- 1Key Laboratory of Western China’s Environmental Systems (MOE), College of Earth and Environmental Sciences, Lanzhou University, Lanzhou, China
- 2Shiyang River Basin Scientific Observing Station, Lanzhou University, Lanzhou, China
Glaciers have melted rapidly in the Yarlung Zangbo River Basin due to recent climate change, which has accelerated the expansion of glacial lakes. We performed a systematic survey of glacial lakes in the Yarlung Zangbo River Basin using the Google Earth Engine platform. This allowed us to map the distribution and monitor the evolution of glacial lakes over seven time periods from 1990 to 2020, using more than 140 Landsat Thematic Mapper and Operational Land Imager images at a 30-m resolution. The results show that there were 2088 glacial lakes in 2020, with a total area of 245.87 km2, mainly distributed at elevations of 3,800–5,800 m above sea level. From 1990 to 2020, the Yarlung Zangbo River Basin glacial lakes expanded by approximately 1.81 km2·a−1. A total of 153 glacial lakes in the Yarlung Zangbo River Basin were assessed. The assessment shows that 153 glacial lakes were divided into very high, high, medium, low, and very low level. The number of glacial lakes in each level is 23, 22, 32, 32, and 44, respectively. The very high and high level glacial lakes were mainly found in the Yi’ong Zangbo River Basin, Parlung Zangbo River Basin, the western of the Yarlung Zangbo River Basin and Nianchu River Basin. These glacial lakes deserve further attention and monitoring.
1 Introduction
Glacial lakes are natural water bodies where either the main recharge source is modern glacial melt water or water accumulated in a moraine dam depression (Qin et al., 2014; Yao et al., 2018). The formation and development of glacial lakes are an indicator of climate change (Richardson and Reynolds, 2000; Gardelle et al., 2011; Khanal et al., 2015; Emmer et al., 2016; Duan et al., 2020). The glacial lake are important globally and regionally (Wilson et al., 2018) as a considerable water resource (Haeberli et al., 2016). Furthermore, they have the potential for hydroelectric power generation (Terrier et al., 2015; Farinotti et al., 2019). However, there can also be hazards associated with glacial lakes. The floods and debris flow from glacial lake outburst have the potential to threaten downstream communities and cause serious damage to valuable infrastructure, agricultural land, and property (Beniston et al., 1997; Carey et al., 2012; Sun et al., 2014; Clague and O'Connor, 2015; Haeberli et al., 2016; Wilson et al., 2018).
It is a well-established fact that global warming in recent years has led to a series of cryospheric issues, including glacial retreat and the expansion of glacial lakes (Pritchard, 2019; Shugar et al., 2020). Furthermore, in many regions enhanced glacier mass loss promotes the growth of glacial lakes, which can result in an increase in catastrophic damage and fatalities if the lakes outburst (Nie et al., 2017; Shugar et al., 2020).
Owing to the remote geographical locations of most glacial lakes, field investigations have high material and financial costs. Therefore, the combination of remote sensing and geographic information systems are extremely useful for the study of glacial lakes, especially in glacial lake mapping (Huggel et al., 2002; Bolch et al., 2011; Shugar et al., 2020). A multi-process extraction method based on the water index has been developed to extract glacial lakes using various remote sensing images (Huggel et al., 2002; Nie et al., 2017; Wang et al., 2020). However, there are some shortcomings, especially when the number of images is large, which requires significant labor and computer performance. Fortunately, the Google Earth Engine (GEE), one of the big data platforms for remote sensing, is a relatively robust platform that harnesses cloud computing to analyze massive quantities of geospatial data (Pekel et al., 2016; Gorelick et al., 2017). Several studies on glacial lakes have been reported based on the GEE platform (Chen et al., 2017; Shugar et al., 2020; Khadka et al., 2021; Rinzin et al., 2021).
Current studies on glacial lakes on the Tibetan Plateau and its surrounding areas mainly include the distribution and variation of glacial lakes (Nie et al., 2013; Nie et al., 2017; Duan et al., 2020), the causes of changes to glacial lakes (Xin Wang et al., 2011; Nie et al., 2017), the identification of potential high risk glacial lakes (Weicai Wang et al., 2011; Liu et al., 2019; Duan et al., 2020), and analysis of typical glacial lake outburst mechanisms (Sun et al., 2014; Nie et al., 2020). Glacial lakes are strongly dependent on season; therefore accurate mapping requires images from the same season, preferably autumn (Xie et al., 2013). However, owing to the extreme weather conditions, it is difficult to capture a sufficient quantity of suitable remote sensing images in areas near the Tibetan Plateau (Liu et al., 2019). Consequently, the temporal resolution of most studies on glacial lakes is usually coarse (commonly 2–4 time periods), which results in an overly idealized standard for new and vanished glacial lakes.
The Yarlung Zangbo River Basin (YZRB) is one of the most concentrated areas of mountain glaciers in China (Figure 1). There are 10,816 glaciers, with an area of approximately 14,493 km2, accounting for 25.5% and 33.6% of the total number and area of glaciers in China, respectively (Guo et al., 2015). The glaciers in the YZRB have retreated significantly in response to recent climate change (Yao et al., 2010; Pritchard, 2019), which may have led to variation in glacial lakes and potential hazards (Yao et al., 2019; Zhu et al., 2019).
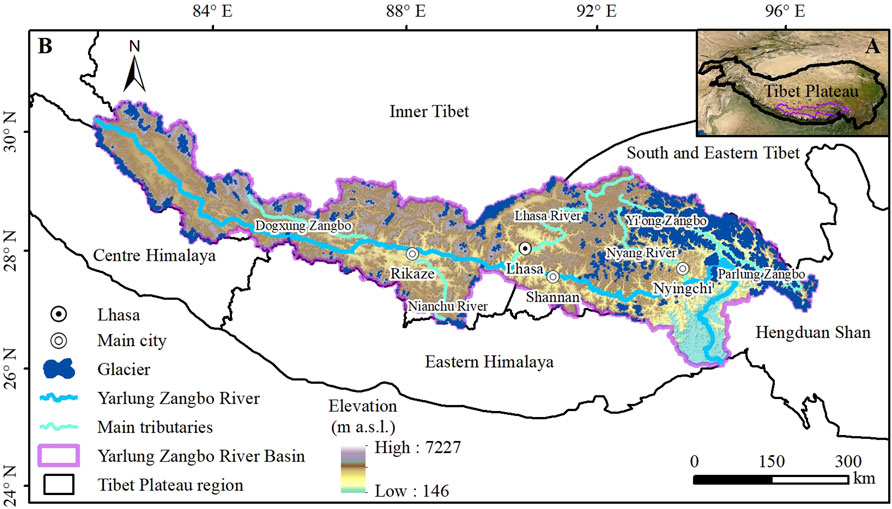
FIGURE 1. (A) Location of the YZRB in relation to the Tibetan Plateau. (B) Overview of the distribution of glaciers in the YZRB.
At present, research on glacial lakes in the YZRB has mainly focused on the eastern region where the concentration of glaciers is highest (Liu et al., 2019; Duan et al., 2020; Qi et al., 2020). However, the type of glacier and the climate change characteristics in the eastern YZRB are very different to the western YZRB. Obtaining a high temporal resolution of glacial lake changes in the YZRB will help analyze the response of glacial lakes to climate and glacier changes, as well as improve risk assessments of glacial lake outbursts. Therefore, this study had three key objectives. The first was to map glacial lakes and analyze the change in characteristics in the YZRB using a series of Landsat images from 1990 to 2020 on the GEE platform. We particularly wished to identify glacial lakes which had recently appeared or disappeared, to a relatively reasonable standard. The second objective was to determine the reasons for changes in glacial lakes in the YZRB, and how the changes are influenced by climate change. Finally, we wished to identify glacial lakes in the YZRB that pose a significant hazard.
2 Study Area
Located in the Tibetan Plateau, the Yarlung Zangbo River is the longest plateau river in China and one of the highest rivers in the world. It originates from the Gyima Yangzoin Glacier on the northern slope of the Himalayas with a total length of 2057 km and a basin area of 240480 km2, ranging from 80.12°–97.38° E to 27.26°–28.54° N (Figure 1). It then merges with the Brahmaputra River and the Ganges in Goelundo to become the Padma River, which also involves the river water of Meghna around the delta before injecting into the Bay of Bengal (Yao et al., 2010).
The total annual runoff of the Yarlung Zangbo River is about 1.40 × 1011 m3, accounting for 42.4% of the outflow river runoff in Tibet (Liu et al., 2007). It has a population of 1.30 × 106 in the YZRB, accounting for 50.7% of the total population of Xizang. The Yarlung Zangbo River has many tributaries, among which the basin area is greater than 1 × 104 km2, from upstream to downstream there are Dogxung Zangbo, Nianchu River, Lhasa River, Nyang River and Parlung Zangbo, among which the Lhasa River basin is the largest with an area of about 3.26 × 104 km2 (Liu et al., 2007).
The precipitation in the YZRB mainly comes from the warm and humid air in the Bay of Bengal in the Indian Ocean (Jia et al., 2008). Affected by topographic factors, precipitation decreases rapidly from the lower reaches to the upper reaches. The precipitation in the basin varies greatly, mainly from June to September (Jia et al., 2008). The runoff is large, the annual distribution is seriously uneven, and the proportion of flood season is large. Snowmelt recharge and groundwater recharge accounted for a large and stable proportion, with little interannual variation (Jia et al., 2008).
3 Materials and Methods
3.1 Data Source
Images that cover the entire YZRB in a given year are difficult to obtain because of the highly frequent cloud cover across the region (Burns and Nolin, 2014; Nie et al., 2017). Therefore, to ensure the temporal accuracy of the changes in glacial lakes, images covering the whole YZRB within a period of 5 years were used instead. Thus, more than 140 Landsat Thematic Mapper (TM) and Landsat Operational Land Imager (OLI) images, with a spatial resolution of 30 m, were used to map the extents of lakes in 1990, 1995, 2000, 2005, 2010, 2015, and 2020 on the GEE platform. All available high-quality images were acquired primarily within ±2 years of each period. If suitable images were still lacking for a certain period, data from adjacent periods were used instead to ensure data integrity. To reduce the influence of season, images from September to November with low cloud cover were selected (Figure 2).
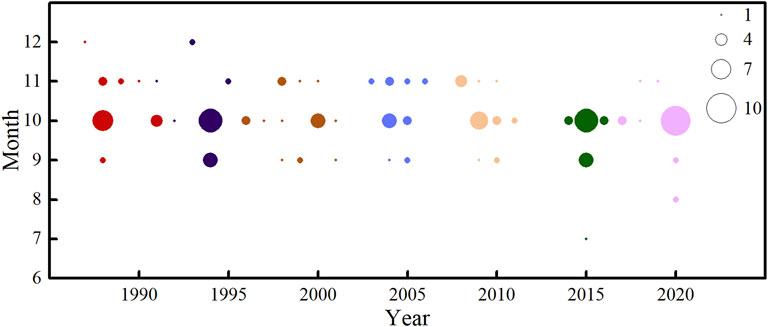
FIGURE 2. Time phase statistics showing the temporal distribution of Landsat images of the study area.
The other datasets used in this study were the Advanced Spaceborne Thermal Emission and Reflection Radiometer (ASTER) Global Digital Elevation Model (GDEM) V2, the Second Chinese Glacier Inventory, and the fifth-generation ECMWF atmospheric reanalysis of the global climate (ERA5) (Table 1). The ASTER GDEM was used to assist in the extraction of glacial lakes and the division of altitude zones, while data from the Glacier Inventory were used to extract glacier attributes. Based on the data, a buffer distance of 10 km was used on glacier outlines to exclude glacial lakes that were assumed to have weak interactions with current glaciers (Wang et al., 2013; Zhang et al., 2015; Nie et al., 2017). ERA5 data were used to explore the impact of climate on glacial lake changes.
3.2 Glacial Lake Extraction
A traditional method used to extract glacial lakes is the normalized difference water index (NDWI) that is based on the green and near infrared bands (McFeeters, 1996; Shugar et al., 2020; Wang et al., 2020). However, this method cannot easily distinguish water from snow and shadows. Therefore, in this study we used a modified NDWI (MNDWI) method, which can reduce the effect of shadows on water bodies (Xu, 2006; Chen et al., 2017; Qi et al., 2020). The MNDWI method is expressed as (BGreen−BSWIR)/(BGreen+BSWIR), where BGreen and BSWIR are the green and short-wave infrared bands of the image (Xu, 2006). After repeated experiments, we set the MNDWI threshold to 0.3. An additional threshold in Bred was included for improved mapping in the snow. Bred is mainly used to distinguish between snow and water. Within red band wavelength range, snow has a high reflectivity (usually > 0.5), and the reflectance of water with low chlorophyll content is very low (usually < 0.1).
Because lakes are flat, GDEMv2 data were used to calculate the slope in the study area, and only pixels in which the slope was less than 20° were retained (Zhang et al., 2019). This resulted in many detrital pixels in the images. Therefore, morphological image processing was used to filter detrital pixels and small water bodies by opening (erosion first and then dilation). Finally, the results were manually modified to correct, add, and delete non-glacial lake water bodies (such as river channels), and set the minimum mapping unit to 0.01 km2 (Wang et al., 2020). Then, all lakes within 10 km buffer zone from the nearest glacier were included and classified into three types based on their positions in relation to the glaciers: supraglacial lakes on the surface of glaciers, connected lakes at the lateral or terminal position of the glacier and unconnected lakes far from glaciers (Wang et al., 2016). The classification process is based on the relative position of glacial lakes and glaciers. The entire process was divided into two parts, based on the GEE and ArcGIS platforms (Figure 3).
Previous studies have typically estimated the uncertainties in glacial lake area from the linear error and the lake’s perimeter (Wang et al., 2012b; Nie et al., 2017; Duan et al., 2020). In this study, considering the interference of mixed pixels at the lake boundaries, lake outline uncertainty was determined based on ± 0.5 pixels:
where ua is the area uncertainty of a glacial lake, r is the image’s pixel resolution, and p is the perimeter of the lake.
3.3 Standard of Newly Formed and Vanished Glacial Lakes
To investigate the regularity of expansion and reduction of glacial lakes, a linear regression method (Zhang et al., 2019) for each glacial lake was used to estimate the trend of multi-year lake measurements (Figure 4). A rate of glacial lake change (Rgl) greater than 50 m2·a−1 was considered to be expansion, while a Rgl less than −50 m2·a−1 was reduction. A Rgl between 50 and −50 m2·a−1 indicated a stable glacial lake. This method allowed us to discriminate between new and vanished glacial lakes. In this study, new glacial lakes were defined as lakes that were present in images from 2005 to 2020, but were not observed in three consecutive periods from 1990 to 2000. Vanished glacial lakes were defined as those that existed in four periods from 1990 to 2005, but were not found in three consecutive periods from 2010 to 2020 (Figure 4).
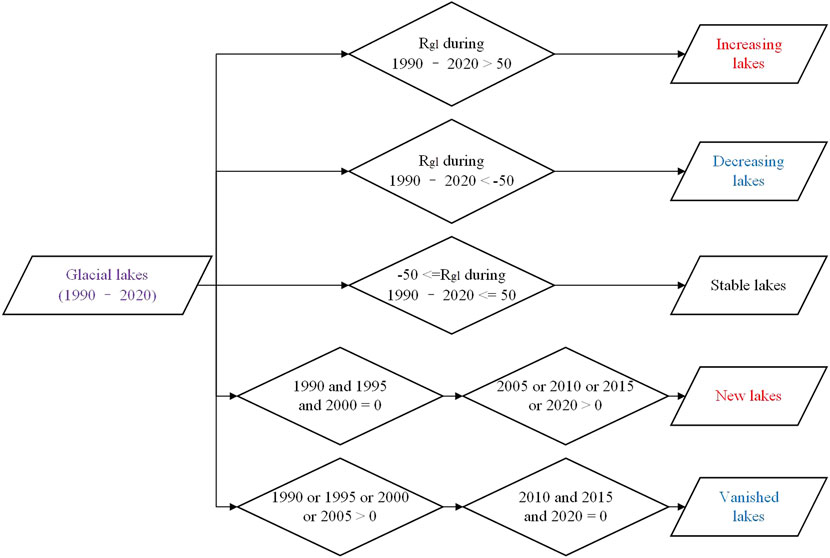
FIGURE 4. Criteria for categorizing glacial lakes as increasing, decreasing, stable, newly appeared, and vanished. The units in the figure are m2·a−1.
3.4 Identification of Dangerous Glacial Lakes
Glacial lake outburst flood (GLOF) can bring serious disasters and terrible consequences (Haeberli et al., 2016; Wilson et al., 2018). In the YZRB, GLOF has brought several disasters (Lv et al., 1999; Sun et al., 2014; Liu et al., 2021). Therefore, in order to prevent or reduce the disasters and serious consequences caused by glacial lake outburst, it is necessary to carry out hazard assessment research on the glacial lake in the YZRB. At present, studies on the risk assessment of glacial lakes in the YZRB mainly focus on some sub-basins, such as Parlung Zangbo River Basin (Liu et al., 2019), Yi’ong Zangbo River Basin (Duan et al., 2020) and Nyang River Basin (Qi et al., 2020). In addition, there are also some assessment studies in the Tibetan Plateau (Allen et al., 2019; Zheng et al., 2021). Indicators such as lake area, mass movement entering into glacial lakes, glacier size and retreat, expansion rate of glacial lake, and distance between the lake and the glacier terminus are commonly used (Bolch et al., 2011; Allen et al., 2019; Liu et al., 2019; Duan et al., 2020; Qi et al., 2020; Zheng et al., 2021). For first-order hazard assessment, attributes such as causes of historical GLOF, availability and applicability of remote sensing data, and suitability of automation are commonly employed (Bolch et al., 2011; Rinzin et al., 2021). Accordingly, here we selected the following hazard assessment factors: 1) glacial lake area, 2) lake expansion rate, 3) distance from glacier to lake, 4) total watershed area and 5) topographic potential. These five factors all meet the following three criteria: 1) easy to obtain from existing remote sensing data; 2) according to the characteristics of lake outburst in the YZRB; 3) objectivity. In this study, each glacial lake has a different ID for tracking research. Assuming that the differing intensity of the particular factor poses a varying degree of GLOF hazard to the lake, the quantified value of each element was categorized into two or three alternative classes: high, medium, and low (Table 2). Based on the previous literature, we assigned an index value of 1 to high, 0.5 to medium, and 0.25 to the low alternative classes (Bolch et al., 2011; Khadka et al., 2021; Rinzin et al., 2021).
Glacial lake area is a commonly used index for glacial lake hazard assessment. Considering the reported minimum area of glacial lake outburst in the YZRB is 0.045 km2 (Nie et al., 2018; Zheng et al., 2021), the 0.045 km2 area is the lower limit of our research in this study therefore. While some glacial lakes larger than 0.5 km2 will cause serious disasters when outburst occurs (Jiweng Co. and Ranzeria Co.) (Sun et al., 2014; Liu et al., 2021), so glacial lakes larger than 0.5 km2 are classified as high alternative class.
Previous studies adopted the relative threshold of glacial lake expansion to evaluate the risk of lake outburst, which has also been proved to be one of the important criteria for hazard assessment (Bolch et al., 2011; Khadka et al., 2021; Rinzin et al., 2021). Most of the glacial lakes experienced significant expansion process before outburst (Lv et al., 1999; Liu et al., 2019; Khadka et al., 2021). Therefore, only glacial lakes with an expansion rate greater than 10% are considered in this assessment. Higher expansion rate will be given a higher index value.
The dynamics of the mother glaciers are directly associated with the occurrence of GLOF, and the distance between the lake and the mother glacier is the most direct measure of the degree of their linkage (Wang et al., 2012a). In relevant studies on the Tibet Plateau, the glacial lakes which are 1 km away from the glacier were taken into account (Allen et al., 2019). Weicai Wang et al. (2011) used less than 500 m threshold and considered it to be more favorable value for a lake to outburst. Therefore, we adopted the distance of 500 m as the research threshold in the study. If glacial lakes are in contact with glaciers, they will be given a higher index value.
Total watershed area of the lake, recognizing the potential for runoff from heavy rainfall or glacier/snow melt to drain into and overwhelm a glacial lake (Worni et al., 2012; Allen et al., 2015). A larger upstream watershed is considered to increase the potential for runoff (Allen et al., 2019). Therefore, a larger watershed area would be classified as a higher alternative class and vice versa.
The last is the threat posed by topographic potential around glacial lake. Potential for ice and rock avalanches to strike a lake, thereby capturing the main process responsible for pervious GLOF triggering in the TP (Weicai Wang et al., 2011). At the basin scale, we do not distinguish between whether the slope is bedrock, ice-covered or debris. Here, following Allen et al. (2019), the topographic potential area was defined as a 30° slope within the lake’s watershed area and with a threshold reach angle of 14°. We calculated the area of each glacial lake watershed that reached the extreme slope threshold (30°) and divided in different alternative class.
In summary, a total of 153 glacial lakes greater than 0.045 km2, with an area expansion greater than 10% and within 500 m from the glacier in the YZRB were assessed. To further evaluate the level of potential risk posed by glacial lakes, it was necessary to quantify the risk score of each glacial lake using the potential risk score index (PRSI) (Weicai Wang et al., 2011; Khadka et al., 2021; Rinzin et al., 2021):
where n is the number of factors, wi is the weight of the factor, and vi is the index value. Because each factor has different impacts on the potential risk of a glacial lake outburst, it was also necessary to assign weight to each variable. The Analytic Hierarchy Process (AHP) method was used to assign the weights of each factor. The AHP is a semi-quantitative decision-making approach using weights through pairwise comparison between different factors without inconsistencies (Saaty, 1990). The AHP method has been used in the risk assessment research related to glacial lakes to determine the weight of each risk factor (Khadka et al., 2021; Rinzin et al., 2021).
Here, our expert judgment for the priority ranking of factors was influenced by the previous causes of GLOFs in the YZRB and other existing researches (Bolch et al., 2011; Allen et al., 2019; Khadka et al., 2021; Rinzin et al., 2021). We assigned the highest rank to topographic potential include ice/snow avalanche and landslide/rockfall, which is the most important factor leading to glacial lake outburst (Wang et al., 2016; Nie et al., 2018). We also assigned a relatively high ranking to watershed area, considering it a dangerous factor of lake outburst (Allen et al., 2019). In the initial screening, indexes such as glacial lake area, glacial lake expansion and distance from glacier to lake were used, so the weight of these three indexes was relatively low (Table 3). Finally, the PRSI of each lake ranged from 0 to 1. Here, based on the PRSI, the hazard potential of the lake was categorized into five groups, namely, very low (0–0.3), low (0.3–0.4), medium (0.4–0.6), high (0.6–0.9) and very high (0.9–1.0). Considering the number of glacial lakes distributed in each range, the division of each group is not equally spaced.
4 Results
4.1 Distribution and Variation of Glacial Lakes
4.1.1 Distribution Characteristics of Glacial Lakes
In 2020, there were a total of 2088 developed glacial lakes in the YZRB, with a total area of 245.87 km2. They were mainly distributed in the eastern region of the basin, which was also an area with a high concentration of glaciers (Figure 5A). There was also a strong asymmetry in the number and area of relatively large and small glacial lakes. Glacial lakes smaller than 0.1 km2 accounted for 78.5% of the total number of glaciers in the YZRB, yet represented only 25.3% of the total glacial lake area. In contrast, glacial lakes larger than 1 km2 constituted only 1.5% of the total number of lakes, but 31.5% of the total area (Figure 5B). Among the lakes, 36 (2.08 ± 0.37 km2) are supraglacial, 146 (47.52 ± 3.11 km2) are connected-glacier and 1900 (193.81 ± 23.58 km2) are unconnected-glacier.
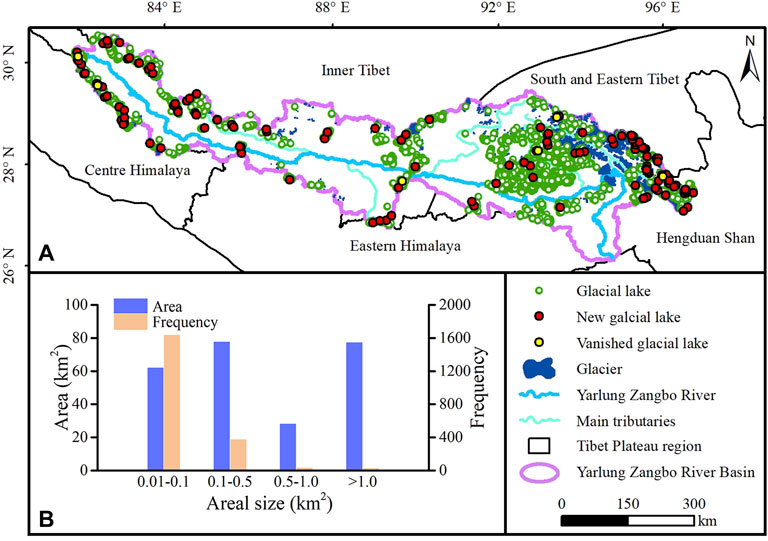
FIGURE 5. (A) Distribution of glacial lakes in the YZRB in 2020 (The new and vanished glacial lakes are between 1990 and 2020); (B) frequency and area of glacial lakes of different sizes in 2020.
Hypsography can provide useful information to understand how regional topography and glacier distribution influences glacial lakes. Figure 6 shows the hypsography of glacial lake frequency, lake area, and glacier area, at 100 m elevation intervals. Overall, glacial lakes and glaciers had very similar elevation distributions, with more than 80% of the glaciers and glacial lakes in the YZRB distributed between 3,800 and 5,800 m (Figure 6). There were very few glacial lakes below 3,800 m and above 5,800 m, and those that were had small areas. It is worth noting that in the YZRB there was a low frequency of glacial lakes between 3,800–4,100 m, but the total glacial lake area was high, indicating that individual lakes at this elevation range had large areas (Figure 6).
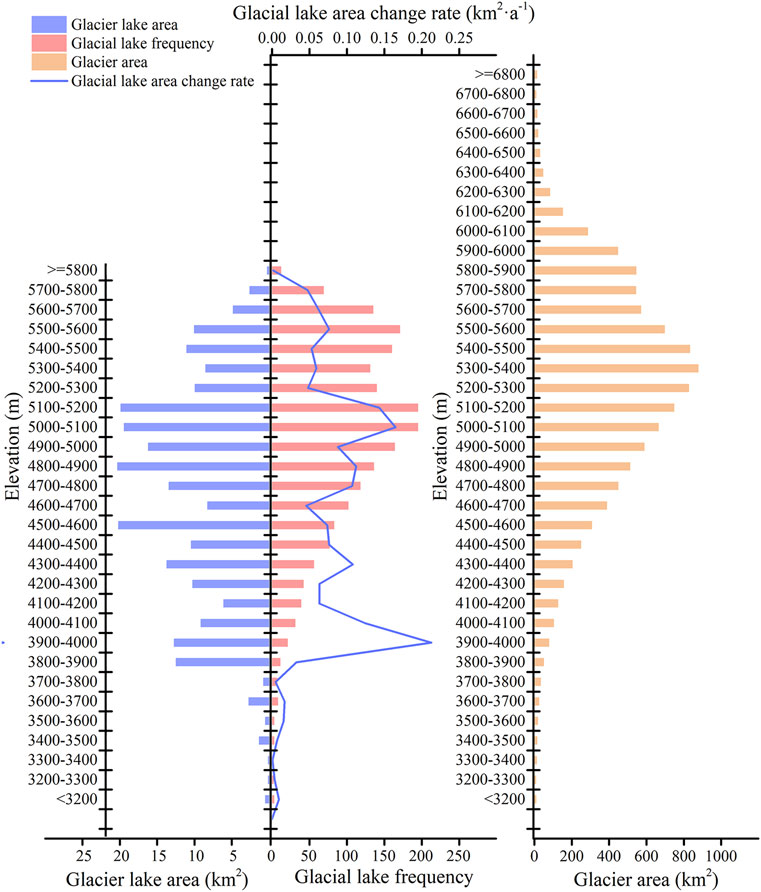
FIGURE 6. Altitudinal distribution showing the total glacial lake area, glacial lake frequency, total glacier area, and the area change rate of glacial lakes at different elevations in the YZRB in 2020.
4.1.2 Variation Characteristics of Glacial Lakes
Overall, the total area of glacial lakes in the YZRB increased from 1990 to 2020, with an average area change rate of 1.81 km2·a−1. However, this change rate varied during that period, with a decrease in glacial lake area observed from 1990 to 1995, and a rapid increase in area after 2010 (Table 4).
The area change rate of glacial lakes in the YZRB also varied greatly at different elevations, with the highest area change rate observed between 5,000 and 5,200 m (Figure 6). The results also show that the distribution trend of the number of glacial lakes and the trend of area change rate are similar in different altitude zones; the higher the frequency of lakes at a specific elevation, the higher the change rate. The same was also seen for the distribution of glaciers. However, it is worth noting that the change rate of glacial lakes between 3,800 and 4,100 m was abnormally high. This may be due to the individual contribution of large glacial lakes. For example, Jiongpu Co. (approximately 3,950 m above sea level) has expanded by approximately 3.7 km2 in the past 30 years, which accounts for half of the expansion of glacial lakes in the 3,800–4,100 m altitude range.
4.2 Newly Formed and Vanished Glacial Lakes
According to the method outlined in Section 3, we determined the specific change status of glacial lakes in the YZRB. Approximately 1,607, 184, and 325 glacial lakes in the basin were determined to be expanding, shrinking, and remaining relatively stable, respectively. Of the expanding glacial lakes, 153 were determined to be new, while six of the shrinking glacial lakes were determined to have vanished (Figure 5A). There were 23, 35, 64, and 31 new glacial lakes in 2005, 2010, 2015, 2020, respectively. There were 1, 4 and 1 vanished glacial lakes in 1995, 2005, 2010, respectively. Glacial lakes that did not appear in the first three periods and appeared in the last four periods were defined as new glacial lakes (Figures 7A,B). Glacial lakes that appeared in the first four periods and did not appear in the last three periods were defined as vanished glacial lakes (Figures 7C,D). However, if a glacial lake disappeared only in a certain period, it will not be defined as a new or vanished glacial lake.
4.3 Dangerous Glacial Lakes
Based on the criteria established in Section 3.4, we assessed 153 glacial lakes in the YZRB. The assessment shows that 153 glacial lakes were divided into very high, high, medium, low and very low level. The number of glacial lakes in each level is 23, 22, 32, 32, and 44, respectively. The very high and high level glacial lakes were mainly found in the Yi’ong Zangbo River Basin, Parlung Zangbo River Basin and the western of the YZRB, which also a few of the two levels distributed in the Nianchu River Basin. The medium level glacial lakes have similar distribution with the former two. The low level glacial lakes and the very low glacial lakes have more extensive distribution (Figure 8).
Among 23 very high level glacial lakes, typical glacial lakes include Jiongpu Co., Shimo Co., Laigu Co., Guangxie Co., and Jiweng Co. (Figures 9A–E). Jiongpu Co. is located in the Yi’ong Zangbo River Basin. It covered an area of about 5.40 km2 in 2020, which is the largest glacial lake assessed in the YZRB. In the last 3 decades, it has expanded by more than 200%. The lake’s watershed area is large and has the highest possibility of ice and rock avalanche. Shimo Co. is located in the Nianchu River Basin, covering an area of 1.94 km2. The lake has expanded by more than 100% in the past 30 years. The watershed area and the possibility of ice and rock avalanche are both high. Laigu Co. is located at the end of Yanong Glacier in the Parlung Zangbo River Basin. It covered an area of 2.86 km2 and has expanded by about 163% in the last 30 years. The lake has the largest watershed area of glacial lakes assessed. Located in the Parlung Zangbo River Basin, Guangxie Co. is the lake at the end of Midui Glacier. It occurred outburst event in 1988 (Lv et al., 1999). After that, the area gradually expanded and even exceeded the area before the outburst. The watershed area and the risk of ice and rock avalanche also belong to the high group. It is worth noting that, a very high level glacial lake, named Jiweng Co. located in the Yi’ong Zangbo River Basin, has not unexpectedly outburst in June 2020. Unfortunately, the image used here was captured in 2019 (no suitable images were available in 2020).
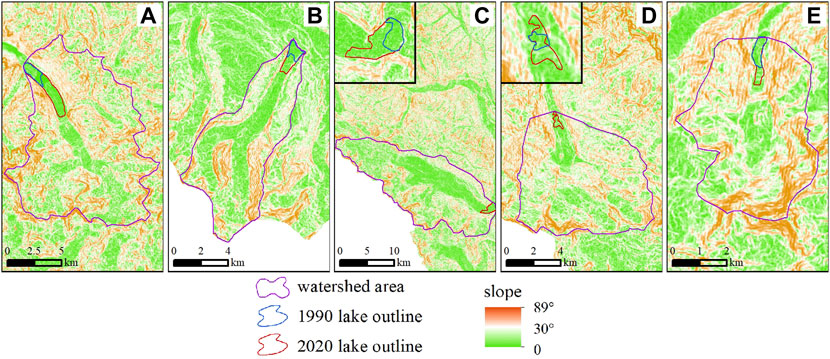
FIGURE 9. Examples of dangerous glacial lakes in the YZRB: (A) Jiongpu Co., (B) Shimo Co., (C) Laigu Co., (D) Guangxie Co., (E) Jiweng Co.
5 Discussion
5.1 Reasons for Glacial Lake Variation
There are both external and internal causes for the changes to the glacial lakes in the YZRB between 1990 and 2020. External causes for glacial lake changes mainly include glacier distribution, and climate factors such as temperature and precipitation. ERA5 meteorological reanalysis data with a spatial resolution of 0.25° (Hersbach et al., 2020), were used to analyze the influence of climate on the changes to glacial lakes. Analysis of interannual variation shows that precipitation has decreased over the past 30 years, especially from 1987 to 1997. Air temperature also decreased from 1987 to 1997; however, there was a marked increase over the subsequent 20 years, resulting in overall warming (Figure 10A). A significant inverse correlation was also observed between the distance of the glacial lake to the mother glacier and the change rate of the glacial lake (Figure 10B). Glacial lakes near to their mother glacier had a significantly higher expansion rate than glacial lakes far from their mother glacier. Therefore, we infer that the expansion of glacial lakes in the YZRB during the investigated period, was primarily caused by melting of the glaciers, which occurred due to the increase in air temperature. Analysis and research on the causes of changes to glacial lakes in other areas of the Tibetan Plateau agree with our results (Yao et al., 2010; Nie et al., 2017). The decrease in the area of glacial lakes from 1990 to 1995 can be explained by the decrease in temperature and precipitation during this time, which reduced glacial melting. Furthermore, many glacial lakes appeared in the YZRB and Himalayas in 2015 (Nie et al., 2017), which may be related to the sudden increase in temperature in 2015–2016.
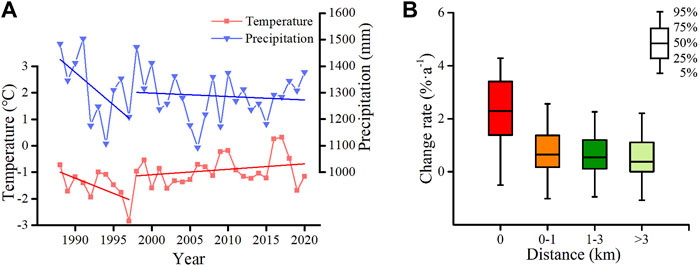
FIGURE 10. (A) Annual change in temperature and precipitation in the YZRB between 1988 and 2020. (B) Relationship between the distance of a glacial lake to its mother glacier and its change rate.
While recent temperature increases have been relatively uniform across the YZRB, precipitation has increased in the west but decreased in the east (Figures 11A,B). This data, combined with the spatial distribution of glacial lake change rate (Figure 11C), suggests three conditions that lead to more rapid expansion of glacial lakes: 1) A rapid increase in air temperature, 2) a rapid increase in precipitation, and 3) a higher concentration of glaciers.
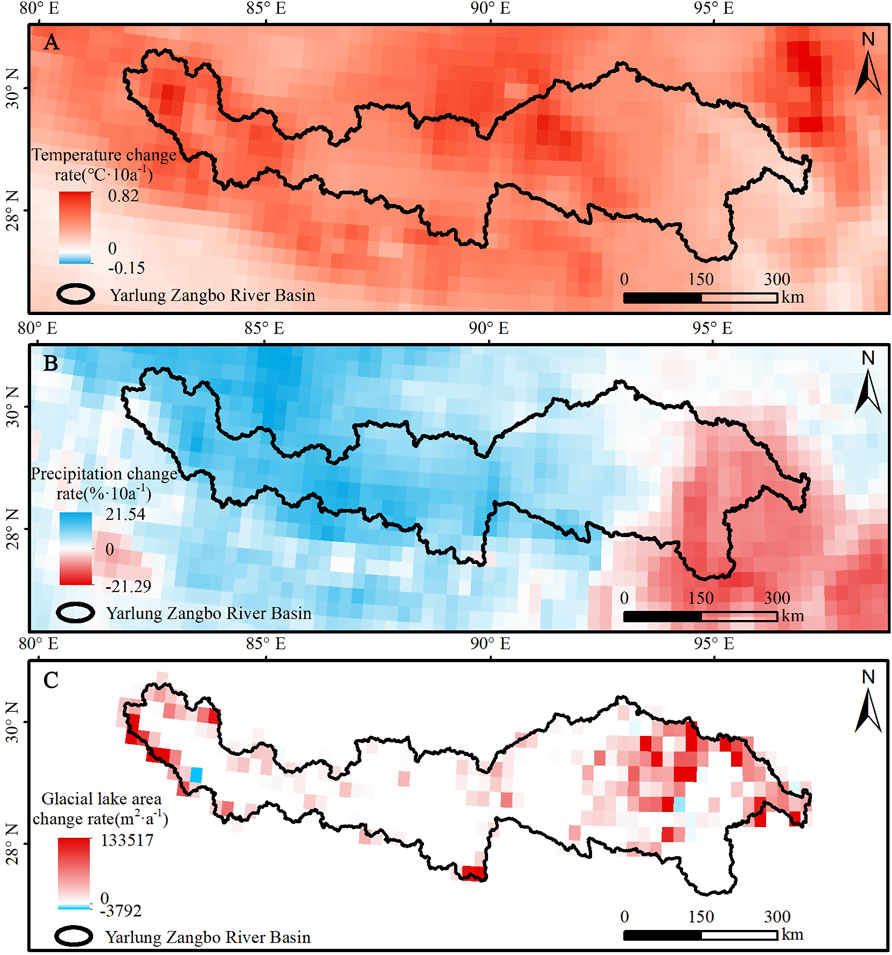
FIGURE 11. Spatial variations in temperature, precipitation and glacial lake area change rate in the YZRB from 1990 to 2020. (A) Rate of temperature change (°C·10a−1), (B) rate of precipitation change (%·10a−1), and (C) rate of glacial lake area change (m2·a−1).
Internal reasons for changes to glacial lakes are mainly the chain reactions caused by glacial lake outburst. Glacial lake outburst is typically accompanied by a sudden decrease in the area of the glacial lake and may even lead to the disappearance of the lake. Furthermore, the outburst of one glacial lake can sometimes result in the change of another glacial lake (Nie et al., 2020).
Glacial lakes have also been observed to be expanding in recent years in the Tibetan Plateau and its surrounding areas, such as the Himalayan (Xin Wang et al., 2011; Nie et al., 2013; Nie et al., 2017), Tianshan (Wang et al., 2013), and West Kunlun Mountain regions (Li et al., 2015). This expansion is likely due to glacier retreat caused by climate warming (Nie et al., 2017). Previous studies have also found that increasing air temperatures were statistically significant to the expansion of glacial lakes, while increases in precipitation were only significant in some areas (Wang et al., 2013). Researchers also suggested that the positions of mother glaciers relative to glacial lakes also contribute to the expansion of glacial lakes (Xin Wang et al., 2011). The interaction between glacial lakes and glaciers will further promote the change of both (Song et al., 2016; Song et al., 2017).
5.2 Risks and Identification of Dangerous Lakes
Several glacial lakes have experienced outburst events in the YZRB in recent decades, including Guangxie Co. in 1988 (Figure 9D; Lv et al., 1999) and Ranzeria Co. in 2013 (Sun et al., 2014). Some researchers have suggested that glacial lake outburst disasters have become more frequent over the past century (Wang et al., 2016). Glacial lake outbursts are a serious risk to downstream areas and human safety, and can cause severe damage to houses, vehicles, roads, bridges, and other infrastructure. In our study, we identified rapidly expanding glacial lakes that require further risk assessment. Except for Jiweng Co., some glacial lakes have also been mentioned in other studies, which further validates our method, such as Jiongpu Co. (Duan et al., 2020) and Shimo Co. (Li et al., 2019). By comparison, the assessment results in this study are in good agreement with Zheng et al. (2021). 16 (total of 23) of very high level glacial lakes in this study also belong to very high group from Zheng et al. (2021), which contains Jiongpu Co., Shimo Co., Guangxie Co., and Jiweng Co. Our assessment method and the dangerous lakes provide a basis for future monitoring studies and priorities.
However, the hazard assessment in this study largely focus on the possibility of ice and rockfall avalanche and watershed area. Less attention has been paid to glacial lake dams. Most existing studies have considered only the slope downstream of the glacial lake (Allen et al., 2019; Khadka et al., 2021; Rinzin et al., 2021). We believe that the material, looseness and other physical characteristics of the dam body are related to the hazard of glacial lakes, which cannot be obtained directly from images and are more dependent on field investigation. In addition, when identifying the potential risks of glacial lakes, lake volume rather than area must be known to realistically estimate potential peak discharge of a possible outburst (Huggel et al., 2002). Unfortunately, volume cannot be directly extracted from optical images. Although some studies have made efforts to estimate glacial lake reserves (Huggel et al., 2002; Yao et al., 2012; Shangguan et al., 2017), it is still not possible to widely determine glacial lake volumes from aerial images due to the different shapes of glacial lake bottoms. In the future, a combination of appropriate field investigations and simulation analysis is essential for determination of the potential risks of glacial lakes.
5.3 Glacial Lake Mapping, Comparison and Limitation
The GEE platform is a big data platform for remote sensing that has emerged in recent years and can conduct batch data processing on a cloud platform (Gorelick et al., 2017). The GEE platform has been proven to be feasible for glacial lake mapping in previous studies (Chen et al., 2017; Chen et al., 2020; Shugar et al., 2020; Khadka et al., 2021; Rinzin et al., 2021). In addition, the use of GEE to carry out glacial lake research increased significantly in last few years. The platform was mainly used in this study for the automatic extraction of glacial lakes in the YZRB.
By comparison with glacial lake inventory data of the Third Pole region (Zhang et al., 2015) and the HMA (Chen et al., 2020; Wang et al., 2020). We found that: in 1990, only 889 glacial lakes with a total area of 109.7 km2 were recorded by Zhang et al. (2015); while there were 4,173 glacial lakes with a total area of 294.5 km2 catalogued by Wang et al. (2020). And there were 1827 glacial lakes with a total area of 197.9 km2 were recorded in this study. In 2010, 1,028 glacial lakes with a total area of 116.0 km2 were recorded by Zhang et al. (2015), whereas we recorded 1,794 glacial lakes with a total area of 212.0 km2 in the YZRB. In 2015, 2,783 glacial lakes with a total area of 300.0 km2 were recorded by Chen et al. (2020), whereas we recorded 2017 glacial lakes with a total area of 236.3 km2. In 2018, 4,459 glacial lakes with a total area of 332.5 km2 were recorded by Wang et al. (2020), whereas we recorded 2,088 glacial lakes with a total area of 245.9 km2 in 2020.
The overall change trend of glacial lakes was similar in these studies, while there are some differences in the number and area of glacial lakes. We consider the discrepancies attributable to four primary factor: 1) first of all, the threshold of glacial lakes of Zhang et al. (2015), Wang et al. (2020), Chen et al. (2020) and this study were different, 0.0027, 0.0054, 0.0081, and 0.01 km2, respectively; 2) the buffer zone of the modern glacier extent was inconsistent between the data sets as the glacier inventories used were also different; 3) the images used from different season may result in various characteristics of the glacial lakes; 4) at last, in the process of visual correction, different operatives with their own experience also can vary outlines of glacial lakes.
6 Conclusion
In this study, we used remote sensing images in the GEE platform from 1990 to 2020, to extract glacial lake distribution and changes in the YZRB. A method was then used to identify new and vanished glacial lakes in the basin. The results show that there was a total glacial lake area of 245.87 ± 27.24 km2 in the YZRB in 2020. There was also a strong asymmetry in the number and area of large and small glacial lakes. Small lakes (<0.1 km2) accounted for 78.5% and 25.3% of the total number and area of lakes, respectively. However, large lakes (>1 km2) accounted for only 1.5% of the number of lakes, but 31.5% of the total lake area. The elevation distribution of glacial lakes and glaciers in the YZRB was similar, with more than 80% of glacial lakes and glaciers distributed between elevations of 3,800 and 5,800 m. Over the past 30 years, the areas of the glacial lakes in the YZRB have changed significantly, with an area variation rate of 1.81 km2 a−1. Most glacial lakes expanded during the observed time period, while only a few shrank, and a total of 153 new glacial lakes and six vanished glacial lakes were identified. Our analysis suggests that the main cause for the expansion of glacial lakes in the YZRB is the melting of glaciers caused by increases in atmospheric temperature.
We assessed 153 glacial lakes in the YZRB. The assessment shows that 153 glacial lakes were divided into very high, high, medium, low, and very low level. The number of glacial lakes in each level is 23, 22, 32, 32, and 44, respectively. The very high and high level glacial lakes were mainly found in the Yi’ong Zangbo River Basin, Parlung Zangbo River Basin and the western of the YZRB, which also a few of the two distributed in the Nianchu River Basin. The medium level glacial lakes have similar distribution with the former two. The low level glacial lakes and the very low glacial lakes have more extensive distribution. If these lakes were to outburst, the resultant floods and debris flows could result human fatalities and severe damage to downstream buildings, roads, and infrastructure. These lakes therefore require further monitoring and research to prevent or minimize the consequences if they burst.
Data Availability Statement
The raw data supporting the conclusion of this article will be made available by the authors, without undue reservation.
Author Contributions
XS designed the study, processed and analyzed data, and wrote the manuscript. BC designed the study, guided XS as a supervisor in processing and analyzing data, and writing this manuscript. BP designed this study and helped in writing the manuscript. KL helped in processing ERA5 data. XZ and WG helped in giving suggestions for the research. All authors read this manuscript and agreed to submit it to this journal.
Funding
This study is financially supported by the Second Tibetan Plateau Scientific Expedition and Research Program (STEP, grant No. 2019QZKK0205), Science and technology Project of Tibet Autonomous Region (grant No. XZ202101ZY0001G), and National Natural Science Foundation of China (grant No. 42071077).
Conflict of Interest
The authors declare that the research was conducted in the absence of any commercial or financial relationships that could be construed as a potential conflict of interest.
Publisher’s Note
All claims expressed in this article are solely those of the authors and do not necessarily represent those of their affiliated organizations, or those of the publisher, the editors and the reviewers. Any product that may be evaluated in this article, or claim that may be made by its manufacturer, is not guaranteed or endorsed by the publisher.
Acknowledgments
The authors thank editage for improving language during the preparation of this article.
References
Allen, S. K., Rastner, P., Arora, M., Huggel, C., and Stoffel, M. (2015). Lake Outburst and Debris Flow Disaster at Kedarnath, June 2013: Hydrometeorological Triggering and Topographic Predisposition. Landslides 13 (6), 1479–1491. doi:10.1007/s10346-015-0584-3
Allen, S. K., Zhang, G., Wang, W., Yao, T., and Bolch, T. (2019). Potentially Dangerous Glacial Lakes across the Tibetan Plateau Revealed Using a Large-Scale Automated Assessment Approach. Sci. Bull. 64 (7), 435–445. doi:10.1016/j.scib.2019.03.011
Beniston, M., Diaz, H. F., and Bradley, R. S. (1997). Climatic Change at High Elevation Sites: an Overview. Climatic Change 36, 233. doi:10.1023/A:1005380714349
Bolch, T., Peters, J., Yegorov, A., Pradhan, B., Buchroithner, M., and Blagoveshchensky, V. (2011). Identification of Potentially Dangerous Glacial Lakes in the Northern Tien Shan. Nat. Hazards 59 (3), 1691–1714. doi:10.1007/s11069-011-9860-2
Burns, P., and Nolin, A. (2014). Using Atmospherically-Corrected Landsat Imagery to Measure Glacier Area Change in the Cordillera Blanca, Peru from 1987 to 2010. Remote Sensing Environ. 140, 165–178. doi:10.1016/j.rse.2013.08.026
Carey, M., Huggel, C., Bury, J., Portocarrero, C., and Haeberli, W. (2012). An Integrated Socio-Environmental Framework for Glacier hazard Management and Climate Change Adaptation: Lessons from Lake 513, Cordillera Blanca, Peru. Climatic Change 112 (3-4), 733–767. doi:10.1007/s10584-011-0249-8
Chen, F., Zhang, M., Tian, B., and Li, Z. (2017). Extraction of Glacial Lake Outlines in Tibet Plateau Using Landsat 8 Imagery and Google Earth Engine. IEEE J. Sel. Top. Appl. Earth Obs. Remote Sensing 10 (9), 4002–4009. doi:10.1109/jstars.2017.2705718
Chen, F., Zhang, M., Guo, H., Allen, S., Kargel, J. S., Haritashya, U. K., et al. (2020). Annual 30-meter Dataset for Glacial Lakes in High Mountain Asia from 2008 to 2017. Earth Syst. Sci. Data. 13, 741–766. doi:10.5194/essd-2020-57
Clague, J. J., and O'Connor, J. E. (2015). “Glacier-Related Outburst Floods,” in Snow and Ice-Related Hazards, Risks, and Disasters. Pittsburgh: Academic Press, 487–519. doi:10.1016/b978-0-12-394849-6.00014-7
Duan, H., Yao, X., Zhang, D., Qi, M., and Liu, J. (2020). Glacial Lake Changes and Identification of Potentially Dangerous Glacial Lakes in the Yi'ong Zangbo River Basin. Water 12 (2), 538. doi:10.3390/w12020538
Emmer, A., Klimeš, J., Mergili, M., Vilímek, V., and Cochachin, A. (2016). 882 Lakes of the Cordillera Blanca: An Inventory, Classification, Evolution and Assessment of Susceptibility to Outburst Floods. Catena 147, 269–279. doi:10.1016/j.catena.2016.07.032
Farinotti, D., Round, V., Huss, M., Compagno, L., and Zekollari, H. (2019). Large Hydropower and Water-Storage Potential in Future Glacier-free Basins. Nature 575 (7782), 341–344. doi:10.1038/s41586-019-1740-z
Gardelle, J., Arnaud, Y., and Berthier, E. (2011). Contrasted Evolution of Glacial Lakes along the Hindu Kush Himalaya Mountain Range between 1990 and 2009. Glob. Planet. Change 75 (1-2), 47–55. doi:10.1016/j.gloplacha.2010.10.003
Gorelick, N., Hancher, M., Dixon, M., Ilyushchenko, S., Thau, D., and Moore, R. (2017). Google Earth Engine: Planetary-Scale Geospatial Analysis for Everyone. Remote Sensing Environ. 202, 18–27. doi:10.1016/j.rse.2017.06.031
Guo, W., Liu, S., Xu, J., Wu, L., Shangguan, D., Yao, X., et al. (2015). The Second Chinese Glacier Inventory: Data, Methods and Results. J. Glaciol. 61 (226), 357–372. doi:10.3189/2015JoG14J209
Haeberli, W., Buetler, M., Huggel, C., Friedli, T. L., Schaub, Y., and Schleiss, A. J. (2016). New Lakes in Deglaciating High-Mountain Regions - Opportunities and Risks. Climatic Change 139 (2), 201–214. doi:10.1007/s10584-016-1771-5
Hersbach, H., Bell, B., Berrisford, P., Hirahara, S., Horányi, A., Muñoz‐Sabater, J., et al. (2020). The ERA5 Global Reanalysis. Q. J. R. Meteorol. Soc. 146 (730), 1999–2049. doi:10.1002/qj.3803
Huggel, C., Kääb, A., Haeberli, W., Teysseire, P., and Paul, F. (2002). Remote Sensing Based Assessment of Hazards from Glacier lake Outbursts: a Case Study in the Swiss Alps. Can. Geotech. J. 39 (2), 316–330. doi:10.1139/t01-099
Jia, J., Lv, S., and Wang, Z. (2008). Characteristics of Water Resources in the Yarlung Zangbo River Basin. Yangtze River 39, 3. doi:10.16232/j.cnki
Khadka, N., Chen, X., Nie, Y., Thakuri, S., Zheng, G., and Zhang, G. (2021). Evaluation of Glacial Lake Outburst Flood Susceptibility Using Multi-Criteria Assessment Framework in Mahalangur Himalaya. Front. Earth Sci. 8, 601288. doi:10.3389/feart.2020.601288
Khanal, N. R., Mool, P. K., Shrestha, A. B., Rasul, G., Ghimire, P. K., Shrestha, R. B., et al. (2015). A Comprehensive Approach and Methods for Glacial lake Outburst Flood Risk Assessment, with Examples from Nepal and the Transboundary Area. Int. J. Water Resour. Develop. 31 (2), 219–237. doi:10.1080/07900627.2014.994116
Li, C., Yang, T., and Tian, h. (2015). Variation of Western Kunlun Mountain Glaciers Monitored by Remote Sensing During 1976-2010. Mountain Res. 33 (2), 157–165. doi:10.16089/j.cnki.1008-2786.000021
Li, L., Bianba, C., Zhao, W., Luosang, L., Da, P., and Zhaxi, O. (2019). Analysis of Change and Outburst Feature of Glacial lake in the Middle Himalayas of Tibet: Take Sangwang lake and Shimo lake as Examples. J. Glaciol. Geocryol. 41 (5), 1–10. doi:10.7522/j.issn.1000-0240.2019.0532
Liu, J., Yao, Z., and Chen, C. (2007). Evolution Trend and Causation Analysis of the Runoff Evolution in the Yarlung Zangbo River Basin. J. Nat. Resour. 22 (3), 471–477. doi:10.3321/j.issn:1000-3037.2007.03.017
Liu, J., Yao, X., Gao, Y., Qi, M., Duan, H., and Zhang, D. (2019). Glacial lake Variation and hazard Assessment of Glacial Lakes Outburst in the Parlung Zangbo River Basin. J. Lake Sci. 31 (4), 1132–1143. doi:10.18307/2019.0420
Liu, J., Zhou, L., Zhang, J., and Zhao, W. (2021). Characteristics of Jiwengcuo GLOF, Lhari County, Tibet. Geol. Rev. 67 (S01), 2. doi:10.16509/j.georeview
Lv, R., Tang, B., and Zhu, P. (1999). Debris Flow and Environment in Tibet. Chengdu: University of Science and Technology of Chengdu Press.
McFeeters, S. K. (1996). The Use of the Normalized Difference Water Index (NDWI) in the Delineation of Open Water Features. Int. J. Remote Sensing 17 (7), 1425–1432. doi:10.1080/01431169608948714
Nie, Y., Liu, Q., and Liu, S. (2013). Glacial lake Expansion in the central Himalayas by Landsat Images, 1990-2010. PLoS One 8 (12), e83973. doi:10.1371/journal.pone.0083973
Nie, Y., Sheng, Y., Liu, Q., Liu, L., Liu, S., Zhang, Y., et al. (2017). A Regional-Scale Assessment of Himalayan Glacial lake Changes Using Satellite Observations from 1990 to 2015. Remote Sensing Environ. 189, 1–13. doi:10.1016/j.rse.2016.11.008
Nie, Y., Liu, Q., Wang, J., Zhang, Y., Sheng, Y., and Liu, S. (2018). An Inventory of Historical Glacial lake Outburst Floods in the Himalayas Based on Remote Sensing Observations and Geomorphological Analysis. Geomorphology 308, 91–106. doi:10.1016/j.geomorph.2018.02.002
Nie, Y., Liu, W., Liu, Q., Hu, X., and Westoby, M. J. (2020). Reconstructing the Chongbaxia Tsho Glacial lake Outburst Flood in the Eastern Himalaya: Evolution, Process and Impacts. Geomorphology 370, 107393. doi:10.1016/j.geomorph.2020.107393
Pekel, J.-F., Cottam, A., Gorelick, N., and Belward, A. S. (2016). High-resolution Mapping of Global Surface Water and its Long-Term Changes. Nature 540 (7633), 418–422. doi:10.1038/nature20584
Pritchard, H. D. (2019). Asia's Shrinking Glaciers Protect Large Populations from Drought Stress. Nature 569 (7758), 649–654. doi:10.1038/s41586-019-1240-1
Qi, M.-m., Liu, S.-y., Yao, X.-j., Grünwald, R., Gao, Y.-p., Duan, H.-y., et al. (2020). Lake Inventory and Potentially Dangerous Glacial Lakes in the Nyang Qu Basin of China between 1970 and 2016. J. Mt. Sci. 17 (4), 851–870. doi:10.1007/s11629-019-5675-5
Qin, D., Yao, T., Ding, Y., and Ren, J. (2014). Glossary of Cryosphere Science. Beijing: China Meteorological Press.
Richardson, S. D., and Reynolds, J. M. (2000). An Overview of Glacial Hazards in the Himalayas. Quat. Int. 65-66, 31–47. doi:10.1016/S1040-6182(99)00035-X
Rinzin, S., Zhang, G., and Wangchuk, S. (2021). Glacial Lake Area Change and Potential Outburst Flood Hazard Assessment in the Bhutan Himalaya. Front. Earth Sci. 9, 775195. doi:10.3389/feart.2021.775195
Saaty, T. L. (1990). Multicriteria Decision Making: The Analytic Hierarchy Process: Planning, Priority Setting Resource Allocation. 2nd ed. Pittsburgh, Pa. USA: RWS Publications, 287.
Saaty, T. L. (2008). Decision Making with the Analytic Hierarchy Process. Int. J. Serv. Sci. 1 (1), 83. doi:10.1504/ijssci.2008.017590
Shangguan, D., Ding, Y., Liu, S., Xie, Z., Pieczonka, T., Xu, J., et al. (2017). Quick Release of Internal Water Storage in a Glacier Leads to Underestimation of the Hazard Potential of Glacial Lake Outburst Floods from Lake Merzbacher in Central Tian Shan Mountains. Geophys. Res. Lett. 44 (19), 9786–9795. doi:10.1002/2017gl074443
Shugar, D. H., Burr, A., Haritashya, U. K., Kargel, J. S., Watson, C. S., Kennedy, M. C., et al. (2020). Rapid Worldwide Growth of Glacial Lakes since 1990. Nat. Clim. Chang. 10 (10), 939–945. doi:10.1038/s41558-020-0855-4
Song, C., Sheng, Y., Ke, L., Nie, Y., and Wang, J. (2016). Glacial lake Evolution in the southeastern Tibetan Plateau and the Cause of Rapid Expansion of Proglacial Lakes Linked to Glacial-Hydrogeomorphic Processes. J. Hydrol. 540, 504–514. doi:10.1016/j.jhydrol.2016.06.054
Song, C., Sheng, Y., Wang, J., Ke, L., Madson, A., and Nie, Y. (2017). Heterogeneous Glacial lake Changes and Links of lake Expansions to the Rapid Thinning of Adjacent Glacier Termini in the Himalayas. Geomorphology 280, 30–38. doi:10.1016/j.geomorph.2016.12.002
Sun, M., Liu, S., Yao, X., and Li, L. (2014). The Cause and Potential hazard of Glacial lake Outburst Flood Occurred on July 5, 2013 in Jiali County, Tibet. J. Glaciol. Geocryol. 36 (1), 158–165. doi:10.7522/j.issn.1000-0240.2014.0020
Terrier, S., Bieri, M., Jordan, F., and Schleiss, A. J. (2015). Impact du retrait glaciaire et adaptation du potentiel hydroélectrique dans les Alpes suisses. La Houille Blanche 101 (1), 93–101. doi:10.1051/lhb/2015012
Wang, X., Liu, S., Ding, Y., Guo, W., Jiang, Z., Lin, J., et al. (2012a). An Approach for Estimating the Breach Probabilities of Moraine-Dammed Lakes in the Chinese Himalayas Using Remote-Sensing Data. Nat. Hazards Earth Syst. Sci. 12 (10), 3109–3122. doi:10.5194/nhess-12-3109-2012
Wang, X., Shiyin, L., Wanqin, G., Xiaojun, Y., Zongli, J., and Yongshun, H. (2012b). Using Remote Sensing Data to Quantify Changes in Glacial Lakes in the Chinese Himalaya. Mountain Res. Develop. 32 (2), 203–212. doi:10.1659/mrd-journal-d-11-00044.1
Wang, X., Ding, Y., Liu, S., Jiang, L., Wu, K., Jiang, Z., et al. (2013). Changes of Glacial Lakes and Implications in Tian Shan, central Asia, Based on Remote Sensing Data from 1990 to 2010. Environ. Res. Lett. 8 (4), 044052. doi:10.1088/1748-9326/8/4/044052
Wang, X., Liu, S., and Ding, Y. (2016). Study on Evaluation Method of Moraine lake Outburst Disaster in Himalaya of China and its Application. Beijing: Science Press.
Wang, X., Guo, X., Yang, C., Liu, Q., Wei, J., Zhang, Y., et al. (2020). Glacial lake Inventory of High-Mountain Asia in 1990 and 2018 Derived from Landsat Images. Earth Syst. Sci. Data 12 (3), 2169–2182. doi:10.5194/essd-12-2169-2020
Wang, W., Yao, T., Gao, Y., Yang, X., and Kattel, D. B. (2011). A First-Order Method to Identify Potentially Dangerous Glacial Lakes in a Region of the Southeastern Tibetan Plateau. Mountain Res. Develop. 31 (2), 122. doi:10.1659/mrd-journal-d-10-00059.1
Wilson, R., Glasser, N. F., Reynolds, J. M., Harrison, S., Anacona, P. I., Schaefer, M., et al. (2018). Glacial Lakes of the Central and Patagonian Andes. Glob. Planet. Change 162, 275–291. doi:10.1016/j.gloplacha.2018.01.004
Worni, R., Stoffel, M., Huggel, C., Volz, C., Casteller, A., and Luckman, B. (2012). Analysis and Dynamic Modeling of a Moraine Failure and Glacier lake Outburst Flood at Ventisquero Negro, Patagonian Andes (Argentina). J. Hydrol. 444-445, 134–145. doi:10.1016/j.jhydrol.2012.04.013
Xie, Z., ShangGuan, D., Zhang, S., Ding, Y., and Liu, S. (2013). Index for hazard of Glacier Lake Outburst Flood of Lake Merzbacher by Satellite-Based Monitoring of lake Area and Ice Cover. Glob. Planet. Change 107, 229–237. doi:10.1016/j.gloplacha.2012.05.025
Wang, X., Liu, S., Mo, H., Yao, X., Jiang, Z., and Guo, W. (2011). Expansion of Glacial Lakes and its Implication for Climate Changes in the Chinese Himalaya. Acta Geograph. Sin. 66 (7), 895–904. doi:10.11821/xb201107003
Xu, H. (2006). Modification of Normalised Difference Water index (NDWI) to Enhance Open Water Features in Remotely Sensed Imagery. Int. J. Remote Sensing 27 (14), 3025–3033. doi:10.1080/01431160600589179
Yao, T., Li, Z., Yang, W., Guo, X., Zhu, L., Kang, S., et al. (2010). Glacial Distribution and Mass Balance in the Yarlung Zangbo River and its Influence on Lakes. Chin. Sci. Bull. 55 (20), 2072–2078. doi:10.1007/s11434-010-3213-5
Yao, X., Liu, S., Sun, M., Wei, J., and Guo, W. (2012). Volume Calculation and Analysis of the Changes in Moraine-Dammed Lakes in the north Himalaya: a Case Study of Longbasaba lake. J. Glaciol. 58 (210), 753–760. doi:10.3189/2012JoG11J048
Yao, X., Liu, S., Han, L., Sun, M., and Zhao, L. (2018). Definition and Classification System of Glacial lake for Inventory and Hazards Study. J. Geogr. Sci. 28 (2), 193–205. doi:10.1007/s11442-018-1467-z
Yao, T., Li, S., Zhao, H., Yu, W., Liu, S., Wang, N., et al. (2019). Glacier Anomalies and Relevant Disaster Risks on the Tibetan Plateau and Surroundings. Chin. Sci. Bull. 64 (27), 2770–2782. doi:10.1360/tb-2019-0246
Zhang, G., Yao, T., Xie, H., Wang, W., and Yang, W. (2015). An Inventory of Glacial Lakes in the Third Pole Region and Their Changes in Response to Global Warming. Glob. Planet. Change 131, 148–157. doi:10.1016/j.gloplacha.2015.05.013
Zhang, G., Yao, T., Chen, W., Zheng, G., Shum, C. K., Yang, K., et al. (2019). Regional Differences of lake Evolution across China during 1960s-2015 and its Natural and Anthropogenic Causes. Remote Sensing Environ. 221, 386–404. doi:10.1016/j.rse.2018.11.038
Zheng, G., Allen, S. K., Bao, A., Ballesteros-Cánovas, J. A., Huss, M., Zhang, G., et al. (2021). Increasing Risk of Glacial lake Outburst Floods from Future Third Pole Deglaciation. Nat. Clim. Chang. 11 (5), 411–417. doi:10.1038/s41558-021-01028-3
Keywords: Google Earth Engine platform, Yarlung Zangbo River Basin, distribution of glacial lake, glacial lake change, identification of dangerous glacial lake
Citation: Sun X, Cao B, Pan B, Li K, Zhao X and Guan W (2022) Identification of Hazardous Glacial Lakes in the Yarlung Zangbo River Basin Based on Lakes Changes Determined Using Google Earth Engine. Front. Earth Sci. 10:825482. doi: 10.3389/feart.2022.825482
Received: 30 November 2021; Accepted: 31 January 2022;
Published: 18 February 2022.
Edited by:
Xin Wang, Hunan University of Science and Technology, ChinaReviewed by:
Guoqing Zhang, Institute of Tibetan Plateau Research (CAS), ChinaChunqiao Song, Nanjing Institute of Geography and Limnology (CAS), China
Copyright © 2022 Sun, Cao, Pan, Li, Zhao and Guan. This is an open-access article distributed under the terms of the Creative Commons Attribution License (CC BY). The use, distribution or reproduction in other forums is permitted, provided the original author(s) and the copyright owner(s) are credited and that the original publication in this journal is cited, in accordance with accepted academic practice. No use, distribution or reproduction is permitted which does not comply with these terms.
*Correspondence: Bo Cao, Y2FvYm9AbHp1LmVkdS5jbg==; Baotian Pan, cGFuYnRAbHp1LmVkdS5jbg==