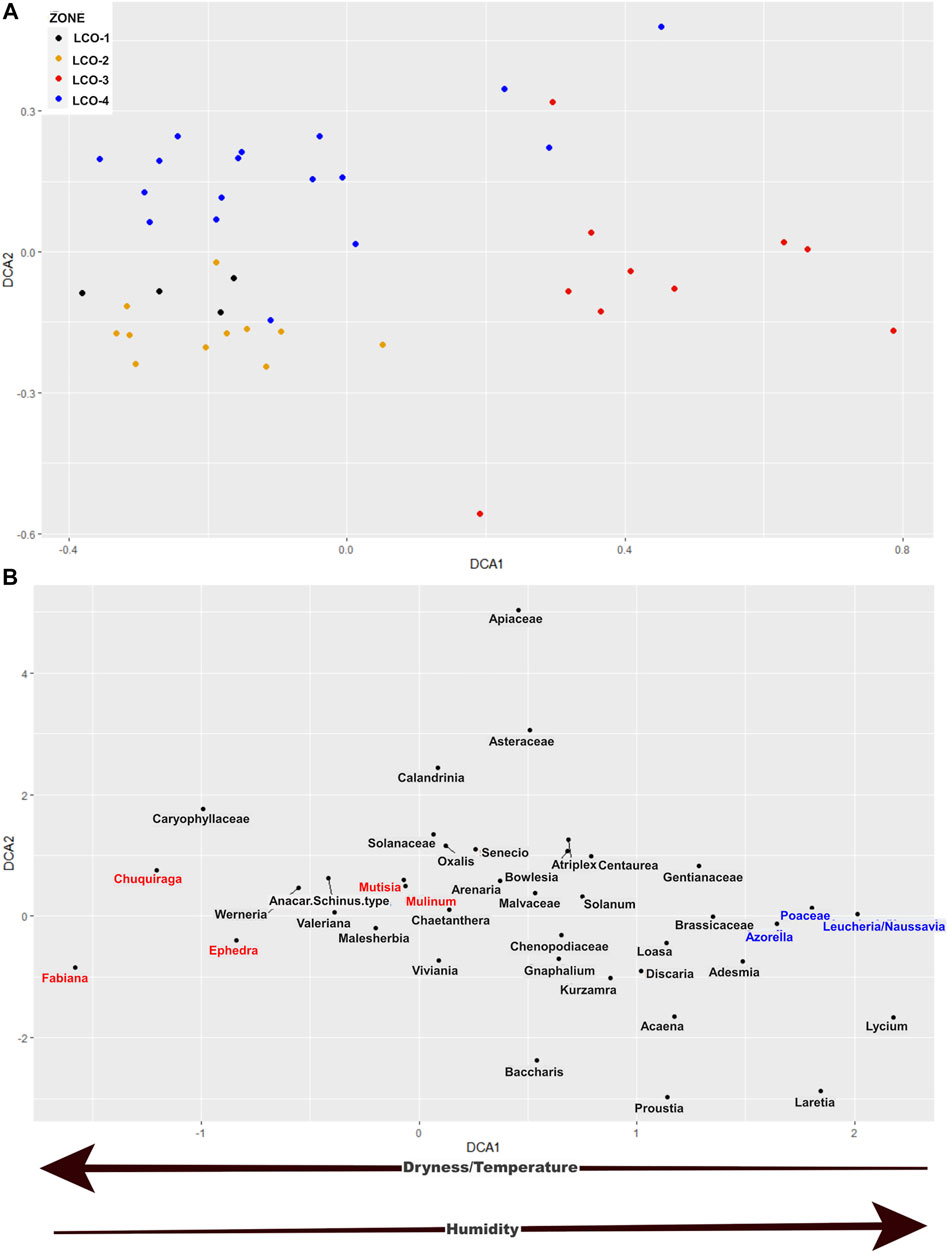
FIGURE 6. DCA results from the pollen abundance of Laguna Corralito. (A) Ordering of the levels (colored points) grouped in the zones, obtained by the cluster analysis; on the left, are the levels with environmental conditions of lower humidity and on the right, the levels with higher humidity. (B) Ordering of pollen types with a similar arrangement in the multivariate space as the levels. Pollen types related to warmer and less humid conditions are highlighted in red. Pollen types related to cool and humid conditions are highlighted in blue. The arrows below indicate how the humidity and temperature conditions vary along the DCA 1 axis.
The pollen types related to species of the sub-Andean belt, associated with drier and/or less cold conditions, are at the left of the graphs (e.g., j. Asteraceae Chuquiraga-type, Solanaceae Fabiana-type, and Ephedra). The values for beta diversity (ecological change) had standard deviations less than one for both lakes; the most important changes coincide with the periods of transition between the different periods (Figures 6, 7). The values remained relatively constant in LCO-1 up to the transition to LCO-2 around ∼2,600 cal. yr BP, when the ecological change increased. LCO-2 showed oscillations during the entire period, one of which coincided with the transition to LCO-3. LCO-3 had the highest values of change, with the most important maximum around ∼850 cal. yr BP (Figure 4).
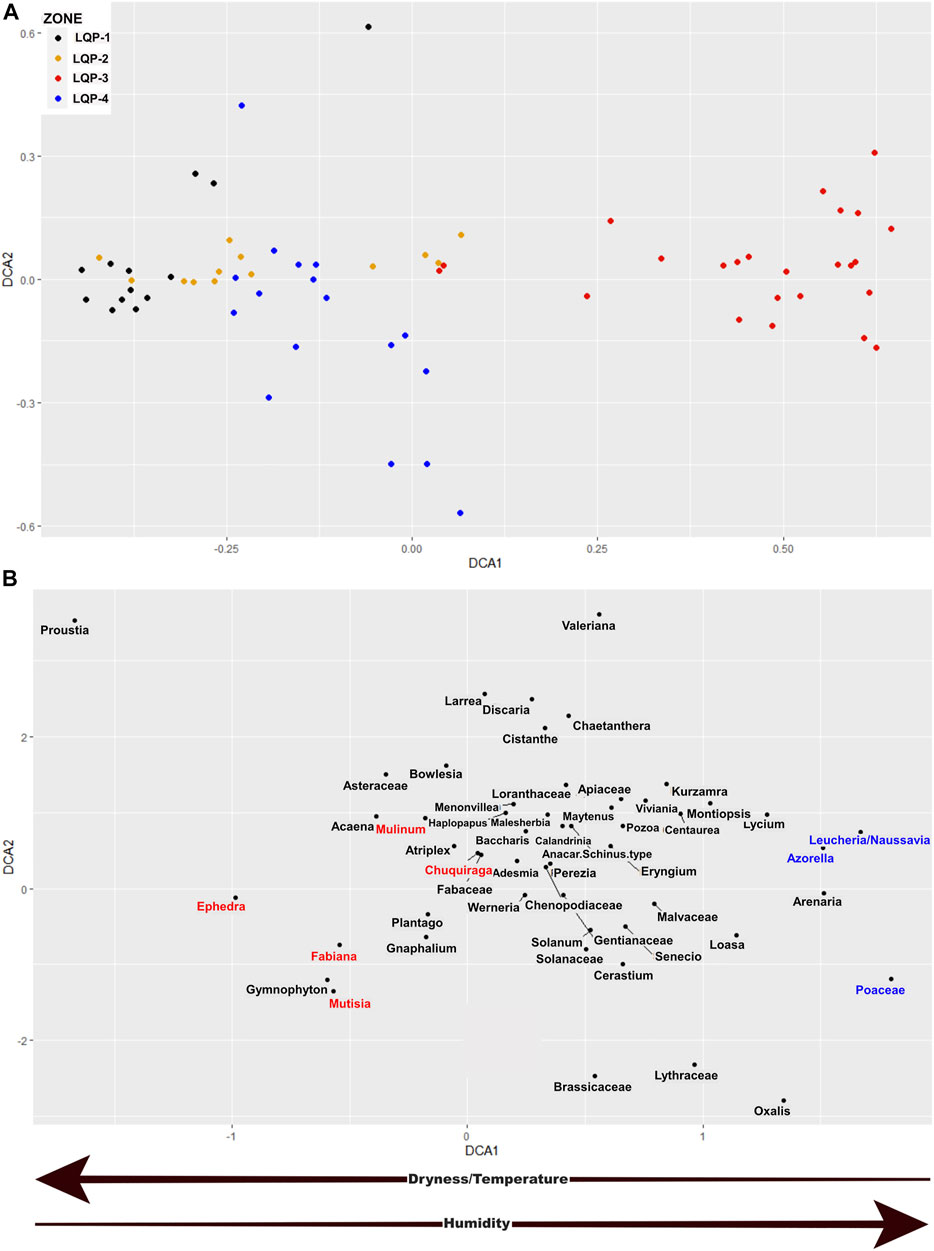
FIGURE 7. DCA results from the pollen abundance of Laguna Quebrada Parada. (A) Ordering of the levels (colored points) grouped in the zones, obtained by the cluster analysis; on the left, are the levels with environmental conditions of lower humidity and on the right, the levels with higher humidity. (B) Ordering of pollen types, with a similar arrangement in the multivariate space as the levels. Pollen types related to warmer and less humid conditions are highlighted in red. Pollen types related to cool and humid conditions are highlighted in blue. The arrows below indicate how the humidity and temperature conditions vary along the DCA 1 axis.
The Laguna Quebrada Parada, as in the LCO, showed relatively constant values of ecological change in the LQP-1 period, which deceased during the transition to LQP-2 with minimum values around ∼2,600 cal. yr BP. The LQP-3 period had the highest values of ecological change; however, there were oscillations. The LQP-4 period showed relatively low values of beta diversity, which increased starting about ∼200 cal. yr BP (Figure 5).
The alpha diversity did not show large oscillations of pollen richness. LCO had a mean of 26 pollen types during the whole record (Figure 4), varying from 25 to 28 in the four zones, while LQP had a mean of 29 pollen types, varying from 28 to 30 in the four zones (Figure 5).
5 Discussion
5.1 Interpretation of the Paleoclimate
The pollen ensembles of LCO and LQP were interpreted according to the altitudinal gradient of vegetation and climate conditions. Thus, for example, the sub-Andean belt is associated with lower precipitation and higher temperature than the lower Andean belt, which has more precipitation and temperatures below 0°C (Cepeda and Novoa, 2006). The main pollen types associated with the sub-Andean zone are Ephedra, Asteraceae Mutisia-type, and Apiaceae Mulinum-type. The genus Ephedra is anemophilous; thus, it produces and disperses a large amount of pollen, which explains its high presence in both records; it is also a genus vulnerable to freezing temperatures (Cavieres et al., 2000). Thus, the presence and abundance of Ephedra in the record is an indicator of less humid and cold conditions. The pollen types Poaceae, Apiaceae Azorella-type, and Asteraceae Leucheria/Nassauvia-type are associated with the lower Andean belt. The presence of grasses in the arid and semiarid Andes is related to soil humidity; more humid soils have a greater diversity of Poaceae. The species of this family tolerate freezing temperatures (Cavieres et al., 2000). Thus, the presence and abundance of Poaceae pollen in the sediments are a good indicator of humid and cold conditions, which are characteristics of the lower Andean zone.
The first axis of the DCA may be related to the gradient of precipitation and temperature (Figures 6, 7). In this axis, the pollen types and dry and less cold periods have negative values and are on the left of the axis, while the pollen types and more humid and cold periods have positive values and are on the right of the axis. Thus, pollen of shrubs such as Fabiana, Ephedra, and Chuquiraga are associated with relatively dry periods, while Poaceae and Leucheria/Nassauvia are related to relatively humid periods.
The carbon analysis showed the greatest activity between ∼1,900 and ∼600 cal. yr BP. The presence of macroscopic carbon particles in the sediment cores is associated with fire events (Whitlock et al., 2010). In the north–central region of Chile, there is a relation between fires, climate, and ENSO (Altamirano et al., 2013). Rainy years favor vegetation growth; in dry periods, this is converted into biomass that may burn (Gonzáles et al., 2011), and thus humid periods with marked seasonality may favor the propagation of fires (Gonzáles et al., 2011). The record of macroscopic carbon particles may thus serve to help identify periods with humid conditions but marked seasonality (rainy winters and very dry and/or hot summers) or strong interannual variation in precipitation and temperature which are mainly associated with the dynamics of the ENSO (Montecinos and Aceituno, 2003).
At the beginning of the records, there was a higher proportion of inorganic sediments in both lakes, especially LCO. The sediment record began with gray clay, barren of pollen, of glacial origin, which suggests that the beginning of organic deposits at ∼4,000 cal. yr BP is associated with a small glacial retreat at 3,800–3,900 m a.s.l., which may have liberated the area for the formation of the current lakes. Later, both lakes showed alternation of dry and humid periods in the last ∼4,500 years. From ∼4,500 to ∼2,700 cal. yr BP, the domination of Ephedra, Asteraceae Mutisia-type, and Apiaceae Mulinum-type in LQP suggests a relatively dry period. The low fire activity period and negative values in the DCA 1 axis reinforce the idea of dry conditions that did not favor plant growth and thus little accumulation of biomass that could generate fires. From ∼2,700 to ∼1,900 cal. yr BP, the same taxa continued to dominate; however, the decrease of elements of the sub-Andean belt and the gradual increase of elements of the lower Andean belt and the first record of fire in the LCO suggest a period of transition toward more humid conditions.
Between ∼1,900 and ∼600 cal. yr BP, there was an increase in pollen associated with the lower Andean belt, represented by Poaceae, Asteraceae Leucheria/Nassauvia-type, and Apiaceae Azorella-type and a concomitant decrease in pollen of species of the sub-Andean belt. This suggests a wetter and colder period than the anterior. This period also had the greatest carbon accumulation and the most fire events. This suggests a period with greater seasonality and/or greater interannual variability, which concurs with reports that this period had the greatest frequency of ENSO events in the entire Holocene (Moy et al., 2002; Rein et al., 2005).
The last ∼600 years again showed an increase in pollen associated with the sub-Andean belt and the accumulation of carbon decreased, suggesting a new dry period. The tendency of an increase in temperature and a reduction in precipitation in recent years in the study area is registered not only in paleoclimatic studies. For example, Souvignet et al. (2012) observed that in the last 30 years in the study area there is an increase in temperature and an annual reduction in precipitation; this could be related to climate change caused by human activities.
5.2 Regional Correlations
The beginning of the records in LCO and LQP lakes has been associated with a glacial retreat in the high mountains, which may be related with the global event at 4,200 cal. yr BP that in some records of the Northern Hemisphere has been indicated as a cold event (Orme et al., 2018) and that marks the beginning of the late Holocene (Wanner et al., 2015). There is not much evidence of new glacial advances in the sub-tropical Andes; however, there is a report of an advance at 35°S in the Andes of Argentina at 4,700–4,300 cal. yr BP (Espizua, 2005), which could be related to the beginning of more organic deposition in our study area. The cores of both LCO and LQP showed the alternation of relatively dry and humid periods from the beginning of the records. The first period, from ∼4,500 to ∼1,900 cal. yr BP, had relatively dry conditions, with a transition phase from ∼2,700 to ∼1,900 cal. yr BP. This result is coherent with the geochemical and physical indicators of the Laguna El Cepo and Cerritos Blancos near the study area (30°S), which suggest relatively dry conditions in this period (Tiner et al., 2018).
These results are somewhat different than those obtained by Martel-Cea et al. (2016), who analyzed the record of the Laguna Chepical in the high Andean zone of central Chile (32°S); they found that the period 3,100–2,000 cal. yr BP was relatively humid and cold, followed by the maximum values of humidity indicators from 2,000 to 1,200 cal. yr BP, possibly associated with higher summer temperatures. The record of the Laguna Aculeo (34°S) in the lowlands showed an important increase in the humidity indicators beginning 3,000 cal. yr BP and an important increase in torrential rains associated with El Niño starting 1,800 cal. yr BP (Jenny et al., 2002; Villa-Martínez et al., 2003). Considering the beginning of the decrease of indicators of the low vegetation belt (sub-Andean) in LCO and LQP, there is some correspondence in the chronology of this humid phase, although at different times and intensity or maximum values from those found in the Laguna Chepical and Laguna Aculeo. Relatively humid conditions at ∼4,000 cal. yr BP were observed in the coast of central Chile (32°S), which became more arid from 3,000 to 2,200 cal. yr BP (Maldonado and Villagrán, 2006; Méndez et al., 2015). In spite of the differences in the dates of change, most of the records suggest relatively dry conditions at least until ∼2,700 or ∼2,000 cal. yr BP (Figure 8). On the other hand, it is possible that the differences found are related to the different latitudes of the studies mentioned.
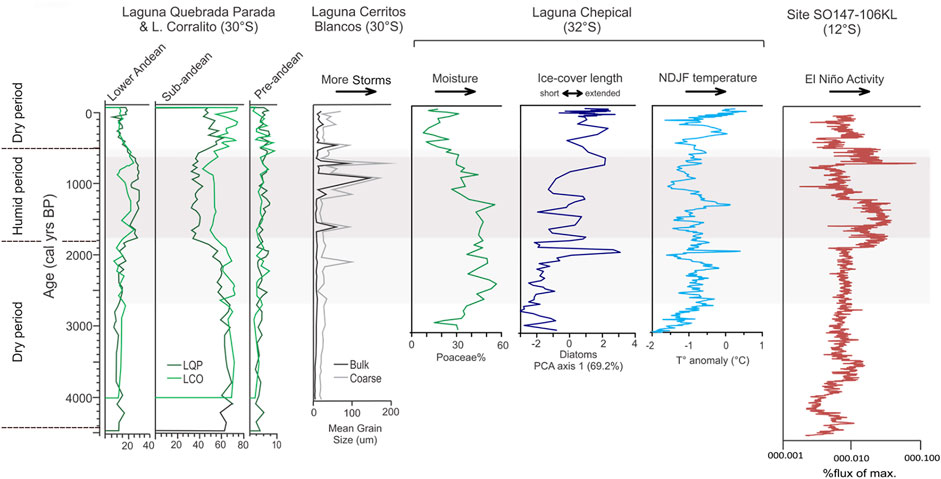
FIGURE 8. Comparison of the pollen record summary from Laguna Corralito and Laguna Quebrada Parada, with Laguna Cerritos Blancos (Tiner et al., 2018), Poaceae pollen % (Martel-Cea et al., 2016), diatom record axis 1 PCA scores (Martel-Cea et al., 2016), NDJF temperature anomalies (de Jong et al., 2013) from Laguna Chepical and lithic content in the marine record, site SO 147-106KL, and offshore Peru (Rein et al., 2005). Wetter periods are highlighted in gray.
From ∼1,900 to ∼600 cal. yr BP, the records of LCO and LQP suggest relatively humid conditions, with an increase in the indicators of the lower Andean belt. This result concurs with the records of Laguna Chepical, Cerritos Blancos and El Cepo (Martel-Cea et al., 2016; Tiner et al., 2018), and the coastal area of the same region (Maldonado and Villagrán, 2006). The greatest influx of carbon was found in this period, and thus it is the period in which the largest number of fire events is inferred. The greatest activity of ENSO was found from 2,000 to 600 cal. yr BP (Jenny et al., 2002; Moy et al., 2002; Rein et al., 2005) and thus high interannual variability in the study area, which would favor the generation of fires. The differences in the times of the most humid period of the last 3,000 years between the records at 30°S and those further south may be related to a greater impact of the precipitations associated with ENSO in the north. Although the ENSO is also important further south, the dynamics of the westerly winds independent of the ENSO years may also explain the humid period beginning at 3,000 cal. yr BP.
Our records of the last ∼600 years again show an increase in the indicators of the sub-Andean belt, associated with a relatively dry condition. This result coincides with studies performed in high Andean lakes in north–central Chile (Martel-Cea et al., 2016; Tiner et al., 2018) and in the coastal area of central Chile (Maldonado and Villagrán, 2006). The record of fossil pollen, in addition to detecting changes in the climate, is capable of giving clues about the origin of these changes (Li et al., 2008). Several studies show that changes in vegetation can be due to natural factors or related to human activities (Zhao et al., 2010; Paus, 2013). Thus, current climate change can be addressed through pollen analysis, and this information can be used to better analyze the consequences of climate change for biodiversity.
5.3 Alpha and Beta Diversity
The changes in the composition of the communities (ecological changes), used as a proxy of beta diversity (Correa-Metrio et al., 2014), were not constant during the last ∼4,500 years. Ecological changes were related to environmental conditions in both lakes. The dry/humid/dry transition which occurred at ∼1,900 and ∼600 cal. yr BP is related to the decrease or increase in ecological change values. This variation in the composition of taxa may be because the new conditions generated by environmental changes generate ecological filters, which only allow some taxa to establish (Püttker et al., 2014). The coincidences between transition periods (niche changes) and increases or decreases in ecological change may indicate that when there are environmental changes, communities assemble due to deterministic processes related to the niche more than due to random processes (Püttker et al., 2014). The humid period showed greater values of ecological changes than the dry periods in both lakes.
Our study is the first to analyze changes in plant communities in terms of alpha and beta diversity in northern Chile, which makes it hard to compare with patterns found in other studies. However, studies of community ecology may be considered to analyze paleoecological patterns, since both disciplines study community dynamics with respect to spatial or environmental changes (Jackson and Blois, 2015). For example, studies in grasslands in semiarid areas of Africa (Anderson, 2008), California (Eskelinen and Harrison, 2015), and Asia (Zhang et al., 2014) show that sites with higher humidity have greater change in the community composition. One of the patterns observed in beta diversity is that it increases with productivity (Chase and Leibold, 2002; Chase, 2010). Productivity in our study area is mainly related to water availability; more precipitation yields more productivity (Jaksic, 2001; Rudloff et al., 2021). The relation between productivity and beta diversity may be one of the reasons that the humid period from ∼1,900 to ∼600 cal. yr BP had the highest values of ecological changes in the two lakes studied, since higher humidity would have produced greater productivity.
Species richness (alpha diversity) in both lakes oscillated slightly during the last 4,000 years but did not undergo abrupt changes. This stability of richness over time was described in pollen records (Haskell, 2001; Giesecke et al., 2012) and in rodents (Brown et al., 2001; Sax, 2002), amphibians, birds, and invertebrates (Sax, 2002). The stability of alpha diversity in many organisms was found at scales from tens to thousands of years. This may be related to the carrying capacity of ecosystems, which is related to resource availability; as long as the latter does not undergo large changes, richness is expected to be stable (Brown et al., 2001). Although the records of both lakes showed changes in environmental conditions, they were not sufficiently great to change stability in richness. The period of our study (∼4,500 years) did not have extreme changes in environmental conditions compared to other periods such as the end of the Pleistocene (Kull and Grosjean, 2000) or the middle Holocene (Maldonado et al., 2010). The stability of richness may also be influenced by problems of taxonomic resolution of pollen grains. In our study, this would have reduced the number of taxa, especially of the Poaceae, in which there were probably various species, which would not allow us to distinguish the true richness of plant species in the study area, and thus its variation in time. The problem of taxonomic resolution may be overcome using molecular techniques, for example, the use of sedimentary DNA, which improves the detection of species in paleoecological studies (Liu et al., 2020).
6 Conclusion
The sedimentary composition of the cores showed glacial activity before ∼4,000 cal. yr BP, suggesting glacial retreat around this time above 3,800 m a.s.l.. Although we cannot use these records to decide when the last period of glacial advance began, we can indicate that it ended around 4,000 years ago, which may be related to the global event around ∼4,200 cal. yr BP.
The records of pollen and carbon analyzed differentiated three contrasting periods during the last ∼4,500 years. From the beginning of the sequences until ∼1,900 cal. yr BP, the pollen ensembles suggest relatively dry climate conditions, with a slight tendency to more humid conditions after 2,700 cal. yr BP. From ∼1,900 to ∼600 cal. yr BP, the pollen records suggest more humid conditions than the present day, with more winter rains, which would be associated with greater influence of westerly winds and/or a decrease in the south Pacific subtropical anticyclone, which also coincides with greater ENSO activity and greater fire events in the study area, associated with high climatic interannual and/or intra-annual variability. Finally, in the last ∼600 years, relatively arid conditions have appeared again. This chronology and direction of changes concurs with other records available for the region, showing that climate oscillations had a regional influence in the last ∼4,500 years. Differences with the records from south of 32°S may be explained by a more important influence of ENSO activity in the study area than further south.
The analysis of diversity shows that the climate influences mainly the composition of taxa in the communities (beta diversity) but not in richness (alpha diversity). Beta diversity increases in dry–humid transition periods and decreases in humid–dry transition periods. Richness in transition periods remained relatively constant, suggesting equilibrium between extinction and local migration, which marks changes in species composition but not large oscillations in richness.
The results obtained in this study show how the Andean flora has behaved from a glacial retreat, passing to relatively dry and/or warm, humid and/or cold conditions, and again to relatively dry and/or warm conditions, associated with fluctuations in the intensity of the westerly wind belt in the area, suggesting increases in the vegetation belts in the warm and/or dry periods and decreases in the cold and/or humid periods, maintaining the alpha diversity but changing the beta diversity. These changes, mainly those associated with increased dry and/or warm conditions, may show how the vegetation is expected to behave in the future considering the current climate change scenarios for the area, which suggest an increase in the zero isotherm, temperatures in general, and decreases in precipitation.
Data Availability Statement
The original contributions presented in the study are included in the article/Supplementary Material, further inquiries can be directed to the corresponding author.
Author Contributions
CM and AM contributed to the conception and design of the study. CM wrote the first draft of the manuscript. CM and AM contributed to manuscript revision and approved the submitted version.
Funding
The funding for this work was provided by the ANID-FONDECYT #1180413, CONICYT-R16A10003, ANID-Millennium Science Initiative Program—NCN19_153, and ANID-“Concurso de Fortalecimiento al Desarrollo Científico de Centros Regionales 2020-R20F0008-CEAZA.”
Conflict of Interest
The authors declare that the research was conducted in the absence of any commercial or financial relationships that could be construed as a potential conflict of interest.
Publisher’s Note
All claims expressed in this article are solely those of the authors and do not necessarily represent those of their affiliated organizations, or those of the publisher, the editors, and the reviewers. Any product that may be evaluated in this article, or claim that may be made by its manufacturer, is not guaranteed or endorsed by the publisher.
Acknowledgments
We thank Marigen Heise and Jeremy Acevedo for their assistance during the coring collection campaign.
Supplementary Material
The Supplementary Material for this article can be found online at: https://www.frontiersin.org/articles/10.3389/feart.2022.833219/full#supplementary-material
References
Aceituno, P. (1988). On the Functioning of the Southern Oscillation in the South American Sector. Part I: Surface Climate. Mon. Wea. Rev. 116, 505–524. doi:10.1175/1520-0493(1988)116<0505:OTFOTS>2.0.CO;2
Altamirano, A., Salas, C., Yaitul, V., Smith-Ramirez, C., and Ávila, A. (2013). Influencia de la heterogeneidad del paisaje en la ocurrencia de incendios forestales en Chile Central. Rev. Geogr. Norte Gd. 55, 157–170. doi:10.4067/s0718-34022013000200011
Birks, H. J. B., Heiri, O., Seppä, H., and Bjune, A. (2011). Strengths and Weaknesses of Quantitative Climate Reconstructions Based on Late-Quaternary Biological Proxies. Toecolj 3, 68–110. doi:10.2174/1874213001003020068
Blaauw, M., and Christen, J. A. (2011). Flexible Paleoclimate Age-Depth Models Using an Autoregressive Gamma Process. Bayesian Anal. 6 (3), 457–474. doi:10.1214/11-BA61810.1214/ba/1339616472
Blarquez, O., Carcaillet, C., Frejaville, T., and Bergeron, Y. (2014). Disentangling the Trajectories of Alpha, Beta and Gamma Plant Diversity of North American Boreal Ecoregions since 15,500 Years. Front. Ecol. Evol. 2, 1–8. doi:10.3389/fevo.2014.00006
Brown, J. H., Ernest, S. K. M., Parody, J. M., and Haskell, J. P. (2001). Regulation of Diversity: Maintenance of Species Richness in Changing Environments. Oecologia 126 (3), 321–332. doi:10.1007/s004420000536
Cavieres, L. A., Peñaloza, A., and Kalin Arroyo, M. (2000). Altitudinal Vegetation Belts in the High-Andes of central Chile (33°S). Rev. Chil. Hist. Nat. 73, 331–344. doi:10.4067/S0716-078X2000000200008
Cepeda, J., and Novoa, J. (2006). “La cordillera altoandina de la cuenca del Río Elqui,” in Los Sistemas Naturales de la Cuenca del Río Elqui. Editor J. Cepeda (La Serena: Ediciones Universidad de La Serena), 551.
Chase, J. M., and Leibold, M. A. (2002). Spatial Scale Dictates the Productivity-Biodiversity Relationship. Nature 416, 427–430. doi:10.1038/416427a
Chase, J. M. (2010). Stochastic Community Assembly Causes Higher Biodiversity in More Productive Environments. Science 328, 1388–1391. doi:10.1126/science.1187820
Comunidad Agrícola Estancia Estero Derecho (CAEED) (2017). Plan de Manejo para la Conservación del Área Protegida Privada y Santuario de la Naturaleza Estero Derecho. La Serena, Chile: DocPlayer, 77.
Correa Metrio, A., Dechnik, Y., Lozano García, S., and Caballero, M. (2014). Detrended Correspondence Analysis: A Useful Tool to Quantify Ecological Changes from Fossil Data Sets. Bsgm 66 (1), 135–143. doi:10.18268/bsgm2014v66n1a10
de Jong, R., von Gunten, L., and Maldonado, A. (2013). Late Holocene Summer Temperatures in the Central Andes Reconstructed From the Sediments of High-Elevation Laguna Chepical, Chile (32° S). Clim.Past. 9, 1921–1932. doi:10.5194/cp-9-1921-2013
de Porras, M., and Maldonado, A. (2018). Metodologías Y Avances De La Palinología Del Cuaternario Tardío a Lo Largo De La Diagonal Árida Sudamericana. Peapa 18 (2), 18–38. doi:10.5710/PEAPA.08.07.2018.255
Eskelinen, A., and Harrison, S. P. (2015). Resource Colimitation Governs Plant Community Responses to Altered Precipitation. Proc. Natl. Acad. Sci. USA 112 (42), 13009–13014. doi:10.1073/pnas.1508170112
Espizua, L. E. (2005). Holocene Glacier Chronology of Valenzuela Valley, Mendoza Andes, Argentina. The Holocene 15 (7), 1079–1085. doi:10.1191/0959683605hl866rr
Faegri, K., and Iversen, J. (1989). Textbook of Pollen Analysis (Fourth). New York: John Wiley & Sons.
Giesecke, T., Wolters, S., Jahns, S., and Brande, A. (2012). Exploring Holocene Changes in Palynological Richness in Northern Europe-Ddid Postglacial Immigration Matter? PLOS ONE 7 (12), e51624–11. doi:10.1594/PANGAEA.73842910.1371/journal.pone.0051624
González, M. E., Lara, A., Urrutia, R., and Bosnich, J. (2011). Cambio climático y su impacto potencial en la ocurrencia de incendios forestales en la zona centro-sur de Chile (33° - 42° S). Bosque (Valdivia) 32 (3), 215–219. doi:10.4067/S0717-92002011000300002
Gosling, W. D., Julier, A. C. M., Adu-Bredu, S., Djagbletey, G. D., Fraser, W. T., Jardine, P. E., et al. (2018). Pollen-vegetation Richness and Diversity Relationships in the Tropics. Veget Hist. Archaeobot 27, 411–418. doi:10.1007/s00334-017-0642-y
Haskell, J. (2001). The Latitudinal Gradient of Diversity through the Holocene as Recorded by Fossil Pollen in Europe. Evol. Ecol. Res. 3, 345–360.
Heusser, C. J., and Moar, N. T. (1973). Pollen and Spores of Chile: Modern Types of the Pteridophyta, Gymnospermae, and Angiospermae. New Zealand J. Bot. 11. 389–391. doi:10.1080/0028825X.1973.10430287
Higuera, P. E., Brubaker, L. B., Anderson, P. M., Hu, F. S., and Brown, T. A. (2009). Vegetation Mediated the Impacts of Postglacial Climate Change on Fire Regimes in the South-central Brooks Range, Alaska. Ecol. Monogr. 79 (2), 201–219. doi:10.1890/07-2019.1
Hogg, A. G., Heaton, T. J., Hua, Q., Palmer, J. G., Turney, C. S., Southon, J., et al. (2020). SHCal20 Southern Hemisphere Calibration, 0-55,000 Years Cal BP. Radiocarbon 62, 759–778. doi:10.1017/RDC.2020.59
Jackson, S. T., and Blois, J. L. (2015). Community Ecology in a Changing Environment: Perspectives from the Quaternary. Proc. Natl. Acad. Sci. USA 112 (16), 4915–4921. doi:10.1073/pnas.1403664111
Jaksic, F. M. (2001). Ecological Effects of El Niño in Terrestrial Ecosystems of Western South America. Ecography 24, 241–250. doi:10.1111/j.1600-0587.2001.tb00196.x
Jenny, B., Valero-Garcés, B. L., Villa-Martínez, R., Urrutia, R., Geyh, M., and Veit, H. (2002). Early to Mid-holocene Aridity in Central Chile and the Southern Westerlies: The Laguna Aculeo Record (34°S). Quat. Res. 58, 160–170. doi:10.1006/qres.2002.2370
Kaiser, J., Schefuß, E., Lamy, F., Mohtadi, M., and Hebbeln, D. (2008). Glacial to Holocene Changes in Sea Surface Temperature and Coastal Vegetation in north central Chile: High versus Low Latitude Forcing. Quat. Sci. Rev. 27, 2064–2075. doi:10.1016/j.quascirev.2008.08.025
Kull, C., and Grosjean, M. (2000). Late Pleistocene Climate Conditions in the north Chilean Andes Drawn from a Climate-Glacier Model. J. Glaciol. 46 (155), 622–632. doi:10.3189/172756500781832611
Li, Y., Zhou, L., and Cui, H. (2008). Pollen Indicators of Human Activity. Sci. Bull. 53, 1281–1293. doi:10.1007/s11434-008-0181-0
Liu, S., Stoof-leichsenring, K. R., Kruse, S., Pestryakova, L. A., and Herzschuh, U. (2020). Holocene Vegetation and Plant Diversity Changes in the North-Eastern Siberian Treeline Region from Pollen and Sedimentary Ancient DNA. Front. Ecol. Evol. 8, 1–17. doi:10.3389/fevo.2020.560243
López-Angulo, J., Pescador, D. S., Sánchez, A. M., Mihoč, M. A. K., Cavieres, L. A., and Escudero, A. (2018). Determinants of High Mountain Plant Diversity in the Chilean Andes: From Regional to Local Spatial Scales. PLOS ONE 13 (7), e0200216–16. doi:10.1371/journal.pone.0200216
Losapio, G., Cerabolini, B. E. L., Maffioletti, C., Tampucci, D., Gobbi, M., and Caccianiga, M. (2021). The Consequences of Glacier Retreat Are Uneven between Plant Species. Front. Ecol. Evol. 8, 1–11. doi:10.3389/fevo.2020.616562
Maldonado, A., Méndez, C., Ugalde, P., Jackson, D., Seguel, R., and Latorre, C. (2010). Early Holocene Climate Change and Human Occupation along the Semiarid Coast of north-central Chile. J. Quat. Sci. 25, 985–988. doi:10.1002/jqs.1385
Maldonado, A., and Villagrán, C. (2006). Climate Variability over the Last 9900 Cal Yr BP from a Swamp forest Pollen Record along the Semiarid Coast of Chile. Quat. Res. 66, 246–258. doi:10.1016/j.yqres.2006.04.003
Mander, L., and Punyasena, S. W. (2014). On the Taxonomic Resolution of Pollen and Spore Records of Earth's Vegetation. Int. J. Plant Sci. 175, 931–945. doi:10.1086/677680
Markgraf, V., and D’Antoni, H. (1978). Pollen flora of Argentina. Tucson: The University of Arizona Press.
Martel-Cea, A., Maldonado, A., Grosjean, M., Alvial, I., de Jong, R., Fritz, S. C., et al. (2016). Late Holocene Environmental Changes as Recorded in the Sediments of High Andean Laguna Chepical, Central Chile (32°S; 3050 M a.s.l.). Palaeogeogr. Palaeoclimatol. Palaeoecol. 461, 44–54. doi:10.1016/j.palaeo.2016.08.003
Méndez, C., Gil, A., Neme, G., Nuevo Delaunay, A., Cortegoso, V., Huidobro, C., et al. (2015). Mid Holocene Radiocarbon Ages in the Subtropical Andes (∼29°-35° S), Climatic Change and Implications for Human Space Organization. Quat. Int. 356, 15–26. doi:10.1016/j.quaint.2014.06.059
Michael Anderson, T. (2008). Plant Compositional Change over Time Increases with Rainfall in Serengeti Grasslands. Oikos 117, 675–682. doi:10.1111/j.0030-1299.2008.16516.x
Montecinos, A., and Aceituno, P. (2003). Seasonality of the ENSO-Related Rainfall Variability in Central Chile and Associated Circulation Anomalies. J. Clim. 16 (2), 281–296. doi:10.1175/1520-0442(2003)016<0281:SOTERR>2.0.CO;2
Moy, C. M., Seltzer, G. O., Rodbell, D. T., and Anderson, D. M. (2002). Variability of El Niño/Southern Oscillation Activity at Millennial Timescales during the Holocene Epoch. Nature 420, 162–165. doi:10.1038/nature01163.110.1038/nature01194
Oksanen, J., Blanchet, F. G., Friendly, M., Kindt, R., Legendre, P., Mcglinn, D., et al. (2015). Vegan. Community Ecology Package (Version 2.2-1). Available at: https://cran.r-project.org/web/packages/vegan/index.html.
O’Reilly, B. C., Finkelstein, S. A., and Bunbury, J. (2014). Pollen-Derived Paleovegetation Reconstruction and Long-Term Carbon Accumulation at a Fen Site in the Attawapiskat River Watershed, Hudson Bay Lowlands, Canada. Arctic, Antarctic, Alpine Res. 46 (1), 6–18. doi:10.1657/1938-4246-46.1.6
Orme, L. C., Miettinen, A., Divine, D., Husum, K., Pearce, C., Van Nieuwenhove, N., et al. (2018). Subpolar North Atlantic Sea Surface Temperature since 6 Ka BP: Indications of Anomalous Ocean-Atmosphere Interactions at 4-2 Ka BP. Quat. Sci. Rev. 194, 128–142. doi:10.1016/j.quascirev.2018.07.007
Paus, A. (2013). Human Impact, Soil Erosion, and Vegetation Response Lags to Climate Change: Challenges for the Mid-scandinavian Pollen-Based Transfer-Function Temperature Reconstructions. Veget Hist. Archaeobot 22 (3), 269–284. doi:10.1007/s00334-012-0360-4
Pellicciotti, F., Ragettli, S., Carenzo, M., and McPhee, J. (2014). Changes of Glaciers in the Andes of Chile and Priorities for Future Work. Sci. Total Environ. 493, 1197–1210. doi:10.1016/j.scitotenv.2013.10.055
Püttker, T., de Arruda Bueno, A., Prado, P. I., and Pardini, R. (2014). Ecological Filtering or Random Extinction? Beta-Diversity Patterns and the Importance of Niche-Based and Neutral Processes Following Habitat Loss. Oikos 124 (2), 206–215. doi:10.1111/oik.01018
Quintana, J. (2012). Changes in the Rainfall Regime along the Extratropical West Coast of South America ( Chile ): 30-43o S. Atmósfera 25 (1), 1–22.
Rein, B., Lückge, A., Reinhardt, L., Sirocko, F., Wolf, A., and Dullo, W.-C. (2005). El Niño Variability off Peru during the Last 20,000 Years. Paleoceanography 20, a–n. doi:10.1029/2004PA001099
Rudloff, V. M., Rutllant, J. A., Martel-Cea, A., and Maldonado, A. (2021). Hydrothermal Modulation of NDVI in the High-Altitude Semiarid Andes of Chile (30-34°S). J. Arid Environments 186, 104397. doi:10.1016/j.jaridenv.2020.104397
Sarricolea, P., Herrera-ossandon, M., and Meseguer-ruiz, Ó. (2016). Climatic Regionalisation of continental Chile. J. Maps 13 (2), 66–73. doi:10.1080/17445647.2016.1259592
Sax, D. F. (2002). Equal Diversity in Disparate Species Assemblages: a Comparison of Native and Exotic Woodlands in California. Glob. Ecol. Biogeogr. 11, 49–57. doi:10.1046/j.1466-822x.2001.00262.x
Schickhoff, U., Singh, R. B., and Mal, S. (2016). “Climate Change and Dynamics of Glaciers and Vegetation in the Himalaya: An Overview,” in Climate Change, Glacier Response, and Vegetation Dynamics in the Himalaya. Editors R. Singh, U. Schickhoff, and S. Mal (Cham: Springer), 1–26. doi:10.1007/978-3-319-28977-910.1007/978-3-319-28977-9_1
Souvignet, M., Oyarzún, R., Verbist, K. M. J., Gaese, H., and Heinrich, J. (2012). Hydro-meteorological Trends in Semi-arid north-central Chile (29-32°S): Water Resources Implications for a Fragile Andean Region. Hydrological Sci. J. 57 (3), 479–495. doi:10.1080/02626667.2012.665607
Squeo, F., Tracol, Y., López, D., León, M., and Gutiérrez, J. (2009). “Vegetación nativa y variación temporal de la productividad en la Provincia del Elqui,” in Los Sistemas Naturales de la Cuenca del Río Elqui. Editor J. Cepeda (La Serena, Chile: Ediciones Universidad de La Serena).
Stockmarr, J. (1971). Tablets with Spores Used in Absolute Pollen Analysis. Pollen Spores 13, 614–621. doi:10.1046/j.1466-822x.2001.00262.x
Tiner, R. J., Negrini, R. M., Antinao, J. L., McDonald, E., and Maldonado, A. (2018). Geophysical and Geochemical Constraints on the Age and Paleoclimate Implications of Holocene Lacustrine Cores from the Andes of central Chile. J. Quat. Sci. 33 (2), 150–165. doi:10.1002/jqs.3012
Valois, R., Schaffer, N., Figueroa, R., Maldonado, A., Yáñez, E., Hevia, A., et al. (2020). Characterizing the Water Storage Capacity and Hydrological Role of Mountain Peatlands in the Arid Andes of North-Central Chile. Water 12, 1071. doi:10.3390/w12041071
Veblen, T., Young, K., Orme, A., and Hinojosa, L. F. (2007). “The Physical Geography of South America,” in The Physical Geography of South America. Editors T. T. Velben, K. R. Young, and A. R. Orme (New York: Oxford University Press), 184–199. doi:10.1093/oso/9780195313413.001.0001
Velásquez, R., Coloma, F., Murillo, I., Merino, R. N., and Ortiz, M. (2021). Geología de las áreas Pisco Elqui y Paso del Agua Negra, región de Coquimbo. Servicio Nacional de Geología y Minería, Carta Geológica de Chile. Serie Geología Básica 211-212, 201.
Villagrán, C., Arroyo, M., and Marticorena, C. (1983). Efectos de la desertización en la distribución de la flora andina de Chile. Revista Chilena de Historia Natural 56, 137–157.
Villa-Martínez, R., Villagrán, C., and Jenny, B. (2003). The Last 7500 Cal Yr B.P. Of westerly Rainfall in Central Chile Inferred from a High-Resolution Pollen Record from Laguna Aculeo (34°S). Quat. Res. 60, 284–293. doi:10.1016/j.yqres.2003.07.007
Wanner, H., Mercolli, L., Grosjean, M., and Ritz, S. P. (2015). Holocene Climate Variability and Change; a Data-Based Review. J. Geol. Soc. 172, 254–263. doi:10.1144/jgs2013-101
Whitlock, C., Higuera, P. E., Mcwethy, D. B., and Briles, C. E. (2010). Paleoecological Perspectives on Fire Ecology: Revisiting the Fire-Regime Concept∼!2009-09-02∼!2009-11-09∼!2010-03-05∼!. Toecolj 3, 6–23. doi:10.2174/1874213001003020006
Whitlock, C., and Larsen, C. (2002). “Charcoal as a Fire Proxy,” in Tracking Environmental Change Using Lake Sediments. Editors J. P. Smol, H. J. B. Birks, and W. M. Last (Dordrecht: Springer), 75–97. doi:10.1007/0-306-47668-1_5
Woodward, F. I., and Williams, B. G. (1987). Climate and Plant Distribution at Global and Local Scales. Vegetatio 69, 189–197. doi:10.1007/BF00038700
World Water Council (2009). Vulnerability of Arid and Semi-arid Regions to Climate Change. Cairo, Egypt: Perspectives on Water and Climate Change Adaptation.
Zhang, Q., Hou, X., Li, F. Y., Niu, J., Zhou, Y., Ding, Y., et al. (2014). Alpha, Beta and Gamma Diversity Differ in Response to Precipitation in the Inner Mongolia Grassland. PLOS ONE 9 (3), e93518–9. doi:10.1371/journal.pone.0093518
Zhao, Y., Chen, F., Zhou, A., Yu, Z., and Zhang, K. (2010). Vegetation History, Climate Change and Human Activities over the Last 6200years on the Liupan Mountains in the Southwestern Loess Plateau in central China. Palaeogeogr. Palaeoclimatol. Palaeoecol. 293, 197–205. doi:10.1016/j.palaeo.2010.05.020
Keywords: subtropical Andes, pollen records, late Holocene (∼4 ka), southern westerly wind belt, alpine vegetation, alpha diversity, beta diversity
Citation: Mayta C and Maldonado A (2022) Climatic and Ecological Changes in the Subtropical High Andes During the Last 4,500 Years. Front. Earth Sci. 10:833219. doi: 10.3389/feart.2022.833219
Received: 10 December 2021; Accepted: 08 February 2022;
Published: 04 March 2022.
Edited by:
Neil Franklin Glasser, Aberystwyth University, United KingdomReviewed by:
Maarten Blaauw, Queen’s University Belfast, United KingdomAlberto Saez, University of Barcelona, Spain
Copyright © 2022 Mayta and Maldonado. This is an open-access article distributed under the terms of the Creative Commons Attribution License (CC BY). The use, distribution or reproduction in other forums is permitted, provided the original author(s) and the copyright owner(s) are credited and that the original publication in this journal is cited, in accordance with accepted academic practice. No use, distribution or reproduction is permitted which does not comply with these terms.
*Correspondence: Antonio Maldonado, antonio.maldonado@ceaza.cl