- Birbal Sahni Institute of Palaeosciences, Lucknow, India
The origin, antiquity, and affinity of benthic seaweeds (multicellular algae) in the geological past are shrouded in mystery due to their preservation bias. In this study, we present a new material of well-preserved carbonaceous compression fossils in shale horizons of the Mesoproterozoic (ca. ∼1,500–1,300 Ma) Singhora Group of the Chhattisgarh Supergroup. Eleven distinct taxa, including one new taxon, Palaeoscytosiphon shuklaii, n. gen. et. sp., and one new species, Jiuqunaoella sergeevii, n. sp., are established. Four unidentified morphologies are also reported. Morphologically, the carbonaceous fossils are fan-shaped, palmate, elongated, leaf-like algal thalli with/without holdfast at the base, isolated or dichotomously branched long filaments, along with multicellular reproductive structures. The results of laser Raman spectroscopy and energy dispersive X-ray spectroscopy (EDX) are also presented in support of their biogenicity. Collectively, the preservation mode of the Singhora carbonaceous fossils suggests multicellular algal affinity and adds to a Burgess Shale-type (BST) taphonomic window in the Pre-Ediacaran biosphere.
Introduction
Macroalgae (seaweeds) are multicellular marine algae easily observed by the unaided eye, whose “thallus” is characterized by holdfasts for attachment, and by “laminae,” reproductive “sori,” gas bladders, and stipes (Xiao and Dong, 2006; Brodie and Lewis, 2007). They are considered macroscopic photosynthetic eukaryotes in the Precambrian biosphere (Xiao and Dong, 2006; Bykova et al., 2020). Gray to black shales in Ediacaran sedimentary rocks contain unusually well-preserved Burgess Shale-type preservation of carbonaceous compressions (macroalga) (Yuan et al., 1999; Xiao et al., 2002; Dornbos et al., 2016; Ye et al., 2019) and cast and mold preservation (Laflamme et al., 2013). Notable carbonaceous fossils are known from the Lantian, Doushantuo, and Liuchapo Formations in South China (Steiner, 1994; Xiao et al., 2002; Zhao et al., 2004; Yuan et al., 2011; Ye et al., 2019); the Perevalok Formation in the Central Urals (Grazhdankin et al., 2007; Marusin et al., 2011); the Khatyspyt Formation in Siberia (Grazhdankin et al., 2008); the Deep Spring Formation in the United States (Rowland and Rodriguez, 2014); and the Zuun-Arts Formation in Western Mongolia (Dornbos et al., 2016). Most of these assemblages are characterized by low taxonomic diversity and are dominated by multicellular benthic algae (Xiao et al., 2002), and some are identified as putative macro-metazoans (Wan et al., 2016; Yuan et al., 2016; Ye et al., 2019). These records have gradually contributed to our comprehensive understanding of the evolution, emergence, and diversification of macroalgae before the Cambrian explosion. Globally, they are considered ecologically important primary producers in marine benthic ecosystems and have played an important role in the evolution of eukaryotic organisms in the late Proterozoic biosphere (Ediacaran) (Brocks et al., 2017; Isson et al., 2018).
In recent years, considerable progress has been made in understanding the evolution and diversification of Proterozoic macroalgal fossils preserved on the surface of siliciclastic sediments. However, their evolutionary timing and recognition in deep time is crucial due to preservational factors (taphonomy) in both palaeontological data and molecular clock analyses (Xiao and Dong, 2006; Parfrey et al., 2011). Geographically, the Proterozoic macroalgal fossils (Chuaria-Tawuia assemblage) and multicellular metaphyte fossils have been extensively documented from the sedimentary successions of Africa (Amard, 1992), Antarctica (Cooper et al., 1982), Australia (Haines, 1998), Canada (Hofmann and Rainbird, 1994), China (Xiao et al., 2002; Tang et al., 2017; Ye et al., 2019; Tang et al., 2020; Tang et al., 2021), Namibia (Leonov et al., 2009), Russia (Gnilovskaya, 1971; Gnilovskaya et al., 2000), Spain (Amard, 1992; Jensen et al., 2007), Spitsbergen (Butterfield et al., 1994), Siberia (Grazhdankin et al., 2008), Ukraine (Gnilovskaya et al., 1988; Steiner, 1997), Ural (Marusin et al., 2011), United States (Ford and Breed, 1973; Rowland and Rodriguez, 2014), Yakutia (Vidal et al., 1993), and India (Sharma et al., 1991, 2009; Sharma, 2006; Sharma and Shukla, 2009a, 2009b; Singh et al., 2009; Sharma and Singh, 2019). These carbonaceous megascopic fossils have drawn the attention of various researchers seeking to understand their affinity, taxonomic position (both debatable) (Hofmann, 1992; Xiao and Dong, 2006; Lamb et al., 2007; Wan et al., 2016; Sharma and Singh, 2019; Ye et al., 2019) and possible natural experimentation in response to the advent of oxygen in the palaeoenvironment (Lenton et al., 2014; Lyons et al., 2014; Tang et al., 2017; Muscente et al., 2019; Tang et al., 2020; Zhang F. et al., 2021; Maloney et al., 2021).
Such fossils are also recorded from the Palaeoproterozoic to Mesoproterozoic sediments of the United States: the ca. 1.87 Ga Negaunee Iron-Formation (Tyler et al., 1957; Han and Runnegar, 1992) and the ca. 1.4 Ga Greyson Formation (Walter et al., 1990); China, including the ca. 1.65 Ga Changzhougou Formation (Zhu et al., 2000), the ca. 1.63 Ga Tuanshanzi Formation (Zhu and Chen, 1995; Yan and Liu, 1997; Qu et al., 2018), the ca. 1.56 Ga Gaoyuzhuang Formation (Du et al., 1986; Zhu et al., 2016), the ca. 1.4 Ga Xiamling Formation (Zhang F. et al., 2021) and the ca. 1.0 Ga Nanfen Formation (Tang et al., 2020); and India, including the ca. 1.63 Ga Olive Shale (Rai and Singh, 2006; Sharma, 2006), and the ca. ∼1.5 Ga Saraipali Shale (Babu and Singh, 2011; Babu and Singh, 2013). Although the Palaeo- and Mesoproterozoic fossil record of macroalgae (eukaryotes) is infrequent, they play an important role in the evolution of multicellular life in the Precambrian Era. The cellular structures and phylogenetic affinities of these fossil remains are uncertain, due to the preservation of limited morphological details (Kumar, 2001; Bykova et al., 2020), modes of preservation (Lamb et al., 2007), broad taphonomic variability (Samuelsson and Butterfield, 2001), and the current paucity of biochemical information (Arouri et al., 1999; Dutta et al., 2006; Sharma et al., 2009). A few demonstrate cyanobacterial affinity (Sharma and Shukla, 2009b). Recently, a coenocytic eukaryotic and macroalgal affinity has been assigned to tomaculate fossil Tawuia based on recalcitrant cell walls, asexual reproduction through asymmetric constrictions, and possible abscission structures (Tang et al., 2021). Their evolutionary patterns and ecological influences have been mostly disregarded (Bykova et al., 2020). To improve our understanding of macroalgal evolution in the Mesoproterozoic biosphere, the present study systematically describes and discusses the biogenicity, antiquity, and affinity of new material from carbonaceous megascopic fossils found in the rocks of the Singhora Group, Chhattisgarh Supergroup, India.
Geology and Age of the Singhora Group
In the peninsular region of central India, the Palaeoproterozoic—Mesoproterozoic succession of unmetamorphosed, less-deformed sedimentary succession of the Chhattisgarh Supergroup lies over the crystalline rocks of the Bastar Craton (Mukherjee et al., 2014; Chakraborty et al., 2020). Lithostratigraphically, it is divided into four groups, viz., the Singhora, the Chandarpur, the Raipur, and the Kharsia, in ascending order (Das et al., 1992; Mukherjee et al., 2014; Chakraborty et al., 2015). In the ∼2,300 m thick litho package of the Chhattisgarh Supergroup, the Singhora Group is designated as the lowermost stratigraphic unit, containing mixed siliciclastic and carbonate litho-associations (Das et al., 2003; Mukherjee et al., 2014). The Singhora Group of rocks is located in the southeastern and western parts of the Chhattisgarh Basin (Figure 1.1). It is well-exposed in the Singhora Township, situated in parts of the Mahasamund District in Chhattisgarh State and the Barapahar area of the Bargarh District, Odisha (Das et al., 2003). With ∼400 m thickness, the litho package of the Singhora Group (200 km2 in aerial extent) is further subdivided into four Formations, viz., the Rehatikhol, the Saraipali, the Bhalukona, and the Chhuipali, in order of superposition (Figure 1.2) (Table 1).
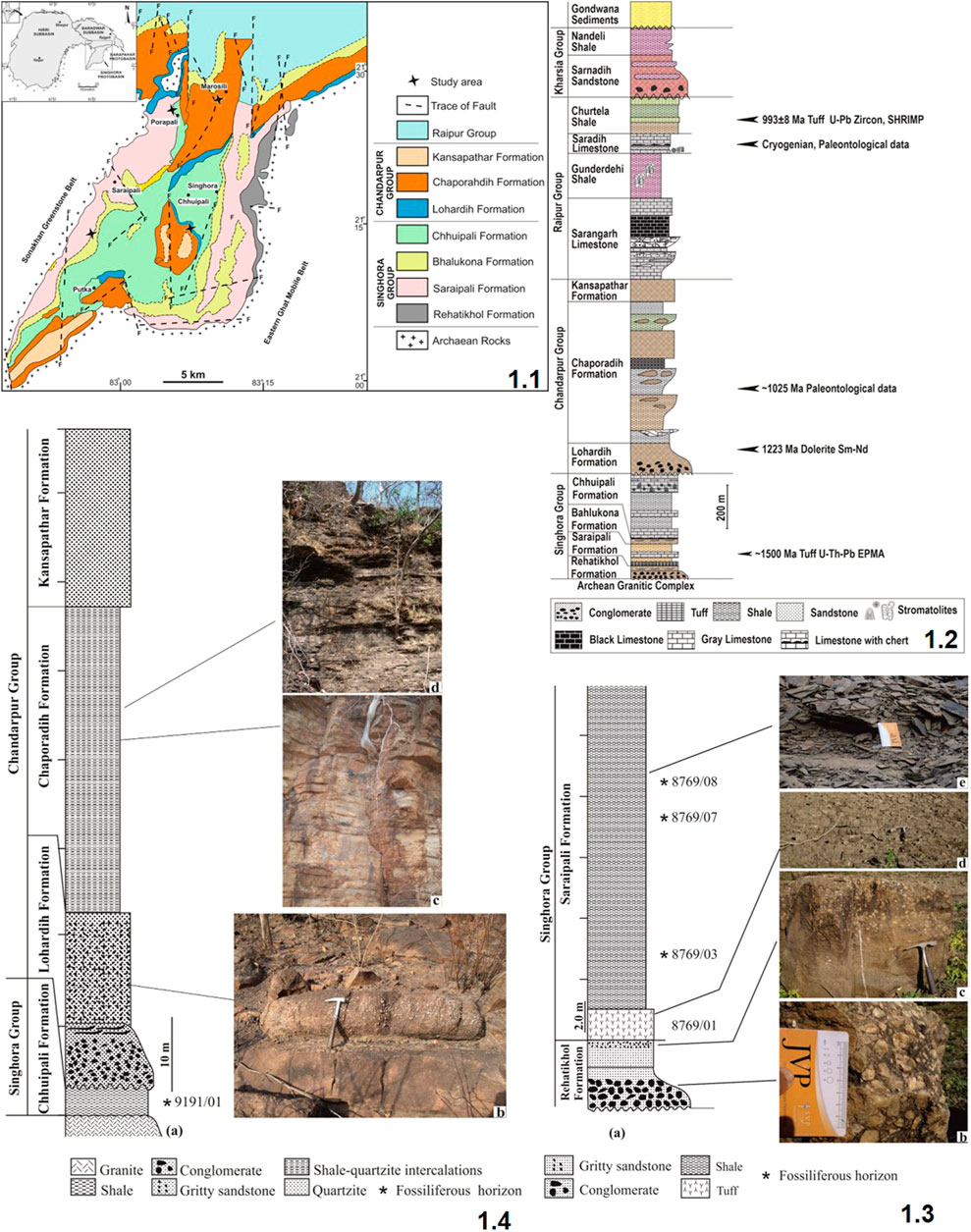
FIGURE 1. Generalized geological map of the Baradwar Subbasin in and around the Singhora and Sarangarh areas, Chhattisgarh (after Das et al., 2001); 1.2: Lithostratigraphic succession of the Chhattisgarh Supergroup in the Baradwar Subbasin (modified after Das et al., 1992; Patranabis deb and Chaudhury, 2008); 1.3: (A) Generalized lithology of the Singhora Group, Surangi River section; (B) Conglomerate of the Rehatikhol Formation; (C) Gritty sandstone of the Rehatikhol Formation; (D) Tuff/porcellanite of the Saraipali Formation; (E) Fossil bearing carbonaceous shale of the Saraipali Formation. Card and geological hammer used for scale; 1.4: (A) Generalized lithology of Bendla Dongar section; (B) Gritty sandstone of the Lohardih Formation; (C,D) Shale-quartzite intercalation heterolithic of the Chaporadih Formation. Hammer is used for scale.
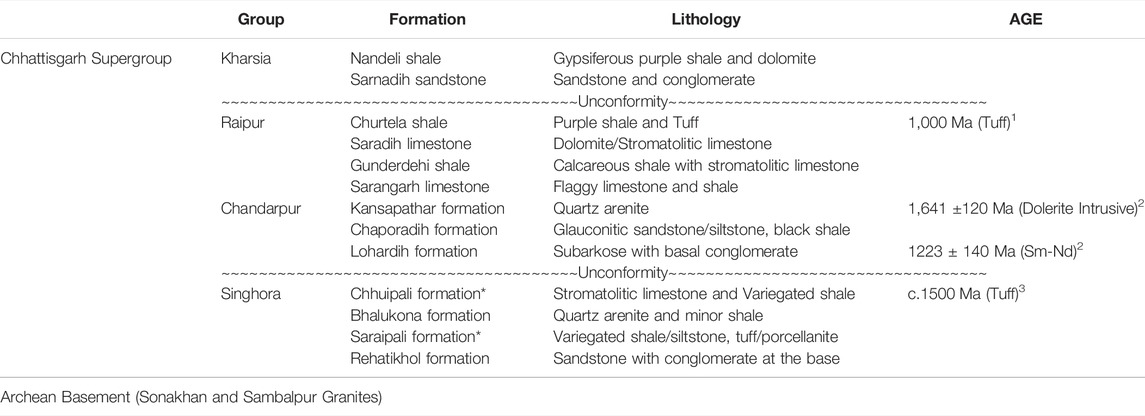
TABLE 1. Generalized lithostratigraphic succession of the Chhattisgarh Supergroup (Das et al., 1992; Patranabis-Deb and Chaudhuri, 2008; Mukherjee and Ray, 2010) Age data source: 1. Bickford et al., (2011), 2. Pandey et al., (2012), 3. Das et al., (2009). * Fossiliferous unit.
With ∼20 m maximum thickness, the Rehatikhol Formation of the Singhora Group marks the initiation of sedimentation in the Baradwar Subbasin, resting over the granitic/gneissic basement of Bastar Craton. It is represented by the repetitive sequence of conglomerate and arkose with minor intercalations of siltstone and shale. More than ∼60 m thick, the Saraipali Formation is the second unit of the Singhora Group (Mukherjee et al., 2014). The finely-laminated shale, siltstone, volcaniclastic tuffs, and limestone-dominated Saraipali Formation overlie the basal Rehatikhol Formation. The arenaceous sediment dominated Bhalukona Formation (∼20 m) overlies the Saraipali Formation. It is constituted of quartz arenite with minor shale and siltstone intercalations in its basal part representing a coarsening upward sequence. The shale and carbonate-dominated Chhuipali Formation is the topmost unit of the Singhora Group, resting over the Bhalukona Formation. It is about ∼300 m thick in the central part of the basin. Rocks of this unit are characterized by variegated shale with intercalations of siltstone, minor sandstone, chert, and stromatolitic limestone/dolomite. It is both bedded and stromatolitic and occurs as pockets as well as lenses. The limestone is light gray to dark gray and is composed of sparry calcite forming a mosaic with patches of micritic carbonate. In places, it is associated with ferruginous arenite. Chertified limestone is further overlain by low-dipping variegated shale which dominates without (>100 m thick), which is laminated and highly friable; 5–20 m thick stromatolitic limestone and dolomite comprise the upper part of this formation and occur as pockets and lenses. The limestone is light gray, impure, and bedded, characterized by the presence of thin chert partings. Stromatolite sections are conspicuous on the horizontal surface parallel to the bedding. Available studies suggest alluvial fan, braid plain, shelf (storm infested), shallow marine, and shelf depositional conditions for the Singhora Group of rocks in stratigraphic order (Das et al., 1992; Chakraborty et al., 2010; Chakraborty et al., 2012; Chakraborty et al., 2015). Carbonaceous fossils are documented from the Saraipali and Chhuipali Formations of the Singhora Group.
The age of the Singhora Group of rocks is constrained by U-Th-Pb SHRIMP EPMA dating of monazite and zircon in the Khariar and Shingora tuffs, which provide a minimum age around ∼1,500 Ma (Das et al., 2009; Bickford et al., 2011; Chakraborty and Barkat, 2020). About ∼80 Ma of depositional timeframe has been suggested after Sm-Nd isochron dating (∼1,420 Ma) of dibasic intrusives piercing the Saraipali Formation. SHRIMP II and LA-ICP-MS zircon dating from the different stratigraphic units of the Singhora Group yields the youngest age population, between c. 1,619 Ma and c. 2,543 Ma, implying sedimentation initiation of >1,600 Ma (Das et al., 2017). However, Babu and Singh (2011) have suggested the latest Palaeoproterozoic (∼1750 Ma) age based on the carbonaceous remains of eukaryotic affinity from the Saraipali Formation of the Singhora Group. The occurrence of acanthomorphic acritarch Tappania plana in the Saraipali Formation also suggest an early Mesoproterozoic age for the Singhora Group of rocks (Singh et al., 2019).
Material and Methods
The fossils described here were collected from the siliciclastic unit of the Saraipali Formation and the Chhuipali Formation of the Singhora Group, Mahasamund District, Chhattisgarh State, India.
The Surangi River section (Latitude: N21°14′30.53″; Longitude: E82°58′05.25″) is situated 25 km southwest of Saraipali Township in the Mahasamund District. The sedimentary succession of this section is characterized by up to 2.0 m thick low-dipping dark grey to black fine-grained porcellanite/tuffs followed by ∼ 20 m thick black and gray shale with minor sandy intercalations at places (Das et al., 1992; Mukherjee et al., 2014). Shale with a gentle dip is the dominant lithology of this formation. Most shale horizons from this unit contain Saraipali preserved as carbonaceous compressions within and on the surface. Specimens described here come from the lower part of this section, as illustrated in Figure 1.3.
The Bendla Dongar section (Latitude: N21°26′3.45″; Longitude: E83°05′5.44″) is situated 18.5 km north of Saraipali Township near Porapali Village in the Mahasamund District. This hill is about 150 m high (Figure 1.4). The sedimentary succession in this section is characterized by the low-dipping khaki-coloured fine-grained shale of the Chhuipali Formation in the Singhora Group. The sequence is further characterized by the Chandarpur Group rocks, represented by the bedded quartzite with glauconite and thin arkosic conglomerate of the Lohardih Formation at the base (Figure 1.4B), shale-mudstone—sandstone lower heterolithics more than 20 m thick (Figures 1.4C, D) and about 10–15 m thick brown-coloured ferruginous sandstone at the top, which distinctly belongs to the Chaporadih Formation. Khaki shale of this unit contains carbonaceous remains preserved as compressions/impressions on the bedding plane (Figure 1.4). More than 200 carbonaceous films (compressions/impressions) hosted in shale slabs were collected for the present study from the Saraipali Shale and Chhuipali Shale. All illustrated specimens, photomicrographs, and associated samples have been reposited in the Museum at Birbal Sahni Institute of Palaeosciences, Lucknow. These can be retrieved vide statement no. BSIP 1584.
Fossil Measurements and Analytical Microscopy
All fossiliferous slabs were thoroughly cleaned and prepared before morphological studies. Megascopic carbonaceous fossils were analyzed and morphologically identified on an OLYMPUS SZ61 Stereoscopic microscope and were photographed and measured on a software-supported OLYMPUS SC50 digital camera. Digital Photographic images were further processed on Olympus Cell Sense Standard software version 2.1 for detailed linear measurements. Approximately 20 samples of carbonaceous films were analyzed with Energy Dispersive X-ray Spectroscopy (EDX) to make elemental mapping and Laser Raman Spectroscopy (LRS) analyzing the macroalgae’s chemical characterization.
Laser Raman Spectroscopy (LRS)
Selected specimens were analyzed for chemical characterization using a RENISHAW inVia Reflex Raman Microscope at BSIP, which permits the acquisition of both point spectra and Raman images, which display the two-dimensional spatial distribution of molecular-structural components of the specimens and their associated mineral matrix. The optics of the Raman system are based on a Leica DM-2700M REN RL/TL microscope. The instrument was calibrated against the Raman signal for Si obtained from an internal silicon wafer reference at 521 cm−1. Spectral acquisition was performed over an extended range from ∼200 to ∼2000 cm−1. On each point of the specimen, Raman spectra were collected by using 0.5% laser power with 20 s of exposure time. The samples were exposed to a Coherent Infrared (IR) laser, which provided excitation at a 785-nm wavelength at a power of ∼1–5 mW over 1.5 μm, to obtain a good signal-to-noise ratio, avoid radiation damage, and minimize laser-induced heating (Schopf et al., 2006). In these conditions, the analysis time was a few minutes (an accumulated time of 30 s and 10 scans). A ×50 objective lens (with an extended working distance of 10.6 mm and a numerical aperture of 0.5) was used to obtain a horizontal spectral resolution of 1.5 μm.
Energy Dispersive X-ray Spectroscopy (EDX)
To determine the elemental constitution of carbonaceous fossils, selected specimens were analyzed under the JEOL JSM-7610F Field Emission Scanning Electron Microscope with Energy Dispersive X-ray Spectroscopy (FESEM-EDX) instrument at 7.05 keV with a platinum coating. The instrument, equipped with Gentle Beam (GB) mode, provides high-resolution images even at a low accelerating voltage from 100 V to 3.9 kV without damaging the specimen surface. The EDX documents the quantitative and qualitative elemental analysis of fossil materials, such as C, O, P, S, Si, Fe, Al, O, K, and many others present in Mendeleev’s Periodic Table.
Results
Systematic Palaeontology
Systematic descriptions, interpretations, and affinities of identified macrofossils of carbonaceous remains are typically based on gross morphological characteristics, as in most treatment of fossil taxa. Distinct morphological characters are an essential tool to establish the taxonomy of any fossils at the genus and species level. Morphologically, they vary in size (from millimeters to a few centimeters), orientation, their degree of twisting/folding, and, in general, appear to be in situ remains. The carbonaceous fossils are fan-shaped, palmate, elongated, leaf-like, ribbon-like, algal thalli with/without holdfast at the base, with rounded and angular distal ends, isolated or dichotomously branched long tubular filaments with a cylindrical axis, along with multicellular reproductive structures (Supplementary Figures S1, S2). New taxa are described following the rules of the International Code of Nomenclatures established for Algae, Fungi, and Plants (Shenzhen Code 2018). The taxonomic details of the identified fossils have been provided at the genus and species level based on characteristics and descriptions proposed by Xiao et al. (2002) and Ye et al. (2019).
Genus: Palaeoscytosiphon n. gen.
Type species: Palaeoscytosiphon shuklaii n. sp.
Etymology: With reference to the Scytosiphon-a modern phaeophyte.
Diagnosis: It is a branched and compressed thallus comprised of long multiple ribbons, with a holdfast at the base. The holdfast is occasionally rhizoidal (root-like). Ribbons are long and gradually taper to the distal end. Tapering angles are 2–3°. Ribbons are 1.0–6.0 mm long and 0.1–0.3 mm wide. Edges of the ribbons are parallel and sharp; each thallus contains 4–12 stalks arising from the holdfast. Filaments are often branched, more or less symmetrically dichotomous in a few specimens. Ribbons in some of the specimens are curved and twisted, perhaps due to taphonomic processes (Figures 3.1, 3.3).
Comparison: The morphological characteristics of described carbonaceous fossils can be compared to the brown alga Scytosiphon lomentaria—an irregularly arranged weed with a tubular, hollow, unbranched thallus growing on a rhizoid-like holdfast (Figure 4). It is a member of the order Scytosiphonales of the algal group Phaeophyceae.
Palaeoscytosiphonella shuklaii n. sp.
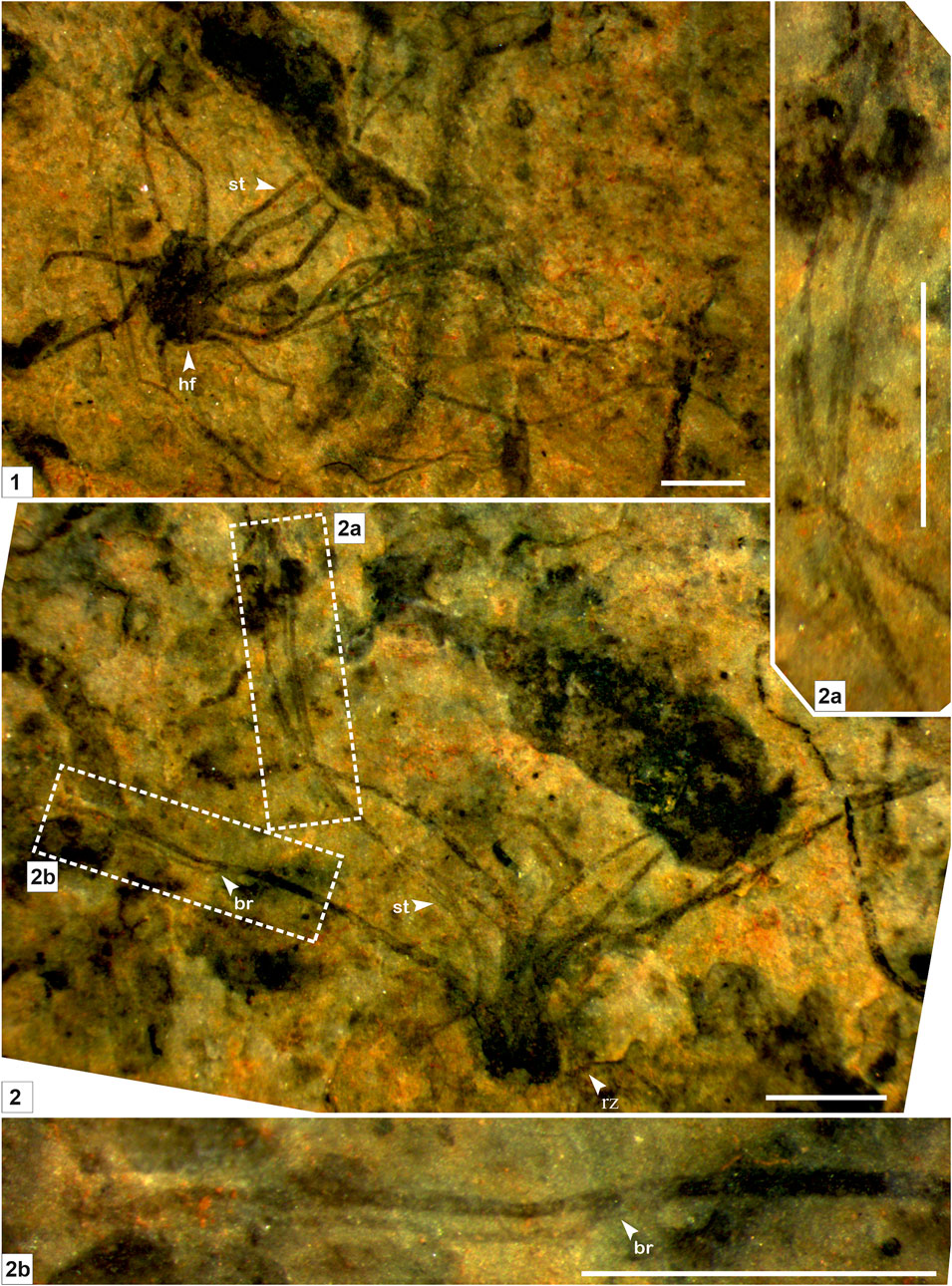
FIGURE 2. Macroscopic fossil Palaeoscytosiphon shuklaii n. gen., n. sp. from the Saraipali Formation. (Arrow indicates the prominent features: st. stalk; br. branching; hf. holdfast; rz. rhizoid.) (1–2). 1. Type specimen comprised of compressed thallus with long multiple tubular stalks attached with a discoidal holdfast at the base; 2. Longitudinal preservation of Palaeoscytosiphon shuklaii; 2.a, 2.b. Enlarged view of Panel 2 showing secondary branching in stalks. 1. Specimen no. BSIP 41897; 2. Specimen no. BSIP 42022. Scale bar is 2 mm for each specimen.
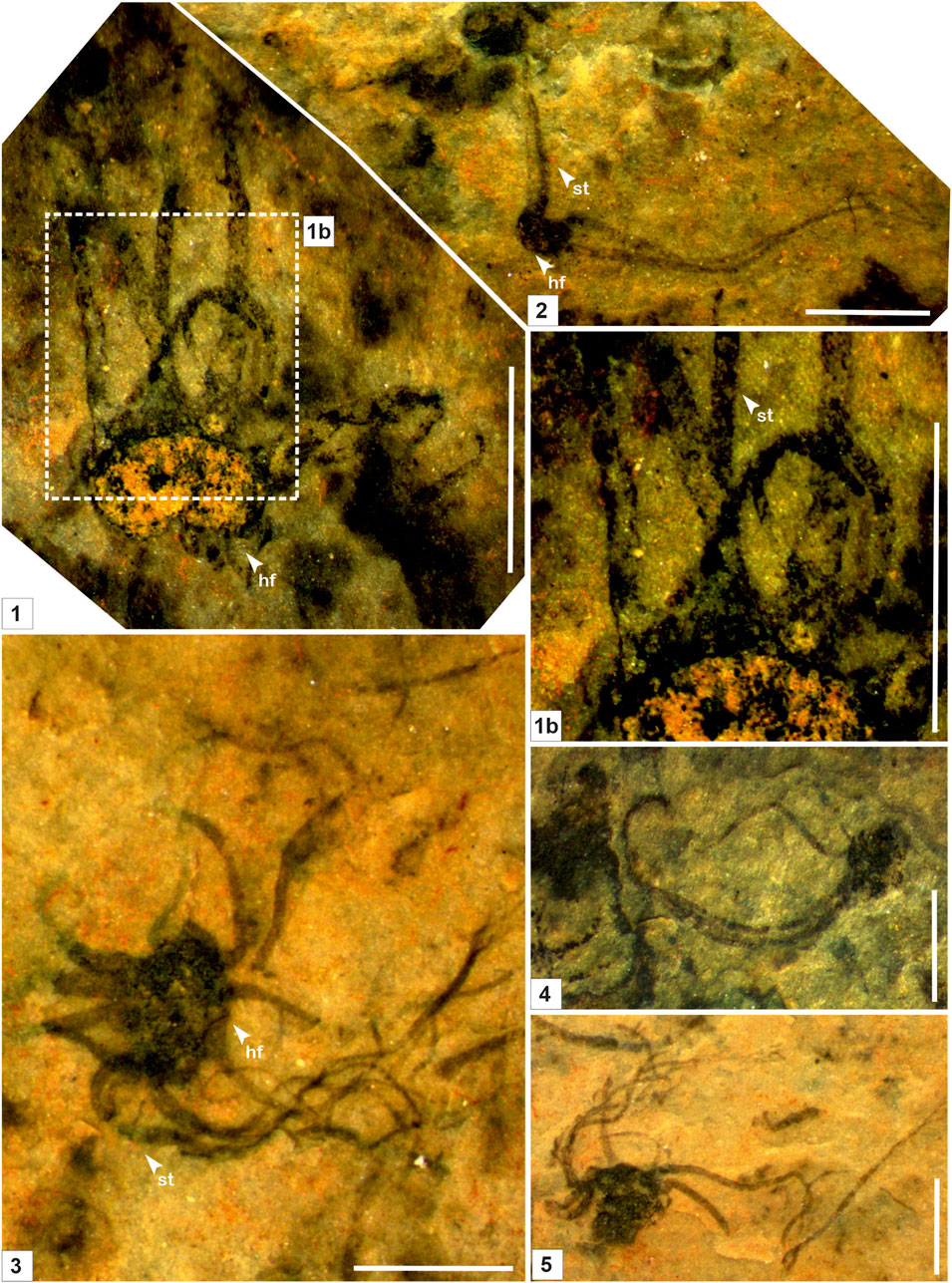
FIGURE 3. Macroscopic fossil Palaeoscytosiphon shuklaii n. gen., n. sp. from the Saraipali Formation (arrow indicates the prominent features: st. stalk; br. branching; hf. holdfast) (1–5). 1b. Enlarged view of connection between stalk and holdfast. 1, 2. Specimen no. BSIP 42022; 3. Specimen no. BSIP 42023; 4. Specimen no. BSIP 42021; 5. Specimen no. BSIP 42024. Scale bar is 2.0 mm for each specimen.
Stratigraphic position: The Saraipali Formation, the Singhora Group.
Derivation of Name: In honour of the Late Dr. Manoj Shukla, a Precambrian palaeobiologist from India.
Type Specimen: Figure 2.1, Specimen No. 8769/82, BSIP Museum.
Material: 10 well-preserved specimens from the Surangi River section, southwest of Saraipali Township, Mahasamund District, Chhattisgarh, India.
Description: As for genus/type species.
Remarks: Stalks of modern Scytosiphons are up to 400 mm long and 3–10 mm wide. Generally, it grows on stones and hard mud in shallow rock pools (up to 17 m deep), the mid-to-low tide region of exposed shores, and in tide pools (Figure 4). Specimens assigned to Palaeoscytosiphon shuklaii are well-preserved, constituting multiple carbonaceous ribbons (Figures 2.1, 2.2; Figure 3.1). A rhizoid-like holdfast (Figure 2.2, Figure 3.3) and dichotomous branching in ribbons (Figures 2.2A, 2.2B) can be distinctly seen in specimens. Carbonaceous ribbons in some specimens are broken and ill-preserved (Figures 3.2–3.5). The mode of preservation of P. shuklaii is illustrated in Supplementary Figure S2. Additionally, the thread-like elongated filamentous compression fossil form Proterotaenia montana, known from the Palaeoproterozoic (∼1,637 Ma) Tuanshanzi Formation of China, has also been compared with the tubular filaments of the extant brown algae Scytosiphon lomentaria (Yan and Liu, 1997). Singh et al. (2009) have reported specimens such as Palaeochorda vindhyansis from the Neoproterozoic Bhander Group of the Vindhyan Supergroup, India. Palaeochorda vindhyansis is characterized by smooth, hollow, unbranched long tubular cord-like ribbons attached by a holdfast-like structure similar to Chorda in morphology. In modern morphology, the Chordafilum is characterized by hollow, unbranched, long cord-like ribbons attached by a small discoid holdfast. However, in Palaeochorda vindhyansis, the discoidal holdfast is absent. In this case, P. vindhyansis can be considered a junior synonym of Palaeoscytosiphon shuklaii. Conversely, the specimens of P. shuklaii have a distinct rhizoidal holdfast (Figure 2.2).
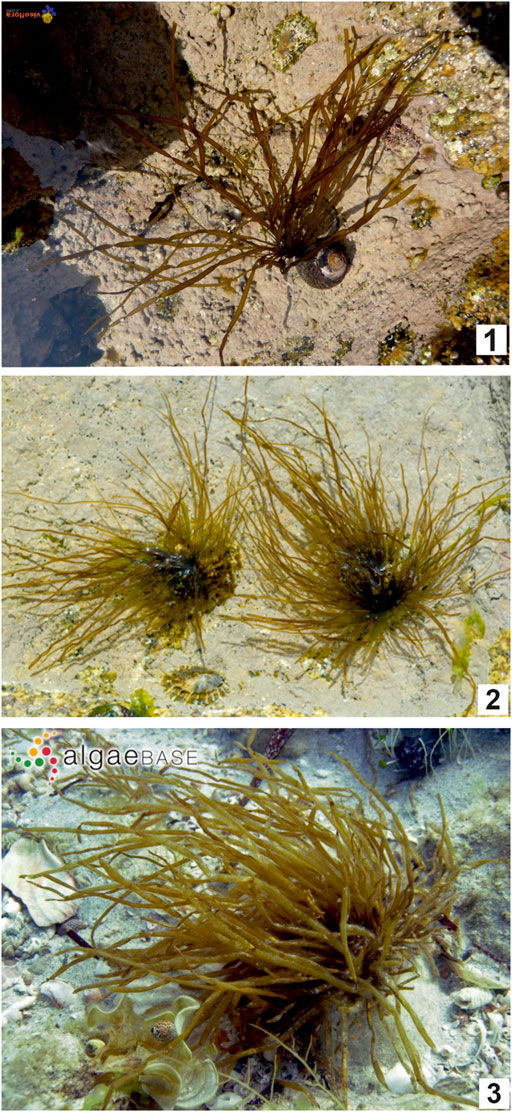
FIGURE 4. Photographs of modern brown algae (Phaeophyta) Scytosiphon lomentaria (1–4). Source – 1. https://www.visoflora.com/photos-nature/photo-scytosiphon-lomentaria-1.html; 2. http://www.seaweed.ie/descriptions/Scytosiphon_lomentaria.php; 3. https://alchetron.com/Scytosiphon-lomentaria.
Genus: Baculiphyca. Yuan, Li, and Chen, 1995, emend. Xiao et al. 2002.
Type species: Baculiphyca taeniata. Yuan, Li, and Chen, 1995, emend. Xiao et al. 2002.
Baculiphyca taeniata. Yuan, Li, and Chen, 1995, emend. Xiao et al. 2002.
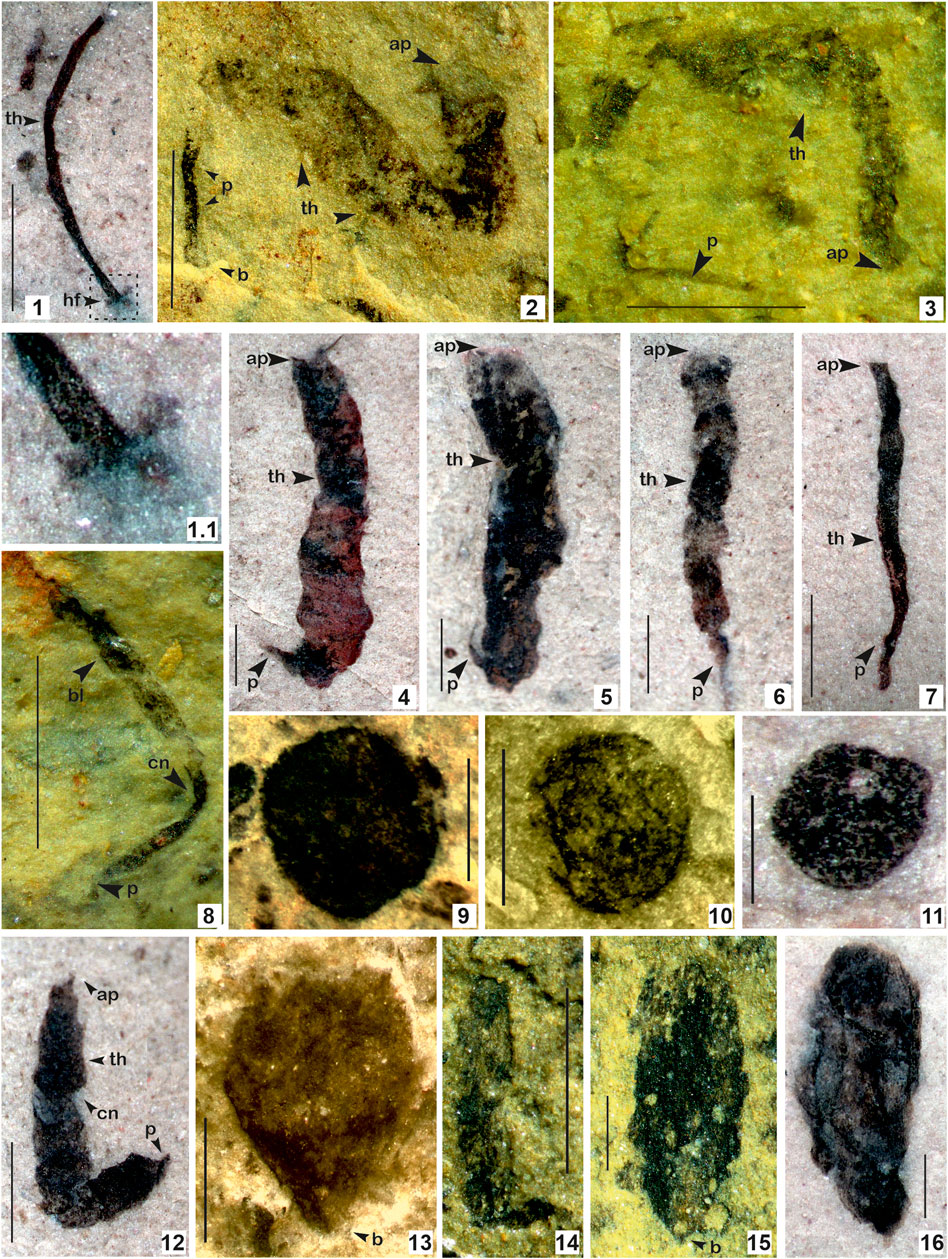
FIGURE 5. 1. Baculiphyca taeniata Yuan et al., 1995, emend. Xiao et al., 2002; 1.1. Enlarged view of (B) taeniata holdfast; 2–8, 12. Changchengia stipitata Yan, 1997 in Yan and Liu, 1997 with distinct parastem ate base; 9–11. Chuaria circularis Walcott, 1899; Vidal and Ford, 1985; 13–15. Eopalmaria pristina Yan, 1995. (Arrow indicates the prominent features: ap. apex; b. base; bd. bead; th. thallus; cn. constrictions, p. parastem; hf. holdfast). 1. Specimen no. BSIP 418983; 2. Specimen no. BSIP 39782; 3. Specimen no. BSIP 39779; 4. Specimen no. BSIP 42026; 5. Specimen no. BSIP 41899. 6. Specimen no. BSIP 42038; 7. Specimen no. BSIP 42033a; 8. Specimen no. BSIP 39776; 9. Specimen no. BSIP 42022; 10. Specimen no. BSIP 41897; 11. Specimen no. BSIP 42042; 12. Specimen no. BSIP 42036; 13. Specimen no. BSIP 42040; 14. Specimen no. BSIP 39774; 15. Specimen no. BSIP 39778; 16. Specimen no. BSIP 42030. Specimens 1, 4–7, 11, 12, 16 from the Bendla Dongar section and 2, 3, 8-10, 13–15 from the Surangi River section. Scale bar is 1.0 mm for each specimen.
Stratigraphic position: Mesoproterozoic Chhuipali Formation, the Singhora Group.
Material: One specimen from the Bendla Dongar section, north of Saraipali Township, Mahasamund District, Chhattisgarh, India.
Description: Elongate, unbranched clavate algal thallus, attached to the substrate by a basal rhizoidal holdfast. The holdfast typically bears fine (ca. 0.1–0.3 mm in diameter) filamentous rhizoids at its base. The thallus expands gradually to the distal end at an apical divergence angle of 2°–6°. The upper portion is more or less blade-like. The terminus is straight. Elongated thalli are 2.5 mm long and 0.2 mm thick.
Remarks: Yuan et al. (1995) instituted the genus Baculiphyca with its type species Baculiphyca taeniata assigned to carbonaceous fossils composed of a clavate, bent and folded thallus attached with a rhizoidal holdfast. Later, in a taxonomic assessment, Xiao et al (2002) emended the diagnosis of Baculiphyca based on both rhizoidal and globose holdfasts. Subsequently, based on complex morphology, Xiao et al. (2002) have also suggested possible eukaryotic algal affinity for the genus Baculiphyca. Recently, Ye et al. (2019) established a new species, Baculiphyca brevistipitata, from the upper Ediacaran Doushantuo Formation, China. A specimen from the Chhuipali Formation is characterized by a dark black elongated stipe with a globose holdfast, showing a close resemblance to Miaohe specimens (Xiao et al., 2002; Ye et al., 2019), but is comparatively smaller (Figure 5.1). The presence of a holdfast in Baculiphyca proves their benthic habit as microorganisms. Holdfasts may play an important role, anchoring them to strengthen Baculiphyca in mud and making their thallus/stipe flexible for movement according to currents.
In the fossil records, Baculiphyca is widely known in the Ediacaran carbonaceous assemblages, specifically in China and India (Singh et al., 2009; Ye et al., 2019). This is the first report of Baculiphyca from the Mesoproterozoic succession and suggests their antiquity is possibly ∼400 Ma deeper in the Proterozoic biosphere.
Genus: Changchengia Yan. Yan and Liu, 1997.
Type species: Changechengia stipitata. Yan and Liu, 1997.
Changechengia stipitata Yan. Yan and Liu, 1997.
Stratigraphic position: The Saraipali and Chhuipali Formations, the Singhora Group.
Material: Twenty (20) specimens from the Surangi River section, southwest of Saraipali Township; and fifteen (15) specimens from the Bendla Dongar section, north of Saraipali Township, Mahasamund District, Chhattisgarh, India.
Description: Unbranched, lanceolate, broad ribbon-like thalli, widest at the middle, wider toward the apex, narrowing toward the base. Algal thalli are folded and twisted with depressed margins, smooth and prominent. A distinct differential type parastem-like structure (Figures 5.2–5.8) is present at the base of the thalli. The measured thallus is 3.0–8.0 mm in length, and up to 1.0 mm wide. The parastem is up to 0.5 mm long. In one specimen, the parastem-like structure is attached to a disc-like base (Figure 5.8).
Remarks: Specimens described here are morphologically similar to, but relatively smaller than, the C. stipitata known from the Tuanshanzi Formation (∼1,637 Ma) of Yanshan Basin in Jinxian, Hubei, China (Yan and Liu, 1997). Specimens with similar morphologies have been documented from the Palaeoproterozoic Olive Shale of the Vindhyan Supergroup, India (Rai and Singh, 2006; Sharma, 2006). Based on the preservation mode of C. stipitata, Sharma (2006) has suggested their growth in a lagoonal environment.
A parastem is the main morphological characteristic feature of Changchengia stipitata, occurring at the base of the thalli. Such structures are well-preserved in both Saraipali (Figures 5.2, 5.3, 5.8) and Chhuipali specimens (Figures 5.4–5.7). The presence of a disc-like structure at the parastem base suggests C. stipitata belonged to a benthic habitat. Our interpretation for the parastem of Changchengia stipitata is that it helped to anchor the algal thalli on the bottom, protecting it from current movement in the water.
Genus: Chuaria. Walcott, 1899; Vidal and Ford, 1985.
Type species: Chuaria circularis. Walcott, 1899; Vidal and Ford, 1985.
Chuaria circularis. Walcott, 1899; Vidal and Ford, 1985.
Stratigraphic position: The Saraipali and Chhuipali Formations, the Singhora Group.
Material: Twenty (20) specimens from the Surangi River section, southwest of Saraipali Township; and fifteen (15) specimens from the Bendla Dongar section, north of Saraipali Township, Mahasamund District, Chhattisgarh, India.
Description: Isolated, flattened, smooth, circular to subcircular black carbonaceous compressions or impressions. Two-dimensionally preserved discs, ranging from 0.5 to 0.8 mm in diameter.
Remarks: Specimens of Chuaria circularis recorded from the Chhattisgarh Supergroup are morphologically similar in type and material to those from China, Canada, Russia, and other localities, including India. The specimens consist of impressions and compressions of carbonized material, deposited and compacted between the bedding planes or parallel to the laminations of rock. It is one of the few globally-distributed carbonaceous specimens extensively reported from Palaeoproterozoic to Neoproterozoic sediments (Hofmann, 1994; Dutta et al., 2006; Sharma et al., 2009). Carbonaceous discs without wrinkles, documented by the Uinta Mountain Group, were also designated as Chuaria circularis (Hofmann, 1977). In later reports, carbonaceous discs with or without wrinkles were globally demonstrated as Chuaria circularis (Yuan et al., 2001; Kumar and Srivastava, 2003; Sharma et al., 2009; Ye et al., 2019). It has also been interpreted as a colonial cyanobacterium, based on its putative association with Nostoc-like filaments (Sun, 1987; Steiner, 1997); or as a multicellular eukaryote because of its excystment opening and a potentially complex life cycle (Kumar, 2001; Sharma et al., 2009; Wang et al., 2011; Tang et al., 2017). Specimens with medial structures are demonstrated as polyphyletic in origin (Butterfield et al., 1994; Sharma et al., 2009). Sharma et al. (2009) proposed a hybrid model for the Chuaria-Tawuia association and suggested that the biogeopolymer of Chuaria is similar to the algaenan macro-molecules of many algae species. Recent analysis using backscattered-electron scanning electron microscopy (BSE-SEM) revealed that Chuaria may have had a multicellular vegetative stage in its life cycle (Tang et al., 2017).
Genus: Eopalmaria. Yan, 1995.
Type species: Eopalmaria pristina. Yan, 1995.
Eopalmaria pristina. Yan, 1995.
Stratigraphic position: The Saraipali and Chhuipali Formations, the Singhora Group.
Material: Eight (08) specimens from the Surangi River section, southwest of Saraipali Township, and five (05) specimens from the Bendla Dongar section, north of Saraipali Township, Mahasamund District, Chhattisgarh, India.
Description: Unbranched elongate, flat, sheet-like algal thalli, with a wide and uneven apex, foliate, angular to subangular at the base. The measured specimens range between 0.5–6.0 mm long and 0.5–1.50 mm wide. Parastem-like stipes are absent at its base.
Remarks: Eopalmaria pristina was originally described as a palmate-shaped carbonaceous compression from the ca. 1,637 Ma Tuanshanzi Formation of the Changcheng Group of China (Yan, 1995). In size and morphological characteristics, the present specimens are similar to the biota known from the latest Palaeoproterozoic Olive Shale, Semri Group, Vindhyan Supergroup, India (Sharma, 2006). E. pristina is morphologically comparable to the modern algae Rhodomenia palmata of the algal group Rhodophyta, Spathoglossum of the Phaeophyta, and Monostroma among the Chlorophyta (Yan and Liu, 1997).
Genus: Eoholynia. Gnilovskaya, 1975.
Type species: Eoholynia mosquensis. Gnilovskaya, 1975.
Eoholynia corumbensis. Gaucher et al., 2003.
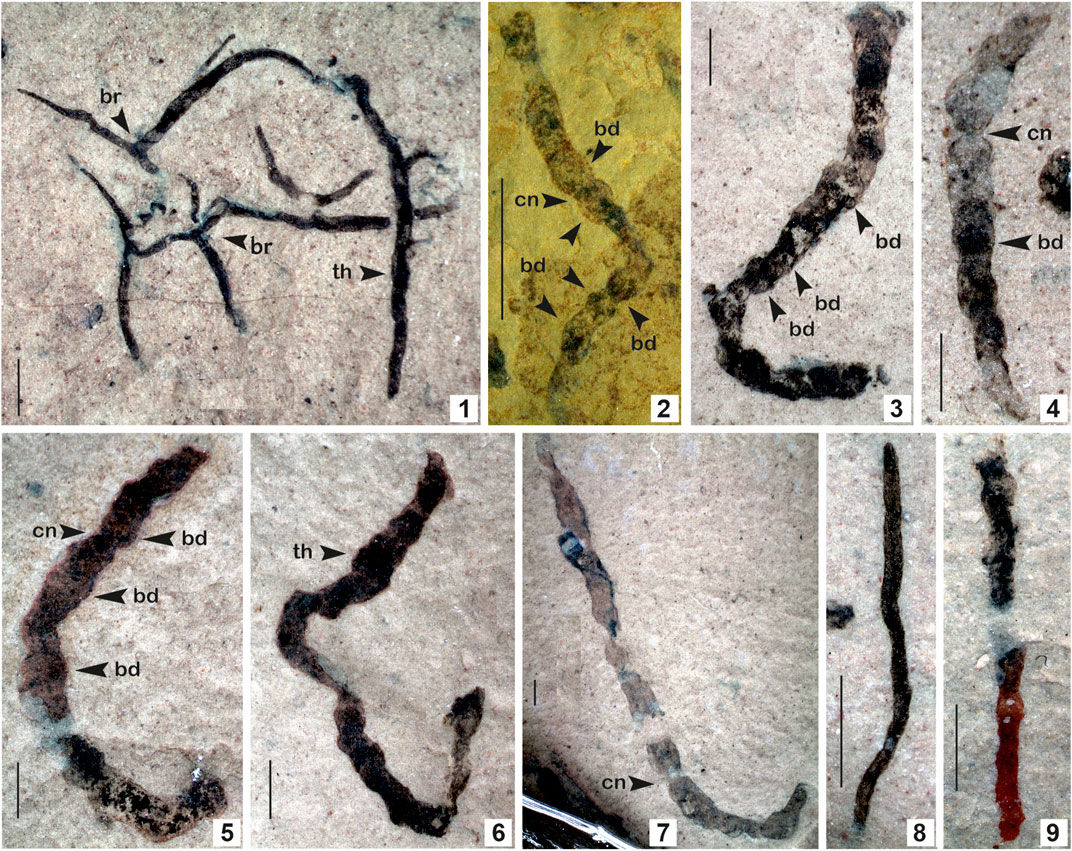
FIGURE 6. 1. Eoholynia corumbensis Gaucher et al., 2003; 2–7. Jiuqunaoella sergeevii n. sp.; 8–9. Synocylindra yunnanensis Chen and Erdtmann, 1991. (Arrow indicates the prominent features: br. branching; bd. bead; cn. constriction; th. thallus). 1. Specimen no. BSIP 42028; 2. Specimen no. BSIP 39782; 3. Specimen no. BSIP 42032; 4. Specimen no. BSIP 41898; 5. Specimen no. BSIP 42029; 6. Specimen no. BSIP 42035; 7. Specimen no. BSIP 42027; 8. Specimens no. BSIP 42037; 9. Specimens no. BSIP 42041. Specimens 1, 3-9 from the Bendla Dongar section and 2 from the Surangi River section. Scale bar is 1.0 mm for each specimen.
Stratigraphic position: The Chhuipali Formation, the Singhora Group.
Material: Single specimen from the Bendla Dongar section, north of Saraipali Township, Mahasamund District, Chhattisgarh, India.
Description: Dichotomously branched, non-septate, ribbon-like carbonaceous thallus, 05–06 branches in the thallus. Primary branches give rise to secondary branches. Secondary branches are sharply tapered at the end. The length of the complete specimen is 3–18 mm, approximately 0.1–0.4 mm wide.
Remarks: Similar carbonaceous compressions have been documented from the Neoproterozoic Guaicurus Formation of the Corumba Group, Brazil (Gaucher et al., 2003). Branches of E. corumbensis are wider than E. mosquensis (Gnilovskaya, 1979). Based on morphological characters, the biological affinity of Eoholynia is assigned to eukaryotic algae, probably of Phaeophyta or Rhodophyta (Hofmann, 1994; Burzin, 1996). E. corumbensis is considered probably benthic, as has been suggested for E. mosquensis (Gnilovskaya, 1975). Miaohephyton bifurcatum Steiner (1994)—a morphologically similar fossil to E. corumbensis—has also been placed among the brown algae (Phaeophyta) (Xiao et al., 1998). Subsequently, Eoholynia was placed among the vendotaenid algae (Gaucher et al., 2003).
Genus: Jiuqunaoella Chen. Chen and Xiao, 1991, emend. Xiao in Xiao et al., 2002.
Type species: Jiuqunaoella simplicis Chen. Chen and Xiao, 1991, emend. Xiao et al., 2002.
Jiuqunaoella sergeevii n. sp.
Type locality: Surangi River section, southwest of Saraipali Township, Mahasamund District, Chhattisgarh, India.
Type specimen: Figure 6.2, Specimen No. BSIP 39782.
Stratigraphic position: Carbonaceous shale of the Saraipali and Chhuipali Formations, the Singhora Group.
Material: 10 specimens: two (02) specimens from the Surangi River section, southwest of Saraipali Township; and eight (08) specimens from the Bendla Dongar section, north of Saraipali Township, Mahasamund District, Chhattisgarh, India.
Derivation of species name: In honour of the Late Prof. Vladimir N. Sergeev, Russian Academy of Sciences, Moscow, for his significant contribution to the field of Precambrian Palaeobiology.
Diagnosis: Ribbon-like carbonaceous compressions consist of a regular series of distinct chamber-like segments arranged in a beaded manner. Filaments are twisted and folded, having more or less parallel margins.
Description: Refer to the diagnosis. Ribbons are 4.0–11.0 mm long and 0.5–1.0 mm wide. The diameter of the chamber ranges from 0.5 to 1.0 mm.
Remarks: Chen (in Chen and Xiao, 1991) established the genus Jiuqunaoella with its type species Jiuqunaoella simplicis, from the Ediacaran Doushantuo Formation, Miaohe Village, in Hubei Province, China. In the absence of a type repository for the species, it was considered an invalid taxon. Later, Chen (in Xiao et al., 2002) revised the diagnosis of this genus and retained it as a genuine fossil in the Ediacaran Miaohe biota, assigned and compared with coenocytic green algae. The carbonaceous compression assigned to the new species Jiuqunaoella sergeevii in the Lower Mesoproterozoic Saraipali Formation differs from the type species Jiuqunaoella simplicis by its chamber-like segments arranged in a beaded manner (Figure 6.2). Such morphotypes are also known as Eosolenides from upper Mesoproterozoic Lakhanda mudstone. Morphologically, Eosolenides fossils are fragments of an elongate, benthic, apparently soft-bodied, double-walled, tubular organism attached to its underlying substrate (German and Podkovyrov, 2009). A possible interpretation is that the ribbons of J. sergeevii may be a remnant of Eosolenides. In size, it is different from other ribbon-like carbonaceous compressions, such as Tyrasotaenia and Cyanocylindra. We assigned it to coenocytic green algae, as suggested by Xiao et al. (2002). Carbonaceous ribbons with similar features are recorded from the Chhuipali Formation of the Singhora Group exposed at the Bendla Dongar section of the study area (Figures 6.3–6.7).Genus: Sinocylindra. Chen and Erdtmann, 1991.Type species: Sinocylindra yunnanensis. Chen and Erdtmann, 1991.Sinocylindra yunnanensis. Chen and Erdtmann, 1991.Stratigraphic position: The Chhuipali Formations, the Singhora Group.Material: Two specimens from the Bendla Dongar section, north of Saraipali Township, Mahasamund District, Chhattisgarh, India.Description: Smooth, ribbon-like carbonaceous compression, twisted and folded. Both margins are more or less parallel. Specimens bear uneven and closely-spaced transverse nodes. Terminus ends of the ribbon are rounded. The ribbons are 3.0–4.0 mm long and up to 0.2 mm wide.Remarks: The genus Sinocylindra differs from other ribbon-like carbonaceous compressions, such as Tyrasotaenia and Jiuqunaoella in the Chhuipali biota, by its rounded terminus and more or less parallel wall margins. The carbonaceous ribbons assigned to Sinocylindra yunnanensis show a close resemblance to Miaohe specimens of China (Xiao et al., 2002). Chen and Erdtmann (1991) established the genus Sinocylindra with its species Sinocylindra yunnanensis from the Ediacaran Doushantuo Formation of China (Chen and Erdtmann, 1991). Xiao et al. (2002) suggested that it may be part of multicellular algae such as Chaetomorpha (green), Nemalion (red), or Chorda (brown), and also suggested an individual status rather than the cyanobacterial sheath Siphonophycus. Sinocylindra is well-known in the carbonaceous fossil assemblages of the Ediacaran to Cambrian periods. Its occurrence in the Mesoproterozoic Chhuipali Formation (1.3 Ga) indicates its antiquity is ∼400 Ma earlier in the Proterozoic biosphere.Genus: Tuanshanzia. Yan, 1995.Type species: Tuanshanzia fasciaria (Yan). Yan and Liu, 1997.Tuanshanzia fasciaria (Yan). Yan and Liu, 1997.Figures 7.1–7.6.Stratigraphic position: The Saraipali and Chhuipali Formations, the Singhora Group.Material: Twenty-five (25) specimens from the Surangi River section, southwest of Saraipali Township, and ten (10) specimens from the Bendla Dongar section, north of Saraipali Township, Mahasamund District, Chhattisgarh, India.Description: Unbranched, taeniate algal thallus, with rotundate apex, slightly tapering toward its base, smooth margins, thin, parastem absent. The thallus is moderately folded or twisted. Measured specimens are 4.0–18.5 mm in length and 0.25–0.5 mm in width.Remarks: The specimens of the present species in Singhora macroalgae are smaller than those from the Tuanshanzi Formation of the Changcheng Group (∼1,637 Ma) in Jinxian, Hubei, China (Yan and Liu, 1997). In size, they are similar to specimens of the Olive Shale, Semri Group, Vindhyan Supergroup (Sharma, 2006). Parastem-like structures are absent in both the algal thalli of T. fasciaria and T. lanceolata. Yan and Liu (1997) emended the genus Tuanshanzia, pointing out it can be distinguished from Changchengia by its lack of stalk-like parastem structure. They suggested that its species can be differentiated bytheir distinct morphology. It represents sessile frond-like algal thalli with bilaterally symmetrical and flat structures.Tuanshanzia lanceolata. Yan, 1995.Figures 7.7–7.11Stratigraphic position: The Saraipali and Chhuipali Formations, the Singhora Group.Material: Ten specimens from the Surangi River section, southwest of Saraipali Township, and fifteen specimens from the Bendla Dongar section, north of Saraipali Township, Mahasamund District, Chhattisgarh, India.Description: Broad sheet-like lanceolate algal thallus, moderately folded, smooth edges, widest in the middle, twisted, narrowing, and tapering toward both ends (Figures 7.7, 7.9, 7.10). The distal end of the thallus is incomplete in some specimens (Figures 7.8, 7.11), its parastem absent. Sheets are 3.0–8.0 mm long and 0.25–0.8 mm wide.Remarks: The present form is morphologically comparable to known specimens of the Tuanshanzi Formation (∼1637 Ma) of the Changcheng Group, China (Yan, 1995), the Olive Shale Semri Group, and the Vindhyan Supergroup (Sharma, 2006). It is comparatively smaller than original specimens. Flattened sheet-like compression resembles the tubular thallus of some of the Phaeophyta, viz., Siphonales (Dictyosiphonales) and green algae Ulvales. Some specimens in the Bendla Dongar section are characterized by a rusty color, probably resulting from oxidation or removal of organic matter.Genus: Tyrasotaenia. Gnilovskaya 1971.Type species: Tyrasotaenia podolica. Gnilovskaya, 1971.Tyrasotaenia podolica. Gnilovskaya, 1971.Figure 7.12.Stratigraphic position: The Chhuipali Formations, the Singhora Group.Material: Only six (6) specimens from the Bendla Dongar section, north of Saraipali Township, Mahasamund District, Chhattisgarh, India.Description: Solitary, unbranched, long cylindrical filaments, more or less straight and parallel, occasionally folded and curved. Measured specimens are 2–4 mm long and 0.1–0.2 mm thick.Remarks: The ribbon-like carbonaceous fossil Tyrasotaenia was initially recorded from the fine-grained clastic sediments of the 900 Ma Russian Platform (Gnilovskaya, 1971) and later from the 1,300 Ma Belt Supergroup, Montana (Walter et al., 1976); Little Dal Group, Mackenzie Mountains, Northwestern Canada (Hofmann and Aitken, 1979). In India, such types of carbonaceous filaments are known from the Proterozoic Vindhyan Supergroup (Shukla and Sharma, 1990; Sharma, 2006; Sharma et al., 2016). Based on its morphological similarity to stalks of Chorda and Scytosiphon belonging to the Phaeophyta, Tyrasotaenia podolica may be a remnant of benthic macroalga/seaweeds.
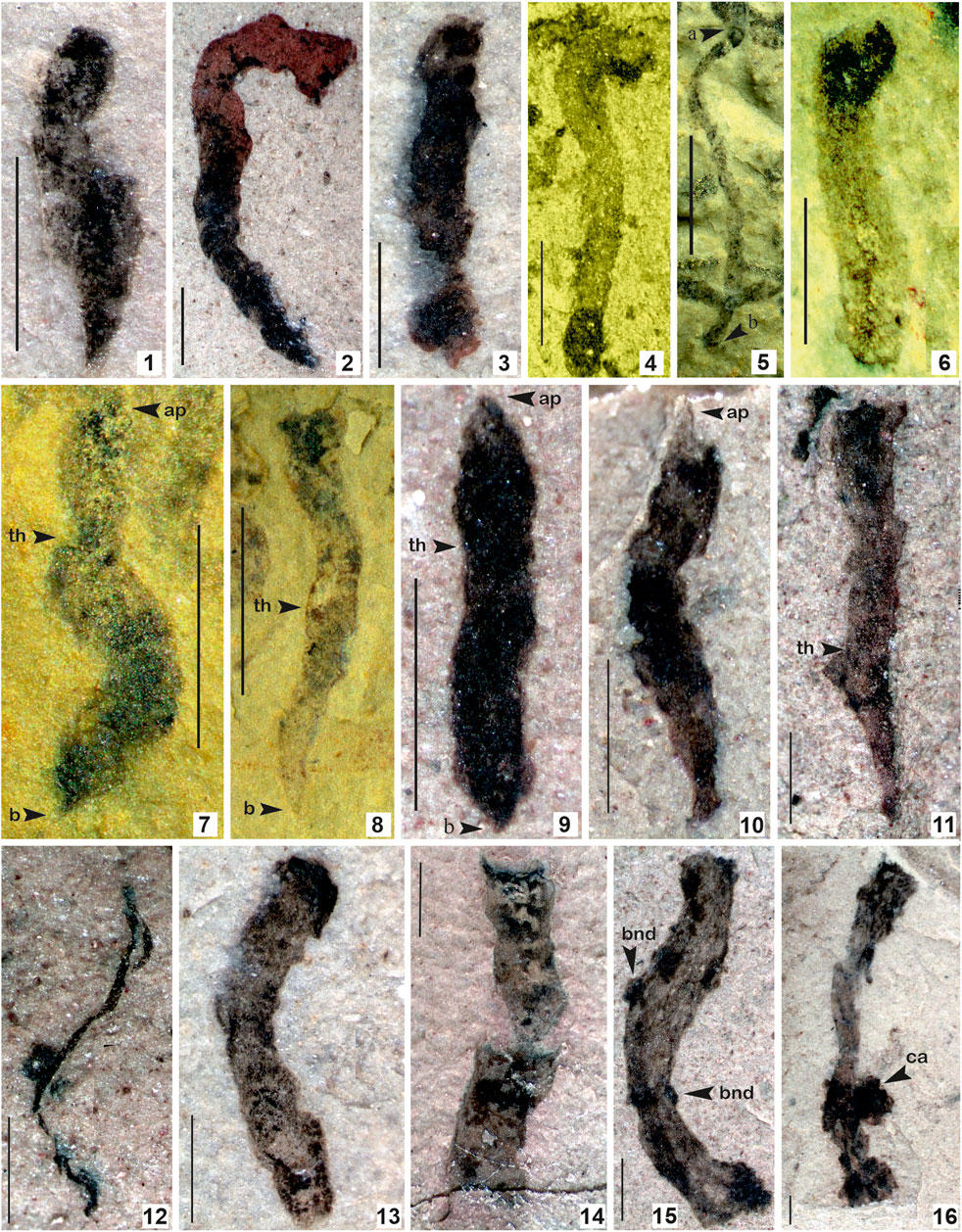
FIGURE 7. 1–6. Tuanshanzia fasciaria (Yan) Yan and Liu, 1997; 7–11. Tuanshanzia lanceolata Yan, 1995; 12. Tyrasotaenia podolica Gnilovskaya, 1971; 13–16. Unnamed ribbon-like film comprised of small conical/circular projection on the surface. (Arrow indicates the prominent features: bnd. Branching node; ca. colonial aggregates; th. thallus) 1. Specimen no. BSIP 42040; 2. Specimen no. BSIP 42039; 3. Specimens no. BSIP 42041; 4. Specimen no. BSIP 39777; 5. Specimen no. BSIP 39780; 6. Specimen no. BSIP 39769; 7. Specimen no. BSIP 39769; 8. Specimen no. BSIP 39782; 9. Specimen no. BSIP 42028; 10. Specimen no. BSIP 42036; 11. Specimen no. BSIP 42043; 12. Specimen no. BSIP 42025; 13. Specimen no. BSIP 42033b; 14. Specimen no. BSIP 42031; 15. Specimen no. BSIP 42045a; 16. Specimen no. BSIP 42044. Specimens 1–3, 9–16 from the Bendla Dongar section and 4–8 from the Surangi River section. Scale bar is 1.0 mm for each specimen.
Unnamed Forms
Stratigraphic position: The Chhuipali Formation, the Singhora Group.
Material: Five incomplete specimens from the Bendla Dongar section, north of Saraipali Township, Mahasamund District, Chhattisgarh, India.
Description: Compressed ribbon-like tubular structures with more or less parallel sidewalls, comprised of small protrusions projected and evenly distributed over the ribbon. Terminal margins of ribbon are straight. Projections in Figure 7.15 seem to be broken nodes of branching. Protrusions seem to be colonial aggregates of small coccoids (Figure 7.16). The thickness of the ribbon is up to 1.0 mm and the protrusion is up to 0.2 mm in diameter. Specimens are incomplete.
Remarks: The Bendla Dongar section contains many incompletely preserved ribbon-like tubular compressions. Some ribbons are comprised of more or less parallel margins similar to filamentous cyanobacteria Siphonophycus. However, ribbons are much larger than the Siphonophycus (Figures 7.13, 7.14). Some ribbon-like compressions comprise small protrusions projected and evenly distributed over the ribbon (Figure 7.15). These seem to be a node of further branching in the organism. The terminal margins of the ribbons are straight. Some specimens hold bunches of carbonaceous aggregates projected on the terminal end or sometimes in the middle (Figure 7.16).
Raman Spectroscopy
Over the last few decades, in situ techniques, including Laser Raman Spectroscopy (LRS), Fourier Transform Infrared Spectroscopy (FTIR), Secondary Ion Mass Spectroscopy (SIMS), and Atomic Force Microscopy (AFM) have been employed to understand the cellular morphology, ultrastructure, and chemical composition of organic matter preserved in these carbonaceous compressions (Javaux and Marshal, 2006; Oehler et al., 2006; Marshall et al., 2007; Schopf et al., 2010; Kilburn and Wacey, 2015; Delarue et al., 2018; Wacey et al., 2019). To understand the biogenicity and geochemical maturity of the carbonaceous films, selected specimens were analyzed using Laser Raman Spectroscopy. Laser Raman Spectroscopy is a non-intrusive, non-destructive analytical approach to investigate carbon compounds in Precambrian carbonaceous matter (Schopf et al., 2005). In situ Raman analysis on fossil materials at different targeted regions revealed the presence of 1,322 cm−1–1,356 cm−1 and 1,599 cm−1–1,608 cm−1 spectral bands, which indicate the level of graphitization in the form of D (Disordered peak) and G (Graphite peak) bands of organic carbon. Additionally, micro-Raman spectra of newly instituted carbonaceous fossil P. shuklaii included the D-band (Disordered band) at 1,339 cm−1 and G-band (Graphite band) at 1,603 cm−1 (Figure 8). This variation in the spectral band is caused by due vibration of complex carbon molecular structures, uneven surfaces, and dangling bonds (Qu et al., 2018). The obtained spectra and the positions and width of the D and G bands are characteristic of organic carbon, as revealed in Figure 8. The other best-matched minerals are glassy carbon and Shungite. Shungite is an elementary noncrystalline mineraloid of biogenic origin comprised of 98% carbon. The Raman spectra analysis and comparisons with available records (Noffke et al., 2013; Qu et al., 2018; Shukla et al., 2019) show that the Singhora films are typically carbonaceous and have a biogenic origin. The carbonaceous fossil composition is also corroborated by Raman first-order spectra organic-walled microfossils (OWMs) embedded in the host rock, which are dominated by distinct D and G bands of carbon (Supplementary Figure S3) supporting the syngenecity of cellular remains within the host rock. On the basis of fossil colour preservation fidelity, the Raman Index of Preservation (RIP) value of kerogen is 8.5 (Schopf et al., 2005).
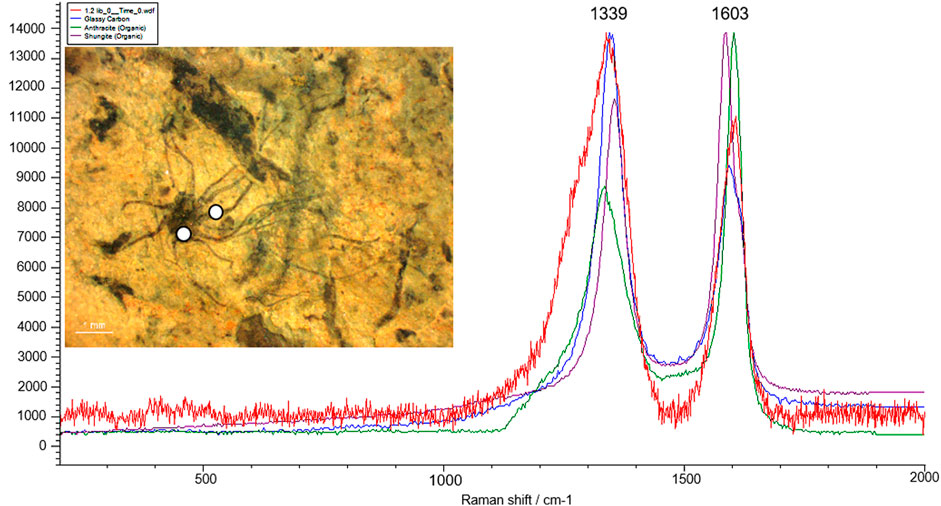
FIGURE 8. Micro-Raman spectra of the Singhora carbonaceous fossil Palaeoscytosiphon shuklaii reflecting the concentration of D (disorder) and G (Graphite) band. Specimen no. BSIP 41897, Scale bar is 2.0 mm.
Energy Dispersive X-ray Spectroscopy (EDX) in the SEM
The Energy Dispersive X-ray Spectroscopy (EDX) technique was applied to understand the chemical composition of the carbonaceous macroalgae. The results of EDX elemental mapping conducted on the Saraipali macroscopic carbonaceous fossils are illustrated in Figure 9. Elemental mapping reveals distinctive differences in composition between the matrix and the fossils. Analysis of the fossiliferous shale matrix demonstrates consistently high concentrations of aluminum (Al-11.28%), oxygen (O-50.98%), and silicon (Si-26.27%) relative to other elements (K-3.01% and Fe-1.93%). However, the concentration of carbon (C-6.54%) in shale matrix silicon (Si) is not enriched relative to the matrix (Figure 9.2). On the other hand, EDX analysis of carbonaceous fossil material demonstrates strong carbon enrichment (C-63.40%) relative to other elements, viz., oxygen (O-32.33%), silicon (Si-2.54%), aluminum (Al-1.06%), potassium (K-0.32%) and iron (Fe-0.36%) (Figure 9.1). Similarly, the Chhuipali macrofossils are preserved on a khaki shale bedding surface and are easily distinguishable from the shale matrix by their darker colour. The elemental composition of the shale matrix is the same as Saraipali Shale (Figure 9.2). Most of the fossils preserved are robust, continuous carbonaceous films as compressions/impressions.
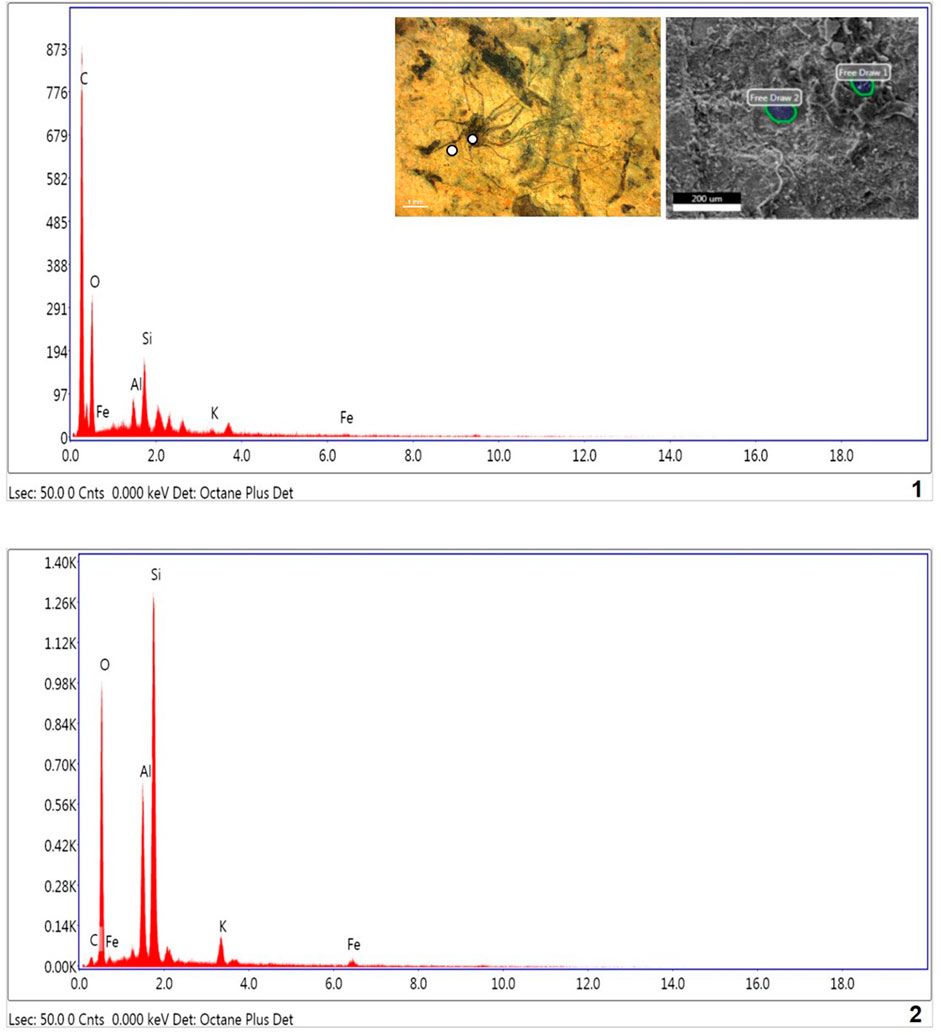
FIGURE 9. EDX spectra of Singhora carbonaceous compressions and host rock. 1. Macroscopic film shows high concentration of C and low concentration of other elements respectively. Presence of high carbon in fossils indicated carbonaceous and organic nature of fossils.; 2. Shale matrix: showing high concentration of Al, SI, O, K, and Fe elements and partial concentration of Si, indicating host rock is aluminosilicate clay.
EDX results reveal that the studied fossil material of macroalgae are typically composed of carbon, and indicates in situ preservation in aluminosilicate clay mineral-bearing rocks. Weathered macrofossils are easily visible due to the strong contrast between the dark fossils and the buff-coloured shale matrix. Aluminosilicate clay elements (Si and Al) are exhausted in the fossils relative to the matrix. Both the acid maceration and EDX elemental mapping analyses show elevated organic carbon enrichment in the fossils relative to the rock matrix. Further, this mode of preservation suggests Burgess Shale-type preservation for these macroscopic fossils (Butterfield, 1995; Gaines et al., 2008; Orr et al., 2009; Wang et al., 2014). Recently, based on morphological and EDX studies, Dornbos et al. (2016) have also suggested Burgess Shale-type fossil preservation for macroscopic filaments Chinggiskhaania bifurcata and Zuunartsphyton delicatum recorded from the upper Ediacaran and Cambrian Zuun-Arts Formations, Mongolia.
Discussion
Biogenicity of the Singhora Carbonaceous Fossils
In the present study, the extensively well-preserved Singhora carbonaceous compressions and impressions show distinct ribbon-shaped, leaf-shaped, fan-shaped morphology with putative stipes and/or holdfast structures preserved on the bedding surface of the host rock. These carbonaceous remains are found preserved in multiple layers in regularly repeated forms (see Supplementary Figures S4–S6). Gross morphology and preservation behaviour of the Singhora assemblage show close resemblance to ∼1,637 Ma Tuanshanzi and ∼1,560 Ma Gaoyuzhuang carbonaceous assemblages of China (Zhu and Chen 1995; Yan and Liu, 1997; Zhu et al., 2016). The Tuanshanzi Formation in Northern China is characterized by millimeter to centimeter-sized scattered carbonaceous compressions of irregular or indeterminate shape (ribbons and blades), interpreted as seaweed specifically attributed to Phaeophyta (Yan 1995; Zhu and Chen 1995; Yan and Liu, 1997) mainly based on varied morphology. The Gaoyuzhuang carbonaceous fossils are decimeter sized, regular, and strongly elongated (Zhu et al., 2016). Based on their size, shape, and chemical structure investigation, both the Chinese assemblages are considered benthic multicellular eukaryotes (Zhu et al, 2016; Qu et al., 2018).
Due to the lack of well-preserved cellular structures, carbonaceous compression fossils are often challenged for their biogenecity (Zhu et al., 2016). Among them, the Tuanshanzi carbonaceous fossils are the most debated. Knoll et al. (2006) have questioned the seaweed affinity proposed for the Tuanshanzi fossils, interpreting them as rare, fortuitously-shaped fragments re-deposited among irregular mat shards. They have also been interpreted as macroalgae with holdfast-stipe-blade differentiation, but their variable morphologies appear to suggest that some of them may be fragmented algal mats (Xiao and Dong, 2006). Butterfield (2009), based on their lack of regularly repeated forms and angular margins, considered the carbonaceous films a part of the fragmented microbial mats. Likewise, the biogenicity of the Singhora carbonaceous compression fossils can also be questioned. But due care was applied in recovering the Singhora fossil material. The Singhora carbonaceous compressions have rounded algal thalli (Figures 5.14–5.16; Figure 7.1), angular distal ends (Figures 5.4–5.7, 5.12; Figures 7.9–7.11) and twisted and folded sheets (Figures 5.2, 5.3, 5.8; Figure 7.7). These features make the Singhora carbonaceous fossils true Phaeophytic fossils. Two possible interpretations of the Tuanshanzi fossils’ angular margins are that they either represent the original shape of the algae, or their distal ends were twisted and overlapped during or after the burial process. The same interpretation may hold for the Singhora carbonaceous fossils.
A substantial number of macroscopic compressions, impressions, and casts are globally known in rocks of the latest Palaeoproterozoic and early Mesoproterozoic ages (Hofmann and Chen, 1981; Han and Runnegar, 1992; Kumar, 1995; Zhu and Chen, 1995; Yan and Liu, 1997; Zhu et al., 2000; Rai and Singh, 2006; Sharma, 2006; Zhu et al., 2016). Millimeter to centimeter-sized carbonaceous compressions with a diverse range of shapes, varying from circular, elongate, filamentous, to complex branching, recorded on the bedding plane surfaces of the Proterozoic successions, are reviewed by Hofmann (1992). Most of them are claimed as “multicellular eukaryotes” (Xiao et al., 2002; Knoll et al., 2006; Xiao and Dong, 2006; Zhu et al., 2016; Sharma and Singh, 2019; Bykova et al., 2020). Palaeoproterozoic carbonaceous macroscopic fossils, including the circular, elliptical, elongate, and irregularly shaped carbonaceous compressions often described as Chuaria, Shouhsienia, and Tawuia from the ca. 1,800 Ma Changzhougou and Chuanlinggou Formations in northern China (Hofmann and Chen 1981; Zhu et al., 2000), were claimed as megascopic eukaryotes and the oldest representatives of multicellular organisms (Zhu et al., 2000). Further investigations of the original material, including plane light investigations of thin sections and acid maceration, the petrographic study of thin sections, SEM, EDS, CHN, XRD, and biomarker analyses, indicate that Changzhougou compressions are clasts composed of clays or phosphates with little carbon, as typically found in pseudo-fossils instead of multicellular structures (Lamb et al., 2007). However, interpreting biogenic origins and affinities for many of these carbonaceous fossils remains relatively controversial because they lack well-preserved pigments and cellular structures (Hofmann, 1994; Knoll et al., 2006; Xiao and Dong, 2006; Lamb et al., 2007; Butterfield, 2009; El Albani et al., 2010; Knoll, 2011).
Recently, Qu et al. (2018) have reinvestigated chemical, isotopic, and molecular-structural features of organic matter from the carbonaceous films of the Tuanshanzi Formation, using in situ Raman Spectroscopy, FTIR, and organic carbon isotopic composition, to determine the affinity of carbonaceous compressions. These were interpreted as the oldest putative macroscopic multicellular eukaryotes by Zhu and Chen (1995). The results of Raman Spectroscopy and carbon isotopic composition of organic remains from the Tuanshanzi carbonaceous compressions demonstrate unambiguous benthic eukaryotic macroalgae and have experienced only advanced diagenesis (Qu et al., 2018). They further suggest that the Tuanshanzi Formation carbonaceous compressions imply higher oceanic oxygen concentrations during the Palaeo- and Mesoproterozoic than previously estimated. Similarly, well-preserved sheets of organic fragments extracted through acid maceration of the fossiliferous samples infer organized multicellularity for the Singhora fossils (Supplementary Figure S3). The organic fragments are dark brown, show various optical densities under transmitted light microscopy, demonstrating thermally-altered organic matter composition (anthracitic carbon). Further, the Raman spectra (D and G bands) of the carbonaceous material approximately equate organic fragments within the host rock. The Singhora carbonaceous compressions record a modest diversity of macroscopic photosynthetic multicellular eukaryotes syngeneic in nature.
Mode of Preservation and Taphonomy of Singhora Carbonaceous Fossils
Several different modes of preservation, specifically silicification, pyritization, phosphatization, carbonaceous compressions/impressions, and cast and mold preservation are established for most exceptionally-preserved micro and macroorganisms. Carbonaceous compressions/impressions are mainly responsible for preserving most macroalgae and organic-walled microfossils (OWMs) embedded in shales and siltstone of the Proterozoic successions. Our understanding of carbonaceous compression fossil preservation from the Palaeo- and Mesoproterozoic strata is inadequate due to limited records of macroalgae, particularly non-calcified macroalgae, unusual taphonomic conditions, and conflicts in taxonomic assignments (Lamb et al., 2007). The taphonomic biases contribute to the scanty fossil record of Proterozoic macroalgae.
We analyzed more than 200 specimens of macrofossils of the Singhora Group of rocks containing various branched and unbranched carbonaceous films with two-dimensional to three-dimensional morphological details (Table 2). Taphonomically, the Singhora macroalgal fossils described here are typically preserved as two-dimensional compressional elements (i.e., branches and stipes). Many of them have an elevated concentration of organic carbon, attesting to their preservation as carbonaceous compressions. Both the Saraipali and Chhuipali specimens are also enriched in aluminosilicates. Framboidal pyrite is conspicuously missing in both assemblages. Some specimens in the Chhuipali Shale show a reddish colour from where the carbon film has been detached, but they are easily distinguished from the rock matrix (Figure 5.4, Figure 6.9, Figure 7.2). Most macrofossils in the Singhora assemblage are preserved as carbonaceous compressions through kerogenization, as confirmed by acid macerations, Laser Raman Spectroscopy (Singh et al., 2019) and Energy Dispersive X-ray Spectroscopy elemental mapping analysis, which resulted in high organic carbon enrichment in the fossils compared to the rock matrix (discussed in the text). EDX results revealed that the studied macroalgae fossil material from the Singhora Group is typically composed of carbon and indicates in situ preservation in aluminosilicate clay mineral-bearing rocks. Additionally, Singhora carbonaceous compression macrofossils are characterized by mixed keroginization and aluminosilicification. Overall, this mode of preservation meets the requirements of Burgess Shale-type (BST) preservation for the carbonaceous compressions of the Singhora Group (Butterfield, 1995; Gaines et al., 2008; Orr et al., 2009; Wang et al., 2014; Ye et al., 2019). Globally, BST is considered exemplary of non-mineralizing organism preservation in fully marine siliciclastic sediments (Butterfield, 1995; Anderson et al., 2011). Several hypotheses for taphonomic pathways, viz., kerogenization, pyritization, and aluminosilicification provide insights to understanding BST preservation in most recorded fossil assemblages. However, in many cases, these processes are found in the same fossil assemblage and sometimes even in the same specimen (Cai et al., 2012).
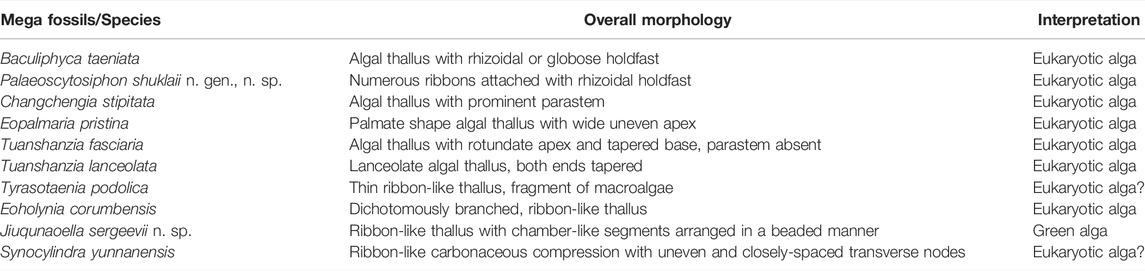
TABLE 2. Megascopic carbonaceous macroalgae from the Saraipali and Chhuipali Formations of the Singhora Group.
Affinity and Biostratigraphic Significance
An extensively well-preserved variety of macroscopic carbonaceous compressions and impressions are recorded from the Saraipali and Chhuipali Formations of the Mesoproterozoic Singhora Group. These are demonstrably macroalgal fossils and provide information about a new level of algal evolution in the Proterozoic biosphere. Similar megascopic carbonaceous fossils were recorded earlier, from the ca. 1,637 Ma Ma Tuanshanzi Formation from the Changcheng Group of Jixian, China, and termed Tuanshanzian Macroscopic Algae (Yan, 1995; Yan and Liu, 1997; Knoll et al., 2006; Xiao and Dong, 2006; Butterfield, 2009). Based on their considerably large size and varied shapes, they were considered the oldest benthic and eukaryotic multicellular algae (Yan, 1995). The lanceolate thalli of Tuanshanzia lanceolata and Tuanshanzia fasciaria were described as sessile frond-like algal thalli with bilaterally symmetrical and flat structures compared to certain species of Petalomia among the Phaeophyta and Enteromorpha or Monostroma among the Chlorophyta (Yan and Liu, 1997). Similarly, the palmate shape thallus of Eopalmaria pristina was linked with Palmaria/Porphyra/Rhodymenia among the Rhodophyta (Yan and Liu, 1997) and also with Monostroma among the Chlorophyta (Yan, 1995) and Spathoglossum among the Phaeophyta (Yan and Liu, 1997). The affinity of broad ribbon-like thallus with short parastem Changchengia stipitata was interpreted as a benthic metaphyte (Yan and Liu, 1997). Based on diversification, Yan and Liu (1997) have suggested a neritic, lagoon-like environment under a brackish-water habitat for the Tuanshanzian assemblage. The Saraipali and Chhuipali megascopic algae closely resemble Tuanshanzian specimens, but are relatively smaller in size. The ribbon-like thin tubular films of Tyrasotaenia podolica are also considered benthic remnants of Scytosiphon among the Phaeophyta (Yan and Liu, 1997). Based on megascopic size, thallus nature, and possible parastem-like features, carbonaceous films recorded from the Late Palaeoproterozoic to Early Mesoproterozoic Olive Shale were attributed to eukaryotic algae (Sharma, 2006). Based on its thallus, Steiner (1994) has placed Tyrasotaenia in synonimy with Vendotaenia.
The Saraipali and Chhuipali assemblages also contain taxonomically well-established megascopic carbonaceous films: namely, Baculiphyca taeniata (Yuan et al., 1995), Cyanocylindra yunnanensis (Chen and Erdtmann, 1991), and Eoholynia corumbensis (Gaucher et al., 2003), primarily known from the Ediacaran successions. A rhizoidal holdfast-bearing carbonaceous film, Baculiphyca taeniata, was compared with remnants of green algae, including the Siphonocladales and Dasycladales. However, due to their simple tubular morphology, Xiao et al. (2002) interpreted the ribbon-like tubular films of Cyanocylindra yunnanensis as filamentous cyanobacteria, although they have also placed this form under eukaryotic algae based on a size larger than the Siphonophycus Schopf. Similarly, the multi-branched carbonaceous film Eoholynia corumbensis is considered an eukaryotic algae, probably remnants of Phaeophyta or Rhodophyta (Hofmann 1994; Burzin, 1996). The occurrence of such Neoproterozoic complex morphologies in the Mesoproterozoic shales of the Singhora Group of the Chhattisgarh Supergroup predates their antiquity to earlier in time.
Apart from these fossils, the Saraipali Shale contains two new carbonaceous fossil taxa, namely Palaeoscytosiphon shuklaii n. gen., n. sp., and Jiuqunaoella sergeevii, n. sp. Preservation behaviour suggests that P. shuklaii is attributed to benthic multicellular algae Scytosiphon lomentaria belonging to family Scytosiphonaceae among the class Phaeophyceae (brown algae) (Figure 4). Scytosiphon lomentaria is a yellowish-brown or dark brown alga, comprised of hollow unbranched cylindrical stipes, up to 400 mm long and 3–10 mm wide. Stalks narrow at the end, with a wider base attached by a rhizoid-like short holdfast (Figure 2). Scytosiphon lomentaria most commonly occurs in the upper shore pool and grows on limpets, rocks, and wave-exposed shores. It is considered a model species for life history and molecular studies of brown algae.
The ribbon-like carbonaceous film Jiuqunaoella sergeevii consists of many bladder-shaped cells arranged in a beaded manner. In its global occurrence, Jiuqunaoella is widely known for the Ediacaran successions of China (Xiao et al., 2002; Ye et al., 2019). Its bladder-shaped beaded arrangement distinguishes Jiuqunaoella sergeevii. These bladder-like structures occur in the thallus, which enable it to receive more light for photosynthesis. In the Miaohe assemblage of the Doushantuo Formation, Jiuqunaoella simplicis was reconstructed as a sausage-like cylindrical tube, similar to some coenocytic green algae (Xiao et al., 2002; Ye et al., 2019). The specimens of Jiuqunaoella in the Singhora carbonaceous compressions are also characterized by ribbon-like structures with distinct chamber-like circular segments arranged in a beaded manner. Such complex morphological characteristics, including cell-to-cell connections, qualify J. sergeevii as a multicellular microorganism. Its degree of morphological differentiation and preservation behaviour reveals that the Saraipali and Chhuipali carbonaceous fossils are attributed to multicellular eukaryotes, similar to Palaeoproterozoic–Mesoproterozoic Tuanshanzi and Gaoyuzhuang carbonaceous fossil assemblages (Yan and Liu, 1997; Zhu et al., 2016), although their exact phylogenetic affinities are comparable to benthic seaweeds belonging to modern algae Phaeophyceae and Chlorophyceae (Xiao and Dong, 2006; Ye et al., 2019).
In this study, the Singhora carbonaceous compressions exhibit various morphologies, including fan-shaped, palmate, elongated, leaf-like, and ribbon-like, their algal thalli with/without holdfast at the base, with rounded and angular distal ends, isolated or dichotomously branched long tube-like cylindrical axis, along with multicellular reproductive structures. The holdfast’s role is to anchor the algal thallus on a hard substrate, protecting it from the currents that qualify their benthic habit, which is also consistent with brown algae (Zhu and Chen, 1995; Qu et al., 2018). Furthermore, their micro-macro morphologies and the results of Laser Raman Spectroscopy of the Singhora carbonaceous compressions share similarities with previously-known fossil materials, which are interpreted as multicellular eukaryotes (Xiao et al., 2002; Zhu et al., 2016; Qu et al., 2018; Zhang S. et al., 2021) and biogenic. Assignment to the multicellular eukaryotic fossils for the Singhora macroalgal carbonaceous compression also supports interpretations of early macroscopic multicellular eukaryote evolution in sulphidic and low-oxygenated Palaeo- and Mesoproterozoic oceans (Zhu et al., 2016; Qu et al., 2018; Bykova et al., 2020).
Antiquity of Carbonaceous Films
Our understanding of eukaryotes’s early evolution and diversification in Precambrian Palaeobiology, specifically multicellular macroalgae, is meager in both fossil and biomarker records. It is difficult to define the antiquity and evolution of fossilized remains in deep time, due to the rare occurrence of morphological and anatomical characters with those found in living forms (Butterfield, 2007). Available phylogenetic records suggest that eukaryotes may have first evolved in freshwater environments at the Palaeoproterozic- Mesoproterozoic transition (Blank, 2013). However, most of the fossil record supports their evolution in marine environments (Yan and Liu, 1997; Rai and Singh, 2006; Sharma, 2006; Bengtson et al., 2017; Qu et al., 2018). They extended into marine environments in the Tonian Period due to dramatic changes in the marine biological pump, food webs, and benthic habitats (Sanchez-Baracaldo et al., 2017; Del Cortona et al., 2020; Tang et al., 2020; Maloney et al., 2021).
The antiquity of macroalgae in deep time has also been discussed in several publications (Butterfield, 2001; Knoll et al., 2006; Butterfield, 2009, 2015; Knoll, 2015; Zhu et al., 2016; Bengtson et al., 2017; Tang et al., 2020; Zhang F. et al., 2021; Zhang S. et al., 2021; Tang et al., 2021). Globally, there are only a handful of published Proterozoic records of higher multicellular algae, as illustrated in Figure 10. Of these, the antiquity of some reports is considered confirmed (genuine) on the basis of distinct morphological and ultrastructural studies. Whereas the antiquity of a few macroalgae is unclear (doubtful) in the absence of distinct skeleton and taphonomic biases.
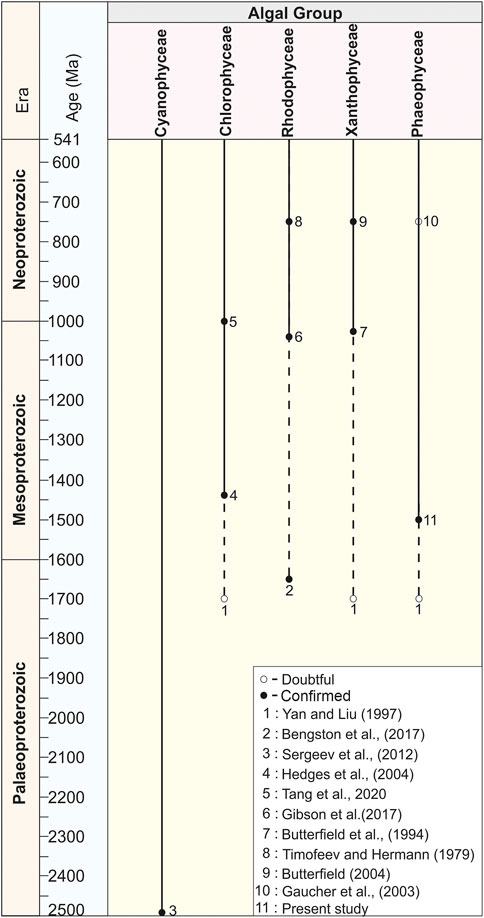
FIGURE 10. Chart showing the antiquity of higher algae in Proterozoic time span compiled on the basis of published records.
Several distinct types of micro and macro fossils are recorded after the Great Oxidation Event (GOE) from the Proterozoic succession and claimed as eukaryotes of the oldest antiquity (Retallack et al., 2013; Singh and Sharma, 2014). Eukaryotic fossils recorded from different formations of the world trace their antiquity from ∼2,200 Ma to ∼1800 Ma, such as the urn-shaped discoidal body of the Diskagma buttonii from the ∼2,200 Ma Palaeosols of South Africa (Retallack et al., 2013); the coiled carbonaceous megascopic fossil Grypania from the ∼1870 Ma Negaunee Iron-Formation, Michigan (Han and Runnegar, 1992; Schneider et al., 2002) and the string-of-beads morphology of Horodyskia from the 1,500 Ma Backdoor Formation of the Collier Group, Australia are assigned as the Oldest Known Tissue-Grade Colonial Eukaryote (Fedonkin and Yochelson, 2002; Grey et al., 2010). However, Sharma and Shukla (2009b) have established that Grypania was prokaryotic. Later, in a review, Knoll et al. (2006) considered the Horodyskia as a problematic macrofossil whose eukaryotic affinity is probable but not beyond debate. Similarly, decimeter to millimeter-scale carbonaceous ribbons and blades were recorded from the ∼1700 Ma to ∼1,600 Ma shales of the Changcheng Group, China (Yan and Liu, 1997; Gao et al., 2011; Zhu et al., 2016) and ∼1,600 Ma Olive Shale Formation, India (Sharma, 2006), and ∼1,500 Ma Singhora Group, India (Babu and Singh, 2013) were also claimed as eukaryotic fossils. However, their affinity with green, brown, and red algae is not confirmed in the absence of favorable diagnostics (Figure 10). Existing compilations of global taxonomic diversity suggest that eukaryotic fossils with complex morphology began to appear in the late Palaeoproterozoic. An eukaryotic nature has also been claimed for the large (>100 µm) sphaeromorphic acritarchs documented from the ∼1800 Ma Changzhougou Formation, North China (Lamb et al., 2009). Singh and Sharma (2014) have documented the oldest eukaryotic body fossil, Shuiyousphaeridium, from the latest Palaeoproterozoic-early Mesoproterozoic (>1,600 Ma) succession of the Chitrakoot Formation of the Vindhyan Supergroup, India. Similar fossils were known from younger sequences (1744 Ma–1,639 Ma) of the Ruyang Group, China (Yin, 1997; Pang et al., 2020), and (1,500 Ma – 1,400 Ma) Roper Group, Australia (Javaux, 2007; Javaux and Knoll, 2017). Therefore, at present, the Chitrakoot acritarchs are possibly the oldest eukaryotic remains in the world. Attempts have been made in this direction after the first record of extant Bangiophyte red algae Bangiomorpha pubescens from the late Mesoproterozoic (∼1,200 Ma) Hunting Formation of Canada (Butterfield, 2000; Gibson et al., 2018). In the fossil records before the Cryogenian (c. 800 Ma), the Bangiomorpha pubescens was the only taxonomically unresolved crown-group eukaryote considered the earliest known expression of extant multicellular forms and eukaryotic photosynthesis (Butterfield, 2000; Cohen and Macdonald, 2015). Recently, based on Re-Os geochronology, a precise ∼1,047 Ma age has been set for the origin of Bangiomorpha pubescens (Gibson et al., 2018) (Figure 10). In addition, Bengtson et al. (2017) have reported uniquely well-preserved fossils interpreted as probable crown-group rhodophytes from the ∼1,650 Ma Tirohan Dolomite of the lower Vindhyan (Semri Group) sediments of Son Valley in the Chitrakoot region (Figure 10). This report includes the discovery of two new fossils: namely, Rafatazmia chitrakootensis, Denaricion mendox and lobate form Ramathallus lobatus. Demonstrably, Rafatazmia is a non-branching filamentous thallus that has uniserial rows of large cells, which grow through diffusely distributed septation. This report predated the minimum age of the last eukaryotic common ancestor (LECA) and increased the antiquity of rhodophytes by about 400 Ma (Butterfield, 2015). However, in the absence of distinct pit plugs within the cells, red algae’s affinity with the fossil Rafatazmia is questionable (Carlisle et al., 2021). Recently, Tang et al. (2020) have predated the antiquity of chlorophyte algae (green algae) ∼200 Ma after reporting the multicellular macroscopic carbonaceous compression filamentous fossil Proterocladus antiquus from the ∼1,000 Ma Nanfen Formation of the Xihe Group, China (Figure 10). The Proterocladus was originally known from the early Neoproterozoic (Tonian) Swanbergfjellet Formation, Spitsbergen (Butterfield et al., 1994) (Figure 10). Together with its large size and morphology, integrated isotopic, geochemical, and ultrastructural studies on carbonaceous compressions of the ∼1,630 Ma Tuanshanzi Formation of North China support that the early benthic macroscopic multicellular eukaryotes evolved and prevailed in the sulphidic and low-oxygenated Palaeo- and Mesoproterozoic oceans (Qu et al., 2018). Cyanobacteria is long-ranging and considered a primary producer in Proterozoic Photosynthetic microorganisms (Sergeev et al., 2012) (Figure 10).
The fossil records of Phaeophyta (brown algae) evolution in the Precambrian successions are not well established. This is due to a generally soft-bodied nature and little occurrence of calcified taxa. A few millimeters of long carbonaceous compressions, typically preserved as flattened outlines or fragments, have been described from Ediacaran successions and their possible affinity as Phaeophyta claimed. The carbonaceous macroalga Eoholynia (Gnilovskaya, 1975)—–a cord or ribbon-like branched thallus, known from Vendian deposits in Russia, was considered eukaryotic algae remains. Its possible affinity was assigned as Phaeophyta or Rhodophyta (Gaucher et al., 2003). Similarly, based on the morphological and taphonomic assessment of Miaohephyton bifurcatum carbonaceous compressions―dichotomously branched multicellular thalli, known from the Ediacaran Doushantuo Formation, China―Steiner placed them among the brown algae (Xiao et al., 1998). Available records suggest that Phaeophytes may have diverged much later (the Mesozoic) in the geological past compared with red and green algae. In previous publications, claims have been made for many carbonaceous compressions as Phaeophyta, documented from the Mesozoic, Palaeozoic, and Ediacaran successions (Loeblich, 1974; Fry, 1983). However, the taxonomic affinity of these impression fossils is far from certain and has been discarded in the absence of key morphological characteristics (Kawai and Henry, 2016).
In the present study, two well-preserved macroscopic carbonaceous compression fossils: namely, Palaeoscytosiphon shuklaii n. gen, n. sp., and Jiuqunaoella sergeevii, n. sp., are described for the first time from the Saraipali Formation of the Singhora Group. Carbonaceous compressions of the fossil P. shuklaii represent all morphological characters in a single specimen, characterized by ribbon/tubular filaments with a distinct holdfast, sharing a similar morphology to the modern brown algae Scytosiphon lomentaria (Figure 7). Nevertheless, our present observations on Palaeoscytosiphon’s palaeobiologic affinities require a more detailed study that would exceed the scope of this study.
Similarly, carbonaceous compressions of Eoholynia corumbensis Gaucher et al. assigned as remains of eukaryotic algae are probably the class Phaeophyceae (brown algae)/Rhodophyceae (red algae) and considered as benthic seaweeds (Gnilovskaya, 1979; Gnilovskaya et al., 1988; Hofmann, 1994). Further, the present findings also suggest that the Phaeophyta evolved during the early Mesoproterozoic Era (∼1,500 Ma) instead of the previously suggested late Neoproterozoic (Gnilovskaya, 1975) (Figure 10). In addition, these studies also support the molecular clock analyses that suggest the divergence of Phaeophyta, Rhodophyta, and Viridiplantae in the Palaeoproterozoic–Mesoproterozoic Eras. Further, the crown-group Chlorophyta diverged during the late Mesoproterozoic to early Neoproterozoic eras; however, siphonous, multicellular, and siphonocladous chlorophytes evolved repeatedly in the late Neoproterozoic and Palaeozoic (Tang et al., 2020; Maloney et al., 2021).
Conclusions
In summary, abundant macroscopic carbonaceous compressions and impressions of macroalgal fossils, attributed to benthic seaweeds, are recorded from the shales and siltstone of the Saraipali and Chhuipali Formations of the Mesoproterozoic Singhora Group, Chhattisgarh Supergroup, India. The presence of exceptionally well-preserved distinct complex morphological features, including holdfasts, parastem, and thalli of different shapes and sizes suggest that the Singhora carbonaceous compressions are derived from the stem group of eukaryotic macroalgae. Two new taxa, Palaeoscytosiphon shuklaii n. gen., n. sp. (Phaeophyta), and Jiuqunaoella sergeevii n. sp. are established for the first time in the present finding. P. shuklaii n. gen., n. sp., Eoholynia corumbensis and Tyrasotaenia podolica most likely belong to brown algae (Phaeophyceae). If our interpretations are correct, both forms may predate the antiquity of Phaeophyta in the early Mesoproterozoic Era (∼1,500 Ma). Analysis using Laser Raman Spectroscopy (LRS) and Energy Dispersive X-ray Spectroscopy (EDX) of fossiliferous material demonstrate that the Singhora carbonaceous compressions of macroalgae are typically made up of organic carbon. Additionally, high carbon enrichment and depletion of Al, Si, K, and O in the carbonaceous compressions, as well as high enrichment of Al, Si, Fe, O, and K and low carbon in the shale matrix qualifies Burgess Shale-type preservation (BST) for the studied carbonaceous remains. Further, the carbonaceous macrofossils from the rocks of the Singhora Group provide evidence of benthic macroalgae inhabited in the shallow water of the low-oxygenated Mesoproterozoic Ocean.
Data Availability Statement
The original contributions presented in the study are included in the article/Supplementary Material. Further inquiries can be directed to the corresponding author.
Author Contributions
VS and MS conceived and designed the project. VS undertook multiple field visits in different localities for the collection of samples, placed them in the proper stratigraphic order, completed the initial scanning of the carbonaceous films from the rock slab. Both authors discussed the implications of the data and finalized the manuscript.
Funding
The field and laboratory work of the authors was supported by the Birbal Sahni Institute of Palaeosciences.
Conflict of Interest
The authors declare that the research was conducted in the absence of any commercial or financial relationships that could be construed as a potential conflict of interest.
Publisher’s Note
All claims expressed in this article are solely those of the authors and do not necessarily represent those of their affiliated organizations, or those of the publisher, the editors, and the reviewers. Any product that may be evaluated in this article, or claim that may be made by its manufacturer, is not guaranteed or endorsed by the publisher.
Acknowledgments
The authors are indebted to the Director of the Birbal Sahni Institute of Palaeosciences (BSIP) for providing necessary laboratory and library facilities and giving permission to publish this study (BSIP/RDCC/03/2022–23). Archana Sonker, Shivalee Srivastava, and Subodh Kumar are acknowledged for their assistance during geochemical characterization. The authors are grateful to reviewers for providing critical reviews and suggestions.
Supplementary Material
The Supplementary Material for this article can be found online at: https://www.frontiersin.org/articles/10.3389/feart.2022.825430/full#supplementary-material
Supplementary Figure S1 | Schematic drawings showing carbonaceous remains described from the Chhattisgarh Supergroup.1–3, 5. Tuanshanzia lanceolata; 4. Tuanshanzia fasciaria; 6. Baculiphyca taeniata; 7, 8. Changchengia stipitata; 9. Chuaria circularis; 10. Eopalmaria pristina; 11. Tyrasotaenia podolica; 12, 17. Jiuqunaoella sergeevii n. sp.; 13. Eoholynia corumbensis; 14, 15. Unnamed forms; 16. Synocylindra yunnanensis. Scale bar is 1.0 mm for each.
Supplementary Figure S2 | Schematic drawing showing mode of preservation of Palaeoscytosiphon shuklaii at different stages (1–4). Scale bar is 2.0 mm for each.
Supplementary Figure S3 | Raman spectra of organic matter of Saraipali fossiliferous shale extracted from HF maceration. Organic matter shows a distinct D- (1344 cm-1) and G- (1607 cm-1) band of organic carbon.
Supplementary Figure S4 | 1–2. Large fossiliferous specimens from the Saraipali Shale showing both large carbonaceous films and fragments on the bedding plane. Scale bar: 2.0 mm. 1. Specimen no. BSIP 42034; 2. Specimen no. BSIP 42045b.
Supplementary Figure S5 | 1–2. Large fossiliferous specimens from the Saraipali Shale showing both large carbonaceous films and fragments on the bedding plane. Scale bar: 2.0 mm. 1. Specimen no. BSIP 42045c; 2. Specimen no. BSIP 42045d.
Supplementary Figure S6 | 1–2. Fossiliferous slab from the Saraipali Formation showing the repeated morphology of carbonaceous films on the bedding plane. 1. Specimen no. BSIP 42045e; 2. Specimen no. BSIP 42045f.
References
Albani, A. E., Bengtson, S., Canfield, D. E., Bekker, A., Macchiarelli, R., Mazurier, A., et al. (20102002). Large Colonial Organisms with Coordinated Growth in Oxygenated Environments 2.1 Gyr ago Middle Proterozoic (1.5 Ga) Horodyskia Moniliformis Yochelson and Fedonkin, the Oldest Known Tissue Grade Colonial Eukaryote. NatureSmithsonian Contributions Paleobiology 46694 (73021-29), 100–104. doi:10.1038/nature09166
Amard, B. (1992). Ultrastructure of Chuaria (Walcott) Vidal and Ford (Acritarcha) from the Late Proterozoic Pendjari Formation, Benin and Burkina-Faso, West Africa. Precambrian Res. 57, 121–133. doi:10.1016/0301-9268(92)90096-7
Anderson, E. P., Schiffbauer, J. D., and Xiao, S. (2011). Taphonomic Study of Ediacaran Organic-Walled Fossils Confirms the Importance of Clay Minerals and Pyrite in Burgess Shale−type Preservation. Geology 39 (7), 643–646. doi:10.1130/G31969.1
Arouri, K., Greenwood, P. F., and Walter, M. R. (1999). A Possible Chlorophycean Affinity of Some Neoproterozoic Acritarchs. Org. Geochem. 30 (10), 1323–1337. doi:10.1016/S0146-6380(99)00105-9
Babu, R., and Singh, V. K. (2013). An Evaluation of Carbonaceous Metaphytic Remains from the Proterozoic Singhora Group of Chhattisgarh Supergroup, India. Special Publication- Geol. Soc. India 2013, 325–338.
Babu, R., and Singh, V. K. (2011). Record of Aquatic Carbonaceous Metaphytic Remains from the Proterozoic Singhora Group of Chhattisgarh Supergroup, India and Their Significance. J. Evol. Res. 3 (5), 47–66.
Bengtson, S., Sallstedt, T., Belivanova, V., and Whitehouse, M. (2017). Three-dimensional Preservation of Cellular and Subcellular Structures Suggests 1.6 Billion-Year-Old Crown-Group Red Algae. PLoS Biol. 15 (3), e2000735. doi:10.1371/journal.pbio.2000735
Bickford, M. E., Basu, A., Patranabis-Deb, S., Dhang, P. C., and Schieber, J. (2011). Depositional History of the Chhattisgarh Basin, Central India: Constraints from New SHRIMP Zircon Ages. J. Geol. 119 (1), 33–50. doi:10.1086/657300
Blank, C. E. (2013). Origin and Early Evolution of Photosynthetic Eukaryotes in Freshwater Environments: Reinterpreting Proterozoic Paleobiology and Biogeochemical Processes in Light of Trait Evolution. J. Phycol. 49 (6), 1040–1055. doi:10.1111/jpy.12111
Brocks, J. J., Jarrett, A. J. M., Sirantoine, E., Hallmann, C., Hoshino, Y., and Liyanage, T. (2017). The Rise of Algae in Cryogenian Oceans and the Emergence of Animals. Nature 548 (7669), 578–581. doi:10.1038/nature23457
Brodie, J., and Lewis, J. (2007). Unravelling the Algae: The Past, Present, and Future of Algal Systematic. 1st ed. Florida, US: CRC Press.
Burzin, M. B. (1996). Late Vendian (Neoproterozoic III) Microbial and Algal Communities of the Russian Platform: Models of Facies-dependent Distribution, Evolution and Reflection of Basin Development. Riv. Ital. Paleontol. Stratigr. 102 (3), 307–316.
Butterfield, N. J. (2001). Bias and Bonus: Fossil Preservation through the Precambrian-Cambrian Transition. Am. Zoologist 41 (6), 1404.
Butterfield, N. J. (2000). Bangiomorpha Pubescensn. gen., N. sp.: Implications for the Evolution of Sex, Multicellularity, and the Mesoproterozoic/Neoproterozoic Radiation of Eukaryotes. Paleobiology 26 (3), 386–404. doi:10.1666/0094-8373(2000)026<0386:bpngns>2.0.co;2
Butterfield, N. J. (2015). Early Evolution of the Eukaryota. Palaeontology 58 (1), 5–17. doi:10.1111/pala.12139
Butterfield, N. J., Knoll, A. H., and Swett, K. (1994). Paleobiology of the Neoproterozoic Svanbergfjellet Formation, Spitsbergen. Fossils Strata 34, 84. doi:10.1111/j.1502-3931.1994.tb01558.x
Butterfield, N. J. (2007). Macroevolution and Macroecology through Deep Time. Palaeontology 50 (1), 41–55. doi:10.1186/1741-7007-2-1310.1111/j.1475-4983.2006.00613.x
Butterfield, N. J. (2009). Modes of Pre-ediacaran Multicellularity. Precambrian Res. 173 (1-4), 201–211. doi:10.1016/j.precamres.2009.01.008
Butterfield, N. J. (1995). Secular Distribution of Burgess Shale-type Preservation. Lethaia 28 (1), 1–13. doi:10.1111/j.1502-3931.1995.tb01587.x
Bykova, N., LoDuca, S. T., Ye, Q., Marusin, V., Grazhdankin, D., and Xiao, S. (2020). Seaweeds through Time: Morphological and Ecological Analysis of Proterozoic and Early Paleozoic Benthic Macroalgae. Precambrian Res. 350, 105875. doi:10.1016/j.precamres.2020.105875
Cai, Y., Schiffbauer, J. D., Hua, H., and Xiao, S. (2012). Preservational Modes in the Ediacaran Gaojiashan Lagerstätte: Pyritization, Aluminosilicification, and Carbonaceous Compression. Palaeogeogr. Palaeoclimatol. Palaeoecol. 326-328, 109–117. doi:10.1016/j.palaeo.2012.02.009
Carlisle, E. M., Jobbins, M., Pankhania, V., Cunningham, J. A., and Donoghue, P. C. J. (2021). Experimental Taphonomy of Organelles and the Fossil Record of Early Eukaryote Evolution. Sci. Adv. 7 (5), eabe9487. doi:10.1126/sciadv.abe9487
Chakraborty, P. P., and Barkat, R. (2020). A Status Report on Age, Depositional Motif and Stratigraphy of Chhattisgarh, Indravati, Kurnool and Bhima Basins, Peninsular India. Pinsa 86. doi:10.16943/ptinsa/2020/49801
Chakraborty, P. P., Dey, S., and Mohanty, S. P. (2010). Proterozoic Platform Sequences of Peninsular India: Implications towards Basin Evolution and Supercontinent Assembly. J. Asian Earth Sci. 39 (6), 589–607. doi:10.1016/j.jseaes.2010.04.030
Chakraborty, P. P., Saha, S., and Das, P. (2015). Chapter 13 Geology of Mesoproterozoic Chhattisgarh Basin, Central India: Current Status and Future Goals. Geol. Soc. Lond. Memoirs 43, 185–205. doi:10.1144/M43.13
Chakraborty, P. P., Sarkar, S., and Patranabis-Deb, S. (2012). Tectonics and Sedimentation of Proterozoic Basins of Peninsular India. Proc. Indian Natl. Sci. Acad. 78 (3), 393–400.
Chakraborty, P. P., Tandon, S. K., Roy, S. B., Saha, S., and Paul, P. P. (2020). “Proterozoic Sedimentary Basins of India,” in Geodynamics of the Indian Plate (Berlin, Germany: Springer), 145–177. doi:10.1007/978-3-030-15989-4_4
Chen, J., and Erdtmann, B. D. (1991). The Early Evolution of Metazoa and the Significance of Problematic Taxa. Cambridge, UK: Cambridge University Press.
Chen, M., and Xiao, Z. (1991). Discovery of the Macrofossils in the Upper Sinain Doushantuo Formation at Miaohe, Eastern Yangtze Gorges. Sci. Geol. Sin. 4, 317–324.
Cohen, P. A., and Macdonald, F. A. (2015). The Proterozoic Record of Eukaryotes. Paleobiology 41 (4), 610–632. doi:10.1017/pab.2015.25
Cooper, R. A., Jago, J. B., Mackinnon, D. I., Shergold, J. H., and Vidal, G. (1982). “Late Precambrian and Cambrian Fossils from Northern Victoria Land and Their Stratigraphic Implications,” in Antarctic Geoscience. 3rd Symposium on Antarctic Geology and Geophysics (Madison: University of Wisconsin Press), 629–633.
Das, D. P., Dutta, N. K., Dutta, D. R., Thanavellu, C., and Baburao, K. (2003). Singhora Group - the Oldest Proterozoic Lithopackage of Eastern Bastar Craton and its Significance. Indian Miner. 57 (3-4), 127–138.
Das, D. P., Kundu, A., Das, N., Dutta, D. R., Kumaran, K., Ramamurthy, S., et al. (1992). Lithostratigraphy and Sedimentation of Chhattisgarh Basin. Indian Miner. 46 (3-4), 271–288.
Das, K., Chakraborty, P. P., Horie, K., Tsutsumi, Y., Saha, S., and Balakrishnan, S. (2017). “Detrital Zircon U-Pb Geochronology, Nd Isotope Mapping, and Sediment Geochemistry from the Singhora Group, Central India,” in Sediment Provenance. Editor R. Mazumder (Amsterdam, Netherlands: Elsevier), 403–451. doi:10.1016/b978-0-12-803386-9.00015-0
Das, K., Yokoyama, K., Chakraborty, P. P., and Sarkar, A. (2009). Basal Tuffs and Contemporaneity of the Chattisgarh and Khariar Basins Based on New Dates and Geochemistry. J. Geol. 117 (1), 88–102. doi:10.1086/593323
Del Cortona, A., Jackson, C. J., Bucchini, F., Van Bel, M., D’hondt, S., Škaloud, P., et al. (2020). Neoproterozoic Origin and Multiple Transitions to Macroscopic Growth in Green Seaweeds. Proc. Natl. Acad. Sci. U.S.A. 117 (5), 2551–2559. doi:10.1073/pnas.1910060117
Delarue, F., Robert, F., Tartèse, R., Sugitani, K., Tang, Q., Duhamel, R., et al. (2018). Can NanoSIMS Probe Quantitatively the Geochemical Composition of Ancient Organic-Walled Microfossils? A Case Study from the Early Neoproterozoic Liulaobei Formation. Precambrian Res. 311, 65–73. doi:10.1016/j.precamres.2018.03.003
Dornbos, S. Q., Oji, T., Kanayama, A., and Gonchigdorj, S. (2016). A New Burgess Shale-type Deposit from the Ediacaran of Western Mongolia. Sci. Rep. 6, 23438. doi:10.1038/srep23438
Du, R., Tian, L., and Li, H. (1986). Discovery of Megafossils in the Gaoyuzhuang Formation of the Chancheng System, Jixian. Acta Geol. Sin. 60, 115–119.
Dutta, S., Steiner, M., Banerjee, S., Erdtmann, B.-D., Jeevankumar, S., and Mann, U. (2006). Chuaria Circularis from the Early Mesoproterozoic Suket Shale, Vindhyan Supergroup, India: Insights from Light and Electron Microscopy and Pyrolysis-Gas Chromatography. J. Earth Syst. Sci. 115 (1), 99–112. doi:10.1007/BF02703028
Ford, T. D., and Breed, W. J. (1973). probletical Precambrian fossils Chuaria Palaeontol. 16, 533–550.
Fry, W. L. (1983). An Algal Flora from the Upper Ordovician of the Lake Winnipeg Region, Manitoba, Canada. Rev. Palaeobot. Palynology 39 (3), 313–341. doi:10.1016/0034-6667(83)90018-0
Gaines, R. R., Briggs, D. E. G., and Yuanlong, Z. (2008). Cambrian Burgess Shale-type Deposits Share a Common Mode of Fossilization. Geol 36 (10), 755–758. doi:10.1130/g24961a.1
Gao, L., Liu, P., Yin, C., Zhang, C., Ding, X., Liu, Y., et al. (2011). Detrital Zircon Dating of Meso- and Neoproterozoic Rocks in North China and its Implications. Acta Geol. Sin. Engl. Ed. 85, 271–282. doi:10.1111/j.1755-6724.2011.00397.x
Gaucher, C., Boggiani, P., Sprechmann, P., Sial, A., and Fairchild, T. (2003). Integrated correlation of the Vendian to Cambrian Arroyo del Soldado and Corumbá Groups (Uruguay and Brazil): palaeogeographic, palaeoclimatic and palaeobiologic implications. Precambrian Res. 120 (3–4), 241–278. doi:10.1016/S0301-9268(02)00140-7
German, T. N., and Podkovyrov, V. N. (2009). New Insights into the Nature of the Late Riphean Eosolenides. Precambrian Res. 173 (1-4), 154–162. doi:10.1016/j.precamres.2009.03.018
Gibson, T. M., Shih, P. M., Cumming, V. M., Fischer, W. W., Crockford, P. W., Hodgskiss, M. S. W., et al. (2018). Precise Age of Bangiomorpha Pubescens Dates the Origin of Eukaryotic Photosynthesis. Geology 46 (2), 135–138. doi:10.1130/G39829.1
Gnilovskaya, M. B. (1971). Drevnejshie Vodnye Rasteniya Venda Russkoj Platformy (The Oldest Vendian Aquatic Plants on the Russian Platform). Palaeontol. Zhurnal 3, 101–107.
Gnilovskaya, M. B., Istchenko, A. A., Kolesniko, C. M., Korenchuk, L. V., and Udalstov, A. P. (1988). Vendotaenids of the East European Platform.
Gnilovskaya, M. B. (1975). Novye Dannye O Prirode Vendotenid (New Data on the Nature of Vendotaenids). Dokl. Akad. Nauk. SSSR 221 (4), 953–955.
Gnilovskaya, M. B. (1979). The Vendian Metaphyta. Bull. Centres Rech. Exploration-Production Elf-Aquitaine 3 (2), 611–618.
Gnilovskaya, M. B., Veis, A. F., Bekker, Y. R., Olovyanishnikov, V. G., and Raaben, M. E. (2000). Pre-Ediacarian Fauna from Timan (Annelidomorphs of the Late Riphean). Stratigr. Geol. Correl. 8 (4), 327–352.
Grazhdankin, D. V., Balthasar, U., Nagovitsin, K. E., and Kochnev, B. B. (2008). Carbonate-hosted Avalon-type Fossils in Arctic Siberia. Geol 36 (10), 803–806. doi:10.1130/G24946A.1
Grazhdankin, D. V., Nagovitsin, K. E., and Maslov, A. V. (2007). Late Vendian Miaohe-type Ecological Assemblage of the East European Platform. Dokl. Earth Sc. 417 (1), 1183–1187. doi:10.1134/s1028334x07080107
Grey, K., Yochelson, E. L., Fedonkin, M. A., and Martin, D. M. (2010). Horodyskia Williamsii New Species, a Mesoproterozoic Macrofossil from Western Australia. Precambrian Res. 180 (1-2), 1–17. doi:10.1016/j.precamres.2010.02.006
Haines, P. W. (1998). ChuariaWalcott, 1899 in the Lower Wessel Group, Arafura Basin, Northern Australia. Alcheringa Australas. J. Palaeontol. 22 (1), 1–8. doi:10.1080/03115519808619235
Han, T.-M., and Runnegar, B. (1992). Megascopic Eukaryotic Algae from the 2.1-Billion-Year-Old Negaunee Iron-Formation, Michigan. Science 257 (5067), 232–235. doi:10.1126/science.1631544
Hofmann, H. J., and Altken, J. D. (1979). Precambrian Biota from the Little Dal Group, Mackenzie Mountains, Northwestern Canada. Can. J. Earth Sci. 16 (1), 150–166. doi:10.1139/e79-014
Hofmann, H. J., and Jinbiao, C. (1981). Carbonaceous Megafossils from the Precambrian (1800 Ma) Near Jixian, Northern China. Can. J. Earth Sci. 18 (3), 443–447. doi:10.1139/e81-038
Hofmann, H. J. (1992). “Proterozoic and Selected Cambrian Megascopic Carbonaceous Films,” in The Proterozoic Biosphere, a Multidisciplinary Study. Editors J. W. Schopf, and C. Klein (Cambridge: Cambridge University Press), 957–998.
Hofmann, H. J. (1994). Proterozoic Carbonaceous Compressions ("metaphytes" and "worms"). Early life earth 84, 342–357.
Hofmann, H. J., and Rainbird, R. H. (1994). Carbonaceous Megafossils from the Neoproterozoic Shaler Supergroup of Arctic Canada. Palaeontology 37 (4), 721–731.
Hofmann, H. J. (1977). The Problematic Fossil Chuaria from the Late Precambrian Uinta Mountain Group, Utah. Precambrian Res. 4 (1), 1–11. doi:10.1016/0301-9268(77)90009-2
Isson, T. T., Love, G. D., Dupont, C. L., Reinhard, C. T., Zumberge, A. J., Asael, D., et al. (2018). Tracking the Rise of Eukaryotes to Ecological Dominance with Zinc Isotopes. Geobiology 16 (4), 341–352. doi:10.1111/gbi.12289
Javaux, E. J., and Marshal, C. P. (2006). A New Approach in Deciphering Early Protist Paleobiology and Evolution: Combined Microscopy and Microchemistry of Single Proterozoic Acritarchs. Rev. Palaeobot. Palynology 139 (1-4), 1–15. doi:10.1016/j.revpalbo.2006.01.005
Jensen, S., Palacios, T., and Martí Mus, M. (2007). A brief review of the fossil record of the Ediacaran-Cambrian transition in the area of Montes de Toledo-Guadalupe, Spain. Geol. Soc. Lond. Spec. Publ. 286, 223–235. doi:10.1144/SP286.16
Kawai, H., and Henry, E. C. (2016). “Phaeophyta,” in Handbook of the Protists. Editors J. M. Archibald, A. G. B. Simpson, C. H. Slamovits, L. Margulis, M. Melkonian, D. J. Chapmanet al. (Cham: Springer International Publishing), 267–304. doi:10.1007/978-3-319-32669-6_31-1
Kilburn, M., and Wacey, D. (2015). Nanoscale Secondary Ion Mass Spectrometry (NanoSIMS) as an Analytical Tool in the Geosciences. RSC Detect. Sci. 2015, 1–34.
Knoll, A. H., Javaux, E. J., Hewitt, D., and Cohen, P. (2006). Eukaryotic Organisms in Proterozoic Oceans. Phil. Trans. R. Soc. B 361 (1470), 1023–1038. doi:10.1098/rstb.2006.1843
Knoll, A. H. (2015). Paleobiological Perspectives on Early Microbial Evolution. Cold Spring Harb. Perspect. Biol. 7 (7), a018093. doi:10.1101/cshperspect.a018093
Knoll, A. H. (2011). The Multiple Origins of Complex Multicellularity. Annu. Rev. Earth Planet. Sci. 39, 217–239. doi:10.1146/annurev.earth.031208.100209
Kumar, S. (1995). Megafossils from the Mesoproterozoic Rohtas Formation (The Vindhyan Supergroup), Katni Area, Central India. Precambrian Res. 72 (3-4), 171–184. doi:10.1016/0301-9268(94)00085-6
Kumar, S. (2001). Mesoproterozoic Megafossil Chuaria–Tawuia Association May Represent Parts of a Multicellular Plant, Vindhyan Supergroup, Central India. Precambrian Res. 106 (3–4), 187–211. doi:10.1016/S0301-9268(00)00093-0
Kumar, S., and Srivastava, P. (2003). Carbonaceous Megafossils from the Neoproterozoic Bhander Group, Central India. J. Palaeontol. Soc. India 48, 139–154.
Laflamme, M., Darroch, S. A. F., Tweedt, S. M., Peterson, K. J., and Erwin, D. H. (2013). The End of the Ediacara Biota: Extinction, Biotic Replacement, or Cheshire Cat? Gondwana Res. 23 (2), 558–573. doi:10.1016/j.gr.2012.11.004
Lamb, D. M., Awramik, S. M., Chapman, D. J., and Zhu, S. (2009). Evidence for Eukaryotic Diversification in the ∼1800 Million-Year-Old Changzhougou Formation, North China. Precambrian Res. 173 (1-4), 93–104. doi:10.1016/j.precamres.2009.05.005
Lamb, D. M., Awramik, S. M., and Zhu, S. (2007). Paleoproterozoic Compression-like Structures from the Changzhougou Formation, China: Eukaryotes or Clasts? Precambrian Res. 154 (3–4), 236–247. doi:10.1016/j.precamres.2006.12.012
Lenton, T. M., Boyle, R. A., Poulton, S. W., Shields-Zhou, G. A., and Butterfield, N. J. (2014). Co-evolution of Eukaryotes and Ocean Oxygenation in the Neoproterozoic Era. Nat. Geosci. 7 (4), 257–265. doi:10.1038/ngeo2108
Leonov, M., Fedonkin, M., Vickers-Rich, P., Ivantsov, A. Y., and Trusler, P. (2009). Discovery of the First Macroscopic Algal Assemblage in the Terminal Proterozoic of Namibia, Southwest Africa. Commun. Geol. Surv. Namib. 14 (null), 87–93.
Loeblich, A. R. (1974). Protistan Phylogeny as Indicated by the Fossil Record. TAXON 23 (2-3), 277–290. doi:10.2307/1218707
Lyons, T. W., Reinhard, C. T., and Planavsky, N. J. (2014). The Rise of Oxygen in Earth's Early Ocean and Atmosphere. Nature 506 (7488), 307–315. doi:10.1038/nature13068
Maloney, K. M., Halverson, G. P., Schiffbauer, J. D., Xiao, S., Gibson, T. M., Lechte, M. A., et al. (2021). New Multicellular Marine Macroalgae from the Early Tonian of Northwestern Canada. Geology 49 (6), 743–747. doi:10.1130/g48508.1
Marshall, C. P., Love, G. D., Snape, C. E., Hill, A. C., Allwood, A. C., Walter, M. R., et al. (2007). Structural Characterization of Kerogen in 3.4Ga Archaean Cherts from the Pilbara Craton, Western Australia. Precambrian Res. 155 (1-2), 1–23. doi:10.1016/j.precamres.2006.12.014
Marusin, V. V., Grazhdankin, D. V., and Maslov, A. V. (2011). Redkino Stage in Evolution of Vendian Macrophytes. Dokl. Earth Sc. 436 (2), 197–202. doi:10.1134/S1028334X11020176
Mukherjee, A., and Ray, R. K. (2010). An Alternate View on the Stratigraphic Position of the ∼1‐Ga Sukhda Tuff Vis‐à‐vis Chronostratigraphy of the Precambrians of the Central Indian Craton. J. Geol. 118 (3), 325–332. doi:10.1086/651502
Mukherjee, A., Ray, R. K., Tewari, D., Ingle, V. K., Sahoo, B. K., and Y Khan, M. W. (2014). Revisiting the Stratigraphy of the Mesoproterozoic Chhattisgarh Supergroup, Bastar Craton, India Based on Subsurface Lithoinformation. J. Earth Syst. Sci. 123 (3), 617–632. doi:10.1007/s12040-014-0418-z
Muscente, A. D., Bykova, N., Boag, T. H., Buatois, L. A., Mángano, M. G., Eleish, A., et al. (2019). Ediacaran Biozones Identified with Network Analysis Provide Evidence for Pulsed Extinctions of Early Complex Life. Nat. Commun. 10 (1), 911. doi:10.1038/s41467-019-08837-3
Noffke, N., Christian, D., Wacey, D., and Hazen, R. M. (2013). Microbially Induced Sedimentary Structures Recording an Ancient Ecosystem in theca.3.48 Billion-Year-Old Dresser Formation, Pilbara, Western Australia. Astrobiology 13 (12), 1103–1124. doi:10.1089/ast.2013.1030
Oehler, D. Z., Robert, F., Mostefaoui, S., Meibom, A., Selo, M., and McKay, D. S. (2006). Chemical Mapping of Proterozoic Organic Matter at Submicron Spatial Resolution. Astrobiology 6 (6), 838–850. doi:10.1089/ast.2006.6.838
Orr, P. J., Kearns, S. L., and Briggs, D. E. G. (2009). Elemental Mapping of Exceptionally Preserved ‘carbonaceous Compression’ Fossils. Palaeogeogr. Palaeoclimatol. Palaeoecol. 277 (1), 1–8. doi:10.1016/j.palaeo.2009.02.009
Pang, K., Tang, Q., Wu, C., Li, G., Chen, L., Wan, B., et al. (2020). Raman Spectroscopy and Structural Heterogeneity of Carbonaceous Material in Proterozoic Organic-Walled Microfossils in the North China Craton. Precambrian Res. 346, 105818. doi:10.1016/j.precamres.2020.105818
Parfrey, L. W., Lahr, D. J. G., Knoll, A. H., and Katz, L. A. (2011). Estimating the Timing of Early Eukaryotic Diversification with Multigene Molecular Clocks. Proc. Natl. Acad. Sci. U.S.A. 108 (33), 13624–13629. doi:10.1073/pnas.1110633108
Patranabis-Deb, S., and Chaudhuri, A. K. (2008). Sequence Evolution in the Eastern Chhattisgarh Basin; Constraints on Correlation and Stratigraphic Analysis. Palaeobotanist 57 (1-2), 15–32.
Qu, Y., Zhu, S., Whitehouse, M., Engdahl, A., and McLoughlin, N. (2018). Carbonaceous Biosignatures of the Earliest Putative Macroscopic Multicellular Eukaryotes from 1630 Ma Tuanshanzi Formation, North China. Precambrian Res. 304, 99–109. doi:10.1016/j.precamres.2017.11.004
Rai, V., and Singh, V. K. (2006). Discovery of Megascopic Multicellularity in Deep Time: New Evidences from the ∼1.63 Billion Years Old Lower Vindhyan Succession, Vindhyan Supergroup, Uttar Pradesh, India. J. Appl. Biosci. 32 (2), 196–203.
Retallack, G. J., Krull, E. S., Thackray, G. D., and Parkinson, D. (2013). Problematic Urn-Shaped Fossils from a Paleoproterozoic (2.2Ga) Paleosol in South Africa. Precambrian Res. 235, 71–87. doi:10.1016/j.precamres.2013.05.015
Rowland, S. M., and Rodriguez, M. G. (2014). A Multicellular Alga with Exceptional Preservation from the Ediacaran of Nevada. J. Paleontol. 88 (2), 263–268. doi:10.1666/13-075
Samuelsson, J., and Butterfield, N. J. (2001). Neoproterozoic Fossils from the Franklin Mountains, Northwestern Canada: Stratigraphic and Palaeobiological Implications. Precambrian Res. 107 (3–4), 235–251. doi:10.1016/S0301-9268(00)00142-X
Sánchez-Baracaldo, P., Raven, J. A., Pisani, D., and Knoll, A. H. (2017). Early Photosynthetic Eukaryotes Inhabited Low-Salinity Habitats. Proc. Natl. Acad. Sci. U.S.A. 114 (37), E7737–E7745. doi:10.1073/pnas.1620089114
Schneider, D. A., Bickford, M. E., Cannon, W. F., Schulz, K. J., and Hamilton, M. A. (2002). Age of Volcanic Rocks and Syndepositional Iron Formations, Marquette Range Supergroup: Implications for the Tectonic Setting of Paleoproterozoic Iron Formations of the Lake Superior Region. Can. J. Earth Sci. 39 (6), 999–1012. doi:10.1139/e02-016
Schopf, J. W., Kudryavtsev, A. B., Agresti, D. G., Czaja, A. D., and Wdowiak, T. J. (2005). Raman Imagery: A New Approach to Assess the Geochemical Maturity and Biogenicity of Permineralized Precambrian Fossils. Astrobiology 5 (3), 333–371. doi:10.1089/ast.2005.5.333
Schopf, J. W., Kudryavtsev, A. B., and Sergeev, V. N. (2010). Confocal Laser Scanning Microscopy and Raman Imagery of the Late Neoproterozoic Chichkan Microbiota of South Kazakhstan. J. Paleontol. 84 (3), 402–416. doi:10.1666/09-134.1
Schopf, J. W., Tripathi, A. B., and Kudryavtsev, A. B. (2006). Three-dimensional Confocal Optical Imagery of Precambrian Microscopic Organisms. Astrobiology 6 (1), 1–16. doi:10.1089/ast.2006.6.1
Sergeev, V. N., Sharma, M., and Shukla, Y. (2012). Proterozoic Fossil Cyanobacteria. Palaeobotanist 61 (2), 189–358.
Sharma, M. (2006). Late Palaeoproterozoic (Statherian) Carbonaceous Films from the Olive Shale (Koldaha Shale), Semri Group, Vindhyan Supergroup, India. J. Palaeontol. Soc. India 51 (2), 27–35.
Sharma, M., Mishra, S., Dutta, S., Banerjee, S., and Shukla, Y. (2009). On the Affinity of Chuaria-Tawuia Complex: A Multidisciplinary Study. Precambrian Res. 173 (1-4), 123–136. doi:10.1016/j.precamres.2009.04.003
Sharma, M., Shukla, M., and Venkatachala, B. S. (1991). Metaphyte and Metazoan Fossils from Precambrian Sediments of India; a Critique. Palaeobotanist 40, 8–51.
Sharma, M., and Shukla, Y. (2009a). Mesoproterozoic Coiled Megascopic Fossil Grypania Spiralis from the Rohtas Formation, Semri Group, Bihar, India. Curr. Sci. 96, 1636–1640.
Sharma, M., and Shukla, Y. (2009b). Taxonomy and Affinity of Early Mesoproterozoic Megascopic Helically Coiled and Related Fossils from the Rohtas Formation, the Vindhyan Supergroup, India. Precambrian Res. 173 (1-4), 105–122. doi:10.1016/j.precamres.2009.05.002
Sharma, M., and Singh, V. K. (2019). “Megascopic Carbonaceous Remains from Proterozoic Basins of India,” in Geological Evolution of the Precambrian Indian Shield. Editor M. E. A. Mondal (Cham: Springer International Publishing), 725–749. doi:10.1007/978-3-319-89698-4_27
Sharma, M., Tiwari, M., Ahmad, S., Shukla, R., Shukla, B., Singh, V. K., et al. (2016). Palaeobiology of Indian Proterozoic and Early Cambrian Successions- Recent Developments. Proc. Indian Natl. Sci. Acad. 82 (3), 559–579. doi:10.16943/ptinsa/2016/48468
Shukla, M., and Sharma, M. (1990). Palaeobiology of Suket Shale, Vindhyan Supergroup; Age Implications. Special Publ. Geol. Surv. India 28, 411–434.
Shukla, Y., Sharma, M., Noffke, N., and Callefo, F. (2019). Biofilm Microfacies in Phosphoritic Units of the Neoproterozoic Halkal Shale, Bhima Basin, South India. Precambrian Res. doi:10.1016/j.precamres.2019.105501
Singh, V. K., Babu, R., and Shukla, M. (2009). Discovery of Carbonaceous Remains from the Neoproterozoic Shales of Vindhyan Supergroup, India. J. Evol. Bioology Res. 1 (1), 001–017.
Singh, V. K., and Sharma, M. (2014). Morphologically Complex Organic-Walled Microfossils (OWM) from the Late Palaeoproterozoic - Early Mesoproterozoic Chitrakut Formation, Vindhyan Supergroup, Central India and Their Implications on the Antiquity of Eukaryotes. J. Palaeontol. Soc. India 59 (1), 89–102.
Singh, V. K., Sharma, M., and Sergeev, V. N. (2019). A New Record of Acanthomorphic Acritarch Tappania Yin from the Early Mesoproterozoic Saraipali Formation, Singhora Group, Chhattisgarh Supergroup, India and its Biostratigraphic Significance. J. Geol. Soc. India 94 (5), 471–479. doi:10.1007/s12594-019-1343-1
Steiner, M. (1997). Chuaria Circularis Walcott 1899 – ‘megasphaeromorph Acritarch’ or Prokaryotic Colony? Acta Univ. Carol. Geol. 40 (null), 645–665.
Steiner, R. (1994). “Einführung,” in Die Neoproterozoischen Megaalgen Sudchinas (Berlin: Fachbereich Geowissenschaften), 1–4. doi:10.1007/978-3-322-84265-7_1
Sun, W. (1987). Palaeontology and Biostratigraphy of Late Precambrian Macroscopic Colonial Algae: Chuaria Walcott and Tawuia Hofmann. Palaeontogr. Abt. A 203 (null), 109–134.
Tang, Q., Pang, K., Li, G., Chen, L., Yuan, X., Sharma, M., et al. (2021). The Proterozoic Macrofossil Tawuia as a Coenocytic Eukaryote and a Possible Macroalga. Palaeogeogr. Palaeoclimatol. Palaeoecol. 576, 110485. doi:10.1016/j.palaeo.2021.110485
Tang, Q., Pang, K., Yuan, X., and Xiao, S. (2020). A One-Billion-Year-Old Multicellular Chlorophyte. Nat. Ecol. Evol. 4 (4), 543–549. doi:10.1038/s41559-020-1122-9
Tang, Q., Pang, K., Yuan, X., and Xiao, S. (2017). Electron Microscopy Reveals Evidence for Simple Multicellularity in the Proterozoic fossilChuaria. Geology 45 (1), 75–78. doi:10.1130/G38680.1
Tyler, S. A., Barghoorn, E. S., and Barrett, L. P. (1957). Anthracitic Coal from Precambrian Upper Huronian Black Shale of the Iron River District, Northern Michigan. Geol. Soc. Am. Bull. 68 (10), 1293–1304. doi:10.1130/0016-7606(1957)68[1293:ACFPUH]2.0.CO;2
Vidal, G., and Ford, T. D. (1985). Microbiotas from the Late Proterozoic Chuar Group (Northern Arizona) and Uinta Mountain Group (Utah) and Their Chronostratigraphic Implications. Precambrian Res. 28 (3-4), 349–389. doi:10.1016/0301-9268(85)90038-5
Vidal, G., Moczydłowska, M., and Rudavskaya, V. A. (1993). Biostratigraphical Implications of a Chuaria-Tawuia Assemblage and Associated Acritarchs from the Neoproterozoic of Yakutia. Palaeontology 36 (2), 387–402.
Wacey, D., Saunders, M., McPherson, A., Gain, S., Sirantoine, E., and Eiloart, K. (2019). Correlative Microscopy of Diverse Filamentous Microfossils from 850 Ma Rocks. Microsc. Microanal. 25 (S2), 2466–2467. doi:10.1017/S1431927619013060
Walcott, C. D. (1899). Pre-Cambrian Fossiliferous Formations. Geol. Soc. Am. Bull. 10, 199–244. doi:10.1130/gsab-10-199
Walter, M., Oehler, J. H., and Oehler, D. Z. (1976). Megascopic Algae 1300 Million Years Old from the Belt Supergroup, Montana: a Reinterpretation of Walcott's Helminthoidichnites. J. Paleontology 50 (5), 872–881.
Walter, M. R., Du, R., and Horodyski, R. J. (1990). Coiled Carbonaceous Megafossils from the Middle Proterozoic of Jixian (Tianjin) and Montana. Am. J. Sci. 290 A, 133–148.
Wan, B., Yuan, X., Chen, Z., Guan, C., Pang, K., Tang, Q., et al. (2016). Systematic Description of Putative Animal Fossils from the Early E Diacaran L Antian F Ormation of S Outh C Hina. Palaeontology 59 (4), 515–532. doi:10.1111/pala.12242
Wang, W. E. I., Guan, C., Zhou, C., Wan, B. I. N., Tang, Q., Chen, X., et al. (2014). Exceptional Preservation of Macrofossils from the Ediacaran Lantian and Miaohe Biotas, South China. PALAIOS 29 (3/4), 129–136. doi:10.2110/palo.2013.085
Wang, X., Yuan, X., Zhou, C., Du, K., and Gong, M. (2011). Anatomy and Plant Affinity of Chuaria. Chin. Sci. Bull. 56 (null), 693–699. doi:10.1007/s11434-011-4370-x
Xiao, S., and Dong, L. (2006). On the Morphological and Ecological History of Proterozoic Macroalgae. Top. Geobiol. 27, 57–90.
Xiao, S., Knoll, A. H., and Yuan, X. (1998). Morphological Reconstruction of Miaohephyton Bifurcatum, a Possible Brown Alga from the Neoproterozoic Doushantuo Formation, South China. J. Paleontol. 72 (6), 1072–1086. doi:10.1017/s0022336000027414
Xiao, S., Yuan, X., Steiner, M., and Knoll, A. H. (2002). Macroscopic Carbonaceous Compressions in a Terminal Proterozoic Shale: A Systematic Reassessment of the Miaohe Biota, South China. J. Paleontol. 76 (2), 347–376. doi:10.1666/0022-3360(2002)076<0347:Mcciat>2.0.Co;2
Yan, Y. (1995). Discovery and Preliminary Study of Megascopic Algae (1700 Ma) from the Tuanshanzi Formation in Jixian, Hebei. Acta Micropalaeontologica Sin. 12, 107–126.
Yan, Y., and Liu, Z. (1997). Tuanshanzian Macroscopic Algae of 1700 Ma B. P. From Changcheng System of Jixian, China. Acta Palaeontol. Sin. 36, 18–41.
Ye, Q., Tong, J., An, Z., Hu, J., Tian, L., Guan, K., et al. (2019). A Systematic Description of New Macrofossil Material from the Upper Ediacaran Miaohe Member in South China. J. Syst. Palaeontol. 17 (3), 183–238. doi:10.1080/14772019.2017.1404499
Yuan, X., Chen, Z., Xiao, S., Zhou, C., and Hua, H. (2011). An Early Ediacaran Assemblage of Macroscopic and Morphologically Differentiated Eukaryotes. Nature 470 (7334), 390–393. doi:10.1038/nature09810
Yuan, X., Li, J., and Cao, R. (1999). A Diverse Metaphyte Assemblage from the Neoproterozoic Black Shales of South China. Lethaia 32 (null), 143–155.
Yuan, X., Li, J., and Chen, M. (1995). Development and Their Fossil Records of Metaphytes from Late Precambrian. Acta Palaeontol. Sin. 34 (null), 90–102.
Yuan, X., Wan, B., Guan, C., Chen, Z., Zhou, C., Xiao, S., et al. (2016). The Lantian Biota. Shanghai: Shanghai Science and Technology Press, 138.
Yuan, X., Xiao, S., Li, J., Yin, L., and Cao, R. (2001). Pyritized Chuarids with Excystment Structures from the Late Neoproterozoic Lantian Formation in Anhui, South China. Precambrian Res. 107 (3-4), 253–263. doi:10.1016/S0301-9268(00)00144-3
Zhang, F., Wang, H., Ye, Y., Deng, Y., Lyu, Y., Wang, X., et al. (2021a). The Environmental Context of Carbonaceous Compressions and Implications for Organism Preservation 1.40 Ga and 0.63 Ga. Palaeogeogr. Palaeoclimatol. Palaeoecol. 573, 110449. doi:10.1016/j.palaeo.2021.110449
Zhang, S., Su, J., Ma, S., Wang, H., Wang, X., He, K., et al. (2021b). Eukaryotic Red and Green Algae Populated the Tropical Ocean 1400 Million Years Ago. Precambrian Res. 357, 106166. doi:10.1016/j.precamres.2021.106166
Zhao, Y., Chen, M. e., Peng, J., Yu, M., He, M., Wang, Y., et al. (2004). Discovery of a Miaohe-type Biota from the Neoproterozoic Doushantuo Formation in Jiangkou County, Guizhou Province, China. Chin. Sci. Bull. 49 (20), 2224–2226. doi:10.1360/982004-4710.1007/bf03185792
Zhu, S., and Chen, H. (1995). Megascopic Multicellular Organisms from the 1700-Million-Year-Old Tuanshanzi Formation in the Jixian Area, North China. Science 270 (5236), 620–622.
Zhu, S., Zhu, G., Sun, S., Sun, L., Huang, X., Zhang, K., et al. (2000). Discovery of Carbonaceous Compressions and Their Multicellular Tissues from the Changzhougou Formation (1 800 Ma) in the Yanshan Range, North China. Chin. Sci. Bull. 45 (9), 541–547. doi:10.1007/bf02887415
Keywords: multicellular, eukaryotes, macroalgae, carbonaceous compressions, Mesoproterozoic, Chhattisgarh Supergroup, India
Citation: Singh VK and Sharma M (2022) New Material of Carbonaceous Compressions from the ∼1.5 Ga Singhora Group, Chhattisgarh Supergroup, India, and their Interpretation as Benthic Algae. Front. Earth Sci. 10:825430. doi: 10.3389/feart.2022.825430
Received: 30 November 2021; Accepted: 06 June 2022;
Published: 21 July 2022.
Edited by:
Shuhai Xiao, Virginia Tech, United StatesReviewed by:
Qin Ye, China University of Geosciences, ChinaKe Pang, State Key Laboratory of Palaeobiology and Stratigraphy, Nanjing Institute of Geology and Palaeontology (CAS), China
Copyright © 2022 Singh and Sharma. This is an open-access article distributed under the terms of the Creative Commons Attribution License (CC BY). The use, distribution or reproduction in other forums is permitted, provided the original author(s) and the copyright owner(s) are credited and that the original publication in this journal is cited, in accordance with accepted academic practice. No use, distribution or reproduction is permitted which does not comply with these terms.
*Correspondence: Veeru Kant Singh, dmVlcnVrYW50c2luZ2hAYnNpcC5yZXMuaW4=